- 1Department of Medical and Surgical Sciences and Translational Medicine, Sapienza—University of Rome, Rome, Italy
- 2Molecular Genetics Section, Department of Cellular Biotechnology and Hematology, Sapienza—University of Rome, Rome, Italy
- 3FIMM Institute for Molecular Medicine Finland, Helsinki, Finland
HIGHLIGHTS
• Preliminary results of this work were presented at the 2016 Academic Surgical Congress, Jacksonville (FL), February 2–4 2016 (Original title: Selective Smo-Inhibition Interferes With Cellular Energetic Metabolism In Colorectal Cancer)
• This study was funded by “Sapienza—University of Rome” (Funds for young researchers) and “AIRC” (Italian Association for Cancer Research)
• Hedgehog inhibitor was kindly provided by Genentech, Inc.®.
Colon Cancer (CC) is the fourth most frequently diagnosed tumor and the second leading cause of death in the USA. Abnormalities of Hedgehog pathway have been demonstrated in several types of human cancers, however the role of Hedgehog (Hh) in CC remain controversial. In this study, we analyzed the association between increased mRNA expression of GLI1 and GLI2, two Hh target genes, and CC survival and recurrence by gene expression microarray from a cohort of 382 CC patients. We found that patients with increased expression of GLI1 showed a statistically significant reduction in survival. In order to demonstrate a causal role of Hh pathway activation in the pathogenesis of CC, we treated HCT 116, SW480 and SW620 CC cells lines with GDC-0449, a pharmacological inhibitor of Smoothened (SMO). Treatment with GDC-0449 markedly reduced expression of Hh target genes GLI1, PTCH1, HIP1, MUC5AC, thus indicating that this pathway is constitutively active in CC cell lines. Moreover, GDC-0449 partially reduced cell proliferation, which was associated with upregulation of p21 and downregulation of CycD1. Finally, treatment with the same drug reduced migration and three-dimensional invasion, which were associated with downregulation of Snail1, the EMT master gene, and with induction of the epithelial markers Cytokeratin-18 and E-cadherin. These results were confirmed by SMO genetic silencing. Notably, treatment with 5E1, a Sonic Hedgehog-specific mAb, markedly reduced the expression of Hedgehog target genes, as well as inhibited cell proliferation and mediated reversion toward an epithelial phenotype. This suggests the existence of a Hedgehog autocrine signaling loop affecting cell plasticity and fostering cell proliferation and migration/invasion in CC cell lines. These discoveries encourage future investigations to better characterize the role of Hedgehog in cellular plasticity and invasion during the different steps of CC pathogenesis.
Introduction
In colon carcinoma (CC) as for other cancers, the understanding of cellular and molecular mechanisms involved in tumor progression is essential to provide the rationale for novel therapies. According to the 2016 NCCN Panel, CC is the fourth most frequently diagnosed tumor and the second leading cause of death in the USA. Incidence in Italy raised from 27.06% in 1970 to 52.65% in 2010 (Ciatto, 2007). Reactivation or alteration of molecular pathways that control cellular differentiation and proliferation play a role in the development and progression of both familiar and sporadic CC (Al-Sohaily et al., 2012; Bertrand et al., 2012). Numerous studies demonstrated that chemotherapy may be helpful both for primary and for metastatic tumors (Nappi et al., 2016). However, the persistence of cases of local recurrence in patients treated with current protocols impels to study the impact of novel pathways active in CC pathogenesis.
Hh pathway plays an important role in tissue development and organogenesis (Jiang and Hui, 2008; Rimkus et al., 2016). However, in mature organisms, Hh pathway remains selectively active, controlling cell proliferation and differentiation.
Hh ligands are expressed by various stromal and parenchymal tissues and act generally in a paracrine way by binding to the plasma membrane receptor Patched (PTCH1). Upon binding of Hh ligands to PTCH1, the G-protein–coupled receptor, Smoothened (SMO) is activated and promotes nuclear translocation of GLI family of zinc finger transcription factors. GLI activation induces the transcription of Hh target gene products, including ubiquitous genes such as GLI1, PTCH1, and Hh-interacting protein (HIP1) (Benvenuto et al., 2016; Rimkus et al., 2016). This canonical Hh signaling cascade plays a role in the normal gastrointestinal development, regulating the differentiation of normal intestinal villi and of the adjacent mesenchymal stromal cells. In particular, in the normal adult gastrointestinal tract, induction of the Hh pathway protects the differentiated epithelial cells of the villous surface at the top of the crypts of Lieberkuhn (the structural unit of the normal colon), counteracting the canonical Wnt signaling in the basal cells of the crypt. In the absence of Hh ligand, PTCH1 suppresses the activation of SMO.
Since Hh pathway is involved in the control of proliferation/differentiation status in many tissues, it is not surprising that Hh expression and activity are altered in tumors (Scales and de Sauvage, 2009).
Aberrant Hh signaling, which can be achieved by mutational inactivation of PTCH1, aberrant expression of its ligand, constitutive activation of SMO or gene amplification of GLI-associated transcription factors, has been implicated in the initiation and/or maintenance of different cancer types, including basal cell carcinoma (BCC), lung, and brain tumors and rhabdomyosarcoma (Gupta et al., 2010).
The involvement of Hh pathway in the pathogenesis of CC and the potential relevance for therapy has been already addressed by different studies. It has been demonstrated that Hh-GLI activity in epithelial tumor cells of CC is essential for tumor growth, recurrence and metastasis, and regulates the behavior of human CC stem cells in vivo (Varnat et al., 2009). However, a randomized phase II trial using a pharmacological inhibitor of Hh failed to show an incremental benefit respect to standard treatments in a population of previously untreated patients with metastatic CC (Berlin et al., 2013). In spite of this, recent research based on in vitro and in vivo experimental systems, led to controversial results (Gerling et al., 2016; Kangwan et al., 2016; Lee T. Y. et al., 2016; Wang et al., 2016).
The aim of the present study was to analyze whether pharmacological inhibition of Hh pathway, and specifically of SMO, impacts epithelial plasticity and invasiveness in different CC cell lines, and to explore the molecular mechanisms involved. Starting from our evidence correlating Hh pathway effectors (i.e., GLI1 and GLI2) with CC patients survival, we first treated CC cell lines with a pharmacological SMO inhibitor, GDC-0449, a small molecule already approved by the US Food and Drug Administration (FDA) for the treatment of advanced basal cell carcinoma (BCC) (Dijkgraaf et al., 2011; Takebe et al., 2015). In addition, we investigated the effects of SMO-specific genetic silencing as well as 5E1, a Shh-specific Monoclonal Antibody (mAb). Our results show that Hh pathway impacts epithelial/mesenchymal features and invasion capability of CC cell lines.
Materials and Methods
Cells
Primary (HCT 116, SW480) and metastatic (SW620) human CC cell lines were grown in DMEM supplemented with 10% FBS (GIBCO® Life Technology, Monza, Italy) and antibiotics. These cellular lines were reported to express detectable levels of SMO and GLI1 (Sun et al., 2013; Sénicourt et al., 2016; Wang et al., 2017). MeT5A cells, a human mesothelium non-tumorigenic cell line were grown in M199 supplemented with 10% FBS (GIBCO® Life Technology, Monza, Italy) and antibiotics.
RNA Extraction, Reverse Transcription (RT), and Real-Time Polymerase Chain Reaction (RT-qPCR)
RNA, extracted from cell cultures with ReliaPrep™ RNA Tissue Miniprep System (Promega, Madison, WI, USA), was reverse transcribed with iScriptTM c-DNA Synthesis Kit (Bio-Rad Laboratories, Inc., Hercules, CA, USA) according to the manufacturer's instructions. cDNAs were amplified by qPCR reaction using GoTaq® qPCR Master Mix (Promega, Madison, WI, USA). The specific primer pairs are listed in Table 1. Relative amounts, obtained with 2−ΔCt method, were normalized with respect to the housekeeping gene L32. Statistical significance was determined with a t-test with GraphPad Prism version 5.0 (La Jolla, CA, USA). Differences were considered significant at P < 0.05 (*p < 0.05; **p < 0.01; ***p < 0.001).
Antibodies and Chemicals
Monoclonal antibody against E-cadherin was from BD (Becton-Dickinson Laboratories, Mountain View, CA). Monoclonal antibodies against Snail1 and against GLI1 were from Cell signaling (Danvers, MA). Monoclonal antibody against pan-cytokeratin was from Sigma (St Louis, MO). Polyclonal antibodies against SMO (N-19) and tubulin were from Santa Cruz biotechnology (Dallas, USA). GDC-0449, a Hedgehog/Smoothened pharmacological inhibitor, was a kind gift from Genentech (South San Francisco, CA). 5E1, Shh specific mAb was purchased by Developmental Studies Hybridoma Bank (Iowa City, Iowa, US) (Ericson et al., 1996).
siRNA-Mediated SMO Knockdown
200 × 103 cells were seeded on 12-well plates 24 h prior transfection. Cells were transfected with either 40 pmol ON-TARGETplus SMARTpool against human SMO (Dharmacon Ref#SO-2600349G) or the same amount of ON-TARGETplus NontargetingPool (Dharmacon Ref#D-001206-14-05) and 2 μl Lipofectamine® RNAiMAX Reagent from Thermo Fisher Scientific (Waltham, MA USA) in 200 μl Optimem from Gibco (Waltham, MA USA). 1 ml of supplemented medium per well was also added. Forty-eight hours after transfection, knockdown efficiency was determined by RT-PCR and western blot.
Western Blotting
Monolayers of HCT 116 cells were lysed in modified RIPA buffer containing: 50 mM Tris-HCl, pH 7.4; 1% NP-40; 0.1% SDS; 0.25% Nadeoxycholate; 150 mM NaCl; 1 mM EDTA; 1 mM EGTA; 1 mM PMSF; 1 μg/ml each of aprotinin, leupeptin, and pepstatin; and 25 mM NaF (all from Sigma). Equal amounts of proteins were resolved by SDS-PAGE. Proteins were transferred to nitrocellulose membranes, probed with antibodies and detected as in Battistelli et al. (2017).
Scratch Assay
CC cell lines were pretreated with DMSO or 1 μM GDC-0449 for 24 h in culture medium until reaching 100% confluency. A scratch wound was created on the cell surface using a micropipette tip in low serum (0.5% FBS) culture medium to inhibit cell proliferation. Micrographs were taken at time 0 and 24 h after the scratch. Cell devoid areas at time 0 and 24 h after the scratch were quantified through ImageJ software (NIH, Bethesda, MD, USA). Three independent experiments for each cell line were performed
3-Dimensional Invasion Assay and Immunofluorescence
3-dimensional invasion assays were performed as in Strippoli et al. (2015). HCT 116 cells (1.5 × 105) were treated with 1 μM GDC-0449 or vehicle for 12 h and then seeded in triplicate in Ibidi 15-well chambers and allowed to attach for 3 h. 40% matrigel (40 μl) in serum-free medium was laid over the cells. After 1 h, 50 μl full medium containing 20% serum (and GDC-0449 or vehicle) was added and cells incubated for 72 h. Cells were fixed with 4% paraformaldehyde (PFA), permeabilized with 0.25% Triton in PBS, and stained for 12 h with rhodamine-phalloidin (to stain F-actin) and Hoechst (to stain nuclei) in PBS. Confocal images were captured with a Leica SP5 fitted with a 40 × oil objective. Maximum projection images consist of 30 individual images with a z-distance of 120 μm. Imaris image analysis software (Bitplane Scientific Software) was used to create a 3D view of the same cells and to quantify invading cells. Three independent experiments were performed.
Statistical Analysis
Survival analysis significance was based on Logrank Test. Statistical significance of experiments performed with cell lines was determined with a t-test using GraphPad Prism version 5.0 (La Jolla, CA, USA). Differences were considered significant at P < 0.05.
Results
GLI Altered Expression Correlates with Reduced Survival in CC Patients
To determine the clinical relevance of Hh-GLI pathway in CC, we examined the association between increased GLI mRNA expression and CC survival by exploring gene expression data microarray from a cohort of 382 CC patients in the cancer genome atlas (TCGA) database (Cerami et al., 2012; Gao et al., 2013).
Notably, patients with increased GLI1 expression showed a statistically significant reduction in overall survival (OS) (Figure 1) and a trend toward reduced disease-free survival (DFS) (Figure S1A). Patients with increased GLI2 expression showed tendency to reduced survival (Figure S1B). These findings support a role for GLI1 and GLI2 in CC pathogenesis, and prompted us to investigate if pharmacological inhibition of Hh pathway could affect the proliferation or the invasive capacity of CC cell lines.
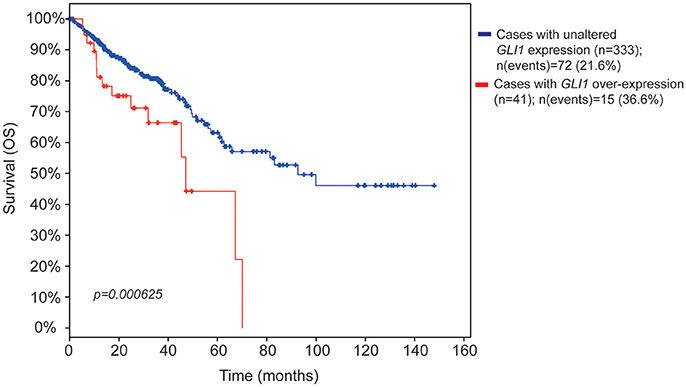
Figure 1. Kaplan-Meier survival estimate plots for increased GLI mRNA expression in CC patients. The survival estimate was analyzed in http://www.cbioportal.org (Cerami et al., 2012; Gao et al., 2013) and is based on Colorectal adenocarcinoma TCGA provisional dataset, which is generated by http://cancergenome.nih.gov/. The cases were set to include tumor samples with mRNA data (RNA Seq V2, n = 382 patients). The red line shows overall survival estimate for patients with increased GLI1 expression (11% of the patients) as compared to patients with no alteration (blue line, the rest of the patients). Eight patients were missing in the survival analysis. The threshold for altered expression was set to z-score value = 1.2 The z-score value is used to define the cut-off for patient dichotomization in the TCGA datasets (see http://www.cbioportal.org/). The median months survival for the increased GLI1 patient group and the reference group were 47.04 and 92.67, respectively. Survival analysis significance was based on Logrank Test. P < 0.05 was considered significant.
GDC-0449 Limits Cellular Proliferation of Several CC Cell Lines
We analyzed whether treatment with GDC-0449 affects the expression of Hh pathway specific genes in CC lines. We exposed HCT 116, SW480, primary CC lines, and SW620, a metastatic CC cell line, to GDC-0449 for 24 h at a concentration (1 μM) that is compatible with cellular viability. As shown in Figures 2A–D, treatment with GDC-0449 significantly reduced the expression of GLI1, PTCH1, HIP1, and MUC5AC, four of the known Hh target genes, thus demonstrating that Hh pathway is constitutively active in all three cell lines. GLI1 protein expression upon treatment with GDC-0449 was shown in Figures S2A–C. Raw data of the original western blots shown in this study are displayed in Figures S5–S7.
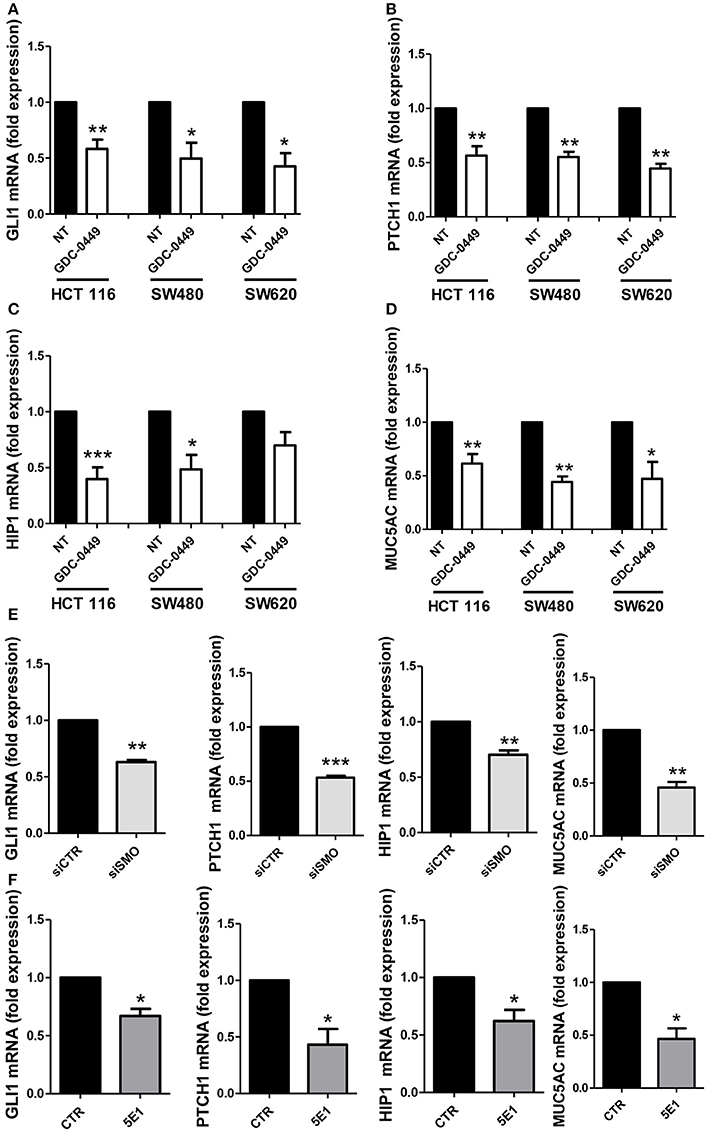
Figure 2. Effect of Hh inhibition on Hh pathway-induced genes. HCT 116, SW480 and SW620 were treated with DMSO vehicle (NT) or with 1 μM GDC-0449 for 24 h. Expression of GLI1 (A), PTCH1 (B), HIP1 (C), MUC5AC (D) was evaluated on total RNA by qRT-PCR. Bars represent means ± SEM of 7 experiment for HCT 116 cells, three experiments for SW480 and SW620 cells. Expression of GLI1, PTCH1, HIP1, MUC5AC in HCT 116 cells transfected with either control or specific SMO-targeting siRNAs (E). Expression of GLI1, PTCH1, HIP1, MUC5AC in HCT 116 cells treated with either isotype-control mAb or 5E1 mAB (F). Bars represent means ± SEM of three experiments. *P < 0.05, **P < 0.01, ***P < 0.001.
Similar results were obtained by genetically silencing SMO, and by using 5E1, a mAb specifically binding to Shh (Figures 2E,F). The extent of SMO knockdown at mRNA level is shown in Figure S3A. Notably, the efficacy of 5E1 mAb, in the absence of exogenous stimulation, suggests the existence of an autocrine loop maintaining Hh pathway constitutively active in these cells.
Conversely in the MeT5A cell line, a non-tumorigenic mesothelium cell line, the same genes were found not responsive to the treatment with GDC-0449, therefore suggesting that these cells do not maintain a constitutively active SMO-GLI pathway (Figure S3B).
In order to evaluate the effect of SMO inhibition on cell proliferation, we exposed HCT 116, SW480, SW620, and MeT5A cells, to GDC-0449 for 24–48 h. At the concentration of 1 μM, GDC-0449 significantly inhibited cell proliferation in HCT 116, SW480, and SW620 while causing negligible effect in MeT5A cells (Figures 3A–D.) At the molecular level, treatment with GDC-0449 reduced the expression of cell cycle promoters (Cyclin D1) while inducing the expression of cell cycle inhibitors (p21) in all of the three CC lines analyzed (Figures 3E,F). Accordingly, both genetic silencing of SMO and treatment with 5EI mAb led to a reduction of Cyclin D1 (Figure 3G), while increasing the expression of p21 (Figure 3H). In contrast, no significative changes in the expression of these genes where detected when MeT5A were treated with GDC-0449 at the same concentrations (Figure S3C). These results demonstrate that activation of Hh pathway delivers proliferative signals in CC cell lines.
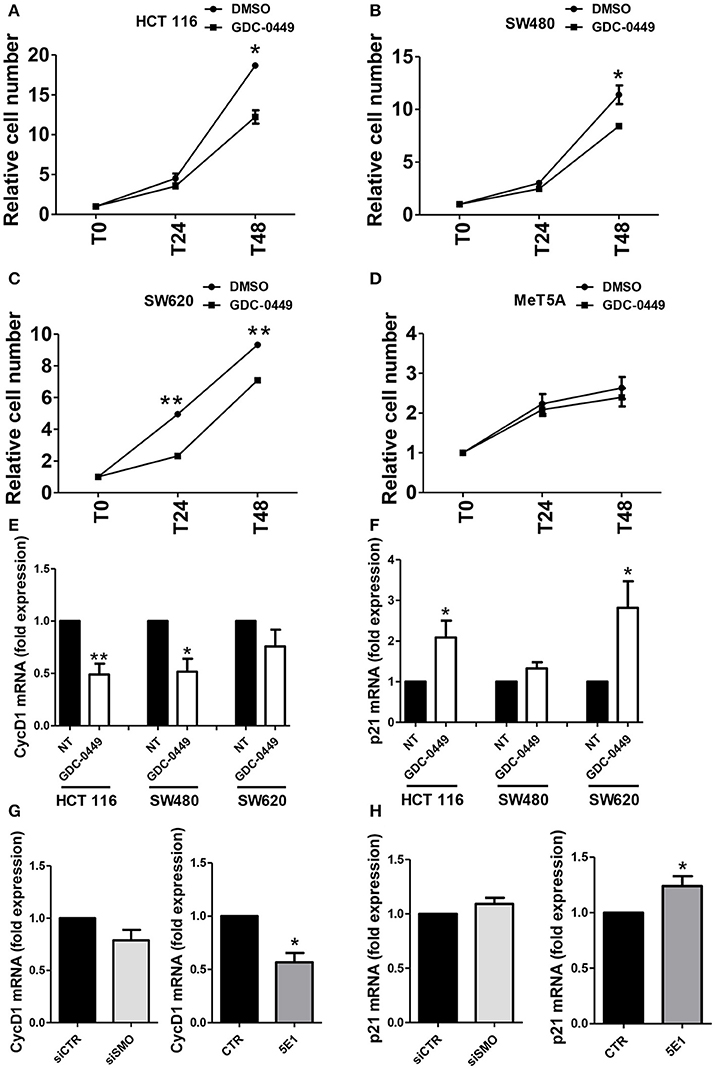
Figure 3. Effect of Hh inhibition on cell proliferation. (A) HCT 116, (B) SW480, (C) SW620 or (D) MeT5A cells were treated with vehicle (DMSO) or with GDC-0449 (1 μM) for 24–48 h. Cell proliferation was evaluated through microscopic count of living cells. Three independent experiments for each cell line were performed. Expression of Cyclin D1 (E) and p21 (F) was evaluated on total RNA by qRT-PCR. Bars represent means ± SEM of 7 experiment for HCT 116 cells, three experiments for SW480 and SW620 cells. (G) Expression of Cyclin D1 mRNA was evaluated in HCT 116 cells transfected with either control or specific SMO-targeting siRNAs (left), or in HCT 116 cells treated with either isotype-control mAb or 5E1 mAb (10 μg/ml) (right). (H) Expression of p21 mRNA was evaluated in HCT 116 cells transfected with either control or specific SMO-targeting siRNAs (left), or in HCT 116 cells treated with either isotype-control mAb or 5E1 mAb (10 μg/ml) (right). Bars represent means ± SEM of three independent experiments. *P < 0.05, **P < 0.01.
GDC-0449 Inhibits Cell-Directed Motility and Invasion and Induces Met
We then analyzed whether GDC-0449 potentially inhibits tumor invasion. In order to analyze the effect of GDC-0449 in controlling cell directed motility, which is relevant for tumor invasion, we performed a scratch assay. Confluent HCT 116 monolayers were pre-treated with GDC-0449 for 24 h, a scratch was performed and the scratch closure was evaluated after 24 h. As shown in Figure 4A, treatment with GDC-0449 significantly limited scratch closure.
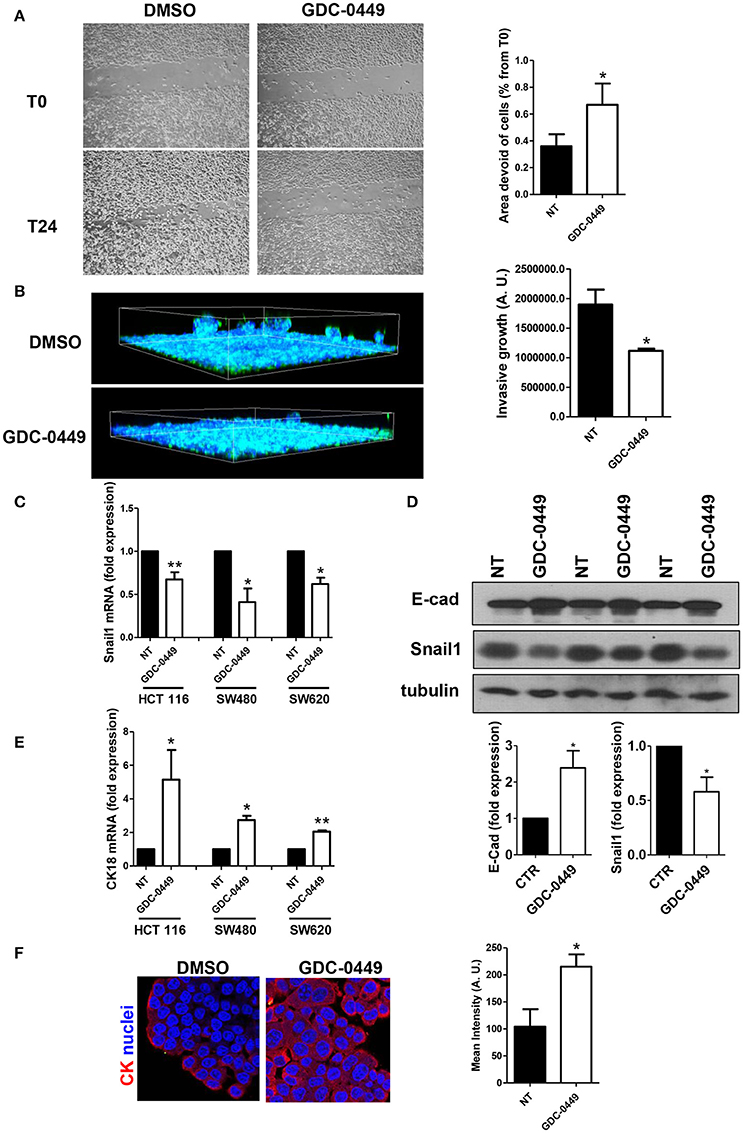
Figure 4. Effect of Hh inhibition on cell directed migration and on EMT-MET dynamics. (A) HCT 116 cells were treated with vehicle (DMSO) or with GDC-0449 (1 μM) in culture medium supplemented with 0.5% FCS. A scratch was performed and micrographs were taken 24 h after the scratch. Wound closure rate was quantified (right). Three independent experiments were performed. (B) HCT 116 cells were pretreated with GDC-0449 for 24 h and then overlaid with a Matrigel matrix. Invasion was monitored over 72 h. Three-dimensional invasion was enhanced by adding 20% FCS to the wells on top of Matrigel. Cells were stained with phalloidin (green) and Hoechst 33342 (cell nuclei, blue). Scale bar: 100 μm. 3 dimensional invasion rate was quantified (right). Three independent experiments were performed. (C,D) Effect of GDC-0449 on genes related to EMT-MET dynamics. (C) HCT 116, SW480, and SW620 cells were treated with GDC-0449 (1 μM) or DMSO for 24 h. Expression of Snail1 mRNA was evaluated on total RNA by qRT-PCR. Bars represent means ± SEM of 7 experiment for HCT 116 cells, three experiments for SW480 and SW620 cells. (D) Expression of Snail1 and E–cadherin in cells treated as above by western blot on total protein lysates of HCT 116 cells treated as stated in the figure. Tubulin was used as a loading control. Three independent experiments were performed. Quantification of E-cadherin and Snail1 protein expression is shown below. (E) Expression of Cytokeratin 18 (CK18) mRNA from total RNA of HCT 116 cells treated as in (C). (F) Cytokeratin expression and localization in HCT 116 cells was evaluated through immunofluorescence analysis. Nuclei were stained in blue with Hoechst. Quantification of Cytokeratin mean intensity is shown on the right. Two independent experiments were performed. *P < 0.05, **P < 0.01.
Similar results were obtained by using SW480 and SW620 cells (Figures S4A,B). By contrast GDC-0449 treatment had no effect on the Hh-irresponsive cell line MeT5A (Figure S4C).
Notably, the use of a 3-dimensional invasion assay on Matrigel matrices highlighted also a reduced invasive capacity of GDC-0449-treated HCT 116 cells (Figure 4B).
At the molecular level, treatment with GDC-0449 markedly reduced the expression of Snail1, a main driver of EMT and tumor invasion, in all CC cell lines analyzed, while increasing the expression of the typical epithelial marker E-cadherin (Figures 4C,D). At the same time, Cytokeratin 18 (CK18) expression was increased both at mRNA and at protein level (Figures 4E,F). These results were confirmed by genetic silencing of SMO and by the use of 5E1 mAb (Figures 5A–D).
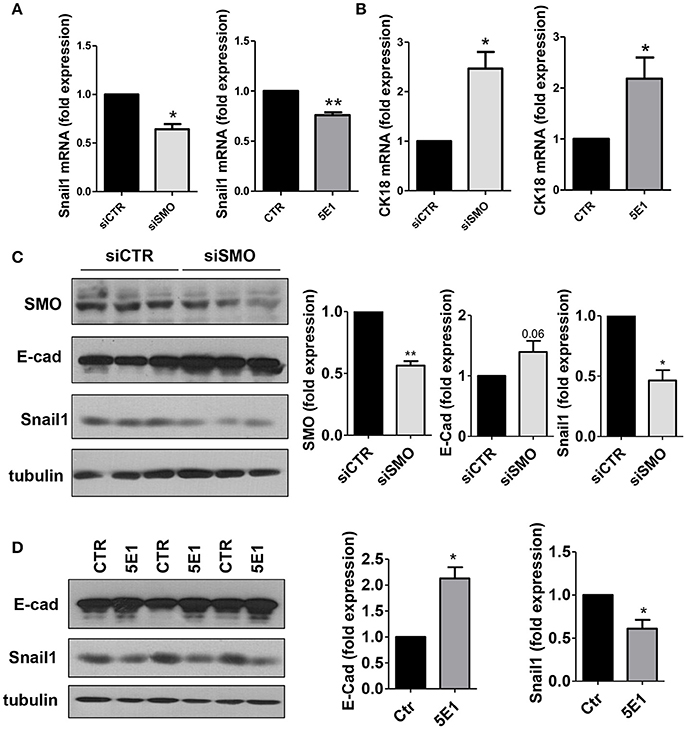
Figure 5. (A) Snail1 and (B) Cytokeratin 18 expression was evaluated on total RNA by qRT-PCR from HCT 116 cells transfected with either control or specific SMO-targeting siRNAs (left), or from cells treated with either isotype-control mAb or 5E1 mAb (10 μg/ml) (right) (n = 3). (C) Western blot showing expression of SMO, E-cadherin, Snail1 in HCT 116 cells transfected with either control or specific SMO-targeting siRNAs. Quantification of SMO, E-cadherin and Snail1 is shown on the left. (n = 3). (D) Western blot showing the expression of E-cadherin, Snail1 in HCT 116 cells treated with either isotype-control mAb or 5E1 mAb (10 μg/ml). Tubulin was used as loading control. E-cadherin and Snail1 quantifications are shown on the right. Bars represent means ± SEM of three independent experiments.*P < 0.05, **P < 0.01.
These data are in accordance with a data set from CC patients (Tables 2, 3), from the cancer genome atlas (TCGA) database (Cerami et al., 2012; Gao et al., 2013), where increased expression of Hh pathway genes (GLI1 and SMO) negatively correlates with protein expression of CDH1 (E-cadherin, epithelial marker) and positively correlate with CDH2 (N-Cadherin) and FN (Fibronectin), mesenchymal markers.
Overall, these results demonstrate that in primary and metastatic CC cell lines, the functional blockade of autocrine Hh pathway leads to a reduction of cellular growth and invasion through a reacquisition of epithelial-like features. These results may be helpful to more completely understand the complex biology of CC in light of future therapeutic strategies.
Discussion
The involvement of Hh in CC tumorigenesis is presently object of controversy. CC has a complex pathogenesis; during its progression, alterations of multiple genes and pathways are required. The observation that Hh pathway is tightly connected with other pathways that are often abnormally activated in CC, such as Wnt/β-catenin or Ras, strongly suggests its involvement in the multistep process of CC pathogenesis (Brechbiel et al., 2014). In fact, recent evidence indicates that Hh signaling may act in CC in autocrine, paracrine or cancer stem cell fashion (Scales and de Sauvage, 2009).
Notably, RNA Seq analysis in 382 patients highlighted a significative correlation between GLI1 increased expression and reduced survival in CC patients. These data support the results obtained by other studies (Xu et al., 2012), and prompted us to overcome the correlative analysis in pinpointing a mechanism; this attempt implied to inhibit Hh pathway in CC cell lines.
We first evaluated the effect of GDC-0449, a specific SMO inhibitor, on Hh signaling in three primary and metastatic CC cell lines. In all cells analyzed, treatment with GDC-0449 significantly reduced the expression of four known target genes (GLI1, PTCH1, HIP1, MUC5AC), this implying a constitutive activation of Hh pathway. The activity of Hh pathway in CC cell lines has been already analyzed in other studies, with controversial results (Douard et al., 2006; Chatel et al., 2007; Alinger et al., 2009).
The observed discrepancies with some of these studies may be explained by the different cell lines analyzed (Alinger et al., 2009) or by the usage of semi-quantitative techniques (Chatel et al., 2007).
In our experimental system, treatment of all three lines with GDC-0449 also significantly reduced cell proliferation, whereas there was no effect in a not-responsive cell line used as a negative control.
At the molecular level, cell cycle inducers (Cyclin D1) were decreased, whereas cell cycle blockers (p21) were increased. Therefore, the use of GDC-0449 directly impacts cellular proliferation and potentially tumor growth. These results were confirmed by performing SMO genetic silencing and by using a 5E1 Shh-specific inhibitory mAb. The effect observed using 5E1 presupposes the existence of an autocrine activatory loop involving Shh-SMO-dependent canonical pathway activation. A previous report analyzing loss-of-function mutation in PTCH1 suggested a role of autocrine Hh signaling in colorectal tumorigenesis (Chung and Bunz, 2013). The autocrine loop observed by us in CC cell lines may complement other studies supporting a model in which paracrine Hh ligands released by CC cells take a role in instructing the stromal components of the tumor (Yauch et al., 2008).
Our results emphasize the role of canonical Hh signaling in CC cell lines, although we do not exclude that other pathways may play a role. Indeed, besides the canonical Hh-PTCH1-SMO-driven signals, ligand-independent pathways have been demonstrated (Merchant et al., 2004; Amakye et al., 2013). For instance, in tumors, GLI transcription may be decoupled from upstream Shh–PTCH1–SMO signaling being regulated by TGF-β and KRAS (Nolan-Stevaux et al., 2009).
Treatment with GDC-0449 also led to reduced cellular motility in both a 2D and a 3D migration assays. These functional data correlated with an increased expression of epithelial markers (E-cadherin, Cytokeratin-18) and with a significant downregulation of Snail1, a main effector of TGF-β pathway. With respect to cellular motility and Snail1 expression, our results appear equally provocative in the light of the fact that in several circumstances TGF-β facilitates tumor invasiveness through induction of epithelial-mesenchymal transition (EMT). EMT is a complex phenotypic conversion of epithelial cells leading to the acquisition of a highly motile phenotype and other mesenchymal traits, and is a key mechanism by which pre-malignant epithelial cells acquire a highly invasive phenotype that leads to metastatic spreading. The evidence of EMT in CC in vivo was firstly demonstrated by Brabletz (Brabletz et al., 2005). Subsequently, genomics studies identified EMT as a major program in CC (Loboda et al., 2011). Moreover, expression of EMT-related genes correlated with poorer survival in CC, but not in other cancers (Tan et al., 2014).
Cell migration and invasiveness are driven by actin cytoskeleton dynamic polarization, which is controlled by the members of the Rho GTPase superfamily Rho, Rac, and Cdc42 (Ridley, 2015). At the same time, EMT dynamics, as well as the acquisition of stem-like phenotypes are characterized by selective activation of Rho GTPases (Yoon et al., 2017). Interestingly, recent studies link Hh pathway to Rho GTPases activation, providing potential molecular mechanisms for our observations (Peng et al., 2017).
Hh pathway has been shown to intersect and cooperate with other pathways by regulating the differentiation/proliferation status of the tissue. In the intestinal mucosa, Bone Morphogenetic Protein (BMP) and Hh pathways are preferentially activated in differentiated layers, whereas Notch and WNT pathways are activated in the basal cells of the crypt (Bertrand et al., 2012). Hh controls the expression of the Notch ligand Jagged2. Moreover, cross talks between TGF-β-induced pathways and Hh have been demonstrated (Perrot et al., 2013). There is evidence of an interplay between Hh and TGF-β pathways in both normal and malignant tissues. In particular, TGF-β may induce GLI1 expression in a GLI2-dependent manner independently from SMO in breast cancer (Hu et al., 2008). Furthermore, pharmacologic blockade of TGF-β signaling leads to a reduction of GLI1 expression in cyclopamine-resistant pancreatic adenocarcinoma (Dennler et al., 2007).
We hypothesize that in our cells Snail1 downregulation, mediated by Hh-pathway inhibition, contributes to the stabilization of an epithelial phenotype (underlined by the increase in E-cadherin and Cytokeratin 18 expression). Besides the well-known effect on tumor invasion, Snail1 downregulation may be effective in limiting chemoresistance, as recently demonstrated in CC (Lee T. Y. et al., 2016). Accordingly, Hh pathway inhibition by GDC-0449 was demonstrated to reduce the expression of a mesenchymal marker and to restore sensitivity to 5-fluorouracil in a 5-fluorouracil-resistant CC cell line (Liu et al., 2015).
While the role of Hh pathway in EMT induction in CC has already been demonstrated by Ruiz i Altaba and other groups (Varnat et al., 2009; Wang et al., 2012; Liu et al., 2015), our study firstly described the maintenance of an invasive “EMT like state” by an autocrine secretion of Hh ligands in CC cell lines. This cellular mechanism may play a role in promoting cellular invasion and metastatic spreading of CC to the liver, consistently with our previous findings on tumor-stroma interactions in liver neoplasms (Magistri et al., 2013). The impact of Hh pathway on CC tumorigenesis has been recently questioned in mouse models (Gerling et al., 2016; Lee J. J. et al., 2016). Using different experimental systems such as the AOM/DSS model of colitis-associated CC, it was demonstrated that Hh signaling is downregulated in CC, and that Hh pathway inhibition may intensify colon inflammation (colitis) in mice, thus promoting tumorigenesis.
Conversely, other recent studies linked the same pathway activation to pro-tumor effects and to the acquisition of a cancer stem-like behavior (Kangwan et al., 2016; Wang et al., 2016).
Finally, it has been demonstrated that Hh active pathway may hamper tumor immunosurveillance (for example downregulating MHC1 expression in epithelial tumor cells), inhibiting the immune response against the tumor (Otsuka et al., 2015; Hanna and Shevde, 2016). Therefore, the interpretation of in vivo models is so far extremely challenging.
In conclusion, our in vitro study established a causal link between constitutive Hh activity and the acquisition of pro-invasive, mesenchymal-like properties in CC cell lines.
When looking for a possible translational relevance of these discoveries, one may hypothesize that subsets of colorectal tumors endowed with abnormal Hh signaling due to mutations, such as a recently reported PTCH1 loss-of-function mutation (Chung and Bunz, 2013), that may not have been represented in the cohorts previously treated with Hh inhibitors (Berlin et al., 2013), may benefit from drugs inhibiting Hh activity such as GDC-0449.
Author Contributions
PM, CB, and RS: equally contributed to the final manuscript and are responsible for study design, lab experiments, and manuscript drafting; GN, MT, and GR: supervised all the study process and revised the final manuscript; NP, LR, and FD: are responsible for literature review and data analysis; PA and LM: are responsible for lab experiments duplicates and statistical analysis. TP is responsible for the analysis of TCGA database.
Funding
This study was funded by “Sapienza—University of Rome” (Funds for young researchers) and “AIRC” (Italian Association for Cancer Research) 2016 (n. 18843) (MT).
Conflict of Interest Statement
The authors declare that the research was conducted in the absence of any commercial or financial relationships that could be construed as a potential conflict of interest.
Acknowledgments
Authors want to thank Prof. Timothy Pawlik and Prof. Jason K. Sicklick for their passionate support and useful tips and Prof. Lucia Di Marcotullio for critically discussing the results.
Supplementary Material
The Supplementary Material for this article can be found online at: https://www.frontiersin.org/articles/10.3389/fphar.2017.00956/full#supplementary-material
References
Alinger, B., Kiesslich, T., Datz, C., Aberger, F., Strasser, F., Berr, F., et al. (2009). Hedgehog signaling is involved in differentiation of normal colonic tissue rather than in tumor proliferation. Virchows Archiv. 454, 369–379. doi: 10.1007/s00428-009-0753-7
Al-Sohaily, S., Biankin, A., Leong, R., Kohonen-Corish, M., and Warusavitarne, J. (2012). Molecular pathways in colorectal cancer. J. Gastroenterol. Hepatol. 27, 1423–1431. doi: 10.1111/j.1440-1746.2012.07200.x
Amakye, D., Jagani, Z., and Dorsch, M. (2013). Unraveling the therapeutic potential of the Hedgehog pathway in cancer. Nat. Med. 19, 1410–1422. doi: 10.1038/nm.3389
Battistelli, C., Cicchini, C., Santangelo, L., Tramontano, A., Grassi, L., Gonzalez, F. J., et al. (2017). The Snail repressor recruits EZH2 to specific genomic sites through the enrollment of the lncRNA HOTAIR in epithelial-to-mesenchymal transition. Oncogene 36, 942–955. doi: 10.1038/onc.2016.260
Benvenuto, M., Masuelli, L., De Smaele, E., Fantini, M., Mattera, R., Cucchi, D., et al. (2016). In vitro and in vivo inhibition of breast cancer cell growth by targeting the Hedgehog/GLI pathway with SMO (GDC-0449) or GLI (GANT-61) inhibitors. Oncotarget 7, 9250–9270. doi: 10.18632/oncotarget.7062
Berlin, J., Bendell, J. C., Hart, L. L., Firdaus, I., Gore, I., Hermann, R. C., et al. (2013). A randomized phase II trial of vismodegib versus placebo with FOLFOX or FOLFIRI and bevacizumab in patients with previously untreated metastatic colorectal cancer. Clin. Cancer Res. 19, 258–267. doi: 10.1158/1078-0432.CCR-12-1800
Bertrand, F. E., Angus, C. W., Partis, W. J., and Sigounas, G. (2012). Developmental pathways in colon cancer: crosstalk between WNT, BMP, Hedgehog and Notch. Cell Cycle 11, 4344–4351. doi: 10.4161/cc.22134
Brabletz, T., Hlubek, F., Spaderna, S., Schmalhofer, O., Hiendlmeyer, E., Jung, A., et al. (2005). Invasion and metastasis in colorectal cancer: epithelial-mesenchymal transition, mesenchymal-epithelial transition, stem cells and beta-catenin. Cells Tissues Organs 179, 56–65. doi: 10.1159/000084509
Brechbiel, J., Miller-Moslin, K., and Adjei, A. A. (2014). Crosstalk between hedgehog and other signaling pathways as a basis for combination therapies in cancer. Cancer Treat. Rev. 40, 750–759. doi: 10.1016/j.ctrv.2014.02.003
Cerami, E., Gao, J., Dogrusoz, U., Gross, B. E., Sumer, S. O., Aksoy, B. A., et al. (2012). The cBio cancer genomics portal: an open platform for exploring multidimensional cancer genomics data. Cancer Discov. 2, 401–404. doi: 10.1158/2159-8290.CD-12-0095
Chatel, G., Ganeff, C., Boussif, N., Delacroix, L., Briquet, A., Nolens, G., et al. (2007). Hedgehog signaling pathway is inactive in colorectal cancer cell lines. Int. J. Cancer 121, 2622–2627. doi: 10.1002/ijc.22998
Chung, J. H., and Bunz, F. (2013). A loss-of-function mutation in PTCH1 suggests a role for autocrine hedgehog signaling in colorectal tumorigenesis. Oncotarget 4, 2208–2211. doi: 10.18632/oncotarget.1651
Ciatto, S. (2007). Current cancer profiles of the Italian regions: should cancer incidence be monitored at a national level? Tumori 93, 529–531.
Dennler, S., André, J., Alexaki, I., Li, A., Magnaldo, T., ten Dijke, P., et al. (2007). Induction of sonic hedgehog mediators by transforming growth factor-beta: Smad3-dependent activation of Gli2 and Gli1 expression in vitro and in vivo. Cancer Res. 67, 6981–6986. doi: 10.1158/0008-5472.can-07-0491
Dijkgraaf, G. J., Alicke, B., Weinmann, L., Januario, T., West, K., Modrusan, Z., et al. (2011). Small molecule inhibition of GDC-0449 refractory smoothened mutants and downstream mechanisms of drug resistance. Cancer Res. 71, 435–444. doi: 10.1158/0008-5472.CAN-10-2876
Douard, R., Moutereau, S., Pernet, P., Chimingqi, M., Allory, Y., Manivet, P., et al. (2006). Sonic Hedgehog-dependent proliferation in a series of patients with colorectal cancer. Surgery 139, 665–670. doi: 10.1016/j.surg.2005.10.012
Ericson, J., Morton, S., Kawakami, A., Roelink, H., and Jessell, T. M. (1996). Two critical periods of Sonic Hedgehog signaling required for the specification of motor neuron identity. Cell 87, 661–673.
Gao, J., Aksoy, B. A., Dogrusoz, U., Dresdner, G., Gross, B., Sumer, S. O., et al. (2013). Integrative analysis of complex cancer genomics and clinical profiles using the cBioPortal. Sci. Signal. 6:pl1. doi: 10.1126/scisignal.2004088
Gerling, M., Buller, N. V., Kirn, L. M., Joost, S., Frings, O., Englert, B., et al. (2016). Stromal Hedgehog signalling is downregulated in colon cancer and its restoration restrains tumour growth. Nat. Commun. 7:12321. doi: 10.1038/ncomms12321
Gupta, S., Takebe, N., and Lorusso, P. (2010). Targeting the Hedgehog pathway in cancer. Ther. Adv. Med. Oncol. 2, 237–250. doi: 10.1177/1758834010366430
Hanna, A., and Shevde, L. A. (2016). Hedgehog signaling: modulation of cancer properies and tumor mircroenvironment. Mol. Cancer 15, 24. doi: 10.1186/s12943-016-0509-3
Hu, M., Yao, J., Carroll, D. K., Weremowicz, S., Chen, H., Carrasco, D., et al. (2008). Regulation of in situ to invasive breast carcinoma transition. Cancer Cell 13, 394–406. doi: 10.1016/j.ccr.2008.03.007
Jiang, J., and Hui, C. C. (2008). Hedgehog signaling in development and cancer. Dev. Cell 15, 801–812. doi: 10.1016/j.devcel.2008.11.010
Kangwan, N., Kim, Y. J., Han, Y. M., Jeong, M., Park, J. M., Go, E. J., et al. (2016). Sonic hedgehog inhibitors prevent colitis-associated cancer via orchestrated mechanisms of IL-6/gp130 inhibition, 15-PGDH induction, Bcl-2 abrogation, and tumorsphere inhibition. Oncotarget 7, 7667–7682. doi: 10.18632/oncotarget.6765
Lee, T. Y., Liu, C. L., Chang, Y. C., Nieh, S., Lin, Y. S., Jao, S. W., et al. (2016). Increased chemoresistance via snail-raf kinase inhibitor protein signaling in colorectal cancer in response to a nicotine derivative. Oncotarget 7, 23512–23520. doi: 10.18632/oncotarget.8049
Lee, J. J., Rothenberg, M. E., Seeley, E. S., Zimdahl, B., Kawano, S., Lu, W. J., et al. (2016). Control of inflammation by stromal Hedgehog pathway activation restrains colitis. Proc. Natl. Acad. Sci. U.S.A. 113, E7545–E7553. doi: 10.1073/pnas.1616447113
Liu, Y., Du, F., Zhao, Q., Jin, J., Ma, X., and Li, H. (2015). Acquisition of 5-fluorouracil resistance induces epithelial-mesenchymal transitions through the Hedgehog signaling pathway in HCT-8 colon cancer cells. Oncol. Lett. 9, 2675–2679. doi: 10.3892/ol.2015.3136
Loboda, A., Nebozhyn, M. V., Watters, J. W., Buser, C. A., Shaw, P. M., Huang, P. S., et al. (2011). EMT is the dominant program in human colon cancer. BMC Med. Genom. 4:9. doi: 10.1186/1755-8794-4-9
Magistri, P., Leonard, S. Y., Tang, C. M., Chan, J. C., Lee, T. E., and Sicklick, J. K. (2013). The glypican 3 hepatocellular carcinoma marker regulates human hepatic stellate cells via Hedgehog signaling. J. Surg. Res. 187, 377–385. doi: 10.1016/j.jss.2013.12.010
Merchant, M., Vajdos, F. F., Ultsch, M., Maun, H. R., Wendt, U., Cannon, J., et al. (2004). Suppressor of fused regulates Gli activity through a dual binding mechanism. Mol. Cell. Biol. 24, 8627–8641. doi: 10.1128/MCB.24.19.8627-8641.2004
Nappi, A., Nasti, G., Romano, C., Cassata, A., Silvestro, L., Ottaiano, A., et al. (2016). Multimodal treatment of recurrent colorectal cancer. World Cancer Res. J. 3:e719.
Nolan-Stevaux, O., Lau, J., Truitt, M. L., Chu, G. C., Hebrok, M., Fernández-Zapico, M. E., et al. (2009). GLI1 is regulated through Smoothened-independent mechanisms in neoplastic pancreatic ducts and mediates PDAC cell survival and transformation. Genes Dev. 23, 24–36. doi: 10.1101/gad.1753809
Otsuka, A., Dreier, J., Cheng, P. F., Nägeli, M., Lehmann, H., Felderer, L., et al. (2015). Hedgehog pathway inhibitors promote adaptive immune responses in basal cell carcinoma. Clin. Cancer Res. 21, 1289–1297. doi: 10.1158/1078-0432.CCR-14-2110
Peng, W. X., Zhu, S. L., Zhang, B. Y., Shi, Y. M., Feng, X. X., Liu, F., et al. (2017). Smoothened regulates migration of fibroblast-like synoviocytes in rheumatoid arthritis via activation of Rho GTPase signaling. Front. Immunol. 8:159. doi: 10.3389/fimmu.2017.00159
Perrot, C. Y., Javelaud, D., and Mauviel, A. (2013). Overlapping activities of TGF-beta and Hedgehog signaling in cancer: therapeutic targets for cancer treatment. Pharmacol. Ther. 137, 183–199. doi: 10.1016/j.pharmthera.2012.10.002
Ridley, A. J. (2015). Rho GTPase signalling in cell migration. Curr. Opin. Cell Biol. 36, 103–112. doi: 10.1016/j.ceb.2015.08.005
Rimkus, T. K., Carpenter, R. L., Qasem, S., Chan, M., and Lo, H. W. (2016). Targeting the sonic hedgehog signaling pathway: review of smoothened and GLI inhibitors. Cancers 8:22. doi: 10.3390/cancers8020022
Scales, S. J., and de Sauvage, F. J. (2009). Mechanisms of Hedgehog pathway activation in cancer and implications for therapy. Trends Pharmacol. Sci. 30, 303–312. doi: 10.1016/j.tips.2009.03.007
Sénicourt, B., Boudjadi, S., Carrier, J. C., and Beaulieu, J. F. (2016). Neoexpression of a functional primary cilium in colorectal cancer cells. Heliyon 2:e00109. doi: 10.1016/j.heliyon.2016.e00109
Strippoli, R., Loureiro, J., Moreno, V., Benedicto, I., Perez Lozano, M. L., Barreiro, O., et al. (2015). Caveolin-1 deficiency induces a MEK-ERK1/2-Snail-1-dependent epithelial-mesenchymal transition and fibrosis during peritoneal dialysis. EMBO Mol. Med. 7, 102–123. doi: 10.15252/emmm.201404127
Sun, K., Deng, H. J., Lei, S. T., Dong, J. Q., and Li, G. X. (2013). miRNA-338-3p suppresses cell growth of human colorectal carcinoma by targeting smoothened. World J. Gastroenterol. 19, 2197–2207. doi: 10.3748/wjg.v19.i14.2197
Takebe, N., Miele, L., Harris, P. J., Jeong, W., Bando, H., Kahn, M., et al. (2015). Targeting notch, Hedgehog, and Wnt pathways in cancer stem cells: clinical update. Nat. Rev. Clin. Oncol. 12, 445–464. doi: 10.1038/nrclinonc.2015.61
Tan, T. Z., Miow, Q. H., Miki, Y., Noda, T., Mori, S., Thiery, J. P., et al. (2014). Epithelial-mesenchymal transition spectrum quantification and its efficacy in deciphering survival and drug responses of cancer patients. EMBO Mol. Med. 6, 1279–1293. doi: 10.15252/emmm.201404208
Varnat, F., Duquet, A., Malerba, M., Zbinden, M., Mas, C., Gervaz, P., et al. (2009). Human colon cancer epithelial cells harbour active HEDGEHOG-GLI signalling that is essential for tumour growth, recurrence, metastasis and stem cell survival and expansion. EMBO Mol. Med. 1, 338–351. doi: 10.1002/emmm.200900039
Wang, D., Hu, G., Du, Y., Zhang, C., Lu, Q., Lv, N., et al. (2017). Aberrant activation of hedgehog signaling promotes cell proliferation via the transcriptional activation of forkhead Box M1 in colorectal cancer cells. J. Exp. Clin. Cancer Res. 36:23. doi: 10.1186/s13046-017-0491-7
Wang, R., Wei, J., Zhang, S., Wu, X., Guo, J., Liu, M., et al. (2016). Peroxiredoxin 2 is essential for maintaining cancer stem cell-like phenotype through activation of Hedgehog signaling pathway in colon cancer. Oncotarget 7, 86816–86828. doi: 10.18632/oncotarget.13559
Wang, T. P., Hsu, S. H., Feng, H. C., and Huang, R. F. (2012). Folate deprivation enhances invasiveness of human colon cancer cells mediated by activation of sonic hedgehog signaling through promoter hypomethylation and cross action with transcription nuclear factor-kappa B pathway. Carcinogenesis 33, 1158–1168. doi: 10.1093/carcin/bgs138
Xu, M., Li, X., Liu, T., Leng, A., and Zhang, G. (2012). Prognostic value of hedgehog signaling pathway in patients with colon cancer. Med. Oncol. 29, 1010–1016. doi: 10.1007/s12032-011-9899-7
Yauch, R. L., Gould, S. E., Scales, S. J., Tang, T., Tian, H., Ahn, C. P., et al. (2008). A paracrine requirement for hedgehog signalling in cancer. Nature 455, 406–410. doi: 10.1038/nature07275
Keywords: colon cancer, hedgehog pathway, SMO inhibition, EMT, cell plasticity, cell invasiveness
Citation: Magistri P, Battistelli C, Strippoli R, Petrucciani N, Pellinen T, Rossi L, Mangogna L, Aurello P, D'Angelo F, Tripodi M, Ramacciato G and Nigri G (2018) SMO Inhibition Modulates Cellular Plasticity and Invasiveness in Colorectal Cancer. Front. Pharmacol. 8:956. doi: 10.3389/fphar.2017.00956
Received: 01 October 2017; Accepted: 15 December 2017;
Published: 02 February 2018.
Edited by:
Giovanni Li Volti, Università degli Studi di Catania, ItalyReviewed by:
Richard T. Premont, Duke University, United StatesBarbara Stecca, Azienda Ospedaliero-Universitaria Careggi, Italy
Massimo Donadelli, University of Verona, Italy
Copyright © 2018 Magistri, Battistelli, Strippoli, Petrucciani, Pellinen, Rossi, Mangogna, Aurello, D'Angelo, Tripodi, Ramacciato and Nigri. This is an open-access article distributed under the terms of the Creative Commons Attribution License (CC BY). The use, distribution or reproduction in other forums is permitted, provided the original author(s) and the copyright owner are credited and that the original publication in this journal is cited, in accordance with accepted academic practice. No use, distribution or reproduction is permitted which does not comply with these terms.
*Correspondence: Giuseppe Nigri, Z2l1c2VwcGUubmlncmlAdW5pcm9tYTEuaXQ=
†These authors have contributed equally to this work.