- 1Research and Innovation Centre, Fondazione Edmund Mach, San Michele all’Adige, Italy
- 2Laboratory of Phytogeography and Applied Geobotany, Section Environment and Landscape, Department of Planning, Design, and Technology of Architecture, Sapienza University of Rome, Rome, Italy
- 3Centre Agriculture Food Environment, University of Trento, Trento, Italy
During the last two decades incidences of fungal infections dramatically increased and the often accompanying failure of available antifungal therapies represents a substantial clinical problem. The urgent need for novel antimycotics called particular attention to the study of natural products. The genus Hypericum includes many species that are used in the traditional medicine to treat pathological states like inflammations and infections caused by fungi. However, despite the diffused use of Hypericum-based products the antifungal potential of the genus is still poorly investigated. In this study five Hypericum species autochthonous of Central and Eastern Europe were evaluated regarding their polyphenolic content, their toxicological safety and their antifungal potential against a broad panel of clinical fungal isolates. LC-MS analysis led to the identification and quantification of 52 compounds, revealing that Hypericum extracts are rich sources of flavonols, benzoates and cinnamates, and of flavan-3-ols. An in-depth screen of the biological activity of crude extracts clearly unveiled H. hircinum subsp. majus as a promising candidate species for the search of novel antifungals. H. hircinum is diffused in the Mediterranean basin from Spain to Turkey where it is traditionally used to prepare a herbal tea indicated for the treatment of respiratory tract disorders, several of which are caused by fungi. Noteworthy, the infusion of H. hircinum subsp. majus excreted broad antifungal activity against Penicillium, Aspergillus and non-albicans Candida isolates comprising strains both sensitive and resistant to fluconazole. Additionally, it showed no cytotoxicity on human cells and the chemical characterization of the H. hircinum subsp. majus infusion revealed high amounts of the metabolite hyperoside. These results scientifically support the traditional use of H. hircinum extracts for the treatment of respiratory tract infections and suggest the presence of exploitable antifungal principles for further investigations aimed at developing novel antifungal therapies.
Introduction
The rising incidence of fungal infections is an emerging serious health problem, especially in cases of immune compromised patients caused either by diseases like AIDS, or by cancer therapies and prolonged antibiotic treatments (Lass-Flörl, 2009; Weil et al., 2017). Estimates of global mortality rates suggest that fungi are responsible for more deaths than either tuberculosis or malaria (Brown et al., 2012). Millions more suffer from severe illness caused by chronic or allergic fungal infections. Candidiasis is the most prevalent fungal disease worldwide difficult and extremely costly to treat (World Health Organization [WHO], 2014). The available antifungal drugs like polyenes, pyrimidines, azoles, and echinocandins often provoke significant toxicity including fever, nausea and vomiting, dose-limiting nephrotoxicity, liver toxicity, and controversial interactions with co-administered drugs (Dismukes, 2000; Zager, 2000). Another crucial problem is represented by the increasing rate of drug resistance that invalidates the clinical treatments. The alarming gap in the anti-fungal pipeline makes the discovery of new active compounds a priority for the scientific community. The strong request of new classes of compounds has renewed the interest in the screening of natural products (Abreu et al., 2012; Atanasov et al., 2016). Especially, ethnobotany represents an important guide in the search of novel antifungal agents. Due to their ability to synthesize a limitless variety of chemical structures, plants have represented a source of bioactive remedies for centuries (Arif et al., 2009). Despite of this, it is estimated that only a small proportion of plants are used by humans. Therefore, bioactivity screening programs and research on new plant-derived compounds are of prime importance for the search of novel leads. Plants belonging to the genus Hypericum, are characteristic components of the European flora. In particular, Europe and the Mediterranean basin represent a hot spot of diversity for Hypericum species (Nürk and Crockett, 2011). The best characterized species is H. perforatum L., which was already described by Hippocrates as a remedy against possession in the Ancient Greece (Dugoua et al., 2006). During the centuries it has been used for the treatment of various pathological states, like neurological disorders, wounds, inflammations, and infections. The use of H. perforatum extracts is so popular that this species is considered the most important medicinal plant of the 20th century (Gurib-Fakim, 2006). The German Commission E approved its usage for several indications, including treatment and post-therapy of acute and contused injuries, myalgia and first-degree burns as well as dyspeptic complaints (Arsic et al., 2010). In the Balkan Countries it is considered as one of the oldest remedies and in the Serbian folk medicine it is indicated for the treatment of cuts, burns, hemorrhoids and also as an antiseptic, for liver and stomach complaints, diarrhea, and gastric ulcers. Its sale represents a huge part of the overall herbal market (Crockett et al., 2011), exceeding $570 million/annum worldwide (Ayan et al., 2006). Regarding its antifungal potential, inhibitory properties against Candida spp., (Tocci et al., 2013) and fungal plant pathogens (Crockett et al., 2011) have been reported. Nevertheless, a growing number of scientific evidences underlines the importance to investigate other Hypericum species that might exert a more promising antifungal activity (Radulović et al., 2007; Stojanovic et al., 2013). In this regard the species H. carinatum, H. myrianthum, H. linoides (Barros et al., 2013), H. garciae (Tocci et al., 2018a) have been shown to have the potential to inhibit the growth of Candida spp. Cryptococcus neoformans and Rhodotorula mucilaginosa growth.
In Central and Eastern Europe several different Hypericum species co-occur and the area is considered a significant source of plant material for the market of herbal products (Raclariu et al., 2017).
Amongst others, H. maculatum Crantz subsp. maculatum, H. hircinum L. s. lat., H. hirsutum L., and H. montanum L., as plants used to treat wounds, skin inflammations and burns (Menkovic et al., 2011), as well as infections of the respiratory (Sagratini et al., 2008) and urinary tract (Sarić-Kundalić et al., 2010). These pathologies are often caused by fungi, with Candida as the main causative agent and by dermatophytes in the case of external infections (Borgers et al., 2005; Johnson, 2012; Ritchie and Eltahawy, 2014). To screen for new candidates suitable for the development of novel antimycotic solutions, we studied extracts deriving from the aerial parts of several Hypericum taxa (H. perforatum L., H. maculatum Crantz subsp. maculatum, H. hircinum L. s.lat., H. hirsutum L., and H. montanum L.) with respect to their chemical profile, their antifungal activity against a broad panel of clinical fungal isolates of the human gut and their toxicological safety on human skin fibroblasts and peripheral blood mononuclear cells (PBMCs).
Materials and Methods
Plant Material
Samples of H. hirsutum subsp. hirsutum, H. hircinum subsp. majus, H. maculatum, H. montanum, and H. perforatum have been collected during spring-summer of 2015 in the Alpine region (Northern-Eastern Italy). Species were identified according to the most recent Flora of Italy (Pignatti, 1982, 2017), nomenclature follows Bartolucci et al. (2018) and type specimens were deposited in the Department of Food Quality and Nutrition at the Fondazione Edmund Mach in San Michele all’Adige, Trento, Italy (Reference number: NT0001 H. perforatum, NT0002 H. montanum, NT0003 H. maculatum, NT0004 H. hirsutum, NT0005 H. hircinum subsp. majus).
Preparation of Crude Plant Extracts
For each species, 500 mg of dried plant biomass, represented by stems and leaves, were powdered by grinding and extracted in the dark with methanol (drug/solvent ratio = 1:20 w/v) by maceration (3 × 24 h). H. hircinum subsp. majus was also subjected to extractions with boiling water (100°C) for 20 min, and ethanol 80% by maceration for 24 h. The obtained extracts were evaporated to dryness, weighed and stored at -20°C until analysis.
Preparation of Samples for Chemical Analysis
Prior to analysis methanolic extracts were resuspended in 50% methanol/water and filtered using sterile (0.2 μm) PTFE filters.
LC-MS Analysis
Analysis of phenolic metabolites was performed using a Waters Acquity UPLC system (Milford, MA, United States) which consists of a binary pump, an online vacuum degasser, an autosampler and a column compartment as previously described (Vrhovsek et al., 2012). Phenolic compounds were separated at a temperature of 40°C on a Waters Acquity HSS T3 column 1.8 μm, 150 mm × 2.1 mm (Milford, MA, United States). The mobile phase was composed of component A (0.1% formic acid in water) and component B (0.1% formic acid in acetonitrile). Acetonitrile was of LC-MS grade and was purchased from Sigma–Aldrich (St. Louis, MO, United States). Pure standards of hypericin from Hypericum perforatum ∼95% (HPLC), hyperoside and hyperforin were purchased from Sigma-Aldrich (St. Louis, MO, United States). Milli-Q water was used for the chromatography. The flow was set to 0.4 mL/min, and the gradient profile was: 0 min, 5% B; from 0 to 3 min, linear gradient to 20% B; from 3 to 4.3 min, isocratic 20% B; from 4.3 to 9 min, linear gradient to 45% B; from 9 to 11 min, linear gradient to 100% B; from 11 to 13 min, wash at 100% B; from 13.01 to 15 min, the re-equilibrated to the initial conditions of 5% B. Analysis of hypericin, hyperforin and hyperoside was performed using the same Waters Acquity UPLC system (Milford, MA, United States) and conditions, by means of a different gradient. The gradient profile in this case was: 0 min, 10% B; from 0 to 4,5 min, linear gradient to 100% B; from 4,5 to 12 min, isocratic 100% B; finally the column was re-equilibrated to the initial conditions of 10% B for 4 min. MRM transitions for hypericin, hyperforin and hyperoside are reported in Supplementary Table S1. Each sample was analyzed in triplicate. Samples were kept at 6°C during the analysis. Mass spectrometry detection for both methods was performed on a Waters Xevo TQMS (Milford, MA, United States) instrument equipped with an electrospray (ESI) source. Capillary voltage was 3.5 kV in positive mode and -2.5 kV in negative mode; the source was kept at 150°C; the desolvation temperature was 500°C; cone gas flow, 50 L/h; and desolvation gas flow, 800 L/h. Further MS parameters are reported in Vrhovsek et al., 2012.
Data Analysis
Quantification was done using Waters MassLynx 4.1 and TargetLynx software.
Microorganisms and Media
For antifungal susceptibility testing, the reference strain C. albicans ATCC MYA-2876 from the American Type Culture Collection (ATCC), Rockville, MD, United States was used. The human gut clinical isolates, C. albicans YN7, C. parapsilosis YB1, C. parapsilosis YB3 (Di Paola et al., unpublished), C. albicans YHS254, C. albicans YHS89, C. lusitaniae YHS217, C. lusitaniae YHS72, C. parapsilosis YHS133, C. parapsilosis YHS312, C. parapsilosis YHS301, Aspergillus glaucus YHS165, Penicillium paneum YHS245 (Strati et al., 2016), C. glabrata MFB004, C. tropicalis MFB035-1, C. tropicalis RTT037-3 (Strati et al., unpublished) were tested for their susceptibility against the applied drugs. All strains were grown on Sabouraud agarized medium for 48 h at 30°C before testing.
Antifungal Susceptibility Testing
Candida strains were tested for their susceptibility to Hypericum spp. extracts (7 dilution series, ranging from 500 to 8 μg/ml) following the European Committee for Antimicrobial Susceptibility Testing protocol (EUCAST DEFINITIVE DOCUMENT EDef 7.2 Revision; European Committee for Antimicrobial Susceptibility Testing, 2012). Briefly, cells were grown in RPMI1640 medium supplied with 2.0% glucose, counted and inoculated at a concentration of 1–5 × 105 CFU/ml. MIC50 and MIC90 values were determined at 530 nm using a spectrophotometer, as the lowest concentration of the drug that resulted in ≥50% and ≥90% inhibition of growth relative to the growth control after 48 h incubation.
PBMC Isolation
Peripheral blood mononuclear cells were obtained from buffy coat of healthy donors (as approved by the Ethical Committee of the local health centre, Azienda Provinciale per I Servizi Sanitari, Provincia Autonoma di Trento) within 4 h from sample collection. PBMC isolation was performed using Lympholite (CEDARLANE) density gradient (1.077 g/liter, pH 6,9), according to manufacturer’s instructions, and incubated in complete nutrient RPMI 1640 medium (Euroclone) supplemented with glutamine (2 mol/L), 10% fetal calf serum (FCS) and antibiotics (10000 U/ml Penicillin, 10000 μg/ml Streptomycin) (Biological Industries), at 37°C and 5% CO2.
Cytotoxicity Assay
The cytotoxicity of H. hircinum subsp. majus infusion, methanolic and hydro-alcoholic extracts was tested using the WST-8 (4-[3-(2-methoxy-4-nitrophenyl)-2-(4-nitrophenyl)-2H-5-tetrazolio]-1,3-benzene disulfonate sodium salt) conversion assay. The cytotoxicity of the extracts was evaluated on two normal cell lines, namely PBMC and human foreskin fibroblast Hs27. Cells were seeded in 96-well culture plates at concentration of 5000 cells/well and incubated for 24 h before treatment with H. hircinum subsp. majus extracts. Plant extracts were diluted in DMSO and added to a final concentration of 500 μg/ml (final volume of DMSO 0,5% v/v). To measure the cytotoxic effects 10 μl of Cell Counting Kit-8 (Sigma-Aldrich) (containing the WST-8 solution) were added to each well and incubated for 2–4 h for fibroblasts and PBMCs, respectively, at 37°C before reading the absorbance at 450 nm.
The results were analyzed by Student’s t-test. The data are expressed as the percentage of viability and standard error of the mean.
Microscopy
To estimate the impact of plant extracts on cell morphology and health, cultures were visualized and recorded using an inverted microscope Motic AE31 equipped with a camera Moticam 580 5.0 MP.
Results
Extract Composition
The extracts have been chemically characterized by the identification and quantification of 52 low molecular weight polyphenols belonging to the classes: flavonols, benzoates and cinnamates, chalcones, flavan-3-ols, flavones, stilbens, coumarins, phloroglucinols, and naphtodianthrones (Table 1). LC-MS analysis revealed that species were characterized by different content of the detected metabolites, yet in all of the extracts, quercetin derivatives represented the most prevalent group of compounds.
Quercetin and quercetin glycosides ranged from 5,21 mg/g in H. montanum to 9,4 mg/g in H. hircinum subsp. majus, with hyperoside being the major compounds in the extracts representing the 45,48% of the detected compounds in H. perforatum and more than the 99% in the other Hypericum species. Quercetin-3-glucoside was abundant in H. maculatum and in H. montanum, while quercetin 3-rhamnoside was highly present in H. perforatum (132,9 μg/g). Quercetin-3-glucuronide only occurred in H. hircinum subsp. majus (28,8 μg/g) and in H. perforatum (10-fold less concentrated). Chlorogenic acid derivatives (chlorogenic acid, neochlorogenic acid, and cryptochlorogenic acid) ranged from 38 μg/g for H. hirsutum and H. perforatum to 456,7 μg/g in H. hircinum subsp. majus. Also the flavan-3-ols were relatively abundant ranging from 53,12 μg/g in H. hircinum subsp. majus to 100,12 μg/g in H. maculatum, with epicatechin being the principal compound of this class (from 19 μg/g in H. hircinum subsp. majus up to 68,5 μg/g in H. maculatum).
Antifungal Activity of Hypericum Crude Extracts
Hypericum extracts were screened against a panel of clinical Candida isolates (Table 2 and Supplementary Table S2) and the results are hereafter given as GM of MIC values. The screening unveiled that H. hircinum subsp. majus crude methanolic extract had a strong anti-Candida activity against all tested fungal strains, with inhibition properties against C. parapsilosis (MIC50 53,5 μg/ml) in the same magnitude of fluconazole (MIC50 22,63 μg/ml).
All extracts exerted antifungal properties against C. albicans and C. lusitaniae while only H. hircinum subsp. majus was also active against C. tropicalis and C. glabrata (MIC50 353,55 and 16 μg/ml, respectively).
Also, with respect to the to the MIC90 values, H. hircinum subsp. majus extract showed higher inhibitory properties than the other tested extracts (Supplementary Table S2).
Antifungal Activity of H. hircinum subsp. majus Extracts
The antifungal activity of H. hircinum subsp. majus was further investigated against a broader panel of Candida, including fluconazole resistant strains, and other fungi (Table 3 and Supplementary Table S3). In addition to crude methanolic extract, infusion and hydroalcoholic extracts have been tested. Notably, all H. hircinum subsp. majus extracts showed antifungal properties and, in particular a remarkable activity against fluconazole resistant C. parapsilosis and C. tropicalis strains. The methanolic extract showed the most pronounced cytotoxic properties. Yet, in general the antifungal activity of the infusion outcompeted those of the hydroalcoholic extract, also with respect to the MIC90 values.
Cytotoxicity of H. hircinum subsp. majus Extracts
The cytototoxicity of H. hircinum subsp. majus extracts was tested on PBMC and human skin fibroblast Hs27 cell lines by using the WST-8 conversion assay. The extracts were tested at a dose of 500 μg/ml, which corresponds to a 1- (for C. tropicalis) to 10-fold higher concentration than those showing inhibition of 50% of Candida growth. As shown in Figure 1, only the plant infusion showed no cytotoxic effect on both human cell lines, while treatment with methanolic and hydroalcoholic extracts inhibited cell viability by 50% for Hs27 cell line and by 65–70% for PBMCs.
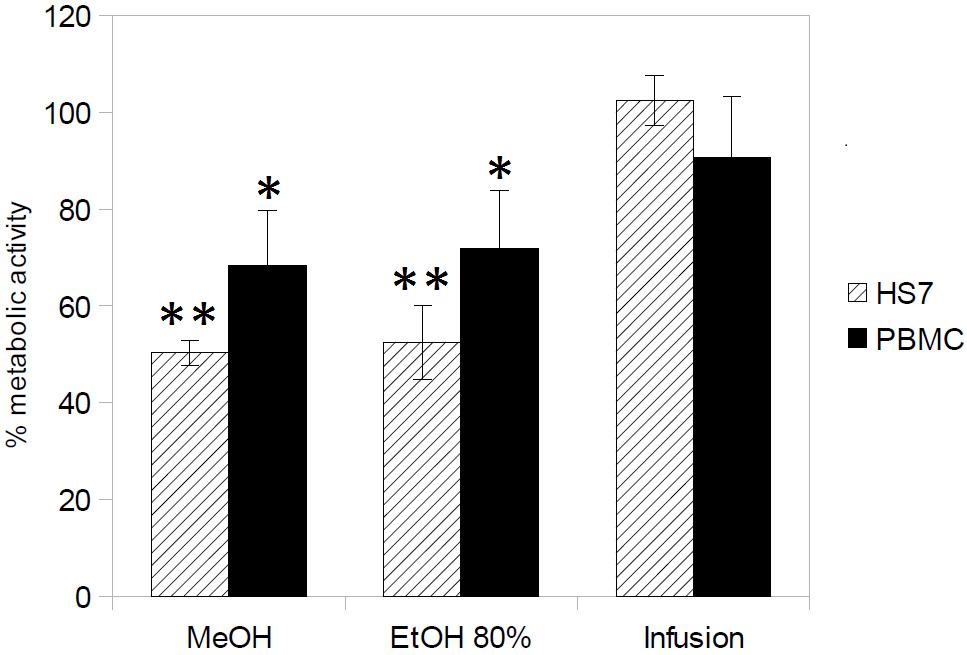
FIGURE 1. Cytotoxicity of H. hircinum subsp. majus extracts (measured using the WST-8 conversion assay). The metabolic activity is given as the endpoint of toxicity in human peripheral mononucleate cells (PBMC) and human skin fibroblast cell line (Hs27), following 24 h exposure to H. hircinum subsp. majus extracts. Values represent standard error of the mean (n = 3); Asterisks denote statistically significant differences from control (∗p < 0.05 and ∗∗p < 0.01).
The effect of H. hircinum subsp. majus on human cells was also observed microscopically (Figure 2). The imagines confirmed the cytotoxicity data. Fibroblast treated with plant infusion showed a normal phenotype, while under treatment with methanolic and hydro-alcoholic extracts the cell morphology was markedly altered. The same alteration was observed for PBMCs (data not shown).
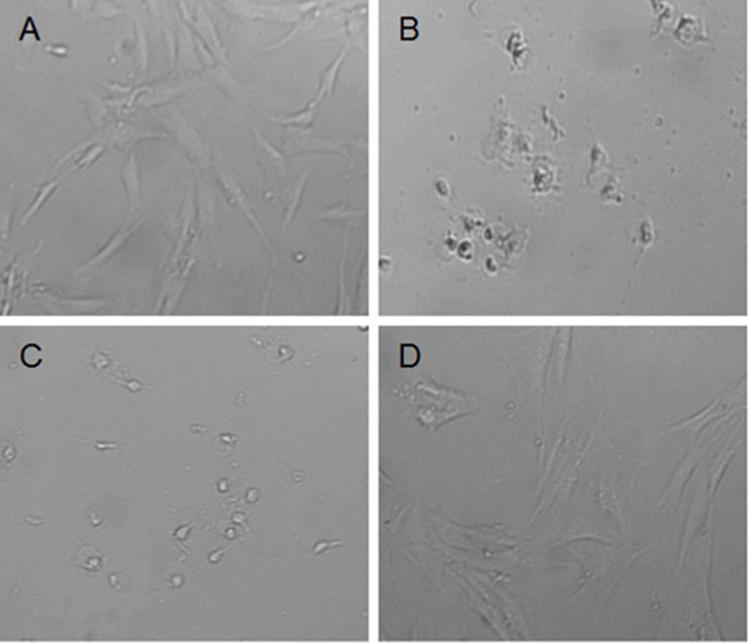
FIGURE 2. Morphology change of normal fibroblast Hs27 after 24 h treatment with H. hircinum subsp. majus extracts (observed under inverted microscope, magnification 100×): (A) control (DMSO), (B) methanolic extract, (C) hydroalcoholic extract, and (D) infusion.
Chemical Composition of H. hircinum susbps. majus Infusion
The chemical composition of the aqueous extract of H. hircinum subsp. majus is reported in Table 4. The analysis revealed that the infusion process allowed the extraction of higher amounts of phenols in comparison to the extraction by maceration in methanol. The aqueous extract was mainly characterized by derivatives of quercetin (24,18 mg/g), of chlorogenic acid (4,51 mg/g) and by flavan-3-ols (2,26 mg/g). Hyperoside was the most abundant compound, representing the 2,23% of the extract. Abundant were also quercetin-3-glucoside (1,65 mg/g), neochlorogenic acid (2,61 mg/g), chlorogenic acid (1,68 mg/g), procyanidin B2 (0,89 mg/g), epicatechin (0,59 mg/g) and procyanidin B1(0,40 mg/g).
Discussion
Plant-based treatments of the traditional medicine could bring forth new solutions for antifungal therapies. In particular polyphenol-rich plants represent an import source of bioactive products for human health (Marchelak et al., 2017) including antifungal treatments. Among polyphenols, flavan-3-ols, flavonols and tannins show a high antimicrobial potential (Daglia, 2012). Flavan-3-ols isolated from green and black tea exert important anti-Candida activity (Hirasawa and Takada, 2004; Sitheeque et al., 2009), ellagitannins obtained from Punica granatum inhibit the growth of plant pathogenic fungi (Glazer et al., 2012), the flavonols myricitrin and fisetin are considered effective agents against Candida glabrata and Cryptococcus neoformans, respectively (Salazar-Aranda et al., 2015; Reis et al., 2016). The mechanisms of action of phenols against Candida have been intensively studied and include inactivation of enzyme production (Evensen and Braun, 2009) and anti-biofilm effects (Evensen and Braun, 2009; Shahzad et al., 2014). Species belonging to the Hypericum genus are able to synthesize a broad array of such bioactive phenolic compounds, mainly as products of the polyketide metabolism (Tocci et al., 2018b), and therefore might contain novel antifungal remedies.
Here we report new data on the inhibitory activity of extracts deriving from H. montanum, H. maculatum, H. hirsutum, and H. hircinum subsp. majus and additional data on the activity of H. perforatum on the growth of clinical isolates of Candida spp. The crude extracts obtained from H. maculatum, H. montanum, H. hirsutum, and H. perforatum showed a moderate antifungal activity against C. albicans, in the range of 250–500 μg/ml, good activity against C. lusitaniae (64–125 μg/ml) and no or only weak activity against C. parapsilosis, C. tropicalis and C. glabrata. Our data are in accordance with those of Radulović et al., 2007, showing that extracts of H. hirsutum, H. maculatum, and H. perforatum exerted comparable antifungal activity against C. albicans using the disk diffusion method assay. Discordant data are reported in Cecchini et al., 2007 which found no activity of extracts obtained from H. hirsutum or H. montanum against C. albicans. Most interesting, the antifungal screening of crude extracts unveiled the promising anti-Candida properties of H. hircinum subsp. majus. The test showed that extracts obtained from this species are able to inhibit 50% of growth of C. albicans at concentrations that are fourfold to eightfold lower than other Hypericum extracts and with a more pronounced activity against C. parapsilosis and C. lusitaniae. Moreover H. hircinum methanolic extract was the only treatment able to inhibit the growth of C. tropicalis and C. glabrata. These results are important especially in the light of the increasing incidence of infections caused by emerging Candida pathogens like C. glabrata, C. parapsilosis, C. tropicalis, and C. krusei, which are less susceptible to the commonly used antifungal drugs (Tortorano et al., 2006; Papon et al., 2013). Previous data on the antifungal activity of H. hircinum are scarce and limited to susceptibility tests against just a single reference strain of C. albicans. Using the disk diffusion test method Pistelli et al., 2000 found no antifungal activity against the tested strain, while Cecchini et al., 2007 reported a higher activity of H. hircinum subsp. majus in comparison to other Hypericum species.
From a chemical point of view, the extracts applied as treatments in our tests have been deeply characterized by investigating the presence of 52 phenolic compounds. In general, the most abundant classes of detected compounds were flavonols, benzoate and cinnamates (chlorogenic acid and neochlorogenic acid), and flavan-3-ols. The major metabolite in all the extracts was the flavonol hyperoside, ranging from 4940 ± 66,3 μg/g in H. montanum to 9306 ± 385 μg/g in H. hircinum subsp. majus, and very abundant were the neochlorogenic acid and the flavan-3-ol epicatechin. Hyperoside is known for its anticancer, anti-inflammatory and antimicrobial properties (Ogawa et al., 2014). The extract of H. hircinum subsp. majus, showed several chemical peculiarities. It contained the highest amount of hyperoside, higher amounts of chlorogenic acid, quercetin-3-glucuronide, kampferol-3-glucuronide, catechin, epigallogatechin, gallocatechin, and no traces of the phloroglucinol hyperforin and of the naphtodianthrone hypericin.
In the light of its pronounced growth inhibition properties against Candida spp. and considering the ethnobotanical knowledge available for this species, H. hircinum subsp. majus has been further subjected to a deeper antifungal screen. H. hircinum is a shrub native to the areas of the Mediterranean Basin comprising Greece and Turkey (Euro+Med, 2006). The infusion of its aerial part is applied in the traditional medicine to treat chronic catarrhal affections, asthma, sore throats and cough (Pieroni et al., 2004). Recent scientific evidences indicate that fungi of the phyla Basidiomycetes and Ascomycetes (e.g., Bjerkandera, Aspergillus, Candida, and Saccharomyces) are causative agents of these pathologies (Raza et al., 2017).
To investigate whether the beneficial effect of H. hircinum treatments may be attributed to its antifungal activity, we studied, for the first time, the activity of extracts obtained from the aerial parts of the plant with a hydroalcoholic solution and, in accordance with the traditional preparations, by infusion in boiling water. Additionally, we characterized the chemical profile. The assay was performed on a broad panel of clinical Candida strains, a strain of Aspergillus glaucus and a strain of Penicillium paneum (Table 3 and Supplementary Table S3). The extract obtained from the herbal tea inhibited 50% of the growth of all tested fungi, showing strong activity against both fluconazole sensitive and resistant Candida strains and moderate activity against Aspergillus and Penicillium strains. The chemical profile revealed an enrichment in the polyphenol content in comparison with the crude methanolic extract. Particularly abundant (content higher than 40 μg/g extract) were caffeic acid, chlorogenic, neochlorogenic and cryptochlorogenic acid, quercetin, quercetin-3-glucoside, quercetin-3-glucuronide, kaempferol-3-glucuronide, all the flavan-3-ols investigated and extraordinary high levels of hyperoside were detected. Even if the bioactivity may be due to the synergistic action of the phytocomplex, it appears reasonable to exclude caffeic acid, chlorogenic acid, quercetin-3-glucuronide, kaempferol-3-glucuronide, and gallocatechin as secondary metabolites to which the antifungal activity may be attributed, since these compounds are not present in the other Hypericum extracts showing growth inhibitory properties on Candida strains. Also, cryptochlorogenic acid, neochlorogenic acid quercetin, quercetin-3-glucoside, epicatechin, have been detected in all the crude extract, but they were less present in H. hircinum subsp. majus and thus their presence cannot be considered correlated to the bioactivity. According to our data a positive correlation between the amount of catechin, epigallocatechin, procyanidin B1 and hyperoside and the antifungal activity exerted can be individuated. The anti-Candida properties of flavan-3-ols, and in particular of catechin and epigallocatechin, are well known and documented (Hirasawa and Takada, 2004; Saito et al., 2013). Nevertheless, our data suggest that the hyperoside, being detected in such high amounts in the extract obtained by the infusion of H. hircinum subsp. majus in water, may play a fundamental role in the observed fungal growth inhibition properties. The hypothesis is supported by studies proposing hyperoside as a lead for the development of new fungicides (Li et al., 2005).
Finally, the aqueous extract of H. hircinum subsp. majus showed another promising characteristic exploitable for the development of a new antifungal therapy. When tested at high concentrations on human PBMCs and human skin fibroblast Hs27cell lines, no cytotoxicity was detected.
Conclusion
Our work reports a comprehensive phenolic profile of five European Hypericum species, highlighting the presence of important exploitable bioactive metabolites. The infusion of H. hircinum subsp. majus used in the popular medicine for the treatment of respiratory tract affections has been chemically and biologically investigated for the first time. The chemical analysis unveiled a new source of the bioactive metabolite hyperoside that might be involved in the observed bioactivity of the extract. The biological tests suggest that the beneficial effects of the traditional remedies based on H. hircinum could be attributed to its antiseptic, and in particular to its antifungal properties against fungal pathogens of the respiratory tract. Moreover, the lack of cytotoxicity indicates the presence of exploitable and promising antimycotic principles for the development of an antifungal therapy with limited cytotoxic side effects. Hence it encourages future studies to assess the effective therapeutic properties of H. hircinum subsp. majus with particular attention to the treatment of fungal-associated pathologies of the respiratory tract.
Ethics Statement
PBMCs were obtained from buffy coats prepared from blood donations collected at the Centro Trasfusionale c/o Ospedale Santa Chiara, Trento, after donor’s signed informed consent. Study procedures were approved by the Comitato Etico per le Sperimentazioni Cliniche, Azienda Provinciale per i Servizi Sanitari di Trento on the 12th February 2015.
Author Contributions
NT, TW, and FM conceived the study and wrote the manuscript with contributions from all authors. NT, DP, FF, and TW performed the experiments. DI identified the species. NT, DP, and TW analyzed the data. NT drafted the manuscript. All authors approved the final manuscript.
Funding
This work was supported by the Project ADP 2016, funded by the Autonomous Province of Trento, Italy.
Conflict of Interest Statement
The authors declare that the research was conducted in the absence of any commercial or financial relationships that could be construed as a potential conflict of interest.
The reviewer LA declared a shared affiliation, though no other collaboration, with one of the authors DI to the handling Editor.
Supplementary Material
The Supplementary Material for this article can be found online at: https://www.frontiersin.org/articles/10.3389/fphar.2018.00382/full#supplementary-material
Abbreviations
CFU, colony forming units; GM, geometric mean; LC-MS, liquid chromatography-mass spectrometry; MIC, minimal inhibitory concentration.
References
Abreu, A. C., McBain, A. J., and Simões, M. (2012). Plants as sources of new antimicrobials and resistance-modifying agents. Nat. Prod. Rep. 29, 1007–1021. doi: 10.1039/c2np20035j
Arif, T., Bhosale, J. D., Kumar, N., Mandal, T. K., Bendre, R. S., Lavekar, G. S., et al. (2009). Natural products–antifungal agents derived from plants. J. Asian Nat. Prod. Res. 11, 621–638. doi: 10.1080/10286020902942350
Arsic, I., Žugic, A., Antic, D. R., Zdunic, G., Dekanski, D., Markovic, G., et al. (2010). Hypericum perforatum L. Hypericaceae/Guttiferae sunflower, olive and palm oil extracts attenuate cold restraint stress - induced gastric lesions. Molecules 15, 6688–6698. doi: 10.3390/molecules15106688
Atanasov, A. G., Waltenberger, B., Eva-Maria Pferschy-Wenzig, T. L., Wawrosch, C., Uhrin, P., Temml, V., et al. (2016). Discovery and resupply of pharmacologically active plant- derived natural products: a review. Biotechnol. Adv. 33, 1582–1614. doi: 10.1016/j.biotechadv.2015.08.001.Discovery
Ayan, A. K., Kizilkaya, R., Cirak, C., and Kevseroglu, K. (2006). Heavy metal contents of St. John’s wort (Hypericum perforatum L.) Growing in Northern Turkey. J. Plant Sci. 1, 182–186. doi: 10.3923/jps.2006.182.186
Barros, F. M., Pippi, B., Dresch, R. R., Dauber, B., Luciano, S. C., Apel, M. A., et al. (2013). Antifungal and antichemotactic activities and quantification of phenolic compounds in lipophilic extracts of Hypericum spp. native to South Brazil. Ind. Crops Prod. 44, 294–299. doi: 10.1016/j.indcrop.2012.11.017
Bartolucci, F., Peruzzi, L., Galasso, G., Albano, A., Alessandrini, A., Ardenghi, N. M. G., et al. (2018). An updated checklist of the vascular flora native to Italy. Plant Biosyst. 152, 179–303. doi: 10.1080/11263504.2017.1419996
Borgers, M., Degreef, H., and Cauwenbergh, G. (2005). Fungal infections of the skin: infection process and antimycotic therapy. Curr. Drug Targets 6, 849–862. doi: 10.2174/138945005774912726
Brown, G. D., Denning, D. W., Gow, N. A., Levitz, S. M., Netea, M. G., and White, T. C. (2012). Hidden killers: human fungal infections. Sci. Transl. Med. 4:165rv13. doi: 10.1126/scitranslmed.3004404
Cecchini, C., Cresci, A., Coman, M. M., Ricciutelli, M., Sagratini, G., Vittori, S., et al. (2007). Antimicrobial activity of seven Hypericum entities from central Italy. Planta Med. 73, 564–566.
Crockett, S. L., Poller, B., Tabanca, N., Pferschy-Wenzig, E. M., Kunert, O., Wedge, D. E., et al. (2011). Bioactive xanthones from the roots of Hypericum perforatum (common St John’s wort). J. Sci. Food Agric. 91, 428–434. doi: 10.1002/jsfa.4202
Daglia, M. (2012). Polyphenols as antimicrobial agents. Curr. Opin. Biotechnol. 23, 174–181. doi: 10.1016/j.copbio.2011.08.007
Dismukes, W. E. (2000). Introduction to antifungal drugs. Clin. Inf. Dis. 30, 653–657. doi: 10.1086/313748
Dugoua, J.-J., Mills, E., Perri, D., and Koren, G. (2006). Safety and efficacy of St. John’s wort (hypericum) during pregnancy and lactation. Can. J. Clin. Pharmacol. 13, e268–76.
Euro+Med (2006). Euro+Med Plant Base the Information Resource for Euro-Mediterranean Plant Diversity. Available at: http://ww2.bgbm.org/EuroPlusMed/ [accessed December 15, 2017].
European Committee for Antimicrobial Susceptibility Testing (2012). Method for the Determination of Broth Dilution Minimum Inhibitory Concentrations of Antifungal Agents for Yeasts. EUCAST DEFINITIVE DOCUMENT EDef 7. 2 Revision. Brussels: European Commission.
Evensen, N. A., and Braun, P. C. (2009). The effects of tea polyphenols on Candida albicans: inhibition of biofilm formation and proteasome inactivation. Can. J. Microbiol. 55, 1033–1039. doi: 10.1139/w09-058
Glazer, I., Masaphy, S., Marciano, P., Bar-Ilan, I., Holland, D., Kerem, Z., et al. (2012). Partial identification of antifungal compounds from Punica granatum peel extracts. J. Agric. Food Chem. 60, 4841–4848. doi: 10.1021/jf300330y
Gurib-Fakim, A. (2006). Medicinal plants: traditions of yesterday and drugs of tomorrow. Mol. Aspects Med. 27, 1–93. doi: 10.1016/j.mam.2005.07.008
Hirasawa, M., and Takada, K. (2004). Multiple effects of green tea catechin on the antifungal activity of antimycotics against Candida albicans. J. Antimicrob. Chem. 53, 225–229. doi: 10.1093/jac/dkh046
Johnson, D. C. (2012). Chronic candidal bronchitis: a consecutive series. Open Respir. Med. J. 6, 145–149. doi: 10.2174/1874306401206010145
Lass-Flörl, C. (2009). The changing face of epidemiology of invasive fungal disease in Europe. Mycoses 52, 197–205. doi: 10.1111/j.1439-0507.2009.01691.x
Li, S., Zhang, Z., Cain, A., Wang, B., Long, M., and Taylor, J. (2005). Antifungal activity of camptothecin, trifolin, and hyperoside isolated from Camptotheca acuminata. J. Agric. Food Chem. 53, 32–37. doi: 10.1021/jf0484780
Marchelak, A., Owczarek, A., Matczak, M., Pawlak, A., Kolodziejczyk-Czepas, J., Nowak, P., et al. (2017). Bioactivity potential of Prunus spinosa L. Flower extracts: phytochemical profiling, cellular safety, pro-inflammatory enzymes inhibition and protective effects against oxidative stress in vitro. Front. Pharmacol. 8:680. doi: 10.3389/fphar.2017.00680
Menkovic, N., Savikin, K., Tasic, S., Zdunic, G., Stesevic, D., Milosavljevic, S., et al. (2011). Ethnobotanical study on traditional uses of wild medicinal plants in Prokletije Mountains (Montenegro). J. Ethnopharmacol. 133, 97–107. doi: 10.1016/j.jep.2010.09.008
Nürk, N. M., and Crockett, S. L. (2011). Morphological and phytochemical diversity among Hypericum species of the Mediterranean Basin. Med. Aromat. Plant Sci. Biotechnol. 5, 14–28.
Ogawa, H., Fujimura, M., Ohkura, N., and Makimura, K. (2014). It is time to call attention to the clinical significance of fungal colonization in chronic cough. Allergol. Int. 63, 611–612. doi: 10.2332/allergolint.14-LE-0691
Papon, N., Courdavault, V., Clastre, M., and Bennett, R. J. (2013). Emerging and emerged pathogenic candida species: beyond the Candida albicans paradigm. PLoS Pathog. 9:e1003550. doi: 10.1371/journal.ppat.1003550
Pieroni, A., Quave, C. L., and Santoro, R. F. (2004). Folk pharmaceutical knowledge in the territory of the Dolomiti Lucane, inland southern Italy. J. Ethnopharmacol. 95, 373–384. doi: 10.1016/j.jep.2004.08.012
Pistelli, L., Bertoli, A., Zucconelli, S., Morelli, I., Panizzi, L., and Menichini, F. (2000). Antimicrobial activity of crude extracts and pure compounds of Hypericum hircinum. Fitoterapia 71, S138–S140. doi: 10.1016/S0367-326X(00)00189-1
Raclariu, A. C., Paltinean, R., Vlase, L., Labarre, A., Manzanilla, V., Ichim, M. C., et al. (2017). Comparative authentication of Hypericum perforatum herbal products using DNA metabarcoding, TLC and HPLC-MS. Sci. Rep. 7:1291. doi: 10.1038/s41598-017-01389-w
Radulović, N., Stankov-Jovanović, V., Stojanović, G., Šmelcerović, A., Spiteller, M., and Asakawa, Y. (2007). Screening of in vitro antimicrobial and antioxidant activity of nine Hypericum species from the Balkans. Food Chem. 103, 15–21. doi: 10.1016/j.foodchem.2006.05.062
Raza, A., Xu, X., Sun, H., Tang, J., and Ouyang, Z. (2017). Pharmacological activities and pharmacokinetic study of hyperoside: a short review. Trop. J. Pharm. Res. 16, 483–489. doi: 10.4314/tjpr.v16i2.30
Reis, M. P. C., Carvalho, C. R. C., Andrade, F. A., Fernandes, O. D. F. L., Arruda, W., and Silva, M. R. R. (2016). Fisetin as a promising antifungal agent against Cryptococcus neoformans species complex. J. Appl. Microbiol. 121, 373–379. doi: 10.1111/jam.13155
Ritchie, T., and Eltahawy, E. (2014). Diagnosis and management of fungal urinary tract infections. Curr. Bladder Dysfunct. Rep. 9, 161–166. doi: 10.1007/s11884-014-0238-7
Sagratini, G., Ricciutelli, M., Vittori, S., Öztürk, N., Öztürk, Y., and Maggi, F. (2008). Phytochemical and antioxidant analysis of eight Hypericum taxa from Central Italy. Fitoterapia 79, 210–213. doi: 10.1016/j.fitote.2007.11.011
Saito, H., Tamura, M., Imai, K., Ishigami, T., and Ochiai, K. (2013). Catechin inhibits Candida albicans dimorphism by disrupting Cek1 phosphorylation and cAMP synthesis. Microb. Pathog. 56, 16–20. doi: 10.1016/j.micpath.2013.01.002
Salazar-Aranda, R., Granados-Guzmán, G., Pérez-Meseguer, J., González, G. M., and de Torres, N. W. (2015). Activity of polyphenolic compounds against Candida glabrata. Molecules 20, 17903–17912. doi: 10.3390/molecules201017903
Sarić-Kundalić, B., Dobes, C., Klatte-Asselmeyer, V., and Saukel, J. (2010). Ethnobotanical study on medicinal use of wild and cultivated plants in middle, south and west Bosnia and Herzegovina. J. Ethnopharmacol. 131, 33–55. doi: 10.1016/j.jep.2010.05.061
Shahzad, M., Sherry, L., Rajendran, R., Edwards, C. A., Combet, E., and Ramage, G. (2014). Inhibitory effect of plant (poly) phenolics on growth and biofilm formation by Candida albicans. Proc. Nutr. Soc. 73:E28. doi: 10.1017/S0029665114000421
Sitheeque, M. A. M., Panagoda, G. J., Yau, J., Amarakoon, A. M. T., Udagama, U. R. N., and Samaranayake, L. P. (2009). Antifungal activity of black tea polyphenols (catechins and theaflavins) against Candida species. Chemotherapy 55, 189–196. doi: 10.1159/000216836
Stojanovic, G., Dordevic, A., and Smelcerovic, A. (2013). Do other Hypericum species have medical potential as St. John’s Wort (Hypericum perforatum)? Curr. Med. Chem. 20, 2273–2295. doi: 10.2174/0929867311320180001
Strati, F., Di Paola, M., Stefanini, I., Albanese, D., Rizzetto, L., Lionetti, P., et al. (2016). Age and gender affect the composition of fungal population of the human gastrointestinal tract. Front. Microbiol. 7:1277. doi: 10.3389/fmicb.2016.01227
Tocci, N., Gaid, M., Kaftan, F., Belkheir, A. K., Belhadj, I., Liu, B., et al. (2018b). Exodermis and endodermis are the sites of xanthone biosynthesis in Hypericum perforatum roots. New Phytol. 217, 1099–1112. doi: 10.1111/nph.14929
Tocci, N., Weil, T., Perenzoni, D., Narduzzi, L., Madriñán, S., Crockett, S., et al. (2018a). Phenolic profile, chemical relationship and antifungal activity of Andean Hypericum species. Ind. Crops Prod. 112, 32–37. doi: 10.1016/j.indcrop.2017.10.030
Tocci, N., Simonetti, G., D’Auria, F. D., Panella, S., Palamara, A. T., Ferrari, F., et al. (2013). Chemical composition and antifungal activity of Hypericum perforatum subsp. angustifolium roots from wild plants and plants grown under controlled conditions. Plant Biosyst. 147, 557–562. doi: 10.1080/11263504.2013.806964
Tortorano, A. M., Kibbler, C., Peman, J., Bernhardt, H., Klingspor, L., and Grillot, R. (2006). Candidaemia in Europe: epidemiology and resistance. Int. J. Antimicrob. Agents 27, 359–366. doi: 10.1016/j.ijantimicag.2006.01.002
Vrhovsek, U., Masuero, D., Gasperotti, M., Franceschi, P., Caputi, L., Viola, R., et al. (2012). A versatile targeted metabolomics method for the rapid quantification of multiple classes of phenolics in fruits and beverages. J. Agric. Food Chem. 60, 8831–8840. doi: 10.1021/jf2051569
Weil, T., Santamaría, R., Lee, W., Rung, J., Tocci, N., Abbey, D., et al. (2017). Adaptive mistranslation accelerates the evolution of fluconazole resistance and induces major genomic and gene expression alterations in Candida albicans. mSphere 2, e00167–17.
World Health Organization [WHO] (2014). Antimicrobial Resistance Global Report on Surveillance 2014. Geneva: WHO Press.
Keywords: Hypericum, antifungal, Candida, cytotoxicity, LC-MS, phenolic compounds
Citation: Tocci N, Perenzoni D, Iamonico D, Fava F, Weil T and Mattivi F (2018) Extracts From Hypericum hircinum subsp. majus Exert Antifungal Activity Against a Panel of Sensitive and Drug-Resistant Clinical Strains. Front. Pharmacol. 9:382. doi: 10.3389/fphar.2018.00382
Received: 15 December 2017; Accepted: 03 April 2018;
Published: 20 April 2018.
Edited by:
Atanas G. Atanasov, Institute of Genetics and Animal Breeding (PAS), PolandReviewed by:
Letizia Angiolella, Sapienza Università di Roma, ItalyKannan R. R. Rengasamy, Alagappa University, India
Copyright © 2018 Tocci, Perenzoni, Iamonico, Fava, Weil and Mattivi. This is an open-access article distributed under the terms of the Creative Commons Attribution License (CC BY). The use, distribution or reproduction in other forums is permitted, provided the original author(s) and the copyright owner are credited and that the original publication in this journal is cited, in accordance with accepted academic practice. No use, distribution or reproduction is permitted which does not comply with these terms.
*Correspondence: Tobias Weil, dG9iaWFzLndlaWxAZm1hY2guaXQ= Fulvio Mattivi, ZnVsdmlvLm1hdHRpdmlAdW5pdG4uaXQ=; ZnVsdmlvLm1hdHRpdmlAZm1hY2guaXQ=