- 1Pharmacogenomics Laboratory, Centre Hospitalier Universitaire (CHU) de Québec Research Center, Québec, QC, Canada
- 2Faculty of Pharmacy, Laval University, Québec, QC, Canada
- 3Clinical and Experimental Pharmacology, IRCCS National Cancer Institute ‘Centro di Riferimento Oncologico’, Aviano, Italy
- 4Centre Hospitalier Universitaire (CHU) de Québec Research Center, Québec, QC, Canada
- 5Faculty of Medicine, Laval University, Québec, QC, Canada
- 6Division of Medical Oncology, Department of Medicine, Ottawa Hospital, University of Ottawa, Ottawa, ON, Canada
- 7Medical Oncology Unit, IRCCS National Cancer Institute ‘Centro di Riferimento Oncologico’, Aviano, Italy
- 8Medical Oncology Unit, San Filippo Neri Hospital, Rome, Italy
Hepatocyte nuclear factor 1-alpha (HNF1A) is a liver-enriched transcription factor that plays a key role in many aspects of hepatic functions including detoxification processes. We examined whether HNF1A polymorphisms are associated with clinical outcomes in two independent cohorts combining 417 European ancestry patients with metastatic colorectal cancer (mCRC) treated with irinotecan-based chemotherapy. The intronic rs2244608A>G marker was predictive of an improved progression-free survival with a trend in the Canadian cohort and reaching significance in the Italian cohort, with hazard ratios (HR) of 0.74 and 0.72, P = 0.076 and 0.038, respectively. A strong association between rs2244608A>G and improved PFS was found in the combined analysis of both cohorts (HR = 0.72; P = 0.002). Consistent with an altered HNF1A function, mCRC carriers of the rs2244608G minor allele displayed enhanced drug exposure by 45% (P = 0.032) compared to non-carriers. In Caucasians, rs2244608A>G is in strong linkage with the coding variant rs1169288c.79A>C (HNF1A p.I27L). In healthy donors, we observed an altered hepatic (ABCC1, P = 0.009, ABCC2, P = 0.048 and CYP3A5, P = 0.001; n = 89) and intestinal (TOP1, P = 0.004; n = 75) gene expression associated with the rs1169288C allele. In addition, the rs1169288C polymorphism could significantly increase the ABCC1 promoter activity by 27% (P = 0.008) and 15% (P = 0.041) in the human kidney HEK293 and the human liver HepG2 cell lines, respectively. Our findings suggest that the HNF1A rs2244608, or the tightly linked functional coding variant p.I27L, might be a potential prognostic marker with irinotecan-based regimens.
Introduction
The camptothecin derivative irinotecan (CPT-11) is a widely used chemotherapeutic agent for the treatment of solid tumors, particularly for metastatic carcinoma of the colon or rectum. A variety of first-line regimens such as irinotecan, 5-fluorouracil (5-FU) and leucovorin (FOLFIRI) used for mCRC in combination with targeted therapy improves outcomes (Fujita et al., 2015). Its active metabolite SN-38 inhibits the replication enzyme topoisomerase I (TOP1), inducing DNA damage and cell death in actively replicating cells leading to S-phase-specific cell killing. The clinical benefits from irinotecan-based regimens are variable among patients whereas sequencing and method of administration influence toxicity. Predictive and prognostic biomarkers are critical in identifying those who would most benefit from the chemotherapy. A number of genetic variants in genes involved in irinotecan pharmacology have been described and may help predict benefit of irinotecan-based chemotherapy. Most notably, the polymorphism UGT1A1∗28, leading to a decreased UGT1A1 enzyme expression and a reduced glucuronidation of the active metabolite SN-38, is a well-established marker of irinotecan-induced severe neutropenia and help guide dosing for treatment of mCRC (Beutler et al., 1998; Barbarino et al., 2014). In addition to help in decreasing the incidence of adverse drug reactions, pharmacogenetic markers can also be useful in predicting irinotecan responsiveness. Irinotecan pharmacology is complex and involves activation into SN-38 by carboxylesterases (CES), inactivation of SN-38 by UDP-glucuronosyltransferases (UGT)-dependent glucuronidation and cytochrome P450 (CYP)-dependent oxidation and hepatic transport of irinotecan and its metabolites by multiple transporters, all potentially contributing to the bioavailability and pharmacological activity of SN-38 (Smith et al., 2006).
Whereas genetic variants in genes regulating irinotecan pharmacology have been investigated, the role of nuclear receptors and other transcriptional factors has not been investigated, despite their potentially broader influence on drug metabolism (Cecchin et al., 2016; De Mattia et al., 2016). Hepatocyte nuclear factor 1-alpha (HNF1A) is a master hepatic transcriptional regulator that influences the expression of numerous genes critical to liver functions. Consistent with an important role in hepatic functions, HNF1A locus and genetic variations in HNF1A are associated with maturity-onset diabetes of the young (MODY3) (Yamagata et al., 1996), type 2 diabetes (Holmkvist et al., 2006; Voight et al., 2010), and coronary artery disease (Liu et al., 2014; Zhou et al., 2017). HNF1A also regulates a number of key genes involved in drug metabolism and disposition including UGT and ATP-binding cassette sub-family C member 2 (ABCC2) expression (Odom et al., 2004, 2007; Aleksunes et al., 2009; Qadri et al., 2009; Hu et al., 2014). Here, we hypothesized that polymorphisms in HNF1A may influence irinotecan pathways and predict clinical outcomes in mCRC patients. We tested the predictive significance of htSNPs in two independent cohorts of mCRC patients of European descent from Canada and Italy treated with the FOLFIRI regimen. We also studied the link with drug exposure in mCRC patients and tissular expression of genes potentially targeted by HNF1A and relevant to irinotecan pharmacology.
Materials and Methods
Patient Characteristics
A first cohort of 167 mCRC Canadian patients and a second cohort comprising 250 mCRC Italian patients were studied. Eligibility criteria included no prior irinotecan-based chemotherapy, histologically confirmed mCRC, a life expectancy of at least 3 months and a good performance status (Eastern Cooperative Oncology Group/World Health Organization Performance Status ≤ 2). White patients from the Canadian cohort were recruited from 2003 to 2012 in three medical centers of eastern Canada and those from the Italian cohort were enrolled from 2002 to 2005 in thirteen medical centers of Northeast Italy. All the patients from the Canadian cohort and the majority (>90%) of the Italian patients were treated with the modified FOLFIRI regimen (irinotecan 180 mg/m2 intravenously for 2 h on day 1 plus 5-fluorouracil (5-FU) 400 mg/m2 bolus followed by continuous infusion for 46 h of 5-FU 2400 mg/m2 plus leucovorin 200 mg/m2) every 2 weeks. The remaining Italian patients received a FOLFIRI regimen (irinotecan 180 mg/m2 intravenously for 2 h on day 1 plus 5-FU 400 mg/m2 bolus followed by 5-FU 600 mg/m2 continuous infusion during 22 h on days 1 and 2 + leucovorin 200 mg/m2 on days 1 and 2 every 2 weeks). Sixty-nine patients from the Canadian cohort received bevacizumab as a co-treatment and six others received another drug or a placebo. Genomic DNA isolation from blood samples was as described (Toffoli et al., 2006; Levesque et al., 2013). For a subset of Italian mCRC patients with available HNF1A genotype (n = 49), pharmacokinetics data including total plasma concentration of irinotecan and its metabolites, SN-38 and SN-38G assessed on serial blood samples collected after drug administration and using high-performance liquid chromatography, were available (Toffoli et al., 2006). Biliary index was calculated with the formula: [CPT-11 AUC X (SN-38 AUC/SN-38G AUC)] and glucuronidation ratio with the formula: (SN-38G AUC/SN-38 AUC). OS, PFS and response rate data in relation to patient characteristics and toxicity in both cohorts are shown in Table 1. This study was carried out in accordance with the regulatory framework for chemical and biological hazards of the CHU de Québec and Centro di Riferimento Oncologico di Aviano. All subjects provided written informed consent in accordance with the Declaration of Helsinki. The protocol was approved by the Comitato Etico Indipendente- Centro di Riferimento Oncologico di Aviano and the CHU de Québec ethics committees.
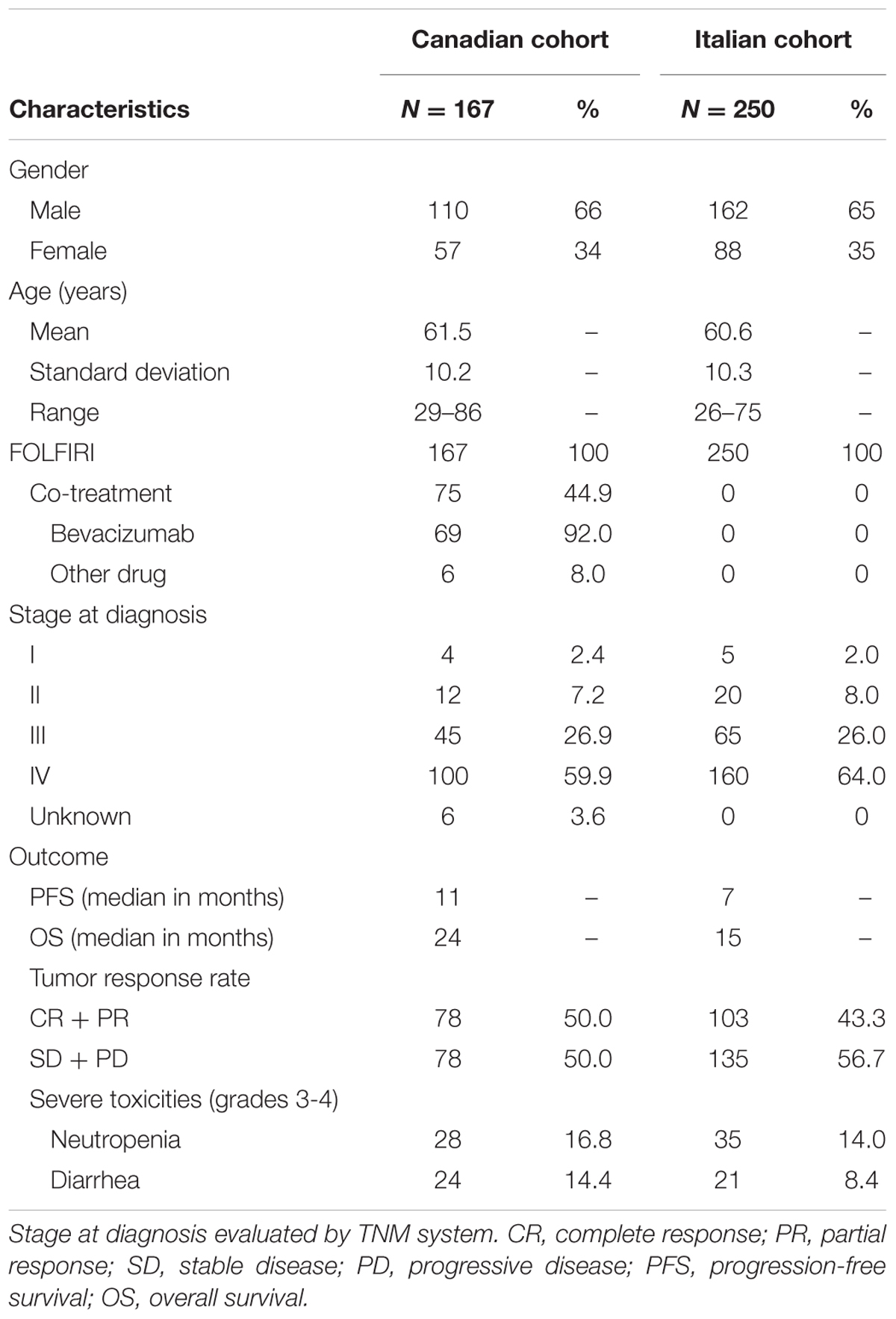
TABLE 1. Characteristics of the study cohorts of mCRC Caucasian patients treated with irinotecan-based chemotherapy (FOLFIRI regimens).
Genetic Analysis
Single-nucleotide polymorphisms in the HNF1A gene and 5 kb flanking regions were identified using the CEU population of the International HapMap Project information1. htSNPs were selected using Haploview v4.2 to tag for SNPs in high LD (r2 > 0.8) (Broad Institute, Cambridge, MA, United States) (Barrett et al., 2005). Allele frequencies and LD data from the 1000 Genomes Project Phase 3 were obtained through the 1000 Genomes Browser – Ensembl2, assembly GRCh37.p13, accessed on March 22, 2017. The list of htSNPs (n = 13) and their associated SNPs is provided in Supplementary Table 1. Genotyping of htSNPs was performed by Sequenom iPLEX matrix-assisted laser desorption/ionization time-of-flight mass spectrometry (Sequenom, San Diego, CA, United States) and using the Illumina BeadXpress platform (Illumina Inc., San Diego, CA, United States). Experimental design of PCR primers, extension primers and genotyping conditions were defined with the SpectroDESIGNER software (Sequenom).
Statistical Analysis
Deviation from Hardy-Weinberg equilibrium was calculated for each htSNP using PLINK v1.07 (Purcell et al., 2007) and those deviating from the equilibrium (P < 0.05) were not retained for further analysis. OS was defined as the period of time between initiation of irinotecan treatment and death. PFS was defined as the period of time between initiation of irinotecan treatment and the first evidence of disease progression, death or last-follow-up. For response rate, patients with complete or partial response were compared to patients with stable disease or progression. Genetic associations with OS and PFS were tested using Cox proportional hazards model (SAS version 9.2, SAS Institute Inc., Cary, NC, United States). Associations with response rate and severe toxicities (neutropenia and diarrhea) were assessed by logistic regression. HRs and odds ratios (ORs) were adjusted for covariates including age, and co-treatment for the Canadian cohort and adjusted for gender, age, cancer primary site, stage at diagnosis, radical surgery and adjuvant chemotherapy in the Italian cohort, as performed in previous reports (Toffoli et al., 2006; Cecchin et al., 2009; Levesque et al., 2013; Chen et al., 2015a,b). Additive, dominant and recessive models were fitted independently. Each marker was tested independently in the two cohorts and then the results were compared. A marker was considered validated when the same effect using the same genetic model was observed in both populations, a trend observed in the Canadian cohort (P < 0.1) replicated in the Italian cohort with a P-value < 0.05. Analysis of pharmacokinetics parameters was performed using GraphPad Prism 5 (GraphPad Software Inc., La Jolla, CA, United States) and differences according to genotypes were tested using a two-tailed Mann–Whitney test. Gene expression data in tissues and associated genotypes were obtained from the GTEx Project portal (http://www.gtexportal.org/home/ on March 16, 2017). Analyses were carried out with GraphPad Prism 5 and differences among groups were tested using a two-tailed Mann–Whitney test. The datasets were obtained from dbGaP at http://www.ncbi.nlm.nih.gov/gap through dbGaP accession number phs000424.v6.p1; project ID 13346. Gene expression values were quantile normalized, as detailed on the GTEx portal. Median expression of genes relevant to irinotecan metabolism in RPKM (reads per kilobase per million mapped reads) from 119 normal liver samples was collected from the GTEx portal. Spearman’s correlation was used to assess correlation between gene expression.
In Vitro Functional Assays
Sequences of primers used in this study are provided in Supplementary Table 4. The HNF1A cDNA was PCR amplified from a construct (FR_HNF1A #31104) kindly provided by Gerhart Ryffel (Addgene, Cambridge, MA, United States) (Senkel et al., 2005) that was subcloned into pcDNA3 EcoRI-EcoRV (Invitrogen, Carlsbad, CA, United States). This construct (pHNF1A_L27_N487) carried the minor alleles rs1169288C and rs2264196A, encoding respectively a leucine at position 27 and an asparagine at position 487. Mutagenesis was performed to obtain the major allele at position 487 (rs2464196G) and either the minor or the major allelle of rs1169288 (pHNF1A_L27_S487 or pHNF1A_I27_S487) using the Q5 Site-directed mutagenesis kit (New England Biolabs, Whitby, ON, Canada). A portion of the ABCC1 promoter (-2.1 kb to -0.3 kb) was PCR amplified from genomic DNA and subcloned into pGL3-Basic vector (Promega Corp., Madison, WI, United States) between SacI and XhoI restriction sites upstream of the firefly luciferase gene. Sanger sequencing was used to verify the sequence of all constructs. HEK293 and HepG2 cells were obtained from ATCC (Rockville, MD, United States). Cell lines were grown as recommended and plated into 24-wells plates at a density of 1.0 × 105 cells/well prior to transfection 24 h later. The pcDNA3_HNF1A constructs (200 ng) were co-transfected with the promoter/pGL3-Basic firefly luciferase reporter vector (200 ng) and pRL-CMV renilla luciferase vector as an internal control (20 ng, Promega Corp., Madison, WI, United States) using Lipofectamine 2000 (Invitrogen, Carlsbad, CA, United States) and OptiMEM-reduced serum media (Life Technologies, Burlington, ON, Canada) as per the manufacturer’s instructions. Cells were lysed 48h after transfection and assessed for luciferase activity using Dual-luciferase reporter assay kit (Promega Corp., Madison, WI, United States). Firefly luciferase activity is expressed as the ratio of firefly/renilla activities relatively to the corresponding control. Differences among conditions were tested by a Student’s two-tailed t-test.
Results
Two cohorts comprising 167 mCRC Canadian patients and 250 mCRC Italian patients were studied, for a total of 417 mCRC patients all treated with the FOLFIRI regimen (leucovorin, 5-fluorouracil and irinotecan) (Table 1). Thirteen HNF1A htSNPs were assessed for their association with OS, PFS, response rate, severe gastrointestinal toxicities and severe neutropenia (listed in Supplementary Table 1). None of the tested HNF1A markers were associated with severe diarrhea in any of the two cohorts examined (data not shown). A few htSNPs were significantly associated with severe neutropenia in the Canadian cohort but not in the Italian cohort (Supplementary Table 2) whereas some htSNPs associated with OS and response rate were significant only in the Italian cohort (Supplementary Table 3).
For HNF1A intronic variant rs2244608A>G, a trend for an improved PFS in the Canadian population with a HR of 0.74 (95% CI = 0.53 – 1.03, P = 0.076) was observed. The same effect using the same genetic model was significant in the Italian cohort with a HR of 0.72 (95% CI = 0.53 – 0.98, P = 0.038) (Table 2). A strong association between rs2244608A>G and improved PFS was also found when both cohorts were combined and analyzed with the same genetic model, adjusted for age and co-treatment, with a HR of 0.72 (95% CI = 0.59 – 0.89, P = 0.002). Consistent with a potential significant influence of this genetic variation, in the analysis of its association with the pharmacokinetics parameters of irinotecan in a subset of patients of the prospective Italian cohort, carriers of the rs2244608G allele (n = 24) presented an enhanced blood exposure to the active metabolite SN-38 (increase of 45% of SN-38 AUC, P = 0.032) compared to carriers of homozygous rs2244608AA genotype (n = 25) whereas no significant changes were noted for CPT-11 AUC and SN-38G AUC (Figures 1A–C). Carriers of the rs2244608G allele also had a 41% increased biliary index (P = 0.021) and a 24% decreased glucuronidation ratio (P = 0.035) compared to homozygote carriers of rs2244608A allele (Figures 1D,E).
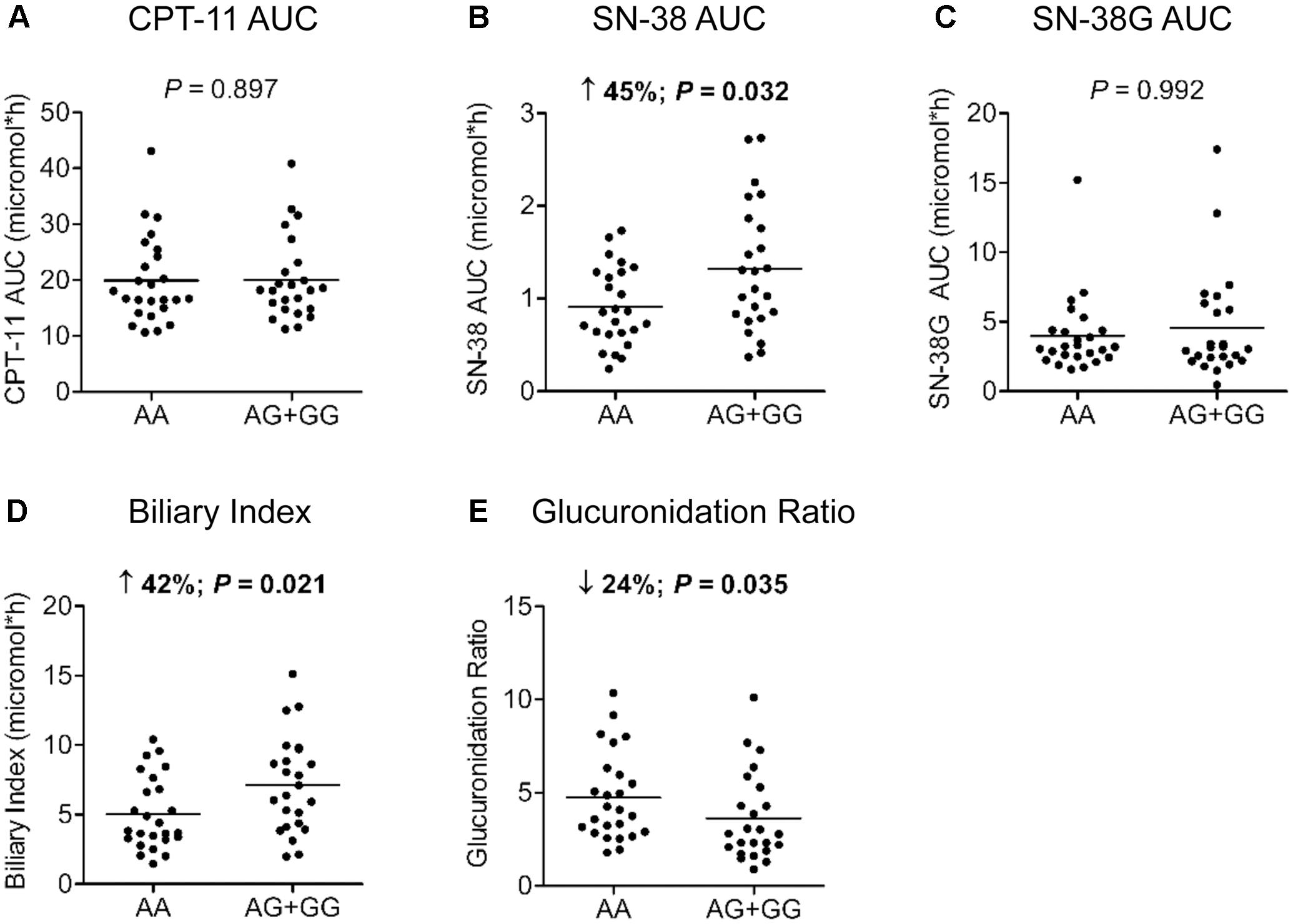
FIGURE 1. Association of HNF1A rs2244608A>G with pharmacokinetics parameters. Differences according to genotypes of 49 mCRC patients from the Italian cohort were tested for (A) irinotecan (CPT-11) AUC; (B) SN-38 AUC; (C) SN-38 glucuronide (SN-38G) AUC; (D) biliary index and (E) glucuronidation ratio using a two-tailed Mann–Whitney test. The bars indicate mean values for each group. Biliary index was calculated with the formula: [CPT-11 AUC X (SN-38 AUC/SN-38G AUC)] and glucuronidation ratio with the formula: (SN-38G AUC/SN-38 AUC). AUC, area under the curve.
The minor allele frequency of rs2244608A>G is 0.32 in the CEU population, 0.34 in the Canadian cohort and 0.33 in the Italian cohort. The htSNP rs2244608A>G is located in the first intron of HNF1A and is in strong LD with 24 other SNPs in individuals of European descent (r2 > 0.80 from 1000 Genomes Phase 3 CEU population) (Figure 2), namely with the non-synonymous coding variant rs1169288c.79A>C located in the first exon of HNF1A, with r2 of 0.98. The minor allele rs1169288C introduces the substitution of the isoleucine 27 to a leucine (p.I27L) in the dimerization domain of HNF1A (Figure 2B) and therefore could functionally contribute to changes observed in circulating SN-38 levels for carriers of the minor rs2244608G allele in almost complete linkage with the minor rs1169288C (p.27L) allele. However, the degree of linkage between the positive marker rs2244608A>G and the coding variant rs1169288A>C varies significantly across ethnic groups. The strongest linkage was observed in individuals of European descent (r2 = 0.98), followed by Asians (Japanese r2 = 0.77 and Chinese r2 = 0.69) and Africans (r2 = 0.23) (Figure 3). All other linked polymorphisms are intronic or located in the untranscribed region upstream of the HNF1A gene, and may influence the expression of HNF1A.
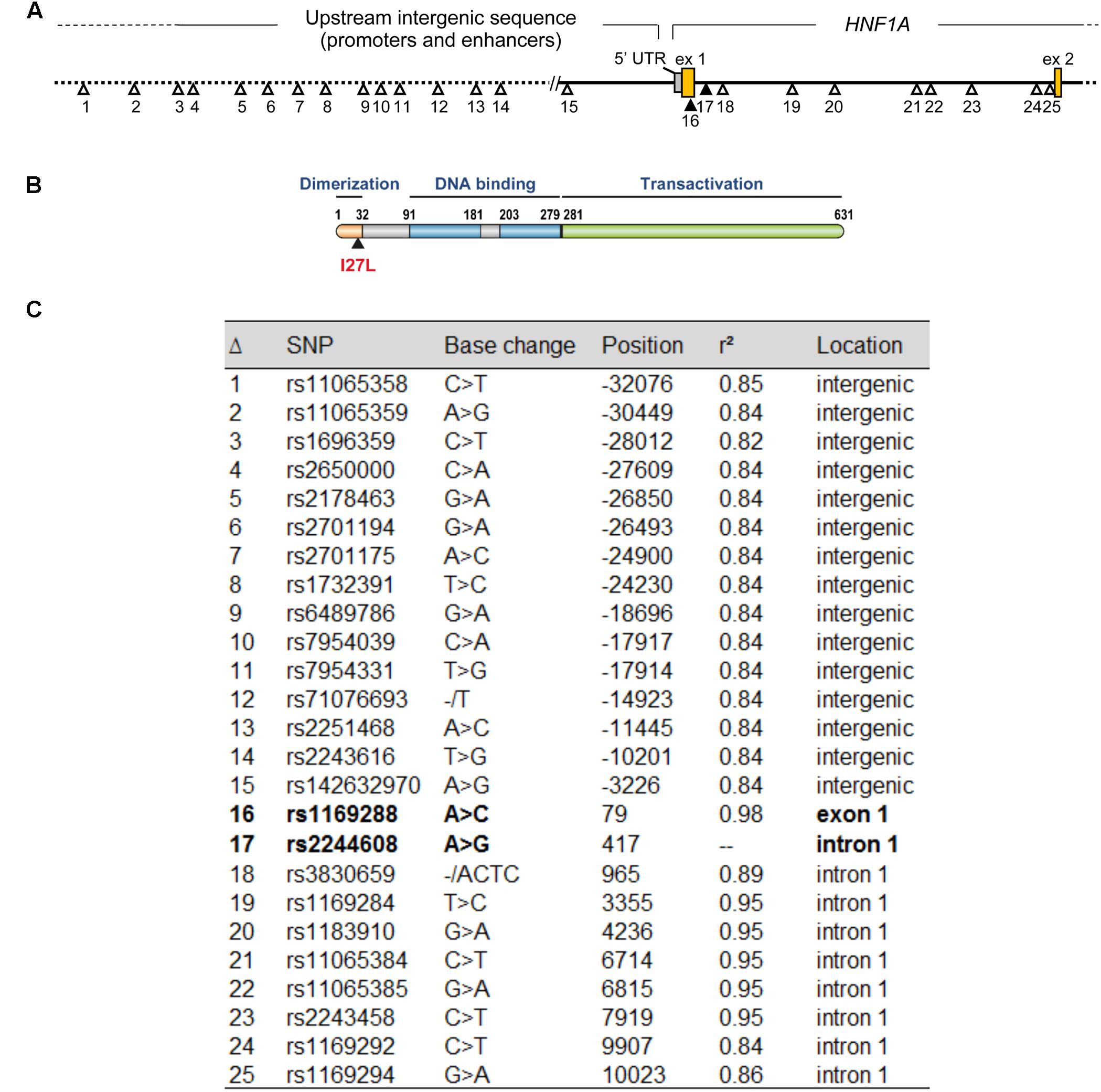
FIGURE 2. The HNF1A marker rs2244608A>G associated with improved PFS is tightly linked (r2 = 0.98) to a number of other SNPs in the HNF1A gene including the non-synonymous coding variant rs1169288A>C (p.I27L). (A) Genetic variants in strong linkage disequilibrium (LD) (r2 > 0.80) with the intronic marker rs2244608 significantly associated with outcome in both populations of mCRC patients treated with irinotecan are represented on the HNF1A gene locus. The positive intronic marker rs2244608 and the non-synonymous rs1169288 variants, represented in bold, are tightly linked (r2 = 0.98) in individuals of European descent. (B) Representation of the HNF1A protein and coordinates in amino acids of its main protein domains. (C) Position (relative to the translation start site) and LD values are shown for polymorphisms presented in A. LD values are from the 1000 Genomes Phase 3 CEU population (European descent).
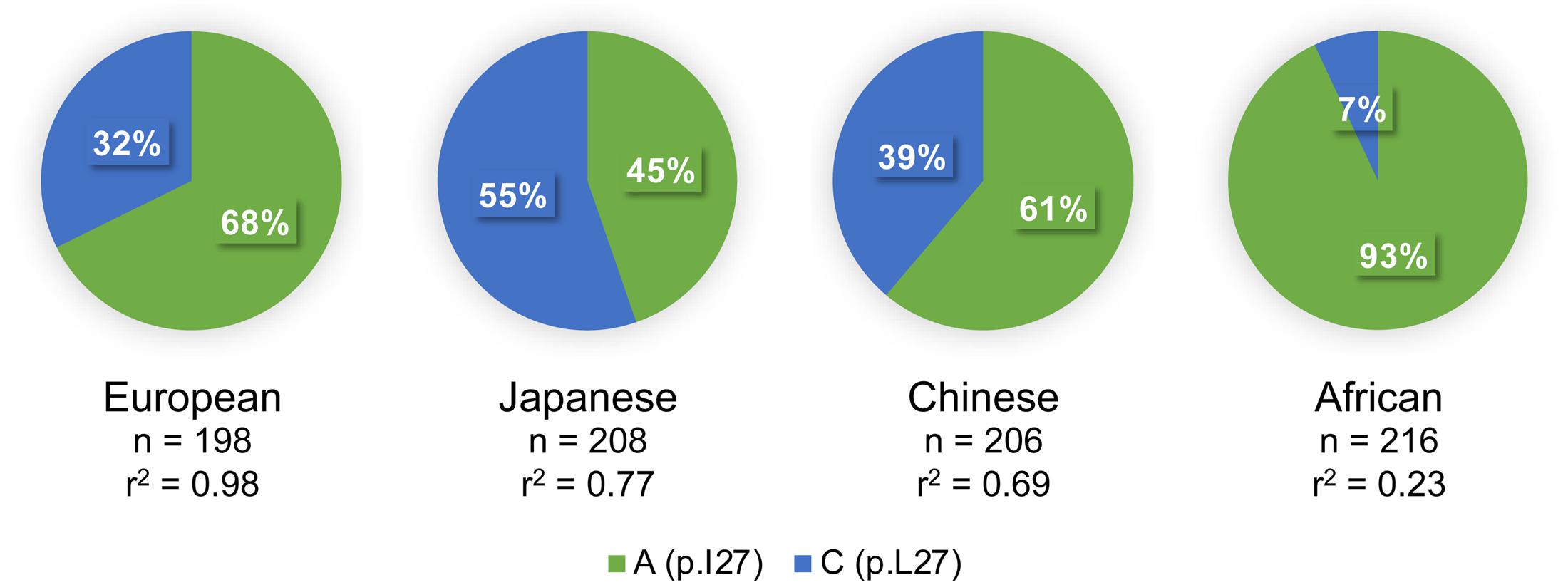
FIGURE 3. Frequency of the non-synonymous rs1169288A>C variation in diverse ethnic groups and its linkage with the intronic marker rs2244608A>G associated with outcome. Linkage disequilibrium (r2) is given for each population. Data were obtained from the 1000 Genomes Phase 3 project. n, number of genotyped alleles. The rs2244608A>G marker is associated with outcome in mCRC patients of European descent.
The analysis of liver tissues from 119 healthy donors indicated that HNF1A as well as ABCC1 and ABCC2 are expressed (data not shown). In support of a potential functional significance of the coding HNF1A variant rs1169288 and based on the analysis of liver samples from 89 healthy donors with available genotype data, we observed an enhanced hepatic expression of ABCC1 (P = 0.009) and a lower expression of ABCC2 (P = 0.048) and CYP3A5 (P = 0.001) in carriers of the minor rs1169288C allele (n = 38 individuals) compared to those carrying the rs1169288AA genotype (n = 51 individuals) (Figures 4A–C). In addition, compared to homozygous carriers of rs1169288A allele (n = 42 individuals), expression of the irinotecan drug target gene TOP1 was significantly higher in the small intestine (P = 0.004) in carriers of the minor allele rs1169288C (n = 33 individuals) (Figure 4D). We also noted that the hepatic expression of HNF1A is inversely correlated to ABCC1 (Spearman ρ = -0.571, P < 0.0001) and a trend for a positive correlation with ABCC2 (Spearman ρ = 0.193, P = 0.058) was observed (Figures 5A,B). In line, a negative correlation between ABCC1 and ABCC2 expressions was observed (Spearman ρ = -0.310, P = 0.002, Figure 5C). When accounting for the rs1169288 genotype, the negative correlation between expression of ABCC1 and HNF1A remained significant in both genotype groups. The negative correlation between ABCC1 and ABCC2 was also preserved but was significant only in homozygous carriers of the reference rs1169288A allele (n = 51), likely due the smaller sample size for rs1169288C carriers (n = 38) (Figure 5D). Relationship to other pathways relevant to irinotecan is provided in Supplementary Figure 1, but none reached significance. A trend was observed for carriers of rs1169288C allele and a decreased HNF1A expression (P = 0.082).
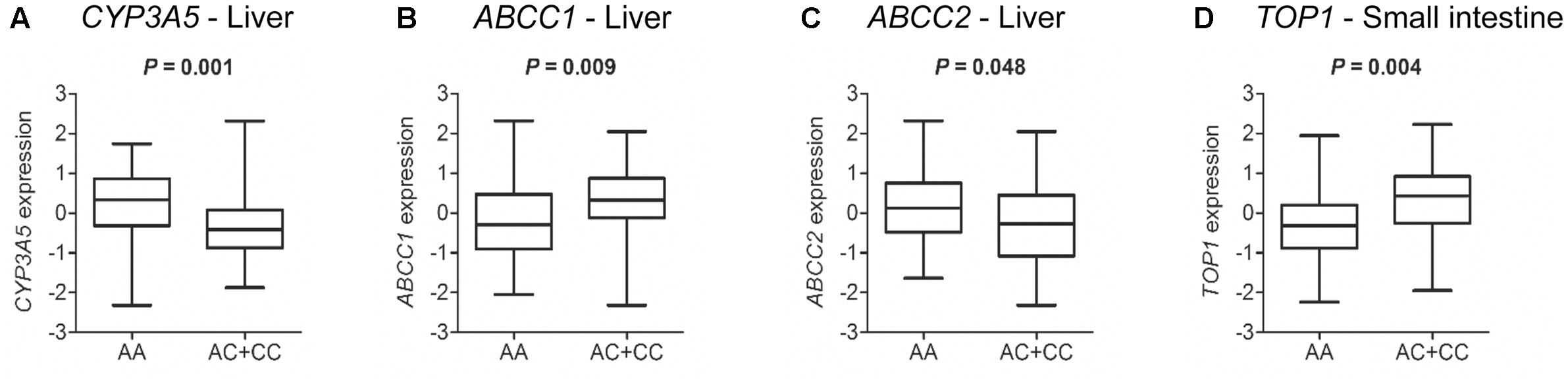
FIGURE 4. The HNF1A rs1169288C variant allele (p.L27) is associated with altered expression of CYP3A5, ABCC1 and ABCC2 transporter genes, and irinotecan drug target TOP1. Gene expression and genotypes were from individuals of the GTEx cohort. P-values were calculated by a two-tailed Mann–Whitney test. (A–C) Normal livers (n = 89 individuals): rs1169288AA, n = 51; rs1169288AC/CC, n = 38; (D) Normal small intestines (n = 75 individuals): rs1169288AA, n = 42; rs1169288AC/CC, n = 33.
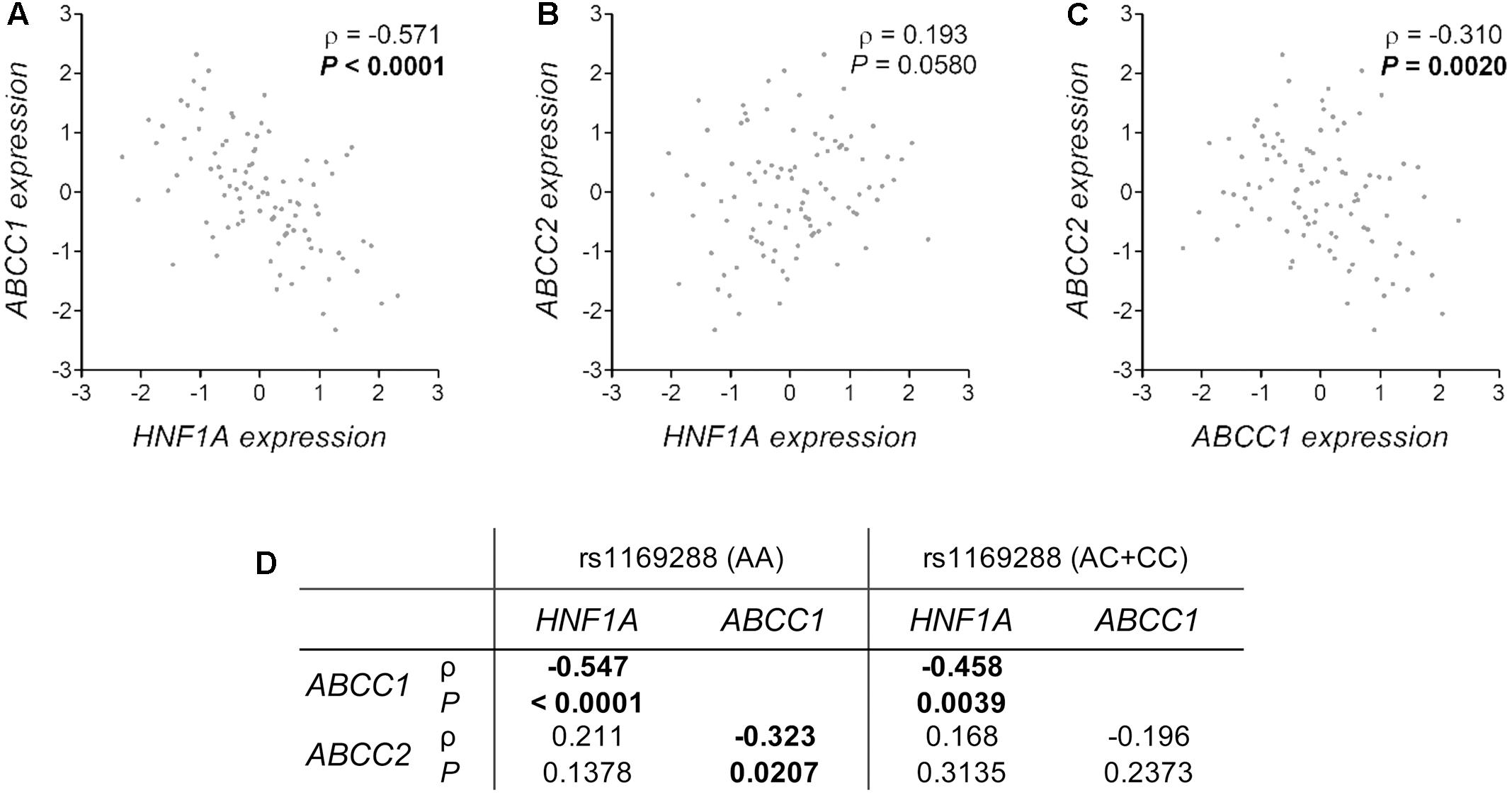
FIGURE 5. Correlation of the hepatic expression of HNF1A, ABCC1 and ABCC2 and the influence of the rs1169288 genotype. (A–C) Correlations are shown for 97 individuals of the GTEx cohort. (D) Spearman correlation ρ coefficients and P-values for genotyped individuals carrying rs1169288AA (homozygous for p.I27; n = 51) and rs1169288AC/CC (heterozygous and homozygous p.L27; n = 38) genotypes.
Based on the prominent association between HNF1A and ABCC1 observed in human liver samples, we then tested whether the p.I27L substitution affects the ability of transcription factor HNF1A to induce transcription of a reporter gene construct containing the ABCC1 promoter. In vitro luciferase assays revealed a significant impact of the p.I27L substitution when tested in the human HEK293 kidney cells and the liver HepG2 cell line with a significant increased in reporter gene activity associated with HNF1A p.L27 by 27% (P = 0.008) and 15% (P = 0.041), respectively, compared to cells expressing the HNF1A p.I27 protein (Figure 6).
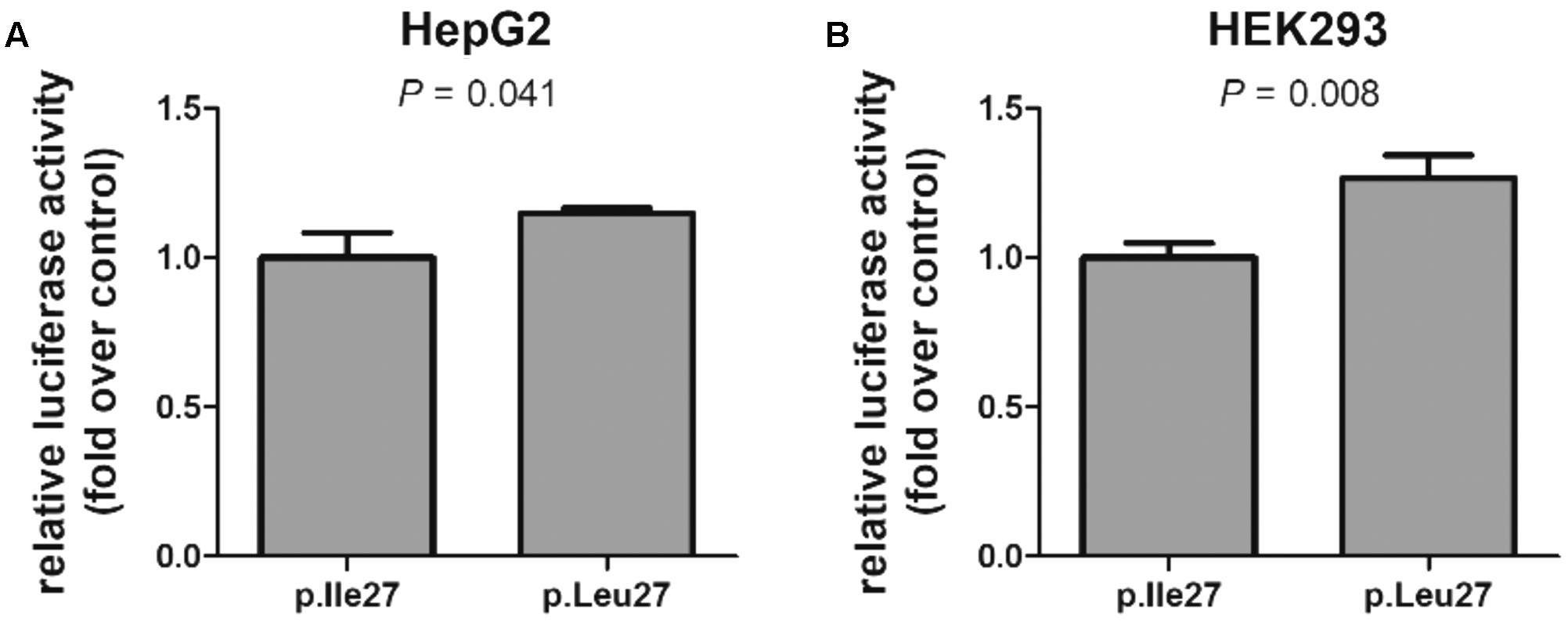
FIGURE 6. Functional impact of the HNF1A rs1169288 coding variant. Transcriptional induction of the ABCC1 promoter by HNF1A p.I27 (p.Ile27) and p.L27 (p.Leu27) in liver (A) and kidney (B) cells. Cells were co-transfected with a reporter vector containing the firefly luciferase gene downstream of the ABCC1 promoter and an HNF1A construct (p.Ile27 or p.Leu27). The bars represent the mean ± SD of three independent experiments performed in triplicate. Statistical analysis used Student’s two-tailed t-test.
Discussion
We report that the intronic htSNP rs2244608A>G located in the HNF1A gene may be predictive of an improved PFS in two independent cohorts of 417 mCRC white patients treated with irinotecan-based regimen. In support of a better response to chemotherapy, an enhanced systemic exposure to the active metabolite of irinotecan, SN-38, was observed in a subset of mCRC patients carrying the minor rs2244608G allele associated with prolonged PFS. The rs2244608A>G was not associated with severe toxicities in the Canadian and the Italian cohorts, suggesting that the increase in SN-38 exposure for carriers of rs2244608G allele may not induce severe side effects. In individuals of European descent, rs2244608A>G marker is almost in complete linkage (r2 = 0.98) with the non-synonymous coding variant rs1169288A>C (p.I27L) located in exon 1, which could explain the observed phenotype. This is reinforced by an altered hepatic expression of metabolic and transport genes involved in irinotecan pharmacology, in favor of superior blood levels of the anticancer drug coupled with enhanced expression of irinotecan target gene TOP1 in the intestine, in carriers of the rs1169288C allele strongly linked to the rs2244608G marker. In functional in vitro studies, the HNF1A rs1169288C p.L27 polymorphism could significantly increase the ABCC1 promoter activity in human HepG2 hepatocellular carcinoma cell line and HEK293 kidney cells. This is the first study to demonstrate the relationship between variability in the transcription factor HNF1A gene and clinical outcome in the context of irinotecan-based regimen.
HNF1A plays an important part in the pathogenesis of various diseases particularly diabetes and cardiovascular diseases. Genetic variations in the HNF1A gene, and especially the coding variant rs1169288A>C (p.I27L), have been previously linked to type 2 diabetes (T2D) (Holmkvist et al., 2006; Gaulton et al., 2015), gestational diabetes mellitus (Shaat et al., 2006), cholelithiasis (Richter et al., 2012), coronary artery diseases (CAD) (Wang et al., 2015; Zhou et al., 2017), serum lipid levels (Babaya et al., 2003; Zhou et al., 2017) and levels of the biomarker of inflammation C-reactive protein (CRP) (Reiner et al., 2008; Reiner et al., 2009), suggesting a functional impact of the codon 27 amino acid substitution. However, this does not preclude the potential impact of the intronic rs2244608 marker (or other SNPs in high LD), which can influence gene expression, stability or splicing of the mRNA. The p.I27L substitution is located in the N-terminal dimerization domain of HNF1A that mediates its homodimerization and heterodimerization with HNF1B and the dimerization cofactor DcoH (Narayana et al., 2001). This conservative substitution may be anticipated to have little impact on the structure of HNF1A; however, isoleucine 27 is highly conserved across species, supporting a functional significance (Narayana et al., 2001; Setoh et al., 2015).
Of key genes involved in irinotecan pharmacology, the hepatic expression of CYP3A5 and the transporters ABCC1 and ABCC2 was significantly influenced by the rs1169288A>C. The expression of these genes may be regulated by HNF1A, either directly or indirectly. In line, the expression of ABCC2 is regulated by HNF1A in liver cells (Odom et al., 2004; Qadri et al., 2009). In addition, results of our in vitro investigations allowed to further substantiate evidence for a functional impact of variant HNF1A p.I27L (rs1169288A>C) on the expression of ABCC1 in human liver and kidney cells, with an increased transcriptional induction by HNF1A p.L27 using the ABCC1 promoter. Consistent with the elevated SN-38 AUC, higher ABCC1 (MRP1) expression and lower ABCC2 (MRP2) and CYP3A5 expressions were observed for rs224608G carriers, strongly linked to the rs1169288C allele. MRP1 is on the basolateral membrane of hepatocytes (Emi et al., 2013) and mediates efflux of SN-38 into blood (Chen et al., 1999) whereas MRP2 is located on the apical membrane of hepatocytes (Emi et al., 2012) and is involved in the efflux of several endogenous compounds and drugs, including irinotecan and its metabolites, to the bile (Chu et al., 1997; Sugiyama et al., 1998). The superior exposure to SN-38 in carriers of rs2244608G may thus be explained through an enhanced redirection of SN-38 from hepatocytes to blood circulation and a lower excretion of irinotecan metabolites in the bile. In line with this hypothesis, Wang et al. (2016) demonstrated that hepatic inhibition of MRP2 expression with hesperidin in rats lead to an increased SN-38 exposure while biliary excretion of SN-38 was reduced. Furthermore, the rs1169288 polymorphism has been linked to altered irinotecan pharmacokinetics in a study of 85 patients with solid tumors (Rosner et al., 2008). The strong negative correlation between hepatic expression of ABCC1 and HNF1A and the nearly significant positive correlation between ABCC2 and HNF1A observed in the GTEx cohort sustain a functional interaction, yet an inverse regulatory influence of HNF1A on these two transporters. We could thus speculate that a decreased expression or function of HNF1A would lead to increase ABCC1 expression and potentially decreased ABCC2 expression. This might contribute to an enhancement of SN-38 systemic exposure and a reduced elimination of the drug, leading to a prolonged PFS, as represented in Figure 7. Consistent with this notion, Holmkvist et al. (2006) demonstrated a decreased transcriptional induction of SLC2A2 promoter (Solute Carrier Family 2 Member 2 or GLUT2) by HNF1A p.L27 in HeLa cells. Although there are inconsistencies in the literature, previous reports also support a link between genetic variations in the ABCC1 and ABCC2 genes, altered irinotecan pharmacokinetics and clinical outcomes in colon and lung cancer patients (Rosner et al., 2008; Innocenti et al., 2009; Akiyama et al., 2012; Li et al., 2016).
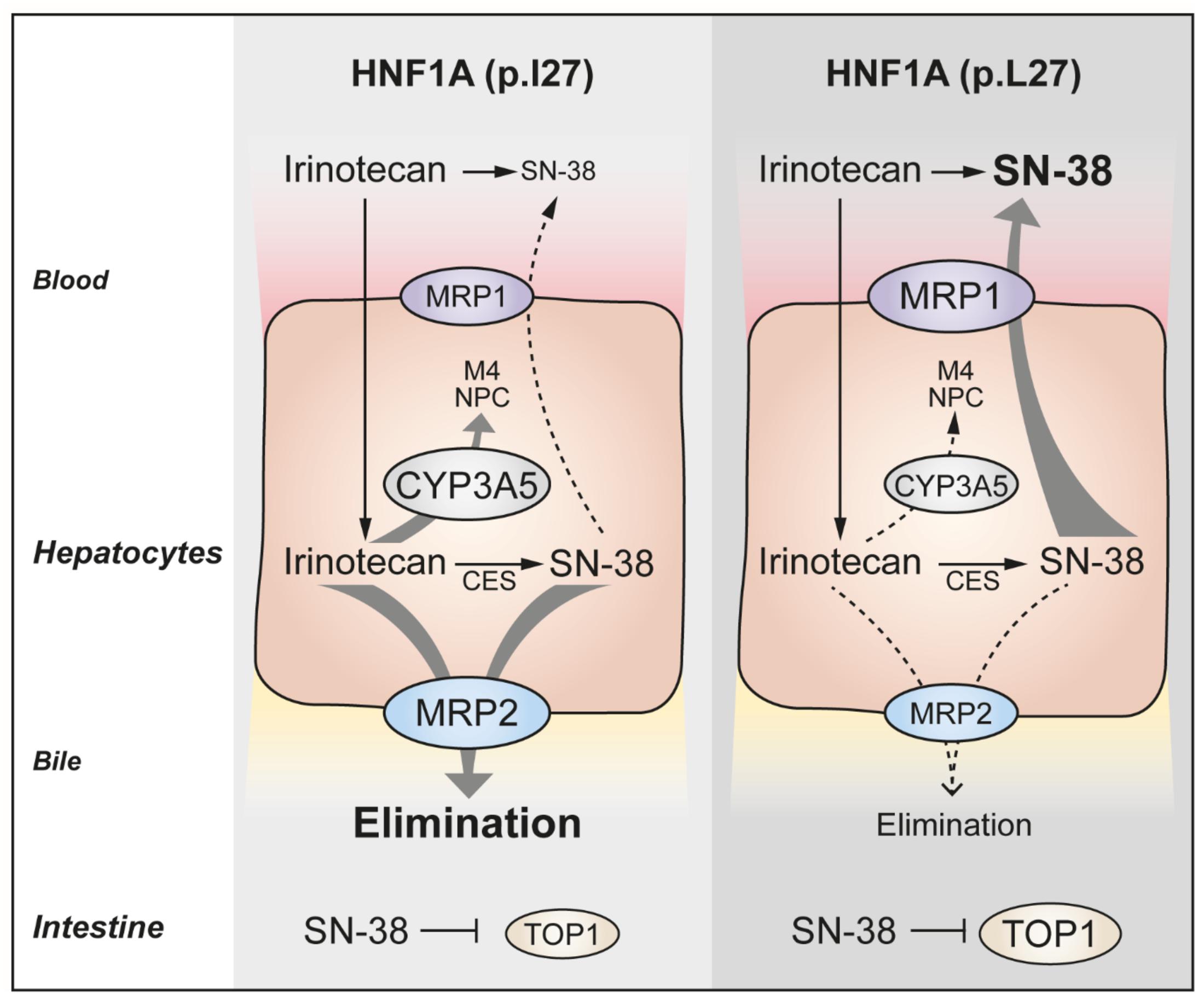
FIGURE 7. Proposed model for the underlying mechanism of an improved progression-free survival associated with the HNF1A rs1169288C allele.
The impact of variations in HNF1A expression and/or function is likely to be multifactorial and may affect multiple pathways relevant to irinotecan pharmacokinetics and pharmacodynamics (Smith et al., 2006). In the liver of healthy individuals, we further found a positive correlation between HNF1A and CYP3A5 expressions, in line with a previous observation by Noll et al. (2016), and an association with reduced expression of CYP3A5 for the rs1169288C allele. As CYP3A5 oxidizes irinotecan in inactive metabolites, reduction of its expression may increase the proportion of irinotecan available for conversion into SN-38 by carboxylesterases, resulting in higher SN-38 exposure. Lastly, carriers of rs1169288C also presented higher expression of the irinotecan drug target gene TOP1 in the small intestine, which could explain, at least in part, a better response to TOP1 inhibitor treatment and improved PFS (Figure 7). Additional functional studies will be required to test these hypotheses.
The strengths of our study include the examination of two independent cohorts of mCRC comprising 417 white patients and the analysis of pharmacokinetics and transcriptomic data to further investigate the potential mechanisms underlying the association. Given its exploratory nature, we did not apply correction for multiple testing. Besides, the study design involved an independent set of patients used to replicate findings observed in the training set, which reduces the likelihood of false-positive results while maximizing the detection of true-positive associations. Whereas this may result in potential false-positive associations, the high stringency of multiple testing may have led to false-negative associations due to the number of patients included. The LD between rs2244608A>G and rs1169288A>C was almost complete in individuals of European descent but is lower in African and Asian populations implying that our findings may be most particularly significant in individuals of European descent. While recognizing the limitations of our study, further investigations are warranted to replicate our findings and substantiate the functional impact of the HNF1A p.I27L coding variation on pathways relevant to drug metabolism, transport and action.
Conclusion
We report the identification of rs2244608A>G, strongly linked to the functional coding variant HNF1A rs1169288A>C (p.I27L), as a predictive marker of improved PFS in mCRC patients treated with irinotecan-based chemotherapy. Prolonged PFS may likely be explained by a greater exposure to SN-38, possibly due to an altered transcriptional regulation by HNF1A of genes involved in irinotecan pharmacology including CYP3A5, ABCC1 and ABCC2 transporters and TOP1.
Author Contributions
Conceptualization: CG and GT; Methodology: All authors; Investigation: AL, EDM, EC, EL, DJ, FC, AB, MD, LV, and CG; Formal Analysis: AL, EDM, EC, EL, LV, GT, and CG; Writing – Review and Editing: All authors; Supervision: CG and GT; Funding acquisition: CG and GT.
Funding
This work was supported by the Canadian Institutes of Health Research (CIHR) (CG; CIHR MOP-42392), the Canada Research Chair Program (CG) and the ‘Associazione Italiana per la Ricerca sul Cancro’ (AIRC; Special Program Molecular Clinical Oncology, 5 × 1000, [No. 12214]) (GT). AL was a recipient of a studentship award from ‘Fonds d’Enseignement et de Recherche (FER)’ from Faculty of Pharmacy at Laval University. EL is a recipient of a CIHR clinical-scientist phase II award and Prostate Cancer Canada Rising Star Award (RS2013-55). CG holds a Canada Research Chair in Pharmacogenomics (Tier I).
Conflict of Interest Statement
The authors declare that the research was conducted in the absence of any commercial or financial relationships that could be construed as a potential conflict of interest.
Acknowledgments
The authors would like to thank all study participants as well as Dr. Michèle Rouleau for critical discussion, Frédéric Fournier from the CHU de Québec for retrieving data from The Genotype-Tissue Expression (GTEx) Project and David Simonyan for support with statistics. The GTEx Project was supported by the Common Fund of the Office of the Director of the National Institutes of Health, and by NCI, NHGRI, NHLBI, NIDA, NIMH, and NINDS. The data used for the analyses described in this manuscript were obtained from: the GTEx Portal and dbGaP accession number phs000424.v6.p1 on 03/16/2017.
Supplementary Material
The Supplementary Material for this article can be found online at: https://www.frontiersin.org/articles/10.3389/fphar.2017.00712/full#supplementary-material
Abbreviations
AUC, area under the curve; CEU population, Utah residents with Northern and Western European ancestry population; FOLFIRI, folinic acid (leucovorin) + 5-fluorouracil (5-FU) + irinotecan; HR, hazard ratio; htSNP, haplotype-tagging single nucleotide polymorphism; LD, linkage disequilibrium; mCRC, metastatic colorectal cancer; OR, odds ratio; OS, overall survival; PFS, progression-free survival.
Footnotes
References
Akiyama, Y., Fujita, K., Ishida, H., Sunakawa, Y., Yamashita, K., Kawara, K., et al. (2012). Association of ABCC2 genotype with efficacy of first-line FOLFIRI in Japanese patients with advanced colorectal cancer. Drug Metab. Pharmacokinet. 27, 325–335. doi: 10.2133/dmpk.DMPK-11-RG-128
Aleksunes, L. M., Yeager, R. L., and Klaassen, C. D. (2009). Application of multivariate statistical procedures to identify transcription factors that correlate with MRP2, 3, and 4 mRNA in adult human livers. Xenobiotica 39, 514–522. doi: 10.1080/00498250902952514
Babaya, N., Ikegami, H., Fujisawa, T., Nojima, K., Itoi-Babaya, M., Inoue, K., et al. (2003). Association of I27L polymorphism of hepatocyte nuclear factor-1 alpha gene with high-density lipoprotein cholesterol level. J. Clin. Endocrinol. Metab. 88, 2548–2551.
Barbarino, J. M., Haidar, C. E., Klein, T. E., and Altman, R. B. (2014). PharmGKB summary: very important pharmacogene information for UGT1A1. Pharmacogenet. Genomics 24, 177–183. doi: 10.1097/fpc.0000000000000024
Barrett, J. C., Fry, B., Maller, J., and Daly, M. J. (2005). Haploview: analysis and visualization of LD and haplotype maps. Bioinformatics 21, 263–265. doi: 10.1093/bioinformatics/bth457
Beutler, E., Gelbart, T., and Demina, A. (1998). Racial variability in the UDP-glucuronosyltransferase 1 (UGT1A1) promoter: a balanced polymorphism for regulation of bilirubin metabolism? Proc. Natl. Acad. Sci. U.S.A. 95, 8170–8174.
Cecchin, E., De Mattia, E., and Toffoli, G. (2016). Nuclear receptors and drug metabolism for the personalization of cancer therapy. Expert Opin. Drug Metab. Toxicol. 12, 291–306. doi: 10.1517/17425255.2016.1141196
Cecchin, E., Innocenti, F., D’Andrea, M., Corona, G., De Mattia, E., Biason, P., et al. (2009). Predictive role of the UGT1A1, UGT1A7, and UGT1A9 genetic variants and their haplotypes on the outcome of metastatic colorectal cancer patients treated with fluorouracil, leucovorin, and irinotecan. J. Clin. Oncol. 27, 2457–2465. doi: 10.1200/jco.2008.19.0314
Chen, S., Laverdiere, I., Tourancheau, A., Jonker, D., Couture, F., Cecchin, E., et al. (2015a). A novel UGT1 marker associated with better tolerance against irinotecan-induced severe neutropenia in metastatic colorectal cancer patients. Pharmacogenomics J. 15, 513–520. doi: 10.1038/tpj.2015.12
Chen, S., Villeneuve, L., Jonker, D., Couture, F., Laverdiere, I., Cecchin, E., et al. (2015b). ABCC5 and ABCG1 polymorphisms predict irinotecan-induced severe toxicity in metastatic colorectal cancer patients. Pharmacogenet. Genomics 25, 573–583. doi: 10.1097/fpc.0000000000000168
Chen, Z. S., Furukawa, T., Sumizawa, T., Ono, K., Ueda, K., Seto, K., et al. (1999). ATP-Dependent efflux of CPT-11 and SN-38 by the multidrug resistance protein (MRP) and its inhibition by PAK-104P. Mol. Pharmacol. 55, 921–928.
Chu, X. Y., Kato, Y., Niinuma, K., Sudo, K. I., Hakusui, H., and Sugiyama, Y. (1997). Multispecific organic anion transporter is responsible for the biliary excretion of the camptothecin derivative irinotecan and its metabolites in rats. J. Pharmacol. Exp. Ther. 281, 304–314.
De Mattia, E., Cecchin, E., Roncato, R., and Toffoli, G. (2016). Pregnane X receptor, constitutive androstane receptor and hepatocyte nuclear factors as emerging players in cancer precision medicine. Pharmacogenomics 17, 1547–1571. doi: 10.2217/pgs-2016-0095
Emi, Y., Harada, Y., and Sakaguchi, M. (2013). Involvement of a di-leucine motif in targeting of ABCC1 to the basolateral plasma membrane of polarized epithelial cells. Biochem. Biophys. Res. Commun. 441, 89–95. doi: 10.1016/j.bbrc.2013.10.013
Emi, Y., Yasuda, Y., and Sakaguchi, M. (2012). A cis-acting five-amino-acid motif controls targeting of ABCC2 to the apical plasma membrane domain. J. Cell Sci. 125(Pt 13), 3133–3143. doi: 10.1242/jcs.099549
Fujita, K., Kubota, Y., Ishida, H., and Sasaki, Y. (2015). Irinotecan, a key chemotherapeutic drug for metastatic colorectal cancer. World J. Gastroenterol. 21, 12234–12248. doi: 10.3748/wjg.v21.i43.12234
Gaulton, K. J., Ferreira, T., Lee, Y., Raimondo, A., Magi, R., Reschen, M. E., et al. (2015). Genetic fine mapping and genomic annotation defines causal mechanisms at type 2 diabetes susceptibility loci. Nat. Genet. 47, 1415–1425. doi: 10.1038/ng.3437
Holmkvist, J., Cervin, C., Lyssenko, V., Winckler, W., Anevski, D., Cilio, C., et al. (2006). Common variants in HNF-1 alpha and risk of type 2 diabetes. Diabetologia 49, 2882–2891. doi: 10.1007/s00125-006-0450-x
Hu, D. G., Meech, R., McKinnon, R. A., and Mackenzie, P. I. (2014). Transcriptional regulation of human UDP-glucuronosyltransferase genes. Drug Metab. Rev. 46, 421–458. doi: 10.3109/03602532.2014.973037
Innocenti, F., Kroetz, D. L., Schuetz, E., Dolan, M. E., Ramirez, J., Relling, M., et al. (2009). Comprehensive pharmacogenetic analysis of irinotecan neutropenia and pharmacokinetics. J. Clin. Oncol. 27, 2604–2614. doi: 10.1200/jco.2008.20.6300
Levesque, E., Belanger, A. S., Harvey, M., Couture, F., Jonker, D., Innocenti, F., et al. (2013). Refining the UGT1A haplotype associated with irinotecan-induced hematological toxicity in metastatic colorectal cancer patients treated with 5-fluorouracil/irinotecan-based regimens. J. Pharmacol. Exp. Ther. 345, 95–101. doi: 10.1124/jpet.112.202242
Li, M., Seiser, E. L., Baldwin, R. M., Ramirez, J., Ratain, M. J., Innocenti, F., et al. (2016). ABC transporter polymorphisms are associated with irinotecan pharmacokinetics and neutropenia. Pharmacogenomics J. doi: 10.1038/tpj.2016.75 [Epub ahead of print].
Liu, R., Liu, H., Gu, H., Teng, X., Nie, Y., Zhou, Z., et al. (2014). A Polymorphism in hepatocyte nuclear factor 1 alpha, rs7310409, is associated with left main coronary artery disease. Biochem. Res. Int. 2014:924105. doi: 10.1155/2014/924105
Narayana, N., Hua, Q., and Weiss, M. A. (2001). The dimerization domain of HNF-1alpha: structure and plasticity of an intertwined four-helix bundle with application to diabetes mellitus. J. Mol. Biol. 310, 635–658. doi: 10.1006/jmbi.2001.4780
Noll, E. M., Eisen, C., Stenzinger, A., Espinet, E., Muckenhuber, A., Klein, C., et al. (2016). CYP3A5 mediates basal and acquired therapy resistance in different subtypes of pancreatic ductal adenocarcinoma. Nat. Med. 22, 278–287. doi: 10.1038/nm.4038
Odom, D. T., Dowell, R. D., Jacobsen, E. S., Gordon, W., Danford, T. W., MacIsaac, K. D., et al. (2007). Tissue-specific transcriptional regulation has diverged significantly between human and mouse. Nat. Genet. 39, 730–732. doi: 10.1038/ng2047
Odom, D. T., Zizlsperger, N., Gordon, D. B., Bell, G. W., Rinaldi, N. J., Murray, H. L., et al. (2004). Control of pancreas and liver gene expression by HNF transcription factors. Science 303, 1378–1381. doi: 10.1126/science.1089769
Purcell, S., Neale, B., Todd-Brown, K., Thomas, L., Ferreira, M. A., Bender, D., et al. (2007). PLINK: a tool set for whole-genome association and population-based linkage analyses. Am. J. Hum. Genet. 81, 559–575. doi: 10.1086/519795
Qadri, I., Hu, L. J., Iwahashi, M., Al-Zuabi, S., Quattrochi, L. C., and Simon, F. R. (2009). Interaction of hepatocyte nuclear factors in transcriptional regulation of tissue specific hormonal expression of human multidrug resistance-associated protein 2 (abcc2). Toxicol. Appl. Pharmacol. 234, 281–292. doi: 10.1016/j.taap.2008.10.005
Reiner, A. P., Barber, M. J., Guan, Y., Ridker, P. M., Lange, L. A., Chasman, D. I., et al. (2008). Polymorphisms of the HNF1A gene encoding hepatocyte nuclear factor-1 alpha are associated with C-reactive protein. Am. J. Hum. Genet. 82, 1193–1201. doi: 10.1016/j.ajhg.2008.03.017
Reiner, A. P., Gross, M. D., Carlson, C. S., Bielinski, S. J., Lange, L. A., Fornage, M., et al. (2009). Common coding variants of the HNF1A gene are associated with multiple cardiovascular risk phenotypes in community-based samples of younger and older European-American adults: the coronary artery risk development in young adults study and the cardiovascular health study. Circ. Cardiovasc. Genet. 2, 244–254. doi: 10.1161/circgenetics.108.839506
Richter, D., Harsch, S., Strohmeyer, A., Hirobe-Jahn, S., Schimmel, S., Renner, O., et al. (2012). MALDI-TOF mass spectrometry screening of cholelithiasis risk markers in the gene of HNF1alpha. J. Proteomics 75, 3386–3399. doi: 10.1016/j.jprot.2012.04.036
Rosner, G. L., Panetta, J. C., Innocenti, F., and Ratain, M. J. (2008). Pharmacogenetic pathway analysis of irinotecan. Clin. Pharmacol. Ther. 84, 393–402. doi: 10.1038/clpt.2008.63
Senkel, S., Lucas, B., Klein-Hitpass, L., and Ryffel, G. U. (2005). Identification of target genes of the transcription factor HNF1beta and HNF1alpha in a human embryonic kidney cell line. Biochim. Biophys. Acta 1731, 179–190. doi: 10.1016/j.bbaexp.2005.10.003
Setoh, K., Terao, C., Muro, S., Kawaguchi, T., Tabara, Y., Takahashi, M., et al. (2015). Three missense variants of metabolic syndrome-related genes are associated with alpha-1 antitrypsin levels. Nat. Commun. 6:7754. doi: 10.1038/ncomms8754
Shaat, N., Karlsson, E., Lernmark, A., Ivarsson, S., Lynch, K., Parikh, H., et al. (2006). Common variants in MODY genes increase the risk of gestational diabetes mellitus. Diabetologia 49, 1545–1551. doi: 10.1007/s00125-006-0258-8
Smith, N. F., Figg, W. D., and Sparreboom, A. (2006). Pharmacogenetics of irinotecan metabolism and transport: an update. Toxicol. In Vitro 20, 163–175. doi: 10.1016/j.tiv.2005.06.045
Sugiyama, Y., Kato, Y., and Chu, X. (1998). Multiplicity of biliary excretion mechanisms for the camptothecin derivative irinotecan (CPT-11), its metabolite SN-38, and its glucuronide: role of canalicular multispecific organic anion transporter and P-glycoprotein. Cancer Chemother. Pharmacol. 42(Suppl.), S44–S49. doi: 10.1007/s002800051078
Toffoli, G., Cecchin, E., Corona, G., Russo, A., Buonadonna, A., D’Andrea, M., et al. (2006). The role of UGT1A1∗28 polymorphism in the pharmacodynamics and pharmacokinetics of irinotecan in patients with metastatic colorectal cancer. J. Clin. Oncol. 24, 3061–3068. doi: 10.1200/jco.2005.05.5400
Voight, B. F., Scott, L. J., Steinthorsdottir, V., Morris, A. P., Dina, C., Welch, R. P., et al. (2010). Twelve type 2 diabetes susceptibility loci identified through large-scale association analysis. Nat. Genet. 42, 579–589. doi: 10.1038/ng.609
Wang, X., Rao, Z., Qin, H., Zhang, G., Ma, Y., Jin, Y., et al. (2016). Effect of hesperidin on the pharmacokinetics of CPT-11 and its active metabolite SN-38 by regulating hepatic Mrp2 in rats. Biopharm. Drug Dispos. 37, 421–432. doi: 10.1002/bdd.2024
Wang, X. B., Han, Y. D., Cui, N. H., Gao, J. J., Yang, J., Huang, Z. L., et al. (2015). Associations of lipid levels susceptibility loci with coronary artery disease in Chinese population. Lipids Health Dis. 14:80. doi: 10.1186/s12944-015-0079-1
Yamagata, K., Oda, N., Kaisaki, P. J., Menzel, S., Furuta, H., Vaxillaire, M., et al. (1996). Mutations in the hepatocyte nuclear factor-1alpha gene in maturity-onset diabetes of the young (MODY3). Nature 384, 455–458. doi: 10.1038/384455a0
Keywords: metastatic colorectal cancer, hepatocyte nuclear factor 1-alpha, irinotecan, polymorphism, progression-free survival
Citation: Labriet A, De Mattia E, Cecchin E, Lévesque É, Jonker D, Couture F, Buonadonna A, D’Andrea M, Villeneuve L, Toffoli G and Guillemette C (2017) Improved Progression-Free Survival in Irinotecan-Treated Metastatic Colorectal Cancer Patients Carrying the HNF1A Coding Variant p.I27L. Front. Pharmacol. 8:712. doi: 10.3389/fphar.2017.00712
Received: 28 July 2017; Accepted: 22 September 2017;
Published: 10 October 2017.
Edited by:
Rick Kittles, Irell & Manella Graduate School of Biological Sciences, United StatesReviewed by:
Cheryl D. Cropp, Samford University, United StatesJason Hansen Karnes, University of Arizona, United States
Ken Batai, University of Arizona, United States
Copyright © 2017 Labriet, De Mattia, Cecchin, Lévesque, Jonker, Couture, Buonadonna, D’Andrea, Villeneuve, Toffoli and Guillemette. This is an open-access article distributed under the terms of the Creative Commons Attribution License (CC BY). The use, distribution or reproduction in other forums is permitted, provided the original author(s) or licensor are credited and that the original publication in this journal is cited, in accordance with accepted academic practice. No use, distribution or reproduction is permitted which does not comply with these terms.
*Correspondence: Chantal Guillemette, chantal.guillemette@crchudequebec.ulaval.ca
†These authors have contributed equally to this work.