- 1Laboratory for Neuronal Circuit Dynamics, Department of Medicine, Imperial College London, London, United Kingdom
- 2Centre for Neurotechnology, Institute of Biomedical Engineering, Imperial College London, London, United Kingdom
The serotonin (5-HT) 2A receptor is the primary molecular target of serotonergic hallucinogens, which trigger large-scale perturbations of the cortex. Our understanding of how 5-HT2A activation may cause the effects of hallucinogens has been hampered by the receptor unselectivity of most of the drugs of this class. Here we used 25CN-NBOH (N-(2-hydroxybenzyl)-2,5-dimethoxy-4-cyanophenylethylamine), a newly developed selective 5-HT2A agonist, and tested it with regard to the head-twitch-response (HTR) model of 5-HT2A activity and effects on locomotion. 25CN-NBOH evoked HTRs with an inverted u-shape-like dose-response curve and highest efficacy at 1.5 mg/kg, i.p. HTR occurrence peaked within 5 min after agonist injection, and exponentially decreased to half-maximal frequency at ~11 min. Thorough habituation to the experimental procedures (including handling, saline injection, and exposure to the observational boxes 1 day before the experiment) facilitated the animals' response to 25CN-NBOH. 25CN-NBOH (1.5 mg/kg, i.p.) induced HTRs were blocked by the 5-HT2A antagonist ketanserin (0.75 mg/kg, 30 min pre), but not by the 5-HT2C antagonist SB-242084 (0.5 mg/kg, i.p., 30 min pre). SB-242084 instead slightly increased the number of HTRs occurring at a 3.0-mg/kg dose of the agonist. Apart from HTR induction, 25CN-NBOH also modestly increased locomotor activity of the mice. Repeated once-per-day injections (1.5 mg/kg, i.p.) led to reduced occurrence of 25CN-NBOH induced HTRs. This intermediate tolerance was augmented when a second (higher) dose of the drug (3.0 mg/kg) was interspersed. Short-interval tolerance (i.e., tachyphylaxis) was observed when the drug was injected twice at intervals of 1.0 and 1.5 h at either dose tested (1.5 mg/kg and 0.75 mg/kg, respectively). Inducing ketanserin-sensitive HTRs, which are dependent on environmental valences and which show signs of tachyphylaxis and tolerance, 25CN-NBOH shares striking features common to serotonergic hallucinogens. Given its distinct in vitro selectivity for 5-HT2A over non5-HT2 receptors and its behavioral dynamics, 25CN-NBOH appears to be a powerful tool for dissection of receptor-specific cortical circuit dynamics, including 5-HT2A related psychoactivity.
Introduction
The serotonin (5-HT) 2A receptor is a member of the 5-HT2 seven-transmembrane receptor family and is expressed in various cells and tissues across the mammalian body, with highest expression levels in the brain (see GPCR database; Regard et al., 2008). 5-HT2A receptors are specifically enriched in the cerebral cortex, particularly on the apical dendrites of pyramidal cells in layer V (Weber and Andrade, 2010). By enhancing cortical glutamate release, 5-HT2A receptors may modulate cognitive processes, such as working memory and attention (Williams et al., 2002; Mirjana et al., 2004). The 5-HT2A receptor is considered as an important drug target, with potential implications of both agonists and antagonists in the treatment of various psychiatric conditions, including depression and anxiety (Mascher, 1967; Quesseveur et al., 2012; Buchborn et al., 2014; Carhart-Harris et al., 2016; Ross et al., 2016; Carhart-Harris and Goodwin, 2017). Blockade of 5-HT2A receptors has been shown to counteract alterations of consciousness induced by serotonergic hallucinogens such as lysergic acid diethylamide (LSD) (Kraehenmann et al., 2017), and more specifically, 5-HT2A receptors of layer 5 pyramidal cells are thought to be a key mediator of psychedelic activity (Vollenweider and Kometer, 2010; Muthukumaraswamy et al., 2013; Nichols, 2016). Despite the availability of relatively selective 5-HT2A antagonists (see PDSP Ki database; Roth et al., 2000), our understanding of the general neurophysiology of (cortical) 5-HT2A receptors is limited by the lack of highly selective 5-HT2A agonists. The 4-iodo-2,5-dimethoxy-analog of amphetamine DOI, for instance, which often has been the drug of choice in animal studies related to 5-HT2A functions, seems to exhibit confounded affinity for 5-HT2 as well as adrenergic receptors (Ray, 2010). In search of agonists with higher 5-HT2A selectivity, a series of 48 compounds was recently developed based on the phenethylamine backbone shared by DOI-like serotonergic hallucinogens (Hansen et al., 2014). Amongst these, as indicated by an extensive follow-up in vitro receptor binding screening, the newly designed N-(2-hydroxy)benzyl substituted 4-cyano-2,5-dimethoxyphenethylamine (25CN-NBOH) turned out to be one of the most promising candidates: 25CN-NBOH is characterized by a high affinity for 5-HT2A receptors (in vitro radioligand competition binding, av. Ki: ~1.72 nM), by a ≥ 100-fold selectivity for binding to this receptor as compared to a plethora of non-5-HT2 targets (including other G-protein coupled receptors, ion channels, transporters, and enzymes), and robust 5-HT2A selectivity over 5-HT2B/2C receptors (Ki in vitro [averaged across studies]: ~56 nM for 5-HT2B and ~83 nM for 5-HT2C, respectively) (Hansen, 2010; Halberstadt et al., 2016; Jensen et al., 2017). Therefore, 25CN-NBOH appears advantageous over other currently used 5-HT2A agonists subjected to a similarly scrutinous receptor profiling (Ray, 2010; see also Ki database).
Here, we aimed to determine the suitability of 25CN-NBOH for prospective brain imaging studies in chronic in vivo mouse models. To this end, we employed the head twitch response (HTR) as well as locomotion as a behavioral readout of central 5-HT2A activity. We determined the dose range of 25CN-NBOH required for parenteral administration to induce central 5-HT2A activation, the time-course of the central action, sensitivity to 5-HT2A antagonism and experimental familiarity, a possible involvement of 5-HT2C receptors in 25CN-NBOH induced HTRs, as well as potential development of tachyphylaxis and tolerance.
Materials and Methods
Animals
All experimental procedures performed at Imperial College London were in accordance with the UK Animal Scientific Procedures Act (1986) under Home Office Personal and Project licenses (I5B5A6029, IA615553C; PPL 70/7818), following appropriate ethical review.
Adult mice of both sexes and with mixed genetic background (stock of mainly C57BL/6JxB6CBAF1 background) were bred in-house at the Central Biomedical Services (CBS) of Imperial College London. They were housed in individually ventilated cages with a 12:12 h light/dark cycle and maintained at an ambient temperature of 21 ± 2°C at 55 ± 10% humidity. Mice were provided with standard rodent-chow pellets (Special Diet Services, #RM1) and water ad libitum.
Drugs
25CN-NBOH (a kind gift from Jesper L. Kristensen, University of Copenhagen) and ketanserin (Tocris Biosciences; Avonmouth, UK) were dissolved in isotonic saline, SB-242084 (Tocris Biosciences; Avonmouth, UK) in DMSO (10% in saline). Drugs were applied intraperitoneally or subcutaneously (<10 ml/kg), as indicated.
Behavioral Experiments
Animals were allowed to habituate to the (observational) non-home boxes (25.5 × 12.5 × 12.5 cm [L × W × H]) for 30 min. Observational boxes were shielded with red acrylic plates to minimize the animals' visual awareness of the experimenter.
Head Twitches
For quantification of HTRs, animals were treated with saline or the respective dose of 25CN-NBOH (0.5–3.0 mg/kg, i.p. (or 1.5 mg/kg, s.c.]) and observed for 20–60 min. The frequency of head twitches, defined as rapid and brisk rotational movement of the head around the longitudinal axis of the animals' body (Handley and Singh, 1986) (see supplemental Movie), was counted in 2- or 5-min bins starting directly after injection. The sensitivity of 25CN-NBOH (1.5 mg/kg [vs. 3.0] mg/kg, i.p.) induced head twitches to 5-HT2A and/or 5-HT2C antagonism was tested by pre-treating the animals with ketanserin (0.75 mg/kg, i.p.) and SB-242084 (0.5 mg/kg, i.p.), respectively, 30 min prior to agonist administration. The impact of experimental familiarity was investigated by simulation of aspects of the experimental procedure (i.e., handling, injection of saline, and exposure to the observational non-home cages for 30 min before, and for 20 min after saline injection) 1 day before the actual 25CN-NBOH experiment.
Tachyphylaxis, defined as in-between-session tolerance occurring at repeated short-interval application, was tested by injecting saline or 25CN-NBOH (0.75 or 1.5 mg/kg, i.p.) twice at a 1.0- or 1.5-h interval, and comparing the average HTR frequency in each 30-min observation period. For subchronic tolerance, animals were treated for 4 days with saline or 25CN-NBOH. 25CN-NBOH was injected once (1.5 mg/kg, i.p. [morning]) or twice (1.5 mg/kg, i.p. [morning] and 3.0 mg/kg, i.p. [evening of days 1–3]) per day and HTRs were counted for 20 min after each morning application. The twice-per-day regimen, with the increased dose (i.e., 3.0 mg/kg) in the evening, was chosen based on findings for LSD, which indicate that tolerance development is slightly facilitated by a higher frequency of application, but more strongly facilitated by a higher frequency of application with increased dosing every other application (Buchborn et al., 2016).
Locomotion
Locomotion was quantified for 90 min directly after saline or 25CN-NBOH (1.5 mg/kg, i.p.) injection, averaged across three 30-min bins, and analyzed for distance traveled (in m), mobility time (in s), and average speed (in m/s) using Any-Maze Video Tracking Software (Stoelting). Mobility refers to times animals showed locomotion or rearing (excluding freezing and stationary grooming); speed was calculated as distance traveled against the cumulative mobility time periods.
Statistics
The data from the dose-response, antagonist, and pre-habituation experiments were analyzed using non-parametric Kruskal-Wallis test with Dunn's multiple post-hoc comparisons, or Mann-Whitney U-testing (as a priori planned). Tolerance (HTR) and time-course data (HTR and locomotion) were subjected to a two- and three-factor ANOVA with repeated measures on one factor, respectively, and followed up on by Bonferroni-corrected multiple comparisons. For HTRs, time-course data of interest were fitted by non-linear regression analysis, and the decay constant provided by the best-fit exponential equation (HTRt = e−lambda*t) subsequently used for determination of 25CN-NBOH's half-life (t½ = ln[2]/lambda). Calculations were carried out by SPSS or GraphPad Prism software. Statistical significance was assumed if the null hypothesis (e.g., drug has no action) could be rejected at ≤ 0. 05 probability level.
Results
Dose-Response Relationship, Ketanserin-Sensitivity, and Effect of Experimental Familiarity on 25CN-NBOH Induced Head Twitches
Mice received a single injection of either saline (control) or 25CN-NBOH (0.5–3.0 mg/kg, [i.p. or s.c.]) and were monitored for HTRs during the following 20 min. While control animals in this observation period rarely showed any behaviors rated as HTRs [0.83 ± 0.31 (mean ± SEM)], the incidence of HTRs markedly increased for all tested doses of 25CN-NBOH. Frequencies (mean ± SEM) ranged from 13.67 ± 4.26 for the lowest dose (0.5 mg/kg, i.p.), 37.67 ± 6.04 to 40.67 ± 5.77 for the medium dose (1.5 mg/kg, i.p. vs. s.c.), and 27.60 ± 4.52 for the highest dose tested (3.0 mg/kg, i.p.; Figures 1A,B). Inference-statistically, however, only the 1.5-mg/kg dose significantly differed from the saline group (X2 [10, N = 62] = 31.49, p ≤ 0.001; Dunn's post-hoc [comparison to control], 1.5 mg/kg i.p. or s.c., each p ≤ 0.01). At none of the doses tested, stereotypies other than HTRs (including any symptoms of the serotonin syndrome) were noted.
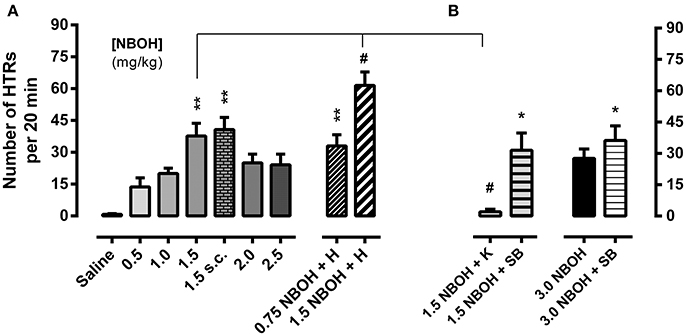
Figure 1. 25CN-NBOH induced head twitches (HTRs) (as observed for the first 20 min post-application). (A) Dose–response relation (0.5–2.5 mg/kg i.p. vs. 1.5 mg/kg s.c.) and effect of experimental habituation (0.75 vs. 1.5 mg/kg NBOH i.p. + H). (B) Effect of the 5-HT2A antagonist ketanserin [1.5 mg/kg NBOH + 0.75 mg/kg K (30 min pre-treatment), i.p.] and the 5-HT2C antagonist SB-242084 [1.5 vs. 3.0 mg/kg NBOH + 0.5 mg/kg SB (30 min pre-treatment), i.p.], respectively. Mean + SEM; n = 4–6 per group. Kruskal-Wallis, post-hoc comparison to saline, *p ≤ 05 and **p ≤ 01; or to agonist w/o habituation and w/o antagonist pre-treatment, respectively, #p ≤.05.
The 5-HT2A antagonist ketanserin (0.75 mg/kg, i.p.), when applied 30 min before the agonist, near-completely prevented the 25CN-NBOH (1.5-mg/kg, i.p.) induced HTRs (mean ± SEM: 2.0 ± 1.29) (X2 [4, N = 30] = 14.47, p ≤ 0.01; Dunn's post-hoc [comparison to 1.5 NBOH w/o antagonist and to control, respectively], p ≤ 0.05 and n.s.). The selective 5-HT2C antagonist SB-242084 (0.5 mg/kg, i.p., 30 min pre-treatment), did not prevent 25CN-NBOH from inducing significant HTRs (mean ± SEM: 31.50 ± 8.25; Dunn's post-hoc (comparison to saline), p ≤ 0.05]. SB-242084, although not affecting the effect of 1.5-mg/kg 25CN-NBOH, increased the animals' response to the 3.0-mg/kg dose of the agonist, leading to HTRs of significant extent (mean ± SEM: 36.20 ± 7.02, p ≤ 0.05; Figure 1B).
Handling the animals and thoroughly habituating them to the experimental procedures (including exposure to observational boxes and saline injection 1 day before the 25CN-NBOH experiment), respectively, facilitated agonist induced HTRs; a 0.75-mg/kg sub-threshold dose was enabled to induce significant twitching (mean ± SEM: 33.0 ± 5.28; p ≤ 0.01) and the response to a 1.5-mg/kg dose was significantly increased (mean ± SEM: 61.5 ± 6.3; p ≤ 0.05; Figure 1A).
Time-Course of 25CN-NBOH Induced Head Twitches
To investigate the time-course of the behavioral effect of the different 25CN-NBOH doses, we counted HTRs in 5-min bins as they occurred over 30 min post-injection (Figure 2). Repeated measures ANOVA with Bonferroni-corrected post-hoc analysis revealed significant increases in HTR frequency as a function of time [F(1.84, 27.66) = 57.52, p ≤ 0.001], treatment [F(2, 15) = 30.41, p ≤ 0.001], as well as of time × treatment interaction [F(3.68, 27.66) = 26.52, p ≤ 0.001]. All but the 0.5- and the 1.0-mg/kg dose elicited significant HTRs in the first observation window (0–5 min), where they peaked (1.5 mg/kg: 14.33 ± 2.67, p ≤ 0.001; 2.0 mg/kg: 9.67 ± 2.6, p ≤ 0.05; 2.5 mg/kg: 11.17 ± 1.68, p ≤ 0.01) and then steadily decreased over time. As depicted in the inset of Figure 2, the temporal course of 25CN-NBOH (1.5 mg/kg, i.p.) induced HTRs is well-fitted by an exponential decay function, with the effect of the drug declining to half after 11 min. The 1.0-, 2.0-, and 2.5-mg/kg doses decayed with similar half-lives between around 9 and 12 min.
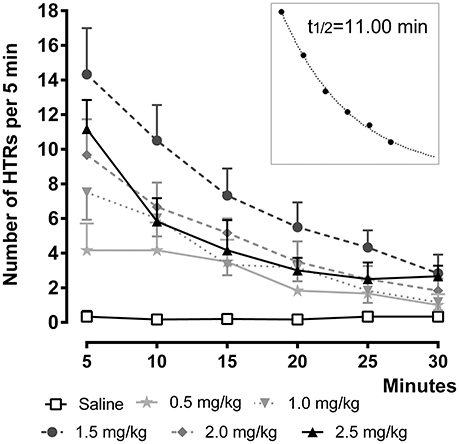
Figure 2. Time-course of 25CN-NBOH (0.5–2.5 mg/kg, i.p.) induced head twitches (HTRs) (as observed for the first 30 min post-application). Mean ± SEM; n = 6 per group. The inset replots the means of the 1.5-mg/kg dose as fitted by an exponential decay function.
In an additional set of experiments, we studied the time-course of the effect produced by the 1.5-mg/kg dose over a longer observation time period. To this end, 25CN-NBOH induced head twitches (i.p. vs. s.c.) were counted over 60 min at a 2-min-observation resolution. After i.p. injection, the frequency of HTRs peaked within the first 2-min bin (mean ± SEM: 6.8 ± 1.32) and then decayed with a t½ of 8.56 min. For s.c. injection, the peak occurred with a slight delay in the second 2-min bin (mean ± SEM: 7.00 ± 0.71) and decayed at a t½ of 13.59 min. Overall, as indicated by repeated measures ANOVA, there were no route-dependent time-course differences. The average (± SEM) of the three half-lives calculated for the 1.5-mg/kg dose is 11.05 ± 1.45.
25CN-NBOH's Effect on Locomotor Activity
To investigate how the dose of 25CN-NBOH that is the most effective in the head-twitch model (i.e., 1.5 mg/kg) would affect the animals' overall behavior, we also monitored locomotion. Splitting the 90-min observational period into three bins of 30 min, we found significant main effects for locomotion [F(1, 14) = 571.22, p ≤ 0.001], treatment [F(1, 14) = 4.62, p ≤ 0.05], time [F(2, 28) = 140.97, p ≤ 0.001], as well as significant interactions in terms of locomotion × treatment [F(1, 14) = 5.44, p ≤ 0.05] and locomotion × time [F(1.71, 23.98) = 111.17, p ≤ 0.001]. Averaged across the three time-bins, 25CN-NBOH treated animals were more mobile [mean ± SEM (% of 30 min): 18.23 ± 2.75 (saline) vs. 26.93 ± 2.50 (NBOH), post-hoc p ≤ 0.05] and traveled a significantly greater distance [mean ± SEM (m per 30 min): 10.17 ± 1.45 (saline) vs. 15.07 ± 1.75 (NBOH), post-hoc p ≤ 0.05] than saline treated mice. However, both treated and untreated mice were more often immobile than mobile (see Figures 3A,B). Also, for the times of mobility, there was no significant difference in average speed [mean ± SEM (m/s per 30 min): 0.029 ± 0.002 (saline) 0.028 ± 0.002 (NBOH), post-hoc, n.s.] (Figure 3C). Following up on the locomotion × time interaction, both groups showed a constant decrease in the locomotion parameter distance traveled (mean ± SEM [m]: 21.56 ± 2.11, 7.48 ± 2.31, and 1.48 ± 0.51 [saline]; 30.62 ± 2.82, 9.67 ± 1.94, and 4.91 ± 1.37 [NBOH]) and mobility time (mean ± SEM [% of 30 min]: 37.86 ± 3.18, 14.12 ± 4.75, and 2.74 ± 1.03 [saline]; 50.46 ± 3.69, 18.04 ± 3.43, and 12.29 ± 2.98 [NBOH]) from the first, to the second, to the third interval (Figures 3A,B). For either parameter, the differences between the three factor levels of time across groups became significant (post-hoc, each p ≤ 0.05). However, no significant time-dependency could be seen for average speed (mean ± SEM [m/s per 30 min]: 0.031 ± 0.001, 0.030 ± 0.002, and 0.024 ± 0.006 [saline]; 0.033 ± 0.001, 0.028 ± 0.002, and 0.023 ± 0.003 [NBOH]) (post-hoc across groups, n.s.) (Figure 3C).
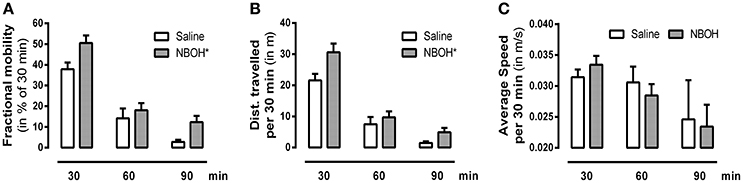
Figure 3. Effect of 25CN-NBOH (1.5 mg/kg, i.p.) on locomotion. Three 30-min intervals for the first 90 min after injection. (A) Fractional mobility (in % of 30 min); (B) distance traveled (in m per 30 min); (C) average speed (in m/s per 30 min). Mean + SEM; n = 8 per group. Repeated measures ANOVA, Bonferroni-corrected across-time post-hoc comparison to control, *p ≤ 05.
Tachyphylaxis and Tolerance to 25CN-NBOH Induced Head Twitches
In an independent set of experiments, we explored whether 25CN-NBOH induced HTRs showed signs of short-term tolerance. We therefore repeatedly applied the drug at different intervals. Similarly, as reported above, 25CN-NBOH (1.5 mg/kg, s.c.) treated mice exhibited an average of 34.67 ± 4.65 HTRs within 30 min, which was significantly more than observed in saline treated mice (mean ± SEM: 1.4 ± 0.25) (p ≤.01). Re-applying the same dose of 25CN-NBOH one or 1½ h after the first application substantially reduced the efficacy of the drug. Main effects for time [F(1, 24) = 64.28, p ≤ 0.001], group [F(1, 19) = 62.1, p ≤ 0.001], and time × group interaction [F(4, 24) = 10.47, p ≤ 0.001] proved significant. Whereas saline treated animals at both intervals, did not show significant differences from the first saline application (not shown), 25CN-NBOH treated mice—with only 24.98 ± 4.54% (1 h) and 19.48 ± 4.59% (1.5 h) of the original responsiveness—twitched significantly less (first vs. resp. second injection, post-hoc each p ≤ 0.01; Figure 4). As shown for the 1.5-h interval, a reduced but still highly significant loss of drug efficacy could likewise be demonstrated when 25CN-NBOH was injected twice at a lower dose (0.75 mg/kg, s.c.) to handling-habituated mice (mean ± SEM [% of the original responsiveness]: 30.66 ± 5.86; first vs. second injection, p ≤ 0.01) (Figure 4).
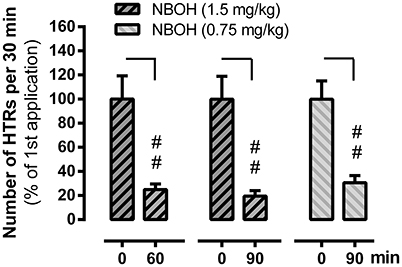
Figure 4. Tachyphylaxis of 25CN-NBOH (0.75 or 1.5 mg/kg, s.c.) induced head twitches (HTRs) (as observed for the first 30 min post-application). Agonist was applied twice at an interval of 60 and 90 min, respectively. Mean + SEM; n = 5–6 per group. Repeated measures ANOVA, Bonferroni-corrected post-hoc comparison to respective first application (0 min), ##p ≤ 01.
In addition to tachyphylaxis occurring at repeated short-interval injection, a substantial loss of responsiveness to 25CN-NBOH could also be demonstrated when the drug (1.5 mg/kg, i.p.) was repeatedly applied at 1-day intervals [main effect time: F(1.84, 27.66) = 57.51, p ≤ 0.001]; main effect group [F(2, 15) = 30.41, p ≤ 0.001]; time × group interaction [F(3.68, 27.66) = 26.52, p ≤ 0.001] (Figure 5). Unlike with the short-interval application, however, the decrease in HTRs only with the third injection of 25CN-NBOH (i.e. on the 3rd and 4th day) became significant [1x NBOH per day: Day 1 (mean ± SEM: 37.67 ± 5.99) vs. day 3 and day 4 [56.19 and 54.87% of day 1, respectively], each p ≤ 0.01]. When a second (higher) dose of the agonist (3.0 mg/kg, i.p.) was applied in the evening of the first 3 days, the onset of tolerance proved significant by day 2 already [2x NBOH per day: Day 1 (mean ± SEM: 61.5 ± 6.34) vs. day 2, day 3, and day 4 (34.15, 18.69, and 17.88% of day 1, respectively), each p ≤ 0.001]. Also, the extent of tolerance was more pronounced. Whereas the HTRs exhibited by the once-per-day-application mice on day 4 still were statistically different from the saline-treated control animals (mean ± SEM: 20.67 ± 3.49, p ≤ 0.001), those in the twice-per-day mice more closely approached the control level (mean ± SEM: 11.00 ± 1.73, n.s.) (Figure 5).
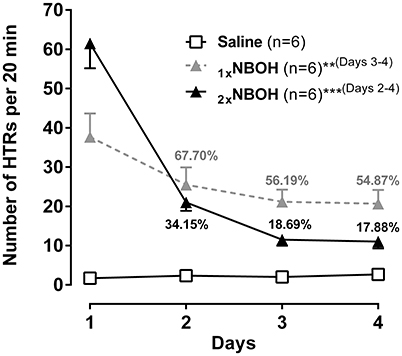
Figure 5. Subchronic tolerance to 25CN-NBOH induced head twitches (HTRs), with a total of four [1xNBOH: Once per day, 1.5 mg/kg (morning)] and seven [2xNBOH: Twice per day, 1.5 mg/kg (morning) vs. 3.0 mg/kg (evening)] i.p. injections, respectively. Head twitches were counted for the first 20 min after the morning application). Percent values indicate percent of HTRs relative to respective first day. Mean ± SEM; n = 6 per group. Repeated measures ANOVA, Bonferroni-corrected post-hoc comparison to respective first-day measurement, **p ≤ 01, ***p ≤ 001 (brackets behind asterisks indicate interval(s) significance applied to).
Discussion
We characterized the selective 5-HT2A agonist 25CN-NBOH in terms of HTRs, a behavioral output suggested to engage (Willins and Meltzer, 1997; González-Maeso et al., 2007), predict and/or model 5-HT2A receptor function in the cortex (Goodwin et al., 1984; Zhang and Marek, 2008; e.g., Buchborn et al., 2015).
25CN-NBOH evoked marked HTRs in mice at the full range of doses tested (i.e., 0.5–3.0 mg/kg). In the context of the multiple-comparisons analysis, however, only the medium dose (1.5 mg/kg) turned out significant, which mirrors previous findings of the drug being most active at around 1.0–3.0 mg/kg (Fantegrossi et al., 2015; Halberstadt et al., 2016). The inverted u-shape-like dose-response curve of 25CN-NBOH (with higher doses entailing a relative loss of responsiveness) might be due to increasing occupancy of 5-HT2C receptors (whose activity may counteract the 5-HT2A–mediated HTR induction), and/or due to 5-HT2 receptors requiring a tuned level of occupancy (compare for DOM, DOB, and DOI: Fantegrossi et al., 2010; Serafine and France, 2014; Buchborn et al., 2015). Consistent with its sensitivity to MDL100907 (Fantegrossi et al., 2015), one of the most selective 5-HT2A antagonists at present (av. Ki [Ki database]: ~1.92 nM for r5-HT2A; ~112 nM for r5-HT2C), 25CN-NBOH (1.5 mg/kg, i.p.) induced HTRs were blocked by the 5-HT2A antagonist ketanserin (av. Ki [Ki database]: ~1.6 nM for r5-HT2A; ~52 nM for r5-HT2C). The selective 5-HT2C antagonist SB-242084 (av. Ki [Ki database]: ~2.85 for h5-HT2C; ~505 nM for h5-HT2A), on the other hand, did not affect HTRs evoked by a 1.5-mg/kg dose of 25CN-NBOH. Interstingly, it slightly increased the behavioral effect of the higher 3.0-mg/kg dose of the agonist. Thus, it might be suggested that low to medium doses of 25CN-NBOH primarily interact with 5-HT2A receptors; but higher doses of 25CN-NBOH may also recruit 5-HT2C receptors, thereby interfering with the actions mediated by 5-HT2A receptors (compare for DOI: Fantegrossi et al., 2010). The finding that 3.0 mg/kg 25CN-NBOH given to mice reach brain concentrations that fall into the range of the agonist's in vitro affinity for 5-HT2C receptors (Jensen et al., 2017), would be in line with such an interpretation.
25CN-NBOH induced HTRs were facilitated when animals had been thoroughly habituated to the experimental procedures on the day before the drug application. The latter finding supports observations made in rats, where the presence of familiar littermates allowed hallucinogens to evoke substantially more HTRs than when observed in isolation (Buchborn et al., 2015). The dependence of 5-HT2A related behavior in rodents on what may be described as state-environment interaction, mirrors characteristics of human psychedelia, which is critically determined by set and setting, i.e., psychological constitution and environmental circumstances (rev. Hartogsohn, 2016).
25CN-NBOH induced HTRs occurred within <1 min after i.p. injection, reached maximal frequencies within 2–5 min, and declined to half-maximal at ~11 min (compare for 25I-NBOMe and 25I-NBMD: Halberstadt and Geyer, 2014). In contrast to the i.p. route, s.c. administration—as shown for the 1.5-mg/kg dose—was associated with a slight delay in peak and somewhat longer duration to half-maximum. 25CN-NBOH, both in plasma and brain, reaches maximum concentrations within 15 min, where it remains relatively stable until at least 30 min post-application (Jensen et al., 2017). The fast decline in the behavioral response observed here therefore does not seem to be a consequence of fast drug clearance. One possible explanation for the apparent disconnect between 25CN-NBOH's brain concentration and behavioral output is the development of acute tolerance. Indeed, DOI which shares the phenethylamine backbone of 25CN-NBOH, internalizes and desensitizes 5-HT2A receptors within 15–20 min in vitro (Porter et al., 2001; Raote et al., 2013).
To follow up on the possibility of tolerance development, we applied 25CN-NBOH (1.5 mg/kg) twice at a 1.0- or a 1.5-h interval and found a rapid loss of responsiveness to the second injection (compare for LSD and DOI: Darmani and Gerdes, 1995; Buchborn et al., 2016). To test the hypothesis that the observed tachyphylaxis was due to substance accumulation (i.e., two single 1.5 mg/kg doses accumulating to a less active 3.0-mg/kg dose), we repeated the experiment injecting a 0.75-mg/kg dose (which after thorough animal habituation induced significant HTRs; see Figure 1A) twice at a 1.5-h interval. If the two 0.75 mg/kg doses had accumulated to form a 1.5-mg/kg dose, the second dose should have induced unchanged or more HTRs than the first. However, as the 0.75-mg/kg regimen led to a similar-degree tachyphylaxis as did the 1.5-mg/kg dose, it is unlikely that drug accumulation is the underlying cause for the rapid tolerance development. Serotonergic hallucinogens have been shown to affect locomotor activity in rodents (e.g., Cohen and Wakeley, 1968; Kabes et al., 1972; Wing et al., 1990), with 5-HT2A activation in mice promoting hyperlocomotion (Halberstadt et al., 2009). 25CN-NBOH administration (1.5 mg/kg), in line with the latter, during the 1.5-h observation increased the distance traveled as well as the overall mobility duration. However, average speed of movement was unchanged. Also, 25CN-NBOH-treated mice (like the control animals) overall spent significantly less time mobile than immobile. Therefore, it appears unlikely that physical exhaustion caused the observed tachyphylaxis to 25CN-NBOH. Instead processes of 5-HT2A regulation might play a role; indeed, DOI applied twice at a 4-h interval in vivo, desensitizes 5-HT2A mediated glutamate release in the cortex (Scruggs et al., 2003). Finally, we investigated subchronic tolerance as it would develop with a once-a-day application of 25CN-NBOH (1.5 mg/kg, i.p.) over 4 days. HTRs steadily decreased (e.g., compare for DOI and 2C-T-7: Smith et al., 2014), with the most pronounced loss of responsiveness exhibited in between the first days. The extent of tolerance could be substantially augmented by applying a second (higher) daily dose of 25CN-NBOH (3.0 mg/kg, i.p.) at an ~8-h interval.
In summary, our data demonstrate that the selective 5-HT2A agonist 25CN-NBOH induces a dose- and time-dependent, ketanserin-sensitive increase in HTRs in mice, which is subject to short-interval and subchronic tolerance. Future research, correlating HTRs with pharmacokinetic-/dynamic adaptations at various time points during tolerance development might provide insight as to a possible mechanism. Furthermore, making use of new technologies to image cortical activities in awake behaving mice using genetically encoded, cell-class specifically targeted voltage and calcium indicators (Carandini et al., 2015; Knöpfel et al., 2015; Song et al., 2017) might take our mechanistic understanding of the underlying processes from basic receptor pharmacology to systemic circuit dynamics.
Author Contributions
TB, TL, and TK: have conceptualized the study design; TB and TL: have collected and plotted the data; TB, TL, and TK: have interpreted the data and written the manuscript.
Funding
This study was supported by the European Commission (TB, TK), funds provided by Imperial College (TK), NIH (TK), and by MRC (TL).
Conflict of Interest Statement
The authors declare that the research was conducted in the absence of any commercial or financial relationships that could be construed as a potential conflict of interest.
Acknowledgments
We would like to thank Jesper L. Kristensen, University of Copenhagen, for a kind gift of 25CN-NBOH. We would like to thank Chenchen Song for advice and supervision, Gemma Oliver for help with animal husbandry, and all members of the Knöpfel lab for critical comments and encouragement. We would also like to thank David Nutt and his group members for advice and inspiration.
Supplementary Material
The Supplementary Material for this article can be found online at: https://www.frontiersin.org/articles/10.3389/fphar.2018.00017/full#supplementary-material
Supplementary Movie 1. Head twitch response (HTR) induced by a 1.5-mg/kg i.p. dose of 25CN-NBOH (recorded at 100 Hz). The arrow indicates on- and offset of the head twitch at ~13 s. The twitch is recapitulated by a slow-motion picture-in-picture replay on the right side of the video. Format: Mp4, H.264+AAC, 720p. Duration: ~20 s.
References
Buchborn, T., Grecksch, G., Dieterich, D. C., and Höllt, V. (2016). “Tolerance to lysergic acid diethylamide: overview, correlates, and clinical implications,” in Neuropathology of Drug Addictions and Substance Misuse, ed V. R. Preedy (San Diego, CA: Academic Press), 846–858.
Buchborn, T., Schröder, H., Dieterich, D. C., Grecksch, G., and Höllt, V. (2015). Tolerance to LSD and DOB induced shaking behaviour: dIfferential adaptations of frontocortical 5-HT 2A and glutamate receptor binding sites. Behav. Brain Res. 281, 62–68. doi: 10.1016/j.bbr.2014.12.014
Buchborn, T., Schröder, H., Höllt, V., and Grecksch, G. (2014). Repeated lysergic acid diethylamide in an animal model of depression: normalisation of learning behaviour and hippocampal serotonin 5-HT2 signalling. J. Psychopharmacol. 28, 545–552. doi: 10.1177/0269881114531666
Carandini, M., Shimaoka, D., Rossi, L. F., Sato, T. K., Benucci, A., and Knöpfel, T. (2015). Imaging the awake visual cortex with a genetically encoded voltage indicator. J. Neurosci. 35, 53–63. doi: 10.1523/JNEUROSCI.0594-14.2015
Carhart-Harris, R. L., Bolstridge, M., Rucker, J., Day, C. M., Erritzoe, D., Kaelen, M., et al. (2016). Psilocybin with psychological support for treatment-resistant depression: an open-label feasibility study. Lancet Psychiatry 3, 619–627. doi: 10.1016/S2215-0366(16)30065-7
Carhart-Harris, R. L., and Goodwin, G. M. (2017). The Therapeutic potential of psychedelic drugs: past, present, and future. Neuropsychopharmacology 42, 2105–2113. doi: 10.1038/npp.2017.84
Cohen, M., and Wakeley, H. (1968). A comparative behavioral study of ditran and LSD in mice, rats, and dogs. Arch. Int. Pharmacodyn. Ther. 173:316.
Darmani, N. A., and Gerdes, C. F. (1995). Temporal differential adaptation of head-twitch and ear-scratch responses following administration of challenge doses of DOI. Pharmacol. Biochem. Behav. 50, 545–550. doi: 10.1016/0091-3057(94)00340-8
Fantegrossi, W. E., Simoneau, J., Cohen, M. S., Zimmerman, S. M., Henson, C. M., Rice, K. C., et al. (2010). Interaction of 5-HT2A and 5-HT2C Receptors in R (-)-2,5-Dimethoxy-4-iodoamphetamine-elicited head twitch behavior in mice. J. Pharmacol. Exp. Ther. 335, 728–734. doi: 10.1124/jpet.110.172247
Fantegrossi, W. E., Gray, B. W., Bailey, J. M., Smith, D. A., Hansen, M., and Kristensen, J. L. (2015). Hallucinogen-like effects of 2-([2-(4-cyano-2,5-dimethoxyphenyl) ethylamino]methyl)phenol (25CN-NBOH), a novel N-benzylphenethylamine with 100-fold selectivity for 5-HT(2)A receptors, in mice. Psychopharmacology 232, 1039–1047. doi: 10.1007/s00213-014-3739-3
González-Maeso, J., Weisstaub, N. V., Zhou, M., Chan, P., Ivic, L., Ang, R., et al. (2007). Hallucinogens recruit specific cortical 5-HT 2A receptor-mediated signaling pathways to affect behavior. Neuron 53, 439–452. doi: 10.1016/j.neuron.2007.01.008
Goodwin, G. M., Green, A. R., and Johnson, P. (1984). 5-HT2 receptor characteristics in frontal cortex and 5-HT2 receptor-mediated head-twitch behaviour following antidepressant treatment to mice. Br. J. Pharmacol. 83, 235–242. doi: 10.1111/j.1476-5381.1984.tb10140.x
Halberstadt, A. L., and Geyer, M. A. (2014). Effects of the hallucinogen 2,5-dimethoxy-4-iodophenethylamine (2C-I) and superpotent N-benzyl derivatives on the head twitch response. Neuropharmacology 77, 200–207. doi: 10.1016/j.neuropharm.2013.08.025
Halberstadt, A. L., Sindhunata, I. S., Scheffers, K., Flynn, A. D., Sharp, R. F., Geyer, M. A., et al. (2016). Effect of 5-HT2A and 5-HT2C receptors on temporal discrimination by mice. Neuropharmacology 107, 364–375. doi: 10.1016/j.neuropharm.2016.03.038
Halberstadt, A. L., Van Der Heijden, I., Ruderman, M. A., Risbrough, V. B., Gingrich, J. A., Geyer, M. A., et al. (2009). 5-HT2A and 5-HT2C receptors exert opposing effects on locomotor activity in mice. Neuropsychopharmacology 34, 1958–1967. doi: 10.1038/npp.2009.29
Handley, S. L., and Singh, L. (1986). Neurotransmitters and shaking behaviour - more than a ‘gut-bath’ for the brain? Trends Pharmacol. Sci. 7, 324–328. doi: 10.1016/0165-6147(86)90371-8
Hansen, M. (2010). Design and Synthesis of Selective Serotonin Receptor Agonists for Positron Emission Tomography Imaging of the Brain. Copenhagen: Faculty of Pharmaceutical Sciences; University of Copenhagen.
Hansen, M., Phonekeo, K., Paine, J. S., Leth-Petersen, S., Begtrup, M., Bräuner-Osborne, H., et al. (2014). Synthesis and structure-activity relationships of N-benzyl phenethylamines as 5-HT2A/2C agonists. ACS Chem. Neurosci. 5, 243–249. doi: 10.1021/cn400216u
Hartogsohn, I. (2016). Set and setting, psychedelics and the placebo response: an extra-pharmacological perspective on psychopharmacology. J. Psychopharmacol. 30, 1259–1267. doi: 10.1177/0269881116677852
Jensen, A. A., McCorvy, J. D., Leth-Petersen, S., Bundgaard, C., Liebscher, G., Kenakin, T. P., et al. (2017). Detailed characterization of the in vitro pharmacological and pharmacokinetic properties of N-(2-hydroxybenzyl)-2, 5-dimethoxy-4-cyanophenylethylamine (25CN-NBOH), a highly selective and brain-penetrant 5-HT2A receptor agonist. J. Pharmacol. Exp. Ther. 361, 441–453. doi: 10.1124/jpet.117.239905
Kabes, J., Fink, Z., and Roth, Z. (1972). A new device for measuring spontaneous motor activity—effects of lysergic acid diethylamide in rats. Psychopharmacology 23, 75–85. doi: 10.1007/BF00414415
Knöpfel, T., Gallero-Salas, Y., and Song, C. (2015). Genetically encoded voltage indicators for large scale cortical imaging come of age. Curr. Opin. Chem. Biol. 27, 75–83. doi: 10.1016/j.cbpa.2015.06.006
Kraehenmann, R., Pokorny, D., Vollenweider, L., Preller, K. H., Pokorny, T., Seifritz, E., et al. (2017). Dreamlike effects of LSD on waking imagery in humans depend on serotonin 2A receptor activation. Psychopharmacology 234, 2031–2046. doi: 10.1007/s00213-017-4610-0
Mascher, E. (1967). “Psycholytic therapy: statistics and indications,” in Neuro-Psychopharmacology, eds H. Brill, J. O. Cole, P. Denker, H. Hippins, and P. B. Bradley (Amsterdam: Excerpta-Medica), 441–444.
Mirjana, C., Baviera, M., Invernizzi, R. W., and Balducci, C. (2004). The serotonin 5-HT2A receptors antagonist M100907 prevents impairment in attentional performance by NMDA receptor blockade in the rat prefrontal cortex. Neuropsychopharmacology 29, 1637–1647. doi: 10.1038/sj.npp.1300479
Muthukumaraswamy, S. D., Carhart-Harris, R. L., Moran, R. J., Brookes, M. J., Williams, T. M., Errtizoe, D., et al. (2013). Broadband cortical desynchronization underlies the human psychedelic state. J. Neurosci. 33, 15171–15183. doi: 10.1523/JNEUROSCI.2063-13.2013
Porter, R. H., Malcolm, C. S., Allen, N. H., Lamb, H., Revell, D. F., and Sheardown, M. J. (2001). Agonist-induced functional desensitization of recombinant human 5-HT2 receptors expressed in CHO-K1 cells. Biochem. Pharmacol. 62, 431–438. doi: 10.1016/S0006-2952(01)00677-3
Quesseveur, G., Nguyen, H. T., Gardier, A. M., and Guiard, B. P. (2012). 5-HT2 ligands in the treatment of anxiety and depression. Expert Opin. Investig. Drugs 21, 1701–1725. doi: 10.1517/13543784.2012.719872
Raote, I., Bhattacharyya, S., and Panicker, M. M. (2013). Functional selectivity in serotonin receptor 2A (5-HT2A) endocytosis, recycling, and phosphorylation. Mol. Pharmacol. 83, 42–50. doi: 10.1124/mol.112.078626
Ray, T. S. (2010). Psychedelics and the human receptorome. PLoS ONE 5:e9019. doi: 10.1371/annotation/e580a864-cf13-40c2-9bd9-b9687a6f0fe4
Regard, J. B., Sato, I. T., and Coughlin, S. R. (2008). Anatomical profiling of G protein-coupled receptor expression. Cell 135, 561–571. doi: 10.1016/j.cell.2008.08.040
Ross, S., Bossis, A., Guss, J., Agin-Liebes, G., Malone, T., Cohen, B., et al. (2016). Rapid and sustained symptom reduction following psilocybin treatment for anxiety and depression in patients with life-threatening cancer: a randomized controlled trial. J. Psychopharmacol. 30, 1165–1180. doi: 10.1177/0269881116675512
Roth, B. L., Lopez, E., Patel, S., and Kroeze, W. K. (2000). The multiplicity of serotonin receptors: uselessly diverse molecules or an embarrassment of riches? Neuroscientist 6, 252–262. doi: 10.1177/107385840000600408
Scruggs, J. L., Schmidt, D., and Deutch, A. Y. (2003). The hallucinogen 1-[2,5-dimethoxy-4-iodophenyl]-2-aminopropane (DOI) increases cortical extracellular glutamate levels in rats. Neurosci. Lett. 346, 137–140. doi: 10.1016/S0304-3940(03)00547-0
Serafine, K. M., and France, C. P. (2014). Restricted access to standard or high fat chow alters sensitivity of rats to the 5-HT2A/2C receptor agonist 1-(2,5-dimethoxy-4-methylphenyl)-2-aminopropane (DOM). Behav. Pharmacol. 25, 44–52. doi: 10.1097/FBP.0000000000000015
Smith, D. A., Bailey, J. M., Williams, D., and Fantegrossi, W. E. (2014). Tolerance and cross-tolerance to head twitch behavior elicited by phenethylamine-and tryptamine-derived hallucinogens in mice. J. Pharmacol. Exp. Ther. 351, 485–491. doi: 10.1124/jpet.114.219337
Song, C., Barnes, S., and Knöpfel, T. (2017). Mammalian cortical voltage imaging using genetically encoded voltage indicators: a review honoring professor Amiram Grinvald. Neurophotonics 4:031214. doi: 10.1117/1.NPh.4.3.031214
Vollenweider, F. X., and Kometer, M. (2010). The neurobiology of psychedelic drugs: implications for the treatment of mood disorders. Nat. Rev. Neurosci. 11, 642–651. doi: 10.1038/nrn2884
Weber, E. T., and Andrade, R. (2010). Htr2a gene and 5-HT2A receptor expression in the cerebral cortex studied using genetically modified mice. Front. Neurosci. 4:36. doi: 10.3389/fnins.2010.00036
Williams, G. V., Rao, S. G., and Goldman-Rakic, P. S. (2002). The physiological role of 5-HT2A receptors in working memory. J. Neurosci. 22, 2843–2854.
Willins, D. L., and Meltzer, H. Y. (1997). Direct injection of 5-HT2A receptor agonists into the medial prefrontal cortex produces a head-twitch response in rats. J. Pharmacol. Exp. Ther. 282, 699–706.
Wing, L. L., Tapson, G. S., and Geyer, M. A. (1990). 5HT-2 mediation of acute behavioral effects of hallucinogens in rats. Psychopharmacology 100, 417–425. doi: 10.1007/BF02244617
Keywords: serotonergic hallucinogen, 25CN-NBOH, 5-HT2A receptor, 5-HT2C receptor, head twitch response, tachyphylaxis, tolerance, locomotion
Citation: Buchborn T, Lyons T and Knöpfel T (2018) Tolerance and Tachyphylaxis to Head Twitches Induced by the 5-HT2A Agonist 25CN-NBOH in Mice. Front. Pharmacol. 9:17. doi: 10.3389/fphar.2018.00017
Received: 19 October 2017; Accepted: 08 January 2018;
Published: 06 February 2018.
Edited by:
Andrew Robert Gallimore, Okinawa Institute of Science and Technology, JapanReviewed by:
A Elizabeth Linder, Universidade Federal de Santa Catarina, BrazilAlfredo Meneses, Centro de Investigación y de Estudios Avanzados del Instituto Politécnico Nacional (CINVESTAV-IPN), Mexico
Copyright © 2018 Buchborn, Lyons and Knöpfel. This is an open-access article distributed under the terms of the Creative Commons Attribution License (CC BY). The use, distribution or reproduction in other forums is permitted, provided the original author(s) and the copyright owner are credited and that the original publication in this journal is cited, in accordance with accepted academic practice. No use, distribution or reproduction is permitted which does not comply with these terms.
*Correspondence: Thomas Knöpfel, dGtub3BmZWxAa25vcGZlbC1sYWIubmV0
†These authors have contributed equally to this work.