- 1The Third Affiliated Hospital, Zhongshan School of Medicine, Sun Yat-sen University, Guangzhou, China
- 2Key Laboratory for Stem Cells and Tissue Engineering, Ministry of Education, Sun Yat-sen University, Guangzhou, China
- 3Edinburgh Cancer Research UK Centre and MRC University of Edinburgh Centre for Reproductive Health, University of Edinburgh, Edinburgh, UK
- 4Department of Pathophysiology, Zhongshan School of Medicine, Sun Yat-sen University, Guangzhou, China
Prostate cancer is the most common cancer among men worldwide. However, current treatments for prostate cancer patients in advanced stage often fail because of relapse. Prostate cancer stem cells (PCSCs) are resistant to most standard therapies, and are considered to be a major mechanism of cancer metastasis and recurrence. In this review, we summarized current understanding of PCSCs and their self-renewal signaling pathways with a specific focus on Wnt signaling. Although multiple Wnt inhibitors have been developed to target PCSCs, their application is still limited by inefficient delivery and toxicity in vivo. Recently, nanotechnology has opened a new avenue for cancer drug delivery, which significantly increases specificity and reduces toxicity. These nanotechnology-based drug delivery methods showed great potential in targeting PCSCs. Here, we summarized current advancement of nanotechnology-based therapeutic strategies for targeting PCSCs and highlighted the challenges and perspectives in designing future therapies to eliminate PCSCs.
Introduction
Prostate cancer continues to be the most frequently diagnosed cancer in males and the third leading malignancy of cancer-related deaths in the USA (Siegel et al., 2017). Advanced/metastatic prostate cancer has been the major clinical challenge for prostate cancer. Recently, several new treatments have led to significant improvement of overall survival. These include novel androgen receptor pathway inhibitors abiraterone acetate (de Bono et al., 2011; Ryan et al., 2013) and enzalutamide (Scher et al., 2012; Beer et al., 2014), chemotherapy drugs taxanes, docetaxel and cabazitaxel (de Bono et al., 2010), an immunotherapeutic agent Sipuleucel-T (Kantoff et al., 2010), and a bone targeting alpha-emitting radionuclide, radium-223 chloride (Parker et al., 2013; Gillessen et al., 2015). However, resistance and recurrence still persists, which limits patient benefit.
Although still controversial, the resistant to the current treatment (hormonal therapy, chemotherapy, or radiotherapy) can be at least partially explained by the existence of prostate cancer stem cells (PCSCs). These cells can self-renew to initiate tumor in vivo in severe combined immunodeficient (SCID) mice (Hurt et al., 2008). PCSCs usually have low or undetectable androgen receptor expression that can lead to the failure of androgen deprivation therapy (hormonal therapy), the standard primary treatment for advanced prostate cancer (Lee et al., 2013; Di Zazzo et al., 2016). The slow growth rate of PCSCs allows them to survive routine chemotherapy and radiotherapy which are designed to attack actively dividing neoplastic cells. Moreover, PCSCs are highly resistant to drugs and toxins through a variety of mechanisms including enhanced drug efflux, expression of anti-apoptosis genes, and active DNA repair (Ni et al., 2014). The surviving PCSCs can regenerate the bulk of the tumor, or give rise to distant metastasis (Hurt et al., 2008; Salvatori et al., 2012; Shiozawa et al., 2016). Therefore, therapeutic strategies that specifically target PCSCs may eradicate tumors more effectively and reduce the risk of relapse and metastasis. PCSCs use various signaling pathways to maintain their self-renewal and differentiation, including Wnt/β-catenin, Hedgehog, TGF-β (Bisson and Prowse, 2009; Chang et al., 2011; Chen et al., 2015). Targeting these pathways to eliminate PCSCs is predicted to have high therapeutic potential in prostate cancer treatment. However, delivering drugs precisely to the vicinity of the tumor to target PCSCs is still a major challenge in clinical treatment.
Recently, developed nanotechnology opens a novel avenue for drug delivery in cancer therapy. Nanotechnology is the engineering and manufacturing of materials from 1 to 100 nanometers in size in at least one dimension. Nanotechnology has been widely used in cancer diagnosis and therapy such as molecular imaging, molecular diagnosis, and targeting therapy (Toy et al., 2014; Wicki et al., 2015). For example, nanovectors are used in the clinic to facilitate the targeted delivery of imaging contrast agents for diagnosis and anticancer drugs for treatment. Nanowires and nanocantilever arrays are used for precancerous and malignant lesion detection in biological fluids (Ferrari, 2005). Some of these nanoparticle-based strategies have already been approved for clinical use, and even more are in clinical trials or in preclinical development (Zhang L. et al., 2008; Van Audenhove and Gettemans, 2016).
Here, we summarized current advances in PCSCs with a focus on their identification, origin, and maintenance signals. Furthermore, we reviewed current advances in the application of nanotechnology toward the diagnosis and therapy of prostate cancer with a specific focus on targeting PCSCs.
Identification of PCSCs
Bonnet and Dick (1997) reported that a small subset of leukemic cells (CD34+CD38-) were capable of initiating human acute myeloid leukemia (AML) in a xenograft mouse model, this provided the first experimental evidence for the existence of cancer stem cells. Since then, although many groups have tried to identify cancer stem cells in solid tumors, it was not achieved until 2003. Al-Hajj et al. (2003) showed that CD44+CD24-/lowLineage- breast cancer cells were able to initiate tumor in immunodeficient mice, which proved the existence of cancer stem cells in solid tumors. Since then, cancer stem cell markers in different solid tumors have been identified, such as brain tumors (CD133+) (Singh et al., 2003), lung cancer (CD133+) (Eramo et al., 2008), colon cancer (CD133+) (O’Brien et al., 2007), pancreatic cancer (CD44+CD24+ESA+) (Li et al., 2007), ovarian cancer (CD44+CD117+) (Zhang S. et al., 2008), hepatic carcinoma (CD45-CD90+) (Yang et al., 2008), and melanoma (ABCB5+) (Schatton et al., 2008).
Prostate cancer stem cells were first identified by Collins et al. (2005). Their studies showed that CD44+α2β1hiCD133+ cells isolated from prostate cancer patients have a high potential for self-renewal and proliferation; these cells were also able to differentiate to heterogeneous cancer cells in ex vivo culture (Collins et al., 2005). Since, CSCs are conceptually considered to share similar self-renewal maintenance signals with normal stem cells, researchers intended to adapt knowledge from normal stem cell studies to explain CSC regulation mechanisms. For example, Hurt et al. (2008) found that CD44+CD24- enriched PCSC population has high level Oct3/4 and BMI-1 expression, which are critical for embryonic and quiescent adult stem cell maintenance (Masui et al., 2007; Tian et al., 2011). These isolated PCSCs have high tumorigenic and metastatic potential in immunodeficient xenograft mouse models (Hurt et al., 2008; Salvatori et al., 2012). This evidence indicated that CSCs might hijack self-renewal maintenance signals from normal stem cells during their evolution. Besides cell surface markers, some intracellular functional proteins can also be used for CSC identification. Increased aldehyde dehydrogenase (ALDH) activity is found in prostate stem/progenitor cells (Burger et al., 2009) and multiple types of CSCs, including PCSCs (Pearce et al., 2005; Ginestier et al., 2007; Jiang et al., 2009; Li et al., 2010). Prostate cancer cells with high ALDH activity showed enhanced tumorigenic and metastatic ability (van den Hoogen et al., 2010). A study identified prostate cancer cells with ALDH+CD44+α2β1+ phenotype could form xenograft tumors in non-obese diabetic (NOD)/SCID mice, which have impaired T and B cell lymphocyte development (SCID mutation) and deficient natural killer (NK) cell function (NOD background) (Qin et al., 2012). In addition, drug resistant genes such as ATP-binding cassette (ABC) transporter ABCG2 was used to further purify PCSCs from CD133+CD44+CD24- population. These purified PCSCs have increased clone and sphere formation ability (Hirschmann-Jax et al., 2004; Pfeiffer et al., 2011; Castellon et al., 2012). Overall, these studies suggest that both self-renewal and drug resistance characteristics should be considered for CSC identification.
The Origin of PCSCs
The origin of CSCs is still controversial. There is experimental evidence to show they could originate from normal stem cells because CSCs share similar cell surface markers with normal stem cells. For example, the first CSC study showed that CD34+CD38- CSCs in AML share the same surface marker with hematopoietic stem cells (HSCs) (Issaad et al., 1993; Petzer et al., 1996; Bonnet and Dick, 1997). In prostate, epithelial stem cells located in the basal layer of prostate gland have cell surface markers such as CD44, α2β1, and CD133 (Collins et al., 2001; Richardson et al., 2004; Garraway et al., 2010). Interestingly, the CD44+α2β1hiCD133+ prostate cancer cells have been shown to be PCSCs (Collins et al., 2005). It seems that during carcinogenesis, normal prostate stem cells gain mutations in oncogenes and tumor suppressor genes that drive them to become PCSCs.
Prostate cancer stem cells can also be derived from reprograming of differentiated cells via epithelial-mesenchymal transition (EMT), in which epithelial cells lose their polarity and cell–cell adhesion and gain migratory and invasive properties of mesenchymal cells (Kong et al., 2010; Talati et al., 2015; Lee et al., 2016). Kong et al. (2010) reported that overexpression of platelet-derived growth factor D (PDGFD) resulted in the loss of epithelial markers and increasing mesenchymal markers in prostate cancer cells. These EMT transformed prostate cancer cells have enhanced clone and sphere (prostasphere)-forming ability in vitro and tumorigenicity in mice. They also have increased stem-cell genes such as Sox2, Nanog, Oct4, Lin28B, and Notch1 (Kong et al., 2010). Suppressing DNA methyltransferase 1 (DNMT1) by 5-azacitidine (5-Aza) in prostate cancer cells can also induce EMT and stimulate transition of PCSCs. 5-Aza treated prostate cancer cells showed enhanced CD133+CD44+ phenotype and prostasphere formation ability, and elevated expression of stem cell-related transcription factors KLF4 and Sox2 (Lee et al., 2016). Activation of Jak2-Stat5a/b signaling promotes metastasis by inducing EMT and stem cell properties in prostate cancer cells, as shown by sphere formation and expression of CSC markers BMI-1, CD44, and Sox2 (Talati et al., 2015). Recently, there is an emerging concept that EMT represents a spectrum of differentiation status ranging from fully epithelial to fully mesenchymal status (Nieto et al., 2016). It is interesting to investigate the specific EMT status that may be associated with stem cell properties. PDGFD and 5-Aza both can induce stemness of prostate cancer cells and expression of mesenchymal markers but no expression of E-cadherin (Kong et al., 2010; Lee et al., 2016); prolactin can induce the stem-like features and an intermediate EMT phenotype, with low levels of E-cadherin and concomitant mesenchymal features (Talati et al., 2015). Therefore, it seems that in prostate cancer, different degrees of EMT can be associated with stem cell properties. Overall, the EMT transformed PCSCs might have more metastatic potential compared to normal stem cell derived PCSCs. More experimental evidence is needed to fully understand the origin of PCSCs.
Prostate cancer cells can also dedifferentiate to PCSCs in bone marrow. Nearly 80–90% of patients with prostate cancer have bone metastasis (Petrylak et al., 2004; Tannock et al., 2004). Although the mechanism of the tendency to metastasize to bone is not clear, experimental evidences suggest that bone marrow may provide a microenvironment to support PCSCs, as it does for HSCs (Lymperi et al., 2010; Zhao and Li, 2015). Interestingly, disseminated tumor cells (DTCs) from prostate cancer, particularly PCSCs, can compete with HSCs to occupy bone marrow osteoblastic niche for their maintenance (Shiozawa et al., 2011). Shiozawa et al. (2011) performed a assay to recover human DTCs grown in SCID mice from bone marrow. Using this approach, they found that after intracardiac injections of non-CSC prostate cancer cells (CD133-CD44-), the CSC population (CD133+CD44+) was observed and accounted for approximately 35% of the total prostate cancer cells isolated from mouse marrow. This suggests that the enrichment of CSCs is due to the conversion of non-CSCs into CSCs. Further mechanistic analysis showed this conversion may be regulated by osteoblastic niche-derived GAS6 through the Mer/mTOR signaling (Shiozawa et al., 2016). Overall, these studies suggest PCSCs can arise from normal stem cells or from differentiated cells depending on the context.
Self-Renewal Signaling Pathways in PCSCs
Wnt signaling is critical for embryonic stem cell transition from the pluripotent state and adult stem cell self-renewal maintenance. This raises the possibility that tightly regulated self-renewal capability in normal stem cells mediated by Wnt signal, could be hijacked by CSCs for malignant progress (Holland et al., 2013). Aberrant Wnt signaling has been reported in various tumors, including prostate cancer (Voeller et al., 1998; Chesire et al., 2000; de la Taille et al., 2003; Takebe et al., 2011). Prostate cancer patients have about 5% β-catenin activation mutation rate and this rate increases to 25–38% in metastatic and androgen-independent prostate cancer patients (Chesire and Isaacs, 2002; de la Taille et al., 2003). Two studies showed that the high incidence of β-catenin activation can induce formation of PCSCs. First, Wnt3a treatment in prostate cancer cells activated Wnt signaling and expanded PCSC numbers and increased their sphere forming ability in vitro (Bisson and Prowse, 2009). Second, activation of the Wnt pathway by AR79, a glycogen synthase kinase 3 (GSK-3) inhibitor, can increase the proportion of ALDH+CD133+ stem-like prostate cancer cells (Jiang et al., 2013). However, certain GSK-3 inhibitors might have varying non-specific effects, which lead to inconsistent results (Kroon et al., 2014). Therefore, targeting Wnt signaling is critical for PCSC treatment. Saikosaponin-d (SSd), a triterpenoid saponin derived from bupleurum, blocks Wnt/β-catenin signaling pathway by decreasing GSK-3β phosphorylation. SSd suppressed prostate cancer cell growth and inhibited their migration and invasion abilities. This was also accompanied by a reversal of the EMT process and inhibition of CSC phenotypes (measured by its ability to reduce tumor sphere formation and CD44 expression) (Zhong et al., 2016). In prostate cancer, PTEN is frequently mutated, which leads to activation of PI3K/Akt pathway that promotes PCSC maintenance and self-renewal (Li et al., 1997; Dubrovska et al., 2009). PI3K/Akt pathway can directly phosphorylate β-catenin at serine 552 to induce its nuclear localization, which leads to activation of Wnt signaling (Fang et al., 2007; He et al., 2007). Akt can also activate Wnt signaling through phosphorylation and inactivation of GSK-3β (Sharma et al., 2002). However, this mechanism is not universally supported. In a traumatic brain injury rat model, the peak time points of Akt and GSK-3β phosphorylation are not synchronous, suggesting GSK-3β may not be phosphorylated by Akt pathway (Zhao et al., 2012). Moreover, simultaneous activation of Wnt/β-catenin and PI3K/Akt signaling is required to drive self-renewal and expansion of HSCs (Perry et al., 2011). These findings indicate that Wnt/β-catenin and PI3K/Akt signaling can cooperatively promote CSC self-renewal. Thus, how to target these two pathways simultaneously may be critical to eliminate PCSCs.
Androgen signaling controls the growth of prostate gland and AR plays important roles throughout the various stages of prostate cancer (Augello et al., 2014). Interestingly, the expression and function of AR in PCSCs are still debatable. In many reported PCSC populations, AR expression is often low or undetectable. For example, the CD44+α2β1+CD133+ cells purified from human prostate tumor samples (Collins et al., 2005), the CD44+ cells in several prostate cancer xenografts (Patrawala et al., 2006), and the BCRP+ putative PCSCs (Huss et al., 2005) are all AR-. However, some studies show conflicting data. It was reported that the CD133+ cancer-initiating population and CD44+CD24- putative PCSCs in prostate cancer cell lines are AR+ (Sharifi et al., 2008; Vander Griend et al., 2008). Deng and Tang provide a hypothesis that PCSCs in primary and untreated tumors and models are mainly AR-, whereas PCSCs in castration resistant tumors could be either AR+ or AR-/lo (Deng and Tang, 2015). Interestingly, androgen signaling can interact with Wnt signaling and PI3K/Akt signaling at multiple levels (Terry et al., 2006; Lee et al., 2015). β-catenin can directly bind to ligand-engaged AR protein to promote its transcription activity. This binding can also facilitate the translocation of β-catenin into the nucleus (Truica et al., 2000; Mulholland et al., 2002; Yang et al., 2002). GSK-3β phosphorylates AR, thereby inhibits AR-driven transcription, which can be abrogated by the GSK-3 inhibitor LiCl (Salas et al., 2004). Human AR gene promoter contains LEF-1/TCF binding elements and activation of Wnt signaling upregulates AR transcription. In contrast, Wnt activation suppresses AR protein level by increasing phosphorylation of Akt and its downstream target MDM2, which promotes degradation of AR protein (Yang et al., 2006). Moreover, AR inhibition can activate Akt signaling by reducing levels of AKT phosphatase PHLPP in prostate PTEN-deficient murine prostate cancer model and in human prostate cancer xenografts (Carver et al., 2011). Overall, these findings indicate that androgen signaling has complex crosstalk with Wnt/β-catenin and PI3K/Akt signaling, and may enable prostate cancer cell stemness through Wnt/β-catenin and PI3K/Akt signaling.
The importance of Wnt/β-catenin signaling in tumors has spurred the development of inhibitors for cancer therapy. Cell-line studies have suggested some Wnt inhibitors exert inhibitory effects on prostate cancer cell proliferation and several Wnt inhibitors have been proven to be effective at inhibiting PCSCs. PKF118-310 suppresses prostate cancer cell growth by inhibiting β-catenin and TCF complex mediated transcription activation (Lepourcelet et al., 2004; Lu et al., 2009). 3289–8625 suppresses prostate cancer cell proliferation and reduces β-catenin level by inhibiting DVL-1 which links frizzled receptors and downstream signals (Grandy et al., 2009). Pyrvinium inhibits AR dependent gene expression and prostate cancer cell growth, which may result from its inhibitory effect on Wnt signaling through potentiating casein kinase 1α (CK1α) kinase activity (Jones et al., 2009; Thorne et al., 2010). Additionally, a study showed niclosamide, a drug used for the treatment of tapeworm, suppresses prostate cancer cell growth by inducing degradation of the Wnt receptor LRP6 (Lu et al., 2011). Importantly, DKK1 and sFRP2, two inhibitors that block Wnt signaling by binding to Wnt receptor LRP5/6 (DKK1) or Wnt proteins (sFRP2) (Kawano and Kypta, 2003), significantly inhibit the self-renewal capacity of PCSCs as evidenced by their ability to decrease prostasphere size and formation (Bisson and Prowse, 2009).
Several other signaling pathways are also implicated in PCSC regulation. Sanchez et al. (2004) found that sonic hedgehog (SHH) pathway components, such as GLI1, PTCH1, and SHH are upregulated in human prostate cancer tissues compared with normal prostatic epithelia. SHH signaling can be activated by androgen deprivation (Chen et al., 2009). Activation of SHH signaling supports androgen independent cell growth in a low androgen environment and enhances therapy resistance by increasing the level of ABC transporter (Chen et al., 2010; Statkiewicz et al., 2014). Blocking SHH pathway with an anti-SHH antibody or cyclopamine, a SMOH inhibitor, suppressed prostate cell proliferation (Chen et al., 2002; Sanchez et al., 2004). Overexpression of hedgehog leads to the formation of PCSCs with increased metastasizing potential (Chang et al., 2011). Darinaparsin, an organic arsenical compound with potent antineoplastic ability (Mann et al., 2009), and Genistein, an isoflavone with inhibitory effect on tyrosine kinases and topoisomerase-II (Salti et al., 2000; Qin et al., 2015), can both inhibit stemness of PCSCs and reduce tumor formation in xenograft models through targeting SHH signaling pathway (Zhang et al., 2012; Bansal et al., 2015).
Prostate carcinoma have high levels of TGF-β and TGF-β receptor expression (Cardillo et al., 2000). During prostate cancer progression, TGF-β plays an inconsistent role. During tumor initiation, TGF-β suppresses tumor growth by inducing apoptosis (Diener et al., 2010), while during tumor progression TGF-β induces EMT for invasion and metastasis (Moustakas and Heldin, 2016). This phenomenon is known as the TGF-β paradox (Tian and Schiemann, 2009). Activation of TGF-β signal expanded the CD44+CD24- population in prostate cancer cells through downregulating poly r(C) binding protein (PCBP)-1 (Chen et al., 2015), which suggested that TGF-β might regulate PCSC maintenance.
Non-coding RNAs are also involved in regulation of PCSCs stemness. Long non-coding RNA (lncRNA) H19 is highly expressed in PCSCs and knockdown of H19 decreases the colony-forming efficiency and reduces the expression of stem-cell genes (Oct4, Sox2, and Notch1). On the other hand, overexpression of H19 favors stemness of PCSCs (Bauderlique-Le Roy et al., 2015). lncRNA Hotair works synchronously with PRC2 to transcriptionally downregulate AR, leading to the increase of the CD133+ stem cell population (Li et al., 2015). In addition, microRNAs (miRNAs) are shown to regulate PCSCs through several stemness-related pathways such as Wnt, Akt, and TGF-β pathway. Increasing β-catenin expression through decreasing miRNA-320 in prostate cancer cells significantly increased their tumor spheres formation and clonogenic capacity, along with an increase in chemotherapy resistance in vitro and tumor growth in prostate cancer xenografts (Hsieh et al., 2013). Reduced miR-708 expression enhances PCSC stemness by upregulating AKT2, while re-expressing miR-708 suppresses the clonogenicity in vitro and leads to tumor regression in prostate cancer xenografts (Saini et al., 2012). MiR-128 overexpression in prostate cancer cells inhibits clonogenic and sphere-forming activities by decreasing stem cell regulatory factors BMI-1, Nanog, and TGFβR1 (Jin et al., 2014).
Conceivably, these self-renewal signaling pathways could serve as PCSC therapeutic targets in the future. However, most of the inhibitors against self-renewal pathways have clinical side effects and toxicities, which limit their clinical use. Since somatic stem cell homeostatic and regenerative processes after injury also rely on the self-renewal pathways for tissue regeneration and stem cells maintenance, inhibitors targeting these pathways may cause systemic toxicities (Pattabiraman and Weinberg, 2014). For example, it is well-known that Wnt signaling is essential for the regulation and homeostasis of intestinal stem cells (Pinto et al., 2003). Wnt inhibitors may lead to a depletion of normal intestinal stem cells (Kahn, 2014). Nanotechnology-based drug delivery systems can greatly improve this situation by increasing targeting specificity and reducing toxicities through restriction of drugs to the immediate vicinity of the tumor.
Application of Nanotechnology in Prostate Cancer
Recently, nanotechnology has been extensively explored in biomedical field to facilitate diagnosis and drug delivery for cancer treatment (Wu et al., 2010; Liao et al., 2011). Nanoparticles are small in size but with large surface-to-volume ratios allowing attachment of various molecules such as drugs and antibodies, which makes them suitable for medical use (Whitesides et al., 1991). Currently, prostate cancer diagnosis methods in the clinic include biochemical assays, digital rectal examination, transrectal ultrasonography, and biopsy. Biochemical assays are usually the first step for prostate cancer screening that examines the serum level of prostate specific antigen (PSA) (Catalona et al., 1991). PSA is a serine protease secreted by normal and malignant prostatic epithelium into seminal fluid, with minor amounts leaking into circulation in normal state, but increased amounts are observed in prostatic cancer (Stenman et al., 1999). According to the guidelines approved by the US Food and Drug Administration (FDA), a concentration of PSA > 4 ng/mL is considered as the gold standard of prostate cancer in initial screening. However, currently used enzyme-linked immunosorbent assay (ELISA) detection method for PSA shows poor sensitivity and specificity, with approximately 70% false-positive rate (Catalona et al., 1991; Bretton, 1994; Kang B.J. et al., 2015). Various nanomaterials with unique properties such as strong electronic, optic, and magnetic properties have been developed for PSA detection with better sensitivity. Among these nanotechnology-based bioassays, the most popular method is the electrochemical assay. In this assay, PSA captured by specific antibody alters the current that runs through carbon nanotubes, which gives this assay a higher sensitivity and a quicker speed than the standard ELISA method (Panini et al., 2008; Kim et al., 2009; Pandey et al., 2012; Huang et al., 2013; Salimi et al., 2013; Wang et al., 2013). Gold nanoparticles, with high surface area to volume ratio allowing more antibodies loading, can significantly improved PSA detection sensitivity in both serum (Thaxton et al., 2009) and urine samples (Yuhi et al., 2006). Besides PSA, other biomarkers such as prostate specific membrane antigen (PSMA), PF-4, IL-6, and ANXA3 can also be used for prostate cancer diagnosis, which have been tested using nanomaterials (Chikkaveeraiah et al., 2009; Kim et al., 2013). miRNAs are expressed in a tissue- and function-specific manner and are protected from nuclease degradation in the bloodstream. This makes them new candidate biomarkers for detecting cancers (Lu et al., 2005; Mitchell et al., 2008). MiR-141, with an elevated level in the blood of patients having metastatic prostate cancer (Mitchell et al., 2008), can be detected by a polymer-based nanomaterial (Tran et al., 2013). Another interesting study used spherical gold nanoparticle-nucleic acid conjugates to develop a microRNA array system for detection of microRNA profiles in prostate cancer samples. Through this system, they found several differentially expressed microRNAs (miR-200c, -21, -210, -205, -20a, -143∗, -143, and -16) that can be used as biomarkers (Alhasan et al., 2012).
Despite many chemotherapeutic agents show promising results in preclinical settings, their application in clinic often meets limitations largely due to inefficient bioavailability. Nanotechnology can improve drug bioavailability by developing a variety of nanoparticles that encapsulate anti-tumor drugs and release drugs in a controlled and time-dependent manner. Green tea polyphenol epigallocatechin-3-gallate (EGCG) can induce apoptosis of prostate cancer cells (Stuart et al., 2006). A polylactic acid-polyethylene glycol nanoparticle that encapsulated EGCG showed better pro-apoptotic and angiogenesis-inhibitory effects in vitro and larger inhibitory effect on prostate tumor growth in xenograft mice model than the non-encapsulated EGCG (Siddiqui et al., 2009). Camptothecin (CPT) is a pentacyclic alkaloid with a wide spectrum of anti-cancer activities, but is poorly soluble and has a fast degradation rate. CPT encapsulated β-cyclodextrin-nanosponges has been reported to improve the inhibitory effect on prostate cancer cell growth (Gigliotti et al., 2016). Besides improving bioavailability, nanotechnology can also specifically deliver chemotherapeutic agents to cancer cells without damaging the healthy cells. This targeted delivery is achieved by conjugating antibodies against tumor antigens to nanoparticles. In prostate cancer, PSMA and prostate stem cell antigen (PSCA) are the mostly used conjugated antibodies, both of which are highly expressed in prostate cancer cells (Reiter et al., 1998; Ghosh and Heston, 2004). The unique magnetic properties of some nanomaterials can be utilized in real-time monitoring of drug distribution. Nanoparticles that contain anti-tumor drugs and targeting ligands/antibodies can be coupled with the real-time imaging for the quantification of targeting efficiency. These reagents are defined as theranostic nanomedicine (Cherian et al., 2014). For example, PSMA targeted and PSCA targeted docetaxel-loaded superparamagnetic iron oxide (SPIO) nanoparticles can be efficiently internalized in prostate cancer cells and exhibit a higher inhibitory effect on cell survival compared with free docetaxel in prostate cancer cells. The distribution of these nanoparticles in cells can be visualized because SPIO is a kind of magnetic resonance imaging (MRI) contrast agent (Ling et al., 2011; Nagesh et al., 2016). Abdalla et al. (2011) engineered an iron oxide nanoparticle that targeted the drug noscapine (Nos) to tumors using urokinase plasminogen activator (uPA), a natural ligand for uPA receptor (uPAR) that is highly expressed by prostate cancer cells. The uPAR-targeted Nos-loaded iron oxide nanoparticles enhance the inhibitory effect of noscapine on prostate cancer cell growth and maintain their T2 MRI contrast effect upon internalization into tumor cells (Abdalla et al., 2011).
To date, nanotechnology has been applied to destroy PCSCs (Table 1). Nanoparticles loaded with self-renewal pathway inhibitors are designed in order to inhibit stemness of PCSCs. The clinical use of cyclopamine, a hedgehog inhibitor, is limited by its high hydrophobicity, systemic toxicity and poor pharmacokinetics (Lipinski et al., 2008). N-(2-hydroxypropyl) methacrylamide (HPMA) copolymers are great drug carriers with the advantage of increased solubility, prolonging circulation time and improved pharmacokinetic profiles of small molecule drugs (Kopecek and Kopeckova, 2010; Zhou and Kopecek, 2013). HPMA copolymer-cyclopamine conjugate treatment significantly decreased prostasphere forming capacity and percentage of CD133+ PCSC enriched population in PC3 and RC-92a/hTERT prostate cancer cells. RC-92a/hTERT cells are human prostate cancer epithelial cells transduced to express human telomerase reverse transcriptase, and exhibit high levels of CD133 (Miki et al., 2007). In vivo experiment showed that HPMA copolymer-cyclopamine conjugate administration reduced tumor volume in PC3 tumor xenograft nude mice. Moreover, combination of HPMA copolymer-cyclopamine conjugate and HPMA copolymer-docetaxel conjugate led to significantly reduced tumor volume over single drug administration (Zhou et al., 2012, 2013). HPMA copolymer-GDC-0980 (PI3K/mTOR inhibitor) conjugate treatment can also decrease the percentage of CD133+ cells and the number of prostaspheres in PC3 cells. In PC3 tumor xenograft model, administration of HPMA copolymer-GDC-0980 conjugate could prolong survival slightly, and combination use of HPMA copolymer-GDC-0980 conjugate and HPMA copolymer-docetaxel conjugate led to significantly prolonged survival compared with either of the single treatments (Zhou et al., 2015). Yang et al. (2016) synthesized poly(ethylene glycol)-block-poly(2-methyl-2-carboxyl-propylene carbonate) (mPEG-b-PCC) for loading cyclopamine and paclitaxel, respectively. Both the cyclopamine and paclitaxel loaded nanoparticles can release drugs slowly and inhibit colony-forming ability of paclitaxel resistant PC3 cells. Administration of either of the two nanoparticles to PC3 tumor xenograft nude mice can lower tumor growth. Significant tumor inhibition was observed in mice treated with the combination of cyclopamine and paclitaxel loaded nanoparticles (Yang et al., 2016). These studies suggest combination therapy targeting both CSCs and bulk tumor cells is a promising approach to improve the therapeutic benefit against prostate cancer. Cis-dichlorodiamminoplatinum (II) (CDDP) is a highly effective anti-tumor agent toward a variety of tumor types. Jafari Malek et al. (2014) generated CDDP loaded glyconanoparticles using hyaluronic acid (HA), the endogenous substrate for CD44. These CDDP loaded glyconanoparticles led to a significant reduction of clonogenicity and sphere formation capacity of prostate cancer DU145 and PC3 cells (Jafari Malek et al., 2014). Alongside the drugs conjugated to nanoparticles, some materials themselves can exert anti-tumor functions. An interesting study found graphene oxide effectively inhibited sphere formation not only in PC3 prostate cancer cells, but also in SKOV3 ovarian cancer cells, U87 glioblastoma cells, A549 lung cancer cells, and MIA-PaCa-2 pancreatic cancer cells, highlighting its efficacy against CSCs across different cancer types. Graphene oxide exerts this effect by inducing CSC differentiation through blocking several key signaling pathways including Wnt, Notch, and STAT (Fiorillo et al., 2015).
Challenges and Perspectives
There is a significant and rapid advancement in our knowledge of PCSCs and their role in prostate cancer initiation and progression. We can target PCSCs through their self-renewal pathways, such as Wnt signaling. Several inhibitors such as DKK1 and sFRP2 for Wnt signaling are effective at inhibiting PCSC self-renewal (Bisson and Prowse, 2009). However, their potential adverse effects on normal stem cell self-renewal and tissue homeostasis are a serious concern. More effective drug delivery system is urgently needed. The application of nanotechnology-based drug delivery such as nanoparticle capsules can improve PCSC targeting specificity and reduce side effects by restricting drugs to tumors and their surrounding areas.
Targeted delivery of drugs to CSCs without damaging normal stem cells is challenging because of shared cell surface markers. A variety of drug-loaded nanoparticles conjugated with antibodies to these markers (CD44, CD133, and ABCG2) have been developed that improve the drug delivery efficiency to CSCs in colon cancer (Bourseau-Guilmain et al., 2012), breast cancer (Swaminathan et al., 2013), and multiple myeloma (Yang et al., 2014). Some nanomaterials with photo-thermal properties, such as single-walled carbon nanotubes (SWNTs), can be used for thermal destruction of glioblastoma stem-like cells when conjugated with CD133 antibody (Wang et al., 2011). Nanomaterials like HA, which has high CD44-binding efficacy, can deliver drugs to CD44-expressing CSCs (Wei et al., 2013). However, it is unknown whether these CSC-targeted nanoparticles will have toxic effects on normal stem cells. One possible solution is to identify markers with specific expression on CSCs but not on normal stem cells.
Since, CSCs and normal stem cells often share the same self-renewal pathways, identifying and targeting the key signaling involved in CSCs but not in normal stem cells is a promising strategy. Kang X. et al. (2015) found that Leukocyte-associated immunoglobulin-like receptor 1 (LAIR1) deficiency exhausts mouse AML stem cells, but does not affect normal hematopoiesis. This discovery provides hope that there may likewise be similar pathways in PCSCs. The hypoxia-inducible factor (HIF) pathway may be one of such pathways in prostate cancer. HIF signaling is elevated in PCSC population and promotes stemness and self-renewal of PCSCs (Ma et al., 2011; Marhold et al., 2015). Considering hypoxia often presents in the tumor microenvironment instead of the normal state, targeting HIF signaling may inhibit PCSCs without damaging normal stem cells.
Overall, there has been much advancement in the field of nanotechnology for prostate cancer treatment. Various approaches have been developed to specifically target PCSCs. In the future, more nanotechnology-based therapeutic strategies are urgently needed to target self-renewal pathways of PCSCs. Wnt signaling with its critical role in PCSCs and existence of a variety of small-molecule inhibitors is an attractive target. Moreover, additional studies are still needed to investigate the specific markers and pathways involved in PCSCs. By targeting these markers and pathways, nanoparticles may avoid the toxic effects on normal stem cells. It should also be noted that targeting the PCSC alone may not be enough to eliminate tumor and combination of a standard chemotherapy and a PCSC specific chemotherapy may be the most efficacious treatment for prostate cancer (Figure 1). Based on the studies mentioned in our review, it is apparent that nanotechnology-based methods holds great potential for the targeted destruction of PCSCs and may lead to significant patient benefit.
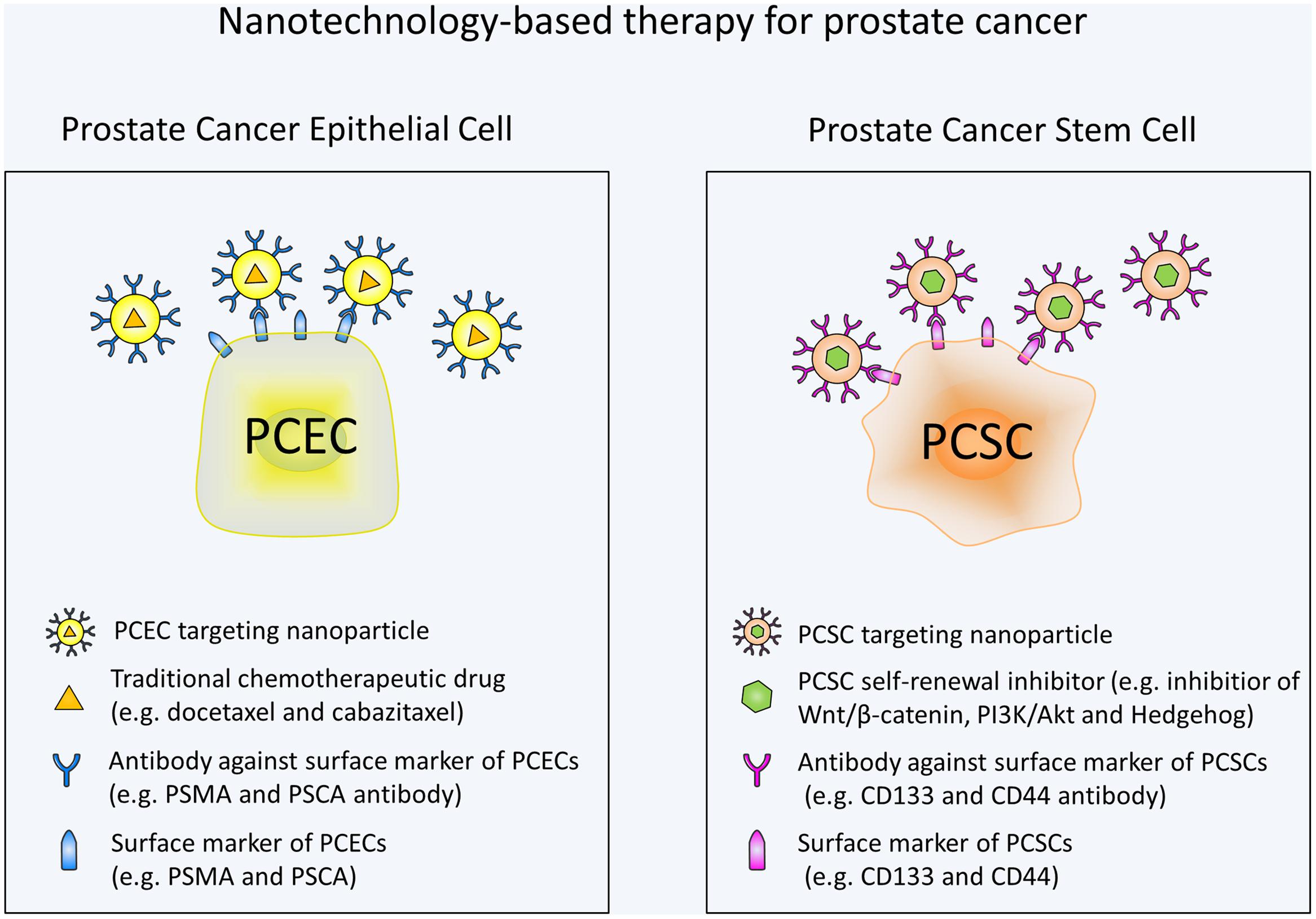
FIGURE 1. Schematic illustration of nanotechnology-based therapy for prostate cancer. Prostate cancer epithelial cell (PCEC) targeting nanoparticles are conjugated with antibodies against PCEC surface markers and contain traditional chemotherapeutic drugs. PCSC targeting nanoparticles are conjugated with antibodies against PCSC surface markers and contain self-renewal signaling pathway inhibitors. Combination use of the nanotechnology-based standard chemotherapy and PCSC specific chemotherapy can eradicate the bulk of the tumor cells and PCSCs at the same time, thus may be the most efficacious treatment for prostate cancer.
Author Contributions
WQ and YZ wrote the manuscript. B-ZQ and MZ provided critical comments and revised the manuscript.
Funding
MZ is supported by Zhongshan School of Medicine, Sun Yat-sen University and the Thousand Talents Plan in China. B-ZQ is supported by Cancer Research UK Career Development Fellowship C49791/A17367.
Conflict of Interest Statement
The authors declare that the research was conducted in the absence of any commercial or financial relationships that could be construed as a potential conflict of interest.
References
Abdalla, M. O., Karna, P., Sajja, H. K., Mao, H., Yates, C., Turner, T., et al. (2011). Enhanced noscapine delivery using uPAR-targeted optical-MR imaging trackable nanoparticles for prostate cancer therapy. J. Control. Release 149, 314–322. doi: 10.1016/j.jconrel.2010.10.030
Al-Hajj, M., Wicha, M. S., Benito-Hernandez, A., Morrison, S. J., and Clarke, M. F. (2003). Prospective identification of tumorigenic breast cancer cells. Proc. Natl. Acad. Sci. U.S.A. 100, 3983–3988. doi: 10.1073/pnas.0530291100
Alhasan, A. H., Kim, D. Y., Daniel, W. L., Watson, E., Meeks, J. J., Thaxton, C. S., et al. (2012). Scanometric microRNA array profiling of prostate cancer markers using spherical nucleic acid-gold nanoparticle conjugates. Anal. Chem. 84, 4153–4160. doi: 10.1021/ac3004055
Augello, M. A., Den, R. B., and Knudsen, K. E. (2014). AR function in promoting metastatic prostate cancer. Cancer Metastasis Rev. 33, 399–411. doi: 10.1007/s10555-013-9471-3
Bansal, N., Farley, N. J., Wu, L., Lewis, J., Youssoufian, H., and Bertino, J. R. (2015). Darinaparsin inhibits prostate tumor-initiating cells and Du145 xenografts and is an inhibitor of hedgehog signaling. Mol. Cancer Ther. 14, 23–30. doi: 10.1158/1535-7163.MCT-13-1040
Bauderlique-Le Roy, H., Vennin, C., Brocqueville, G., Spruyt, N., Adriaenssens, E., and Bourette, R. P. (2015). Enrichment of human stem-like prostate cells with s-SHIP promoter activity uncovers a role in stemness for the long noncoding RNA H19. Stem Cells Dev. 24, 1252–1262. doi: 10.1089/scd.2014.0386
Beer, T. M., Armstrong, A. J., Rathkopf, D. E., Loriot, Y., Sternberg, C. N., Higano, C. S., et al. (2014). Enzalutamide in metastatic prostate cancer before chemotherapy. N. Engl. J. Med. 371, 424–433. doi: 10.1056/NEJMoa1405095
Bisson, I., and Prowse, D. M. (2009). WNT signaling regulates self-renewal and differentiation of prostate cancer cells with stem cell characteristics. Cell Res. 19, 683–697. doi: 10.1038/cr.2009.43
Bonnet, D., and Dick, J. E. (1997). Human acute myeloid leukemia is organized as a hierarchy that originates from a primitive hematopoietic cell. Nat. Med. 3, 730–737. doi: 10.1038/nm0797-730
Bourseau-Guilmain, E., Bejaud, J., Griveau, A., Lautram, N., Hindre, F., Weyland, M., et al. (2012). Development and characterization of immuno-nanocarriers targeting the cancer stem cell marker AC133. Int. J. Pharm. 423, 93–101. doi: 10.1016/j.ijpharm.2011.06.001
Bretton, P. R. (1994). Prostate-specific antigen and digital rectal examination in screening for prostate cancer: a community-based study. South Med. J. 87, 720–723. doi: 10.1097/00007611-199407000-00009
Burger, P. E., Gupta, R., Xiong, X., Ontiveros, C. S., Salm, S. N., Moscatelli, D., et al. (2009). High aldehyde dehydrogenase activity: a novel functional marker of murine prostate stem/progenitor cells. Stem Cells 27, 2220–2228. doi: 10.1002/stem.135
Cardillo, M. R., Petrangeli, E., Perracchio, L., Salvatori, L., Ravenna, L., and Di Silverio, F. (2000). Transforming growth factor-beta expression in prostate neoplasia. Anal. Quant. Cytol. Histol. 22, 1–10.
Carver, B. S., Chapinski, C., Wongvipat, J., Hieronymus, H., Chen, Y., Chandarlapaty, S., et al. (2011). Reciprocal feedback regulation of PI3K and androgen receptor signaling in PTEN-deficient prostate cancer. Cancer Cell 19, 575–586. doi: 10.1016/j.ccr.2011.04.008
Castellon, E. A., Valenzuela, R., Lillo, J., Castillo, V., Contreras, H. R., Gallegos, I., et al. (2012). Molecular signature of cancer stem cells isolated from prostate carcinoma and expression of stem markers in different Gleason grades and metastasis. Biol. Res. 45, 297–305. doi: 10.4067/S0716-97602012000300011
Catalona, W. J., Smith, D. S., Ratliff, T. L., Dodds, K. M., Coplen, D. E., Yuan, J. J., et al. (1991). Measurement of prostate-specific antigen in serum as a screening test for prostate cancer. N. Engl. J. Med. 324, 1156–1161. doi: 10.1056/NEJM199104253241702
Chang, H. H., Chen, B. Y., Wu, C. Y., Tsao, Z. J., Chen, Y. Y., Chang, C. P., et al. (2011). Hedgehog overexpression leads to the formation of prostate cancer stem cells with metastatic property irrespective of androgen receptor expression in the mouse model. J. Biomed. Sci. 18:6. doi: 10.1186/1423-0127-18-6
Chen, J. K., Taipale, J., Cooper, M. K., and Beachy, P. A. (2002). Inhibition of Hedgehog signaling by direct binding of cyclopamine to Smoothened. Genes Dev. 16, 2743–2748. doi: 10.1101/gad.1025302
Chen, M., Feuerstein, M. A., Levina, E., Baghel, P. S., Carkner, R. D., Tanner, M. J., et al. (2010). Hedgehog/Gli supports androgen signaling in androgen deprived and androgen independent prostate cancer cells. Mol. Cancer 9:89. doi: 10.1186/1476-4598-9-89
Chen, M., Tanner, M., Levine, A. C., Levina, E., Ohouo, P., and Buttyan, R. (2009). Androgenic regulation of hedgehog signaling pathway components in prostate cancer cells. Cell Cycle 8, 149–157. doi: 10.4161/cc.8.1.7532
Chen, Q., Cai, Z. K., Chen, Y. B., Gu, M., Zheng, D. C., Zhou, J., et al. (2015). Poly r(C) binding protein-1 is central to maintenance of cancer stem cells in prostate cancer cells. Cell Physiol. Biochem. 35, 1052–1061. doi: 10.1159/000373931
Cherian, A. M., Nair, S. V., and Lakshmanan, V. K. (2014). The role of nanotechnology in prostate cancer theranostic applications. J. Nanosci. Nanotechnol. 14, 841–852. doi: 10.1166/jnn.2014.9052
Chesire, D. R., Ewing, C. M., Sauvageot, J., Bova, G. S., and Isaacs, W. B. (2000). Detection and analysis of beta-catenin mutations in prostate cancer. Prostate 45, 323–334. doi: 10.1002/1097-0045(20001201)45:4<323::AID-PROS7>3.0.CO;2-W
Chesire, D. R., and Isaacs, W. B. (2002). Ligand-dependent inhibition of beta-catenin/TCF signaling by androgen receptor. Oncogene 21, 8453–8469. doi: 10.1038/sj.onc.1206049
Chikkaveeraiah, B. V., Bhirde, A., Malhotra, R., Patel, V., Gutkind, J. S., and Rusling, J. F. (2009). Single-wall carbon nanotube forest arrays for immunoelectrochemical measurement of four protein biomarkers for prostate cancer. Anal. Chem. 81, 9129–9134. doi: 10.1021/ac9018022
Collins, A. T., Berry, P. A., Hyde, C., Stower, M. J., and Maitland, N. J. (2005). Prospective identification of tumorigenic prostate cancer stem cells. Cancer Res. 65, 10946–10951. doi: 10.1158/0008-5472.CAN-05-2018
Collins, A. T., Habib, F. K., Maitland, N. J., and Neal, D. E. (2001). Identification and isolation of human prostate epithelial stem cells based on alpha(2)beta(1)-integrin expression. J. Cell Sci. 114(Pt 21), 3865–3872.
de Bono, J. S., Logothetis, C. J., Molina, A., Fizazi, K., North, S., Chu, L., et al. (2011). Abiraterone and increased survival in metastatic prostate cancer. N. Engl. J. Med. 364, 1995–2005. doi: 10.1056/NEJMoa1014618
de Bono, J. S., Oudard, S., Ozguroglu, M., Hansen, S., Machiels, J. P., Kocak, I., et al. (2010). Prednisone plus cabazitaxel or mitoxantrone for metastatic castration-resistant prostate cancer progressing after docetaxel treatment: a randomised open-label trial. Lancet 376, 1147–1154. doi: 10.1016/S0140-6736(10)61389-X
de la Taille, A., Rubin, M. A., Chen, M. W., Vacherot, F., de Medina, S. G., Burchardt, M., et al. (2003). Beta-catenin-related anomalies in apoptosis-resistant and hormone-refractory prostate cancer cells. Clin. Cancer Res. 9, 1801–1807.
Deng, Q., and Tang, D. G. (2015). Androgen receptor and prostate cancer stem cells: biological mechanisms and clinical implications. Endocr. Relat. Cancer 22, T209–T220. doi: 10.1530/ERC-15-0217
Di Zazzo, E., Galasso, G., Giovannelli, P., Di Donato, M., Di Santi, A., Cernera, G., et al. (2016). Prostate cancer stem cells: the role of androgen and estrogen receptors. Oncotarget 7, 193–208. doi: 10.18632/oncotarget.6220
Diener, K. R., Need, E. F., Buchanan, G., and Hayball, J. D. (2010). TGF-beta signalling and immunity in prostate tumourigenesis. Expert Opin. Ther. Targets 14, 179–192. doi: 10.1517/14728220903544507
Dubrovska, A., Kim, S., Salamone, R. J., Walker, J. R., Maira, S. M., Garcia-Echeverria, C., et al. (2009). The role of PTEN/Akt/PI3K signaling in the maintenance and viability of prostate cancer stem-like cell populations. Proc. Natl. Acad. Sci. U.S.A. 106, 268–273. doi: 10.1073/pnas.0810956106
Eramo, A., Lotti, F., Sette, G., Pilozzi, E., Biffoni, M., Di Virgilio, A., et al. (2008). Identification and expansion of the tumorigenic lung cancer stem cell population. Cell Death Differ. 15, 504–514. doi: 10.1038/sj.cdd.4402283
Fang, D., Hawke, D., Zheng, Y., Xia, Y., Meisenhelder, J., Nika, H., et al. (2007). Phosphorylation of beta-catenin by AKT promotes beta-catenin transcriptional activity. J. Biol. Chem. 282, 11221–11229. doi: 10.1074/jbc.M611871200
Ferrari, M. (2005). Cancer nanotechnology: opportunities and challenges. Nat. Rev. Cancer 5, 161–171. doi: 10.1038/nrc1566
Fiorillo, M., Verre, A. F., Iliut, M., Peiris-Pages, M., Ozsvari, B., Gandara, R., et al. (2015). Graphene oxide selectively targets cancer stem cells, across multiple tumor types: implications for non-toxic cancer treatment, via “differentiation-based nano-therapy”. Oncotarget 6, 3553–3562. doi: 10.18632/oncotarget.3348
Garraway, I. P., Sun, W., Tran, C. P., Perner, S., Zhang, B., Goldstein, A. S., et al. (2010). Human prostate sphere-forming cells represent a subset of basal epithelial cells capable of glandular regeneration in vivo. Prostate 70, 491–501. doi: 10.1002/pros.21083
Ghosh, A., and Heston, W. D. (2004). Tumor target prostate specific membrane antigen (PSMA) and its regulation in prostate cancer. J. Cell. Biochem. 91, 528–539. doi: 10.1002/jcb.10661
Gigliotti, C. L., Minelli, R., Cavalli, R., Occhipinti, S., Barrera, G., Pizzimenti, S., et al. (2016). In vitro and in vivo therapeutic evaluation of camptothecin-encapsulated beta-cyclodextrin nanosponges in prostate cancer. J. Biomed. Nanotechnol. 12, 114–127. doi: 10.1166/jbn.2016.2144
Gillessen, S., Omlin, A., Attard, G., de Bono, J. S., Efstathiou, E., Fizazi, K., et al. (2015). Management of patients with advanced prostate cancer: recommendations of the St Gallen Advanced Prostate Cancer Consensus Conference (APCCC) 2015. Ann. Oncol. 26, 1589–1604. doi: 10.1093/annonc/mdv257
Ginestier, C., Hur, M. H., Charafe-Jauffret, E., Monville, F., Dutcher, J., Brown, M., et al. (2007). ALDH1 is a marker of normal and malignant human mammary stem cells and a predictor of poor clinical outcome. Cell Stem Cell 1, 555–567. doi: 10.1016/j.stem.2007.08.014
Grandy, D., Shan, J., Zhang, X., Rao, S., Akunuru, S., Li, H., et al. (2009). Discovery and characterization of a small molecule inhibitor of the PDZ domain of dishevelled. J. Biol. Chem. 284, 16256–16263. doi: 10.1074/jbc.M109.009647
He, X. C., Yin, T., Grindley, J. C., Tian, Q., Sato, T., Tao, W. A., et al. (2007). PTEN-deficient intestinal stem cells initiate intestinal polyposis. Nat. Genet. 39, 189–198. doi: 10.1038/ng1928
Hirschmann-Jax, C., Foster, A. E., Wulf, G. G., Nuchtern, J. G., Jax, T. W., Gobel, U., et al. (2004). A distinct “side population” of cells with high drug efflux capacity in human tumor cells. Proc. Natl. Acad. Sci. U.S.A. 101, 14228–14233. doi: 10.1073/pnas.0400067101
Holland, J. D., Klaus, A., Garratt, A. N., and Birchmeier, W. (2013). Wnt signaling in stem and cancer stem cells. Curr. Opin. Cell Biol. 25, 254–264. doi: 10.1016/j.ceb.2013.01.004
Hsieh, I. S., Chang, K. C., Tsai, Y. T., Ke, J. Y., Lu, P. J., Lee, K. H., et al. (2013). MicroRNA-320 suppresses the stem cell-like characteristics of prostate cancer cells by downregulating the Wnt/beta-catenin signaling pathway. Carcinogenesis 34, 530–538. doi: 10.1093/carcin/bgs371
Huang, Y. W., Wu, C. S., Chuang, C. K., Pang, S. T., Pan, T. M., Yang, Y. S., et al. (2013). Real-time and label-free detection of the prostate-specific antigen in human serum by a polycrystalline silicon nanowire field-effect transistor biosensor. Anal. Chem. 85, 7912–7918. doi: 10.1021/ac401610s
Hurt, E. M., Kawasaki, B. T., Klarmann, G. J., Thomas, S. B., and Farrar, W. L. (2008). CD44+ CD24(-) prostate cells are early cancer progenitor/stem cells that provide a model for patients with poor prognosis. Br. J. Cancer 98, 756–765. doi: 10.1038/sj.bjc.6604242
Huss, W. J., Gray, D. R., Greenberg, N. M., Mohler, J. L., and Smith, G. J. (2005). Breast cancer resistance protein-mediated efflux of androgen in putative benign and malignant prostate stem cells. Cancer Res. 65, 6640–6650. doi: 10.1158/0008-5472.CAN-04-2548
Issaad, C., Croisille, L., Katz, A., Vainchenker, W., and Coulombel, L. (1993). A murine stromal cell line allows the proliferation of very primitive human CD34++/CD38- progenitor cells in long-term cultures and semisolid assays. Blood 81, 2916–2924.
Jafari Malek, S., Khoshchehreh, R., Goodarzi, N., Khoshayand, M. R., Amini, M., Atyabi, F., et al. (2014). cis-Dichlorodiamminoplatinum (II) glyconanoparticles by drug-induced ionic gelation technique targeted to prostate cancer: preparation, optimization and in vitro characterization. Colloids Surf. B Biointerfaces 122, 350–358. doi: 10.1016/j.colsurfb.2014.06.065
Jiang, F., Qiu, Q., Khanna, A., Todd, N. W., Deepak, J., Xing, L., et al. (2009). Aldehyde dehydrogenase 1 is a tumor stem cell-associated marker in lung cancer. Mol. Cancer Res. 7, 330–338. doi: 10.1158/1541-7786.MCR-08-0393
Jiang, Y., Dai, J., Zhang, H., Sottnik, J. L., Keller, J. M., Escott, K. J., et al. (2013). Activation of the Wnt pathway through AR79, a GSK3beta inhibitor, promotes prostate cancer growth in soft tissue and bone. Mol. Cancer Res. 11, 1597–1610. doi: 10.1158/1541-7786.MCR-13-0332-T
Jin, M., Zhang, T., Liu, C., Badeaux, M. A., Liu, B., Liu, R., et al. (2014). miRNA-128 suppresses prostate cancer by inhibiting BMI-1 to inhibit tumor-initiating cells. Cancer Res. 74, 4183–4195. doi: 10.1158/0008-5472.CAN-14-0404
Jones, J. O., Bolton, E. C., Huang, Y., Feau, C., Guy, R. K., Yamamoto, K. R., et al. (2009). Non-competitive androgen receptor inhibition in vitro and in vivo. Proc. Natl. Acad. Sci. U.S.A. 106, 7233–7238. doi: 10.1073/pnas.0807282106
Kahn, M. (2014). Can we safely target the WNT pathway? Nat. Rev. Drug Discov. 13, 513–532. doi: 10.1038/nrd4233
Kang, B. J., Jeun, M., Jang, G. H., Song, S. H., Jeong, I. G., Kim, C. S., et al. (2015). Diagnosis of prostate cancer via nanotechnological approach. Int. J. Nanomed. 10, 6555–6569. doi: 10.2147/IJN.S91908
Kang, X., Lu, Z., Cui, C., Deng, M., Fan, Y., Dong, B., et al. (2015). The ITIM-containing receptor LAIR1 is essential for acute myeloid leukaemia development. Nat. Cell Biol. 17, 665–677. doi: 10.1038/ncb3158
Kantoff, P. W., Higano, C. S., Shore, N. D., Berger, E. R., Small, E. J., Penson, D. F., et al. (2010). Sipuleucel-T immunotherapy for castration-resistant prostate cancer. N. Engl. J. Med. 363, 411–422. doi: 10.1056/NEJMoa1001294
Kawano, Y., and Kypta, R. (2003). Secreted antagonists of the Wnt signalling pathway. J. Cell Sci. 116(Pt 13), 2627–2634. doi: 10.1242/jcs.00623
Kim, J. P., Lee, B. Y., Lee, J., Hong, S., and Sim, S. J. (2009). Enhancement of sensitivity and specificity by surface modification of carbon nanotubes in diagnosis of prostate cancer based on carbon nanotube field effect transistors. Biosens. Bioelectron. 24, 3372–3378. doi: 10.1016/j.bios.2009.04.048
Kim, Y. J., Rahman, M. M., and Lee, J.-J. (2013). Ultrasensitive and label-free detection of annexin A3 based on quartz crystal microbalance. Sens. Actuators B Chem. 177, 172–177. doi: 10.1016/j.snb.2012.10.117
Kong, D., Banerjee, S., Ahmad, A., Li, Y., Wang, Z., Sethi, S., et al. (2010). Epithelial to mesenchymal transition is mechanistically linked with stem cell signatures in prostate cancer cells. PLoS ONE 5:e12445. doi: 10.1371/journal.pone.0012445
Kopecek, J., and Kopeckova, P. (2010). HPMA copolymers: origins, early developments, present, and future. Adv. Drug Deliv. Rev. 62, 122–149. doi: 10.1016/j.addr.2009.10.004
Kroon, J., in ’t Veld, L. S., Buijs, J. T., Cheung, H., van der Horst, G., and van der Pluijm, G. (2014). Glycogen synthase kinase-3beta inhibition depletes the population of prostate cancer stem/progenitor-like cells and attenuates metastatic growth. Oncotarget 5, 8986–8994. doi: 10.18632/oncotarget.1510
Lee, E., Wang, J., Yumoto, K., Jung, Y., Cackowski, F. C., Decker, A. M., et al. (2016). DNMT1 regulates epithelial-mesenchymal transition and cancer stem cells, which promotes prostate cancer metastasis. Neoplasia 18, 553–566. doi: 10.1016/j.neo.2016.07.007
Lee, S. H., Johnson, D., Luong, R., and Sun, Z. (2015). Crosstalking between androgen and PI3K/AKT signaling pathways in prostate cancer cells. J. Biol. Chem. 290, 2759–2768. doi: 10.1074/jbc.M114.607846
Lee, S. O., Ma, Z., Yeh, C. R., Luo, J., Lin, T. H., Lai, K. P., et al. (2013). New therapy targeting differential androgen receptor signaling in prostate cancer stem/progenitor vs. non-stem/progenitor cells. J. Mol. Cell Biol. 5, 14–26. doi: 10.1093/jmcb/mjs042
Lepourcelet, M., Chen, Y. N., France, D. S., Wang, H., Crews, P., Petersen, F., et al. (2004). Small-molecule antagonists of the oncogenic Tcf/beta-catenin protein complex. Cancer Cell 5, 91–102. doi: 10.1016/S1535-6108(03)00334-9
Li, C., Heidt, D. G., Dalerba, P., Burant, C. F., Zhang, L., Adsay, V., et al. (2007). Identification of pancreatic cancer stem cells. Cancer Res. 67, 1030–1037. doi: 10.1158/0008-5472.CAN-06-2030
Li, J., Yen, C., Liaw, D., Podsypanina, K., Bose, S., Wang, S. I., et al. (1997). PTEN, a putative protein tyrosine phosphatase gene mutated in human brain, breast, and prostate cancer. Science 275, 1943–1947. doi: 10.1126/science.275.5308.1943
Li, L., Dang, Q., Xie, H., Yang, Z., He, D., Liang, L., et al. (2015). Infiltrating mast cells enhance prostate cancer invasion via altering LncRNA-HOTAIR/PRC2-androgen receptor (AR)-MMP9 signals and increased stem/progenitor cell population. Oncotarget 6, 14179–14190. doi: 10.18632/oncotarget.3651
Li, T., Su, Y., Mei, Y., Leng, Q., Leng, B., Liu, Z., et al. (2010). ALDH1A1 is a marker for malignant prostate stem cells and predictor of prostate cancer patients’ outcome. Lab. Invest. 90, 234–244. doi: 10.1038/labinvest.2009.127
Liao, C., Sun, Q., Liang, B., Shen, J., and Shuai, X. (2011). Targeting EGFR-overexpressing tumor cells using Cetuximab-immunomicelles loaded with doxorubicin and superparamagnetic iron oxide. Eur. J. Radiol. 80, 699–705. doi: 10.1016/j.ejrad.2010.08.005
Ling, Y., Wei, K., Luo, Y., Gao, X., and Zhong, S. (2011). Dual docetaxel/superparamagnetic iron oxide loaded nanoparticles for both targeting magnetic resonance imaging and cancer therapy. Biomaterials 32, 7139–7150. doi: 10.1016/j.biomaterials.2011.05.089
Lipinski, R. J., Hutson, P. R., Hannam, P. W., Nydza, R. J., Washington, I. M., Moore, R. W., et al. (2008). Dose- and route-dependent teratogenicity, toxicity, and pharmacokinetic profiles of the hedgehog signaling antagonist cyclopamine in the mouse. Toxicol. Sci. 104, 189–197. doi: 10.1093/toxsci/kfn076
Lu, J., Getz, G., Miska, E. A., Alvarez-Saavedra, E., Lamb, J., Peck, D., et al. (2005). MicroRNA expression profiles classify human cancers. Nature 435, 834–838. doi: 10.1038/nature03702
Lu, W., Lin, C., Roberts, M. J., Waud, W. R., Piazza, G. A., and Li, Y. (2011). Niclosamide suppresses cancer cell growth by inducing Wnt co-receptor LRP6 degradation and inhibiting the Wnt/beta-catenin pathway. PLoS ONE 6:e29290. doi: 10.1371/journal.pone.0029290
Lu, W., Tinsley, H. N., Keeton, A., Qu, Z., Piazza, G. A., and Li, Y. (2009). Suppression of Wnt/beta-catenin signaling inhibits prostate cancer cell proliferation. Eur. J. Pharmacol. 602, 8–14. doi: 10.1016/j.ejphar.2008.10.053
Lymperi, S., Ferraro, F., and Scadden, D. T. (2010). The HSC niche concept has turned 31. Ann. N. Y. Acad. Sci. 1192, 12–18. doi: 10.1111/j.1749-6632.2009.05223.x
Ma, Y., Liang, D., Liu, J., Axcrona, K., Kvalheim, G., Stokke, T., et al. (2011). Prostate cancer cell lines under hypoxia exhibit greater stem-like properties. PLoS ONE 6:e29170. doi: 10.1371/journal.pone.0029170
Mann, K. K., Wallner, B., Lossos, I. S., and Miller, W. H. Jr., (2009). Darinaparsin: a novel organic arsenical with promising anticancer activity. Expert Opin. Investig. Drugs 18, 1727–1734. doi: 10.1517/13543780903282759
Marhold, M., Tomasich, E., El-Gazzar, A., Heller, G., Spittler, A., Horvat, R., et al. (2015). HIF1alpha regulates mTOR signaling and viability of prostate cancer stem cells. Mol. Cancer Res. 13, 556–564. doi: 10.1158/1541-7786.MCR-14-0153-T
Masui, S., Nakatake, Y., Toyooka, Y., Shimosato, D., Yagi, R., Takahashi, K., et al. (2007). Pluripotency governed by Sox2 via regulation of Oct3/4 expression in mouse embryonic stem cells. Nat. Cell Biol. 9, 625–635. doi: 10.1038/ncb1589
Miki, J., Furusato, B., Li, H., Gu, Y., Takahashi, H., Egawa, S., et al. (2007). Identification of putative stem cell markers, CD133 and CXCR4, in hTERT-immortalized primary nonmalignant and malignant tumor-derived human prostate epithelial cell lines and in prostate cancer specimens. Cancer Res. 67, 3153–3161. doi: 10.1158/0008-5472.CAN-06-4429
Mitchell, P. S., Parkin, R. K., Kroh, E. M., Fritz, B. R., Wyman, S. K., Pogosova-Agadjanyan, E. L., et al. (2008). Circulating microRNAs as stable blood-based markers for cancer detection. Proc. Natl. Acad. Sci. U.S.A. 105, 10513–10518. doi: 10.1073/pnas.0804549105
Moustakas, A., and Heldin, C. H. (2016). Mechanisms of TGFbeta-induced epithelial-mesenchymal transition. J. Clin. Med. 5:E63. doi: 10.3390/jcm5070063
Mulholland, D. J., Cheng, H., Reid, K., Rennie, P. S., and Nelson, C. C. (2002). The androgen receptor can promote beta-catenin nuclear translocation independently of adenomatous polyposis coli. J. Biol. Chem. 277, 17933–17943. doi: 10.1074/jbc.M200135200
Nagesh, P. K., Johnson, N. R., Boya, V. K., Chowdhury, P., Othman, S. F., Khalilzad-Sharghi, V., et al. (2016). PSMA targeted docetaxel-loaded superparamagnetic iron oxide nanoparticles for prostate cancer. Colloids Surf. B Biointerfaces 144, 8–20. doi: 10.1016/j.colsurfb.2016.03.071
Ni, J., Cozzi, P., Hao, J., Duan, W., Graham, P., Kearsley, J., et al. (2014). Cancer stem cells in prostate cancer chemoresistance. Curr. Cancer Drug Targets 14, 225–240. doi: 10.2174/1568009614666140328152459
Nieto, M. A., Huang, R. Y., Jackson, R. A., and Thiery, J. P. (2016). Emt: 2016. Cell 166, 21–45. doi: 10.1016/j.cell.2016.06.028
O’Brien, C. A., Pollett, A., Gallinger, S., and Dick, J. E. (2007). A human colon cancer cell capable of initiating tumour growth in immunodeficient mice. Nature 445, 106–110. doi: 10.1038/nature05372
Pandey, B., Demchenko, A. V., and Stine, K. J. (2012). Nanoporous gold as a solid support for protein immobilization and development of an electrochemical immunoassay for prostate specific antigen and carcinoembryonic antigen. Microchim. Acta 179, 71–81. doi: 10.1007/s00604-012-0870-x
Panini, N. V., Messina, G. A., Salinas, E., Fernandez, H., and Raba, J. (2008). Integrated microfluidic systems with an immunosensor modified with carbon nanotubes for detection of prostate specific antigen (PSA) in human serum samples. Biosens. Bioelectron. 23, 1145–1151. doi: 10.1016/j.bios.2007.11.003
Parker, C., Nilsson, S., Heinrich, D., Helle, S. I., O’Sullivan, J. M., Fossa, S. D., et al. (2013). Alpha emitter radium-223 and survival in metastatic prostate cancer. N. Engl. J. Med. 369, 213–223. doi: 10.1056/NEJMoa1213755
Patrawala, L., Calhoun, T., Schneider-Broussard, R., Li, H., Bhatia, B., Tang, S., et al. (2006). Highly purified CD44+ prostate cancer cells from xenograft human tumors are enriched in tumorigenic and metastatic progenitor cells. Oncogene 25, 1696–1708. doi: 10.1038/sj.onc.1209327
Pattabiraman, D. R., and Weinberg, R. A. (2014). Tackling the cancer stem cells – what challenges do they pose? Nat. Rev. Drug Discov. 13, 497–512. doi: 10.1038/nrd4253
Pearce, D. J., Taussig, D., Simpson, C., Allen, K., Rohatiner, A. Z., Lister, T. A., et al. (2005). Characterization of cells with a high aldehyde dehydrogenase activity from cord blood and acute myeloid leukemia samples. Stem Cells 23, 752–760. doi: 10.1634/stemcells.2004-0292
Perry, J. M., He, X. C., Sugimura, R., Grindley, J. C., Haug, J. S., Ding, S., et al. (2011). Cooperation between both Wnt/{beta}-catenin and PTEN/PI3K/Akt signaling promotes primitive hematopoietic stem cell self-renewal and expansion. Genes Dev. 25, 1928–1942. doi: 10.1101/gad.17421911
Petrylak, D. P., Tangen, C. M., Hussain, M. H., Lara, P. N. Jr., Jones, J. A., Taplin, M. E., et al. (2004). Docetaxel and estramustine compared with mitoxantrone and prednisone for advanced refractory prostate cancer. N. Engl. J. Med. 351, 1513–1520. doi: 10.1056/NEJMoa041318
Petzer, A. L., Zandstra, P. W., Piret, J. M., and Eaves, C. J. (1996). Differential cytokine effects on primitive (CD34+CD38-) human hematopoietic cells: novel responses to Flt3-ligand and thrombopoietin. J. Exp. Med. 183, 2551–2558. doi: 10.1084/jem.183.6.2551
Pfeiffer, M. J., Smit, F. P., Sedelaar, J. P., and Schalken, J. A. (2011). Steroidogenic enzymes and stem cell markers are upregulated during androgen deprivation in prostate cancer. Mol. Med. 17, 657–664. doi: 10.2119/molmed.2010.00143
Pinto, D., Gregorieff, A., Begthel, H., and Clevers, H. (2003). Canonical Wnt signals are essential for homeostasis of the intestinal epithelium. Genes Dev. 17, 1709–1713. doi: 10.1101/gad.267103
Qin, J., Liu, X., Laffin, B., Chen, X., Choy, G., Jeter, C. R., et al. (2012). The PSA(-/lo) prostate cancer cell population harbors self-renewing long-term tumor-propagating cells that resist castration. Cell Stem Cell 10, 556–569. doi: 10.1016/j.stem.2012.03.009
Qin, W., Du, N., Zhang, L., Wu, X., Hu, Y., Li, X., et al. (2015). Genistein alleviates pressure overload-induced cardiac dysfunction and interstitial fibrosis in mice. Br. J. Pharmacol. 172, 5559–5572. doi: 10.1111/bph.13002
Reiter, R. E., Gu, Z., Watabe, T., Thomas, G., Szigeti, K., Davis, E., et al. (1998). Prostate stem cell antigen: a cell surface marker overexpressed in prostate cancer. Proc. Natl. Acad. Sci. U.S.A. 95, 1735–1740. doi: 10.1073/pnas.95.4.1735
Richardson, G. D., Robson, C. N., Lang, S. H., Neal, D. E., Maitland, N. J., and Collins, A. T. (2004). CD133, a novel marker for human prostatic epithelial stem cells. J. Cell Sci. 117(Pt 16), 3539–3545. doi: 10.1242/jcs.01222
Ryan, C. J., Smith, M. R., de Bono, J. S., Molina, A., Logothetis, C. J., de Souza, P., et al. (2013). Abiraterone in metastatic prostate cancer without previous chemotherapy. N. Engl. J. Med. 368, 138–148. doi: 10.1056/NEJMoa1209096
Saini, S., Majid, S., Shahryari, V., Arora, S., Yamamura, S., Chang, I., et al. (2012). miRNA-708 control of CD44(+) prostate cancer-initiating cells. Cancer Res. 72, 3618–3630. doi: 10.1158/0008-5472.CAN-12-0540
Salas, T. R., Kim, J., Vakar-Lopez, F., Sabichi, A. L., Troncoso, P., Jenster, G., et al. (2004). Glycogen synthase kinase-3 beta is involved in the phosphorylation and suppression of androgen receptor activity. J. Biol. Chem. 279, 19191–19200. doi: 10.1074/jbc.M309560200
Salimi, A., Kavosi, B., Fathi, F., and Hallaj, R. (2013). Highly sensitive immunosensing of prostate-specific antigen based on ionic liquid-carbon nanotubes modified electrode: application as cancer biomarker for prostate biopsies. Biosens. Bioelectron. 42, 439–446. doi: 10.1016/j.bios.2012.10.053
Salti, G. I., Grewal, S., Mehta, R. R., Das, Gupta T.K., Boddie, A. W. Jr., and Constantinou, A. I. (2000). Genistein induces apoptosis and topoisomerase II-mediated DNA breakage in colon cancer cells. Eur. J. Cancer 36, 796–802. doi: 10.1016/S0959-8049(00)00017-4
Salvatori, L., Caporuscio, F., Verdina, A., Starace, G., Crispi, S., Nicotra, M. R., et al. (2012). Cell-to-cell signaling influences the fate of prostate cancer stem cells and their potential to generate more aggressive tumors. PLoS ONE 7:e31467. doi: 10.1371/journal.pone.0031467
Sanchez, P., Hernandez, A. M., Stecca, B., Kahler, A. J., DeGueme, A. M., Barrett, A., et al. (2004). Inhibition of prostate cancer proliferation by interference with SONIC HEDGEHOG-GLI1 signaling. Proc. Natl. Acad. Sci. U.S.A. 101, 12561–12566. doi: 10.1073/pnas.0404956101
Schatton, T., Murphy, G. F., Frank, N. Y., Yamaura, K., Waaga-Gasser, A. M., Gasser, M., et al. (2008). Identification of cells initiating human melanomas. Nature 451, 345–349. doi: 10.1038/nature06489
Scher, H. I., Fizazi, K., Saad, F., Taplin, M. E., Sternberg, C. N., Miller, K., et al. (2012). Increased survival with enzalutamide in prostate cancer after chemotherapy. N. Engl. J. Med. 367, 1187–1197. doi: 10.1056/NEJMoa1207506
Sharifi, N., Hurt, E. M., and Farrar, W. L. (2008). Androgen receptor expression in prostate cancer stem cells: Is there a conundrum? Cancer Chemother. Pharmacol. 62, 921–923. doi: 10.1007/s00280-007-0659-5
Sharma, M., Chuang, W. W., and Sun, Z. (2002). Phosphatidylinositol 3-kinase/Akt stimulates androgen pathway through GSK3beta inhibition and nuclear beta-catenin accumulation. J. Biol. Chem. 277, 30935–30941. doi: 10.1074/jbc.M201919200
Shiozawa, Y., Berry, J. E., Eber, M. R., Jung, Y., Yumoto, K., Cackowski, F. C., et al. (2016). The marrow niche controls the cancer stem cell phenotype of disseminated prostate cancer. Oncotarget 7, 41217–41232. doi: 10.18632/oncotarget.9251
Shiozawa, Y., Pedersen, E. A., Havens, A. M., Jung, Y., Mishra, A., Joseph, J., et al. (2011). Human prostate cancer metastases target the hematopoietic stem cell niche to establish footholds in mouse bone marrow. J. Clin. Invest. 121, 1298–1312. doi: 10.1172/JCI43414
Siddiqui, I. A., Adhami, V. M., Bharali, D. J., Hafeez, B. B., Asim, M., Khwaja, S. I., et al. (2009). Introducing nanochemoprevention as a novel approach for cancer control: proof of principle with green tea polyphenol epigallocatechin-3-gallate. Cancer Res. 69, 1712–1716. doi: 10.1158/0008-5472.CAN-08-3978
Siegel, R. L., Miller, K. D., and Jemal, A. (2017). Cancer statistics, 2017. CA Cancer J. Clin. 67, 7–30. doi: 10.3322/caac.21387
Singh, S. K., Clarke, I. D., Terasaki, M., Bonn, V. E., Hawkins, C., Squire, J., et al. (2003). Identification of a cancer stem cell in human brain tumors. Cancer Res. 63, 5821–5828.
Statkiewicz, M., Maryan, N., Lipiec, A., Grecka, E., Grygorowicz, M. A., Omiotek, M., et al. (2014). The role of the SHH gene in prostate cancer cell resistance to paclitaxel. Prostate 74, 1142–1152. doi: 10.1002/pros.22830
Stenman, U. H., Leinonen, J., Zhang, W. M., and Finne, P. (1999). Prostate-specific antigen. Semin. Cancer Biol. 9, 83–93. doi: 10.1006/scbi.1998.0086
Stuart, E. C., Scandlyn, M. J., and Rosengren, R. J. (2006). Role of epigallocatechin gallate (EGCG) in the treatment of breast and prostate cancer. Life Sci. 79, 2329–2336. doi: 10.1016/j.lfs.2006.07.036
Swaminathan, S. K., Roger, E., Toti, U., Niu, L., Ohlfest, J. R., and Panyam, J. (2013). CD133-targeted paclitaxel delivery inhibits local tumor recurrence in a mouse model of breast cancer. J. Control. Release 171, 280–287. doi: 10.1016/j.jconrel.2013.07.014
Takebe, N., Harris, P. J., Warren, R. Q., and Ivy, S. P. (2011). Targeting cancer stem cells by inhibiting Wnt, Notch, and Hedgehog pathways. Nat. Rev. Clin. Oncol. 8, 97–106. doi: 10.1038/nrclinonc.2010.196
Talati, P. G., Gu, L., Ellsworth, E. M., Girondo, M. A., Trerotola, M., Hoang, D. T., et al. (2015). Jak2-Stat5a/b signaling induces epithelial-to-mesenchymal transition and stem-like cell properties in prostate cancer. Am. J. Pathol. 185, 2505–2522. doi: 10.1016/j.ajpath.2015.04.026
Tannock, I. F., de Wit, R., Berry, W. R., Horti, J., Pluzanska, A., Chi, K. N., et al. (2004). Docetaxel plus prednisone or mitoxantrone plus prednisone for advanced prostate cancer. N. Engl. J. Med. 351, 1502–1512. doi: 10.1056/NEJMoa040720
Terry, S., Yang, X., Chen, M. W., Vacherot, F., and Buttyan, R. (2006). Multifaceted interaction between the androgen and Wnt signaling pathways and the implication for prostate cancer. J. Cell. Biochem. 99, 402–410. doi: 10.1002/jcb.20983
Thaxton, C. S., Elghanian, R., Thomas, A. D., Stoeva, S. I., Lee, J. S., Smith, N. D., et al. (2009). Nanoparticle-based bio-barcode assay redefines “undetectable” PSA and biochemical recurrence after radical prostatectomy. Proc. Natl. Acad. Sci. U.S.A. 106, 18437–18442. doi: 10.1073/pnas.0904719106
Thorne, C. A., Hanson, A. J., Schneider, J., Tahinci, E., Orton, D., Cselenyi, C. S., et al. (2010). Small-molecule inhibition of Wnt signaling through activation of casein kinase 1alpha. Nat. Chem. Biol. 6, 829–836. doi: 10.1038/nchembio.453
Tian, H., Biehs, B., Warming, S., Leong, K. G., Rangell, L., Klein, O. D., et al. (2011). A reserve stem cell population in small intestine renders Lgr5-positive cells dispensable. Nature 478, 255–259. doi: 10.1038/nature10408
Tian, M., and Schiemann, W. P. (2009). The TGF-beta paradox in human cancer: an update. Future Oncol. 5, 259–271. doi: 10.2217/14796694.5.2.259
Toy, R., Bauer, L., Hoimes, C., Ghaghada, K. B., and Karathanasis, E. (2014). Targeted nanotechnology for cancer imaging. Adv. Drug Deliv. Rev. 76, 79–97. doi: 10.1016/j.addr.2014.08.002
Tran, H. V., Piro, B., Reisberg, S., Tran, L. D., Duc, H. T., and Pham, M. C. (2013). Label-free and reagentless electrochemical detection of microRNAs using a conducting polymer nanostructured by carbon nanotubes: application to prostate cancer biomarker miR-141. Biosens. Bioelectron. 49, 164–169. doi: 10.1016/j.bios.2013.05.007
Truica, C. I., Byers, S., and Gelmann, E. P. (2000). Beta-catenin affects androgen receptor transcriptional activity and ligand specificity. Cancer Res. 60, 4709–4713.
Van Audenhove, I., and Gettemans, J. (2016). Nanobodies as versatile tools to understand, diagnose, visualize and treat cancer. EBioMedicine 8, 40–48. doi: 10.1016/j.ebiom.2016.04.028
van den Hoogen, C., van der Horst, G., Cheung, H., Buijs, J. T., Lippitt, J. M., Guzman-Ramirez, N., et al. (2010). High aldehyde dehydrogenase activity identifies tumor-initiating and metastasis-initiating cells in human prostate cancer. Cancer Res. 70, 5163–5173. doi: 10.1158/0008-5472.CAN-09-3806
Vander Griend, D. J., Karthaus, W. L., Dalrymple, S., Meeker, A., DeMarzo, A. M., and Isaacs, J. T. (2008). The role of CD133 in normal human prostate stem cells and malignant cancer-initiating cells. Cancer Res. 68, 9703–9711. doi: 10.1158/0008-5472.CAN-08-3084
Voeller, H. J., Truica, C. I., and Gelmann, E. P. (1998). Beta-catenin mutations in human prostate cancer. Cancer Res. 58, 2520–2523.
Wang, C. H., Chiou, S. H., Chou, C. P., Chen, Y. C., Huang, Y. J., and Peng, C. A. (2011). Photothermolysis of glioblastoma stem-like cells targeted by carbon nanotubes conjugated with CD133 monoclonal antibody. Nanomedicine 7, 69–79. doi: 10.1016/j.nano.2010.06.010
Wang, H., Zhang, Y., Yu, H., Wu, D., Ma, H., Li, H., et al. (2013). Label-free electrochemical immunosensor for prostate-specific antigen based on silver hybridized mesoporous silica nanoparticles. Anal. Biochem. 434, 123–127. doi: 10.1016/j.ab.2012.11.012
Wei, X., Senanayake, T. H., Warren, G., and Vinogradov, S. V. (2013). Hyaluronic acid-based nanogel-drug conjugates with enhanced anticancer activity designed for the targeting of CD44-positive and drug-resistant tumors. Bioconjug. Chem. 24, 658–668. doi: 10.1021/bc300632w
Whitesides, G. M., Mathias, J. P., and Seto, C. T. (1991). Molecular self-assembly and nanochemistry: a chemical strategy for the synthesis of nanostructures. Science 254, 1312–1319. doi: 10.1126/science.1962191
Wicki, A., Witzigmann, D., Balasubramanian, V., and Huwyler, J. (2015). Nanomedicine in cancer therapy: challenges, opportunities, and clinical applications. J. Control. Release 200, 138–157. doi: 10.1016/j.jconrel.2014.12.030
Wu, Y., Wang, W., Chen, Y., Huang, K., Shuai, X., Chen, Q., et al. (2010). The investigation of polymer-siRNA nanoparticle for gene therapy of gastric cancer in vitro. Int. J. Nanomedicine 5, 129–136. doi: 10.2147/IJN.S8503
Yang, C., Xiong, F., Wang, J., Dou, J., Chen, J., Chen, D., et al. (2014). Anti-ABCG2 monoclonal antibody in combination with paclitaxel nanoparticles against cancer stem-like cell activity in multiple myeloma. Nanomedicine 9, 45–60. doi: 10.2217/nnm.12.216
Yang, F., Li, X., Sharma, M., Sasaki, C. Y., Longo, D. L., Lim, B., et al. (2002). Linking beta-catenin to androgen-signaling pathway. J. Biol. Chem. 277, 11336–11344. doi: 10.1074/jbc.M111962200
Yang, R., Mondal, G., Wen, D., and Mahato, R. I. (2016). Combination therapy of paclitaxel and cyclopamine polymer-drug conjugates to treat advanced prostate cancer. Nanomedicine 13, 391–401. doi: 10.1016/j.nano.2016.07.017
Yang, X., Chen, M. W., Terry, S., Vacherot, F., Bemis, D. L., Capodice, J., et al. (2006). Complex regulation of human androgen receptor expression by Wnt signaling in prostate cancer cells. Oncogene 25, 3436–3444. doi: 10.1038/sj.onc.1209366
Yang, Z. F., Ho, D. W., Ng, M. N., Lau, C. K., Yu, W. C., Ngai, P., et al. (2008). Significance of CD90+ cancer stem cells in human liver cancer. Cancer Cell 13, 153–166. doi: 10.1016/j.ccr.2008.01.013
Yuhi, T., Nagatani, N., Endo, T., Kerman, K., Takata, M., Konaka, H., et al. (2006). Resin-based micropipette tip for immunochromatographic assays in urine samples. J. Immunol. Methods 312, 54–60. doi: 10.1016/j.jim.2006.02.011
Zhang, L., Gu, F. X., Chan, J. M., Wang, A. Z., Langer, R. S., and Farokhzad, O. C. (2008). Nanoparticles in medicine: therapeutic applications and developments. Clin. Pharmacol. Ther. 83, 761–769. doi: 10.1038/sj.clpt.6100400
Zhang, L., Li, L., Jiao, M., Wu, D., Wu, K., Li, X., et al. (2012). Genistein inhibits the stemness properties of prostate cancer cells through targeting Hedgehog-Gli1 pathway. Cancer Lett. 323, 48–57. doi: 10.1016/j.canlet.2012.03.037
Zhang, S., Balch, C., Chan, M. W., Lai, H. C., Matei, D., Schilder, J. M., et al. (2008). Identification and characterization of ovarian cancer-initiating cells from primary human tumors. Cancer Res. 68, 4311–4320. doi: 10.1158/0008-5472.CAN-08-0364
Zhao, M., and Li, L. (2015). Regulation of hematopoietic stem cells in the niche. Sci. China Life Sci. 58, 1209–1215. doi: 10.1007/s11427-015-4960-y
Zhao, S., Fu, J., Liu, X., Wang, T., Zhang, J., and Zhao, Y. (2012). Activation of Akt/GSK-3beta/beta-catenin signaling pathway is involved in survival of neurons after traumatic brain injury in rats. Neurol. Res. 34, 400–407. doi: 10.1179/1743132812Y.0000000025
Zhong, D., Zhang, H. J., Jiang, Y. D., Wu, P., Qi, H., Cai, C., et al. (2016). Saikosaponin-d: a potential chemotherapeutics in castration resistant prostate cancer by suppressing cancer metastases and cancer stem cell phenotypes. Biochem. Biophys. Res. Commun. 474, 722–729. doi: 10.1016/j.bbrc.2016.05.017
Zhou, Y., and Kopecek, J. (2013). Biological rationale for the design of polymeric anti-cancer nanomedicines. J. Drug Target 21, 1–26. doi: 10.3109/1061186X.2012.723213
Zhou, Y., Yang, J., and Kopecek, J. (2012). Selective inhibitory effect of HPMA copolymer-cyclopamine conjugate on prostate cancer stem cells. Biomaterials 33, 1863–1872. doi: 10.1016/j.biomaterials.2011.11.029
Zhou, Y., Yang, J., Rhim, J. S., and Kopecek, J. (2013). HPMA copolymer-based combination therapy toxic to both prostate cancer stem/progenitor cells and differentiated cells induces durable anti-tumor effects. J. Control. Release 172, 946–953. doi: 10.1016/j.jconrel.2013.09.005
Keywords: prostate cancer, prostate cancer stem cell, Wnt signaling, nanotechnology, cancer therapy
Citation: Qin W, Zheng Y, Qian B -Z and Zhao M (2017) Prostate Cancer Stem Cells and Nanotechnology: A Focus on Wnt Signaling. Front. Pharmacol. 8:153. doi: 10.3389/fphar.2017.00153
Received: 02 November 2016; Accepted: 09 March 2017;
Published: 28 March 2017.
Edited by:
Olivier Cuvillier, Centre National de la Recherche Scientifique (CNRS), FranceReviewed by:
Stéphane Terry, Institut Gustave Roussy, FranceBradley Doble, McMaster University, Canada
Copyright © 2017 Qin, Zheng, Qian and Zhao. This is an open-access article distributed under the terms of the Creative Commons Attribution License (CC BY). The use, distribution or reproduction in other forums is permitted, provided the original author(s) or licensor are credited and that the original publication in this journal is cited, in accordance with accepted academic practice. No use, distribution or reproduction is permitted which does not comply with these terms.
*Correspondence: Bin-Zhi Qian, YmluemhpLnFpYW5AZWQuYWMudWs= Meng Zhao, emhhb20zOEBtYWlsLnN5c3UuZWR1LmNu
†These authors have contributed equally to this work.