- 1Longhua Hospital, Shanghai University of Traditional Chinese Medicine, Shanghai, China
- 2State Key Laboratory of Quality Research in Chinese Medicine, Institute of Chinese Medical Sciences, University of Macau, Macau, China
- 3Shuguang Hospital, Shanghai University of Traditional Chinese Medicine, Shanghai, China
- 4Faculty of Health Sciences, University of Macau, Macau, China
- 5Cardiac Rehabilitation Center of Longhua Hospital, Shanghai University of Traditional Chinese Medicine, Shanghai, China
Tetrahydropalmatine (THP) is an active natural alkaloid isolated from Corydalis yanhusuo W.T. Wang which has been widely used for treating pain and cardiovascular disease in traditional Chinese medicine. Previous studies suggested THP have various pharmacological effects in neural and cardio tissue while the vascular reactivity of THP was not fully established. The present study found that THP relaxed rat aorta which contracted by phenylephrine (Phe), KCl, and U46619. The vascular relaxation effect of THP was partially attenuated by PI3K inhibitor wortmannin, Akt inhibitor IV, endothelial nitric oxide synthetase (eNOS) inhibitor L-NAME, guanylate cyclase inhibitors and the mechanical removal of endothelium. Also, the eNOS substrate L-arginine reversed the inhibition effect of L-NAME on THP-induced vascular relaxation. THP also induced intracellular NO production in human umbilical vein endothelial cells. However, Pre-incubation with β-adrenergic receptor blocker propranolol, angiotensin II receptor 1 (AT1) inhibitor losartan, angiotensin II receptor 2 (AT1) inhibitor PD123319 or angiotensin converting enzyme inhibitor enalapril enhanced the vascular relaxation effect of THP. THP did not affect the angiotensin II induced vascular contraction. Cyclooxygenase-2 (COX2) inhibitor indomethacin did not affect the vascular relaxation effect of THP. Furthermore, pre-treatment THP attenuated KCl and Phe induced rat aorta contraction in standard Krebs solution. In Ca2+ free Krebs solution, THP inhibited the Ca2+ induced vascular contraction under KCl or Phe stress and reduced KCl stressed Ca2+ influx in rat vascular smooth muscle cells. THP also inhibited intracellular Ca2+ release induced vascular contraction by blocking Ryr or IP3 receptors. In addition, the voltage-dependent K+ channel (Kv) blocker 4-aminopyridine, ATP-sensitive K+ channel (KATP) blocker glibenclamide and inward rectifying K+ channel blocker BaCl2 attenuated THP induced vascular relaxation regardless of the Ca2+-activated K+ channel (KCa) blocker tetraethylammonium. Thus, we could conclude that THP relaxed rat aorta in an endothelium-dependent and independent manner. The underlying mechanism of THP relaxing rat aorta involved PI3K/Akt/eNOS/NO/cGMP signaling path-way, Ca2+ channels and K+ channels rather than COX2, β-adrenergic receptor and renin-angiotensin system (RAS). These findings indicated that THP might be a potent treatment of diseases with vascular dysfunction like hypertension.
Introduction
Hypertension is a worldwide disease, while the prevalence of hypertension arising and the control rate is less than 20% in China (Armario et al., 2017; Lu et al., 2017). Vascular function plays a vital role in the maintenance of normal blood pressure. Endothelial function, intracellular and extracellular ions in particular balance intracellular Ca2+ concentration and calcium sensitization primary control vascular function (Wang et al., 2015; Garcia et al., 2017; Zhou et al., 2017). Previous studies have suggested that Chinese medicine ameliorated endothelial dysfunction, vascular contractility, and vasodilation, which were important in hypertension therapy. Blood-Letting therapy, Qigong Ba Duan Jin and Tai Chi excises provided an alternative approach for hypertension therapy (Sun and Buys, 2015; Xiao et al., 2016; Ma et al., 2018; Xiong et al., 2019). Chinese herb medicine, such as Ilex hainanensis Merr., Longdanxiegan Decoction, also enhanced the effectiveness of anti-hypertension drug (Xiong et al., 2018; Yang et al., 2018). Thus, traditional Chinese medicine plays a vital role in the prevention and treatment of hypertension, especially in China.
Tetrahydropalmatine (THP, Figure 1A) is a natural alkaloid isolated from Corydalis yanhusuo W.T. Wang which has been widely used in traditional Chinese medicine for treating various pains and cardiovascular disease (Han et al., 2012; Kang et al., 2016). Previous studies have indicated that THP presented multiple pharmacological effects on cardio and neural tissues, such as cardioprotection, neuroprotection, anti-oxidant, anti-apoptosis, and anti-inflammation (Wu et al., 2010; Zhang et al., 2015, 2018). THP improved memory impairment, protected the blood brain barrier and cerebral ischemia-reperfusion injuries in experimental mice models (Mao et al., 2015; Cao et al., 2018; Sun et al., 2018). Chueh et al. found THP could induce hypotension and bradycardia though inhibition of the 5-HT2 and/or D2-receptor in the hypothalamus in rats (Chueh et al., 1995; Fu et al., 1995). THP presented numerous benefits in blood vessels as well. THP reduced the inflammation process of monocyte binding to endothelium by down regulation of ICAM-1 and VCAM-1 in endothelial cells (Yang et al., 2015). THP also inhibited the progression of aortic aneurysms (AAs) though the suppression of matrix metalloproteinase and monocyte chemotactic protein-1 in rat (Wang et al., 2018). Moreover, THP relaxed rabbit atrial strips in a calcium channel blocker like manner (Sun and Li, 1989) and inhibited the kinetic activity of Kv1.5 channels expressed in HEK293 cells (Li et al., 2017). These results suggested that THP presented various pharmacological effects in the vascular system and is potent for anti-hypertension therapy. However, the vascular reactivity of THP and the action mechanism has not yet been fully understood.
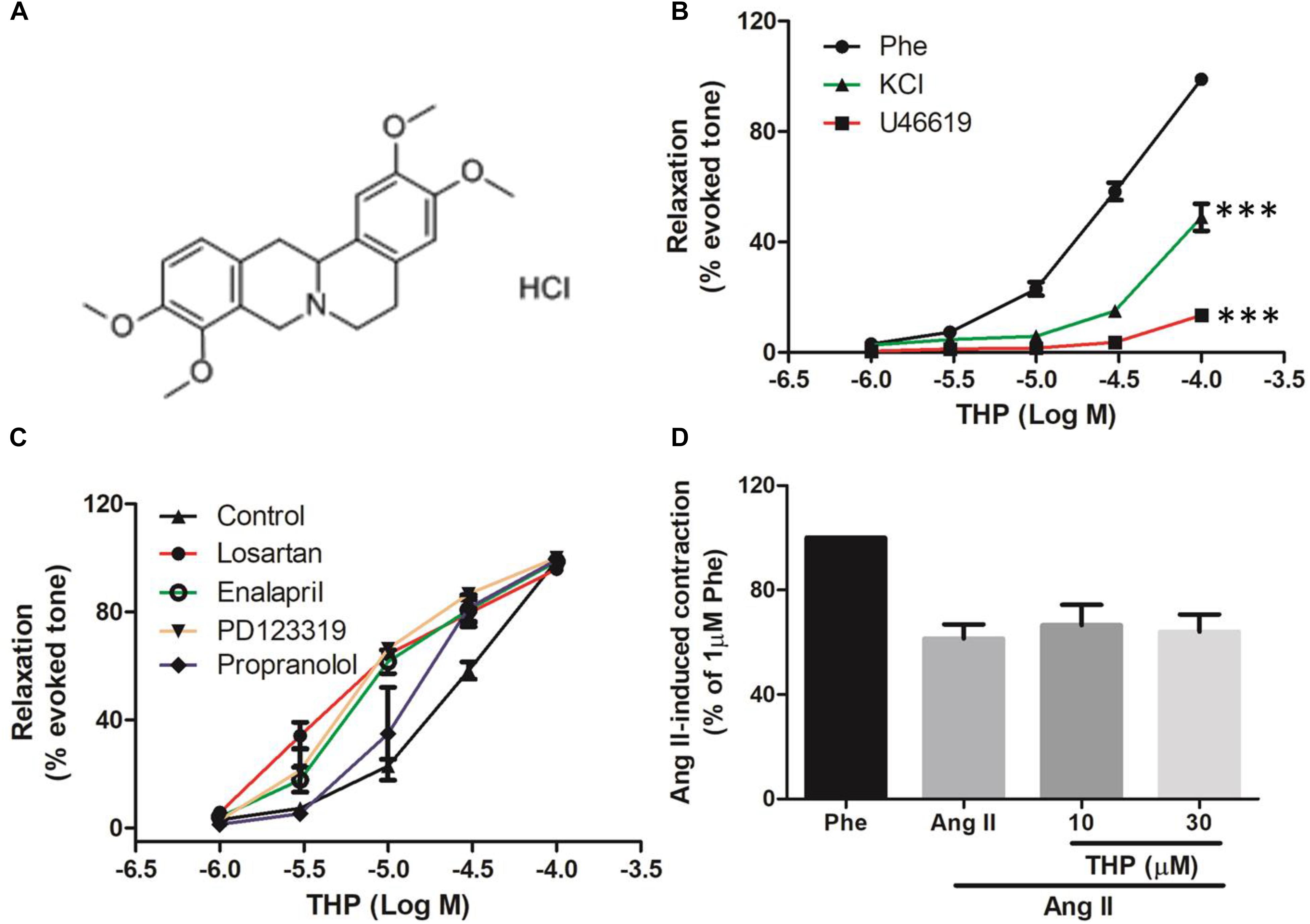
Figure 1. Tetrahydropalmatine (THP) induced vascular relaxation in rat aorta. (A) The chemical structure of Tetrahydropalmatine Hydrochloride (THP, PubChem CID: 6602555). (B) Rat aortic rings were pre-contracted by phenylephrine (Phe, 1 μM), U46619 (30 nM), or KCl (60 mM) and various concentration of THP (1, 3, 10, 30, and 100 μM) were cumulative applied to induce vascular relaxation. (C) Aortic rings were pre-incubated propranolol (1 μM), Losartan (1 μM), Enalapril (1 μM), or PD123319 (1 μM) for 30 min followed by the addition of Phe (1 μM) to induce contraction and relaxed by cumulative addition of THP (1, 3, 10, 30, and 100 μM). (D) Aorta rings were pre-incubated THP (10 and 30 μM) for 30 min then followed by the addition of Ang II (3 μM) to induce vascular contraction. Vascular relaxations or contractions were presented as percentage of the evoked tone. Results were means ± SEM. ∗∗∗P < 0.001 versus control group.
In present study, we examined the vascular relaxation effects of THP under different contractors in rat aorta. The roles of endothelium, vascular smooth muscle cell and related signaling pathway and ion channels in the vascular relaxation effect of THP were also evaluated.
Materials and Methods
Chemicals
Acetylcholine (Ach), phenylephrine (Phe), Indomethacin (Indo), and angiotensin II (Ang II) were bought from Sigma-Aldrich (St. Louis, MO, United States). 9,11-dideoxy-9α,11α-methanoepoxy Prosta-glandin F2α (U46619), L-NAME, nifedipine, 1H-[1,2,4]-oxadiazolo-[4,3-alpha]-quinoxalin-1-one (ODQ) and Losartan, Enalapril, PD123319 were from Cayman Chemical (MI, United States). Fura-2 was supplied by Beyotime Biotechnology (Shanghai, China). THP hydrochloride (purity by HPLC ≥98.0%) was bought from Chengdu Must Bio-Technology Co., Ltd. (Chengdu, China). THP was dissolved in dimethyl sulfoxide (DMSO) while other drugs were prepared in distilled water.
Animals
Male Wistar rats weighting 250 ± 20 g were supplied by the Laboratory Animal Service Center, Shanghai University of Traditional Chinese Medicine. All experiments described below were in accordance with the Animal Experimentation Ethics Committee, Shanghai University of Traditional Chinese Medicine.
Artery Preparation
Rats were killed by carbon dioxide suffocation. After scarification, aortas were quickly isolated and immersed in oxygenated (95% O2/5% CO2) chilled Krebs solution with the following composition (mM):119 NaCl, 4.7 KCl, 2.5 CaCl2, 1 MgCl2, 25 NaHCO3, 1.2 KH2PO2, and 11 D-glucose. Fat and connective tissues were removed carefully. Then aortas were cut into ring segments with a length of 3–4 mm. The endothelium was mechanically removed by gently rubbing the internal surface of the ring using stainless steel wire.
Measurement of Isometric Vascular Tone
Isometric tension of aortic rings was recorded in 15-ml organ bath (Techman Software Co., Ltd., Chengdu, China). The organ chambers were filled with Krebs solution bubbled with 95% O2 and 5% CO2 at 37°C. Each ring was stretched to 1.5 g resting tension. Before each experiment, the rings were equilibrated for 60–90 min and stimulated with 60 mM KCl at least three times to obtain a reproducible maximal contractile response. The integrity of endothelium was assessed by the ability of Ach (10 μM) to induce more than 80% relaxation of rings pre-contracted with Phe (1 μM). In endothelium-denuded rings, the relaxation to Ach was less than 10%. Ca2+-free Krebs solution was prepared by the omission of CaCl2 and the addition of 0.5 mM EGTA. In order to ensure the repeatability of the study, 11–13 g was selected as the inclusion criterion of pre-contractile force.
Experimental Procedures
Effect of THP on Vascular Contraction Induced by Phe, KCl, and U46619
Phe (1 μM), KCl (60 mM), or U46619 (30 nM) was applied to contract rat aorta and the cumulative concentration response of THP (1, 3, 10, and 30, 100 μM) was examined. The experiments were repeated with the addition of the solvent DMSO at 1:1000 v/v (volume of DMSO per volume of final solution volume) to the contracted arteries, though this did not result in any relaxation (data not shown), thus verifying that the relaxations observed are most likely due to the action of THP. Relaxations were expressed as the percentage of the plateau contraction.
Role of β-Adrenergic Receptor and Renin-Angiotensin System (RAS) in THP-Induced Vascular Relaxation
Aortic rings were pre-incubated with β-adrenergic receptor blocker propranolol (1 μM), angiotensin II receptor 1 (AT1) inhibitor losartan (1 μM), angiotensin II receptor 2 (AT2) inhibitor PD123319 (1 μM), or angiotensin converting enzyme inhibitor enalapril (1 μM) for 30 min, then the concentration-response to accumulative addition of THP (1–100 μM) was studied in aortic rings pre-contracted by Phe (1 μM). Aortic rings were pre-incubated with THP (10 and 30 μM) for 30 min which was followed by the addition of Ang II (3 μM) to induce vascular contraction. Relaxations or contractions were expressed as the percentage of the Phe-induced contraction.
Role of Endothelium, PI3K/Akt/eNOS/NO/cGMP, and COX2/PGI2 Signaling Pathway in THP-Induced Vascular Relaxation
Aortic rings were incubated with guanylate cyclase inhibitor Methylene blue (MB, 5 μM), nitric oxide synthase (NOS) inhibitor L-NAME (100 μM), PI3K inhibitor Wortmannin (Wort, 0.5 μM), Akt inhibitor IV (0.5 μM), cyclooxygenase-2 (COX2) inhibitor Indomethacin (Indo, 1 μM), or NO-sensitive guanylyl cyclase inhibitor ODQ (3 μM) for 30 min. Alternatively, we performed mechanical removal of endothelium (-endo), and then the concentration-response to accumulative addition of THP (1–100 μM) was studied in aortic rings pre-contracted by Phe (1 μM). L-arginine (L-Arg, 100 μM) was used to argue the effect of L-NAME on THP induced aorta relaxation. Relaxations were expressed as the percentage of the plateau contraction.
Effect of THP on High K+ or Phe-Induced Vascular Contraction
Aortic rings were pre-treated with THP (10, 30, and 100 μM) or DMSO (1:1000 v/v) which served as solvent control for 30 min, then followed by KCl (60 mM) or Phe (1 μM)-induced vascular contraction. The plateaued contraction force values were recorded. The L-type Ca2+ channel blocker nifedipine (100 nM) was used as positive control. Vascular tensions were expressed as the percentage of the plateau contraction induced by KCl (60 mM) or Phe (1 μM) in the control group.
Effect of THP on Extracellular Ca2+-Induced Vascular Contraction
Aortic rings were challenged with high K+ (60 mM KCl) or Phe (1 μM) containing Ca2+-free Krebs solution. The cumulative concentration-response curves of CaCl2 (0.1–3 mM) were obtained after incubation with THP (10 and 100 μM), nifedipine (100 nM) or DMSO (1:1000 v/v) for 30 min. The contractile responses to CaCl2 were expressed as the percentage of the plateau contraction induced by KCl (60 mM) or Phe (1 μM) in standard Krebs solution.
Roles of K+ Channels in THP-Induced Vascular Relaxation
To elucidate the roles of K+ channels in THP-mediated relaxation, intact or endothelium denuded aortic rings were pre-incubated with the voltage-dependent K+ channel (Kv) blocker 4-aminopyridine (4-AP, 100 μM) and ATP-sensitive K+ channel (KATP) blocker glibenclamide (Gly, 1 μM), Ca2+-activated K+ channel (KCa) blocker tetraethylammonium (TEA, 1 mM), or inward rectifying K+ channel blocker (BaCl2, 100 μM) for 30 min, then followed by Phe (1 μM) to reach a plateaued contraction and cumulative concentration-response to THP (1–100 μM) was measured. Relaxations were expressed as the percentage of the plateau contraction.
Roles of Intracellular Ca2+ Storage in THP-Induced Vascular Relaxation
The rat aortas were immersed in Ca2+ free Krebs solution and pre-incubated with THP (10, 30, and 100 μM) for 30 min, then vascular contracted by KCl (60 mM) or Phe (1 μM). The Ca2+ was released from the intracellular Ca2+ store though Ryr and IP3 receptors in the endoplasmic reticulum. Thus, the endothelium denuded rat aortas were pre-treated with Ryr inhibitor ruthenium red (RR, 10 μM) or IP3 receptor heparin (5 mg/ml) for 30 min, then vascular contracted by Phe (1 μM) and relaxed by the accumulative addition of THP (1–100 μM). Relaxations were expressed as the percentage of the plateau contraction.
Human Umbilical Vein Endothelial Cell (HUVEC) Culture and Detection of Intracellular Nitric Oxide (NO)
The HUVEC cell line was purchased from the American type culture collection (ATCC) and cultured in cultured in F-12K medium supplemented with 0.1 mg/ml heparin, 0.05 mg/ml endothelial cell growth supplement (ECGS), 10% (v/v) FBS and 1% penicillin/streptomycin at 37°C in a humidified atmosphere of 5% CO2 in air. HUVECs were stained with the NO probe DAF-FM DA (5 μM) for 30 min followed by NO imaging every 10 s with a real-time cell imaging system. After 5 min baseline intracellular NO recorded, HUVECs were treated with THP (25 and 100 μM) or 0.01% DMSO and continually record to 1 h. The data calculated as the ratio of fluorescent signal value at indicated time (F1) and the fluorescent signal value at the 0 time point (F0).
Rat Vascular Smooth Muscle Cell A7r5 Culture and Detection of Intracellular Calcium
The A7r5 cell line was purchased from the ATCC and cultured in Dulbecco’s modified Eagle’s medium (DMEM, Gibco) supplemented with 10% FBS (Gibco). A7r5 cells were incubated at 37°C in a humidified atmosphere of 5% CO2 in air. A7r5 cells were stained by the calcium indicator Fura-2 (1 μM) for 15 min in Krebs solution and followed by the incubation of THP (100 μM) or nifedipine (100 nM) for 30 min in Ca2+ free Krebs solution containing KCl (60 mM). Then, the CaCl2 (2.5 mM) was added to trigger the Ca2+ influx. Nifedipine served as a positive control. The values of 550 nm emission were recorded at the 340 nm excitation and 380 nm excitation in the same time by calcium imaging system (Nikon Ti-E, Japan). The intracellular calcium amount was measured as ratio 340 nm/380 nm (R). The calcium imaging system recorded 60 s to obtain the stable baseline. The change of ratio 340 nm/380 nm value (ΔR) was calculated as ratio 340 nm/380 nm value after the addition of CaCl2 for 2 min (R1) minus ratio 340 nm/380 nm value before the addition of CaCl2 (R0).
Data Analysis
Data was mean ± SEM from at least three vascular rings from different rats. The vascular relaxation degree was presented as a percentage of the evoked contraction. Data was analyzed using GraphPad Prism software (version 5.0). The half-maximum effective concentration (EC50) means the concentration of THP that induced 50% of maximal relaxation (Emax). The negative logarithm of the EC50 (pD2) was calculated from the concentration-response curves by non-linear regression (curve fit). The Student’s unpaired t-test or analysis of variance (ANOVA) was used for statistical evaluation of difference between two groups. P < 0.05 indicated a significant difference.
Results
Tetrahydropalmatine (THP) Concentration Dependently Relaxed Rat Aorta Pre-contacted by Phenylephrine (Phe), KCl, or U46619
In order to examine the vascular relaxation effect of THP (Figure 1A) caused by different contractors, rat aorta was pre-contracted by KCl, Phe, or U46619 then relaxed by cumulative addition of THP. As the results shown in Figure 1B and Table 1, THP relaxed rat aortas in a concentration-dependent manner while the relaxation response cures were not consistent between vascular challenged by different contractors. The vascular relaxation effect of THP was most effective in the aorta rings pre-contracted by Phe and the corresponding maximal relaxation values (Emax) and negative logarithm of the half-maximum effective concentration (pD2) were 98.97 ± 1.31 and 4.48 ± 0.07%, respectively (Table 1). In addition, pre-incubation with β-adrenergic receptor blocker propranolol, angiotensin II receptor 1 (AT1) inhibitor losartan, angiotensin II receptor 2 (AT2) inhibitor PD123319 or angiotensin converting enzyme inhibitor enalapril enhanced the vascular relaxation effect of THP in Phe-induced vascular contraction (Figure 1C and Table 1). Pre-treatment THP not affected the vascular contraction induced by angiotensin II (Figure 1D). So, the vascular relaxation effect of THP was not like relating to β-adrenergic receptor and renin-angiotensin system (RAS).
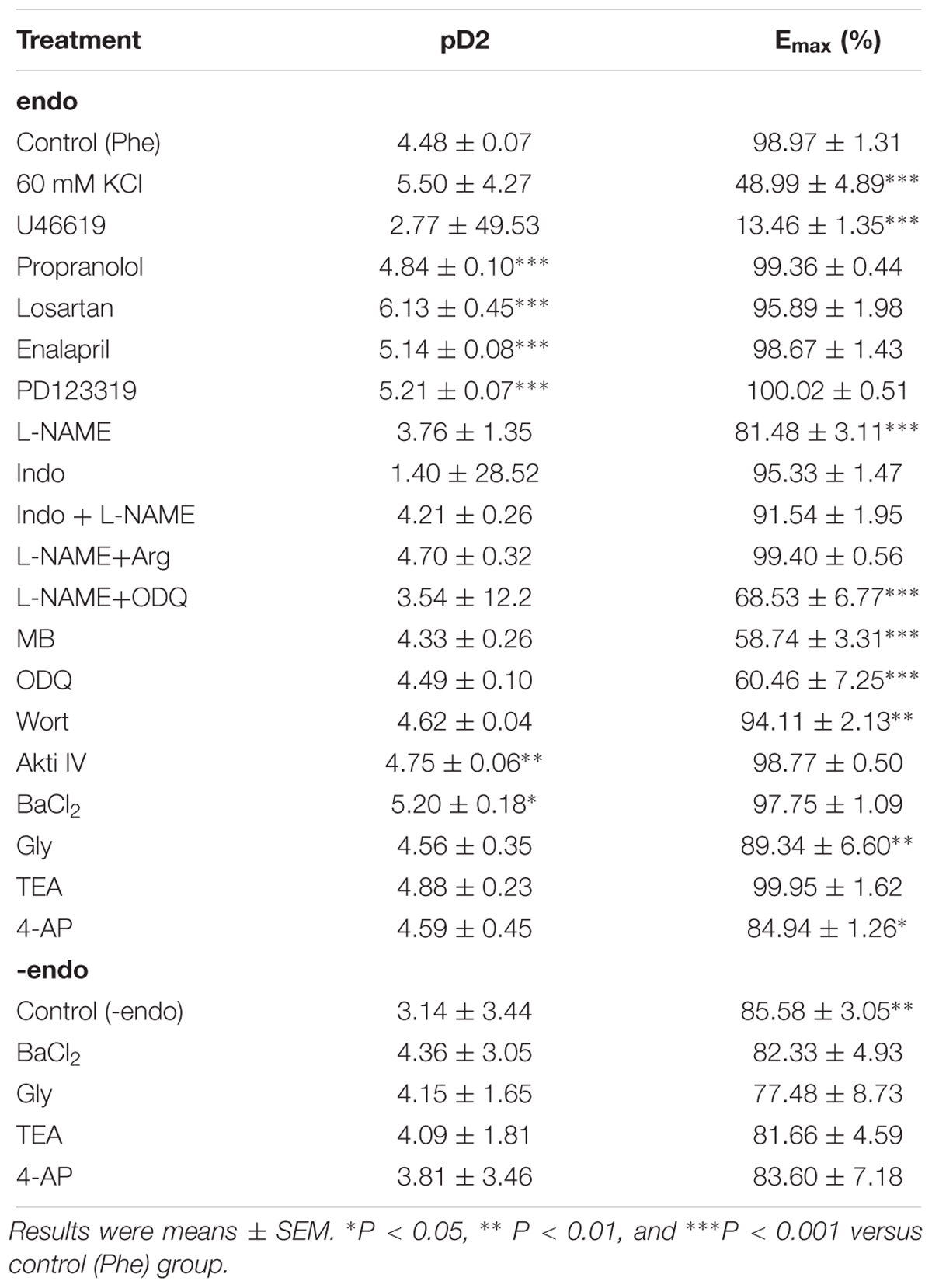
Table 1. Characteristics of THP (1–100 μM)-induced vascular relaxation under different conditions in intact (endo) and endothelium denudated (-endo) rat aorta.
THP-Induced Vascular Relaxation Was Dependent on Endothelium and Related to PI3K/Akt/eNOS/NO/cGMP Signaling Pathway, but Not Involved in COX2/PGI2
Nitric oxide (NO) and Prostacyclin (PGI2) were main endothelium dependent relaxing factors (EDRF). In present study, we found pre-incubation with endothelial nitric oxide synthase (eNOS) inhibitor L-NAME, soluble guanylyl cyclase inhibitor ODQ, guanylyl cyclase inhibitor methylene blue (MB), and mechanical removal of endothelium (-endo) suppressed the vascular relaxation effect of THP (Figures 2A,B). The addition of L-arginine (L-Arg) reversed the inhibition effect of L-NAME in THP induced vasodilation. Also, the combination of L-NAME and ODQ significantly inhibited THP-induced vascular relaxation (Figure 2C). Regarding the Emax, there was no significant difference between the L-NAME-pre-treated group and the mechanical removal of endothelium (-endo) group (Table 1). Moreover, the PI3K inhibitor wortmannin (Wort) and Akt inhibitor IV (Akti IV) slightly suppressed THP-induced vascular relaxation (Figure 2D and Table 1). Also, THP induced intracellular NO production in HUVECs (Figures 2E,F). These results indicated that the vascular relaxation effect of THP was dependent on endothelium and mediated by NO synthesis and bioavailability via PI3K/Akt/eNOS/NO/cGMP signaling pathway. However, cyclooxygenase-2 (COX2) inhibitor indomethacin (Indo) did not affect the vascular relaxation effect of THP. The vascular relaxant inhibition level by L-NAME pre-treatment or mechanical removal of endothelium (-endo) was coincident with the combination treatment of Indo and L-NAME (Figure 2A and Table 1). So, the secretion of PGI2 by endothelium was not likely to be involved in the vascular relaxation effect of THP. The vascular relaxation effect of THP was dependent on endothelium. The underlying mechanism of THP relaxing rat aorta was related to PI3K/Akt/eNOS/NO/cGMP signaling pathway and not dependent on the COX2/PGI2 signaling.
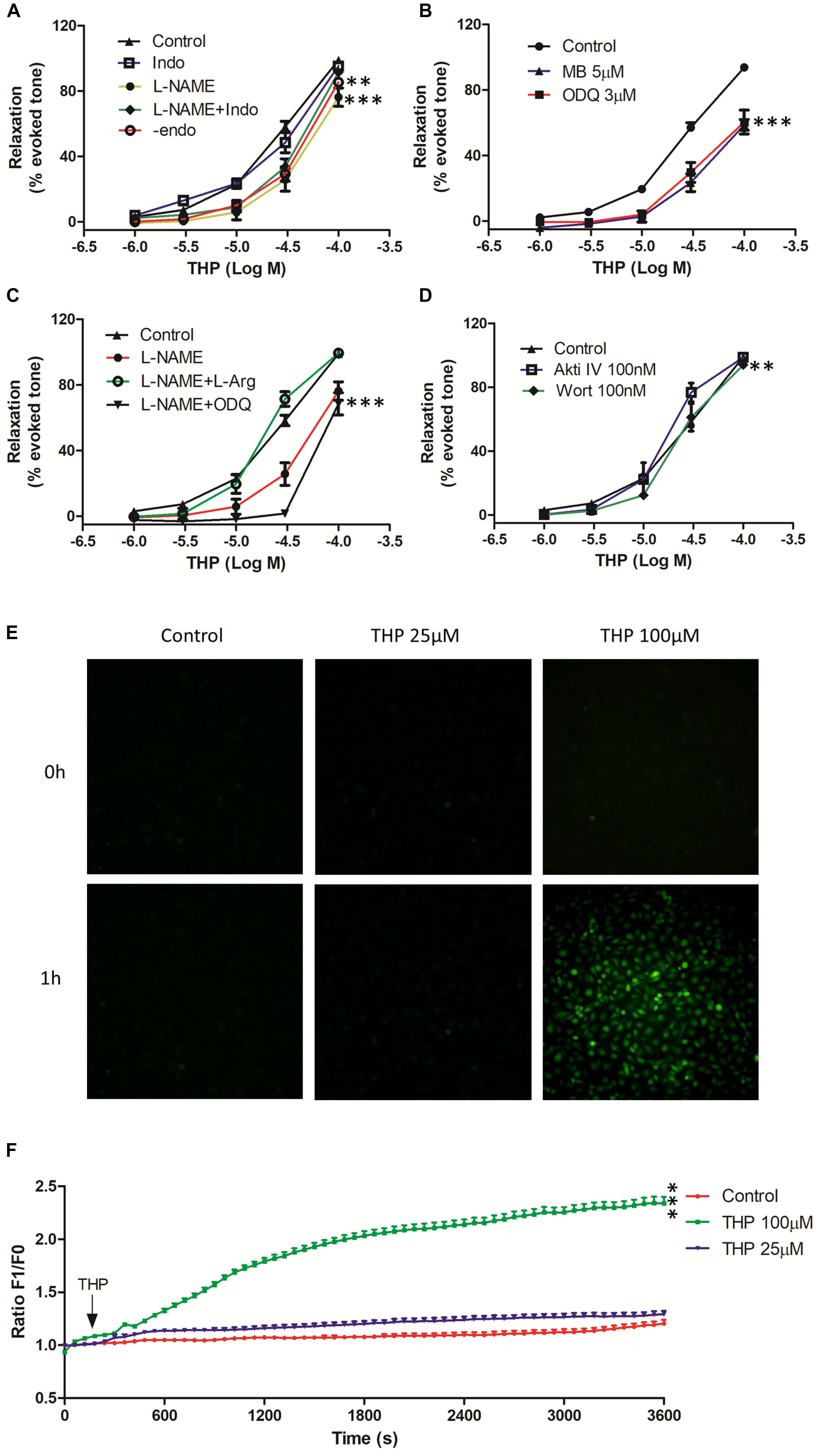
Figure 2. Tetrahydropalmatine-induced vascular relaxation was dependent on endothelium and related to nitric oxide (NO) production and bioavailability. The rat aortas were contracted by Phe (1 μM). (A) The effects of eNOS inhibitor (L-NAME, 100 μM), COX2 inhibitor (Indo, 1 μM) or mechanical removal of endothelium (-endo) on THP-induced vascular relaxation. (B) The effects of soluble guanylate cyclase inhibitors ODQ (3 μM) and methyl blue (MB, 5 μM) on THP-induced vascular relaxation. (C) The addition of L-arginine (L-Arg, 100 μM) reversed the inhibition effect of L-NAME on THP-induced vascular relaxation in Phe pre-contracted rat aorta. L-NAME (100 μM) together with ODQ (3 μM) reduced THP-induced vascular relaxation. (D) The effects of PI3K inhibitor wortmannin (Wort, 0.1 μM) and Akt inhibitor IV (Akti IV, 0.1 μM) on THP-induced vascular relaxation. (E) Representative images of NO staining of HUVECs under different conditions. HUVECs were stained with NO indicator DAF-FM DA (5 μM) for 30 min followed by NO real-time record using a real-time cell imaging system. After 5 min baseline NO recorded, HUVECs were treated with THP (25 and 100 μM) or DMSO (1:1000 v/v) and continually record to 1 h. (F) Statistic graph presented the real-time NO fluorescence change in HUVECs. F1 and F0 represented the fluorescent signal value at the indicated time point and 0 time point, respectively. Vascular relaxation presented as percentage of the evoked tone. Results were means ± SEM of more than three experiments. ∗∗P < 0.01, ∗∗∗P < 0.001 versus control group.
THP Reduced Vascular Tension and Intracellular Ca2+ Concentration by Blocking Calcium Channels
Phe and KCl induced vascular contraction by inducing Ca2+ influx though voltage-dependent calcium channels (VDCC) and receptor-operated calcium channels (ROCC) (Niazmand et al., 2014; Zhou et al., 2017). We found that aortas pre-incubated THP significantly inhibited Phe or KCl-induced contraction in a concentration-dependent manner (Figure 3A). In line with these results, pre-incubation THP also inhibited the cumulative addition of CaCl2 triggered the vascular tension of aorta in Ca2+-free depolarizing Krebs solution containing KCl or Phe (Figure 3B). In rat vascular smooth muscle cell A7r5, we also observed that THP reduced Ca2+ influx which was trigged by CaCl2 in Ca2+-free Krebs solution containing 60 mM KCl (Figures 3C,D). L-type calcium channel blocker nifedipine serve as positive control in these experiments. These results revealed that THP suppressed vascular tension and reduced the intracellular Ca2+ concentration by blocking calcium channels.
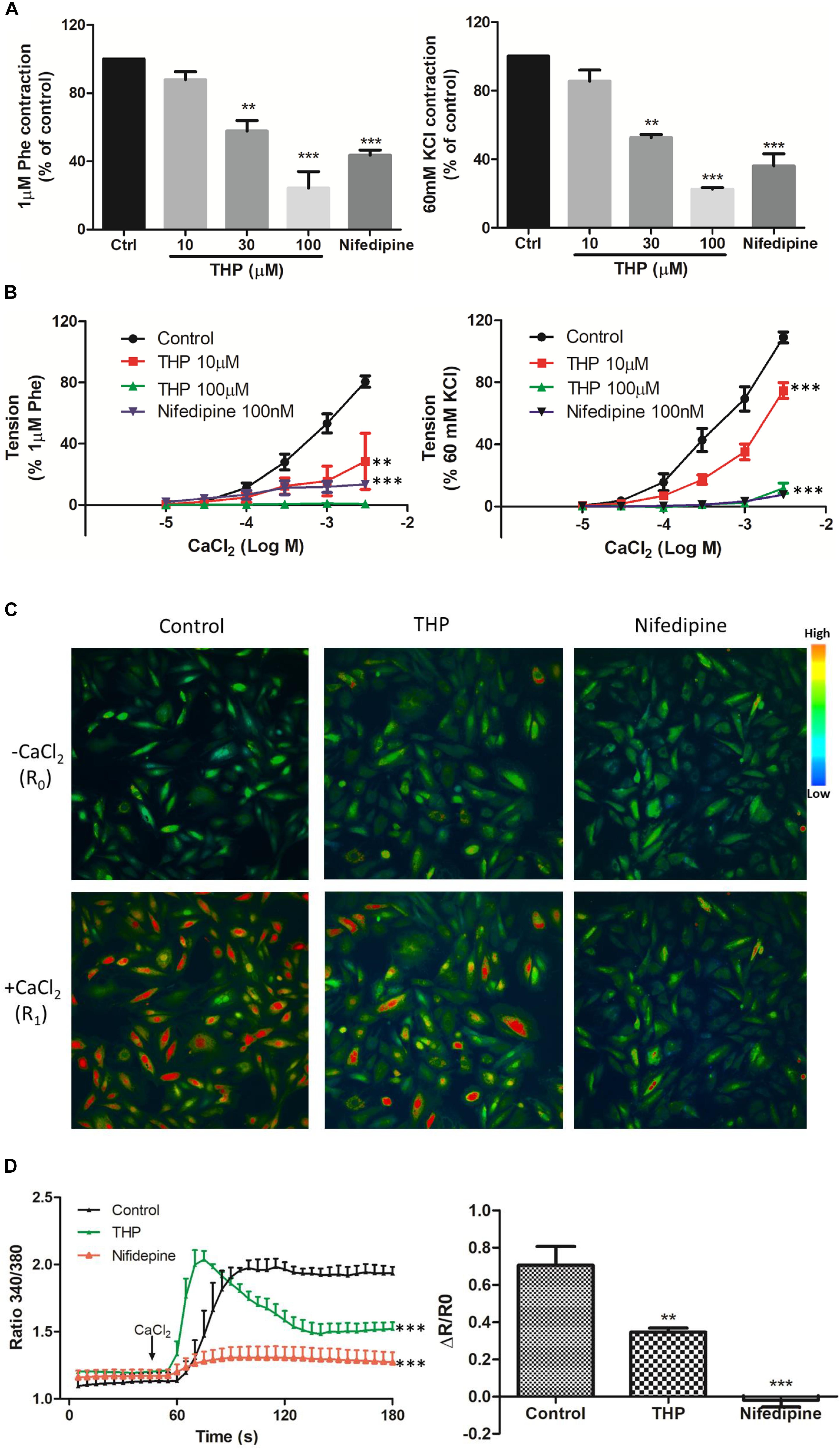
Figure 3. Tetrahydropalmatine-induced vascular relaxation was dependent on inhibition of Ca2+ influx. (A) Pre-treatment THP (10, 30, and 100 μM) inhibited KCl (60 mM) or Phe (1 μM) induced contraction of aorta in a concentration dependent manner. (B) Concentration-response curves showed pre-treatment THP (10 and 100 μM) inhibited CaCl2 (0.01–3 mM) induced contraction of aorta in Ca2+-free Krebs solution containing KCl (60 mM) or Phe (1 μM). (C) Representative images of calcium imaging. In A7r5 cells, pre-treatment THP (100 μM) or nifedipine (100 nM) inhibited the Ca2+ influx triggered by the addition of CaCl2 (2.5 mM) in Ca2+ free Krebs solution containing KCl (60 mM). (D) Time-course response and summarized graph of ratio 340 nm/380 nm (R). The change of ratio 340 nm/380 nm value (ΔR) was calculated as ratio 340 nm/380 nm value after the addition of CaCl2 for 2 min (R1) minus ratio 340 nm/380 nm value before the addition of CaCl2 (R0). Nifedipine served as positive control. Vascular contraction presented as percentage of the evoked tone. Results were means ± SEM of more than three experiments. ∗∗P < 0.01, ∗∗∗P < 0.001 versus control group.
THP Suppressed Intracellular Ca2+ Release-Induced Vascular Tension by Blocking Ryr and IP3 Receptors
The data in Figure 3 indicated THP relaxed rat aorta by blocking Ca2+ channels and inhibited Ca2+ influx. Whether THP affected cellular internal Ca2+ release is unknown. KCl and Phe-induced cellular depolarizing lead to Ca2+ transient releases from intracellular calcium stores in endoplasmic reticulum (Ratz et al., 2005; Qin et al., 2014). So, we immersed aortic rings in Ca2+ free Krebs solution to avoid Ca2+ influx from extracellular medium. We found pre-incubation THP suppressed KCl or Phe-induced contraction of aorta in Ca2+-free Krebs solution (Figures 4A,B). Intracellular Ca2+ mainly released from Ca2+ store through IP3-dependent channels and the Ca2+ signaling amplified by ryanodine receptors (Ryr) (del Valle-Rodríguez et al., 2003). Consistently, pre-treatment Ryr inhibitor ruthenium red (RR) or IP3 receptor inhibitor heparin reduced the vasorelaxation effect of THP in Ca2+ free Krebs solution (Figure 4C and Table 2). Thus, the underlying mechanism of THP inhibiting the vascular tension induced by intercellular Ca2+ release to cytosol was blocking Ryr and IP3 receptors.
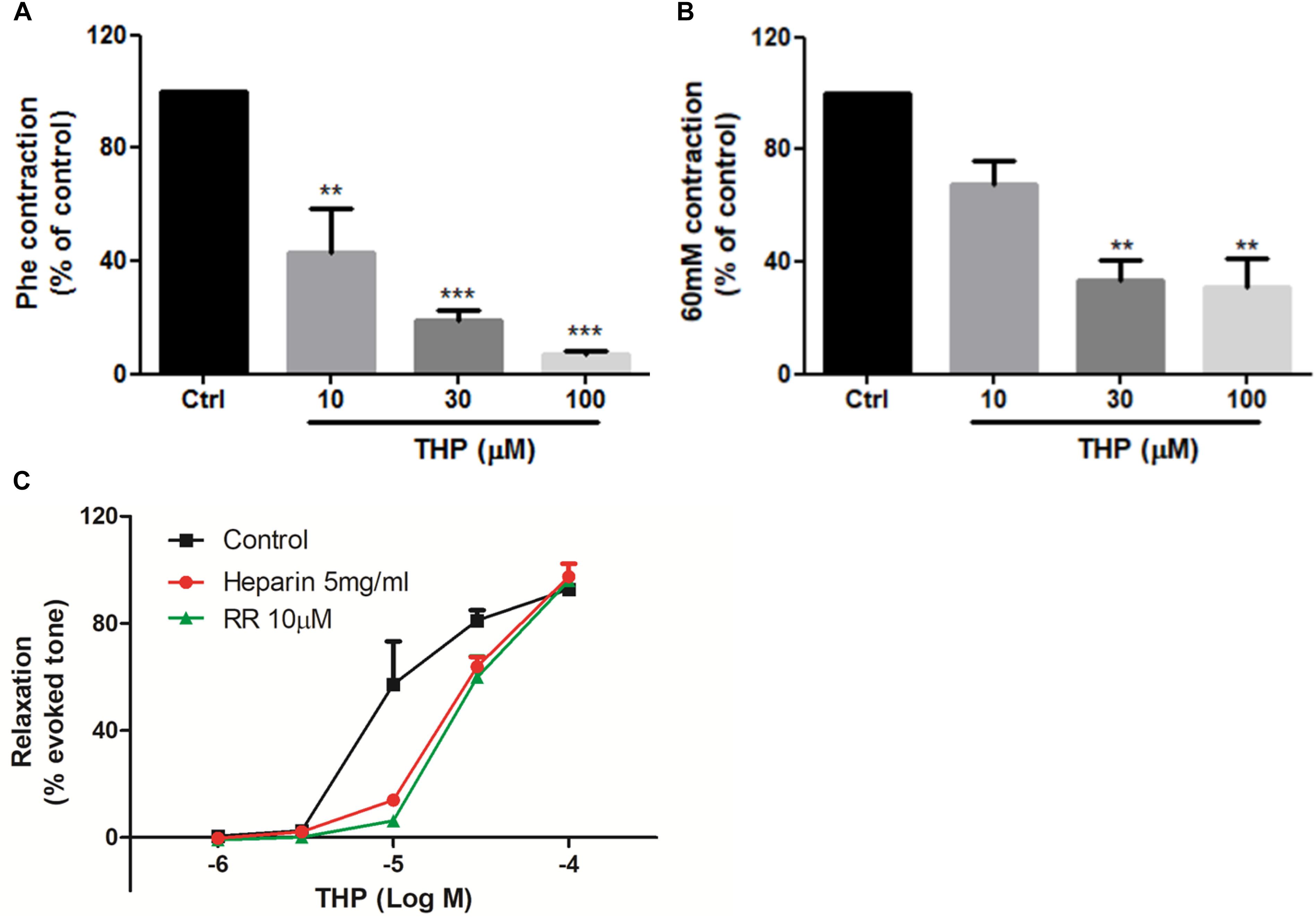
Figure 4. Tetrahydropalmatine inhibited intracellular Ca2+ release-induced vascular contraction. (A) Pre-treatment THP (10, 30, and 100 μM) suppressed KCl (60 mM) induced contraction of aorta in Ca2+-free Krebs solution. (B) Pre-treatment THP (10, 30, and 100 μM) suppressed Phe (1 μM) induced vascular contraction of aorta in Ca2+-free Krebs solution. (C) Pre-incubation Ryr inhibitor ruthenium red (RR, 10 μM) or IP3 receptor inhibitor heparin (5 mg/ml) attenuated THP induced rat aorta relaxation in Phe (1 μM) induced vascular tension in Ca2+-free Krebs solution. Vascular contraction and relaxation presented as percentage of control or evoked tone. Results were means ± SEM of more than three experiments. ∗∗P < 0.01, ∗∗∗P < 0.001 versus control group.
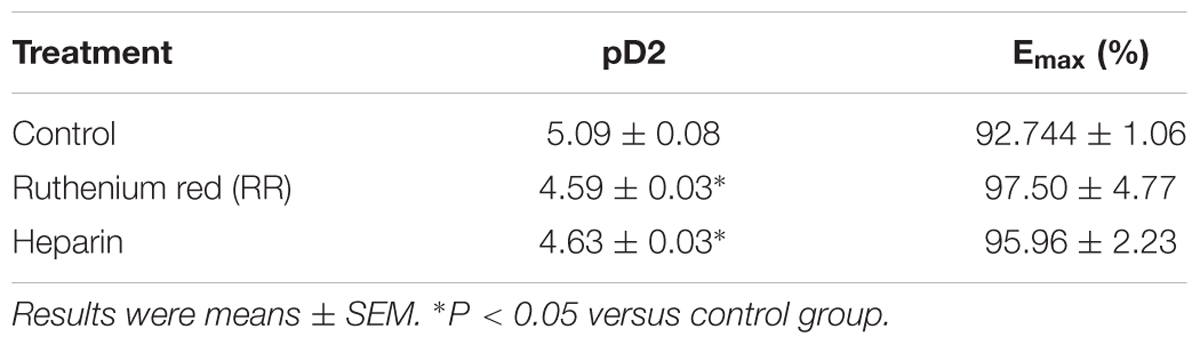
Table 2. The effects of IP3 and Ryr receptor inhibitor on THP (1–100 μM)-induced vascular relaxation in Ca2+ free Krebs solution.
THP Relaxed Rat Aorta by Partially Inhibition of K+ Channels
In this study, we evaluated the effects of K+ channel blockers on the vascular relaxationinduced by THP in both intact and endothelium denuded rat aortas. The results from Figure 5A and Table 1 show that the voltage-dependent K+ channel (Kv) blocker 4-aminopyridine (4-AP), ATP-sensitive K+ channel (KATP) blocker glibenclamide (Gly), and inward rectifying K+ channel blocker (BaCl2) attenuated THP induced vascular relaxation in intact rat aorta regardless of Ca2+-activated K+ channel (KCa) blocker tetraethylammonium (TEA). However, K+ channel blockers did not affect the vascular relaxation effect of THP in endothelium denuded rat aorta (Figure 5B and Table 1). So, we could conclude that the underlying mechanism of vasodilatory effect of THP was partially though blocking K+ channels.
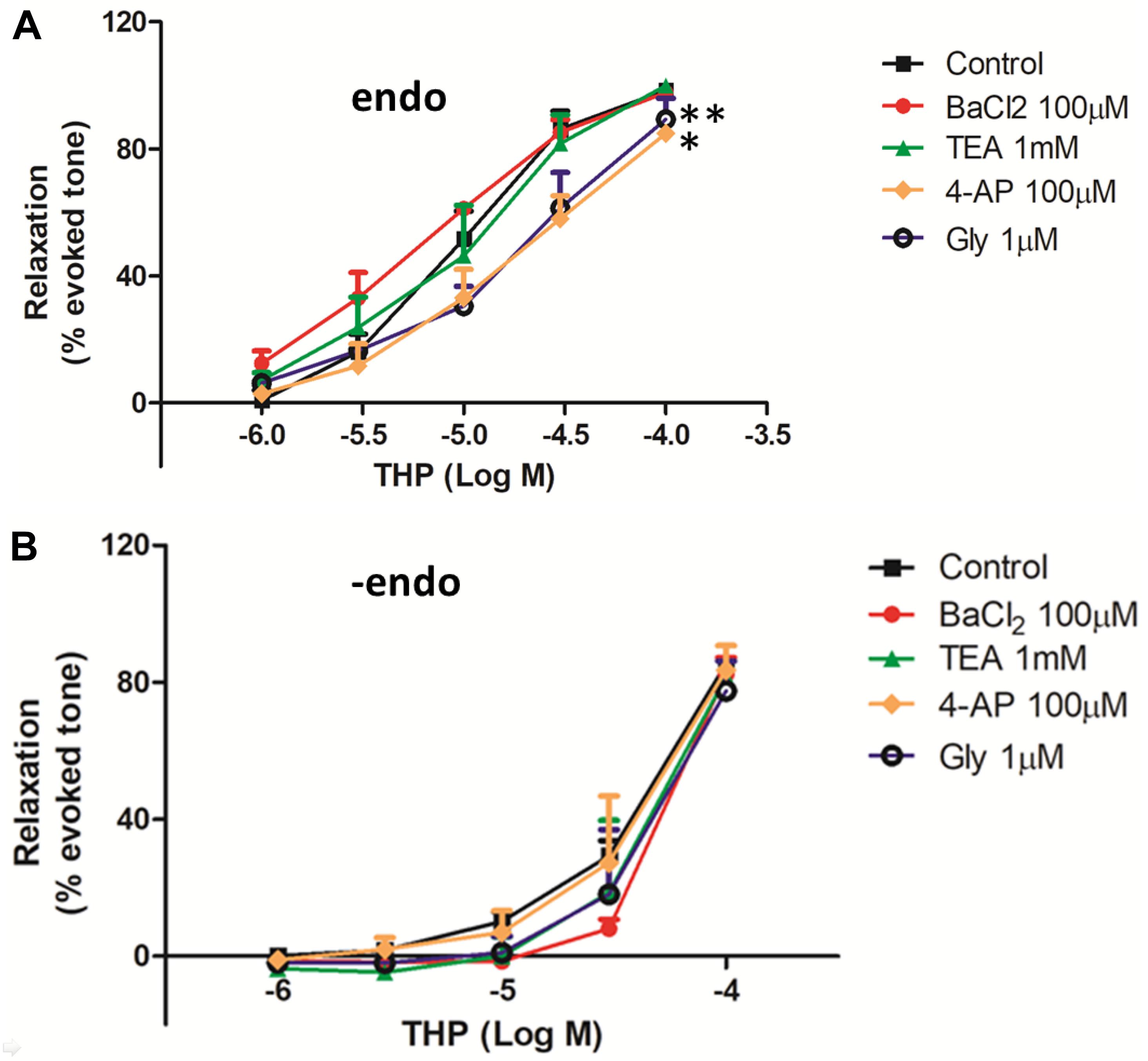
Figure 5. The effect of K+ channels on THP-induced vascular relaxation. The rat aortas were with intact endothelium (endo) (A) or mechanical remove the endothelium (-endo) (B). The aortic rings were pre-incubated with the voltage-dependent K+ channel (Kv) blocker 4-aminopyridine (4-AP), ATP-sensitive K+ channel (KATP) blocker glibenclamide (Gly), Ca2+-activated K+ channel (KCa) blocker tetraethylammonium (TEA), and inward rectifying K+ channel blocker (BaCl2) on the indicated concentration for 30 min, then the vascular contracted with Phe (1 μM) and relaxed by the accumulative addition of THP (1–100 μM). Vascular relaxation presented as a percentage of the evoked tone. Results were means ± SEM of more than three experiments. ∗P < 0.05, ∗∗P < 0.01 versus control group.
Discussion
The present study examined the vascular reactivity of Tetrahydropalmatine (THP, Figure 1A) in rat aorta. We demonstrated that THP relaxed rat aorta evoked by different contractors including phenylephrine (Phe), KCl, and U46619 (9,11-dideoxy-9α,11α-methanoepoxy Prosta-glandin F2α). Also, the underlying mechanisms were involved in PI3K/Akt/eNOS/NO/cGMP signaling pathway, Ca2+ channels and K+ channels.
Hypertension is a common cardiovascular disease and the pathogenesis related to the abnormal vascular contractility and vasodilation. Normal endothelium function plays a vital role in the garden of vascular physiological homeostasis and vascular relaxation (Touyz, 2000; Zhou et al., 2017; Maruhashi et al., 2019). In the present study, we found that THP relaxed KCl, Phe, and U46619 induced vascular contraction and the relaxation effect was most effective in Phe-contracted rat aorta (Figure 1B and Table 1). Also, the pre-treatment of β-adrenergic receptor blocker propranolol, angiotensin II receptor blocker losartan and PD123319 or angiotensin converting enzyme inhibitor enalapril enhanced THP-induced vascular relaxation (Figure 1C and Table 1). THP did not affect the vascular contraction induced by angiotensin II (Figure 1D). These results indicated that THP significantly relaxed vascular aorta and the underlying mechanism was not involved in β-adrenergic receptor blockade and RAS inhibition.
Endothelium dependent relaxing factors (EDRF) including nitric oxide (NO) and prostacyclin (PGl2) play key roles in the maintenance of the normal physical tension and relaxation function of vessel (Vanhoutte, 1989). NO was synthesized by endothelial nitric oxide synthase (eNOS) while cyclooxygenase-2 (COX2) and prostacyclin synthase (PGIS) mediated the production of PGI2 in endothelial cells (Dorris and Peebles, 2012). We found the vasorelaxation effect of THP was partially inhibited by eNOS inhibitor L-NAME in intact rat aorta regardless of COX2 inhibitor indomethacin (Indo). The inhibition effect of L-NAME + Indo was consistent to mechanical removal of endothelium (-endo) (Figure 2A and Table 1). Cyclic guanosine monophosphate (cGMP) which is generated by guanylyl cyclase mediates the bioavailability of NO and drives NO-dependent vascular relaxation in smooth muscle cells (Furchgott and Vanhoutte, 1989). Pre-treatment of guanylyl cyclase inhibitor ODQ and methyl blue (MB) also reduced the vascular relaxation effect of THP. In addition, L-arginine (L-Arg) which has higher binding ability to eNOS than L-NAME reversed the inhibition effect of L-NAME in THP-induced vascular relaxation. Combination of L-NAME and ODQ significantly inhibited THP-induced vascular relaxation (Figure 2C). PI3K/Akt activates the phosphorylation of eNOS and subsequently generates NO in endothelium. Their inhibitors wortmannin (Wort) and Akt inhibitor IV (Akti IV) slightly suppressed the vascular relaxation effect of THP (Figure 2D and Table 1). In addition, THP induced intracellular NO accumulation in HUVECs (Figures 2E,F). Thus, we could conclude that the underlying mechanism of THP-induced vascular relaxation was endothelium-dependently and involved in the PI3K/Akt/eNOS/NO/cGMP signaling pathway rather than COX2/PIG2. A previous study also reported that L-THP increased protein expression of phosphatidylinositol 3-kinase (PI3K) and phosphorylation of Akt and eNOS in myocardium (Han et al., 2012). So, PI3K/Akt/eNOS/NO/cGMP signaling pathway seems like an important pharmacological target of THP in cardiovascular disease.
The results in Figure 2A and Table 1 shown denuding aorta endothelium reduced the Emax of THP from 98.97 ± 1.31 to 85.58 ± 3.05% and indicated THP relaxed rat aorta mainly in an endothelium-independent manner. And the regulation of intracellular Ca2+ concentration by ion channels especially Ca2+ channels dramatically gardens vascular contraction and relaxation. We found THP inhibited the Phe or KCl induced vascular contraction concentration dependently (Figure 3A). And THP reduced CaCl2 triggered vascular tension of aorta in Ca2+-free depolarizing Krebs solution containing KCl or Phe (Figure 3B). Phe and KCl increased intracellular Ca2+ concentration by inducing extracellular Ca2+ influx and internal Ca2+ stores release (Niazmand et al., 2014; Zhou et al., 2017). In order to explore whether THP suppressed intracellular Ca2+ concentration, we measured the effect of THP on the cytosol Ca2+ amount change triggered by CaCl2 in Ca2+-free Krebs solution containing KCl in smooth muscle cells A7r5 by calcium imaging system. We found THP reduced the cytosol Ca2+ concentration in A7r5 cells (Figures 3C,D). L-type channel blocker nifedipine serve as positive control in these experiments. So, we could conclude that THP inhibited the vascular tension by blocking the Ca2+ influx. In addition, THP inhibited Phe and KCl-induced rat aorta contraction in the absence of extracellular Ca2+ concentration-dependently (Figures 4A,B). It seems that THP blocked the Ca2+ released from intracellular Ca2+ store and subsequently reduced the Phe and KCl induced transient vascular tension in Ca2+ free Krebs solution. Consistently, we found the inhibition of the IP3 and Ryr receptor which mediated the Ca2+ release from intracellular Ca2+ store attenuated the vascular relaxation effect of THP (Figure 4C and Table 2). Thus, we could conclude that THP reduced the intracellular Ca2+ release induced vascular tension by blocking IP3 and Ryr receptors. The Ca2+ and Ca2+/Calmodulin (CaM) complex activates smooth muscle myosin light chain kinase (MLCK) and induced calcium sensitization during vascular contraction (Martinsen et al., 2014; Zhou et al., 2017). However, THP relaxed PMA (Phorbol 12-myristate 13-acetate) which trigger calcium sensitization by active PKC and ROCK signaling pathway induced vascular contraction at the concentration of 300 μM (Supplementary Figure S1). THP also enhanced vascular relaxation effect of bradykinin and protein expression of bradykinin in endothelial cells (Supplementary Figure S2). So, THP relaxed vascular tension may also relate to the inhibition of intracellular calcium sensitization and the production of bradykinin.
Vascular tension and relaxation were also regulated by K+ channels. In this study, we found the voltage-dependent K+ channel (Kv) blocker 4-aminopyridine (4-AP), ATP-sensitive K+ channel (KATP) blocker glibenclamide (Gly), and inward rectifying K+ channel blocker (BaCl2) attenuated THP induced vascular relaxation in intact rat aorta rather than endothelium denuded rat aorta (Figure 5). Li et al. (2017) also found that THP inhibited the kinetic activity of Kv1.5 channels. Thus, we could conclude that THP relaxed rat aorta partially though K+ channels and endothelium also plays a key role.
However, this study has some limitations. Mas-receptor (MS-R) which presents opposite function to angiotensin II receptor type 1 plays a vital role in vasodilation (Katsi et al., 2018) and whether THP could activate MS-R is unknown. THP induced the production of bradykinin while the underlying mechanism needs to be further studied. Moreover, whether THP could lower the blood pressure in vivo and protect the hypertension-related endothelium dysfunction and other complications also needs to be studied in the future.
In summary, THP relaxed vessel contracted by different contractors including Phe, KCl, and U46619. The underlying mechanism was both endothelium-dependent and independent and was involved in the PI3K/Akt/eNOS/NO/cGMP signaling pathway as well as the Ca2+ and K+ channels. Thus, THP may be a potential treatment for cardiovascular disease like hypertension in clinics.
Author Contributions
Z-YZ conducted the experiments related to vascular relaxation effect of THP and wrote the manuscript. W-RZ conducted the experiments about the mechanism of vascular relaxation effect of THP. W-TS conducted the cell culture and related experiments. YX, Z-LM, and L-QZ analyzed the data of these experiments. J-YT and J-GX designed the experiments. X-LC and QY designed the experiments and revised the manuscript.
Funding
This study was supported by the Science and Technology Commission of Shanghai (16ZR1437600), Shanghai Hospital Development Center (SHDC12015313), Shanghai University of Traditional Chinese Medicine (A1-U1720501030101), National Natural Science Foundation of China (81603549, 81673726, and 81760860), and National Health Commission of Shanghai [GWIV-28 and ZY-(2018-2020)-FWTX-8001].
Conflict of Interest Statement
The authors declare that the research was conducted in the absence of any commercial or financial relationships that could be construed as a potential conflict of interest.
Supplementary Material
The Supplementary Material for this article can be found online at: https://www.frontiersin.org/articles/10.3389/fphar.2019.00336/full#supplementary-material
References
Armario, P., Calhoun, D. A., Oliveras, A., Blanch, P., Vinyoles, E., Banegas, J. R., et al. (2017). Prevalence and clinical characteristics of refractory hypertension. J. Am. Heart Assoc. 6:e007365. doi: 10.1161/JAHA.117.007365
Cao, G., Zhang, Y., Zhu, L., Zhu, J., Zhao, N., Dong, N., et al. (2018). The inhibitory effect of levo-tetrahydropalmatine on the methamphetamine-induced spatial memory impairment in mice. Neurosci. Lett. 672, 34–39. doi: 10.1016/j.neulet.2018.02.018
Chueh, F. Y., Hsieh, M. T., Chen, C. F., and Lin, M. T. (1995). Hypotensive and bradycardic effects of dl-tetrahydropalmatine mediated by decrease in hypothalamic serotonin release in the rat. Jpn. J. Pharmacol. 69, 177–180. doi: 10.1254/jjp.69.177
del Valle-Rodríguez, A., López-Barneo, J., and Ureña, J. (2003). Ca2+ channel-sarcoplasmic reticulum coupling: a mechanism of arterial myocyte contraction without Ca2+ influx. EMBO J. 22, 4337–4345. doi: 10.1093/emboj/cdg432
Dorris, S. L., and Peebles, R. S. (2012). PGI2 as a regulator of inflammatory diseases. Mediat. Inflamm. 2012:926968. doi: 10.1155/2012/926968
Fu, Y. C., Hsieh, M. T., Chen, C. F., and Lin, M. T. (1995). DL-Tetrahydropalmatine-produced hypotension and bradycardia in Rats through the Inhibition of central nervous dopaminergic mechanisms. Pharmacology 51, 237–244. doi: 10.1159/000139365
Furchgott, R. F., and Vanhoutte, P. M. (1989). Endothelium-derived relaxing and contracting factors. FASEB J. 3, 2007–2018. doi: 10.1096/fasebj.3.9.2545495
Garcia, V., Gilani, A., Shkolnik, B., Pandey, V., Zhang, F. F., Dakarapu, R., et al. (2017). 20-HETE signals through G-protein–coupled receptor GPR75 (Gq) to affect vascular function and trigger hypertension. Circ. Res. 120, 1776–1788. doi: 10.1161/CIRCRESAHA.116.310525
Han, Y., Zhang, W., Tang, Y., Bai, W., Yang, F., Xie, L., et al. (2012). l-Tetrahydropalmatine, an active component of Corydalis yanhusuo W.T Wang, protects against myocardial ischaemia-reperfusion injury in rats. PLoS One 7:e38627. doi: 10.1371/journal.pone.0038627
Kang, D. W., Moon, J. Y., Choi, J. G., Kang, S. Y., Ryu, Y., Park, J. B., et al. (2016). Antinociceptive profile of levo-tetrahydropalmatine in acute and chronic pain mice models: role of spinal sigma-1 receptor. Sci. Rep. 6:37850. doi: 10.1038/srep37850
Katsi, V., Maragkoudakis, S., Marketou, M., Tsioufis, C., Parthenakis, F., and Tousoulis, D. (2018). The role of angiotensin-(1-7)/Mas axis and angiotensin type 2 receptors in the central nervous system in cardiovascular disease and therapeutics: a riddle to be solved. Curr. Vasc. Pharmacol. doi: 10.2174/1570161117666181105154843 [Epub ahead of print].
Li, K., Pi, M. S., and Li, X. T. (2017). The inhibitory effects of levo-tetrahydropalmatine on rat Kv1.5 channels expressed in HEK293 cells. Eur. J. Pharmacol. 809, 105–110. doi: 10.1016/j.ejphar.2017.05.020
Lu, J., Lu, Y., Wang, X., Li, X., Linderman, G. C., Wu, C., et al. (2017). Prevalence, awareness, treatment, and control of hypertension in China: data from 1 ⋅ 7 million adults in a population-based screening study (China PEACE Million Persons Project). Lancet 390, 2549–2558. doi: 10.1016/S0140-6736(17)32478-9
Ma, C., Wei, Z., Tang, Q., and Huang, S. (2018). The impact of group-based Tai chi on health-status outcomes among community-dwelling older adults with hypertension. Heart Lung 47, 337–344. doi: 10.1016/j.hrtlng.2018.04.007
Mao, X. W., Pan, C. S., Huang, P., Liu, Y. Y., Wang, C. S., Yan, L., et al. (2015). Levo-tetrahydropalmatine attenuates mouse blood-brain barrier injury induced by focal cerebral ischemia and reperfusion: involvement of Src kinase. Sci. Rep. 5:11155. doi: 10.1038/srep11155
Martinsen, A., Dessy, C., and Morel, N. (2014). Regulation of calcium channels in smooth muscle: new insights into the role of myosin light chain kinase. Channels 8, 402–413. doi: 10.4161/19336950.2014.950537
Maruhashi, T., Kinoshita, Y., Kajikawa, M., Kishimoto, S., Matsui, S., Hashimoto, H., et al. (2019). Relationship between home blood pressure and vascular function in patients receiving antihypertensive drug treatment. Hypertens. Res. doi: 10.1038/s41440-019-0240-8 [Epub ahead of print].
Niazmand, S., Harandizadeh, F., Mahmoudabady, M., Hosseini, M., Hasanzadeh, M., and Fereidouni, E. (2014). Mechanism of vasorelaxation induced by Achillea wilhelmsii in rat isolated thoracic aorta. Adv. Biomed. Res. 3:91. doi: 10.4103/2277-9175.128470
Qin, X., Hou, X., Zhang, M., Liang, T., Zhi, J., Han, L., et al. (2014). Relaxation of rat aorta by farrerol correlates with potency to reduce intracellular calcium of VSMCs. Int. J. Mol. Sci. 15, 6641–6656. doi: 10.3390/ijms15046641
Ratz, P. H., Berg, K. M., Urban, N. H., and Miner, A. S. (2005). Regulation of smooth muscle calcium sensitivity: KC1 as a calcium-sensitizing stimulus. Am. J. Physiol. Cell Physiol. 288, 769–783. doi: 10.1152/ajpcell.00529.2004
Sun, F., and Li, D. X. (1989). Effects of l-tetrahydropalmatine on isolated rabbit arterial strips. Zhongguo Yao Li Xue Bao 10, 30–33.
Sun, J., and Buys, N. (2015). Community-based mind-body meditative tai chi program and its effects on improvement of blood pressure, weight, renal function, serum lipoprotein, and quality of life in chinese adults with hypertension. Am. J. Cardiol. 116, 1076–1081. doi: 10.1016/j.amjcard.2015.07.012
Sun, R., Song, Y., Li, S., Ma, Z., Deng, X., Fu, Q., et al. (2018). Levo-tetrahydropalmatine attenuates neuron apoptosis induced by cerebral ischemia-reperfusion injury: involvement of c-abl activation. J. Mol. Neurosci. 65, 391–399. doi: 10.1007/s12031-018-1063-9
Touyz, R. M. (2000). Molecular and cellular mechanisms regulating vascular function and structure–implications in the pathogenesis of hypertension. Can. J. Cardiol. 16, 1137–1146.
Vanhoutte, P. M. (1989). Endothelium and control of vascular function. State of the art lecture. Hypertension 13(6 pt 2), 658–667.
Wang, X., San Cheang, W., Yang, H., Xiao, L., Lai, B., Zhang, M., et al. (2015). Nuciferine relaxes rat mesenteric arteries through endothelium-dependent and-independent mechanisms. Br. J. Pharmacol. 172, 5609–5618. doi: 10.1111/bph.13021
Wang, X., Zhao, R., Zhang, H., Zhou, M., Zhang, M., and Qiao, T. (2018). Levo-tetrahydropalmatine attenuates progression of abdominal aortic aneurysm in an elastase perfusion rat model via suppression of matrix metalloproteinase and monocyte chemotactic protein-1. Med. Sci. Monit. 24, 652–660. doi: 10.12659/MSM.906153
Wu, L., Ling, H., Li, L., Jiang, L., and He, M. (2010). Beneficial effects of the extract from Corydalis yanhusuo in rats with heart failure following myocardial infarction. J. Pharm. Pharmacol. 59, 695–701. doi: 10.1211/jpp.59.5.0010
Xiao, C., Yang, Y., and Zhuang, Y. (2016). Effect of health qigong ba duan jin on blood pressure of individuals with essential hypertension. J. Am. Geriatr. Soc. 64, 211–233. doi: 10.1111/jgs.13913
Xiong, X., Yang, X., Liu, W., Duan, L., Wang, P., You, H., et al. (2018). Therapeutic efficacy and safety of traditional chinese medicine classic herbal formulalongdanxiegandecoction for hypertension: a systematic review and meta-analysis. Front. Pharmacol. 9:466. doi: 10.3389/fphar.2018.00466
Xiong, X. J., Wang, P. Q., and Li, S. J. (2019). Blood-letting therapy for hypertension: a(systematic) review and meta-analysis of randomized controlled trials. Chin. J. Integr. Med. 25, 139–146. doi: 10.1007/s11655-018-3009-2
Yang, B. R., Yu, N., Deng, Y. H., Hoi, P. M., Yang, B., Liu, G. Y., et al. (2015). L-tetrahydropalamatine inhibits tumor necrosis factor-alpha-induced monocyte-endothelial cell adhesion through downregulation of intercellular adhesion molecule-1 and vascular cell adhesion molecule-1 involving suppression of nuclear factor-kappa B signaling pathway. Chin. J. Integr. Med. 21, 361–368. doi: 10.1007/s11655-015-2165-7
Yang, X., Yang, G., Li, W., Zhang, Y., and Wang, J. (2018). Therapeutic effect of ilex hainanensis merr. Extract on essential hypertension: a systematic review and meta-analysis of randomized controlled trials. Front. Pharmacol. 9:424. doi: 10.3389/fphar.2018.00424
Zhang, M. Y., Liu, Y. P., Zhang, L. Y., Yue, D. M., Qi, D. Y., Liu, G. J., et al. (2015). Levo-tetrahydropalmatine attenuates bone cancer pain by inhibiting microglial cells activation. Mediat. Inflamm. 2015:752512. doi: 10.1155/2015/752512
Zhang, Y., Sha, R., Wang, K., Li, H., Yan, B., and Zhou, N. (2018). Protective effects of tetrahydropalmatine against ketamine-induced learning and memory injury via antioxidative, anti-inflammatory and anti-apoptotic mechanisms in mice. Mol. Med. Rep. 17, 6873–6880. doi: 10.3892/mmr.2018.8700
Zhou, Z. Y., Xu, J. Q., Zhao, W. R., Chen, X. L., Jin, Y., Tang, N., et al. (2017). Ferulic acid relaxed rat aortic, small mesenteric and coronary arteries by blocking voltage-gated calcium channel and calcium desensitization via dephosphorylation of ERK1/2 and MYPT1. Eur. J. Pharmacol. 815, 26–32. doi: 10.1016/j.ejphar.2017.10.008
Keywords: Tetrahydropalmatine, vasorelaxation, calcium influx, endothelium function, potassium channel, calcium channel, calcium sensitization
Citation: Zhou Z-Y, Zhao W-R, Shi W-T, Xiao Y, Ma Z-L, Xue J-G, Zhang L-Q, Ye Q, Chen X-L and Tang J-Y (2019) Endothelial-Dependent and Independent Vascular Relaxation Effect of Tetrahydropalmatine on Rat Aorta. Front. Pharmacol. 10:336. doi: 10.3389/fphar.2019.00336
Received: 23 October 2018; Accepted: 19 March 2019;
Published: 16 April 2019.
Edited by:
Francesco Rossi, Università degli Studi della Campania “Luigi Vanvitelli”, ItalyReviewed by:
Vincenzo Brancaleone, University of Basilicata, ItalyFrancesco Squadrito, University of Messina, Italy
Copyright © 2019 Zhou, Zhao, Shi, Xiao, Ma, Xue, Zhang, Ye, Chen and Tang. This is an open-access article distributed under the terms of the Creative Commons Attribution License (CC BY). The use, distribution or reproduction in other forums is permitted, provided the original author(s) and the copyright owner(s) are credited and that the original publication in this journal is cited, in accordance with accepted academic practice. No use, distribution or reproduction is permitted which does not comply with these terms.
*Correspondence: Xin-Lin Chen, aGVhbDczNzRAMTYzLmNvbQ== Jing-Yi Tang, ZHJfdGFuZ0AxNjMuY29t
†These authors have contributed equally to this work