- 1Faculty of Pharmacy, Hubei University of Chinese Medicine, Wuhan, China
- 2Key Laboratory of Traditional Chinese Medicine Resources and Chemistry of Hubei Province, Wuhan, China
- 3Collaborative Innovation Center of Traditional Chinese Medicine of New Products for Geriatrics Hubei Province, Wuhan, China
The present study explored the possible bioactive ingredients and target protein of Rostellularia procumbens (L.) Nees. Firstly, we found that the ethyl acetate extraction obtained from R. procumbens could inhibit platelet aggregation. Then, gene chip was used to investigate differentially expressed genes and blood absorption compounds were investigated using high performance liquid chromatography-mass spectrometry characterization (LC-MS). Depending on the results of gene chip and LC-MS, the targets of blood absorption compounds were predicted according to the reverse pharmacophore matching model. The platelet aggregation-related genes were discovered in databases, and antiplatelet aggregation-related gene targets were selected through comparison. The functions of target genes and related pathways were analyzed and screened using the DAVID database, and the network was constructed using Cytoscape software. We found that integrin αIIbβ3 had a highest degree, and it was almost the intersection of all pathways. Then, blood absorption compounds were screened by optical turbidimetry. Western blot (WB) revealed that justicidin B separated from the ethyl acetate fraction may inhibit the expression of integrin αIIbβ3 protein. For the first time, we used Prometheus NT.48 and MST to detect the stability of this membrane protein to optimize the buffer and studied the interaction of justicidin B with its target protein. To our best knowledge, this is the first report to state that justicidin B targets the integrin αIIbβ3 protein. We believe that our findings can provide a novel target protein for the further understanding of the mechanism of R. procumbens on platelet aggregation.
Introduction
Integrin αIIbβ3 is a major platelet-surface receptor for the regulation of platelet aggregation and thrombosis. Fibrinogen is attached to the integrin αIIbβ3 protein of one platelet and is linked to the integrin αIIbβ3 protein of another platelet. At the same time, the platelets are grouped together to cause a platelet aggregation cascade. Therefore, integrin αIIbβ3 is a key protein for platelet aggregation (Coller and Shattil, 2008; Yun et al., 2016; Andrews and Gardiner, 2017; Bender et al., 2017; Chatterjee and Gawaz, 2017).
Rostellularia procumbens (L.) Nees (Acanthaceae) is widely distributed in the Taiwan Province and the southwest provinces and has been proven to have a huge potential for the development of Chinese medicine owing to its plant resources, chemical constituents, pharmacological action, and clinical application. It has complex chemical composition (Savithramma et al., 2007; Joshi and Joshi, 2000; Committee, 2011).
In addition, reports have shown that R. procumbens has significant pharmacological properties such as anti-viral and anti-tumoral (Chen et al., 1995; Corrêa and Alcântara, 2012). It has also been noted that aqueous extracts of R. procumbens decrease platelet aggregation (Chen et al., 1996). According to preliminary experiments, ethyl acetate extract is the active fraction. Animal experimentation conducted analyze this fraction. Then, gene chip was used to investigate differentially expressed genes. Blood absorption compounds were investigated using LC-MS. Targets of blood absorption compounds were predicted according to the reverse pharmacophore matching model. The platelet aggregation-related genes were found in databases, and antiplatelet aggregation-related gene targets were selected through comparison. The functions of target genes and related pathways were analyzed and screened using the DAVID database, and the network of antiplatelet aggregation effect of blood absorption compounds was constructed using Cytoscape software. However, the detailed molecular interaction between justicidin B and its target is still unknown. Firstly, blood absorption compounds were screened by optical turbidimetry. Prometheus NT.48 is used to detect protein stability and screen buffer (Maschberger et al.). Then, we compared the two models of microscale thermophoresis (MST) and used NT.115 to verify the interaction between the compound and the integrin αIIbβ3 protein (van den Bogaart et al., 2012; Batoulis et al., 2016; Sparks and Fratti, 2019; Vinothkannan Ravichandran et al., 2018).
In this study, according to preliminary experiments, blood absorption compounds of R. procumbens were screened by serum pharmacological. Gene chip and network pharmacology were used to find the target. Then, the interaction between justicidin B and the membrane protein integrin αIIbβ3 was verified by Western blot (WB) and MST. It would lay the groundwork for understanding the molecular mechanism involved in the inhibition of platelet aggregation by R. procumbens.
Materials and Methods
Chemical and Materials
The ethyl acetate extract was obtained from the Key Laboratory for Traditional Chinese Medicine Resources and Chemistry of Hubei Province (Chen, 2012; Su, 2014; Wu et al., 2013) [ethyl acetate (EtOAc), Lichrosolv, purity ≥ 99.9%]. Acetonitrile (ACN) was of LC-MS grade and was purchased from Thermo-Fisher (Pittsburgh, PA, USA). Deionized water was made available in-lab using a Milli-Q purification instrument (Millipore, Bedford, MA, USA). 5-Hydroxytryptophan was obtained from National Institutes for Food and Drug Control (China). Thrombin was purchased from BioMed Lublin, Poland. Arachidonic acid (AA), bovine serum albumin (BSA), adenosine diphosphate (ADP), β-acetyl-γ-O-hexadecyl-L-α-phospharidylcholine hydrate (PAF), 12-O-tetradecanoylphorbol-13-acetate (PMA), and dimethyl sulfoxide (DMSO) were purchased from Sigma (St. Louis, MO, USA). RNeasy Mini Kit and RNase-free DNase I were purchased from QIAGEN. Pico Reagent Kit, GeneChip90 Hybridization, Wash, and Stain Kit were purchased from Affymetrix. Integrin αIIbβ3 monoclonal antibody was purchased from SAB (Maryland, USA), and β-actin and anti-mouse IgG were purchased from Cell Signaling (Boston, USA).
Experimental Animals
SD male rats (190–230 g) were randomly divided into two groups of 16 animals per group, treated orally as follows: control group received 0.5% CMC-Na, groups of ethyl acetate extract (97.20 g/ml) (fasting for 12 h before intragastric administration, administered by intragastric administration at 1 ml/200 mg twice a day for 3 days). After the last administration for 1.5 h, we took 5–8 ml of blood from the femoral artery. All experimental procedures were approved by Animal Care and Use Committee of Institute of Materia Medica, People’s Republic of China.
Extraction and Detection of Total RNA in Two Groups of Platelets
The platelet of the control group and ethyl acetate group at 4°C and 12,000 rpm was centrifuged for 10 min using RNeasy Mini Kit separation of total RNA. The RNA concentration and A260/A280 ratio were determined using an SMA 3000 microspectrophotometer (Meriton, China). The results showed that the ratio was 2.00:2.05, indicating a high purity of the extracted RNA, which was deemed suitable for subsequent analysis. After this, a total of 1 µg RNA was used for 1% agarose gel electrophoresis. The ratio of 28S/18S was then determined using a JS-380A Bioanalyzer (Shanghai, China) to determine the quality of RNA. If the RNA integrity number (RIN) > 7.0 and 28S/18S > 0.7, samples were transcribed by a Pico Reagent Kit. Following this, samples were prepared using a GeneChip90 Hybridization, Wash, and Stain Kit (Affymetrix, Thermo Fisher Scientific, Inc.). Then, chips were scanned using a GeneChip Scanner 3000 (Affymetrix, Thermo Fisher Scientific, Inc.).
Detection of Blood Absorption Compounds
Justicidin B, chinensinaphthol methyl ether, and 6’-hydroxy justicidin B were weighed accurately, and 1 mg/ml control solution with methanol was prepared. The control solution was stored at 4°C for use. The supernatant that was treated by solid phase microextraction cartridge was collected and dried by nitrogen after centrifugation (12,000 r/min, 10 min). Before the liquid injection, 200 μl acetonitrile was used for redissolution and the supernatant was determined by HPLC-DAD-ESI-MS after filtration.
The chromatography analysis was performed on an Agilent XDB-C18 column (150 mm × 4.6 mm, 4 µm) at 25°C; the mobile phase was a mixture of aqueous solutions containing water (A) and acetonitrile (B). The gradient elution procedure was made as follows: 0–14 min, 26% B; 14–51 min, 26–38.5% B; 51–80 min, 38.5% B. The injection volume was 100 μl, and the flow rate was 1 ml/min. The wavelength of 190–690 nm was used for the detection. Mass spectrometry detection was set as follows: capillary temperature, 200°C; source voltage, 4.5 kV for the positive ion mode. The mass range was from 50 to 1,600. Blood absorption compounds were identified by accurate mass, MS/MS ion fragment pattern, and retention time of LC and then were validated by available standard.
Prediction and Analysis of Differentially Expressed Target Genes
Based on the results of the gene chip and LC-MS, we conducted a network pharmacology study. The PharmMapper Server (http://lilab.ecust.edu.cn/pharmmapper/index.php) is a freely accessed web server used to identify potential target candidates for given probe blood absorption compounds using a pharmacophore mapping approach. GeneCards (https://www.genecards.org/) and MalaCards (http://www.malacards.org/) were used for potential target screening from the results of gene chip. All potential target genes were collected and uploaded to the DAVID (https://david.ncifcrf.gov/summary.jsp) database. Following this, the functions and signaling pathways of target genes as well as pathway enrichment were investigated via Gene Ontology (GO; http://geneontology.org/) and Kyoto Encyclopedia of Genes and Genomes (KEGG) pathway analyses (http://www.genome.jp/kegg/ko.html).
Construction of the Network
The blood absorption compounds and target genes were imported into Cytoscape 3.6.1 software to build an active compound/target gene/pathway network and an active compound/platelet aggregation-related target gene network. The blood absorption compounds and target genes were input as the node. If there was a connection between two nodes, edge was used to show the connection.
The network was then analyzed with the network analyze function. High degree gene targets in the protein interaction network were analyzed. According to the results of KEGG enrichment, the pathways with higher counts were selected to analyze their key targets. Meanwhile, the genes suitable for the analysis of the targets were obtained through comparative analysis from the literature and database.
Screening of Active Compounds Inhibiting Platelet Aggregation
Platelet-rich plasma (PRP) was prepared by centrifugation of fresh blood at 200×g for 10 min at room temperature and aspirating the supernatant. Platelet-poor plasma was then sedimented by centrifugation of residual blood at 800×g for 10 min at room temperature. Blood platelet aggregation was monitored by platelet turbidity, with 0% aggregation calibrated as the absorbance of platelet-poor plasma and 100% aggregation as the absorbance of PRP. PRP was incubated with the drug at 37°C for 20 min and then stimulated with AA, ADP, thrombin, PAF, and PMA. The aggregation of PRP (preincubated with the tested plant fraction) in response to 10 µM inducer was recorded using an aggregometer (LBY-NJ4).
Western Blotting to Detect Integrin αIIbβ3
Just like 2.7, we got PRP what were split into four groups: blank control group, inducer group, justicidin B induction group, and the last group received aspirin. Different groups were cultured 1.5 h, and the incubator was set to 5% CO2 at 37°C. Then, platelets were washed with PBS twice, harvested, and lysed in RIPA buffer containing protease inhibitors on ice for 60 min. The protein concentration was determined by the BCA method. Next, protein samples (18 μg) were equally loaded onto SDS-PAGE and electrotransferred to PVDF membranes. Subsequently, the membranes were blocked with 5% non-fat milk for 1 h and incubated overnight with primary antibodies. The membranes were washed with TBST buffer three times and then incubated with secondary antibodies for 1 h at 25°C. The membranes were then rinsed three times with blocking solution and visualized by the ECL detection system.
NT. LabelFree Analysis
Since integrin αIIbβ3 is a membrane protein, we used NT. LabelFree to prevent the label from affecting the results. Titration series with ligand concentrations varying between 0 and 1,000 mM were prepared in the Prometheus NT.48 optimized buffer (Figure S1). SD-test (the automatic pre-detection of MST to detect the stability of protein signal) was required before measurement.
Approximately, 3 µl was loaded into NT.LabelFree standard-treated capillaries (Nanotemper). MST experiments were performed at 40% MST (infra-red laser) power and 60% LED power at 25°C using the Monolith NT.LabelFree Instrument (Nanotemper). Ratios between normalized initial fluorescence and after temperature-jump and thermophoresis were calculated and averaged from five to nine independent runs. Means of fluorescence intensity obtained by the MST measurements were fitted, and the resultant Kd values were given together with an error estimation from the fit by the built-in formula of the analysis software.
NT.115 Analysis
The fluorescence of the ligand interfered with the result. This was further exacerbated when using label-free thermophoresis owing to the additional noise present in measuring fluorescence in ranges where inherent fluorescence of the protein itself is measured. After the SD-test experiment verified that the label had little effect on the protein, we used NT.115 for measurement.
All the compounds were analyzed with the concentration gradient of 50 µM with 20 µM of CviR that was labeled Monolith NTTM Protein Labeling Kit RED–NHS (Cat Nr: L001) before instrumental analysis. LED power was 20% and Prometheus NT.48 optimized buffer was used only for the analysis.
Analysis was performed on Monolith Nano Temper (NT)115 and its accessory, i.e., standard-treated 4-µl volume glass capillaries were employed to measure the molecular interaction (Nano Temper Technologies GmbH, Munich, Germany). Means of fluorescence intensity obtained by the MST measurements were fitted, and the resultant Kd values were given together with an error estimation from the fit by the built-in formula of NT1.5.41 analysis software.
Results
Microarray Results
Compared with the control group, hundreds of genes were revealed to be differentially expressed in platelet in EtOAc extract group (Table 1). In EtOAc extract group, PLCB2, PRKCA, GNAQ, MAPK10, MAPK8, MAPK11, MAPK14, GNAI2, PIK3CG, and PIK3R1 were markedly down-regulated in the model group compared with the control group.
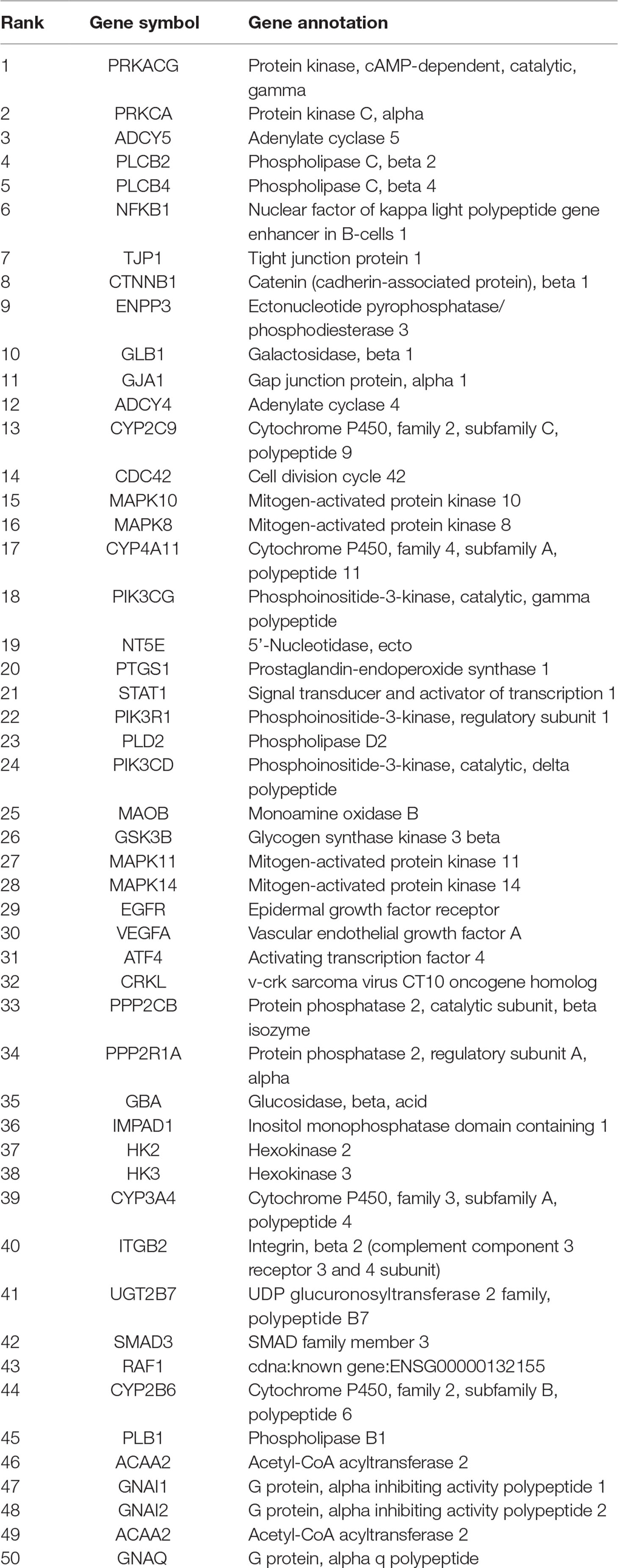
Table 1 The top different 50 genes of platelet expression between the ethyl acetate extract group and blank group.
The Blood Absorptions Compounds of EtOAc Extract
All samples were analyzed by LC-MS according to chromatographic conditions. By comparing the relative retention time and UV absorption spectra of the chromatographic peaks of the test solution and the mixed control solution, the information of the mass spectrometry fragmentation, combined with the preliminary research results and literature of the laboratory, we can confirm that 6’-hydroxy justicidin B, justicidin B, and chinensinaphthol methyl ether are the prototype compounds of the ethyl acetate extract in the blood (Figure 1, Table 2).
Prediction and Analysis of the Target Genes
All potential target genes were synthesized and uploaded to the DAVID database for KEGG pathway annotation and GO enrichment. The threshold was set as P ≤ 0.05, and the pathways or gene functions with higher count were analyzed. The top 10 pathways were graphed by GraphPad Prism 6 (Figure 2).
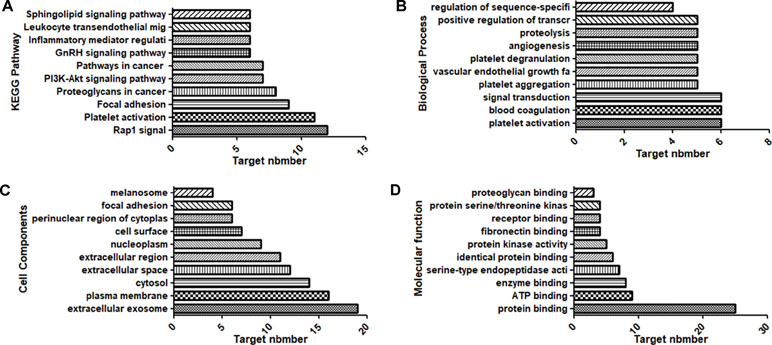
Figure 2 Top 10 components of the KEGG pathway and GO enrichment analyses. (A) KEGG pathways. (B) Biological process. (C) Cell components. (D) Molecular function.
KEGG pathway annotation showed that 30 of the 31 potential target genes were enriched (96.8%) and involved 62 pathways, and 27 of these pathways were significantly correlated with the target genes (P ≤ 0.05). The following pathways had the largest number of genes involved: Platelet activation (11, 35.5%), Rap1 signaling pathway (12, 38.7%), Focal adhesion (9, 29.0%), Proteoglycans in cancer (8, 25.8%), PI3K-Akt signaling pathway (7, 22.6%), and Pathways in cancer (7, 22.6%). GO enrichment analysis showed that the number of genes involved in the CC (Cell Components), MF (Molecular Function), and BP (Biological Process) targets was 31 (100%). CC enrichment was mainly involved in following target genes: extracellular exosome (19, 61.3%), plasma membrane (16, 51.6%), and cytosol (14, 45.2%). MF enrichment was mainly involved in the following target genes: protein binding (25, 80.6%), ATP binding (9, 29.0%), and enzyme binding (8, 25.8%). BP enrichment was mainly involved in the following target genes: platelet aggregation (5, 16.1%), platelet activation (6, 19.4%), and blood coagulation (6, 19.4%).
Construction of the Network
According to the results of gene chip, target genes in the top 10 pathways and compounds were selected to construct an active compound/target gene/pathway network and an active compound/platelet aggregation-related target gene network (Figure 3). The network diagram shows the synergistic effect of various compounds on multiple targets when R. procumbens plays a role in antiplatelet aggregation effects.
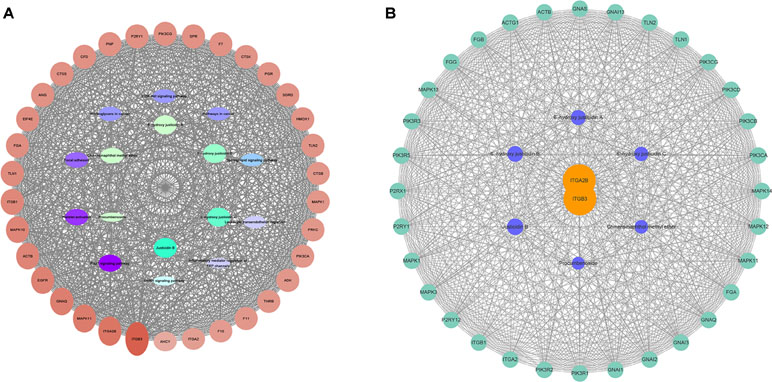
Figure 3 Network diagram constructed by Cytoscape. (A) Network diagram of active components/target genes/enrichment pathways. (B) Network diagram of active components/platelet aggregation-related target genes.
The network analyze tool was used to analyze the network, and genes with higher degree were associated with more genes. It can be considered that the corresponding protein of the genes plays an important role in central correlation when R. procumbens plays an antiplatelet aggregation role. The integrin αIIbβ3 (integrin αIIbβ3 is a protein that in humans is encoded by the ITGA2B and ITGB3 gene) had the highest degree; it was the intersection of all platelet-related pathways.
The results were compared with those of the KEGG pathway analysis and combined with the literature study. Integrin αIIbβ3 was selected as the target for the binding validation experiment.
Screening of Active Compounds
Platelet with four kinds of inducers was stimulated, and platelet aggregation was detected after being incubated by these isolated blood absorption compounds. The platelet aggregation inhibition rate (MIR) was calculated to indicate the inhibitory activity of the drug. Results showed that those justicidin B groups had higher maximum aggregation compared with others. The remaining compounds showed different degrees of antiplatelet aggregation (Table 3, Figure 4).
Integrin αIIbβ3 Expression in PRP
We investigated whether justicidin B had a regulatory effect on integrin αIIbβ3 expression. As shown in Figure 4, the integrin αIIbβ3 level was significantly increased on the inducer group (P < 0.01, versus control group). More remarkably, justicidin B produced a dramatically inhibited effect on integrin αIIbβ3 level (versus inducer group, P < 0.01, Figure 5).
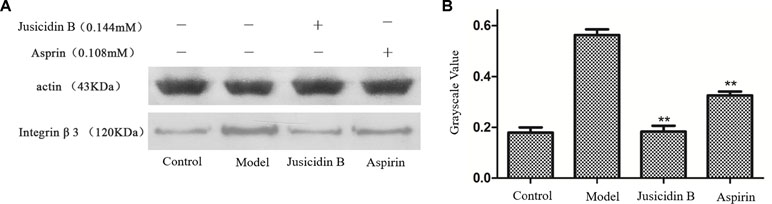
Figure 5 The influence of justicidin B on integrin αIIbβ3 protein. (A) Western blot. (B) Grayscale value.
NT. LabelFree
MST experiments were performed to detect the molecular interaction between inhibitor and integrin αIIbβ3. Owing to the influence of ligand fluorescence, we obtained unsatisfactory results under the SD-test pass condition, accompanied by large errors (Figure 6).
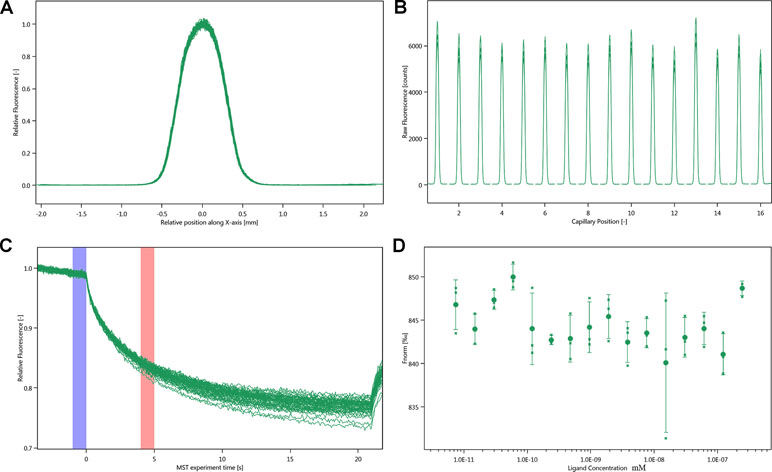
Figure 6 Molecular interaction of integrin αIIbβ3 using NT. LabelFree analysis. (A) SD-TEST capillary shap. (B) SD-TEST capillary scan. (C) MST time traces of 16 different justicin B concentrations (ranging from 0.00748 to 245 µM). (D) Dependence of the MST signal on the justicin B concentration (measured 30 s after turning on heating; data from C).
NT.115
The SD-test verified that the label had less effect on the protein. We used NT.115 for the experiment. As differences in normalized fluorescence of the bound and unbound state allow determination of the fraction bound, the dissociation constant was thus calculated. All values were multiplied by a factor of 1,000, which yielded the relative fluorescence change in per thousand. The Kd values of justicin B were 35.017 ± 5.9014 μm (Figure 7).
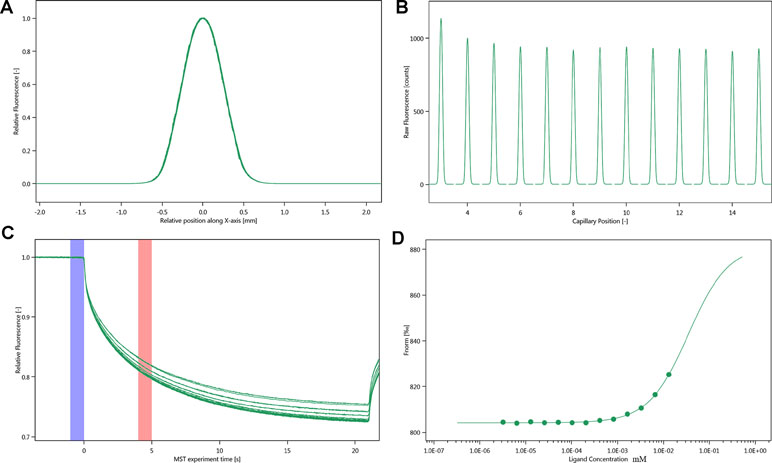
Figure 7 Molecular interaction of integrin αIIbβ3 using NT. 115 analysis. (A) SD-TEST capillary shap. (B) SD-TEST capillary scan. (C) MST time traces of 16 different justicin B concentrations (ranging from 0.0032 to 13.1 mM). (D) Dependence of the MST signal on the justicin B concentration (measured 30 s after turning on heating; data from C).
Discussion
R. procumbens has been used in herbal medicines for promoting blood circulation and pain relief. Modern pharmacological studies have shown that R. procumbens has a good antiplatelet aggregation effect (Chen et al., 1996). According to preliminary experiments, ethyl acetate extract is the active fraction. However, the chemical composition of this plant material is quite complex. Therefore, it was difficult to determine the active ingredient and target protein by using traditional methods.
The animal experiment was utilized for further verification. Results of gene chip illustrated that ethyl acetate extract could inhibit Gq–PLC–PKC pathway and Gi–PI3K–MAPK pathway. The down-regulation of GNAI1 gene related to the regulation of AC kinase was also observed, indicating that the ethyl acetate site can reduce inhibitory effect of Gi and enhance the activity of AC kinase. This result indicated that the ethyl acetate site could regulate the GI–AC–CAMP signaling pathway. Above all, ethyl acetate might inhibit platelet aggregation by inhibiting Gq–PLC–PKC, Gi–PI3K–MAPK, and other signaling pathways.
In order to further explore the material basis of R. procumbens, the blood absorption compounds of R. procumbens were screened by serum pharmacological method. Based on the results of the gene chip and LC-MS, GO enrichment analysis found that the targets involved extracellular exosome, plasma membrane, extracellular space, cytosol, and other cell compartments. At the molecular level, the targets were involved in protein binding, enzyme binding, ATP binding, and other molecular activities, and they were related to platelet aggregation, platelet activation, and platelet degranulation. It indicated that the drug may inhibit platelet aggregation by binding to membrane proteins, affecting its energy utilization and activation. The pathway enrichment results also indicated that R. procumbens may play an antiplatelet aggregation role by inhibiting the key targets of the platelet activation signaling pathway, such as integrin αIIbβ3. It was the intersection of all platelet-related pathways. Proteoglycans in cancer, PI3K–Akt signaling pathway, and so on also suggest that justicidin B may affect the expression and activity of cancer-related proteins by binding integrin αIIbβ3.
Then, we use optical turbidimetry to measure the activity of three isolated blood absorption compounds. We found that justicidin B is the most active compound. Then, WB experiment verified this fact. It is well known that membrane proteins are less stable, and this has shown to have a massive impact on the MST (NT.LabelFree) experiment (Chasis and Mohandas, 1986). To identify optimal test and storage conditions for the membrane protein integrin αIIbβ3, the protein was subjected to a thermal unfolding formulation screen of the Prometheus NT.48 (supplementary material). NanoTemper’s on-the-fly technology allows to measure 48 samples in parallel, providing more than 10 data points per minute. Formulation developments benefit from ultra-high resolution that are not compromised by aggregation but, at the same time, offer ease of use. Prometheus NT.48 results suggest that Hepes is the best detergent. The onset for ratio of Hepes-DDM is 46.1°C, which is higher than other buffers. This attempt was not reported before.
MST is a powerful technique to measure biomolecular interaction that are based on thermophoresis—the movement of molecules in a temperature gradient. This technique was reported to be highly sensitive such that it allows precise quantification of molecular interaction (Jerabek-Willemsen et al., 2014). Because of the influence of ligand fluorescence, we obtained unsatisfactory results, accompanied by relatively large errors. The results of the Prometheus NT.48 experiment showed that the protein stability was relatively good, and the labeled protein SD-test was qualified; hence, we experimented further with NT.115 and obtained better results. MST results suggest that the selected compound has potential molecular interaction with integrin αIIbβ3. The Kd value of justicin B was 35.017 ± 5.9014 μm. These data suggest that justicin B has a good interaction with integrin αIIbβ3. According to Seidel et al. (2013), the fitting curve may be either S-shaped or mirror S-shaped. The standard symbol of MST amplitude (change in normalized fluorescence) depends on the chemistry of the compound that is titrated, its binding site, and the conformational change induced upon binding. Justicin B shows a positive slope suggesting a strong conformational change induced upon complex formation. Probably its interaction plays a major role in conformational change. We speculate that it might interfere with the platelet aggregation mechanism by negatively influencing the conformational changes required for the integrin αIIbβ3 activation.
Taken together, our results demonstrate that the ethyl acetate extract plays an anti-platelet aggregation role through integrin αIIbβ3, and justicin B, which is the most active blood absorption compound, targets it. We believe that our findings would provide a better foundation for further understanding of the mechanism of R. procumbens intervention in platelet aggregation.
Ethics Statement
This study was carried out in accordance with the recommendations of Animal Care and Use Committee of Institute of Materia Medica, China.
Author Contributions
H-ZW conceived the study. H-ZW and Y-FY designed the study. S-TW performed the experiments and the data analysis, and wrote the manuscript. Y-FY, W-CX, BL, P-FH, W-PX, YS, Z-ZA, P-TY, and Z-TX revised the manuscript. All the authors read and approved the final version of the manuscript.
Funding
This research was funded by the National Natural Science Foundation of China, grant number 31570343.
Conflict of Interest Statement
The authors declare that the research was conducted in the absence of any commercial or financial relationships that could be construed as a potential conflict of interest.
Supplementary Material
The Supplementary Material for this article can be found online at: https://www.frontiersin.org/articles/10.3389/fphar.2019.00688/full#supplementary-material
Figure S1 | Thermal unfolding curves. (A) Thermal unfolding curves in presence of Hepes and pbs. (B) Thermal unfolding curves in presence of Ttis. Insets show the detergent dependence of the first unfolding transition midpoint (Tm1).
References
Andrews, R. K., Gardiner, E. E. (2017). Basic mechanisms of platelet receptor shedding. Platelets 28, 319–324. doi: 10.1080/09537104.2016.1235690
Batoulis, H., Schmidt, T. H., Weber, P., Schloetel, J.-G., Kandt, C., Lang, T. (2016). Concentration dependent ion-protein interaction patterns underlying protein oligomerization behaviours. Sci. Rep. 6, 24131. doi: 10.1038/srep24131
Bender, M., Stegner, D., Nieswandt, B. (2017). Model systems for platelet receptor shedding. Platelets 28, 325–332. doi: 10.1080/09537104.2016.1195491
Chasis, J. A., Mohandas, N. (1986). Erythrocyte membrane deformability and stability: two distinct membrane properties that are independently regulated by skeletal protein associations. J. Cell. Biol. 103, 343–350. doi: 10.1083/jcb.103.2.343
Chatterjee, M., Gawaz, M. (2017). Clinical significance of receptor shedding-platelet GPVI as an emerging diagnostic and therapeutic tool. Platelets. 28, 362–371. doi: 10.1080/09537104.2016.1227062
Chen, Q.-J. (2012). The preliminary study of anti-chronic nephritis from Rostellularia procumbens (L.) Nees. [dissertation]. Hubei: Hubei University of Chinese Medicine.
Chen, C.-C., Lin, C.-F., Huang, Y.-L., Ko, F.-N., Teng, C.-M. (1995). Bioactive constituents from the flower buds and peduncles of Lindera megaphylla. J. Natural. Prod. 58, 1423–1425. doi: 10.1021/np50123a013
Chen, C.-C., Hsin, W.-C., Ko, F.-N., Huang, Y.-L., Ou, J.-C., Teng, C.-M. (1996). Antiplatelet arylnaphthalide lignans from Justicia procumbens. J. Natural. Prod. 59, 1149–1150. doi: 10.1021/np960443+
Coller, B. S., Shattil, S. J. (2008). The GPIIb/IIIa (integrin αIIbβ3) odyssey: a technology-driven saga of a receptor with twists, turns, and even a bend. Blood 112, 3011–3025. doi: 10.1182/blood-2008-06-077891
Committee FoCE (2011). Flora of China (Curcurbitaceae through Valerianaceae with Annonaceae and Berberidaceae) vol. 19. Beijing & St Louis: Fl China Science Press & Missouri Botanical Garden Press, 1–884.
Corrêa, G. M., Alcântara, AFdC. (2012). Chemical constituents and biological activities of species of Justicia: a review. Rev. Bras. Farmacogn. 22, 220–238. doi: 10.1590/S0102-695X2011005000196
Jerabek-Willemsen, M., André, T., Wanner, R., Roth, H. M., Duhr, S., Baaske, P., et al. (2014). MicroScale thermophoresis: interaction analysis and beyond. J. Mol. Struc. 1077, 101–113. doi: 10.1016/j.molstruc.2014.03.009
Joshi, A. R., Joshi, K. (2000). Indigenous knowledge and uses of medicinal plants by local communities of the Kali Gandaki Watershed Area, Nepal. J. Ethnopharmacol. 73, 175–183. doi: 10.1016/S0378-8741(00)00301-9
Savithramma, N., Sulochana, C., Rao, K. (2007). Ethnobotanical survey of plants used to treat asthma in Andhra Pradesh, India. J. Ethnopharmacol. 113, 54–61. doi: 10.1016/j.jep.2007.04.004
Seidel, S. A., Dijkman, P. M., Lea, W. A., van den Bogaart, G., Jerabek-Willemsen, M., Lazic, A., et al. (2013). Microscale thermophoresis quantifies biomolecular interactions under previously challenging conditions. Methods. 59, 301–315. doi: 10.1016/j.ymeth.2012.12.005
Sparks, R. P., Fratti, R. (2019). “Use of microscale thermophoresis (MST) to measure binding affinities of components of the fusion machinery,” in: SNAREs. Springer, 191–198. doi: 10.1007/978-1-4939-8760-3_11
Su, W.-Y. (2014). Study on chemical constituents of Rostellularia procumbens (L.) Nees. [dissertation]. Hubei: Hubei University of Chinese Medicine.
van den Bogaart, G., Meyenberg, K., Diederichsen, U., Jahn, R. (2012). Phosphatidylinositol 4, 5-bisphosphate increases Ca2+ affinity of synaptotagmin-1 by 40-fold. J. Biol. Chem. 287, 16447–16453. doi: 10.1074/jbc.M112.343418
Vinothkannan Ravichandran, L. Z., Wang, H., Yu, G., Zhang, Y., Li, A. (2018). Virtual screening and biomolecular interactions of CviR-based quorum sensing inhibitors against Chromobacterium violaceum. Front. Cell. Inf. Microbiol 8, 1–13. doi: 10.3389/fcimb.2018.00292
Wu, H.-Z., Zhang, Y.-Q., Zhang, B.-W., Yan, B., Yang, Y.-F. (2013). Study on chemical constituents of Rostellularia procumbens (L.) Nees. Hubei. J. Trad. Chin. Med. 35, 68–69.
Keywords: justicidin B, integrin αIIbβ3, platelet aggregation, gene chip, LC-MS, network pharmacology, Prometheus NT.48, microscale thermophoresis
Citation: Yang Y-F, Wu S-T, Liu B, Xie Z-T, Xiong W-C, Hao P-F, Xiao W-P, Sun Y, Ai Z-Z, You P-T and Wu H-Z (2019) A Novel Antiplatelet Aggregation Target of Justicidin B Obtained From Rostellularia Procumbens (L.) Nees. Front. Pharmacol. 10:688. doi: 10.3389/fphar.2019.00688
Received: 22 January 2019; Accepted: 27 May 2019;
Published: 14 June 2019.
Edited by:
Yuanjia Hu, University of Macau, ChinaReviewed by:
Jiachun Chen, Huazhong University of Science and Technology, ChinaQun Wei, Beijing Normal University, China
Zhou Benhong, Wuhan University, China
Copyright © 2019 Yang, Wu, Liu, Xie, Xiong, Hao, Xiao, Sun, Ai, You and Wu. This is an open-access article distributed under the terms of the Creative Commons Attribution License (CC BY). The use, distribution or reproduction in other forums is permitted, provided the original author(s) and the copyright owner(s) are credited and that the original publication in this journal is cited, in accordance with accepted academic practice. No use, distribution or reproduction is permitted which does not comply with these terms.
*Correspondence: Song-Tao Wu, c29uZ3Qxd3VAMTYzLmNvbQ==; He-Zhen Wu, aGV6aF93dUAxNjMuY29t
†These authors have contributed equally to this work.