- 1Department of Biomedical Science, University of Nottingham Malaysia Campus, Semenyih, Malaysia
- 2School of Life Sciences, The Chinese University of Hong Kong, Shatin, China
- 3Medicinal Mushroom Research Group, Department of Molecular Medicine, Faculty of Medicine, University of Malaya, Kuala Lumpur, Malaysia
- 4LiGNO Biotech Sdn Bhd, Balakong Jaya, Malaysia
- 5School of Life Sciences, University of Nottingham Medical School, Queen’s Medical Centre, Nottingham, United Kingdom
- 6Center for Natural Products Research and Drug Discovery, University of Malaya, Kuala Lumpur, Malaysia
Lignosus rhinocerotis has a long history of use by the indigenous community within East Asia to treat a range of health conditions including asthma and chronic cough. To date, there is limited scientific evidence to support its therapeutic effects in relieving these airways conditions. In this study, we examined the effects of the different molecular weight fractions [high-molecular-weight (HMW), medium-molecular-weight (MMW), and low-molecular-weight (LMW)] obtained from the cold water sclerotial extract (CWE) of L. rhinocerotis on airways patency using airway segments isolated from Sprague Dawley rat in an organ bath set-up. It is demonstrated that the HMW and MMW fractions exhibited higher efficacy in relaxing the pre-contracted airways when compared to the CWE and LMW fraction. In addition, the HMW fraction markedly supressed carbachol-, 5-hydroxytrptamine-, and calcium-induced airway contractions. CWE demonstrated a lower efficacy than the HMW fraction but it also significantly attenuated carbachol- and calcium-induced airway contractions. Results showed that the bronchorelaxation effect of CWE and fractions is mediated via blockade of extracellular Ca2+ influx. The composition analysis revealed the following parts of carbohydrate and proteins, respectively: HMW fraction: 71 and 4%; MMW fraction: 35 and 1%; and LMW fraction: 22 and 0.3%. Our results strongly suggest that the polysaccharide–protein complex or proteins found in the HMW and MMW fractions is likely to contribute to the bronchorelaxation effect of CWE.
Introduction
Lignosus rhinocerotis (Cooke) Ryvarden, belonging to the Polyporaceae family, is commonly used as folk medicine among indigenous people in Southeast Asia to treat a variety of conditions, including cough, asthma and cancers (Abdullah et al., 2013). It is also known as Polyporus rhinocerus (Cooke), which is the taxonomical synonym of L. rhinocerotis (Huang, 1999). From ethnobotanical surveys, the methods of consumption reported are by boiling the mushroom sclerotium in water and drinking the decoction, or by eating the sclerotium raw (Azliza et al., 2012). The medicinal value of this mushroom is derived from its sclerotium.
Scientific evidence of the biological activity and bioactive components of L. rhinocerotis has been on the rise in recent years. The antiproliferative effect against several human leukemic cell lines (HL-60, K562 and THP-1) is suggested to be attributed to the hot water-soluble polysaccharide–protein complex extracted from the P. rhinocerus (Lai et al., 2008). Since the successful cultivation of L. rhinocerotis on agar, solid and spawn media in year 2009 (Tan, 2009), more chemical profiling and characterization of the bioactive components have been published. Evidence gathered from a number of different assays measuring the anti-inflammatory, anti-proliferative, and immunomodulatory activities of L. rhinocerotis consistently attributed its actions to the HMW components, in particular the polysaccharide–protein complex or proteins, fractionated from water-soluble sclerotial extract of L. rhinocerotis (Lee et al., 2012, 2014; Lau et al., 2013). Shotgun proteomics analysis of L. rhinocerotis has revealed that lectins and serine proteases are the most abundant proteins present in the sclerotium, together with other proteins including immunomodulatory protein 8 and cerato-platanin (Yap et al., 2015). Fungal immunomodulatory protein, FIP-fve, isolated from Flammulina velutipes was shown to attenuate the infiltrating inflammatory cells in the lungs of allergic asthma mouse model and reduced the pulmonary resist-ance measured by whole-body plethysmography (Lee et al., 2013).
Asthma and chronic obstructive pulmonary disease (COPD) are respiratory diseases characterised by airway obstruction, with varying degrees of inflammation (Cukic et al., 2012). One distinguishable physiological characteristic between the diseases is the reversibility of airflow limitation, with asthma being reversible and COPD being incompletely reversible, either spontaneously or with treatment. Bronchodilators, including β2-adrenoceptor agonists and muscarinic receptor antagonist, are the mainstay of treatment for asthma and COPD respectively (Proskocil and Fryer, 2005). One issue concerning the use of β2-adrenoceptor agonists is its diminishing effectiveness due to β2-adrenoceptor desensitisation after prolonged monotherapy (Cooper et al., 2011). Inhaled corticosteroids are often co-administered to treat underlying airway inflammation and to prevent tolerance to β2-adrenoceptor agonists (Barnes and Adcock, 2003). Moreover, development of an effective antimuscarinic bronchodilator has been challenging to balance between clinical effectiveness and side effects of the drug (Moulton and Fryer, 2011). The currently available bronchodilators are not ideal, hence, the need to search for alternative therapies with different mechanisms of action.
The pathophysiology of asthma and COPD has been linked with excessive release of acetylcholine, causing overactivation of muscarinic receptors and subsequently airway smooth muscle contraction (Erle and Sheppard, 2014). Serotonin or 5-hydroxytryptamine (5-HT) is another neurotransmitter which has complex actions on pulmonary function. Activation of 5-HT receptors on airway smooth muscle has been reported to cause smooth muscle contraction or relaxation. Another explanation for the complex action of 5-HT is that its presence could indirectly modulate the acetylcholine level leading to muscle contraction (Cazzolau et al., 1995). In rat, 5-HT activates 5-HT2A receptors on airway smooth muscle and causes airway contraction. In human, 5-HT plasma levels are elevated in asthmatic patients and in vitro evidences showed that it might have a role in asthma (Dupont et al., 1999). Activation of Gαq-protein-coupled M3 and 5-HT2A receptors on airway smooth muscle, results in 1, 4, 5-trisphosphate (IP3) and diacylglycerol (DAG) generation and mobilisation of extracellular and intracellular stored Ca2+. Elevation of cytoplasmic Ca2+ level subsequently leads to airway smooth muscle contraction (Cazzolau et al., 1995; Erle and Sheppard, 2014).
To date, no other group has reported the direct effect of airway relaxation mediated by the bioactive components of L. rhinocerotis. The objectives of this study were to examine the airway relaxation effect of the different molecular weight fractions obtained from the CWE of L. rhinocerotis and to investigate the mechanism of the bronchodilator action.
Materials and Methods
Preparation, Extraction and Fractionation of the Sclerotial Cold Water Extract
Ground mushroom sclerotial powder (0.2 mm sieve) from L. rhinocerotis (TM02), identified by the internal transcribed spacer (ITS) regions of their ribosomal RNA (Tan et al., 2010), was supplied by LiGNO Biotech Sdn Bhd (Selangor, Malaysia). The mushroom specimen voucher is deposited in the Royal Botanic Garden Kew, K(M)177812. The preparation method for the CWE was adopted from our previous paper (Yap et al., 2013). In brief, the sclerotial powder was extracted by stirring in cold water at 4°C for 24 h at a ratio of 1:20 (w/v). CWE obtained by filtration through Whatman No. 1 filter paper was then freeze-dried. CWE was re-dissolved in purified water (Milli-Q quality) to make a stock solution of 100 mg/ml before being fractionated by gel permeation chromatography using a Sephadex G50 column (212 ml). Aqueous elution was carried out at room temperature by gravity and 100 fractions of 2.5 ml volume each were collected. Aliquots from the tubes were sampled for testing the protein and carbohydrate content by measurement of tis absorbance reading at 595 and 490 nm, respectively. Molecular weight (MW) of the pooled fractions was determined by comparing their ratio of elution volume (Ve) and void volume (Vo) to the Ve/Vo of selected protein standards including carbonic anhydrase (29 kDa), soybean trypsin inhibitor (20 kDa), ribonuclease A (14 kDa), cardiotoxin (7 kDa), cobalamin (1.35 kDa), and riboflavin (0.376 kDa).
Determination of Total Carbohydrate and Protein Contents
Carbohydrate and protein contents in the different fractions were determined with phenol-sulfuric acid method as described by DuBois et al. (1956) and Bradford (1976) method, respectively. D-Glucose and bovine serum albumin (BSA) were used as standards, respectively.
Determination of Monosaccharide Composition
The monosaccharide composition of the carbohydrate moiety in the CWE and its different MW fractions were determined by the alditol acetate derivatives of the monosaccharides by gas chromatography (7890B, Agilent Technology, United States) equipped with an Alltech DB-225 capillary column (15 m × 0.25 mm i.d., 0.25 μm films). The oven temperature was increased from 150 to 220°C at the rate of 2°C/min and kept at 220°C for 15 min. The carrier gas was helium. The monosaccharide standards consisted of D-fucose, L-rhamnose, D-ribose, L-arabinose, D-xylose, D-mannose, D-galactose, and D-glucose. D-Allose was used as internal standard. All the monosaccharide standards were purchased from Sigma-Aldrich, United States.
Tissues Preparation
Ethical approval was obtained from the University of Nottingham’s Animal Welfare and Ethics Review Body (UNMC#2kn and UNMC12). All applicable international, national and/or institutional guidelines for the care and use of animals were followed. Experiments were conducted with male Sprague Dawley rats (235–440 g; 2–3 months old). Sprague Dawley rats were purchased from University Putra Malaysia or University Kebangsaan Malaysia and sacrificed on the day of experiment. Rat trachea and bronchus were removed, excised into 2 mm rings, and immediately immersed in standard Krebs-Ringer bicarbonate solution (pH 7.4). All the methods used in this study were adopted from previous study (Loong et al., 2015). Changes in muscle tension responses produced by tissue contraction and relaxation were detected by force transducer (MLTF050/ST, ADInstruments, United States). Data were recorded by a PowerLab data acquisition system (LabChart v7.3.4) and a computer (Hewlett-Packard, United States). Muscle tension was expressed in milliNewtons (mN). Tissues were left to equilibrate to bath conditions for 30 min after the application of 9.8 mN tension to achieve a steady baseline tone. Following equilibration, all tissues were exposed twice to 60 mM potassium chloride (KCl) to assess tissue viability and to provide reference contractions for subsequent data analysis. After the KCl was washed out and a stable basal tone was re-established, experiments were carried out as in Section “Organ Bath Experimental Protocol.”
Organ Bath Experimental Protocol
Concentration-Response Curves to CWE and Fractions Against Pre-contracted Airway Segments
Tracheal and bronchial segments were pre-contracted with carbachol (1 μM), a non-selective acetylcholine receptor agonist, to obtain a stable submaximal contraction between 70 and 80% of the maximum carbachol contraction [determined from previous experiments (data not shown)]. Once a stable contraction was established, cumulative concentrations of CWE, HMW, MMW, or LMW fractions, was added into the preparation every 15 min. The degree of response (tissue relaxation) was measured as a fraction of the contraction achieved by 1 μM of carbachol. The tissue relaxation was expressed as a percentage of carbachol-induced tone (n = 4 to 6 animals). Vehicle control, either purified water or the appropriate concentration of DMSO, was carried out in all the following protocols.
Effect of CWE and Fractions Against Carbachol and 5-Hydroxytrptamine (5-HT) Concentration-Response Curve
Tracheal rings were pre-incubated with 2.5 mg/ml CWE, 2.5 mg/ml HMW fraction, 2.5 mg/ml LMW fraction, or 30 nM ipratropium (muscarinic receptor antagonist) for at least 30 min, prior to the construction of the carbachol or 5-HT concentration-response curve (CRC). Tissue contraction to carbachol or 5-HT was expressed as a percentage of 60 mM KCl-induced tone (n = 5 to 6 animals).
Effect of CWE and Fractions on Calcium-Induced Contraction
Tracheal segments were bathed in calcium-free Krebs solution and pre-incubated with the 2.5 mg/ml CWE, 2.5 mg/ml HMW fraction, 2.5 mg/ml LMW fraction, or 10 μM nifedipine for at least 30 min. KCl (60 mM) was added to depolarize the cell membrane and left to incubate for 10 min before calcium chloride (CaCl) was reintroduced cumulatively. Tissue contraction to CaCl was expressed as a percentage of the 60 mM KCl-induced tone (n = 5 to 7 animals).
Statistical Analysis
Data were analysed and graphs were drawn using PRISM v7.0 (GraphPad software). All data were expressed as mean ± standard deviation (SD) of n number of animals. Maximum tissue contraction or relaxation response (Emax) was derived from non-linear regression analysis of the obtained CRC or from the response generated at the highest concentration tested. Statistical analyses were performed using one-way ANOVA, two-way ANOVA with Bonferroni correction and Student’s unpaired t-test. Results were considered statistically significant if p-value was less than 0.05.
Drugs
Carbamycholine chloride or carbachol (Nacalai Tesque, Japan), 5-hydroxytryptamine (Nacalai Tesque, Japan), and ipratropium bromide (Tocris, United Kingdom) were dissolved in purified water. Nifedipine (Nacalai Tesque, Japan) was dissolved in 100% DMSO (Sigma-Aldrich, United States) to make 100 mM of stock solution and further diluted in 50% DMSO to make 10 mM of nifedipine solution.
Results
Fractionation of Sclerotial Extract From Lignosus rhinocerotis
Cold water extraction of the sclerotial powder of L. rhinocerotis yielded 7.04 ± 0.69 g of freeze-dried solid. Further fractionation of the extract by Sephadex® G-50 gel filtration chromatography yielded a broad protein peak and several carbohydrate peaks (Figure 1). The fraction tubes collected were pooled into three fractions based on their molecular weight range. They are the high-, medium-, and low-molecular-weight fractions designated by HMW, MMW, and LMW, respectively. The MW range of each fraction were: HMW (>29.9 kDa), MMW (7.1 to 27.4 kDa) and LMW (0.8 to 6.5 kDa).
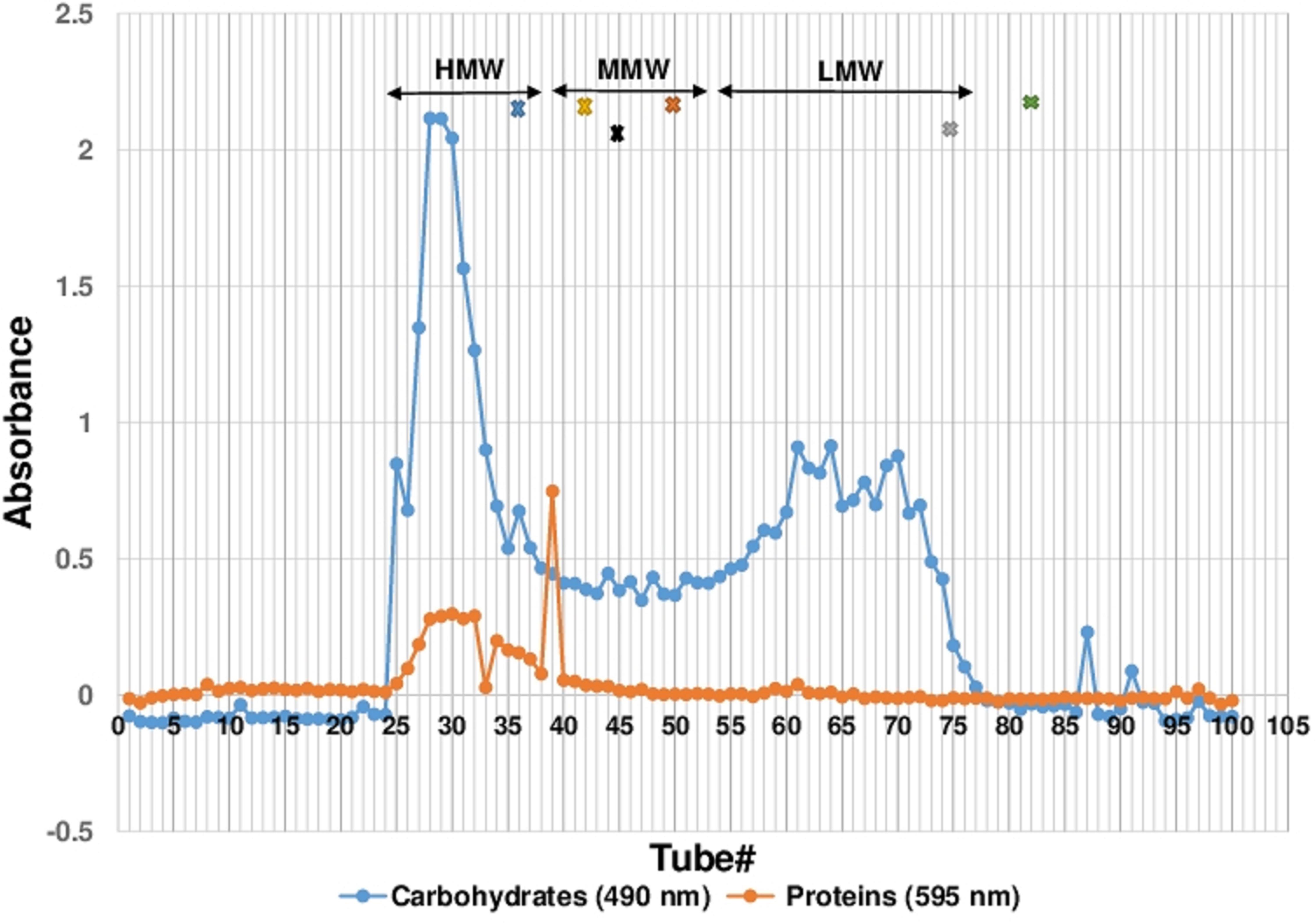
FIGURE 1. Sephadex® G-50 Fractionation of CWE. Fractionation of 100 tubes were collected with each tubes containing 2.5 mL of fraction. Proteins and carbohydrates content were measured as absorbance reading at 595 and 490 nm, respectively. The fractionation of the CWE was separated into three molecular weight ranges, HMW, MMW and LMW. Protein standards used for determining the molecular mass of pooled fraction were carbonic anhydrase (29 kDa), soybean trypsin inhibitor (20 kDa), ribonuclease A (14 kDa), cardiotoxin (7 kDa), cobalamin (1.35 kDa), and riboflavin (0.376 kDa) as indicated as x in the colour of blue, black, gold, orange, grey and green, respectively. CWE, Lignosus rhinocerotis TM02 sclerotial CWE.
Chemical Composition of CWE and Its Fractions
The chemical composition in Table 1 show that the majority of carbohydrate and protein present in CWE is found in the HMW fraction, indicating the presence of polysaccharides and polysaccharide–protein complexes. There was a larger amount of non-protein and non-carbohydrate substances in the LMW and MMW fractions than the HMW fraction. These are LMW molecules such as phenolic compounds found abundantly in CWE (Yap et al., 2013). The monosaccharide profile of CWE and its fractions indicated the presence of different MW carbohydrates having different sugar composition. Glucose was the most dominant sugar found in CWE and its fractions indicating the presence of water-soluble glucans which are commonly found in mushrooms. Other than glucans, there were small amounts of other heteroglycans containing arabinose, mannose and galactose in CWE and its fractions. It was noted that the amount of mannose and galactose in the fractions correlated with the amount of protein in the fractions, suggesting the presence of manno- and/or galacto-glycoprotein linkages in polysaccharide–protein complexes.
Unequivocal Airway Relaxation Effects of CWE, HMW and MMW Fractions
CWE relaxed both tracheal (79.45 ± 39.68%, p = 0.0018) and bronchial (86.90 ± 16.30, p = 0.0119) segments and both responses were statistically different from the corresponding vehicle control (39.68 ± 5.47%) (see Figures 2A,B). Based on the maximum tissue relaxation (Emax) (see Figures 2C,D), the effectiveness of the test samples is similar in trachea (T) and bronchus (B): HMW fraction (T: 117.32 ± 24.62%; B: 101.67 ± 16.34%) and MMW fraction (T: 105.84 ± 17.58%; B: 108.30 ± 19.98%) produced the largest response followed by CWE. The responses produced by the LMW fraction in trachea and bronchus were no different (T: 40.64 ± 35.02%; B: 65.80 ± 13.21%) from vehicle control (T: 39.68 ± 12.23%; B: 49.68 ± 23.70%).
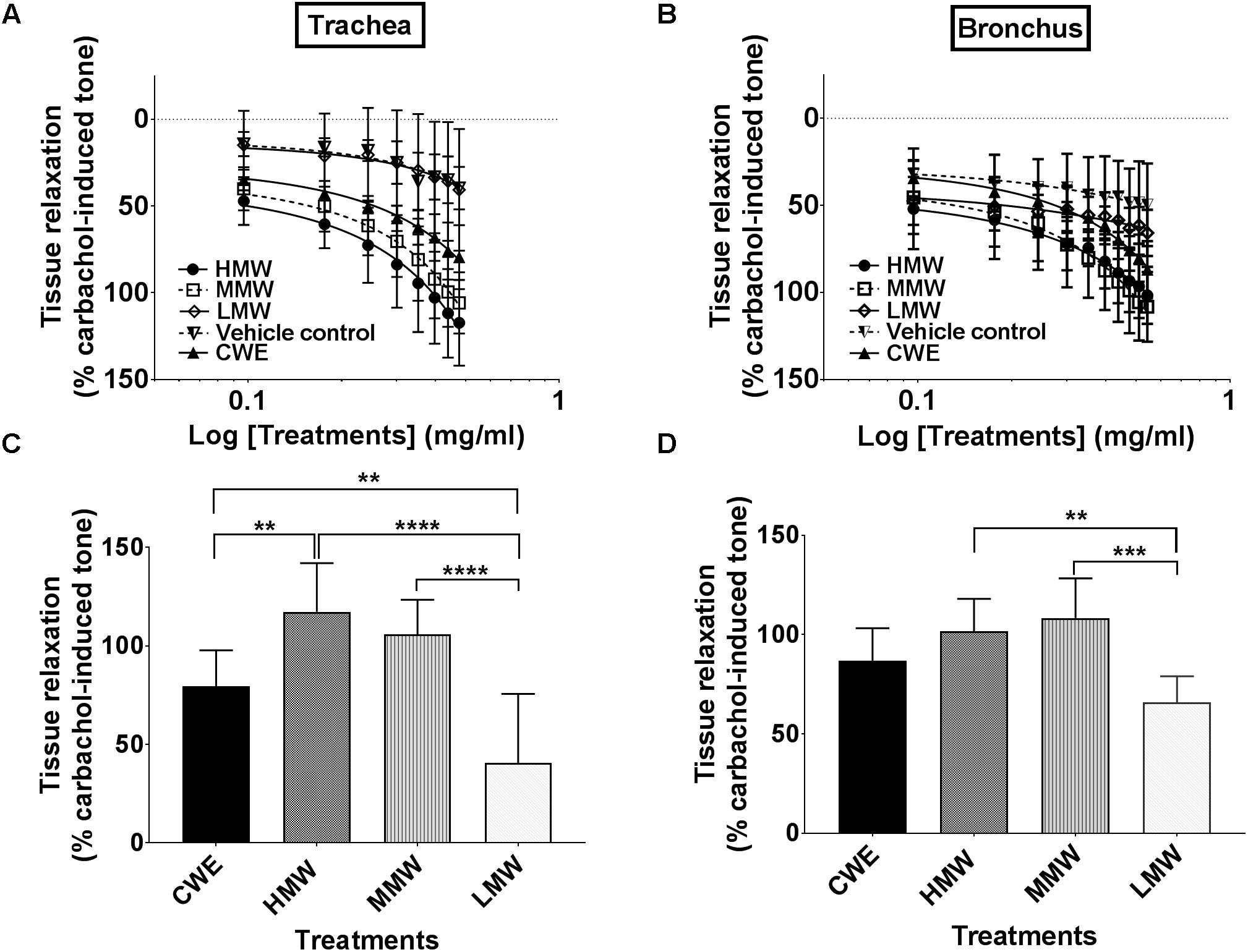
FIGURE 2. Effect of CWE and fractions cumulative CRC against carbachol-induced contractions of the rat isolated (A) trachea and (B) bronchus. Comparison of the bronchorelaxation effects of the fractions at the highest concentrations tested are depicted in (C) trachea at 3.0 mg/ml and (D) bronchus at 3.5 mg/ml. Tissue responses have been expressed as a percentage of the carbachol-induced contraction and are shown as means ± SD of 4 to 6 animals. CWE, cold water extract; HMW, high-molecular-weight fraction; MMW, medium-molecular-weight fraction; LMW, low-molecular-weight fraction (two way ANOVA: ∗∗p < 0.01; ∗∗∗p < 0.001; ∗∗∗∗p < 0.0001).
HMW Fraction Markedly Prevents Carbachol- and 5-HT-Induced Airway Constriction
Based on the above findings (see section “Unequivocal Airway Relaxation Effects of CWE, HMW and MMW Fractions”), subsequent experiments were carried out using 2.5 mg/ml of CWE, HMW and LMW fractions on tracheal rings. HMW fraction almost totally prevented carbachol- (Emax: 44.52 ± 36.61%, p = 0.0002) and 5-HT-(Emax: 2.48 ± 3.41%, p < 0.0001)-induced contraction compared to the vehicle control (Emax of carbachol: 241.20 ± 24.41%; Emax of 5-HT: 85.24 ± 30.95%) (Figure 3) in the airway segments. Although the effect of CWE (Emax: 165.00 ± 45.37%, p = 0.0129) was not as marked as HMW fraction, it also significantly attenuated the Emax of carbachol and exhibited a similar response as ipratropium (Emax: 177.30 ± 46.88%, p = 0.0447). On the contrary, LMW fraction did not affect any of the tissue contractions induced by carbachol (Emax: 187.90 ± 26.88%, p = 0.1497) or 5-HT (Emax: 69.91 ± 24.22%, p > 0.999). Results are summarised in Table 2.
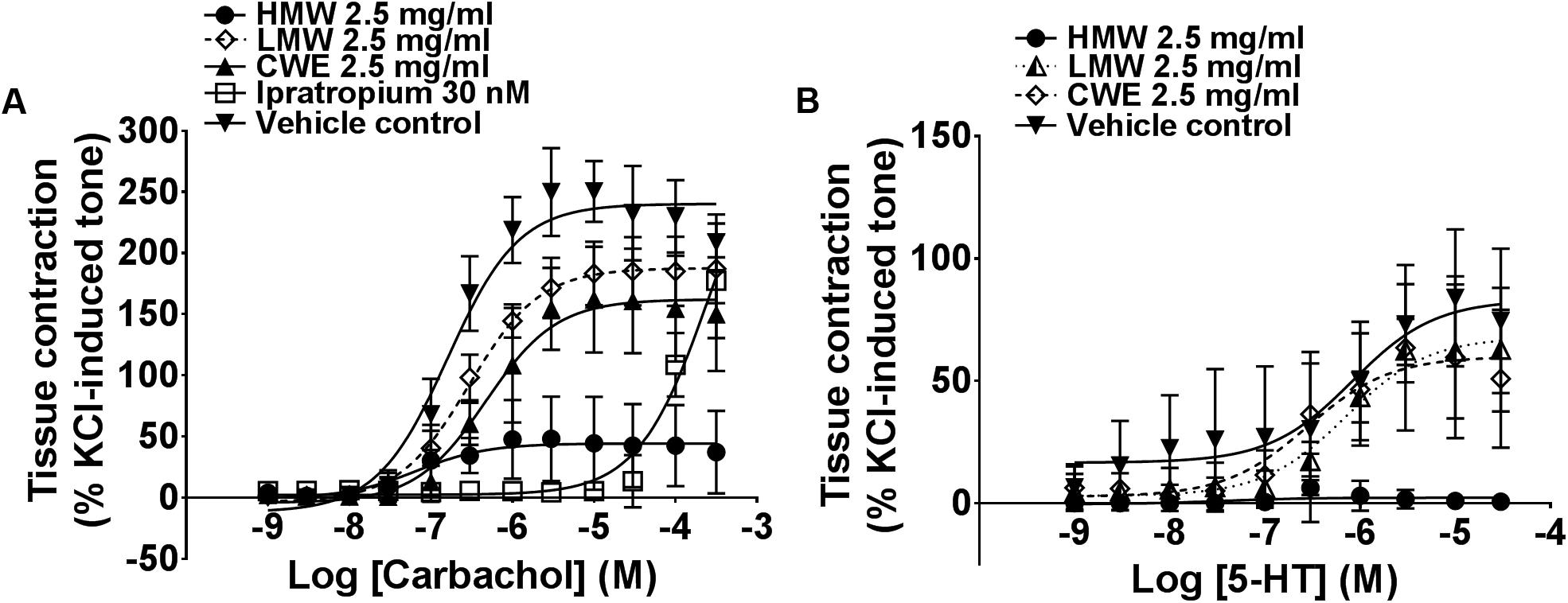
FIGURE 3. Effect of CWE and fractions pre-incubation against (A) carbachol and (B) 5-HT CRC in rat isolated tracheal rings. Concentration-response curves (CRCs) to (A) carbachol and (B) 5-HT in the absence or presence of CWE and fractions in rat isolated tracheal rings. Tissue responses have been expressed as a percentage of KCl-induced contraction and are shown as means ± SD of 5 to 6 animals.
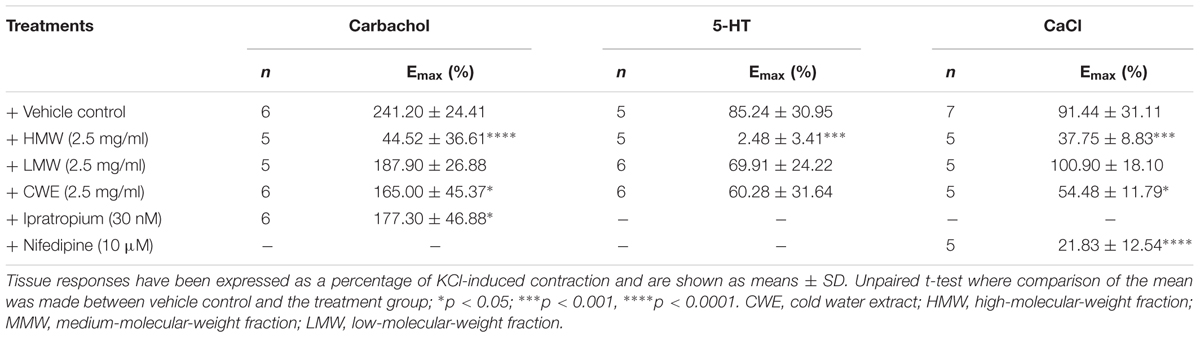
TABLE 2. The maximum response (Emax) of carbachol, 5-HT and CaCl derived from the respective concentration-response curve (CRC) in the presence of different treatment.
HMW Fraction Significantly Limits Calcium-Induced Contraction
Smooth muscle contraction is associated with increased intracellular Ca2+ level. This is mediated via extracellular Ca2+ influx through membrane calcium channel or release of Ca2+ from intracellular sarcoplasmic reticulum stores. The HMW fraction (Emax: 37.75 ± 8.83%, p = 0.0005) significantly attenuated the contraction induced by external calcium, similar to the effect of nifedipine (Emax: 21.83 ± 12.54%, p = 0.0001) compared to vehicle control (Emax: 91.44 ± 31.11%) (Figure 4). CWE (Emax: 54.48 ± 11.79%, p = 0.0149) also significantly reduced the Emax of calcium chloride, although to a lesser extent than HMW fraction and nifedipine. The LMW fraction (Emax: 100.90 ± 18.10%, p = 0.829) had the same effect as vehicle. It was unable to calculate the Emax from non-linear regression curve and therefore Emax was taken as the response at highest concentration tested. Results are summarised in Table 2.
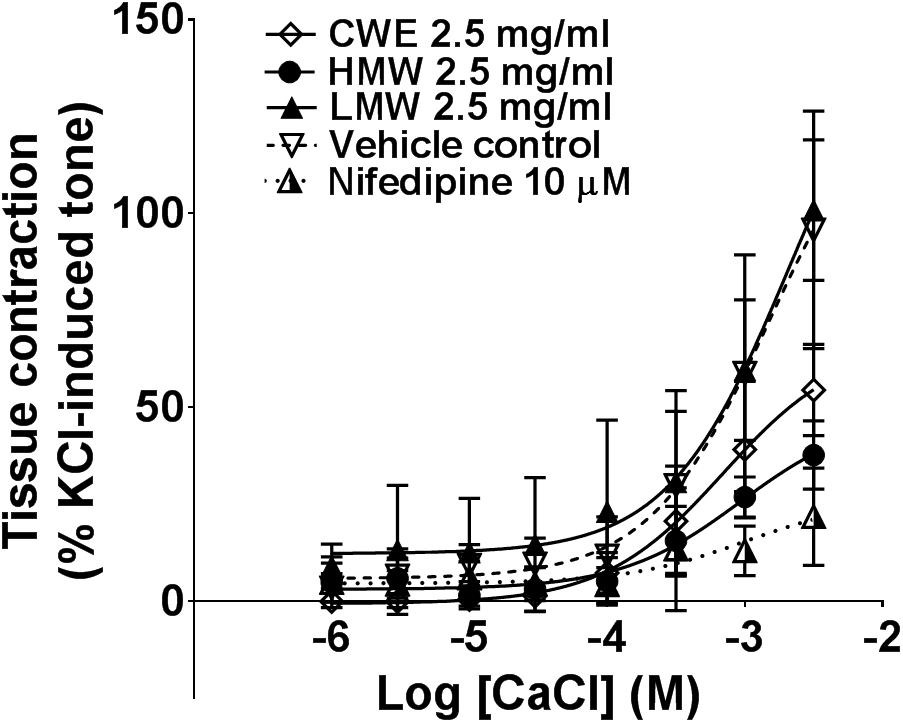
FIGURE 4. Effect of CWE and fractions against CaCl CRC in rat isolated tracheal rings. Concentration-response curves to calcium in the absence or presence of CWE and fractions in rat isolated tracheal rings. Tissue responses have been expressed as a percentage of KCl-induced contraction and are shown as means ± SD of 5 to 7 animals.
Discussion
Lignosus rhinocerotis has a long history of use by the indigenous community to treat asthma and chronic cough (Lau et al., 2015). This is the first study to elucidate the bioactive fractions accounting for the airway relaxation effect elicited by this mushroom. Our earlier study showed that CWE has fully relaxed both the isolated tracheal and bronchial segments pre-contracted with carbachol (Lee et al., 2018). Two most common bronchodilators used to reverse airways constriction act via stimulation of β2-adrenoceptors (e.g., salmeterol) or antagonism of muscarinic receptors (e.g., ipratropium). The relaxation effects of CWE are not mediated through β2-adrenoceptor stimulation, nitric oxide, or K+ channel (see Supplementary Figure 1 and Lee et al., 2018) or blockade of muscarinic receptors (see Figure 3A). Our evidence to date implies that CWE relaxes airways through a mechanism different from that used by the conventional bronchodilators. Interestingly, CWE significantly limits calcium-induced contraction in the tracheal rings. This implies that one of mechanisms of relaxation in the tracheal smooth muscle is via the blockade of external calcium entry similar to the effect exhibited by nifedipine, a L-type calcium channel blocker.
To identify the bronchodilator components, the CWE was fractionated using Sephadex G-50 gel filtration chromatography into HMW, MMW and LMW fractions. We have successfully demonstrated that both HMW and MMW fractions exhibited higher efficacy in relaxing the pre-contracted airways when compared to CWE and LMW fraction. This demonstrates that the bioactive components responsible for the airway relaxation effects are large molecular weight substances present mostly in the HMW and MMW fractions. Responses of the LMW fraction were similar to the vehicle control thus indicating no significant bioactive components are present in this fraction.
Activation of Gαq-protein-coupled M3 and 5-HT2A receptors leads to an overall increase in the intracellular Ca2+ free concentration which results in airway smooth muscle contractions. We showed that pre-incubation with the HMW fraction inhibits the contraction of the airways to both carbachol and 5-HT to a similar degree. The HMW fraction abolished the carbachol- and 5-HT-induced contractions in a non-competitive manner. This suggests that the HMW fraction non-selectively inhibits the downstream mechanism of activated Gαq-coupled receptors. Our postulation is based on our earlier work which showed that carbachol-induced contraction in the trachea is largely dependent on extracellular Ca2+ entry (unpublished data) with little contribution from intracellular Ca2+ stores. Following these observations, we subsequently investigated the effect of the CWE and its fractions on external Ca2+ entry. In the absence of extracellular Ca2+, addition of KCl depolarizes the smooth muscle cell membrane and opens voltage-gated Ca2+ channels, resulting in an influx of Ca2+ upon calcium re-introduction. Nifedipine, a selective voltage-gated Ca2+ channel blocker, was chosen as a positive control in this study to confirm the involvement of these channels in the contraction elicited following re-introduction of calcium. The effectiveness of the HMW fraction in blocking the calcium-induced contraction was similar to nifedipine thus implying the HMW fraction may be blocking voltage-gated Ca2+ channels. This is supported by observations from an earlier calcium imaging study showing that CWE (likely HMW fraction as well) inhibits extracellular Ca2+ influx into rat dorsal root ganglion cells (Lee et al., 2018). Given this evidence, we conclude that one of the mechanisms by which the CWE induces airway relaxation is linked to regulation of calcium signalling in response to Gαq-protein-coupled receptor activation. We are not excluding the possibility that the CWE or the HMW fraction might also influence other regulators that modulate smooth muscle contractility such as IP3, DAG, protein kinase C, and Rho kinase. The precise mechanisms of the CWE and its active fractions remain to be elucidated.
Our observations lead us to postulate that treatment with L. rhinocerotis may confer protection against acute respiratory tract exacerbations and that it has the potential to preserve airways patency. Our results also showed that the efficacy of 2.5 mg/ml CWE and HMW fraction is comparable to or even better than that of 30 nM ipratropium in inhibition of carbachol-induced airway contraction.
The biological activity of other types of medicinal mushroom, in particular the immunomodulatory activity, has been associated with the HMW polysaccharide or polysaccharide–protein complex found in the mushroom extract (Tzianabos, 2000; El Enshasy and Hatti-Kaul, 2013). Oral application of a polysaccharide fraction with a highly branched arabinogalactan protein derived from a medicinal plant, Terminalia chebula, displayed antitussive properties in TRIK strain guinea pigs (Nosalova et al., 2013).
In this study, the largest amount of carbohydrate and protein was found in the HMW fraction (71 and 4%, respectively), followed by the MMW fraction (35 and 1%, respectively) and the LMW fraction (22 and 0.3%, respectively). As the LMW fraction containing an insignificant amount of protein did not display any bronchodilator properties at the concentration tested our results strongly suggest that the polysaccharide–protein complex or proteins found in the HMW and MMW fractions are likely to contribute to the bronchorelaxation effects of the CWE. This is in agreement with another published study using the same TM02 cultivar where the HMW fraction exhibited the greatest anti-inflammatory activity and anti-proliferative properties against human lung and breast cancer cells (Lee et al., 2014).
Our monosaccharide profile analysis revealed that glucose is the most abundant sugar in the CWE and all its fractions. The amount of galactose and mannose corresponds with the bronchorelaxation activities; both sugars are found in the highest amount in the bioactive HMW fraction, followed by CWE. However, mannose does not seem to contribute to the bronchodilator effect as the MMW fraction is devoid of mannose but able to produce pronounced bronchorelaxation similar to the HMW fraction. Furthermore, mannose, but not galactose, is found in the LMW fraction. We postulate that the glucose and galactose consistently found in the HMW fraction, the MMW fraction, and the CWE appeared to be the important residues in the glycosidic linkages of the polysaccharide–protein complex contributing to the bronchorelaxation activities. Recently, a novel polysaccharide–protein complex was isolated from the hot-water-soluble polysaccharide fraction of P. rhinocerus and chemically characterised, indicating that glucose and mannose are the predominant carbohydrate moieties in the polysaccharide–protein complex with antitumor activity (Liu et al., 2016). The slight variations in the sugar composition between our study and the previous report might be attributed to the differences in extraction method, cultivar and geographical origins. In addition, in contrary with the previous findings by Liu et al. (2016), our preliminary experiments with the hot water extract of L. rhinocerotis have showed no bronchorelaxation effect (unpublished data), suggesting that the manno-glycoprotein linkages in the polysaccharide–protein complex are not likely to contribute to the bronchorelaxation effect.
Conclusion
This is the first study to report the direct relaxation effects of active fractions derived from water-soluble sclerotial extract from L. rhinocerotis on airway segments from rats. This study confirms that the bioactive components are the HMW polysaccharide–protein complexes or proteins found in HMW and MMW fractions. The important sugar moieties are glucose and galactose. One of the mechanisms of relaxation is likely to be due to blockade of extracellular Ca2+ influx through the membrane calcium channels downstream of Gαq-protein-coupled receptors. Further investigations are required to identify and elucidate the structure of the large molecular weight bioactive components from the sclerotium of L. rhinocerotis for their potential therapeutic application as bronchodilators.
Author Contributions
SYF, KNT, RR, and PCKC conceived and designed the experiments. MKL, XJL, and ACSY performed the experiments and analyzed the data. STN, CST, KNT, PCKC, and SYF contributed reagents/materials/analysis tools. MKL, SYF, KNT, and PCKC wrote the paper. All authors agreed to be accountable for all aspects of the work in ensuring that questions related to the accuracy or integrity of any part of the work are appropriately investigated and resolved.
Funding
This research was supported by Ministry of Higher Education Malaysia (Grant No. FRGS/1/2014/SKK03/UNIM/02/1) and UMRG Program (Grant No. RP034A,B,C-17AFR) from University of Malaya. MKL receives a scholarship for her Ph.D. studies from the University of Nottingham Malaysia Campus. ACSY was funded by Special Assistance (Grant No. BKS045-2017) and UMRG Program (Grant No. RP034A,B,C-17AFR) from University of Malaya.
Conflict of Interest Statement
LiGNO Biotech Sdn Bhd supplied the sclerotial powder of Lignosus rhinocerotis. The authors declare that the research was conducted in the absence of any commercial or financial relationships that could be construed as a potential conflict of interest.
Acknowledgments
The authors would like to thank Dr. Sue Mian Then for statistical advice.
Supplementary Material
The Supplementary Material for this article can be found online at: https://www.frontiersin.org/articles/10.3389/fphar.2018.00461/full#supplementary-material
Abbreviations
CWE, cold water extract; HMW, high-molecular-weight; LMW, low-molecular-weight; MMW, medium-molecular-weight.
References
Abdullah, N., Haimi, M. Z. D., Lau, B. F., and Annuar, M. S. M. (2013). Domestication of a wild medicinal sclerotial mushroom, Lignosus rhinocerotis (Cooke) Ryvarden. Ind. Crops Prod. 47, 256–261. doi: 10.1016/j.indcrop.2013.03.012
Azliza, M. A., Ong, H. C., Vikineswary, S., Noorlidah, A., and Haron, N. W. (2012). Ethno-medicinal resources used by the Temuan in Ulu Kuang village. Stud. Ethno Med. 6, 17–22. doi: 10.1080/09735070.2012.11886415
Barnes, P. J., and Adcock, I. M. (2003). How do corticosteroids work in asthma? Ann. Intern. Med. 139, 359–370. doi: 10.7326/0003-4819-139-5_Part_1-200309020-00012
Bradford, M. M. (1976). A rapid and sensitive method for the quantitation of microgram quantities of protein utilizing the principle of protein-dye binding. Anal. Biochem. 72, 248–254. doi: 10.1016/0003-2697(76)90527-3
Cazzolau, M., Matera, M. G., D’Amato, G., and Rossi, F. (1995). Effects of serotonin on airways: recent developments. Allergy 50, 1–10. doi: 10.1111/j.1398-9995.1995.tb02476.x
Cooper, P. R., Kurten, R. C., Zhang, J., Nicholls, D. J., Dainty, I. A., and Panettieri, R. A. (2011). Formoterol and salmeterol induce a similar degree of β2-adrenoceptor tolerance in human small airways but via different mechanisms. Br. J. Pharmacol. 163, 521–532. doi: 10.1111/j.1476-5381.2011.01257.x
Cukic, V., Lovre, V., Dragisic, D., and Ustamujic, A. (2012). Asthma and chronic obstructive pulmonary disease (COPD) – Differences and similarities. Mater. Sociomed. 24, 100–105. doi: 10.5455/msm.2012.24.100-105
DuBois, M., Gilles, K. A., Hamilton, J. K., Rebers, P. A., and Smith, F. (1956). Calorimetric method for determination of sugars and related substances. Anal. Chem. 28, 350–356. doi: 10.1021/ac60111a017
Dupont, L. J., Pype, J. L., Demedts, M. G., De Leyn, P., Deneffe, G., and Verleden, G. M. (1999). The effects of 5-HT on cholinergic contraction in human airways in vitro. Eur. Respir. J. 14, 642–649. doi: 10.1034/j.1399-3003.1999.14c26.x
El Enshasy, H. A., and Hatti-Kaul, R. (2013). Mushroom immunomodulators: unique molecules with unlimited applications. Trends Biotechnol. 31, 668–677. doi: 10.1016/j.tibtech.2013.09.003
Erle, D. J., and Sheppard, D. (2014). The cell biology of asthma. J. Cell Biol. 205, 621–631. doi: 10.1083/jcb.201401050
Huang, N. L. (1999). Identification of the scientific name of Hurulingzhi. Acta Edulis Fungi 6, 30–32.
Lai, C. K. M., Wong, K. H., and Cheung, P. C. K. (2008). Antiproliferative effects of sclerotial polysaccharides from Polyporus rhinocerus Cooke (Aphyllophoromycetideae) on different kinds of leukemic cells. Int. J. Med. Mushrooms 10, 255–264. doi: 10.1615/IntJMedMushr.v10.i3.60
Lau, B. F., Abdullah, N., and Aminudin, N. (2013). Chemical composition of the tiger’s milk mushroom, Lignosus rhinocerotis (Cooke) Ryvarden, from different developmental stages. J. Agric. Food Chem. 61, 4890–4897. doi: 10.1021/jf4002507
Lau, B. F., Abdullah, N., Aminudin, N., Lee, H. B., and Tan, P. J. (2015). Ethnomedicinal uses, pharmacological activities, and cultivation of Lignosus spp. (tiger’s milk mushrooms) in Malaysia - A review. J. Ethnopharmacol. 169, 441–458. doi: 10.1016/j.jep.2015.04.042
Lee, M.-K., Lim, K.-H., Millns, P., Mohankumar, S. K., Ng, S.-T., Tan, C.-S., et al. (2018). Bronchodilator effects of Lignosus rhinocerotis extract on rat isolated airways is linked to the blockage of calcium entry. Phytomedicine 42, 172–179. doi: 10.1016/j.phymed.2018.03.025
Lee, M. L., Tan, N. H., Fung, S. Y., Tan, C. S., and Ng, S. T. (2012). The antiproliferative activity of sclerotia of Lignosus rhinocerus (tiger milk mushroom). Evid. Based Complement. Altern. Med. 2012:697603. doi: 10.1155/2012/697603
Lee, S. S., Tan, N. H., Fung, S. Y., Sim, S. M., Tan, C. S., and Ng, S. T. (2014). Anti-inflammatory effect of the sclerotium of Lignosus rhinocerotis (Cooke) Ryvarden, the tiger milk mushroom. BMC Complement. Altern. Med. 14:359. doi: 10.1186/1472-6882-14-359
Lee, Y.-T., Lee, S.-S., Sun, H.-L., Lu, K.-H., Ku, M.-S., Sheu, J.-N., et al. (2013). Effect of the fungal immunomodulatory protein FIP-fve on airway inflammation and cytokine production in mouse asthma model. Cytokine 61, 237–244. doi: 10.1016/j.cyto.2012.09.024
Liu, C., Chen, J., Chen, L., Huang, X., and Cheung, P. C. K. (2016). Immunomodulatory activity of polysaccharide–protein complex from the mushroom sclerotia of Polyporus rhinocerus in murine macrophages. J. Agric. Food Chem. 64, 3206–3214. doi: 10.1021/acs.jafc.6b00932
Loong, B. J., Tan, J. H., Lim, K. H., Mbaki, Y., and Ting, K. N. (2015). Contractile function of smooth muscle retained after overnight storage. Naunyn Schmiedebergs Arch. Pharmacol. 388, 1061–1067. doi: 10.1007/s00210-015-1140-3
Moulton, B. C., and Fryer, A. D. (2011). Muscarinic receptor antagonists, from folklore to pharmacology; finding drugs that actually work in asthma and COPD. Br. J. Pharmacol. 163, 44–52. doi: 10.1111/j.1476-5381.2010.01190.x
Nosalova, G., Jurecek, L., Chatterjee, U. R., Majee, S. K., Nosal, S., and Ray, B. (2013). Antitussive activity of the water-extracted carbohydrate polymer from Terminalia chebula on citric acid-induced cough. Evid. Based Complement. Altern. Med. 2013:650134. doi: 10.1155/2013/650134
Proskocil, B. J., and Fryer, A. D. (2005). Beta2-agonist and anticholinergic drugs in the treatment of lung disease. Proc. Am. Thorac. Soc. 2, 305–310; discussion 311–2. doi: 10.1513/pats.200504-038SR
Tan, C. S. (2009). Setting-up Pilot-Plant for Up-scaling Production of “Tiger-Milk” Mushroom as Dietary Functional Food. Technical Report, MOA TF0109M004. Kuala Lumpur: Government of Malaysia.
Tan, C. S., Ng, S. T., Vikneswary, S., Lo, F. P., and Tee, C. S. (2010). Genetic markers for identification of a Malaysian medicinal mushroom, Lignosus rhinocerus (Cendawan Susu Rimau). Acta Hortic. 859, 161–167. doi: 10.17660/ActaHortic.2010.859.19
Tzianabos, A. O. (2000). Polysaccharide immunomodulators as therapeutic agents: structural aspects and biologic function. Clin. Microbiol. Rev. 13, 523–533. doi: 10.1128/CMR.13.4.523-533.2000
Yap, H. Y. Y., Fung, S. Y., Ng, S. T., Tan, C. S., and Tan, N. H. (2015). Shotgun proteomic analysis of tiger milk mushroom (Lignosus rhinocerotis) and the isolation of a cytotoxic fungal serine protease from its sclerotium. J. Ethnopharmacol. 174, 437–451. doi: 10.1016/j.jep.2015.08.042
Keywords: tiger milk mushroom, Lignosus rhinocerotis, bronchorelaxation, airway diseases, aqueous extract, fractions, medicinal mushroom
Citation: Lee MK, Li X, Yap ACS, Cheung PCK, Tan CS, Ng ST, Roberts R, Ting KN and Fung SY (2018) Airway Relaxation Effects of Water-Soluble Sclerotial Extract From Lignosus rhinocerotis. Front. Pharmacol. 9:461. doi: 10.3389/fphar.2018.00461
Received: 18 December 2017; Accepted: 19 April 2018;
Published: 07 May 2018.
Edited by:
Adolfo Andrade-Cetto, Universidad Nacional Autónoma de México, MexicoReviewed by:
Aslam Khan, King Saud bin Abdulaziz University for Health Sciences, Saudi ArabiaTobias Piegeler, Universitätsklinikum Leipzig, Germany
Copyright © 2018 Lee, Li, Yap, Cheung, Tan, Ng, Roberts, Ting and Fung. This is an open-access article distributed under the terms of the Creative Commons Attribution License (CC BY). The use, distribution or reproduction in other forums is permitted, provided the original author(s) and the copyright owner are credited and that the original publication in this journal is cited, in accordance with accepted academic practice. No use, distribution or reproduction is permitted which does not comply with these terms.
*Correspondence: Shin Yee Fung, ZnVuZ3NoaW55ZWVAZ21haWwuY29t; c3lmdW5nQHVtLmVkdS5teQ== Kang Nee Ting, S2FuZy1OZWUuVGluZ0Bub3R0aW5naGFtLmVkdS5teQ==