- 1School of Pharmacy, Nantong University, Nantong, China
- 2Jiangsu Collaborative Innovation Center of Chinese Medicinal Resources Industrialization, Nanjing University of Chinese Medicine, Nanjing, China
Studies have indicated that Chrysanthemum polysaccharides (CP) could prominently ameliorate colitis rats, but its possible mechanism remains unclear. In this study, the underlying mechanism of CP was explored by the metabolic profiling analysis and correlated signaling pathways. TNBS/ethanol induced colitis was used to investigate the intervention efficacy following oral administration of CP. The levels of cytokines such as TNF-α, IL-6, IFN-γ and IL-1β, and the activities of SOD, MPO, and MDA were determined. We also performed western-blot for p65, TLR4, p-JAK2, and STAT3 protein expression in the colon tissue to probe their mechanisms of correlated signaling pathways. What’s more, the metabolic changes in plasma and urine from colitis rats were investigated based on UPLC-Q-TOF/MS combined with MetabolynxTM software. The potential biomarkers and metabolic pathways were also tentatively confirmed. The metabolic profiles of plasma and urine were clearly improved in model rats after oral administration of CP. Thirty-two (17 in serum and 15 in urine) potential biomarkers were identified. The endogenous metabolites were mainly involved in linoleic acid, retinol, arachidonic acid, glycerophospholipid and primary bile acid metabolism in plasma, and nicotinate and nicotinamide, ascorbate and aldarate, histidine and β-alanine metabolism in urine. After polysaccharides intervention, these markers turned back to normal level at some extent. Meanwhile, the elevated expression levels of pp65, TLR4, p-STAT3, and p-JAK2 were significantly decreased after treatment. Results suggested that CP would be a potential prebiotics for alleviation of TNBS-induced colitis. The study paved the way for the further exploration of the pathogenesis, early diagnosis and curative drug development of the colitis.
Introduction
Inflammatory bowel disease, which is characterized by chronic and progressive inflammation in the gastrointestinal tract, is a chronic recurring condition with a high incidence of morbidity, classified into two distinct clinical forms, ulcerative colitis and Crohn’s disease. IBD manifests some symptoms which includes weight loss, severe diarrhea, debilitating abdominal pain and bloody stools, leading to impairment in quality of life. Patients with IBD also have a significantly increased risk of having a related disease such as colon cancer (Pizzi et al., 2006; Walker et al., 2011). So far, the pathogeny of IBD remains unknown, although several factors have been related to the development of disease, including genetics, abnormal immune regulation, barrier dysfunction, environmental exposures and the changes of the gut microbial community (Packey and Sartor, 2008; Mar et al., 2014; Tao et al., 2017). Dysregulation of immune response of intestinal bacterial flora was associated with the development of IBD (Junghee et al., 2009). The innate immune system could recognize the presence of bacterial invasion through PRRs in the intestinal epithelial cells, and the major PRRs are TLRs (Fort et al., 2005). Out of all the TLRs, induction of TLR4 isoform is implicated in IBD pathogenesis (Venkatesh et al., 2014). Convincing researches have indicated that LPS, a major component of Gram-negative bacterial cytoderm, binds to TLR4 and triggers MyD88-mediated signaling cascades activation of NF-κB, and eventually leads to inflammatory response (Siddique and Khan, 2011; Jang et al., 2013). TLR4 is generally expressed at low level in the intestinal mucosa but is notably up-regulated in IBD patients and colitis rats which implied its important role in the pathogenesis of IBD (Sun et al., 2016). IBD is usually accompanied by a typical imbalance of pro-inflammatory and anti-inflammatory signaling pathways in the intestine. NF-κB is a major regulator of gene transcription in these pathways. The disorder of the NF-κB signaling pathway usually causes overzealous inflammation in patients with IBD (Tsang et al., 2015; Wang et al., 2017). Various inflammatory cytokine genes that involved in the etiologies of IBD, including IL-1β, IL-2, TNF-α and IL-6, have NF-κB binding sites and are transcriptionally regulated by NF-κB (Baldwin, 1996; Barnes and Karin, 1997).
The type of metabolites and changes in metabolic profiles could serve as good indicator of cytokines-mediated inflammatory processes in IBD. Metabolomics is a systems biology approach (Holmes et al., 2008; Su et al., 2015) that measures the dynamic multi-parametric metabolic responses of living systems to pathophysiologic stimuli or genetic modification (Nicholson et al., 2008) by the simultaneous and non-targeted analysis of dynamic changes of endogenous metabolites in complex biological matrices (Phua et al., 2013). Changes of metabolites with low molecular weight usually reflect the end-results of the genomic and protein perturbations in disease and are closely related to phenotypic changes. Additionally, through the identification of biomarkers, analysis of metabolic pathways, discovery of drug-target interactions, etc., it is also possible to elucidate the pathogenesis of diseases and the action mechanisms of therapy. Therefore, metabonomics has attracted an interest for investigating the IBD and evaluating drug treatment outcomes (Holmes et al., 2008; Schicho et al., 2010; Lin et al., 2011; Su et al., 2015).
Unfortunately, therapeutic options currently available for the treatment of IBD are largely limited to symptoms of the disease, with some exceptions to the molecular pathways associated with intestinal inflammation (Jobin, 2010; Okafor et al., 2013). Despite advances in therapeutics, some patients didn’t respond to treatment or suffered from side effects or complications (Dou et al., 2014). And so, recent researches have focused on the use of natural products or dietary supplements as alternatives to patients who are unresponsive or unwilling to take commonly medications (Recio et al., 2012). As a medicinal and edible homologous plant, chrysanthemum was reported to have strong heat-clearing and detoxifying ability, which can effectively treat infectious diseases and some inflammatory diseases such as influenza, colitis, stomatitis, and can also be used clinically to treat cancer (Kim et al., 2013; Luyen et al., 2015; Zheng et al., 2015; Tao et al., 2016, 2017). Our previous studies showed that CP have a significant protective effect on colitis mice by increasing SCFAs production (Tao et al., 2016). However, the mechanism of colonic fermentation is not yet clear, and the effect of CP on the intestinal microbiota is also limited (Tao et al., 2017).
In this study, TNBS/ethanol induced colitis was used to investigate the intervention efficacy after oral administration of CP. The levels of cytokines such as TNF-α, IFN-γ, IL-6 and IL-1β, and the activities of SOD, MDA, and MPO were determined. To elucidate the mechanism, the metabolic changes in plasma and urine from TNBS-induced colitis rats were investigated based on UPLC-Q-TOF/MS combined with MetabolynxTM software. The potential biomarkers and metabolic pathway were also tentatively identified. The data on the basis of this work would be useful to clarify the potential mechanism of CP to improve and alleviate colitis.
Materials and Methods
Animals
Male SD rats (220 ± 20 g) from the Vital River Laboratory Animal Technology Co., Ltd. (Beijing, China) were accommodated in the specific pathogen-free animal center with an air-conditioned animal quarter with 12 h light/12 h dark cycle and a constant temperature of 22 ± 2°C. The rats were allowed to acclimate for 7 days prior to experiments and were fed with standard food and water ad libitum.
Chemical Regents
2, 4, 6-trinitrobenzene sulfonic acid and sulfasalazine were bought from MP Biomedical (Aurora, OH, United States) and Yifeng Pharmacy (Nanjing, China), respectively. ELISA kits (IL-6, IL-1β, IFN-γ, and TNF-α) were purchased from Nanjing Jiancheng Bioengineering Institute Co., Ltd. CP were prepared according to our previous methods (Schicho et al., 2010; Tao et al., 2017).
Induction of Colitis Rat Model and Treatment Protocol
The rats were anesthetized with chloral hydrate and then administered with TNBS/ethanol solution into the colon from the rectum. 24 h later, all the rats were randomly assigned to six groups. N, receiving normal saline; M, receiving ethanol vehicle with TNBS; PP, receiving SASP 0.5 g/kg; HP, MP and LP, receiving CP 200, 100, and 50 mg/kg, respectively (Scheiffele and Fuss, 2002; Tao et al., 2017) (the details were listed in Supplementary Text S1).
Sample Collection and Preparation
After the last treatment day, all experimental rats were put in the metabolism cages for urine collection, and blood samples were gathered into the tubes containing EDTA-2Na on the 16th day from carotid artery after ether deep anesthesia. After collection of urine and blood, all rats were sacrificed. The colon was dissected and incised longitudinally along the mesenterium, rinsed with saline, and then used to assess colon mucosal damage and biochemical tests.
The urine and blood samples were thawed under room temperature prior to analysis. 200 μL of plasma and urine were, respectively, added to 600 μL of acetonitrile, vortexed for 30 s and centrifuged at 13,000 g at 4°C for 10 min to obtain a supernatant. The plasma and urine supernatants were evaporated to dryness in a 37°C water bath under a gentle stream of nitrogen. The residues were reconstituted in 200 μL of a 70% acetonitrile-water solution mobile phase, filtered through a 0.22 μm membrane filter for UPLC/MS analysis (Su et al., 2015).
UPLC-Q-TOF/MS Analysis Conditions
Metabolite separation was performed using a Waters AcquityTM UPLC system equipped with a Waters XevoTM G2 Q/TOF-MS. 2 μL sample solution was injected into an ACQUITYTM UPLC BEH C18 (100 mm × 2.1 mm, 1.7 μm) with the flow rate was 0.4 mL/min at 35°C. The optimal mobile phase consisted of water (A) 0.1% formic acid and (B) acetonitrile. The optimized UPLC elution conditions for serum were: 0 ∼ 3 min, 95% ∼ 55% A; 4 ∼ 13 min, 55% ∼ 5% A; 13 ∼ 14 min, 5% A; The optimized UPLC elution conditions for urine were: 0 ∼ 8 min, 95% ∼ 70% A; 8 ∼ 11 min, 70% ∼ 30% A; 11 ∼ 13 min, 30% ∼ 5% A; 13 ∼ 14 min, 5% A.
Mass spectrometry detection was performed using a quadrupole and orthogonal acceleration time-of-flight tandem mass spectrometer. Data were collected in centroid mode from 100 to 1000 m/z. For both positive and negative electrospray modes, the capillary and cone voltage were set at 3.0 kV and 30 V, respectively. The desolvation gas was set to 600 L/h at a temperature of 350°C, the cone gas was set to 50 L/h and the source temperature was set to 120°C (Zhao et al., 2012).
Determination of Cytokines (TNF-α, IFN-γ, IL-6, and IL-1β)
50 mg of colon tissue was extracted with 500 μL of guanidine hydrochloride and Tris-HCl (50 mM, pH 8.0) containing protease inhibitors. The extracts were then centrifuged at 13,000 g for 10 min at 4°C. The cytokines were then analyzed by ELISA kits using the supernatant fraction according to the manufacturer’s instructions.
MPO, SOD, and MDA Assay
The MPO, SOD, and MDA activity was determined according to the respective assay kit. The supernatant of colon tissue were obtained by the method described above.
Myeloperoxidase which is often over expressed in numerous inflammatory diseases, including IBD (Lau and Baldus, 2006; Pattison and Davies, 2006), has the potential to serve as a viable, non-invasive fecal biomarker for assessing IBD status. MDA is a product of lipid peroxidation and degradation, which reacts with TBA to form a red product that absorbs at a wavelength of 532 nm (Su et al., 2015).
Metabolomic Data Processing and Multivariate Data Analysis
All of the UPLC/MS data acquisition and analyses were controlled by Waters Mass Lynx v4.1 and MarkerLynx software. The multivariate data matrix was analyzed using EZinfo software. Unsupervised segregation was examined with a PCA using Pareto-scaled data. A PLS-DA and an OPLS-DA were used to identify the various meta∖bolites responsible for the separation between the normal and model groups (Su et al., 2013). Potential biomarkers were extracted from the S-plots that were constructed following the OPLS-DA analysis, and the biomarkers were chosen based on their contribution to the variation and correlation within the dataset. The VIP in the projection value was a weighted sum of squares of the PLS weights, and the variables with VIP > 1 were considered to be influential for the separation of samples in the score plots generated from PLS-DA analysis (Salliot and Van, 2009; Su et al., 2015).
Biomarker Identification and Metabolic Pathway Analysis
Potential biomarkers were corroborated by comparing their chromatographic retention times, mass spectra with the standards and a full spectral library with MS/MS data acquired in the negative and/or positive ion modes. MS/MS fragment ions were analyzed by the mass fragment application manager MassLynx v4.1with chemically intelligent peak-matching algorithms. Then, based on the above information, multiple databases were searched and potential metabolic pathways were analyzed by METLIN1, Human Metabolome Database2, and MetPA3 based on the KEGG4.
Quantitative Real-Time Polymerase Chain Reaction (qPCR)
Total RNA was isolated from colon tissues using Trizol reagents following the manufacturer’s specifications [TranScript® All-in-One First-Strand cDNA Synthesis SuperMix for qPCR (One-Step gDNA Removal)]. Real-time quantitative PCR was performed using SYBR Green Master mix and Rox reference dye. Transcript levels were quantified by the ΔΔCt value method (Pan et al., 2000; Su et al., 2015). Calculation was done using the Ct value of GAPDH to normalize the Ct value of target genes in each sample to obtain the ΔΔCt value, which was then used to compare among different samples (Huang et al., 2016). PCR products were analyzed by gel electrophoresis on 1.5% agarose gel and the specificity of amplification was confirmed by the melting curves. The primer sequences of target genes were listed as Supplementary Table S1.
Western Blot Analysis for NF-κB p65, pp65, TLR4, STAT3, p-STAT3, and JAK2
Colonic tissues were disrupted by homogenization in lysis buffer containing protease inhibitor and the supernatants (total protein) were collected after centrifugation. The protein lysates were separated by 10% SDS-PAGE, transferred to PVDF membranes, and blocked with 0.05% (w/v) Tween 20 and 5% (w/v) BSA over night at 4°C. The immunoblots were incubated with primary antibodies at 1:1000 dilution with 1% BSA for 3 h at 4°C. Subsequently, the immunoblots were incubated with the secondary antibody conjugated with IgG-HRP at room temperature for 1 h and then washed with TBST in triplicate, and then the bands were detected using chromogenic substrate. β-actin was used as loading control.
Statistical Analysis
The experimental data were analyzed by GraphPad PrismV5 and the results were expressed as mean ± standard deviation. Comparison of the mean number of groups using one-way ANOVA and Tukey’s true significance difference (HSD) post hoc test (Wu et al., 2016; Tao et al., 2017).
Results
Effection of Chrysanthemum Polysaccharides on TNBS-Induced Colitis
This study established a TNBS-induced colitis model and was successfully used to evaluate the amelioration of CP (Tao et al., 2017). Physiological and pathological changes of colitis rats after oral administration of CP have been detailly described in our previous reported protocol (Supplementary Text S1 and Supplementary Figure S1). The above results indicated that CP strongly protected and notably alleviated the TNBS-induced colitis (Tao et al., 2016).
Effects of CP on MPO, SOD, and MDA Activities in Colon Tissues
Myeloperoxidase is an enzyme which found primarily in neutrophils and has been used as a quantitative indicator of inflammation and neutrophil infiltration in the colonic mucosa. The decrease of MPO activity could be interpreted as the performance of the anti-inflammatory properties (Xing et al., 2012; Chen et al., 2015). In this study, as shown in Figure 1A, compared to the normal group, the activity of MPO was dramatically increased (15.31 ± 2.32 U/g vs. 7.62 ± 1.03 U/g) in TNBS-induced colitis rats (p < 0.001). However, CP with different doses (high dosage, HP 200 mg/kg, middle dosage, MP 100 mg/kg, low dosage, LP 50 mg/kg) and SASP (0.5 g/kg) could significantly inhibit the activity of MPO in the model rats.
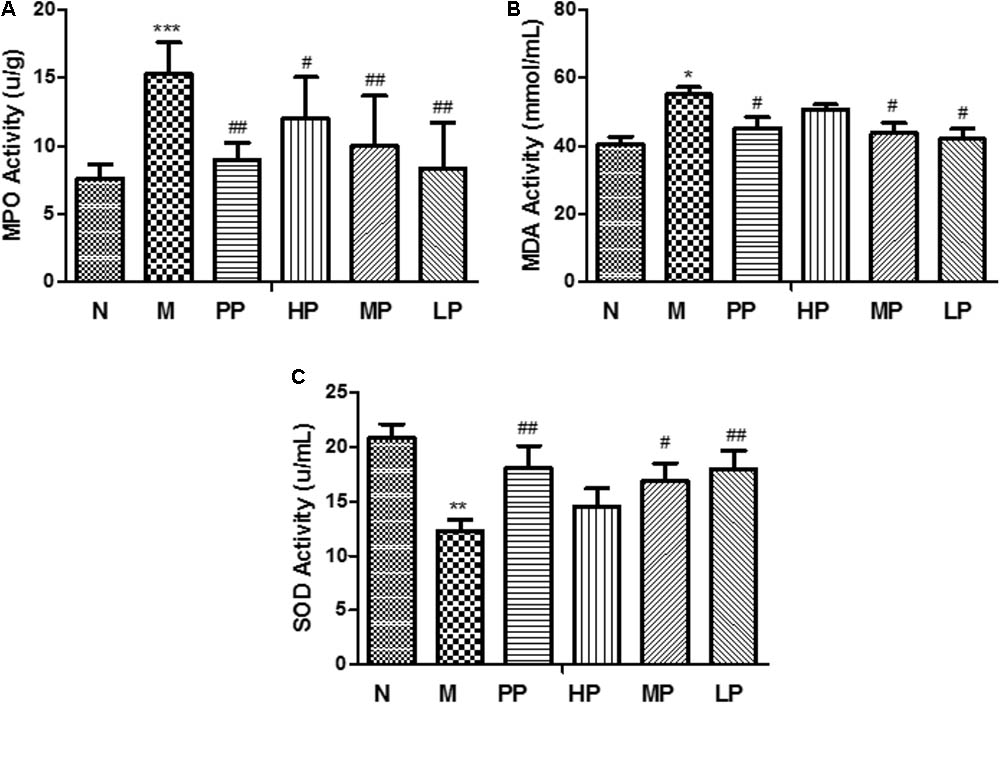
FIGURE 1. MPO (A), SOD (B), and MDA (C) activities in colonic tissue of different rats. N vs. M, ∗p < 0.05, ∗∗p < 0.01, and ∗∗∗p < 0.001. PP, HP, MP, LP vs. M, #p < 0.05 and ##p < 0.01.
Malondialdehyde is a peroxidation product by the attack of free radicals to lipids and its level represents the intensity of body injury (Su et al., 2015). The levels of MDA in colonic tissue had the same trends with the activity of MPO in colitis rats than that of normal rats, and interventional effects of CP were seen in Figure 1B.
Superoxide dismutase is an important antioxidant enzyme in organism, which can effectively scavenge oxygen free radicals and inhibit lipid in colon tissue qualitative peroxidation, and can stabilize the cell membrane and produce specific responses to certain inflammatory stimuli. The level of SOD is the main indicator to reflect the function of cell membrane and the body’s anti-inflammatory reaction (Halliwell, 1996). The SOD activity in colonic tissue indicates the intensity of the antioxidant defense system in UC. Tissue SOD may protect against oxidative stress caused by ROS. Because the antioxidant defense system in chronically inflamed tissues is impaired, this action might confer susceptibility to DNA damage and mutation, resulting in the development of carcinoma (Korenaga et al., 2002). In this study, the SOD activity was obviously decreased after TNBS induction compared with normal group (12.33 ± 1.01 vs. 20.86 ± 1.24 U/mL). After oral administration of with middle and low doses of CP, SOD activity was recovered notably (p < 0.05) (Figure 1C).
Effects of CP on the Levels of TNF-α, IFN-γ, IL-6, and IL-1β
As depicted in Figure 2, TNBS/ethanol instillation significantly promoted the levels of TNF-α, IFN-γ, IL-6, and IL-1β in rats. In contrast with the colitis group, different doses of CP remarkably attenuated the levels of these pro-inflammatory cytokines in various degrees (p < 0.05), which indicated that CP effectively reduced the pro-inflammatory factors in colitis rats.
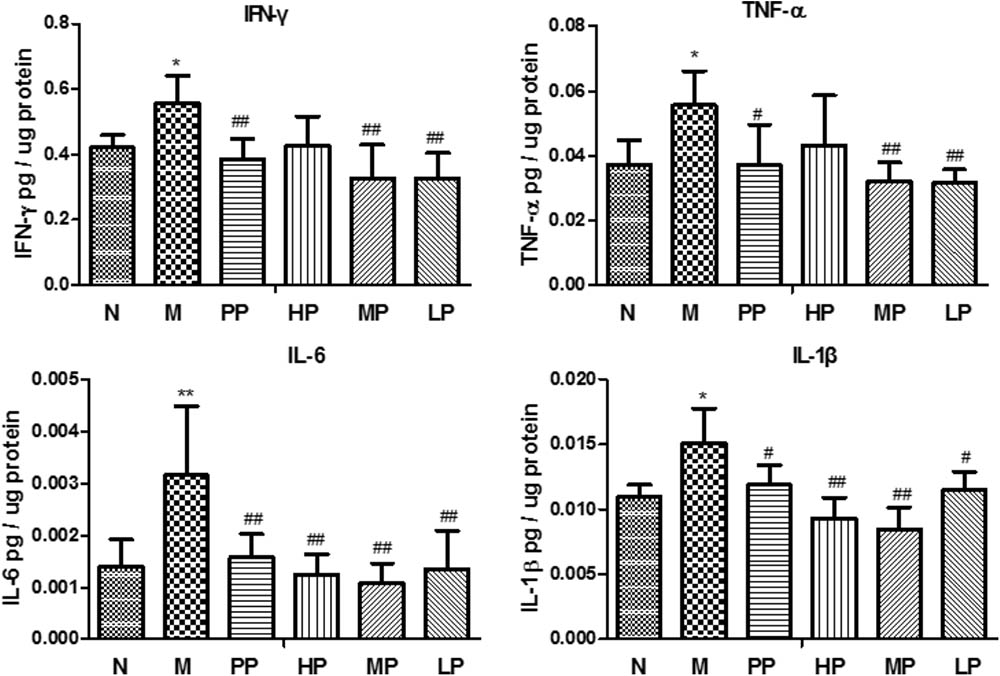
FIGURE 2. Chrysanthemum polysaccharides (CP) regulated pro-inflammatory cytokines in colons mucosa of different groups. Cytokine levels in colonic proteins were assessed by ELISA. All data were presented as mean ± SD. N = 6 for each group. N vs. M, ∗p < 0.05, ∗∗p < 0.01, and ∗∗∗p < 0.001. PP, HP, MP, LP vs. M, #p < 0.05, ##p < 0.01, and ###p < 0.001.
Chrysanthemum Polysaccharides Inhibited mRNA Levels of TLR4, NF-κB, IL-6, STAT3, and JAK2 in TNBS-Induced Colitis Rats
As shown in Figure 3A, compared with the normal group, mRNA levels of TLR4, NF-κB, IL-6, STAT3, and JAK2 in colitis rats remarkably increased (P < 0.001, P < 0.01). However, when treated with different dosages of CP (HP, 200 mg/kg, MP, 100 mg/kg, LP, 50 mg/kg) and Sulfasalazine (0.5g/kg), mRNA expressions of these factors decreased in varied degrees (Tao et al., 2017). The relative mRNA levels of NF-κB and IL-6 significantly decreased by each dosage of CP (P < 0.01, P < 0.001). The expressions of TLR4 and JAK2 mRNA were inhibited by MP and LP, respectively. Both MP and LP could reduce STAT3 mRNA notably (P < 0.05).
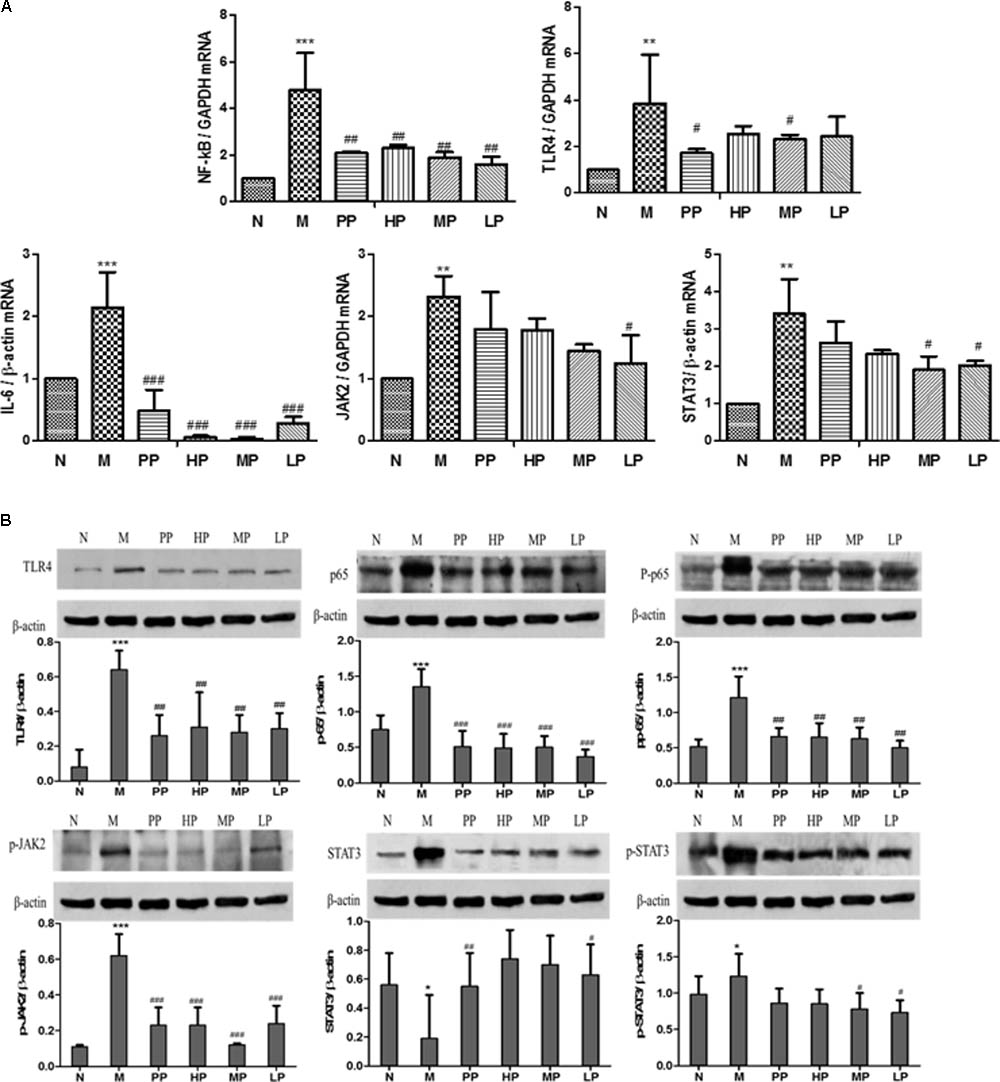
FIGURE 3. (A) Effects of CP on mRNA levels of TLR4, NF-κB, IL-6, STAT3, and JAK2 in TNBS-induced colitis. (B) Effects of CP on the expression of p65, pp65, TLR4, STAT3, p-STAT3, and JAK2 in colitis rats. N vs. M, ∗p < 0.05, ∗∗p < 0.01, ∗∗∗p < 0.001. PP, HP, MP, LP vs. M, #p < 0.05, ##p < 0.01, ###p < 0.001.
Chrysanthemum Polysaccharides Suppressed the Expression of p65, pp65, TLR4, STAT3, p-STAT3, and JAK2 in TNBS-Induced Colitis Rats
An attenuated trend of the expression of p65, pp65, TLR4, STAT3, p-STAT3, JAK2 in TNBS-induced colitis rats was observed in the groups treated with CP. Colitis rats exhibited a remarkable tendency to up-regulate the above target proteins (P < 0.05, P < 0.01), while CP could notably lower the levels of pp65, TLR4, p-STAT3, and p-JAK2 (P < 0.05, P < 0.01, P < 0.001). As shown in Figure 3B, the relative pp65, TLR4, and p-JAK2 levels in TNBS-induced colitis rats were diminished significantly after treatment of HP, MP, and LP. However, there was no significant change in the expression of STAT3 and p-65. The results above illustrated that CP could retrace the expression of target proteins in NF-κB/TLR4 and IL-6/JAK2/STAT3 signaling pathways.
LC-MS Analysis of Metabolic Profiling
All the data containing the retention time, peak intensity and exact mass were imported into the MasslynxTM software for multiple statistical analyses. Typical based peak intensity chromatograms of serum and urine samples, derived from normal controls (N) and colitis rats (M) in positive and negative modes, were presented as Supplementary Figure S2. Based on the data from the two groups, plasma or urine sample were divided into blocks between model and normal rats using the unsupervised PCA model to determine the metabolic changes and characterize the metabolite profile (Figures 4A–D). The supervised OPLS-DA with 100% sensitivity and no less than 95% specificity showed a better discrimination between the two groups (Figure 4), which demonstrated that the colitis model was successfully established. And subsequently, the OPLS-DA score plot (Figures 4E–H) was used to screen potential metabolites related to colitis progress (Cai et al., 2018).
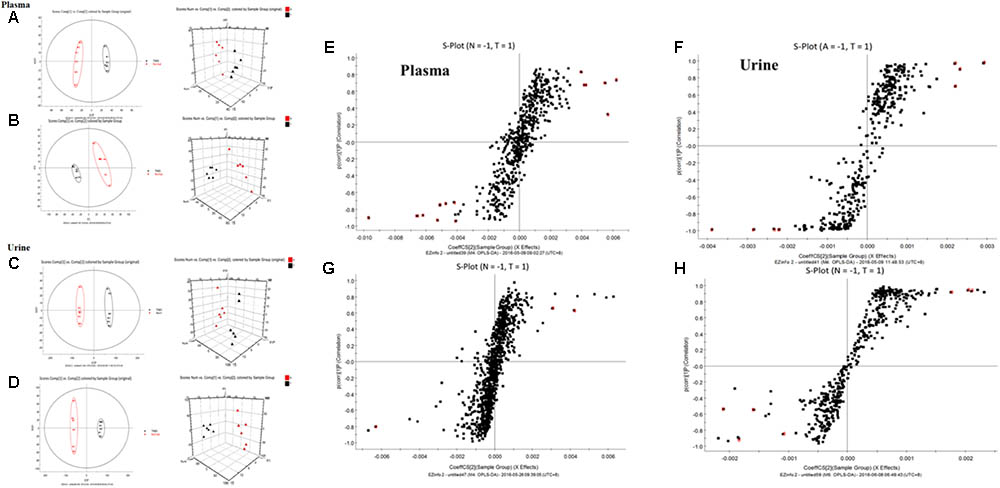
FIGURE 4. Principal component analysis (PCA) results between colitis and normal rats in positive and negative modes. (A) 2-D plot of plasma and 3D PLS-DA scores plot of LC-MS spectral data in ESI-. (B) 2-D plot of plasma and 3D PLS-DA scores plot of LC-MS spectral data in ESI+. (C) 2-D plot of urine and 3D PLS-DA scores plot of LC-MS spectral data in ESI-. (D) 2-D plot of urine and 3D PLS-DA scores plot of LC-MS spectral data in ESI+; S-plot of OPLS-DA model for colitis vs. normal group; (E) plasma, ESI-; (F) Urine, ESI-; (G) plasma, ESI+; (H) Urine, ESI+.
Identification of Potential Biomarkers and Intervention of Chrysanthemum Polysaccharides
The UPLC-Q-TOF/MS analysis provided the retention time, precise molecular mass and the fragments of corresponding production for the structural identification of metabolites. According to the precise molecular mass, the potential element composition was predicted and potential molecular formula could be searched out based on the KEGG5 and Human Metabolome Database6 (Cai et al., 2018). Thirty-two potential biomarkers (17 in serum and 15 in urine) were ultimately identified by comparing with authentic standards or based on the protocol detailed above method. The information about the detected endogenous metabolites was summarized in Table 1.
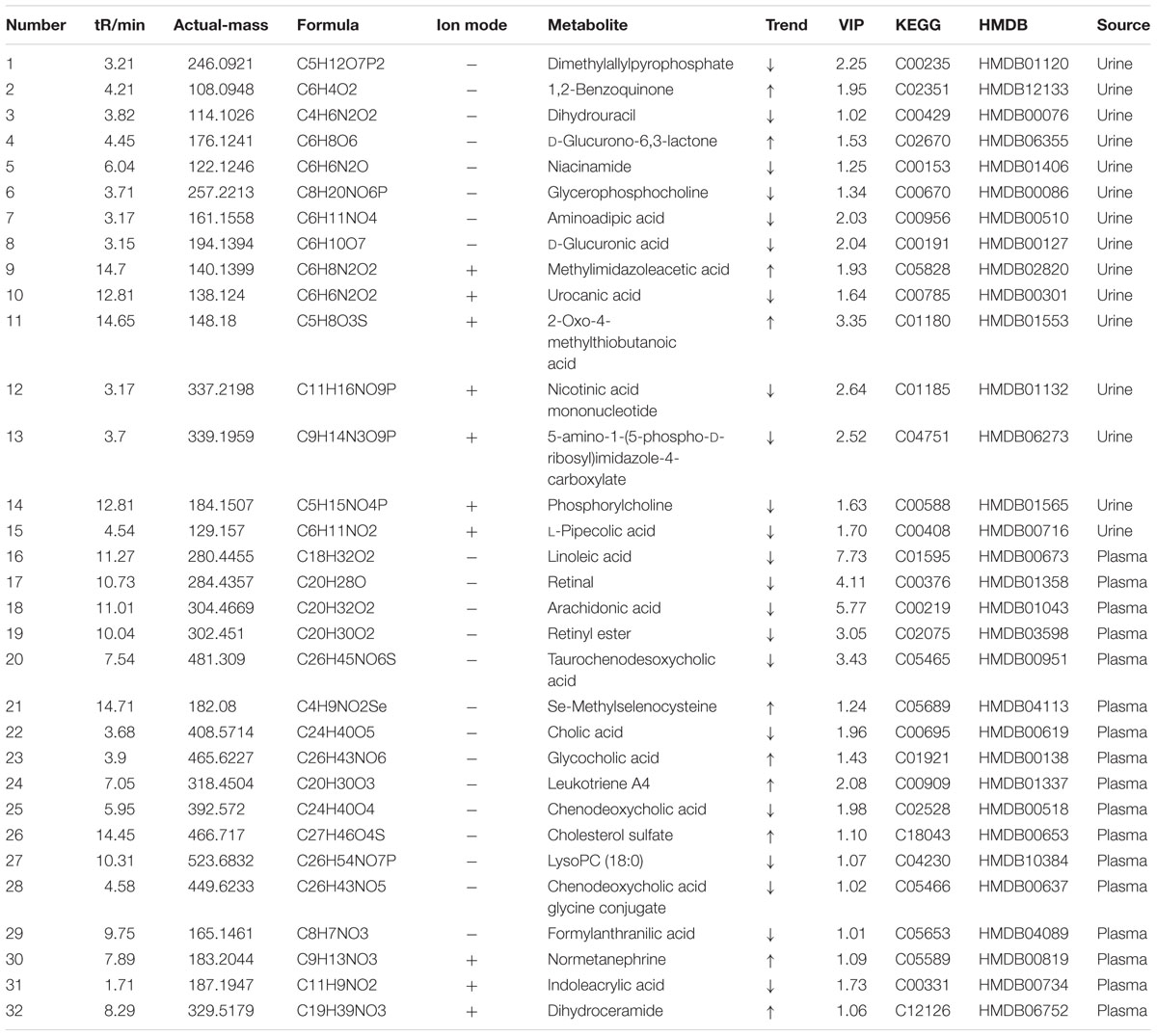
TABLE 1. The identified and change trend of the potential biomarkers between colitis and normal rats.
To investigate the amelioration and action mechanism of CP for curing colitis rats, PLS-DA model analysis was built to obtain the changes during the normal groups, TNBS-induced groups, SASP groups and administration groups. The variations of plasma and urine metabolic profiling of SASP groups and different dosages of CP were restored to the levels of normal controls in the varied degree (Figure 5). Furthermore, the relative quantities of eight potential biomarkers in plasma and four in urine were significantly affected by CP. Especially, the middle and low dosage groups were restored to a control-like level (Figure 6). These changes may not immediately in response to therapeutic effects of CP for the colitis rats, but they are produced by the perturbation of CP from the organism. The contents of the potential biomarkers in Table 1 were considered as biomarkers for therapeutic efficacy.
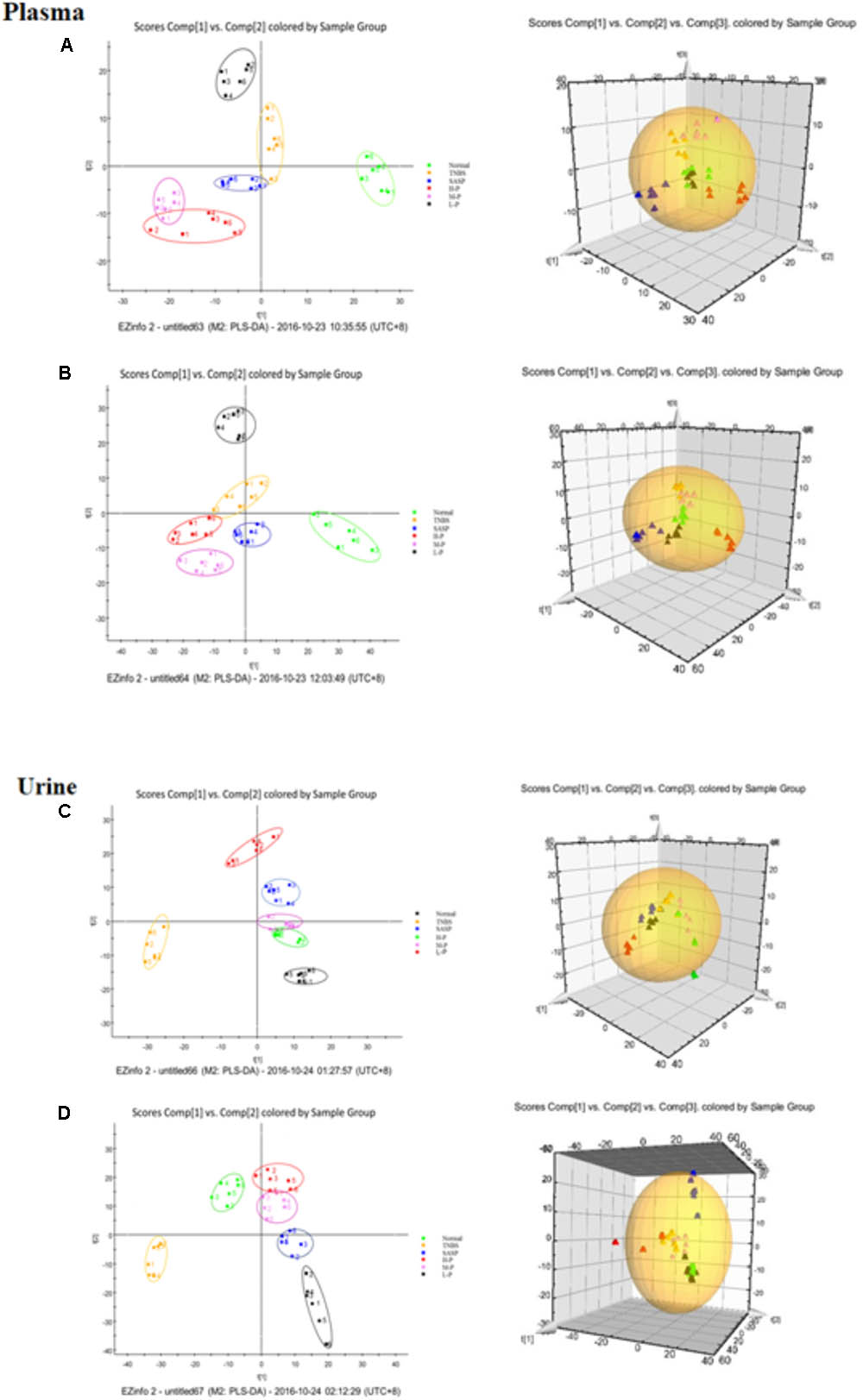
FIGURE 5. Partial least squares discriminant analysis (PLS-DA) plot in normal rats, colitis rats, SASP group and administration group rats. (A) plasma, ESI-; (B) plasma, ESI+; (C) urine, ESI-; (D) urine, ESI+.
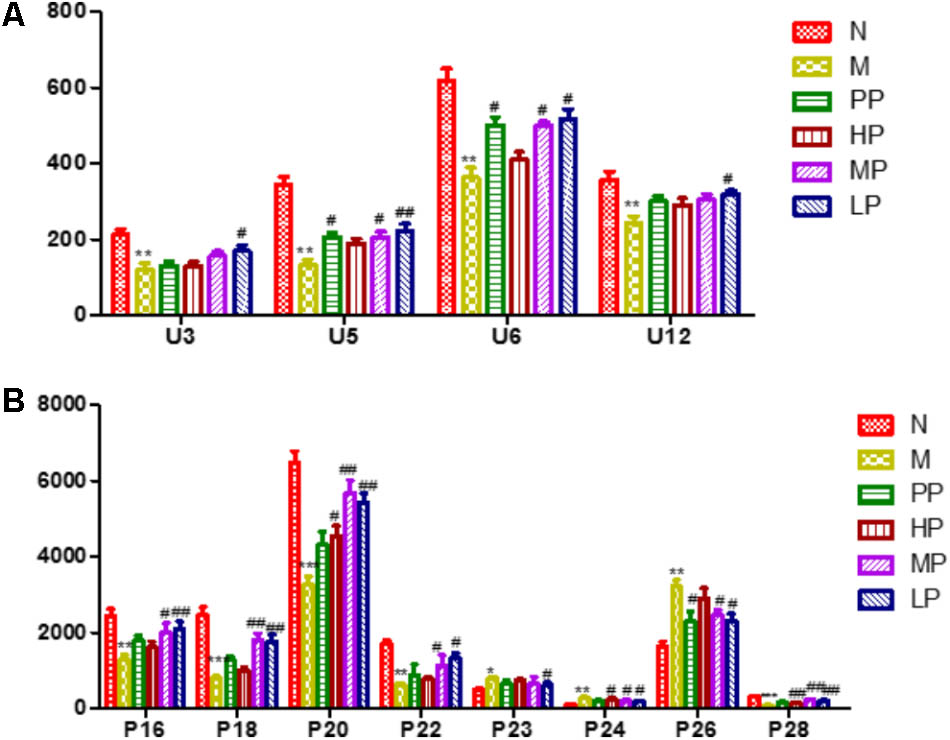
FIGURE 6. The relative quantities of target endogenous metabolites affected by CP. (A) Urine; (B) plasma. The corresponding markers represented to the Table 1. N vs. M, ∗p < 0.05, ∗∗p < 0.01, ∗∗∗p < 0.001. PP, HP, MP, LP vs. M, #p < 0.05, ##p < 0.01, ###p < 0.001.
The metabolic pathway was established by importing the potential metabolites into the web-based database MetPA. The pathway impact value calculated from pathway to topology analysis with MetPA above 0.1 was screened out as the potential target pathway (Cai et al., 2018). As shown in Figure 7A, among the eleven pathways in plasma, (1) Linoleic acid metabolism, (2) Retinol metabolism, (3) Arachidonic acid metabolism, (4) Glycerophospholipid metabolism and (5) Primary bile acid biosynthesis with the impact value 1.0, 0.41, 0.40, 0.14, and 0.10, respectively, were filtered out as the most important metabolic pathways. In Figure 7B, 15 pathways in urine mainly involving (1) Nicotinate and nicotinamide metabolism, (2) Ascorbate and aldarate metabolism, (3) Histidine metabolism and (4) β-Alanine metabolism with the impact value 0.41, 0.4, 0.15, and 0.13, respectively, were filtered out. Results indicated that these target pathways showed the marked perturbations over the time-course of the treatment and could contribute to development of colitis.
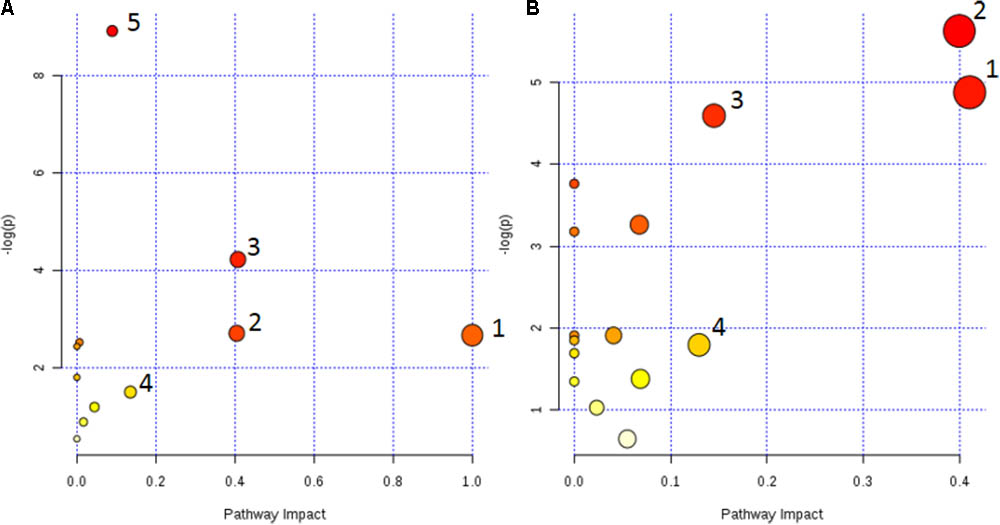
FIGURE 7. Metabolic pathways involved in potential markers in plasma and urine. (A) (1) Linoleic acid metabolism, (2) Retinol metabolism, (3) Arachidonic acid metabolism, (4) Glycerophospholipid metabolism, and (5) Primary bile acid biosynthesis. (B) (1) Nicotinate and nicotinamide metabolism, (2) Ascorbate and aldarate metabolism, (3) Histidine metabolism, and (4) β-Alanine metabolism.
Discussion
Inflammatory bowel disease is a chronic, relapsing disease of which the etiology remains essentially unclear. However, the results from many studies in humans and animal models indicate that it is related to an abnormal immune response in the gastrointestinal tract (Miao et al., 2015). At present, lots of researches have confirmed that the intestinal microflora dysbiosis is closely related to the gastrointestinal disease (Waidmann et al., 2003; Walker et al., 2011). Patients with gastrointestinal disease usually have the phenomenon of intestinal flora imbalance. Under the stimulation of various microbial antigens, the immune system in vivo is activated, resulting in imbalance of cytokine production and secretion, followed by activation of various inflammatory cells and further chronic inflammatory response of intestinal tissue (Danese and Fiocchi, 2006). Our previous studies have demonstrated that the intestinal flora structure of rats with colitis remarkably changed. The abundances of some Gram-negative pathogens such as Escherichia, Enterococcus, and Prevotella were elevated significantly, while some probiotics, such as Butyricicoccus, Clostridium, Lachnospiraceae, Lactobacillus, and Bifidobacterium were decreased (Tao et al., 2017). The break-down of the intestinal flora caused by TNBS inevitably has an important impact on the development of IBD. Based on the above findings, in the present study, we tried to investigate the molecular mechanism of CP on the improvement of the colitis.
Toll-like receptors are the recognition receptors of the innate immune system, which locate on the surface of various immune cells of the body and play an important role in the defense of the pathogen infection and regulation of immune responses (Venkatesh et al., 2014). LPS, a typical bacterial endotoxin, is the major component of the cell wall of Gram-negative bacteria and is responsible for the activation of the innate immune system caused by bacterial infection (Chaluvadi et al., 2009; Sun et al., 2016). TLR4 usually is low expression in normal state of the body, while in IBD condition, the intestinal flora lose tolerance and specific recognition of LPS, then produce excessive signal transduction, and finally activate TLR4 and induce pro-inflammatory cytokines (Zawawi et al., 2010; Robertsthomson et al., 2011).
As a key transcription factor, NF-κB plays an important role in the activation of genes involved in immune and acute phase responses (Schottelius and Baldwin, 1999) and is an attractive candidate for its roles in IBD pathogenesis (Schottelius and Baldwin, 1999) which can increase the expression of many cytokines, enzymes, and adhesion molecules involved in chronic inflammatory diseases, including IL-1β, TNF-a, IL-2, IL-6, IFN-γ, and IL-12 which possess NF-κB binding sites and are transcriptionally regulated by NF-κB (Barnes and Karin, 1997; Schottelius and Baldwin, 1999). NF-κB in normal intestinal epithelial cells generally shows low expression in a certain range, which can be over activated in the inflammatory environment.
In this study, we demonstrated that TNBS up-regulated the protein expression and mRNA levels of TLR4 and NF-κB. Over expression of these signaling proteins promoted the secretion of cytokines, leading to the proliferation and metastasis of tumor cells (Huang et al., 2014; Wang et al., 2017). Compared with the normal group, mRNA levels of TLR4 and NF-κB in colitis rats were significantly increased, which were markedly down-regulated by different dosages of CP. An attenuated trend of the expression of p65, pp65, and TLR4 in TNBS-induced colitis rats was also observed in the groups treated with CP. Meanwhile, CP could notably down-regulate the levels of pro-inflammatory factors such as TNF-α, IFN-γ, IL-6, and IL-1β. These results suggested that CP exerted its effects on amelioration of TNBS-induced colitis via decreasing the expression of TLR4 and blocking the activation of NF-κB signaling pathways.
Lipopolysaccharide can activate multiple intracellular signal pathways, promote the expression of a large number of cytokines and adhesion molecules, and cause inflammation cascade reaction (Herzum and Renz, 2008; Zhao et al., 2015). IL-6 is a pleiotropic cytokine considered to be a major player in inflammation, regulation of T cell responses, and apoptosis (Kishimoto, 2005). IL-6-mediated signaling pathways are reported to play a critical role in the development of IBD (Atreya and Neurath, 2005). Combines to the receptor, IL-6 increased the phosphorylation of JAK2, an upstream activator of STAT3, and IL-6-induced STAT3 phosphorylation was obtained which regulated the downstream target gene transcription and participated in the regulation of inflammation (Lovato et al., 2003). Studies have shown that activated STAT3 was found in human and animal colitis models, and over expression of STAT3 was always accompanied by more serious of the disease state. IL-6-induced JAK2/STAT3 signaling pathways play an important role in the pathogenesis of IBD. Consistent with previous reports, we observed that TNBS-induced colitis invariably promoted inflammation in the colon with elevated levels of IL-1β, IL-6, TNF-α and an increased expression of JAK2, STAT3, and p-STAT3. In colitis rats, the observed up-regulation of pro-inflammatory cytokines including IL-6 was ameliorated by CP, meanwhile, phosphorylation of Stat3 and JAK2 was blocked. The results provided further evidences of the effects of CP on suppression of colitis and indicated the key role of IL-6/JAK2/STAT3 pathway as relevant targets of CP (Huo et al., 2016).
TLR4/NF-κB and IL-6/JAK2/STAT3 signal pathways were investigated to evaluate the alleviation of CP on the TNBS-induced colitis. It was documented that inhibition of STAT3 activation by a JAK2 inhibitor could reduce the nuclear pool of STAT3 resulting in a suppressed NF-κB activation (Lovato et al., 2012; Seavey et al., 2012). JAK2/STAT3 and NF-κB pathways regulated the expression of a wide spectrum of cytokines and chemokines in the tumor micro-environment, which controlled the activation of immune cells as well as growth and survival of premalignant and malignant epithelial cells, including IL-6, IL-1β, IL-11, IL-23, IFN-γ, TNF-α, and so on (Grivennikov et al., 2006). IL-6 has been shown to play a critical role in the development and progression of colitis and colitis-associated cancer. In our study, its levels in colon tissues were dramatically reduced by CP. Combinatorial effects of STAT3 and NF-κB inactivation could explain such a robust inhibition of IL-6 expression (Bollrath and Greten, 2009).
The results of plasma and urine metabolomics study of colitis rats indicated that endogenous metabolites of glycerophosphocholine and phosphorylcholine levels were decreased, which result in the metabolic disorder of phospholipid and choline metabolism in inflammation. The above two endogenous biomarkers are important metabolites of and the major cellular components of the biofilm, and their deletions lead to abnormalities in cell membrane biosynthesis. It is reported that the reduction of nucleotides and bases, such as nicotinic acid mononucleotides, dihydrouracil, could lead to the death of the colonic epithelial cells (Dong, 2013). The level of cholesterol in the plasma of the model group was increased significantly, which indicated the occurrence of abnormal lipid metabolism associated with colitis. Abnormal dyslipidemia is a typical clinical symptom in patients with the colitis which is closely related to the development of inflammation (Martin et al., 2009). The induction of TNBS/ethanol accelerated the expression of lipoprotein lipase and down-regulated the expression of CYP450 and CoA oxidase. Recent studies indicated that CYP450 enzymes played a significant physiological role in the body. They could control the intracellular cholesterol concentration and affect the transcription of low-density lipoprotein receptors and enzymes involved in cholesterol synthesis (Warren et al., 2001; Karlsson et al., 2008). The increase of cholesterol levels in plasma was accompanied by the TNBS-induced colitis due to the biosynthesis of fatty acids was consistent with our previous studies that the content of acetic acid in colon mucosa of colitis rats was decreased. Because acetic acid is easily converted into acetyl coenzyme A by cell uptake, it is the intermediate product of the synthesis of cholesterol and fatty acids (Yoshimoto et al., 2001). The down-regulation of nicotinamide and urocanic acid in the urine of colitis rats indicated that TNBS-induced ulcerative colitis rats reduced the antioxidant function and increased oxidative stress of the host, which was important reasons for the development and continuation of ulcerative colitis (Cho, 2008). Uric acid is produced by the deamination of L- histidine by histidinelyase. In the liver, the urocanic acid is converted to imidazol-4-oxo-5-propionic acid by the urocanic acid hydratase and eventually to glutamic acid, and subsequently, glutamic acid undergoes the decarboxylation by glutamic acid decarboxylase to form γ-aminobutyric acid, which is an important neurotransmitter and also precursor components of glutathione (Grisham, 1993). Compared with the normal group, the levels of niacinamide in TNBS-induced colitis was decreased. Studies have shown that nicotinamide enhanced the antimicrobial activity of neutrophil, improved tissue damage and reduced the activity of MPO of DSS-induced colitis mice significantly (Disher et al., 2014; Dominik et al., 2014). Consistent with previous studies, the levels of linoleic acid and arachidonic acid were decreased and the leukotriene A4 was increased in colitis rats. Arachidonic acid is an unsaturated fatty acid which is transformed from linoleic acid and linolenic acid, and could be converted into prostaglandins, leukotrienes and other inflammatory substances. IBD is bound to be closely related to the metabolic disorder of bile acids, especially to reduce the level of secondary bile acids, while hydrophilic secondary bile acids have cytoprotective effects. In this study, the down-regulation of taurodeoxycholic acid, chenodeoxycholic acid and bile acid in plasma of colitis rats was verified this point (Blackwood et al., 2011; Disher et al., 2014).
The metabolic pathways were established by importing the potential metabolites into the web-based database MetPA (Figure 7). Furthermore, the relative quantities of eight potential biomarkers in plasma and four in urine were significantly affected by CP. Especially, the middle (MP, 100 mg/kg) and low (LP, 50 mg/kg) dosage were restored to a control-like level. The results indicated that CP could notably alleviate the TNBS-induced colitis.
Conclusion
The present study suggested that CP exerted a distinct inhibition effect on TNBS-induced colitis. CP could significantly reduce the levels of inflammatory cytokines, decrease mRNA levels of TLR4, NF-κB, IL-6, STAT3, and JAK2, and down regulate the expression of pp65, TLR4, p-STAT3, and p-JAK2. The anti-inflammation effect of CP in IBD might be associated with TLR4/NF-κB and IL-6/JAK2/STAT3 signaling pathway. Meanwhile, CP altered the biomarkers and the corresponding metabolic pathways in plasma and urine to normal transformation, which indicated its alleviation on TNBS-induced colitis.
Ethics Statement
The animal experiments were performed according to the Regulations of Experimental Animal Administration (State Committee of Science and Technology of the People’s Republic of China). The guidelines of the Committee on the Care and Use of Laboratory Animals in China and the related ethics regulations of Nanjing University of Chinese Medicine. Laboratory animal license number is SYXK (Su)-2012-0042.
Author Contributions
J-HT and J-AD conceived and designed the study. J-HT and D-DW performed the experiments. J-HT, SJ, and J-MG acquired the data. J-HT, J-AD, and J-MG analyzed the data. J-HT, J-AD, SJ, and WZ drafted and critically revised the article. J-HT, J-AD, WZ, SJ, J-MG, and D-DW approved the final manuscript.
Funding
This work was financially supported by the Nature Science Foundation of China (No. 8160141336) and Jiangsu Collaborative Innovation Center of Chinese Medicinal Resources Industrialization (No. ZDXM-1-10).
Conflict of Interest Statement
The authors declare that the research was conducted in the absence of any commercial or financial relationships that could be construed as a potential conflict of interest.
Supplementary Material
The Supplementary Material for this article can be found online at: https://www.frontiersin.org/articles/10.3389/fphar.2018.00746/full#supplementary-material
FIGURE S1 | Effects of CP on colitis rats. (A) Change in body weight during the disease. (B) DAI based on weight loss, hematochezia, and diarrhea. (C) Statistics of colon length in each group. (D) Histopathological changes in the colons.
FIGURE S2 | Ultra-performance liquid chromatography/quadrupole time of flight mass spectrometry (UPLC-TOF/MS) TIC chromatograms of plasma and urine sample in normal and colitis rats. (A) Plasma; (B) Urine.
TABLE S1 | Primer sequences of the target gene.
Abbreviations
CP, Chrysanthemum polysaccharides; HP, TNBS/ethanol + high dosage CP; IBD, inflammatory bowel disease; JAK2, janus kinase 2; LP, TNBS/ethanol + low dosage CP; LPS, lipopolysaccharide; M, TNBS/ethanol group; MDA, malondialdehyde; MP, TNBS/ethanol + middle dosage CP; MPO, myeloperoxidase; MyD88, myeloid differentiation factor 88; N, Normal group; NF-κB, nuclear factor kappa-B; PCA, principal component analysis; PLS-DA, partial least squares discriminant analysis; PP, TNBS/ethanol + Sulfasalazine; PRRs, pattern recognition receptors; SOD, superoxide dismutase; STAT3, signal transduction and transcriptional activators; TBA, thiobarbituric acid; TLRs, toll-like receptors; TNBS, 2, 4, 6-trinitrobenzene sulfonic acid; UPLC-Q-TOF/MS, ultra-performance liquid chromatography/quadrupole time of flight mass spectrometry; VIP, variable importance.
Footnotes
- ^http://metlin.scripps.edu
- ^http://www.hmdb.ca
- ^http://www.metaboanalyst.ca/
- ^http://www.genome.jp/kegg/
- ^http://www.genome.jp/kegg/
- ^http://www.hmdb.ca
References
Atreya, R., and Neurath, M. F. (2005). Involvement of IL-6 in the pathogenesis of inflammatory bowel disease and colon cancer. Clin. Rev. Allergy Immunol. 28, 96–187. doi: 10.1385/CRIAI:28:3:187
Baldwin, A. S. Jr. (1996). The NF-kappa B and I kappa B proteins: new discoveries and insights. Annu. Rev. Immunol. 14, 649–683. doi: 10.1146/annurev.immunol.14.1.649
Barnes, P. J., and Karin, M. (1997). Nuclear Factor-kappa B: a pivotal transcription factor in chronic inflammatory diseases. N. Engl. J. Med. 336, 1066–1071. doi: 10.1056/NEJM199704103361506
Blackwood, B., Alderdice, F., Burns, K., Cardwell, C., Lavery, G., and O’halloran, P. (2011). Use of weaning protocols for reducing duration of mechanical ventilation in critically ill adult patients: cochrane systematic review and meta-analysis. BMJ 342, 7237–7250. doi: 10.1136/bmj.c7237
Bollrath, J., and Greten, F. R. (2009). IKK/NF-κ B and Stat3 pathways: central signalling hubs in inflammation-mediated tumour promotion and metastasis. EMBO Rep. 10, 1314–1319. doi: 10.1038/embor.2009.243
Cai, H., Su, S., Li, Y., Zeng, H., Zhu, Z., Guo, J., et al. (2018). Salvia miltiorrhiza protects adenine-induced chronic renal failure by regulating the metabolic profiling and modulating the nadph oxidase/ros/erk and tgf-Î2/smad signaling pathways. J. Ethnopharmacol. 212, 153–165. doi: 10.1016/j.jep.2017.09.021
Chaluvadi, M. R., Nyagode, B. A., Kinloch, R. D., and Morgan, E. T. (2009). TLR4-dependent and -independent regulation of hepatic cytochrome P450 in mice with chemically induced inflammatory bowel disease. Biochem. Pharmacol. 77, 464–471. doi: 10.1016/j.bcp.2008.10.029
Chen, P., Zhou, X., Zhang, L., Shan, M., Bao, B., and Cao, Y. (2015). Anti-inflammatory effects of huangqin tang extract in mice on ulcerative colitis. J. Ethnopharmacol. 162, 207–214. doi: 10.1016/j.jep.2014.12.039
Cho, J. H. (2008). The genetics and immunopathogenesis of inflammatory bowel disease. Nat. Rev. Immunol. 8, 66–458. doi: 10.1038/nri2340
Danese, S., and Fiocchi, C. (2006). Etiopathogenesis of inflammatory bowel diseases. World J Gastroenterol. 12, 4807–4812. doi: 10.3748/wjg.v12.i30.4807
Disher, B., Wade, L., Hajdok, G., Gaede, S., Battista, J. J., and Palma, D. (2014). Nicotinamide treatment ameliorates the course of experimental colitis mediated by enhanced neutrophil-specific antibacterial clearance. Mol. Nutr. Food Res. 58, 1474–1490. doi: 10.1002/mnfr.201300818
Dominik, B., Nowacki, T. M., Matthias, R., Pierre, K., Daniela, S., Linda, K., et al. (2014). Nicotinamide treatment ameliorates the course of experimental colitis mediated by enhanced neutrophil-specific antibacterial clearance. Mol. Nutr. Food Res. 58, 1474–1490. doi: 10.1002/mnfr.201300818
Dong, F. C. (2013). NMR-Based Metabonomic Studies on Ulcerative Colitis and Bacteremia. Huairou: University of Chinese Academy of Sciences.
Dou, W., Zhang, J., Ren, G., Ding, L., Sun, A., and Deng, C. (2014). Mangiferin attenuates the symptoms of dextran sulfate sodium-induced colitis in mice via NF-κ B and MAPK signaling inactivation. Int. Immunopharmacol. 23, 170–178. doi: 10.1016/j.intimp.2014.08.025
Fort, M. M., Mozaffarian, A., Stöver, A. G., Correia, J. D. S., Johnson, D. A., and Crane, R. T. (2005). A synthetic TLR4 antagonist has anti-inflammatory effects in two murine models of inflammatory bowel disease. J. Immunol. 174, 6416–6423. doi: 10.4049/jimmunol.174.10.6416
Grisham, M. B. (1993). Role of reactive oxygen metabolites in inflammatory bowel disease. Curr. Opin. Gastroenterol. 9, 971–980. doi: 10.1097/00001574-199309060-00013
Grivennikov, S. I., Kuprash, D. V., Liu, Z., and Nedospasov, S. A. (2006). Intracellular signals and events activated by cytokines of the tumor necrosis factor superfamily: from simple paradigms to complex mechanisms. Int. Rev. Cytol. 252, 129–161. doi: 10.1016/S0074-7696(06)52002-9
Halliwell, B. (1996). Antioxidants in human health and disease. Annu. Rev. Nutr. 16, 33–50. doi: 10.1146/annurev.nu.16.070196.000341
Herzum, I., and Renz, H. (2008). Inflammatory markers in sirs, sepsis and septic shock. Curr. Med. Chem. 15, 581–587. doi: 10.2174/092986708783769704
Holmes, E., Wilson, I. D., and Nicholson, J. K. (2008). Metabolic phenotyping in health and disease. Cell 134, 714–717. doi: 10.1016/j.cell.2008.08.026
Huang, H. Y., Zhang, Z. J., Cao, C. B., Wang, N., Liu, F. F., and Peng, J. Q. (2014). The TLR4/NF-κB signaling pathway mediates the growth of colon cancer. Eur. Rev. Med. Pharmacol. Sci. 18, 3834–3843.
Huang, X., Su, S., Duan, J.-A., Sha, X., Zhu, K. Y., Guo, J., et al. (2016). Effects and mechanisms of Shaofu-Zhuyu decoction and its major bioactive component for Cold – Stagnation and Blood – Stasis primary dysmenorrhea rats. J. Ethnopharmacol. 186, 234–243. doi: 10.1016/j.jep.2016.03.067
Huo, X., Liu, D., Li, G., Li, L., and Li, C. (2016). Flavonoids extracted from licorice prevents colitis-associated carcinogenesis in aom/dss mouse model. Int. J. Mol. Sci. 17, 1343–1354. doi: 10.3390/ijms17091343
Jang, S. E., Hyam, S. R., Jeong, J. J., Han, M. J., and Kim, D. H. (2013). Penta-o-galloyl-β-d-glucose ameliorates inflammation by inhibiting myd88/nf-κb and myd88/mapksignalling pathways. Br. J. Pharmacol. 170, 1078–1091. doi: 10.1111/bph.12333
Jobin, C. (2010). Probiotics and ileitis: could augmentation of TNF/NF-κB activity be the answer? Gut Microbes 1, 196–199. doi: 10.4161/gmic.1.3.12485
Junghee, L., Bomi, L., Hyesung, L., Eunah, B., Hoyong, L., and Youngtae, A. (2009). Lactobacillus suntoryeus inhibits pro-inflammatory cytokine expression and tlr-4-linked NF-κbactivation in experimental colitis. Int. J. Colorectal Dis. 24, 231–237. doi: 10.1007/s00384-008-0618-6
Karlsson, A., Jägervall, A., Pettersson, M., Andersson, A. K., Gillberg, P. G., and Melgar, S. (2008). Dextran sulphate sodium induces acute colitis and alters hepatic function in hamsters. Int. Immunopharmacol. 8, 20–27. doi: 10.1016/j.intimp.2007.10.007
Kim, C., Kim, M. C., Kim, S. M., Nam, D., Choi, S. H., Kim, S. H., et al. (2013). Chrysanthemum indicum l. extract induces apoptosis through suppression of constitutive stat3 activation in human prostate cancer du145 cells. Phytother. Res. 27, 30–38. doi: 10.1002/ptr.4689
Kishimoto, T. (2005). Interleukin-6: from basic science to medicine-40 years in immunology. Annu. Rev. Immunol. 23, 1–21. doi: 10.1146/annurev.immunol.23.021704.115806
Korenaga, D., Takesue, F., Kido, K., Yasuda, M., Inutsuka, S., and Honda, M. (2002). Impaired antioxidant defense system of colonic tissue and cancer development in dextran sulfate sodium-induced colitis in mice. J. Surg. Res. 102, 144–149. doi: 10.1006/jsre.2001.6314
Lau, D., and Baldus, S. (2006). Myeloperoxidase and its contributory role in inflammatory vascular disease. Pharmacol. Ther. 111, 16–26. doi: 10.1016/j.pharmthera.2005.06.023
Lin, H. M., Helsby, N. A., Rowan, D. D., and Ferguson, L. R. (2011). Using metabolomic analysis to understand inflammatory bowel diseases. Inflamm. Bowel Dis. 17, 1021–1029. doi: 10.1002/ibd.21426
Lovato, G., Bezerra, R. A., Rodrigues, J. A. D., Ratusznei, S. M., and Zaiat, M. (2012). Effect of feed strategy on methane production and performance of an ansbbr treating effluent from biodiesel production. Appl. Biochem. Biotechnol. 166, 2007–2029. doi: 10.1007/s12010-012-9627-6
Lovato, P., Brender, C., Agnholt, J., Kelsen, J., Kaltoft, K., and Svejgaard, A. (2003). Constitutive Stat3 activation in intestinal T cells from patients with Crohn’s disease. J. Biol. Chem. 278, 16777–16781. doi: 10.1074/jbc.M207999200
Luyen, B. T., Tai, B. H., Thao, N. P., Cha, J. Y., Lee, H. Y., and Lee, Y. M. (2015). Anti-inflammatory components of chrysanthemum indicum flowers. Bioorg. Med. Chem. Lett. 25, 266–269. doi: 10.1016/j.bmcl.2014.11.054
Mar, J. S., Nagalingam, N. A., Song, Y., Onizawa, M., Lee, J. W., and Lynch, S. V. (2014). Amelioration of Dss-induced Murine Colitis By Vsl#3 supplementation is primarily associated with changes in ileal microbiota composition. Gut Microbes 5, 494–503. doi: 10.4161/gmic.32147
Martin, F., Rezzi, S., Montoliu, I., Philippe, D., Tornier, L., and Messlik, A. (2009). Metabolic assessment of gradual development of moderate experimental colitis in Il-10 deficient mice. J. Proteome Res. 8, 2376–2387. doi: 10.1021/pr801006e
Miao, X. P., Sun, X. N., Cui, L. J., Cao, Q. F., Zhuang, G. F., and Deng, T. Z. (2015). Suppressive effect of pectic polysaccharides extracted from Rauwolfia verticillata (lour.) Baill.var.hainanensis Tsiang on inflammation By regulation of Nf– κ B pathway and interleukin–17 in mice with dextran sulphatesodium–induced ulcerative coli. Asian Pac. J. Trop. Med. 8, 147–152. doi: 10.1016/S1995-7645(14)60306-0
Nicholson, J. K., Lindon, J. C., and Holmes, E. (2008). ’Metabonomics’: understanding the metabolic responses of living systems to pathophysiological stimuli via multivariate statistical analysis of biological NMR spectroscopic data. Xenobiotica 29, 1181–1189. doi: 10.1080/004982599238047
Okafor, P. N., Nunes, D. P., and Farraye, F. A. (2013). Pneumocystis jiroveci pneumonia in inflammatory bowel disease: when should prophylaxis be considered? Inflamm. Bowel Dis. 19, 1764–1771. doi: 10.1097/MIB.0b013e318281f562
Packey, C. D., and Sartor, R. B. (2008). Interplay of commensal and pathogenic bacteria, genetic mutations, and immunoregulatory defects in the pathogenesis of inflammatory bowel diseases. J. Intern. Med. 263, 597–606. doi: 10.1111/j.1365-2796.2008.01962.x
Pan, J., Xiang, Q., and Ball, S. (2000). Use of a novel real-time quantitative reverse transcription-polymerase chain reaction method to study the effects of cytokines on cytochrome p450 mrna expression in mouse liver. Drug Metab. Dispos. 28, 709–713.
Pattison, D. I., and Davies, M. J. (2006). Reactions of myeloperoxidase-derived oxidants with biological substrates: gaining chemical insight into human inflammatory diseases. Curr. Med. Chem. 13, 3271–3290. doi: 10.2174/092986706778773095
Phua, L. C., Koh, P. K., Cheah, P. Y., Ho, H. K., and Chan, E. C. (2013). Global Gas chromatography/time-of-flight mass spectrometry (GC-TOF/MS)-based metabonomic profiling of lyophilized human feces. J. Chromatogr. B 937, 13–103. doi: 10.1016/j.jchromb.2013.08.025
Pizzi, L. T., Weston, C. M., Goldfarb, N. I., Moretti, D., Cobb, N., and Howell, J. B. (2006). Impact of chronic conditions on quality of life in patients with inflammatory bowel disease. Inflamm. Bowel Dis. 12, 47–52. doi: 10.1097/01.MIB.0000191670.04605.e7
Recio, M. C., Andujar, I., and Rios, J. L. (2012). Anti-inflammatory agents from plants: progress and potential. Curr. Med. Chem. 19, 2088–2103. doi: 10.2174/092986712800229069
Robertsthomson, I. C., Fon, J., Uylaki, W., Cummins, A. G., and Barry, S. (2011). Cells, cytokines and inflammatory bowel disease: a clinical perspective. Expert Rev. Gastroenterol. Hepatol. 5, 7034807–4812. doi: 10.1586/egh.11.74
Salliot, C., and Van, D. H. D. (2009). Long-term safety of methotrexate monotherapy in patients with rheumatoid arthritis: a systematic literature research. Ann. Rheum. Dis. 68, 1100–1104. doi: 10.1136/ard.2008.093690
Scheiffele, F., and Fuss, I. J. (2002). Induction of tnbs colitis in mice. Curr. Protoc. Immunol. 15:Unit15.19. doi: 10.1002/0471142735.im1519s49
Schicho, R., Nazyrova, A., Shaykhutdinov, R., Duggan, G., Vogel, H. J., and Storr, M. (2010). Quantitative metabolomic profiling of serum and urine in dss-induced ulcerative colitis of mice by (1)HNMR spectroscopy. J. Proteome Res. 9, 6265–6273. doi: 10.1021/pr100547y
Schottelius, A. J. G., and Baldwin, A. S. (1999). A role for transcription factor NF-kB in intestinal inflammation. Int. J. Colorectal Dis. 14, 18–28. doi: 10.1007/s003840050178
Seavey, M. M., Lu, L. D., Stump, K. L., Wallace, N. H., Hockeimer, W., and O’kane, T. M. (2012). Therapeutic efficacy of Cep-33779, a novel selective Jak2 inhibitor, in a mouse model of colitis-induced colorectal cancer. Mol. Cancer Ther. 11, 984–993. doi: 10.1158/1535-7163.MCT-11-0951
Siddique, I., and Khan, I. (2011). Mechanism of regulation of na-hexchanger in inflammatory bowel disease: role of TLR-4 signaling mechanism. Dig. Dis. Sci. 56, 62–1656. doi: 10.1007/s10620-010-1524-7
Su, S., Duan, J., Chen, T., Huang, X., Shang, E., and Li, Y. (2015). Corrigendum: frankincense and myrrh suppress inflammation via regulation of the metabolic profiling and the MAPK signaling pathway. Sci. Rep. 5:15597. doi: 10.1038/srep13668
Su, S., Duan, J., Wang, P., Liu, P., Guo, J., and Shang, E. (2013). Metabolomic study of biochemical changes in the plasma and urine of primary dysmenorrhea patients using uplc-ms coupled with a pattern recognition approach. J. Proteome Res. 12, 852–865. doi: 10.1021/pr300935x
Sun, A., Ren, G., Deng, C., Zhang, J., Luo, X., and Wu, X. (2016). ). C-glycosyl flavonoid orientin improves chemically induced inflammatory bowel disease in mice. J. Funct. Foods 21, 418–430. doi: 10.1016/j.jff.2015.12.029
Tao, J. H., Duan, J. A., Jiang, S., Feng, N. N., Qiu, W. Q., and Ling, Y. (2017). Polysaccharides from chrysanthemum morifolium ramat ameliorate colitis rats by modulating the intestinal microbiota community. Oncotarget 8, 80790–80803. doi: 10.18632/oncotarget.20477
Tao, J. H., Duan, J. A., Shu, J., Guo, J. M., Qian, Y. Y., and Qian, D. W. (2016). Simultaneous determination of six short-chain fatty acids in colonic contents of colitis mice after oral administration of polysaccharides from chrysanthemum morifolium ramat by gas chromatography with flame ionization detector. J. Chromatogr. B 102, 88–94. doi: 10.1016/j.jchromb.2016.07.002
Tsang, S. W., Ip, S. P., Wu, J. C., Ng, S. C., Yung, K. K., and Bian, Z. X. (2015). A Chinese medicinal formulation ameliorates dextran sulfate sodium-induced experimental colitis by suppressing the activity of nuclear factor-kappa B signaling. J. Ethnopharmacol. 162, 20–30. doi: 10.1016/j.jep.2014.12.035
Venkatesh, M., Mukherjee, S., Wang, H., Li, H., Sun, K., and Benechet, A. P. (2014). Symbiotic bacterial metabolites regulate gastrointestinal barrier function via the xenobiotic sensor PXR and toll-like receptor 4. Immunity 41, 296–310. doi: 10.1016/j.immuni.2014.06.014
Waidmann, M., Bechtold, O., Frick, J. S., Lehr, H. A., Schubert, S., and Dobrindt, U. (2003). Bacteroides vulgatus protects against Escherichia coli-induced colitis in gnotobiotic interleukin-2-deficient mice. Gastroenterology 125, 162–177. doi: 10.1016/S0016-5085(03)00672-3
Walker, A. W., Sanderson, J. D., Churcher, C., Parkes, G. C., Hudspith, B. N., and RaymentNetal. (2011). High-throughput clone library analysis of the mucosa-associated microbiota reveals dysbiosis and differences between inflamed and non-inflamed regions of the intestine in inflammatory bowel disease. BMC Microbiol. 11:7. doi: 10.1186/1471-2180-11-7
Wang, H., Gu, J., Hou, X., Chen, J., Yang, N., and Liu, Y. (2017). Anti-inflammatory effect of miltirone on inflammatory bowel disease via TLR4/NF-κB/IQGAP2 signaling pathway. Biomed. Pharmacother. 85, 531–540. doi: 10.1016/j.biopha.2016.11.061
Warren, G. W., Van Ess, P. J., Watson, A. M., Mattson, M. P., and Blouin, R. A. (2001). Cytochrome P450 and antioxidant activity in interleukin-6 knockout mice after induction of the acute-phase response. J. Interferon Cytokine Res. 21, 821–826. doi: 10.1089/107999001753238060
Wu, M., Wu, Y., Deng, B., Li, J., Cao, H., and Yan, Q. (2016). Isoliquiritigenin decreases the incidence of colitis-associated colorectal cancer by modulating the intestinal microbiota. Oncotarget 7, 85318–85331. doi: 10.18632/oncotarget.13347
Xing, J. F., Sun, J. N., Sun, J. Y., You, C. Y., Dong, K., and Lv, J. (2012). Protective effects of 3, 4-oxo-isopropylidene-shikimic acid on experimental colitis induced by trinitrobenzenesulfonic acid in rats. Dig. Dis. Sci. 57, 2045–2054. doi: 10.1007/s10620-012-2155-y
Yoshimoto, M., Waki, A., Yonekura, Y., Sadato, N., Murata, T., and Omata, N. (2001). Characterization of acetate metabolism in tumor cells in relation to cell proliferation: acetate metabolism in tumor cells. Nucl. Med. Biol. 28, 117–122. doi: 10.1016/S0969-8051(00)00195-5
Zawawi, K. H., Kantarci, A., Schulze-späte, U., Fujita, T., Batista, E. L. Jr., and Amar, S. (2010). Moesin-induced signaling in response to lipopolysaccharide in macrophages. J. Periodontal Res. 45, 589–601. doi: 10.1111/j.1600-0765.2010.01271.x
Zhao, Q. C., Shen, H., and Cheng, H. B. (2015). Effect of qingchanghuashi recipe on IL-6/JAK2/STAT3 pathway in HT-29 cells. Chin. Pharmacol. Bull. 6, 883–885. doi: 10.3969/j.issn.1001-1978.2015.06.029
Zhao, Y. Y., Shen, X. F., Cheng, X. L., Wei, F., Bai, X., and Lin, R. C. (2012). Urinary metabonomics study on the protective effects of ergosta-4,6,8(14),22-tetraen-3-one on chronic renal failure in rats using UPLC Q-TOF/MS and a novel MSE data collection technique. Process. Biochem. 47, 1980–1987. doi: 10.1016/j.procbio.2012.07.008
Keywords: Chrysanthemum polysaccharides, colitis, metabonomics, signaling pathway, IBD
Citation: Tao J-H, Duan J-A, Zhang W, Jiang S, Guo J-M and Wei D-D (2018) Polysaccharides From Chrysanthemum morifolium Ramat Ameliorate Colitis Rats via Regulation of the Metabolic Profiling and NF-κ B/TLR4 and IL-6/JAK2/STAT3 Signaling Pathways. Front. Pharmacol. 9:746. doi: 10.3389/fphar.2018.00746
Received: 24 March 2018; Accepted: 19 June 2018;
Published: 10 July 2018.
Edited by:
Lie-Fen Shyur, Agricultural Biotechnology Research Center, Academia Sinica, TaiwanReviewed by:
Wentzel Christoffel Gelderblom, Cape Peninsula University of Technology, South AfricaGeng Wenye, Fudan University, China
Copyright © 2018 Tao, Duan, Zhang, Jiang, Guo and Wei. This is an open-access article distributed under the terms of the Creative Commons Attribution License (CC BY). The use, distribution or reproduction in other forums is permitted, provided the original author(s) and the copyright owner(s) are credited and that the original publication in this journal is cited, in accordance with accepted academic practice. No use, distribution or reproduction is permitted which does not comply with these terms.
*Correspondence: Jin-Hua Tao, dGFvamluaHVhMjAwMEAxNjMuY29t Jin-Ao Duan, ZGphMTcwNjI2QDE2My5jb20=