- 1State Key Laboratory of Natural Medicines, School of Basic Medical Sciences and Clinical Pharmacy, China Pharmaceutical University, Nanjing, China
- 2Department of Pediatrics, Nanjing Integrated Traditional Chinese and Western Medicine Hospital, Nanjing, China
Ma Xing Shi Gan Decoction (MXD), a classical traditional Chinese medicine prescription, is widely used for the treatment of upper respiratory tract infection. However, the effect of MXD against particulate matters with diameter of less than 2.5 μm (PM2.5) induced lung injury remains to be elucidated. In this study, rats were stimulated with PM2.5 to induce lung injury. MXD was given orally once daily for five days. Lung tissues were harvested to assess pathological changes and edema. Myeloperoxidase (MPO) activity and malonaldehyde (MDA) content in lung were determined to evaluate the degree of injury. To assess the barrier disruption, the bronchoalveolar lavage fluid (BALF) was collected to determine the total protein content and count the number of neutrophils and macrophages. For evaluating the activation of macrophage in lung tissue, CD68 was detected using immunohistochemistry (IHC). The levels of inflammatory factors including tumor necrosis factor-alpha (TNF-α), interleukin-1beta (IL-1β), and interleukin-6 (IL-6) in BALF and serum were measured. In vitro, a PM2.5-activated RAW 264.7 macrophages inflammatory model was introduced. To evaluate the protective effect of MXD-medicated serum, the cell viability and the release of inflammatory factors were measured. The effects of MXD on the High mobility group box-1/Toll-like receptor 4/Nuclear factor-kappa B (HMGB1/TLR4/NFκB) pathway in lung tissue and RAW 264.7 cells were assessed by Western blot. For further confirming the protective effect of MXD was mediated by inhibiting the HMGB1/TLR4/NFκB pathway, RAW 264.7 cells were incubated with MXD-medicated serum alone or MXD-medicated serum plus recombinant HMGB1 (rHMGB1). MXD significantly ameliorated the lung injury in rats, as evidenced by decreases in the pathological score, lung edema, MPO activity, MDA content, CD68 positive macrophages number, disruption of alveolar capillary barrier and the levels of inflammatory factors. In vitro, MXD-medicated serum increased cell viability and inhibited the release of inflammatory cytokines. Furthermore, MXD treatment was found to inhibit HMGB1/TLR4/NFκB signal pathway both in vivo and in vitro. Moreover, the protection of MXD could be reversed by rHMGB1 in RAW 264.7. Taken together, these results suggest MXD protects rats from PM2.5 induced acute lung injury, possibly through the modulation of HMGB1/TLR4/NFκB pathway and inflammatory responses.
Introduction
Exposure to respirable urban air particles poses a serious threat to human health across the world (Englert, 2004; Brook et al., 2010; Schneider et al., 2010; Zheng et al., 2015; McGuinn et al., 2019). The toxic effects of particulate matters (PMs) are mainly related to their particle size, and particulate matters with diameter of less than 2.5 µm (PM2.5) can penetrate deeper into the respiratory tract and about 50% of them are retained in the lung parenchyma, causing respiratory infection, cardiovascular disease, and systemic inflammation (Clifford et al., 2018; Nhung et al., 2018; Pope et al., 2018).
Alveolar macrophage, the main phagocyte in lung tissue protects lung tissue against microorganism or particles via phagocytosis (Lukacs et al., 1995). However, when high particulate burdens are deposited and accumulated in the lung, alveolar macrophages will be over-activated and release excessive amounts of inflammatory factors which plays a key factor during the occurrence and development of lung injury (Asimakopoulos et al., 1999; Jacobson et al., 2005; Kallapur and Jobe, 2006; Herold et al., 2011).
Ma Xing Shi Gan Decoction (MXD), a traditional Chinese medicine formula, which was recorded in Treatise on Exogenous Febrile Disease in Eastern Han dynasty has many kinds of pharmacological action including but not limited to anti-asthmatic effect, antiviral effect, and antibacterial effect (Lu et al., 2012; Wang et al., 2012). Currently, MXD is widely used in clinic for treatment of acute upper respiratory tract infection in China (Liao et al., 2017). However, it remains unknown whether MXD has protective effects against PM2.5 induced acute lung injury.
The present study was designed to investigate the protective effect of MXD on PM2.5 induced acute lung injury and to explore the underlying mechanism.
Materials And Methods
Reagents
All crude drugs were provided by the pharmacy of Nanjing Integrated Traditional Chinese and Western Medicine Hospital. Ephedrae herba (ma huang) collected from the province of Inner Mongolia, China, was purchased from Sanyue Traditional Chinese Medicine Co., Ltd (Nantong China). Semen armeniacae amarum (ku xing ren) collected from the province of Hebei, China, was purchased from Jiangsu Huahong Pharmaceutical Technology Co., Ltd (Danyang, China). Glycyrrhizae radix preparata (gan cao; licorice) collected from the province of Gansu, China, was purchased from Hangzhou Zhende Traditional Chinese Medicine Co., Ltd (Hangzhou, China). Gypsum fibrosum (shi gao; calcium sulfate) collected from the province of Shandong, China, was purchased from Jiangsu Huahong Pharmaceutical Technology Co., Ltd (Danyang, China). The quality of crude drugs is consistent with rule of related part of Chinese Pharmacopoeia (2015 edition). Botanical identifications were authenticated by an associate professor Yong Wang (Nanjing Integrated Traditional Chinese and Western Medicine Hospital, China). Voucher specimens of Ephedrae herba (voucher number: No.EH181201), Semen armeniacae amarum (voucher number: No.SAA190410), Glycyrrhizae radix preparata (voucher number: No.GRP181202), and Gypsum fibrosum (voucher number: No.GF181202) have been deposited in the Herbarium of China Pharmaceutical University and registered under the voucher specimen numbers. Standards of Ephedrine hydrochloride (CAS No. 50-98-6, purity 100.0%) and pseudoephedrine hydrochloride (CAS No. 345-78-8, purity 99.8%) were purchased from National Institutes for Food and Drug Control. Standards of Liquiritin (CAS No. 551-15-5, purity ≥98%), Glycyrrhizic acid ammonium salt (CAS No. 53956-04-0, purity ≥98%) and Amygdalin (CAS No. 29883-15-6, purity ≥98%) were purchased from Solarbio Science & Technology Co., Ltd., Beijing, China. PM2.5 was provided by Nanjing Municipal Environmental Monitoring Centre. Rat interleukin-1beta (IL-1β), Interleukin-6 (IL-6) and tumor necrosis factor-alpha (TNF-α) enzyme linked immunosorbent assay (ELISA) kits were obtained from Shanghai MLBIO Biotechnology Co., Ltd., Shanghai, China. The myeloperoxidase (MPO) and malondialdehyde (MDA) assay kits were obtained from Nanjing Jiancheng Bioengineering Institute Nanjing, China. Antibodies to High mobility group box 1 (HMGB1), Toll-like receptor 4 (TLR4), Myeloid differentiation factor 88 (MyD88), and phosphorylated p65 (p-p65) were all purchased from Wanleibio Co., Ltd., Shenyang, China. Antibody to β-actin was purchased from Abways Biotechnology Co., Ltd., Shanghai, China. Antibody to CD68 was purchased from Servicebio Co., Ltd., Wuhan, China. Recombinant HMGB1 (rHMGB1) was purchased from Beyotime Institute of Biotechnology, Shanghai, China. All other reagents were of analytical grade and commercially available.
Preparation of PM2.5
PM2.5 collecting filters were kindly provided by Jiangsu Environmental Monitoring Center. The atmospheric particulates were collected from 2018.1 to 2018.5 in Nanjing, China. After extraction, a total of 6.76 g PM2.5 was collected. Then the PM2.5 was fully dispersed by ultrasonic washer (40 min, 3 times). The liquid was filtered by 8-fold gauze, and the supernatant was discarded after centrifugation. 2.25 g PM2.5 was obtained by drying. After an ultraviolet sterilization for 40 min, the concentration of PM2.5 suspension was adjusted to 10 mg/ml with deionized water. Before use, the suspension was fully suspended.
Animals and Experimental Design
Male Sprague-Dawley (SD) rats of SPF level (180–200 g) were purchased from Qinglongshan Animal Farm of Nanjing, China. Animals were housed under a normal 12/12 h light/dark schedule and housed at 24±2 °C with relative humidity (55 ± 5%). Standard chow and water were supplied ad libitum.
Rats were divided into six groups: control group, intervention group (MXD, 16.4 g/kg), PM2.5 group (model group), and PM2.5+MXD (4.1, 8.2, and 16.4 g/kg) groups. The administration dosage was calculated according to the content of crude herbs. The middle dose (8.2 g/kg) was 6.3 times of the clinical dosage in human, which equivalent dose calculation is based on body surface area (Nair and Jacob, 2016). One hour after the establishment of acute lung injury, drugs were administrated orally (1 ml/100 g body weight) once daily for 5 consecutive days. Correspondingly, rats in the control group and the model group were treated with normal saline.
All procedures for animal care and use were in accordance with the National Institute of Health (NIH) guidelines for the Care and Use of Laboratory Animals, and approved by the Institutional Animal Care and Use Committee of China Pharmaceutical University [license number: SYXK (Su) 2016-0011]. The numbers of animals for different experimental groups were listed in Supplementary Table 1 as follows:
Preparation of MXD
Ephedrae herba (ma huang, 4 g) were added into 1 L of double distilled water and decocted for 60 min. After skimming the scum, Semen armeniacae amarum (ku xing ren, 12 g), Glycyrrhizae radix preparata (gan cao, 8 g), and Gypsum fibrosum (shi gao, 24 g) were added and extracted by refluxing with water for another 40 min. Then the crude extract was filtered and the concentration of extract was enriched to 2 g/ml by evaporating (calculated according to the content of crude drugs).
Quantitative Analysis of MXD By UPLC-MS (Ultra-Performance Liquid Chromatography-Tandem Mass Spectrometry)
Preparation of test solution: MXD was diluted 10,000 times using 50% (v/v) methanol in water. The test solution was obtained after filtration.
Preparation of reference standard solution: The reference standard samples (ephedrine hydrochloride, pseudoephedrine hydrochloride, liquiritin, glycyrrhizic acid ammonium salt, and amygdalin) were weighed accurately and dissolved in 50% (v/v) methanol in water at a concentration of 1 mg/ml. After diluted and pooled, the reference standard solution containing ephedrine hydrochloride (500 ng/ml), pseudoephedrine hydrochloride (500 ng/ml), liquiritin (500 ng/ml), glycyrrhizic acid ammonium salt (500 ng/ml), and amygdalin (500 ng/ml) was obtained.
The preliminary analysis was performed using an Acquity UPLC CSH C18 (2.1×100 mm, 1.7 µm) column in both positive and negative electrospray ionization mode. For positive electrospray ionization mode, mobile phase solvent A was consisted of aqueous phase of ammonium acetate at 5 mmol/L, and mobile phase solvent B was pure methanol. For negative electrospray ionization mode, mobile phase solvent A was ultrapure water, and mobile phase solvent B was methanol. The injection volume was 1 µl. The common elution condition is shown in Supplementary Table 2.
The five major components of MXD (ephedrine hydrochloride, pseudoephedrine hydrochloride, liquiritin, glycyrrhizic acid ammonium salt, and amygdalin) were quantified through a UPLC (Acquity UPLC system, Waters, USA)-tandem MS (Xevo TQ-XS mass spectrometer, Waters).
For the analysis of ephedrine and pseudoephedrine in MXD, The UPLC separation was performed at 40 °C using a SHIMADZU VP-ODS (2.0×150 mm) column. Mobile phase solvent A was 0.1% (v/v) formic acid in water, and mobile phase solvent B was acetonitrile. The injection volume was 1 µl. The elution conditions are shown in Supplementary Table 3. Positive ionization electrospray MS was employed for the quantification, and the optimal instrument conditions were as follows: cone (V): 30, collision (eV): 12. The precursor-product ion combination of m/z 166.00→148.00 was common quantifier ion for ephedrine and pseudoephedrine. The external standard method is employed for quantitative analysis.
For the analysis of liquiritin and glycyrrhizic acid in MXD, the UPLC separation was performed at 50 °C using an Acquity UPLC HSS T3 (2.1×30 mm, 1.8 µm) column. Mobile phase solvent A was consisted of aqueous phase of ammonium acetate in concentration 5 mmol/L, and mobile phase solvent B was pure methanol. The injection volume was 1 µl. The elution conditions are shown in Supplementary Table 4. Positive ionization electrospray MS was employed for the quantification, and the optimal instrument conditions were as follows: cone (V): 42, collision (eV): 8. The precursor–product ion combinations of m/z 419.00→257.00 and 823.41→453.37 were quantifier ion for liquiritin and glycyrrhizic acid. The external standard method is used for quantitative analysis.
For the analysis of amygdalin in MXD, The UPLC separation was performed at 50 °C using an Acquity UPLC HSS T3 (2.1×30 mm, 1.8 µm) column. Mobile phase solvent A was 0.1% (v/v) formic acid in water, and mobile phase solvent B was acetonitrile. The injection volume was 1 µl. The elution conditions are shown in Supplementary Table 5. Negative ionization electrospray MS was employed for the quantification, and the optimal instrument conditions were as follows: cone (V): 30, collision (eV): 12. The precursor–product ion combination of m/z 502.00→456.00 was quantifier ion for amygdalin. The external standard method is employed for quantitative analysis.
Establishment Of Acute Injury
After the grouping, rats were maintained anesthetized with 3% isoflurane in O2 for 0.5 h by a respirator mask and fixed in the supine position at a 45-degree angle to horizontal plane. The model of PM2.5 induced acute lung injury was established as previously described with slight modification (Liu et al., 2018). Briefly, a number 16 trocar was inserted into the rat’s trachea, after which the PM2.5 suspension was injected into the trachea through the trocar at a volume of 0.1 ml/100 g body weight. The rats were gently rolled from side to side for 30 s to distribute suspension equably in both lungs. This operation was done at the first, third, fifth, seventh, and ninth day. One hour after the last instillation of PM2.5 suspension, MXD was administered as described in Animals and Experimental Design section.
Histological Analysis
Rats were sacrificed 2 h after the last administration, the left lungs were fixed in 4% formaldehyde (pH = 7.4) overnight and then embedded in paraffin and sliced into 5 µm-thick sections. The histopathological abnormalities of lung tissue were observed under a light microscope after staining with H&E. The pathological changes of the lungs were scored as previously described (Liu et al., 2014; Bao et al., 2015; Lv et al., 2015). The score was graded according to the sum of the score for degree of damage such as the thickening of alveolar walls, the number of infiltration cells and hemorrhage. Each histological characteristic was scored 0 to 5.
Assessment of Lung Tissue Edema
Two hours after the last administration, rats were sacrificed and lungs were excised and placed on pre-weighed aluminum foil papers. After obtaining wet weight of lungs by weighing, lungs were dried at 90 °C for 48 h in an oven to obtain the dry weight. The water content of the lungs was calculated according to the formula below:
Pulmonary MPO Activity and MDA Content Assay
Five percent homogenate was obtained by homogenizing 0.1 g of right lung tissues in normal saline. The rest right lungs were stored at −80 °C for the following Western blot. MPO which reflects activation of neutrophils and macrophages in lungs was determined using a test kit (Nanjing Jiancheng Bioengineering Institute) according to the instructions (Grattendick et al., 2002; Zhang et al., 2011; Tang et al., 2014). MDA content in lung tissues were detected by an assay kit (Nanjing Jiancheng Bioengineering Institute).
IHC Assay
Immumohistochemical assay was performed to study the effects of MXD on the expression of CD68, a valuable marker of monocyte/macrophages in the histochemical analysis of inflamed tissues. After the sections (5 µm in thickness) were deparaffinized with xylene and rehydrated with graded ethanol, antigen retrieval was performed in an autoclave (10 min, 121 °C), followed by incubation in 3% H2O2 at room temperature for 30 min to block endogenous peroxidase activity. After washed with phosphatic buffer solution (PBS), the sections were incubated with goat serum for 30 min at 37 °C, and subsequently incubated with the primary antibody against CD68 (1: 500) for 15 h at 4 °C. Then, the sections were washed with PBS and stained with peroxidase-labeled secondary antibody at 37 °C for 1 h. Slides were then processed using 3, 3-diaminobenzidine chromogenic solution and counter stained with hematoxylin. The pictures were observed using a light microscope (Olympus, Japan, BX53).
Cell Counting and Protein Concentration in Bronchoalveolar Lavage Fluid (BALF)
Rats were anesthetized 2 h after the last administration. Three milliliters of blood were collected from the abdominal aorta, the serum was then separated at 3,000 g for 15 min and stored at −80 °C for further ELISA tests. The right hilum of the lung was clamped and sutured (to exclude from the lavage) with a 2-0 silk suture thread, after which the right lungs were dissected and stored at −80 °C for MPO assay, MDA assay and Western blot. The tracheae were then cannulated, and the left lungs were lavaged three times with ice-cold PBS (0.5 ml each time) for BALF collection. The mean volume retrieved was 80% of the amount instilled (1.2 ml). The BALF samples were centrifuged at 1,000 g for 5 min. The supernatant was collected and the protein level was measured by a bicinchoninic acid (BCA) assay kit (Jiancheng Bioengineering Institute, Nanjing, China). The cell pellet was resuspended in 100 µl PBS and the number of total cells was counted using a hemocytometer (Qiujing, Shanghai, China). Then cells were centrifuged onto slides and stained with Wright-Giemsa (Wanleibio, Shenyang, China, WLA043a) for 8 min. Differential cell counts were quantified using light microscope by counting a total of 200 cells/slide at 40× magnification. Number of each cell type was calculated as the percentage of cell type multiplied by the total number of cells in the BALF.
Cytokine Assays in BALF And Serum
The concentrations of TNF-α, IL-6, and IL-1β in the BALF supernatant and serum were measured by ELISA kits (Shanghai MLBIO Biotechnology Co., Ltd., Shanghai, China) according to the instructions. Briefly, specific monoclonal antibody was coated on a 96-well plate. Standards and samples were added to the wells. Next, a biotinylated detection antibody was added, followed by avidin-horseradish peroxidase. 3,3′,5,5′-tetramethylbenzidine (TMB) substrate was subsequently added, producing a blue color. Finally, the reaction system color was changed from blue to yellow by adding the stop solution. Then the absorbance value was measured at 450 nm using a microplate reader (Infinite M200 Pro, Tecan).
Cell Culture
RAW 264.7 cells, a macrophage cell line which has been widely used as an in vitro inflammatory model, was kindly provided by Prof. Zhong-lin Yang (China Pharmaceutical University), were cultured at 37 °C in a humidified atmosphere of 5% CO2/95% air and maintained in DMEM (Kaiji, Nanjing, China) with 10% heat inactivated fetal bovine serum (Gibco Laboratories, USA) supplemented with 100 U/100 ml penicillin/streptomycin (Solarbio, Beijing, China). Cells within the logarithmic growth phase were seeded in 96-well plates at a concentration of 1×104 cells and incubated for 24 h to allow for confluency.
Preparation of MXD-Medicated Serum
As MXD contains a variety of complex components, including but not limited to tannin and inorganic salts, the experimental results will be interfered if we add MXD into the culture system directly. Besides, although filtered to remove dregs, MXD still contained small particulate matters, which may activate macrophage and interfere with the efficacy of MXD (Wright et al., 1985; Claudio et al., 1995; Voronov et al., 1998; Islam et al., 2011; Higashisaka et al., 2014). Hence, serum pharmacological method was introduced to investigate the effect of MXD on the PM2.5 stimulated inflammatory model in RAW 264.7.
Twenty rats were randomly divided into control group and MXD-medicated group. Rats in the control group were given 1 ml/100 g of saline; rats in the MXD-medicated group were orally administered MXD at the dose of 16.4 g/kg once daily for 5 consecutive days. Blood was collected aseptically via the abdominal aorta 2 h after the last administration and then centrifuged to obtain serum. The serum from the same group were pooled, filtered through 0.22 µm filters, inactivated at 56 °C for 30 min, split, and stored at −70 °C for future use.
Cell Viability Assay
Cell viability was evaluated with 3-(4,5-Dimethylthiazol-2-yl)-2,5-diphenyltetrazolium bromide (MTT) assay for determining the optimal concentration of PM2.5. Briefly, RAW 264.7 cells (1×104 cells/well) were seeded in 96-well plates. After incubated for 24 h, cells were treated with PM2.5 (1.25, 2.5, 5, 10 and 20 µg/cm2) for 24 h. Normally cultured RAW 264.7 cells were used as blank control. Then 20 µl MTT (5 mg/ml) was added to each well for an additional 4 h. The supernatant was removed and the sediment of formazan was dissolved in 200 µl DMSO and shook for 5 min, after which the absorbance value was measured at 570 nm using a microplate reader. The concentration of PM2.5 which was able to decrease the cell viability to about 50% selected for the following experiment.
The optimal concentration (volume fraction expressed as a percentage) of MXD-medicated serum was determined with MTT assay. RAW 264.7 cells (1×104 cells/well) were seeded in 96-well plates and incubated for 24 h. Then cells were incubated with various concentrations of MXD-medicated serum (5% MXD-medicated serum+15% non-immune serum, 10% MXD-medicated serum+10% non-immune serum, 15% MXD-medicated serum+5% non-immune serum, and 20% MXD-medicated serum) for 24 h. Generally, the volume fraction of serum in the culture system doesn’t exceed 20%. Hence, in this experiment, the volume fraction of serum was restricted at 20% for uniformity. Cells in control group were incubated with 20% non-immune serum.
The protective effect of MXD-medicated serum on PM2.5 induced injury in RAW 264.7 cells was assessed using MTT method. After seeded in 96-well plates and incubated for 24 h, cells were divided into control group, PM2.5 group (model group), PM2.5+MXD-medicated serum (5, 10, and 20%) groups. Cells in control group and PM2.5 group were incubated with 20% non-immune serum for 24 h. PM2.5 was added simultaneously after the addition of serum. After 24 h, cell viability was determined as described above.
Cytokine Assays in Medium
Twenty-four hours after the incubation, the medium was collected and centrifuged at 3,000 g for 10 min to obtain the supernatant. The concentrations of TNF-α, IL-1β, and IL-6 in the culture supernatant were measured by ELISA kits as described above.
Western Blot Analysis
Isolated lung tissue cubes and RAW 264.7 cells were lysed by radio immunoprecipitation assay (RIPA) lysis buffer containing phenylmethylsulfonyl fluoride (PMSF) (1 mM). The protein concentration was measured by the BCA kit. Equal amount of proteins was separated through 12% SDS polyacrylamide gels and transferred onto polyvinylidene fluoride (PVDF) membranes. The membranes were blocked with 5% nonfat milk at room temperature for 1 h then washed three times and incubated with the primary antibodies (anti-HMGB1, 1:1,000; anti-TLR4, 1:1,000; anti-MyD88, 1:1,000; anti-p-p65, 1:1,000; anti-β-actin, 1:1,000) at 4 °C overnight. The next day, the membranes were washed 3 times for 5 min with TBST and then incubated with the Horseradish peroxidase conjugated secondary antibodies for 2 h at room temperature. Finally, the membranes were washed 3 times for 5 min again. Protein bands were detected by an enhanced chemiluminescence (ECL) detection kit (Wanleibio Co., Ltd., Shenyang, China). The ImageJ software Version 1.41 was used for the quantitative densitometric analysis of the protein bands.
Verification Experiment Using rHMGB1
For further confirming the protective effect of MXD was at least partially mediated by inhibiting the HMGB1/TLR4/NFκB pathway, a verification experiment using rHMGB1 was conducted. Briefly, cells were divided into four groups: (1) Control group, cells were incubated with 20% nonimmune serum; (2) PM2.5 group, cells stimulated with PM2.5 was incubated with 20% nonimmune serum; (3) Treatment group, cells stimulated with PM2.5 was treated with 20% MXD-medicated serum; (4) Cells stimulated with PM2.5 was treated with 20% MXD medicated-serum plus rHMGB1 (1 µg/ml). The application concentration of rHMGB1 was determined according to the previously published articles (Lv et al., 2009; Han et al., 2016). PM2.5 was added simultaneously after the addition of serum and rHMGB1. After 24 h, the release of cytokines and the expression of TLR4, MyD88 and p-p65 was determined as described in Cytokine Assays in Medium and Western Blot Analysis section.
Statistical Analysis
All experimental data were analyzed using SPSS software 22.0 (SPSS Inc., Chicago, IL, USA) and GraphPad Prism 5.0 (GraphPad Software, La Jolla, CA, USA) Statistically significant differences between groups were determined by one-way ANOVA followed by Tukey's post hoc test for multigroup comparisons. A value of p < 0.05 was considered statistically significant.
Results
Characterization Of MXD Using Mass Spectroscopy
UPLC-MS/MS results of reference standard compounds and MXD sample were shown in Figures 1–3. Five major components of MXD were verified by comparing individual peak retention time and quantifier ion with that of reference standard compounds. External standard method of peak area was employed for quantitative analysis. The concentrations of major components of MXD are 1.135 mg/ml, 0.643 mg/ml, 1.041 mg/ml, 2.019 mg/ml, and 12.280 mg/ml for ephedrine, pseudoephedrine, liquiritin, glycyrrhizic acid, and amygdalin respectively.
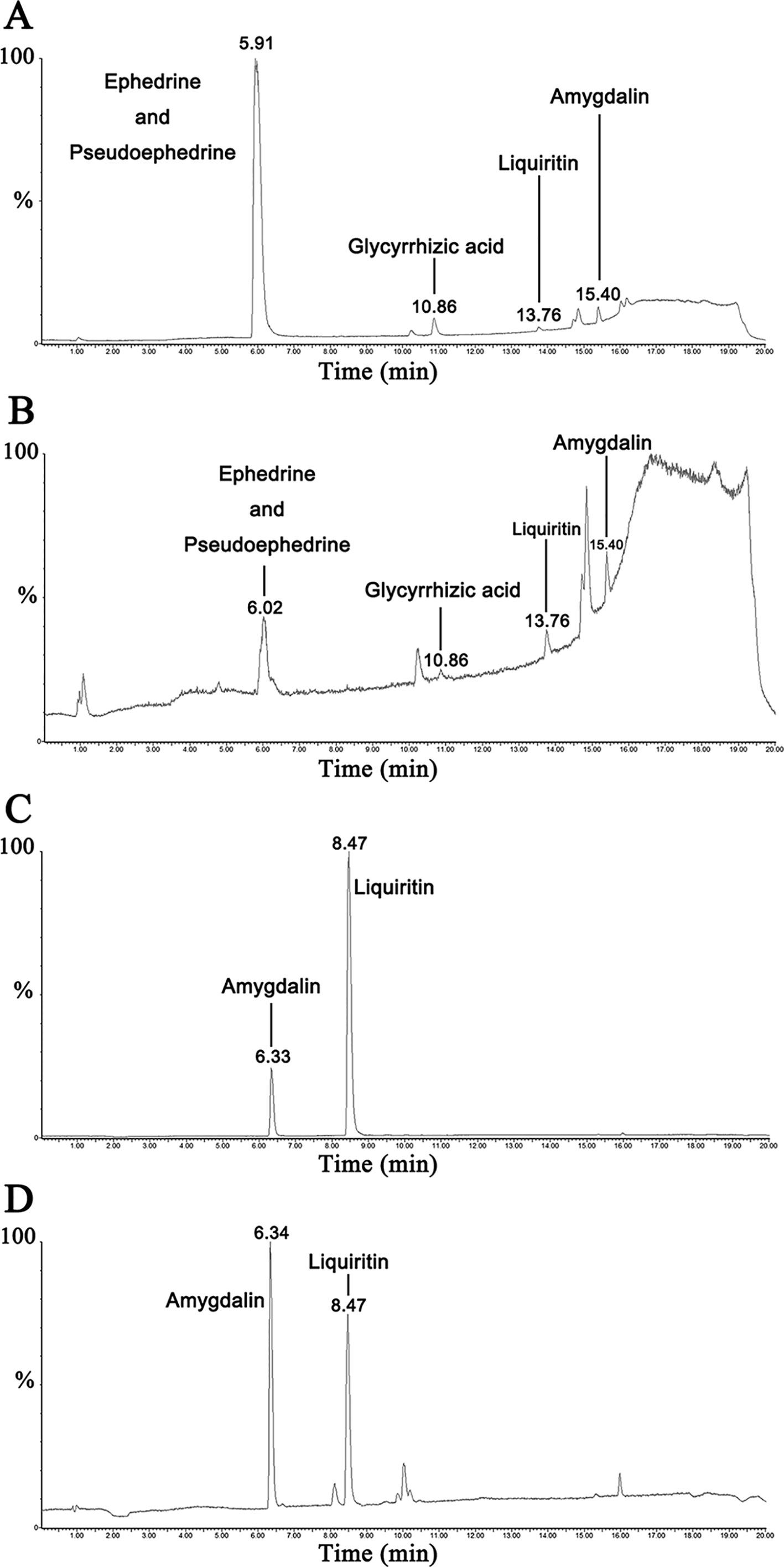
Figure 1 Representative chromatograms of reference standard solution and MXD sample. (A) Representative chromatograms of standard solution in positive electrospray ionization mode. (B) Representative chromatograms of MXD sample in positive electrospray ionization mode. (C) Representative chromatograms of standard solution in negative electrospray ionization mode. (D) Representative chromatograms of MXD sample in negative electrospray ionization mode.
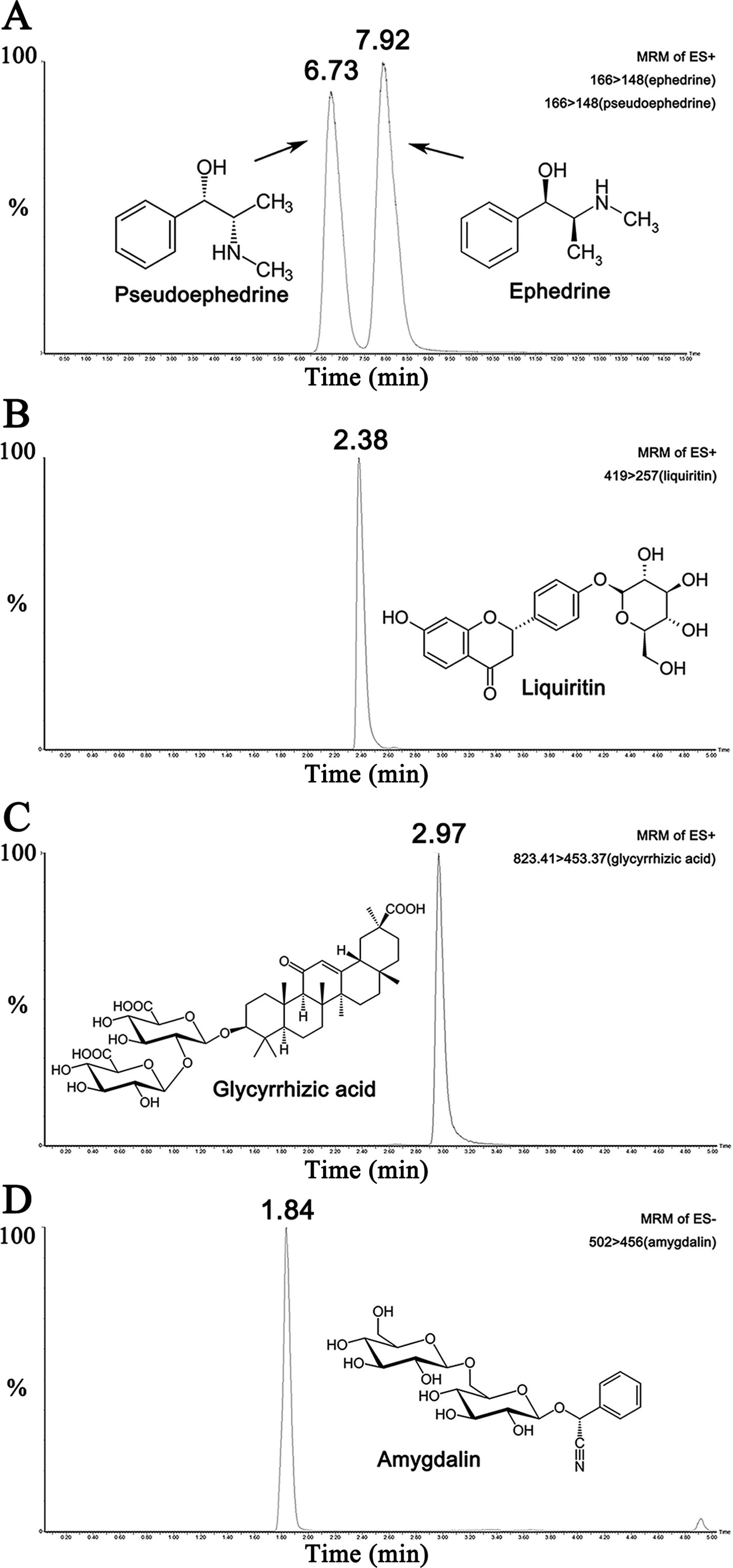
Figure 2 Representative chromatograms of the five compounds in reference standard solution. (A) Representative chromatograms of ephedrine (500 ng/ml) and pseudoephedrine (500 ng/ml) in standard solution. (B) Representative chromatogram of liquiritin (500 ng/ml) in standard solution. (C) Representative chromatogram of glycyrrhizic acid (500 ng/ml) in standard solution. (D) Representative chromatogram of amygdalin (500 ng/ml) in standard solution.
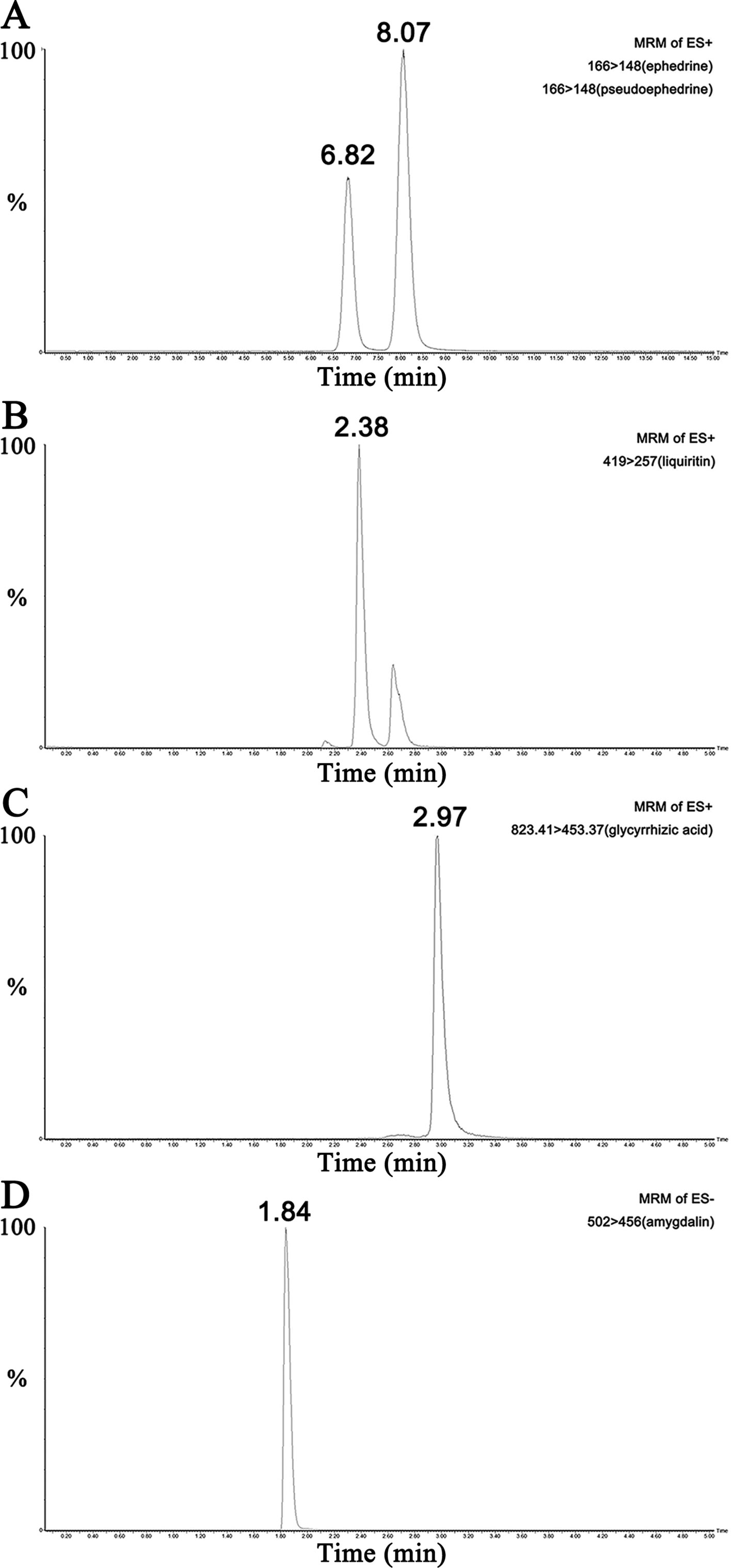
Figure 3 Representative chromatograms of the five compounds in MXD sample. (A) Representative chromatograms of ephedrine and pseudoephedrine in MXD sample. (B) Representative chromatogram of liquiritin in MXD sample. (C) Representative chromatogram of glycyrrhizic acid in MXD sample. (D) Representative chromatogram of amygdalin in MXD sample.
Histopathological Results
H&E staining was used to monitor tissue structures and quantify morphological changes.
As shown in Figure 4A. In control groups, sections of all structures of the lung including bronchioles, alveolus, and pulmonary vessel showed normal morphology. The most severe lung injury was observed in the PM2.5 group. The remarkable changes in the PM2.5 group were the thickening of alveolar walls, lung edema, and alveolar hemorrhage. However, these histopathologic changes were markedly alleviated with the treatment of MXD. The lung damage score was significantly higher in PM2.5 group compared to that of control group (Figure 4B, p < 0.01). MXD (16.4 g/kg) treatment significantly decreased the lung damage score (p < 0.05), indicating that MXD repressed PM2.5 induced histopathological damage of the lung.
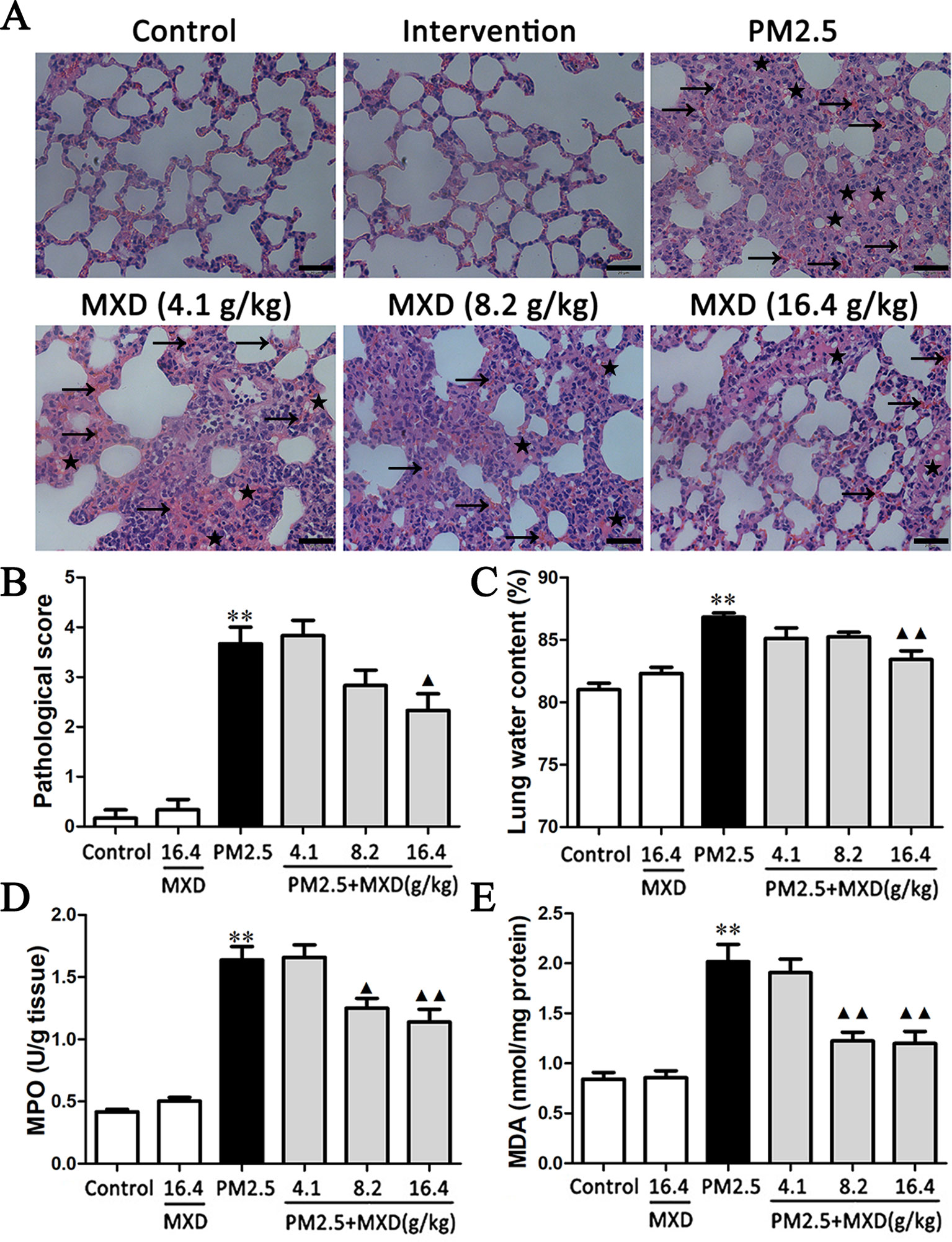
Figure 4 Effects of MXD on PM2.5 induced pathological changes and biochemical indexes changes in lung tissue after a 5-day continuous treatment. (A) Effects of MXD on the pathological changes of the lung tissue induced by PM2.5. The local hemorrhage was marked with arrow, and the local edema was marked with lightning bolt. Scale bar, 20 μm (×400). (B) Quantified scoring of pathological changes in lung tissue (n = 6). (C) Effects of MXD on the lung edema induced by PM2.5 (n = 8). (D) Effects of MXD on MPO activity in lung tissues (n=6). (E) Effects of MXD on MDA content in lung tissues (n = 7). Data are expressed as mean ± SEM. **p < 0.01 vs. Control group; ▴p < 0.05, ▴▴p < 0.01 vs. PM2.5 group.
MXD Inhibited PM2.5 Induced Lung Edema
During lung injury, the increased capillary permeability may lead to lung edema. Two hours after the last drug administration, the lung water content was evaluated.
As shown in Figure 4C, PM2.5 significantly increased the lung water content in comparison with that of the control group (p < 0.01). Treatment with MXD (16.4 g/kg) significantly decreased the lung water content compared with PM2.5 group (p < 0.01), indicating that the PM2.5 induced lung edema was attenuated by MXD.
MXD Reduces PM2.5 Induced MPO Activity and MDA Content
MPO is an index of neutrophil infiltration and MDA content is an indicator of lipid peroxidation, both of which are regarded as an index to evaluate severity of tissue injury.
As shown in Figure 4D, PM2.5 stimulation increased the MPO activity in lung tissue compared with the control group (p < 0.01). In contrast, the levels of lung MPO activity was down-regulated by MXD (8.2 g/kg and 16.4 g/kg) significantly (p < 0.05 and p < 0.01).
As shown in Figure 4E, PM2.5 stimulation increased the content of MDA significantly (p < 0.01), which was reduced significantly by MXD (8.2 g/kg and 16.4 g/kg) (p < 0.01).
Our results suggested that MXD can alleviated the severity of tissue injury induced by PM2.5.
MXD Reduces the Infiltration of CD68 Positive Macrophages in Lung Tissue
The excessive activation of macrophage plays a critical role in the progress of lung injury. CD68 expressed on the surface of macrophage is a well-characterized marker of macrophage activation (Leenen et al., 1994; Dambach et al., 2002; Lloyd et al., 2008; Iwasaki et al., 2011). Hence, the expression of CD68 was detected using IHC to evaluate the activation of macrophage in lung tissue.
As shown in Figure 5A, the accumulation of CD68 positive macrophages in lung tissue was significantly increased in PM2.5 group compared with that of control group. After treatment with MXD, the number of CD68 positive macrophage was significantly reduced, indicating that MXD attenuated the PM2.5 induced activation of macrophage in lung tissue.
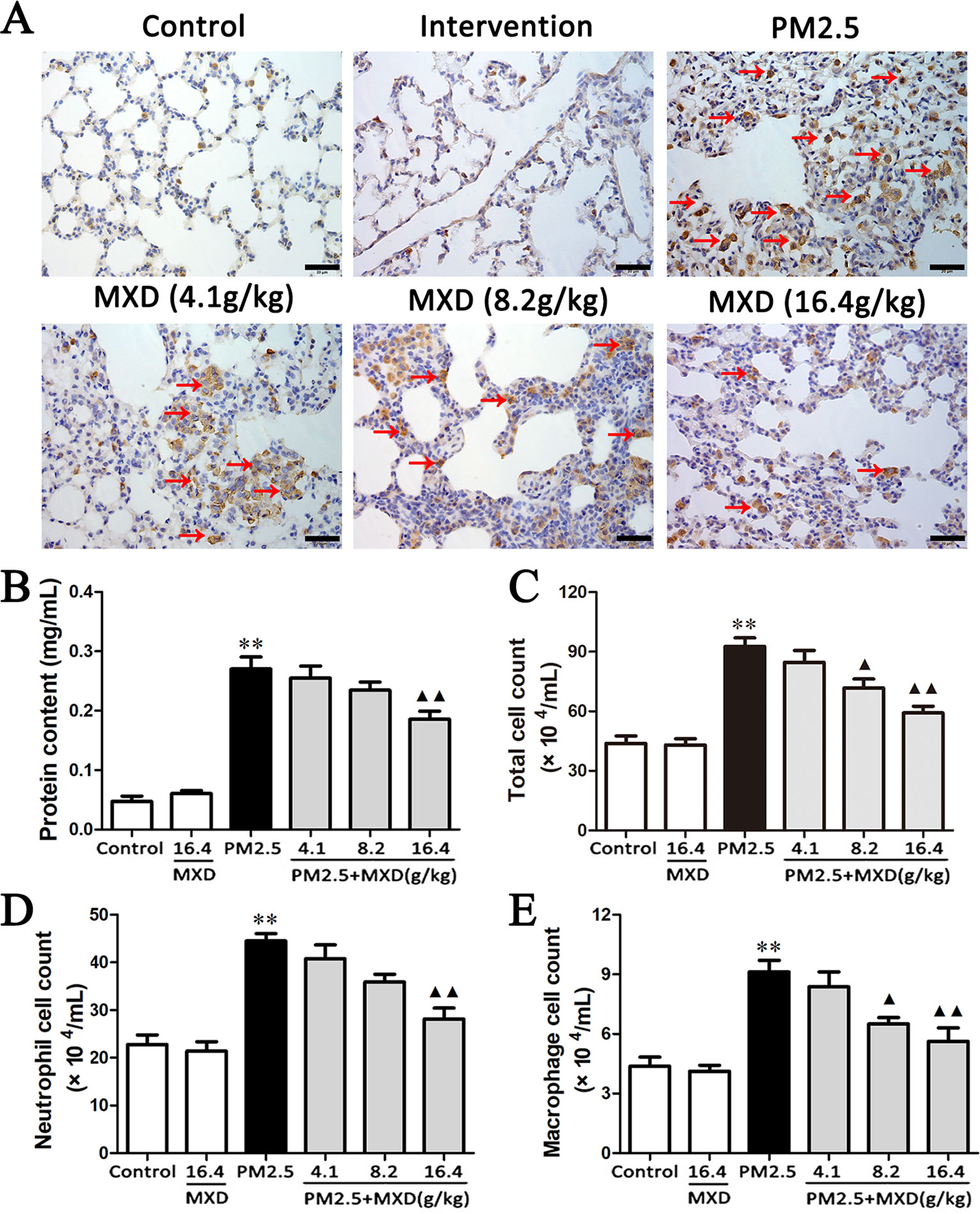
Figure 5 Effects of MXD on PM2.5 induced macrophage activation and alveolar barrier disruption in lung tissue after a 5-day continuous treatment. (A) Effects of MXD on the expression of CD68 in lung tissue. CD68 positive cell were marked with arrow. Scale bar, 20 μm (×400). (B) Effects of MXD on the protein content in BALF (n = 7). (C) Effects of MXD on the total cell count in BALF (n = 8). (D) Effects of MXD on the neutrophil cell count in BALF (n = 8). (E) Effects of MXD on the macrophage cell count in BALF (n = 8). Data are expressed as mean ± SEM. **p < 0.01 vs. Control group; ▴p < 0.05, ▴▴p < 0.01 vs. PM2.5 group.
MXD Alleviates PM2.5 Induced Alveolar Permeability
Severe injury to the alveolar epithelial/endothelial membrane may result in the disruption of alveolar capillary barrier, which is manifested by the leakage of fluid from the vascular circulation into the interstitial and alveolar spaces. The leakage of fluid will result in increased protein concentration and cell number in BALF. Hence, the total protein content and cell number in BALF were detected to evaluate the effect of MXD on lung epithelial barrier function.
As shown in Figure 5B, protein content was significantly increased in PM2.5 group compared with that of control group (p < 0.01). Treatment with MXD (16.4 g/kg) showed a significant decrease in protein content in comparison with that of PM2.5 group (p < 0.01).
As shown in Figure 5C, after PM2.5 stimulation, the total cell count in BALF was significantly increased (p < 0.01), which was significantly reversed by the treatment of MXD (8.2 g/kg and 16.4 g/kg) significantly (p < 0.05 and p < 0.01). For further confirming what type of inflammatory cell is elicited by PM2.5 challenge, the number of neutrophils and macrophages in the BALF was counted respectively using Wright-Giemsa staining. As can be seen in Figures 5D, E, stimulation with PM2.5 increased the number of both neutrophils and macrophages in BALF significantly (p < 0.01). Treatment with MXD (16.4 g/kg) decreased the cell count of both neutrophils and macrophages (p < 0.01).
Taken above, our results showed MXD can alleviate the PM2.5 induced alveolar capillary barrier disruption. Notably, the infiltration of inflammatory cells, such as neutrophils and macrophages, was also reduced.
MXD Attenuates PM2.5 Induced Inflammatory Cytokine Production in Serum and BALF
Inflammatory factors contribute to the development of lung injury, among which TNF-α, IL-1β and IL-6 are the major cytokines (Quay et al., 1998; Smith et al., 1998; Koksoy et al., 2001; Goodman et al., 2003; Saito et al., 2008; Wilson et al., 2010; Pedroza et al., 2011; Patel et al., 2013). Hence, the concentrations of TNF-α, IL-1β and IL-6 in both serum and BALF were determined using ELISA kits.
As shown in Figures 6A–C. The effect of MXD on TNF-α, IL-1β and IL-6 production in serum was analyzed by ELISA after PM2.5 challenge. Compared with the control group, the levels of TNF-α, IL-6, and IL-1β in serum were significantly increased after PM2.5 stimulation (p < 0.01). MXD (16.4 g/kg) reduced TNF-α (p < 0.01), IL-1β (p < 0.01), and IL-6 (p < 0.01) production compared with that of the PM2.5 group.
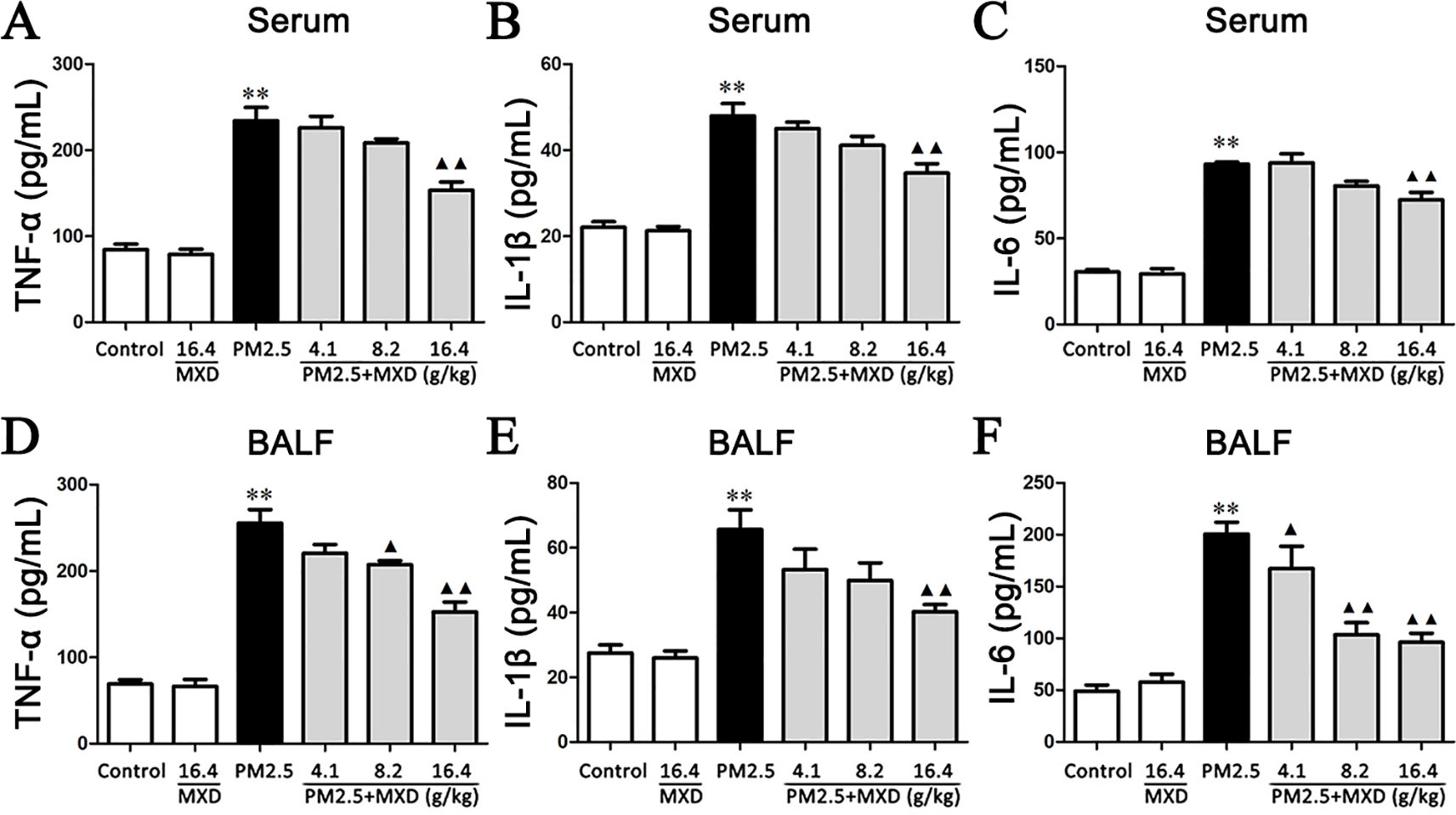
Figure 6 Effects of MXD on the levels of proinflammatory factors in serum and BALF after a 5-day continuous treatment. The levels of TNF-α (A), IL-1β (B) and IL-6 (C) in serum were examined. The levels of TNF-α (D), IL-1β (E), and IL-6 (F) in BALF were examined. Data are expressed as mean ± SEM, n = 5. **p < 0.01 vs. Control group; ▴p < 0.05, ▴▴p < 0.01 vs. PM2.5 group.
As shown in Figures 6D–F, PM2.5 exposure resulted in a marked increase in the levels of TNF-α, IL-1β and IL-6 in BALF compared with control group (p < 0.01). Treatment with MXD (16.4 g/kg) significantly ameliorated the levels of these inflammatory cytokines (p < 0.01).
Our results showed that treatment with MXD reduced the release of pro-inflammatory cytokines after PM2.5 challenge, indicating the anti-inflammatory potential of MXD.
Effect of MXD on Cell Viability of RAW 264.7 Exposed to PM2.5
In order to assess the effect of MXD mediated serum on PM2.5 induced injury in RAW 264.7 cells, we first confirmed the occurrence of PM2.5 induced damage by comparing the viability between the PM2.5-stimulated group and the control group. As shown in Figure 7A, when exposed to PM2.5 at 10 µg/cm2 for 24 h, the cell viability of RAW 264.7 was significantly decreased by 50% in comparison with normal cultured cells (p < 0.01). Hence, the concentration of PM2.5 at 10 µg/cm2 was selected for the following experiment.
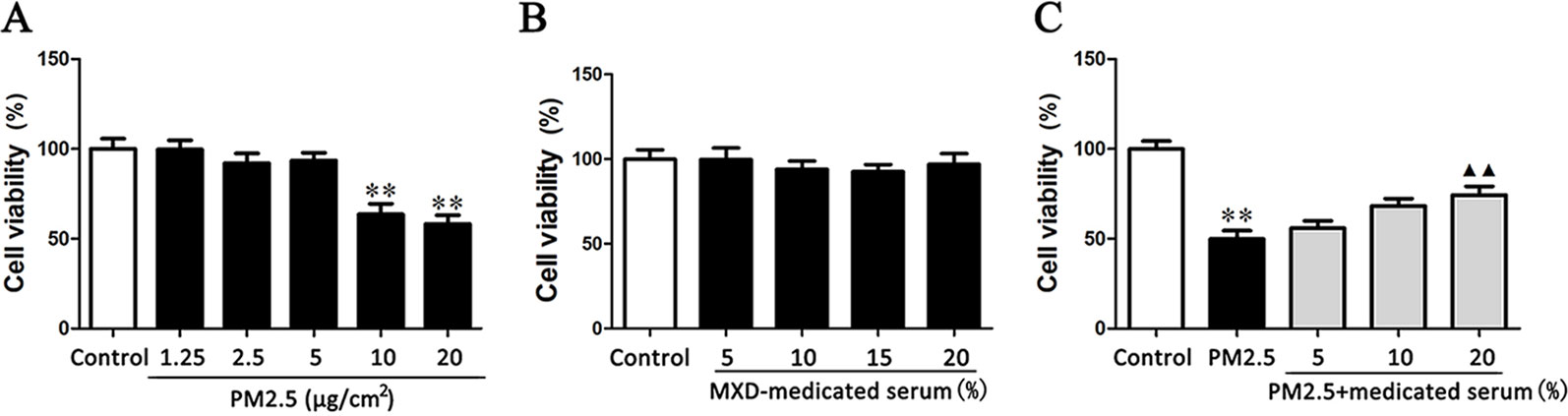
Figure 7 Effects of PM2.5 and MXD-medicated serum on the cell viability of RAW 264.7 after a 24-hour incubation. (A) The cytotoxic effects of PM2.5 on RAW 264.7 cell viability. (B) The confirmation for the non-cytotoxic concentrations (volume fractions) of MXD-medicated serum. (C) Protective effect of MXD-medicated serum on RAW 264.7 exposed to PM2.5. Data are expressed as mean ± SEM, n = 6. **p < 0.01 vs. Control group; ▴▴p < 0.01 vs. PM2.5 group.
Then the non-cytotoxic concentrations (volume fractions) range of MXD-medicated serum was determined by incubating RAW 264.7 cells with MXD-medicated serum at a series of concentrations. As shown in Figure 7B, MXD-medicated serum at the concentrations from 5 to 20% showed no effect on the cell viability (p > 0.05). Considering the volume fraction of serum in culture system doesn’t exceed 20% generally, the concentrations of MXD-medicated serum at 5, 10 and 20% were applicable to all subsequent experiments.
The protective effect of MXD-medicated serum on RAW264.7 cell challenged by PM2.5 was evaluated based on the selected PM2.5 concentration and MXD-medicated serum concentrations. As shown in Figure 7C, MXD-medicated serum at the concentration of 20% significantly reversed the decrease of cell viability induced by PM2.5 (p < 0.01).
Effect of MXD on the Production of Proinflammatory Cytokines In Vitro
The effects of MXD-medicated serum on TNF-α, IL-1β, and IL-6 production in the culture supernatant were detected by ELISA. As shown in Figures 8A–C, PM2.5 challenge increased the level of TNF-α, IL-1β, and IL-6 compared with the control group (p < 0.01). In contrast, incubation with 20% MXD-medicated serum decreased the levels of TNF-α (p < 0.01), IL-1β (p < 0.05), and IL-6 (p < 0.05), indicating the inhibitory effect of MXD-medicated serum on the release of proinflammatory cytokines.
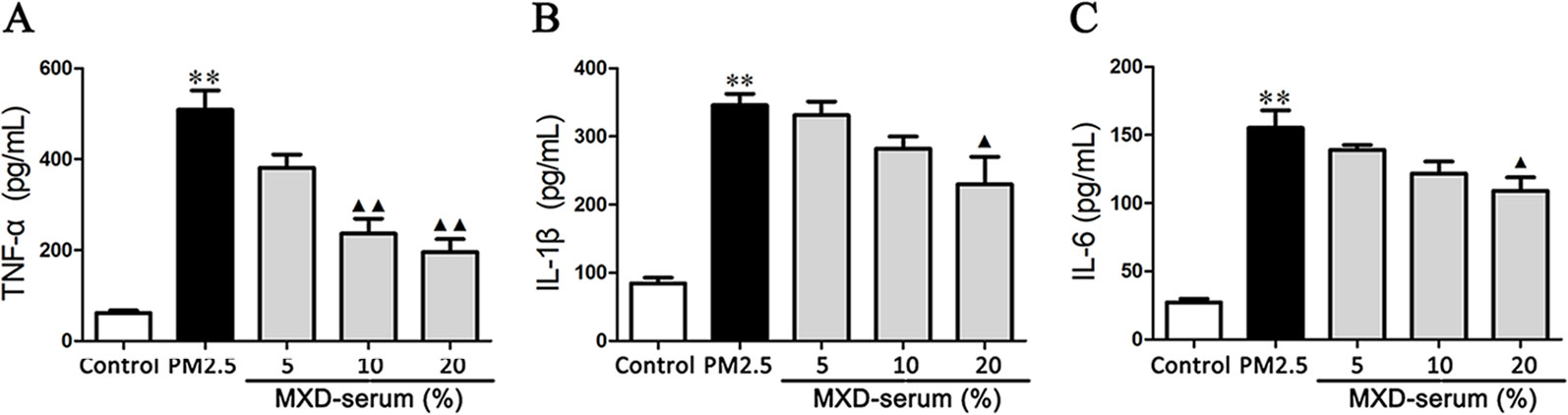
Figure 8 Effects of MXD-medicated serum on the secretion of proinflammatory factors from RAW 264.7 after a 24-hour incubation. The levels of TNF-α (A), IL-1β (B), and IL-6 (C) in the culture supernatant were examined. Data are expressed as mean ± SEM, n = 5. **p < 0.01 vs. Control group; ▴p < 0.05, ▴▴p < 0.01 vs. PM2.5 group.
Effect of MXD on PM2.5 Induced HMGB1/TLR4/NFκB Activation In Vivo and In Vitro
Recent studies suggest that HMGB1 interacts with TLR4 and induces the activation of NFκB signaling pathway, which plays a major role in the release of inflammatory cytokines and the development of organ dysfunction (Yang et al., 2014; Sun et al., 2015). Hence, the expressions of the key proteins in HMGB1/TLR4/NFκB signaling pathway were examined by Western blot both in vivo and in vitro.
As shown in Figure 9, the protein levels of HMGB1, TLR4, MyD88, and p-p65 in lung tissues were significantly increased in PM2.5 group compared with control group (p < 0.01), while treatment with MXD (16.4 g/kg) significantly decreased the protein levels of HMGB1 (p < 0.01), TLR4 (p < 0.05), MyD88 (p < 0.05), and p-p65 (p < 0.01).
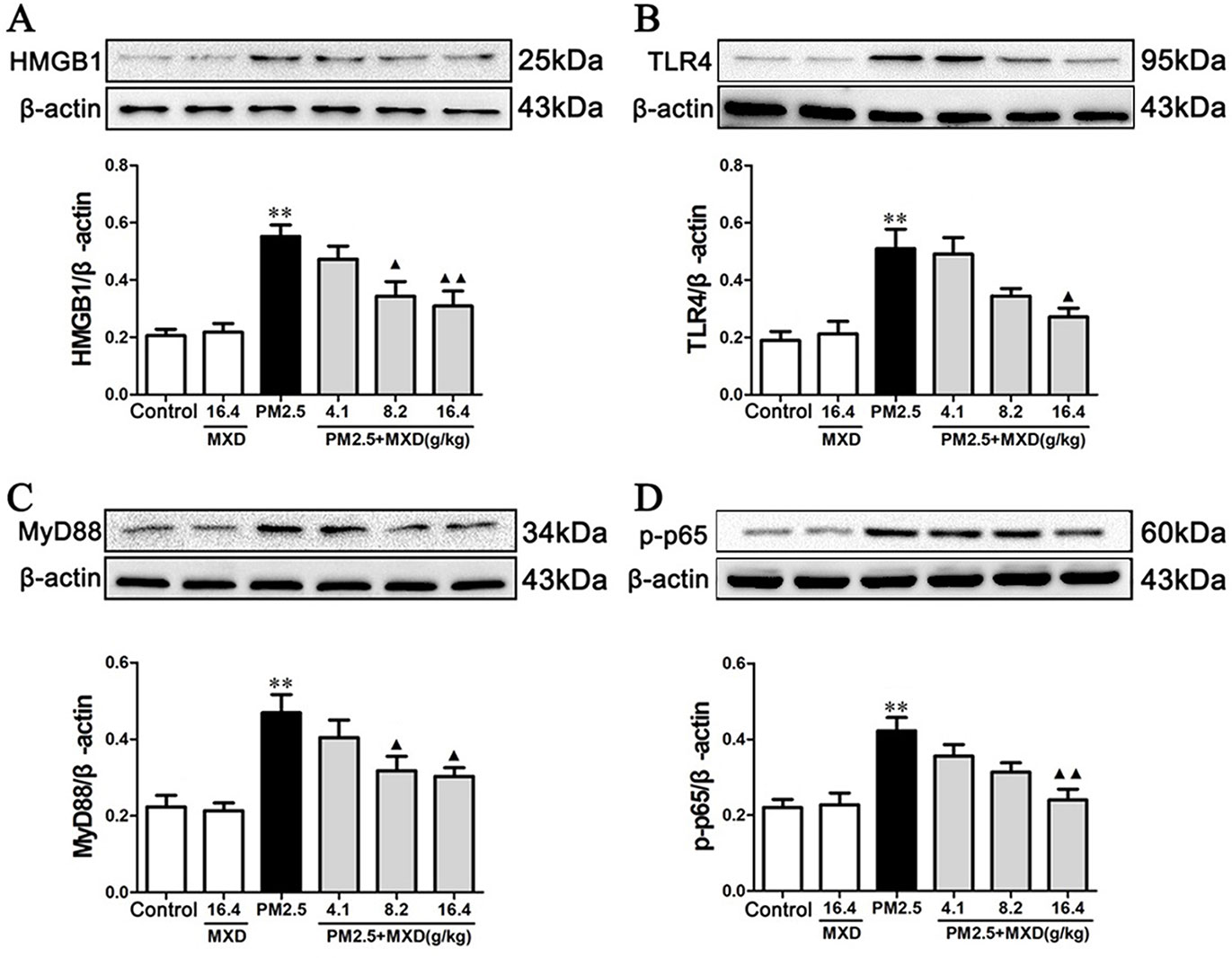
Figure 9 Effects of MXD on the protein levels of HMGB1 (A), TLR4 (B), MyD88 (C), and p-p65 (D) in rat lung tissues after a 5-day continuous treatment. The values presented are the mean ± SEM (n = 5) of three independent experiments. The density values of protein expression were normalized to β-actin. **p < 0.01 vs. Control group; ▴p < 0.05, ▴▴p < 0.01 vs. PM2.5 group.
As shown in Figure 10, stimulation with PM2.5 significantly up-regulated the proteins levels of HMGB1, TLR4, MyD88, and p-p65 in RAW 264.7 cells (p < 0.01). Incubation with 20% MXD-medicated serum significantly down-regulated the expressions of HMGB1 (p < 0.01), TLR4 (p < 0.01), MyD88 (p < 0.01), and p-p65 (p < 0.05), which was in accordance with what we observed in vivo.
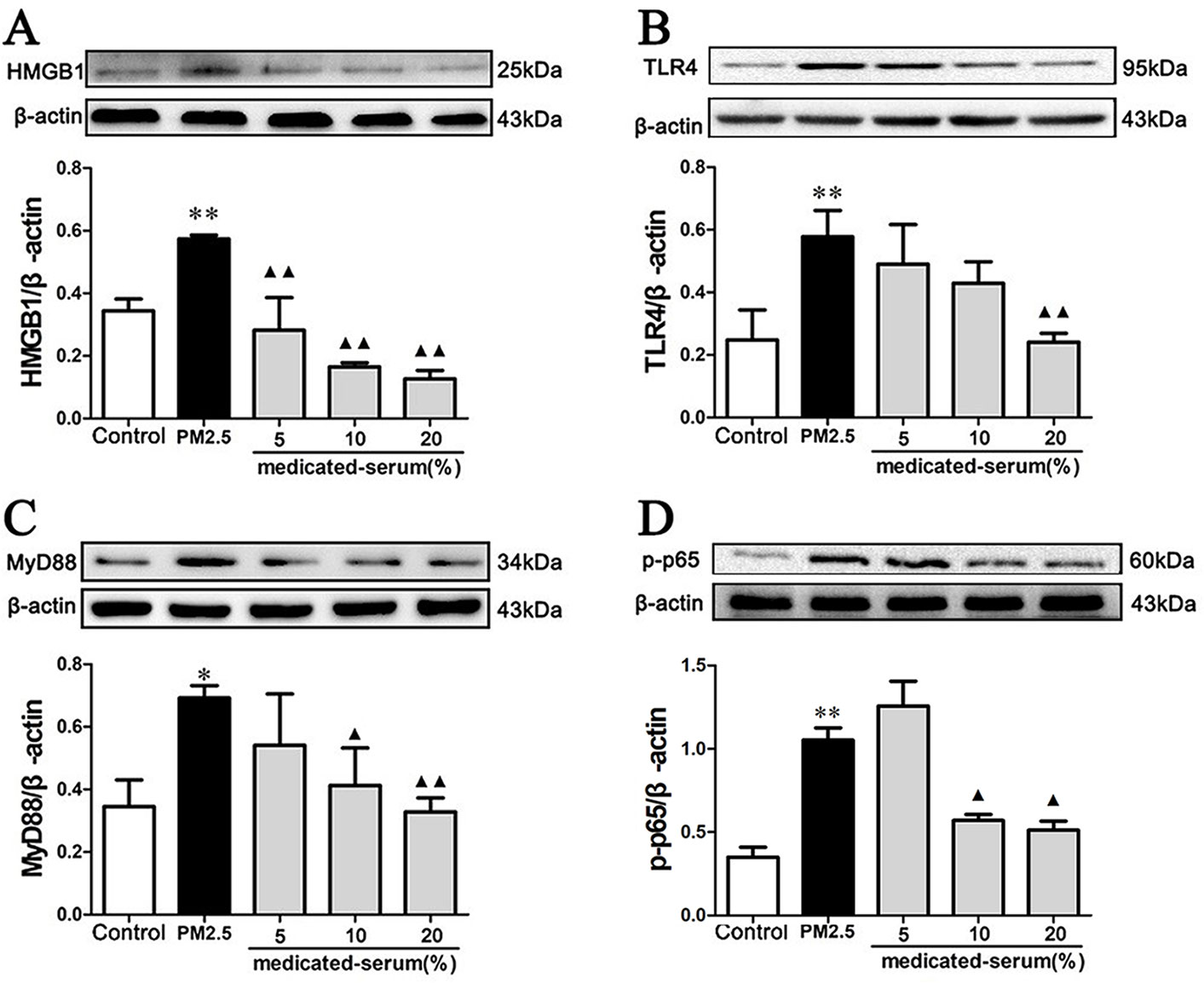
Figure 10 Effects of MXD-medicated serum on the protein levels of HMGB1 (A), TLR4 (B), MyD88 (C), and p-p65 (D) in RAW 264.7 cells after a 24-hour incubation. The values presented are the mean ± SD (n = 3) of three independent experiments. The density values of protein expression were normalized to β-actin. *p < 0.05, **p < 0.01 vs. Control group; ▴p < 0.05, ▴▴p < 0.01 vs. PM2.5 group.
The Effect of MXD-Medicated Serum Was Reversed by rHMGB1
As shown in Figures 11A–C, 20% MXD-medicated serum significantly inhibited the release of proinflammatory factors (p < 0.01), which was consistent with our results above. Notably, co-incubation with rHMGB1 abolished the inhibitory effects of MXD-medicated serum on the release of TNF-α (p < 0.01), IL-1β (p < 0.01), and IL-6 (p < 0.01).
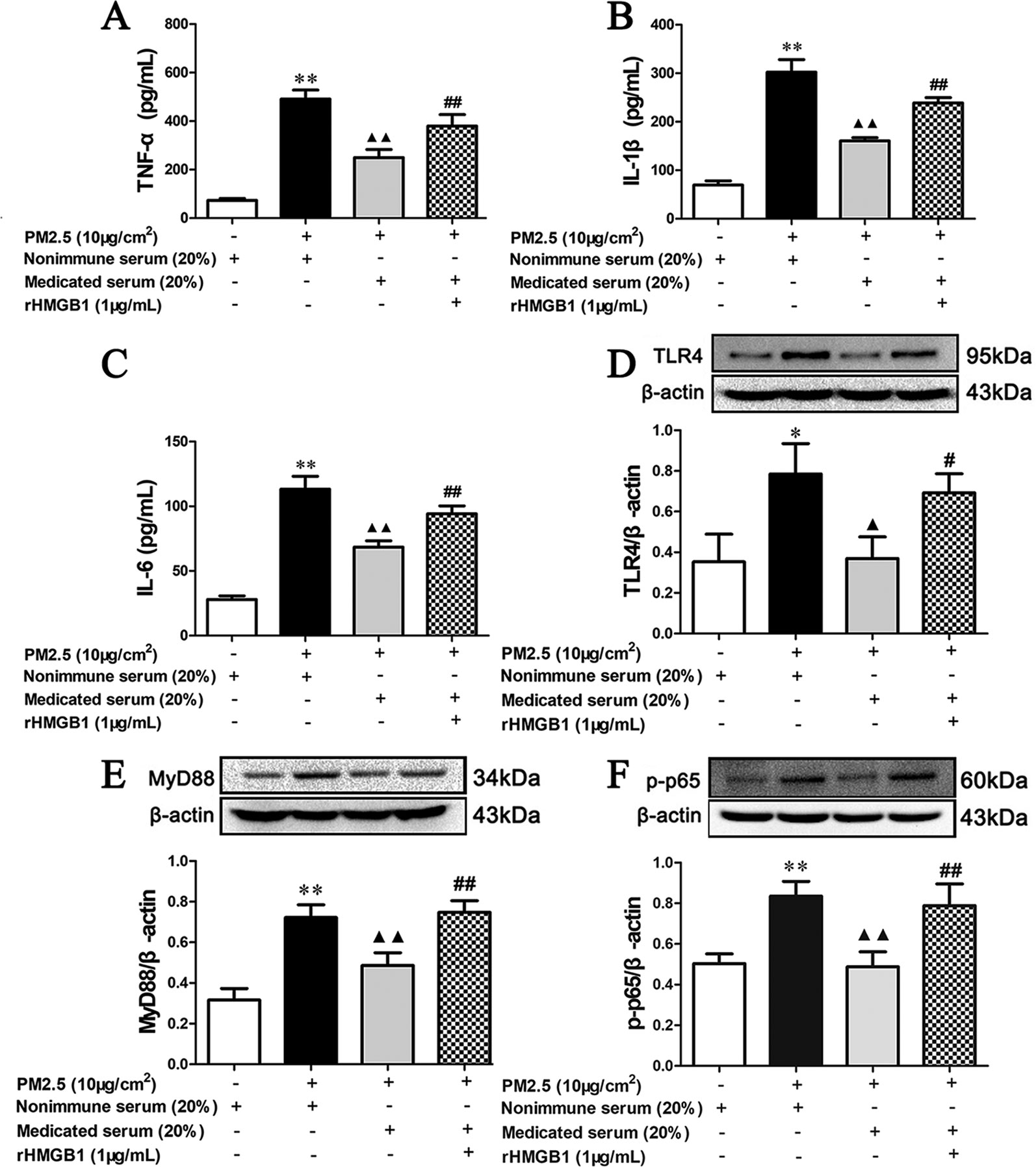
Figure 11 Effects of MXD-medicated serum with or without rHMGB1 treatment on the PM2.5 induced release of inflammatory cytokines and the activation of HMGB1/TLR4/NFκB pathway in RAW 264.7 after a 24-hour incubation. The concentration of TNF-α (A), IL-1β (B), and IL-6 (C) in the supernatant. The expression of TLR4 (D), MyD88 (E), and p-p65 (F) in RAW264.7 cell. For ELISA assay, data are expressed as mean ± SEM, n = 5. For Western blot, the values presented are the mean ± SD (n = 3) of three independent experiments. The density values of protein expression were normalized to β-actin. *p < 0.05, **p < 0.01 vs. Control group; ▴p < 0.05, ▴▴p < 0.01 vs. PM2.5 group; #p < 0.05, ##p < 0.01 vs. Treatment group.
As shown in Figures 11D–F, in accordance with our results above, 20% MXD-medicated serum reduced the expression of TLR4 (p < 0.01), MyD88 (p < 0.01), and p-p65 (p < 0.01). The regulatory effect was again reversed in MXD-medicated serum plus rHMGB1 group (p < 0.01).
These results demonstrate that the incubation of MXD-medicated serum significantly alleviates inflammatory reaction caused by PM2.5 in RAW264.7 cell by antagonizing the function of HMGB1 and the mechanism involved in the regulation of downstream TLR4/MyD88/NFκB signaling pathway.
Discussion
The present study demonstrated that treatment with MXD (or MXD-medicated serum) could protect against PM2.5 induced injury both in vivo and in vitro. MXD could inhibit activation of the HMGB1/TLR4/NFκB signaling pathway and decreased inflammatory cytokine level thereby attenuating inflammatory injury (Figure 12).

Figure 12 Schematic diagram depicting proposed mechanisms for the protection of MXD against PM2.5 induced injury. MXD decreased the expression of HMGB1, TLR4 and downstream MyD88, and inhibited NFκB activation. In addition, the downregulation of proinflammatory cytokines was involved in the protection of MXD. Red arrow and perpendicular line: the effects of MXD.
With the rapid development of modern society, the environment has been seriously polluted. Currently, most northern China areas are deeply trapped in the haze, and the main contaminant of the air is PM (Huang et al., 2014; Yang et al., 2017). PM is composed of inorganic matters, organic matters, metallic minerals, bacteria, and a variety of complex ingredients (Querol et al., 2004; Kleindienst et al., 2010; Huang et al., 2014; Pipal et al., 2014). A most harmful particle to human health is PM2.5. Several studies have shown that PM2.5 can travel deeper into the lungs, and exposure to PM2.5 is associated with pulmonary and cardiovascular diseases and cancer (Vineis and Husgafvel-Pursiainen, 2005; Tecer et al., 2008; Polichetti et al., 2009; Silva et al., 2010; Leiva G et al., 2013; Martínez-Cinco et al., 2016; Xing et al., 2016). During the process of pulmonary diseases, inflammatory reaction plays an important role (Welbourn and Young, 1992; Ghio and Devlin, 2001; Ricard et al., 2001; Peng et al., 2004; Menezes et al., 2005). Many preliminary studies confirmed the anti-inflammatory and immune-regulatory effects of natural products, while several data showed a decreased effect of their metabolites on cytokines for inflammation, such as IL-1, IL-6, and TNF-α (Teng et al., 2016, Teng et al., 2017; Chen et al., 2018, Chen et al., 2019; Gupta et al., 2019; Magalhães et al., 2019; Saudagar and Saokar, 2019; Su et al., 2019; Zhang et al., 2019). Hence, it arouses our interest that whether MXD, a famous traditional Chinese medicine formula widely used in respiratory diseases, can alleviate PM2.5 induced lung injury.
Ma huang, a traditional Chinese herb, is often used to treat asthma, nose and lung congestion, and fever with anhidrosis. The main constituent of ma huang is ephedrine and pseudoephedrine, which showed potential in attenuating inflammatory response (Kasahara et al., 1985; Zheng et al., 2012; Wu et al., 2014).
Ku xing ren is widely used for treatment of cough and asthma. Modern pharmacology indicates that amygdalin is an effective component of ku xing ren (Gao et al., 2012). Recent studies have indicated that amygdalin showed anti-inflammatory activity both in vivo and in vitro (Yang et al., 2007; Lin and Lin, 2011).
Shi gao is often used in combination with Ma huang to exert the antipyretic and anti-asthmatic activities (Mei et al., 2016).
Gan cao is one of the oldest and most popular herbal medicines in the world, and is recorded in the pharmacopoeias of many Asian and European countries including China, Japan, the United Kingdom and others (Zhang and Ye, 2009). The main constituent of gan cao is glycyrrhizic acid which exhibits anti-inflammatory, anti-viral, anti-allergenic, anti-ulcer, and anti-oxidative properties (Gan et al., 2012; Ming and Yin, 2013; Tang et al., 2015). Recent studies have shown that glycyrrhizic acid on the regulation of HMGB1/TLR4/NFκB signaling pathway in many diseases (Lau et al., 2014; Pang et al., 2016).
At present, the most commonly used approach for establishing an animal model of PM2.5 induced lung injury is intratracheal instillation of PM2.5 suspension (Li et al., 2013; Dysart et al., 2014). In this study, we establish the model of acute lung injury by instillation with PM2.5 suspension.
As one of the major characteristics of lung injury, the magnitude of pulmonary edema was quantified by evaluating the lung water content (Liu et al., 2013; Feng et al., 2016). Our results showed that PM2.5 induced edema of the lung, which was reflected by the significant increase in lung water content. Administration of MXD ameliorated PM2.5 induced lung edema.
MPO activity, a marker reflecting the parenchymal infiltration of neutrophils, was assessed as an important index of tissue damage (Lin et al., 2016; Zhou et al., 2016). Increased MPO level may strengthen ability to resist infection, however, it may also increase the synthesis of hypochlorous acid, which can be detrimental to normal tissue (Wei et al., 2004). Overall, the results suggest that MXD attenuates PM2.5 induced elevated MPO level. MDA, a marker of lipid peroxidation, is usually measured as a tissue lesion index. After PM2.5 stimulation, MDA was significantly increased, which was significantly reversed with the treatment of MXD, indicating the effect of MXD against oxidative damage.
Alveolar macrophages have been reported to play important roles in PM2.5 induced lung injury (Meng and Zhang, 2007; Xing et al., 2016). Stimulating alveolar macrophages with PM2.5 induce the production of inflammatory mediators, which contribute to the severity of lung injury by initiating, amplifying, and perpetuating the inflammatory response (Becker et al., 1996; Ning et al., 2000; Hetland et al., 2005). These cytokines not only amplify the inflammatory cascade and cause the inflammatory injury, but also recruit neutrophils into lung and exhibit increased MPO activity in the lungs (Xie et al., 2009). In the present study, increased levels of TNF-α, IL-1β, and IL-6 in serum, BALF and RAW 264.7 cell culture supernatant have been noted in PM2.5 groups. MXD significantly inhibited the production of TNF-α, IL-1β, and IL-6, which indicated that the protective effects of MXD on PM2.5 induced lung injury may be attributed to the inhibition of inflammatory cytokines.
HMGB1 is a highly conserved DNA binding protein. As a nuclear protein, HMGB1 acts as an architectural chromatin-binding factor that bends DNA and promotes protein assembly on specific DNA targets (Scaffidi et al., 2002). In addition to its intranuclear role, HMGB1 also functions as an extracellular signaling molecule during inflammation (Yang et al., 2010a). HMGB1 can be either actively secreted to the extracellular through the activation of macrophages and monocytes or passively released from necrotic or apoptotic cells (Rovere-Querini et al., 2004; Bell et al., 2006). Extracellular HMGB1 activates multiple membrane receptors, including but not limited to receptor for advanced glycation end products, and TLR4, through which it contributes to the pathogenesis of lung injury, stroke, cancer, and so on (Andersson and Erlandsson-Harris, 2004; Kokkola et al., 2005; Muhammad et al., 2008; Sims et al., 2009; Yang and Tracey, 2010; Yang et al., 2010b; Schierbeck et al., 2011; Tolle and Standiford, 2013; Lv et al., 2016; Pandolfi et al., 2016). Furthermore, extracellular HMGB1 is responsible for the progression of PM2.5 induced lung injury (Shoenfelt et al., 2009; Jing et al., 2017; Tang et al., 2018).
TLR4 locating on the cell plasma membrane is one of the best characterized TLRs, which recognizes the Gram-negative product LPS and participates in the recognition of several endogenous ligands including HMGB1 and heat shock protein (Erridge, 2010; Kay et al., 2014). As a canonical downstream adaptor, MyD88 is closely involved in inflammatory signaling pathways mediated by the TLRs. The downstream NFκB is a critical factor in regulating both innate and adaptive immunity, which enhances various gene expressions during inflammatory responses (Chen et al., 2016). HMGB1 binds with TLR4, leading to nuclear factor-kappa B (NFκB) activation recognized by p65 phosphorylation, and promotes the release of inflammatory factors including TNF-α, IL-1β, and IL-6 (Lawrence, 2009; Tang et al., 2009; Shin et al., 2016). Pharmacological inhibition of TLR4/MyD88/NFκB pathway led to decrease of inflammatory cytokines and showed protective effects on acute lung injury (Jiang et al., 2015). In this study, the expression of HMGB1, TLR4, MyD88, and p-p65 were detected by Western blot analysis in vivo and in vitro. The results showed that PM2.5 significantly increased the expression of HMGB1, TLR4 and MyD88, and upregulated the phosphorylation level of p65. However, treatment with MXD decreased expression of HMGB1, TLR4 and MyD88 and inhibited the phosphorylation of p65. In all, these results showed that MXD could inhibit the activation of HMGB1/TLR4/NFκB pathway, alleviate the inflammation reaction and protect the lung from PM2.5 induced injury.
However, several limitations of our study must be acknowledged. This study only focuses on the HMGB1/TLR4/NFκB pathway, other pathways related to inflammation such as JAK/STAT and MAPK should be explored in future studies. Moreover, whether the anti-inflammatory effect of MXD requires five components together still remains unknown. Fangjiomics, a recently emerged new discipline to systematically study myriad compatible combinations that may act through multiple targets and modes of actions, maybe a good choice to elucidate the underlying mechanism (Wang et al., 2013; Duan et al., 2015).
In summary, the present study demonstrated that MXD protected against PM2.5 induced lung injury by inhibiting pulmonary edema, MPO activity, MDA content, inflammatory cytokines (IL-1β, IL-6, and TNF-α) production. The protective mechanism of MXD may be related to the inhibition of HMGB1/TLR4/NFκB signaling pathway.
Data Availability Statement
All datasets generated for this study are included in the article/Supplementary Material.
Ethics Statement
The animal study was reviewed and approved by Institutional Animal Care and Use Committee of China Pharmaceutical University.
Author Contributions
Participated in research design: Y-xF and Y-fW. Methodology: Y-xF, G-HR, and B-WW. Formal analysis: Y-xF, BZ, Q-yY, and Y-yQ. Conducted experiments: Y-xF, BZ, Q-yY, and Y-yQ. Writing original draft preparation: Y-xF. Review and editing: Y-xF, Y-fW, and Y-mL. Supervision: Y-fW, W-rF, and Y-mL. Project administration: Y-mL. Funding acquisition: Y-fW.
Funding
This research was funded by the “Key Project of Nanjing Department of Health,” grant number ZKX17047.
Conflict of Interest
The authors declare that the research was conducted in the absence of any commercial or financial relationships that could be construed as a potential conflict of interest.
Acknowledgments
The authors thank Xiao-nan Ma, Min-hui Sun, and Ying-jian Hou for technical support from Cellular and Molecular Biology Center of China Pharmaceutical University.
Abbreviations
MXD, Ma Xing Shi Gan Decoction; PM2.5, Particulate matters with diameter of less than 2.5 µm; MPO, Myeloperoxidase; MDA, Malondialdehyde; HE Hematoxylin and eosin; BALF, Bronchoalveolar lavage fluid; IHC, Immunohistochemistry; TNF-α, Tumor necrosis factor-alpha; IL-1β, Interleukin-1beta; IL-6, Interleukin-6; ELISA, Enzyme linked immunosorbent assay; HMGB1, High-mobility group box 1; rHMGB1, Recombinant high-mobility group box 1; TLR4, Toll-like receptor 4; MyD88, Myeloid differentiation factor 88; p-p65, Phosphorylated p65; UPLC-MS, Ultra-performance liquid chromatography-tandem mass spectrometry; PBS, Phosphatic buffer solution; TMB, 3,3′,5,5′-tetramethylbenzidine; RIPA, Radio immunoprecipitation assay; PMSF, Phenylmethylsulfonyl fluoride; BCA, Bicinchoninic acid; PVDF, Polyvinylidene fluoride; ECL, Enhanced chemiluminescence; NFκB, Nuclear factor-kappa B.
Supplementary Material
The Supplementary Material for this article can be found online at: https://www.frontiersin.org/articles/10.3389/fphar.2019.01361/full#supplementary-material
References
Andersson, U., Erlandsson-Harris, H. (2004). HMGB1 is a potent trigger of arthritis. J. Intern. Med. 255, 344–350. doi: 10.1111/j.1365-2796.2003.01303.x
Asimakopoulos, G., Smith, P. L., Ratnatunga, C. P., Taylor, K. M. (1999). Lung injury and acute respiratory distress syndrome after cardiopulmonary bypass. Ann. Thorac. Surg. 68, 1107–1115. doi: 10.1016/S0003-4975(99)00781-X
Bao, S., Zou, Y., Wang, B., Li, Y., Zhu, J., Luo, Y. (2015). Ginsenoside Rg1 improves lipopolysaccharide-induced acute lung injury by inhibiting inflammatory responses and modulating infiltration of M2 macrophages. Int. Immunopharmacol. 28, 429–434. doi: 10.1016/j.intimp.2015.06.022
Becker, S., Soukup, J. M., Gilmour, M. I., Devlin, R. B. (1996). Stimulation of human and rat alveolar macrophages by urban air particulates: effects on oxidant radical generation and cytokine production. Toxicol. Appl. Pharmacol. 141, 637–648. doi: 10.1006/taap.1996.0330
Bell, C. W., Jiang, W., Reich, C.F., III., and Pisetsky, D. S. (2006). The extracellular release of HMGB1 during apoptotic cell death. Am. J. Physiol. Cell Physiol. 291, C1318–C1325. doi: 10.1152/ajpcell.00616.2005
Brook, R. D., Rajagopalan, S., Pope, C.A., III, Brook, J., Bhatnagar, A., Diez-Roux, A. V. (2010). Particulate matter air pollution and cardiovascular disease: an update to the scientific statement from the American Heart Association. Circ. 121, 2331–2378. doi: 10.1161/CIR.0b013e3181dbece1
Chen, L., Teng, H., Fang, T., Xiao, J. (2016). Agrimonolide from Agrimonia pilosa suppresses inflammatory responses through down-regulation of COX-2/iNOS and inactivation of NF-κB in lipopolysaccharide-stimulated macrophages. Phytomedicine 23, 846–855. doi: 10.1016/j.phymed.2016.03.016
Chen, L., Teng, H., Jia, Z., Battino, M., Mirron, A., Yu, Z. (2018). Intracellular signaling pathways of inflammation modulated by dietary flavonoids: The most recent evidence. Crit. Rev. Food Sci. Nutr. 58, 2908–2924. doi:10.1080/10408398.2017.1345853
Chen, L., Gnanaraj, C., Arulselvan, P., El-Seedi, H., Teng, H. (2019). A review on advanced microencapsulation technology to enhance bioavailability of phenolic compounds: Based on its activity in the treatment of Type 2 Diabetes. Trends Food Sci. Technol. 85, 149–162. doi: 10.1016/j.tifs.2018.11.026
Claudio, E., Segade, F., Wrobel, K., Ramos, S., Lazo, P. S. (1995). Activation of murine macrophages by silica particles in vitro is a process independent of silica-induced cell death. Am. J. Respir. Cell Mol. Biol. 13, 547–554. doi: 10.1165/ajrcmb.13.5.7576690
Clifford, S., Mazaheri, M., Salimi, F., Ezz, W. N., Yeganeh, B., Low-Choy, S. (2018). Effects of exposure to ambient ultrafine particles on respiratory health and systemic inflammation in children. Environ. Int. 114, 167–180. doi: 10.1016/j.envint.2018.02.019
Dambach, D. M., Watson, L. M., Gray, K. R., Durham, S. K., Laskin, D. L. (2002). Role of CCR2 in macrophage migration into the liver during acetaminophen-induced hepatotoxicity in the mouse. Hepatology 35, 1093–1103. doi: 10.1053/jhep.2002.33162
Duan, D. D., Wang, Z., Zhang, B., Wang, Y. (2015). Fangjiomics: revealing adaptive omics pharmacological mechanisms of the myriad combination therapies to achieve personalized medicine. Acta Pharmacol. Sin. 36, 651–653. doi: 10.1038/aps.2015.33
Dysart, M. M., Galvis, B. R., Russell, A. G., Barker, T. H. (2014). Environmental particulate (PM2.5) augments stiffness-induced alveolar epithelial cell mechanoactivation of transforming growth factor beta. PloS One 9, e106821. doi: 10.1371/journal.pone.0106821
Englert, N. (2004). Fine particles and human health—a review of epidemiological studies. Toxicol. Lett. 149, 235–242. doi: 10.1016/j.toxlet.2003.12.035
Erridge, C. (2010). Endogenous ligands of TLR2 and TLR4: agonists or assistants?. J. Leukoc. Biol. 87, 989–999. doi: 10.1189/jlb.1209775
Feng, G., Jiang, Z. Y., Sun, B., Fu, J., Li, T. Z. (2016). Fisetin alleviates lipopolysaccharide-induced acute lung injury via TLR4-mediated NF-κB signaling pathway in rats. Inflammation 39, 148–157. doi: 10.1007/s10753-015-0233-y
Gan, P., Zhong, M., Huang, X., Sun, M., Wang, Y., Xiao, Y. (2012). Pharmacokinetic comparisons of albiflorin and paeoniflorin after oral administration of Shaoyao-Gancao-Tang and single herb Paeony decoction to rats. Planta Med. 78, 237–243. doi: 10.1055/s-0031-1280366
Gao, L., Wang, J., Li, F., Gao, S., Deng, Y. (2012). Analysis on clinically drug-used law for lung-intestine related diseases. J. Tradit. Chin. Med. 32, 523–528. doi: 10.1016/S0254-6272(13)60064-3
Ghio, A. J., Devlin, R. B. (2001). Inflammatory lung injury after bronchial instillation of air pollution particles. Am. J. Respir. Crit. Care Med. 164, 704–708. doi: 10.1164/ajrccm.164.4.2011089
Goodman, R. B., Pugin, J., Lee, J. S., Matthay, M. A. (2003). Cytokine-mediated inflammation in acute lung injury. Cytokine Growth Factor Rev. 14, 523–535. doi: 10.1016/S1359-6101(03)00059-5
Grattendick, K., Stuart, R., Roberts, E., Lincoln, J., Lefkowitz, S. S., Bollen, A. (2002). Alveolar macrophage activation by myeloperoxidase: a model for exacerbation of lung inflammation. Am. J. Respir. Cell Mol. Biol. 26, 716–722. doi: 10.1165/ajrcmb.26.6.4723
Gupta, S., Khajuria, V., Wani, A., Nalli, Y., Bhagat, A., Ali, A. (2019). Murrayanine attenuates lipopolysaccharide-induced inflammation and protects mice from sepsis-associated organ failure. Basic Clin. Pharmacol. Toxicol. 124, 351–359. doi: 10.1111/bcpt.13032
Han, L., Zhang, M., Wang, M., Jia, J., Zhao, M., Fan, Y. (2016). High mobility group box-1 promotes inflammation-induced lymphangiogenesis via Toll-like receptor 4-dependent signalling pathway. PloS One 11, e0154187. doi: 10.1371/journal.pone.0154187
Herold, S., Mayer, K., Lohmeyer, J. (2011). Acute lung injury: How macrophages orchestrate resolution of inflammation and tissue repair. Front. Immunol. 2, 65. doi: 10.3389/fimmu.2011.00065
Hetland, R. B., Cassee, F. R., Låg, M., Refsnes, M., Dybing, E., Schwarze, P. E. (2005). Cytokine release from alveolar macrophages exposed to ambient particulate matter: heterogeneity in relation to size, city and season. Part. Fibre. Toxicol. 2, 4. doi: 10.1186/1743-8977-2-4
Higashisaka, K., Fujimura, M., Taira, M., Yoshida, T., Tsunoda, S.-I., Baba, T. (2014). Asian dust particles induce macrophage inflammatory responses via mitogen-activated protein kinase activation and reactive oxygen species production. J. Immunol. Res. 2014, 856154. doi: 10.1155/2014/856154
Huang, R. J., Zhang, Y., Bozzetti, C., Ho, K. F., Cao, J. J., Han, Y. (2014). High secondary aerosol contribution to particulate pollution during haze events in China. Nat. 514, 218–222. doi: 10.1038/nature13774
Islam, A. S., Beidelschies, M. A., Huml, A., Greenfield, E. M. (2011). Titanium particles activate toll-like receptor 4 independently of lipid rafts in RAW264. 7 murine macrophages. J. Orthop. Res. 29, 211–217. doi: 10.1002/jor.21199
Iwasaki, Y., Otsuka, H., Yanagisawa, N., Hisamitsu, H., Manabe, A., Nonaka, N. (2011). In situ proliferation and differentiation of macrophages in dental pulp. Cell Tissue Res. 346, 99–109. doi: 10.1007/s00441-011-1231-5
Jacobson, J. R., Barnard, J. W., Grigoryev, D. N., Ma, S. F., Tuder, R. M., Garcia, J. G. (2005). Simvastatin attenuates vascular leak and inflammation in murine inflammatory lung injury. Am. J. Physiol. Lung Cell Mol. Physiol. 288, L1026–L1032. doi: 10.1152/ajplung.00354.2004
Jiang, Q., Yi, M., Guo, Q., Wang, C., Wang, H., Meng, S. (2015). Protective effects of polydatin on lipopolysaccharide-induced acute lung injury through TLR4-MyD88-NF-κB pathway. Int. Immunopharmacol. 29, 370–376. doi: 10.1016/j.intimp.2015.10.027
Jing, Y., Zhang, H., Cai, Z., Zhao, Y., Wu, Y., Zheng, X. (2017). Bufei huoxue capsule attenuates PM2.5-induced pulmonary inflammation in mice. Evid. Based Complement Alternat. Med. 2017, 1575793.doi:10.1155/2017/1575793.
Kallapur, S. G., Jobe, A. H. (2006). Contribution of inflammation to lung injury and development. Arch. Dis. Child. Fetal Neonatal Ed. 91, F132–F135. doi: 10.1136/adc.2004.068544
Kasahara, Y., Hikino, H., Tsurufuji, S., Watanabe, M., Ohuchi, K. (1985). Antiinflammatory actions of ephedrines in acute inflammations. Planta Med. 51, 325–331. doi: 10.1055/s-2007-969503
Kay, E., Scotland, R. S., Whiteford, J. R. (2014). Toll-like receptors: Role in inflammation and therapeutic potential. Biofactors 40, 284–294. doi: 10.1002/biof.1156
Kleindienst, T. E., Lewandowski, M., Offenberg, J. H., Edney, E. O., Jaoui, M., Zheng, M. (2010). Contribution of primary and secondary sources to organic aerosol and PM2. 5 at SEARCH network sites. J. Air Waste Manage. Assoc. 60, 1388–1399. doi: 10.3155/1047-3289.60.11.1388
Kokkola, R., Andersson, A., Mullins, G., Treutiger, C. J., Arnold, B. (2005). RAGE is the major receptor for the proinflammatory activity of HMGB1 in rodent macrophages. Scand. J. Immunol. 61, 1–9. doi: 10.1111/j.0300-9475.2005.01534.x
Koksoy, C., Kuzu, M. A., Kuzu, I., Ergun, H., Gurhan, I. (2001). Role of tumour necrosis factor in lung injury caused by intestinal ischaemia-reperfusion. Br. J. Surg. 88, 464–468. doi: 10.1046/j.1365-2168.2001.01737.x
Lau, A., Wang, S., Liu, W., Haig, A., Zhang, Z. X., Jevnikar, A. M. (2014). Glycyrrhizic acid ameliorates HMGB1-mediated cell death and inflammation after renal ischemia reperfusion injury. Am. J. Nephrol. 40, 84–95. doi: 10.1159/000364908
Lawrence, T. (2009). The nuclear factor NF-κB pathway in inflammation. Cold Spring Harb. Perspect. Biol. 1, a001651. doi: 10.1101/cshperspect.a001651
Leenen, P. J., De Bruijn, M. F., Voerman, J. S., Campbell, P. A., Van Ewijk, W. (1994). Markers of mouse macrophage development detected by monoclonal antibodies. J. Immunol. Methods 174, 5–19. doi: 10.1016/0022-1759(94)90005-1
Leiva G, M. A.Santibañez, D. A. , Ibarra E, S., Matus C, P., and Seguel, R. (2013). A five-year study of particulate matter (PM2.5) and cerebrovascular diseases. Environ. Pollut. 181, 1–6. doi: 10.1016/j.envpol.2013.05.057
Li, R., Mittelstein, D., Kam, W., Pakbin, P., Du, Y., Tintut, Y. (2013). Atmospheric ultrafine particles promote vascular calcification via the NF-kappaB signaling pathway. Am. J. Physiol. Cell Physiol. 304, C362–C369. doi: 10.1152/ajpcell.00322.2012
Liao, Y. N., Hu, W. L., Chen, H. J., Hung, Y. C. (2017). The use of Chinese herbal medicine in the treatment of chronic obstructive pulmonary disease (COPD). Am. J. Chin. Med. 45, 225–238. doi: 10.1142/S0192415X17500148
Lin, W. C., Lin, J. Y. (2011). Five bitter compounds display different anti-inflammatory effects through modulating cytokine secretion using mouse primary splenocytes in vitro. J. Agric. Food Chem. 59, 184–192. doi: 10.1021/jf103581r
Lin, J., Cheng, Y., Wang, T., Tang, L., Sun, Y., Lu, X. (2016). Soyasaponin Ab inhibits lipopolysaccharide-induced acute lung injury in mice. Int. Immunopharmacol. 30, 121–128. doi: 10.1016/j.intimp.2015.12.001
Liu, Z., Yang, Z., Fu, Y., Li, F., Liang, D., Zhou, E. (2013). Protective effect of gossypol on lipopolysaccharide-induced acute lung injury in mice. Inflamm. Res. 62, 499–506. doi: 10.1007/s00011-013-0603-6
Liu, M. H., Lin, A. H., Lee, H. F., Ko, H. K., Lee, T. S., Kou, Y. R. (2014). Paeonol attenuates cigarette smoke-induced lung inflammation by inhibiting ROS-sensitive inflammatory signaling. Mediators Inflamm. 2014, 651890. doi: 10.1155/2014/651890
Liu, Z., Wang, W., Cao, F., Liu, S., Zou, X., Li, G. (2018). Number 2 Feibi recipe reduces PM2.5-induced lung injury in rats. Evid. Based Complement Alternat. Med. 2018, 3674145. doi: 10.1155/2018/3674145
Lloyd, C. M., Phillips, A. R., Cooper, G. J., Dunbar, P. R. (2008). Three-colour fluorescence immunohistochemistry reveals the diversity of cells staining for macrophage markers in murine spleen and liver. J. Immunol. Methods 334, 70–81. doi: 10.1016/j.jim.2008.02.005
Lu, F.-G., Tan, L., Li, L., Yang, S.-H., He, Y.-C., Cao, J.-X. (2012). Effect of Maxing Shigan Decoction to inflammation and ultrastructure in lungs of mice infected by influenza virus. China J. Mod. Med. 22, 5–8. doi: 10.3969/j.issn.1005-8982.2012.08.002
Lukacs, N. W., Strieter, R. M., Kunkel, S. L. (1995). Leukocyte infiltration in allergic airway inflammation. Am. J. Respir. Cell Mol. Biol. 13, 1–6. doi: 10.1165/ajrcmb.13.1.7598934
Lv, B., Wang, H., Tang, Y., Fan, Z., Xiao, X., Chen, F. (2009). High-mobility group box 1 protein induces tissue factor expression in vascular endothelial cells via activation of NF-kappaB and Egr-1. Thromb. Haemost. 102, 352–359. doi: 10.1160/th08-11-0759
Lv, H., Zhu, C., Liao, Y., Gao, Y., Lu, G., Zhong, W. (2015). Tenuigenin ameliorates acute lung injury by inhibiting NF-kappaB and MAPK signalling pathways. Respir. Physiol. Neurobiol. 216, 43–51. doi: 10.1016/j.resp.2015.04.010
Lv, W., Chen, N., Lin, Y., Ma, H., Ruan, Y., Li, Z. (2016). Macrophage migration inhibitory factor promotes breast cancer metastasis via activation of HMGB1/TLR4/NF kappa B axis. Cancer Lett. 375, 245–255. doi: 10.1016/j.canlet.2016.02.005
Magalhães, C. B., Casquilho, N. V., Machado, M. N., Riva, D. R., Travassos, L. H., Leal-Cardoso, J. H. (2019). The anti-inflammatory and anti-oxidative actions of eugenol improve lipopolysaccharide-induced lung injury. Respir. Physiol. Neurobiol. 259, 30–36. doi: 10.1016/j.resp.2018.07.001
Martínez-Cinco, M., Santos-Guzmán, J., Mejía-Velázquez, G. (2016). Source apportionment of PM2.5 for supporting control strategies in the Monterrey Metropolitan Area, Mexico. J. Air Waste Manage. Assoc. 66, 631–642. doi: 10.1080/10962247.2016.1159259
Mcguinn, L. A., Schneider, A., Mcgarrah, R. W., Ward-Caviness, C., Neas, L. M., Di, Q. (2019). Association of long-term PM2.5 exposure with traditional and novel lipid measures related to cardiovascular disease risk. Environ. Int. 122, 193–200. doi: 10.1016/j.envint.2018.11.001
Mei, F., Xing, X. F., Tang, Q. F., Chen, F. L., Guo, Y., Song, S. (2016). Antipyretic and anti-asthmatic activities of traditional Chinese herb-pairs, Ephedra and Gypsum. Chin. J. Integr. Med. 22, 445–450. doi: 10.1007/s11655-014-1952-x
Menezes, S. L., Bozza, P. T., Faria Neto, H. C. C., Laranjeira, A. P., Negri, E. M., Capelozzi, V. L. (2005). Pulmonary and extrapulmonary acute lung injury: inflammatory and ultrastructural analyses. J. Appl. Physiol. 98, 1777–1783. doi: 10.1152/japplphysiol.01182.2004
Meng, Z., Zhang, Q. (2007). Damage effects of dust storm PM2.5 on DNA in alveolar macrophages and lung cells of rats. Food Chem. Toxicol. 45, 1368–1374. doi: 10.1016/j.fct.2007.01.014
Ming, L. J., Yin, A. C. (2013). Therapeutic effects of glycyrrhizic acid. Nat. Prod. Commun. 8, 415–418. doi: 10.1016/j.fitote.2012.09.002
Muhammad, S., Barakat, W., Stoyanov, S., Murikinati, S., Yang, H., Tracey, K. J. (2008). The HMGB1 receptor RAGE mediates ischemic brain damage. J. Neurosci. 28, 12023–12031. doi: 10.1523/JNEUROSCI.2435-08.2008
Nair, A. B., Jacob, S. (2016). A simple practice guide for dose conversion between animals and human. J. Basic Clin. Pharm. 7, 27–31. doi: 10.4103/0976-0105.177703
Nhung, N. T. T., Schindler, C., Dien, T. M., Probst-Hensch, N., Perez, L., Künzli, N. (2018). Acute effects of ambient air pollution on lower respiratory infections in Hanoi children: an eight-year time series study. Environ. Int. 110, 139–148. doi: 10.1016/j.envint.2017.10.024
Ning, Y., Imrich, A., Goldsmith, C. A., Qin, G., Kobzik, L. (2000). Alveolar macrophage cytokine production in response to air particles in vitro: role of endotoxin. J. Toxicol. Environ. Health Part A 59, 165–180. doi: 10.1080/009841000156952
Pandolfi, F., Altamura, S., Frosali, S., Conti, P. (2016). Key role of DAMP in inflammation, cancer, and tissue repair. Clin. Ther. 38, 1017–1028. doi: 10.1016/j.clinthera.2016.02.028
Pang, H., Huang, T., Song, J. (2016). Inhibiting HMGB1 with glycyrrhizic acid protects brain injury after DAI via its anti-inflammatory effect. Mediators Inflamm. 2016, 4569521. doi: 10.1155/2016/4569521
Patel, B. V., Wilson, M. R., O'dea, K. P., Takata, M. (2013). TNF-induced death signaling triggers alveolar epithelial dysfunction in acute lung injury. J. Immunol. 190, 4274–4282. doi: 10.4049/jimmunol.1202437
Pedroza, M., Schneider, D. J., Karmouty-Quintana, H., Coote, J., Shaw, S., Corrigan, R. (2011). Interleukin-6 contributes to inflammation and remodeling in a model of adenosine mediated lung injury. PloS One 6, e22667. doi: 10.1371/journal.pone.0022667
Peng, X., Hassoun, P. M., Sammani, S., Mcverry, B. J., Burne, M. J., Rabb, H. (2004). Protective effects of sphingosine 1-phosphate in murine endotoxin-induced inflammatory lung injury. Am. J. Respir. Crit. Care Med. 169, 1245–1251. doi: 10.1164/rccm.200309-1258OC
Pipal, A. S., Jan, R., Satsangi, P. G., Tiwari, S., Taneja, A. (2014). Study of surface morphology, elemental composition and origin of atmospheric aerosols (PM2.5 and PM10) over Agra, India. Aerosol Air Qual. Res. 14, 1685–1700. doi: 10.4209/aaqr.2014.01.0017
Polichetti, G., Cocco, S., Spinali, A., Trimarco, V., Nunziata, A. (2009). Effects of particulate matter (PM10, PM2.5 and PM1) on the cardiovascular system. Toxicol. 261, 1–8. doi: 10.1016/j.tox.2009.04.035
Pope, C., III, Cohen, A. J., Burnett, R. T. (2018). Cardiovascular disease and fine particulate matter: lessons and limitations of an integrated exposure–response approach. Circ. Res. 122, 1645–1647. doi: 10.1161/CIRCRESAHA.118.312956
Quay, J. L., Reed, W., Samet, J., Devlin, R. B. (1998). Air pollution particles induce IL-6 gene expression in human airway epithelial cells via NF-kappaB activation. Am. J. Respir. Cell Mol. Biol. 19, 98–106. doi: 10.1165/ajrcmb.19.1.3132
Querol, X., Alastuey, A., Ruiz, C. R., Artinano, B., Hansson, H. C., Harrison, R. M. (2004). Speciation and origin of PM10 and PM2.5 in selected European cities. Atmos. Environ. 38, 6547–6555. doi: 10.1016/j.atmosenv.2004.08.037
Ricard, J.-D., Dreyfuss, D., Saumon, G. (2001). Production of inflammatory cytokines in ventilator-induced lung injury: a reappraisal. Am. J. Respir. Crit. Care Med. 163, 1176–1180. doi: 10.1164/ajrccm.163.5.2006053
Rovere-Querini, P., Capobianco, A., Scaffidi, P., Valentinis, B., Catalanotti, F., Giazzon, M. (2004). HMGB1 is an endogenous immune adjuvant released by necrotic cells. EMBO Rep. 5, 825–830. doi: 10.1038/sj.embor.7400205
Saito, F., Tasaka, S., Inoue, K., Miyamoto, K., Nakano, Y., Ogawa, Y. (2008). Role of interleukin-6 in bleomycin-induced lung inflammatory changes in mice. Am. J. Respir. Cell Mol. Biol. 38, 566–571. doi: 10.1165/rcmb.2007-0299OC
Saudagar, R. B., Saokar, S. (2019). Anti-inflammatory natural compounds from herbal and marine origin. J. Drug Delivery Ther. 9, 669–672. doi: 10.22270/jddt.v9i3.2906
Scaffidi, P., Misteli, T., Bianchi, M. E. (2002). Release of chromatin protein HMGB1 by necrotic cells triggers inflammation. Nat. 418, 191–195. doi: 10.1038/nature00858
Schierbeck, H., Lundbäck, P., Palmblad, K., Klevenvall, L., Erlandsson-Harris, H., Andersson, U. (2011). Monoclonal anti-HMGB1 (high mobility group box chromosomal protein 1) antibody protection in two experimental arthritis models. Mol. Med. 17, 1039–1044. doi: 10.2119/molmed.2010.00264
Schneider, A., Neas, L. M., Graff, D. W., Herbst, M. C., Cascio, W. E., Schmitt, M. T. (2010). Association of cardiac and vascular changes with ambient PM2.5 in diabetic individuals. Part. Fibre Toxicol. 7, 14. doi: 10.1186/1743-8977-7-14
Shin, I. S., Ahn, K. S., Shin, N. R., Lee, H. J., Ryu, H. W., Kim, J. W. (2016). Protective effect of EC-18, a synthetic monoacetyldiglyceride on lung inflammation in a murine model induced by cigarette smoke and lipopolysaccharide. Int. Immunopharmacol. 30, 62–68. doi: 10.1016/j.intimp.2015.11.025
Shoenfelt, J., Mitkus, R. J., Zeisler, R., Spatz, R. O., Powell, J., Fenton, M. J. (2009). Involvement of TLR2 and TLR4 in inflammatory immune responses induced by fine and coarse ambient air particulate matter. J. Leukoc. Biol. 86, 303–312. doi: 10.1189/jlb.1008587
Silva, F. S., Cristale, J., André, P. A., Saldiva, P. H. N., Marchi, M. R. R. (2010). PM2.5 and PM10: the influence of sugarcane burning on potential cancer risk. Atmos. Environ. 44, 5133–5138. doi: 10.1016/j.atmosenv.2010.09.001
Sims, G. P., Rowe, D. C., Rietdijk, S. T., Herbst, R., Coyle, A. J. (2009). HMGB1 and RAGE in inflammation and cancer. Annu. Rev. Immunol. 28, 367–388. doi: 10.1146/annurev.immunol.021908.132603
Smith, R. E., Strieter, R. M., Phan, S. H., Lukacs, N., Kunkel, S. L. (1998). TNF and IL-6 mediate MIP-1alpha expression in bleomycin-induced lung injury. J. Leukoc. Biol. 64, 528–536. doi: 10.1002/jlb.64.4.528
Su, X.-D., Jang, H.-J., Wang, C.-Y., Lee, S. W., Rho, M.-C., Kim, Y. H. (2019). Anti-inflammatory potential of saponins from Aster tataricus via NF-κB/MAPK activation. J. Nat. Prod. 82, 1139–1148. doi: 10.1021/acs.jnatprod.8b00856
Sun, J., Shin, S., Wang, Q., Yu, K., Wang, R. (2015). Continuous hemodiafiltration therapy reduces damage of multi-organs by ameliorating of HMGB1/TLR4/NFκB in a dog sepsis model. Int. J. Clin. Exp. Pathol. 8, 1555–1564. doi: PMID: 25973040
Tang, T., Zhang, J., Yin, J., Staszkiewicz, J., Gawronska-Kozak, B., Jung, D. Y. (2009). Uncoupling of inflammation and insulin resistance by NF-κB in transgenic mice through elevated energy expenditure. J. Biol. Chem. 285, 4637–4644. doi: 10.1074/jbc.M109.068007
Tang, Y., Chen, Y., Chu, Z., Yan, B., Xu, L. (2014). Protective effect of cryptotanshinone on lipopolysaccharide-induced acute lung injury in mice. Eur. J. Pharmacol. 723, 494–500. doi: 10.1016/j.ejphar.2013.10.019
Tang, Z. H., Li, T., Tong, Y. G., Chen, X. J., Chen, X. P., Wang, Y. T. (2015). A systematic review of the anticancer properties of compounds isolated from Licorice (Gancao). Planta Med. 81, 1670–1687. doi: 10.1055/s-0035-1558227
Tang, W., Huang, S., Du, L., Sun, W., Yu, Z., Zhou, Y. (2018). Expression of HMGB1 in maternal exposure to fine particulate air pollution induces lung injury in rat offspring assessed with micro-CT. Chem. Biol. Interact. 280, 64–69. doi: 10.1016/j.cbi.2017.12.016
Tecer, L. H., Alagha, O., Karaca, F., Tuncel, G., Eldes, N. (2008). Particulate matter (PM2.5, PM10-2.5, and PM10) and children's hospital admissions for asthma and respiratory diseases: A bidirectional case-crossover study. J. Toxicol. Environ. Health Part A 71, 512–520. doi: 10.1080/15287390801907459
Teng, H., Huang, Q., Chen, L. (2016). Inhibition of cell proliferation and triggering of apoptosis by agrimonolide through MAP kinase (ERK and p38) pathways in human gastric cancer AGS cells. Food Funct. 7, 4605–4613. doi: 10.1039/C6FO00715E
Teng, H., Fang, T., Lin, Q., Song, H., Liu, B., Chen, L. (2017). Red raspberry and its anthocyanins: Bioactivity beyond antioxidant capacity. Trends Food Sci. Technol. 66, 153–165. doi: 10.1016/j.tifs.2017.05.015
Tolle, L. B., Standiford, T. J. (2013). Danger-associated molecular patterns (DAMPs) in acute lung injury. J. Pathol. 229, 145–156. doi: 10.1002/path.4124
Vineis, P., Husgafvel-Pursiainen, K. (2005). Air pollution and cancer: biomarker studies in human populations. Carcinogenesis 26, 1846–1855. doi: 10.1093/carcin/bgi216
Voronov, I., Santerre, J., Hinek, A., Callahan, J., Sandhu, J., Boynton, E. (1998). Macrophage phagocytosis of polyethylene particulate in vitro. J. Biomed. Mater. Res. 39, 40–51. doi: 10.1002/(SICI)1097-4636(199801)39:1<40::AID-JBM6>3.0.CO;2-I
Wang, Y.-M., Cheng, H.-J., Zhu, X.-M. (2012). In vitro effect of MXSGD against Pseudomonas aeruginosa biofilms. Lishizhen Med. Mater. Med. Res. 23, 2961–2963. doi: 10.3969/j.issn.1008-0805.2012.12.006
Wang, D. Z., Liu, D. J., Cheng, D. Y., Wang, M. Y. (2013). Fangjiomics: In search of effective and safe combination therapies. J. Clin. Pharmacol. 51, 1132–1151. doi: 10.1177/0091270010382913
Wei, P. F., Ho, K. Y., Ho, Y. P., Wu, Y. M., Yang, Y. H., Tsai, C. C. (2004). The investigation of glutathione peroxidase, lactoferrin, myeloperoxidase and interleukin-1β in gingival crevicular fluid: implications for oxidative stress in human periodontal diseases. J. Periodont. Res. 39, 287–293. doi: 10.1111/j.1600-0765.2004.00744.x
Welbourn, C., Young, Y. (1992). Endotoxin, septic shock and acute lung injury: neutrophils, macrophages and inflammatory mediators. Am. J. Respir. Cell Mol. Biol. 79, 998–1003. doi: 10.1002/bjs.1800791006
Wilson, M. S., Madala, S. K., Ramalingam, T. R., Gochuico, B. R., Rosas, I. O., Cheever, A. W. (2010). Bleomycin and IL-1beta-mediated pulmonary fibrosis is IL-17A dependent. J. Exp. Med. 207, 535–552. doi: 10.1084/jem.20092121
Wright, B., Zeidman, I., Greig, R., Poste, G. (1985). Inhibition of macrophage activation by calcium channel blockers and calmodulin antagonists. Cell. Immunol. 95, 46–53. doi: 10.1016/0008-8749(85)90293-X
Wu, Z., Kong, X., Zhang, T., Ye, J., Fang, Z., Yang, X. (2014). Pseudoephedrine/ephedrine shows potent anti-inflammatory activity against TNF-alpha-mediated acute liver failure induced by lipopolysaccharide/D-galactosamine. Eur. J. Pharmacol. 724, 112–121. doi: 10.1016/j.ejphar.2013.11.032
Xie, Y. C., Dong, X. W., Wu, X. M., Yan, X. F., Xie, Q. M. (2009). Inhibitory effects of flavonoids extracted from licorice on lipopolysaccharide-induced acute pulmonary inflammation in mice. Int. Immunopharmacol. 9, 194–200. doi: 10.1016/j.intimp.2008.11.004
Xing, Y. F., Xu, Y. H., Shi, M. H., Lian, Y. X. (2016). The impact of PM2.5 on the human respiratory system. J. Thorac. Dis. 8, E69–E74. doi: 10.3978/j.issn.2072-1439.2016.01.19
Yang, H., Tracey, K. J. (2010). Targeting HMGB1 in inflammation. Biochim. Biophys. Acta Gene Regul. Mech. 1799, 149–156. doi: 10.1016/j.bbagrm.2009.11.019
Yang, H. Y., Chang, H. K., Lee, J. W., Kim, Y. S., Kim, H., Lee, M. H. (2007). Amygdalin suppresses lipopolysaccharide-induced expressions of cyclooxygenase-2 and inducible nitric oxide synthase in mouse BV2 microglial cells. Neurol. Res. 29 Suppl 1, S59–S64. doi: 10.1179/016164107x172248
Yang, H., Hreggvidsdottir, H. S., Palmblad, K., Wang, H., Ochani, M., Li, J. (2010a). A critical cysteine is required for HMGB1 binding to Toll-like receptor 4 and activation of macrophage cytokine release. Proc. Natl. Acad. Sci. U.S.A. 107, 11942–11947. doi: 10.1073/pnas.1003893107
Yang, Q. W., Xiang, J., Zhou, Y., Zhong, Q., Li, J. C. (2010b). Targeting HMGB1/TLR4 signaling as a novel approach to treatment of cerebral ischemia. Front. Biosci. (Schol. Ed.) 2, 1081–1091. doi: 10.2741/S119
Yang, Y., Wang, J., Yang, Q., Wu, S., Yang, Z., Zhu, H. (2014). Shikonin inhibits the lipopolysaccharide-induced release of HMGB1 in RAW264.7 cells via IFN and NF-kappaB signaling pathways. Int. Immunopharmacol. 19, 81–87. doi: 10.1016/j.intimp.2014.01.003
Yang, Y., Russell, L. M., Lou, S., Liao, H., Guo, J., Liu, Y. (2017). Dust-wind interactions can intensify aerosol pollution over eastern China. Nat. Commun. 8, 15333. doi: 10.1038/ncomms15333
Zhang, Q., Ye, M. (2009). Chemical analysis of the Chinese herbal medicine Gan-Cao (licorice). J. Chromatogr. A 1216, 1954–1969. doi: 10.1016/j.chroma.2008.07.072
Zhang, B., Liu, Z. Y., Li, Y. Y., Luo, Y., Liu, M. L., Dong, H. Y. (2011). Antiinflammatory effects of matrine in LPS-induced acute lung injury in mice. Eur. J. Pharm. Sci. 44, 573–579. doi: 10.1016/j.ejps.2011.09.020
Zhang, P., Jia, C., Deng, Y., Chen, S., Chen, B., Yan, S. (2019). Anti-inflammatory prenylbenzaldehyde derivatives isolated from Eurotium cristatum. Phytochem. 158, 120–125. doi: 10.1016/j.phytochem.2018.11.017
Zheng, Y., Guo, Z., He, W., Yang, Y., Li, Y., Zheng, A. (2012). Ephedrine hydrochloride protects mice from LPS challenge by promoting IL-10 secretion and inhibiting proinflammatory cytokines. Int. Immunopharmacol. 13, 46–53. doi: 10.1016/j.intimp.2012.03.005
Zheng, S., Pozzer, A., Cao, C. X., Lelieveld, J. (2015). Long-term (2001–2012) concentrations of fine particulate matter (PM 2.5) and the impact on human health in Beijing, China. Atmos. Chem. Phys. 15, 5715–5725. doi: 10.5194/acp-15-5715-2015
Keywords: Ma Xing Shi Gan Decoction, PM2.5, lung injury, macrophage, inflammatory reaction, HMGB1/TLR4/NFκB pathway
Citation: Fei Y-x, Zhao B, Yin Q-y, Qiu Y-y, Ren G-h, Wang B-w, Wang Y-f, Fang W-r and Li Y-m (2019) Ma Xing Shi Gan Decoction Attenuates PM2.5 Induced Lung Injury via Inhibiting HMGB1/TLR4/NFκB Signal Pathway in Rat. Front. Pharmacol. 10:1361. doi: 10.3389/fphar.2019.01361
Received: 17 May 2019; Accepted: 28 October 2019;
Published: 14 November 2019.
Edited by:
Aiping Lu, Hong Kong Baptist University, Hong KongReviewed by:
Rajasekaran Subbiah, ICMR-National Institute for Research in Environmental Health, IndiaLei Chen, Fujian Agriculture and Forestry University, China
Huan Yang, Feinstein Institute for Medical Research, United State
Copyright © 2019 Fei, Zhao, Yin, Qiu, Ren, Wang, Wang, Fang and Li. This is an open-access article distributed under the terms of the Creative Commons Attribution License (CC BY). The use, distribution or reproduction in other forums is permitted, provided the original author(s) and the copyright owner(s) are credited and that the original publication in this journal is cited, in accordance with accepted academic practice. No use, distribution or reproduction is permitted which does not comply with these terms.
*Correspondence: Ye-fang Wang, wangyefang@126.com; Wei-rong Fang, weirongfang@163.com; Yun-man Li, yunmanlicpu@163.com