- 1Acesion Pharma, Copenhagen, Denmark
- 2Department of Biomedical Sciences, Faculty of Health and Medical Sciences, University of Copenhagen, Copenhagen, Denmark
- 3Institute of Biological Psychiatry, Sankt Hans Hospital, Roskilde, Denmark
- 4Department of Molecular and Clinical Medicine/Cardiology, Institute of Medicine, Sahlgrenska Academy, University of Gothenburg, Gothenburg, Sweden
Aims: Small conductance Ca2+-activated K+ channels (SK channels, KCa2) are a new target for treatment of atrial fibrillation (AF). AP30663 is a small molecule inhibitor of KCa2 channels that is currently in clinical development for treatment of AF. The aim of this study is to present the electrophysiological profile and mechanism of action of AP30663 and its efficacy in prolonging atrial refractoriness in rodents, and by bioinformatic analysis investigate if genetic variants in KCNN2 or KCNN3 influence the expression level of these in human heart tissue.
Methods and Results: Whole-cell and inside-out patch-clamp recordings of heterologously expressed KCa2 channels revealed that AP30663 inhibits KCa2 channels with minor effects on other relevant cardiac ion channels. AP30663 modulates the KCa2.3 channel by right-shifting the Ca2+-activation curve. In isolated guinea pig hearts AP30663 significantly prolonged the atrial effective refractory period (AERP) with minor effects on the QT-interval corrected for heart rate. Similarly, in anaesthetized rats 5 and 10 mg/kg of AP30663 changed the AERP to 130.7±5.4% and 189.9±18.6 of baseline values. The expression quantitative trait loci analyses revealed that the genome wide association studies for AF SNP rs13376333 in KCNN3 is associated with increased mRNA expression of KCNN3 in human atrial appendage tissue.
Conclusions: AP30663 is a novel negative allosteric modulator of KCa2 channels that concentration-dependently prolonged rodent atrial refractoriness with minor effects on the QT-interval. Moreover, AF associated SNPs in KCNN3 influence KCNN3 mRNA expression in human atrial tissue. These properties support continued development of AP30663 for treatment of AF in man.
Introduction
Atrial fibrillation (AF) is the most common cardiac arrhythmia affecting more than 30 million people worldwide, a number that is rising partly because of the aging population and better detection. AF is associated with 2- and 1.5 fold increases in risk of all-cause mortality in woman and men respectively, and an increased risk of heart failure and stroke (Kirchhof et al., 2016). Management of patients with AF has improved, but pharmacological rhythm control therapy is still limited by moderately effective drugs with potentially serious extra-cardiac or ventricular adverse effects (Waks and Zimetbaum, 2016). Hence, novel pharmacological rhythm control therapies are warranted.
The small conductance calcium activated potassium channel (SK, KCa2), encoded by the KCNNX gene is a new drug target for treatment of AF (Heijman and Dobrev, 2017). As the name implies, KCa2 channels are potassium channels activated by intracellular calcium. Three subtypes of KCa2 channel alfa-subunits exists (KCa2.1–3, SK1–3) and in the human atria KCa2.2 and KCa2.3 predominate (Skibsbye et al., 2014). Genome wide association studies (GWAS) for AF have identified single nucleotide polymorphisms (SNPs) in KCNN2 and KCNN3 that are highly associated with AF (Ellinor et al., 2010; Ellinor et al., 2012; Christophersen et al., 2017). Preclinical studies showed that KCa2 channels during sinus rhythm as well as during AF play a more prominent role in atria as compared to ventricles in several species including man (Tuteja et al., 2005; Li et al., 2009; Diness et al., 2010; Qi et al., 2014; Skibsbye et al., 2014; Haugaard et al., 2015; Diness et al., 2017), thereby exhibiting a functional atrial specificity. In atrially tachy-paced pigs that were resistant to pharmacological cardioversion of AF with vernakalant, negative allosteric modulation of the KCa2 channel converted AF to sinus rhythm (Diness et al., 2017). In the current study, we first investigate if genetic variants (SNPs) in KCNN2 or KCNN3 found in GWAS to be associated with AF influence the expression level of KCNN2 or KCNN3 in human atrial or ventricular tissue. Next, we present the ion channel profile, mode of action, and in vitro, ex vivo and in vivo effects of the clinical candidate AP30663.
Materials and Methods
Expression Quantitative Trait Loci Analyses
The expression quantitative trait loci analyses (eQTL) effects of the AF GWAS associated SNP rs337711 on KCNN2 and SNP rs13376333 on KCNN3 were investigated in the Genotype-Tissue Expression (GTEx) database, release v05-08-15, using default dashboard analytics setup. GTEx data gene expression was measured using RNA-sequencing with Illumina TruSeq library and genotyping was done using whole genome sequencing on an illumina HiSeq X machine (Carithers et al., 2015). For eQTL replication we used the Advanced Study of Aortic Pathology (ASAP) cohort of left-ventricular heart tissue, measured using Affymetrix ST 1.0 Arrays and genotyping was done using Illumina Human 610W-Quad Beadarrays (Folkersen et al., 2011). The statistical calculation was performed using a linear additive model, where genotype was encoded as 0, 1, or 2 and used as explanatory variable, with gene expression level as response variable.
In Vitro Electrophysiology
The potency of AP30663 was assessed in HEK cells stably expressing rat NaV1.5 or human CaV1.2, KCa2.1, KCa2.2 or KCa2.3 channels and CHO cell line stably expressing human KV11.1 using the automated patch-clamp system, Qpatch 16 (Sophion, Ballerup, Denmark) at room temperature. The effect of AP30663 on heteromeric KV11.1a and KV11.1b channels was addressed by manual patch clamping. Inside out patch-clamp recordings were performed on HEK cells stably expressing human KCa2.3 channels using a HEKA EPC9 amplifier and the Patchmaster software (HEKA Elektronik, Germany). Effects of AP30663 on late NaV1.5 currents were addressed by manual whole-cell patch-clamping using HEK cells transiently transfected with human NaV1.5 in the absence and presence of ATXII. The effect of AP30663 was also assessed on the currents conducted by Kir2.1 (IK1); KV7.1/KCNE1 (Iks); KV4.3/KCHIP2 (Ito), Kir3.1/Kir3.4 (IKACh); and KV1.5 (IKur) using the two-electrode voltage-clamp method on Xenopus oocytes, as previously described (Diness et al.). See details of in vitro electrophysiology methods in Supplementary Methods and Materials.
Animal Experiments
Animal experiments were performed under the license from the Danish Ministry of justice (2018-15-0201-01430 & 2016-15-0201-00850) and in accordance with the Danish guidelines for animal experiments according to the European Commission Directive 86/609/EEC.
Isolated Perfused Guinea Pig Heart Experiments
A total of 12 female and 6 male Guinea pig hearts were used. In brief after anesthesia the hearts were removed and retrogradely perfused with Krebs–Henseleit solution via the aorta. Hearts were mounted in a Langendorff set-up (Hugo Sachs Elektronik, Germany) and electrocardiograms (ECG) were obtained with three ECG electrodes placed near the heart. A pacing electrode on the right atrium was used to stimulate the atria and measure atrial refractory periods (AERP).
After the baseline recording, three 20-min episodes followed in which the heart was perfused with increasing concentrations of AP30663 (1, 3, and 10 µM). Time matched control hearts underwent the same procedure. For details see Supplementary Methods.
Irwin
An observational Irwin test for assessing CNS exposure of AP30663 was performed in mice. A total of three NMRI mice (Taconic Europe, Lille Skensved, Denmark) weighing 40 to 60 g were used. After injection of a bolus dose of 10 mg/kg AP30663 in the tail vein, the mice were observed for up to 30 min.
Closed Chest Rat AERP
A total of seven male Sprague–Dawley rats were used for the closed chest preparations. The rats were anaesthetized with 3 % isofluran/oxygen and catheterized to allow for drug infusion and intra cardiac pacing. The AERP was measured by applying electrical stimulation. Baseline AERP recordings were made every fifth minute for 20 min. Hereafter, two 20-min episodes followed in which the animal was injected with increasing doses of AP30663 (5 and 10 mg/kg) or equivalent volumes of vehicle. AERP was measured 0.5, 2, 4, 6, 10, and 15 min after each injection. For details see Supplementary Methods.
Drugs and Solutions
AP30663 was solubilized in DMSO stock concentrations of 10 mM for in vitro experiments. The highest concentration of DMSO was 0.1%. For in vivo experiments, 5 mg/ml AP30663 were dissolved in a vehicle consisting of 50% polyethylene glycol (PEG) 400 (Merck, Germany) and 50% sterile saline (PanReac AppliChem, Germany).
Data Analysis
In vitro data were extracted from PatchMaster or Sophion QPatch Assay Software and analyzed using GraphPad Prism 7. For detailed description, see Supplementary Methods. Data are summarized as mean ± SEM.
In vivo and ex vivo experiments were analyzed using GraphPad Prism software. LabChart software was used for analyzing the following ECG parameters: QT, PR, RR, QRS. The QT-interval corrected for heart rate, QTcH, was calculated as described in Holzgrefe et al. (2014) using the guinea pig specific formula:
Continuous data are summarized using the mean ± SEM. Multiple unpaired t-tests without assuming consistent standard deviations and using Holm-Sidaks correction for multiple comparisons were used to compare the AP30663 groups to their respective TMC. P values < 0.05 were considered significant and are given with three decimals. Manuscript Formatting.
Results
AF-Associated SNPs in KCNN3 Influences KCNN3 mRNA Expression in Human Atrial Tissue
We found that the minor allele T of SNP rs13376333 was associated with higher mRNA expression of KCNN3 in the GTEx atrial appendage samples (Figure 1) (P = 0.0223, n = 264). In the one other GTEx heart-related tissue available, the left ventricle, there was also a trend towards increased expression with the minor allele A, although not statistically significant (P = 0.0818, n = 272). Additionally, we observed the same effect in the lung tissue (P = 2.13e-8) and in one brain region tissue (the BA24 region, P=0.0162). The cardiac eQTL effect was replicated in independent samples of left ventricular biopsies from patients undergoing surgery in the ASAP study, also with the minor allele T resulting in increased expression (P=0.0162, n= 127). In comparison SNP rs337711 was not associated with atrial or ventricular changes in KCCN2 mRNA expression (P = 0.6 and P = 0.1, respectively).
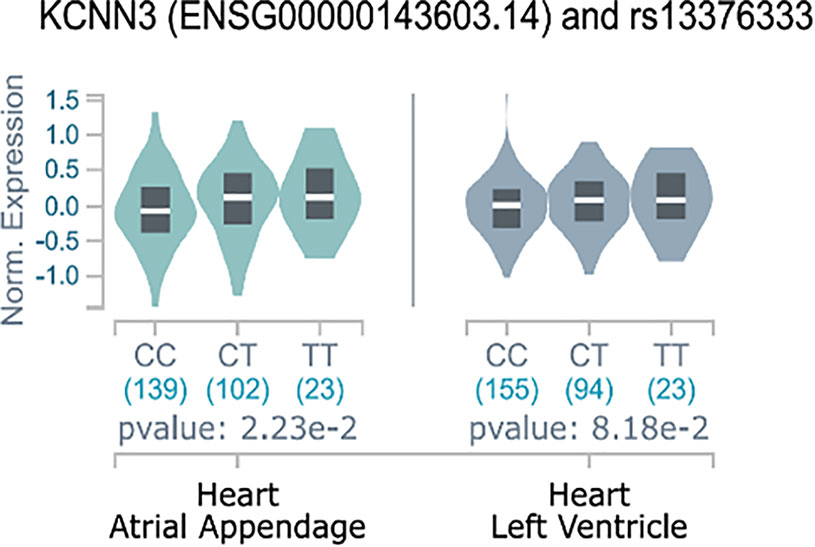
Figure 1 eQTL effects of rs13376333 on KCNN3. Y-axis indicates normalized expression value of KCNN3 according to the GTEx standard analysis pipeline (Carithers et al., 2015). The figure shows that the expression of KCNN3 in atrial appendage is increased in the 23 GTEx individuals with the rs13376333-T/T genotype, as compared to both the 102 heterozygote (C/T) GTEx individuals and the 139 individuals that are major allele homozygote (C/C). Having the T-allele have also been shown to increase risk of AF (Ellinor et al., 2010; Ellinor et al., 2012; Christophersen et al., 2017). These findings were replicated in an independent eQTL data (P = 0.0162, n = 127).
AP30663 Inhibits KCa2 Channels by Modifying the Calcium Sensitivity of the Channel
AP30663 inhibited the KCa2 current in a concentration-dependent manner (Figures 2A–C). The concentration response curves show that AP30663 inhibits all KCa2 channel subtypes but with a slightly lower potency on KCa2.1 (IC50= 2.29 ± 0.22 µM; KCa2.2, IC50 =1.46 ± 0.28 µM; KCa2.3, IC50= 1.09 ± 0.09 µM) (see Figures 2D, E).
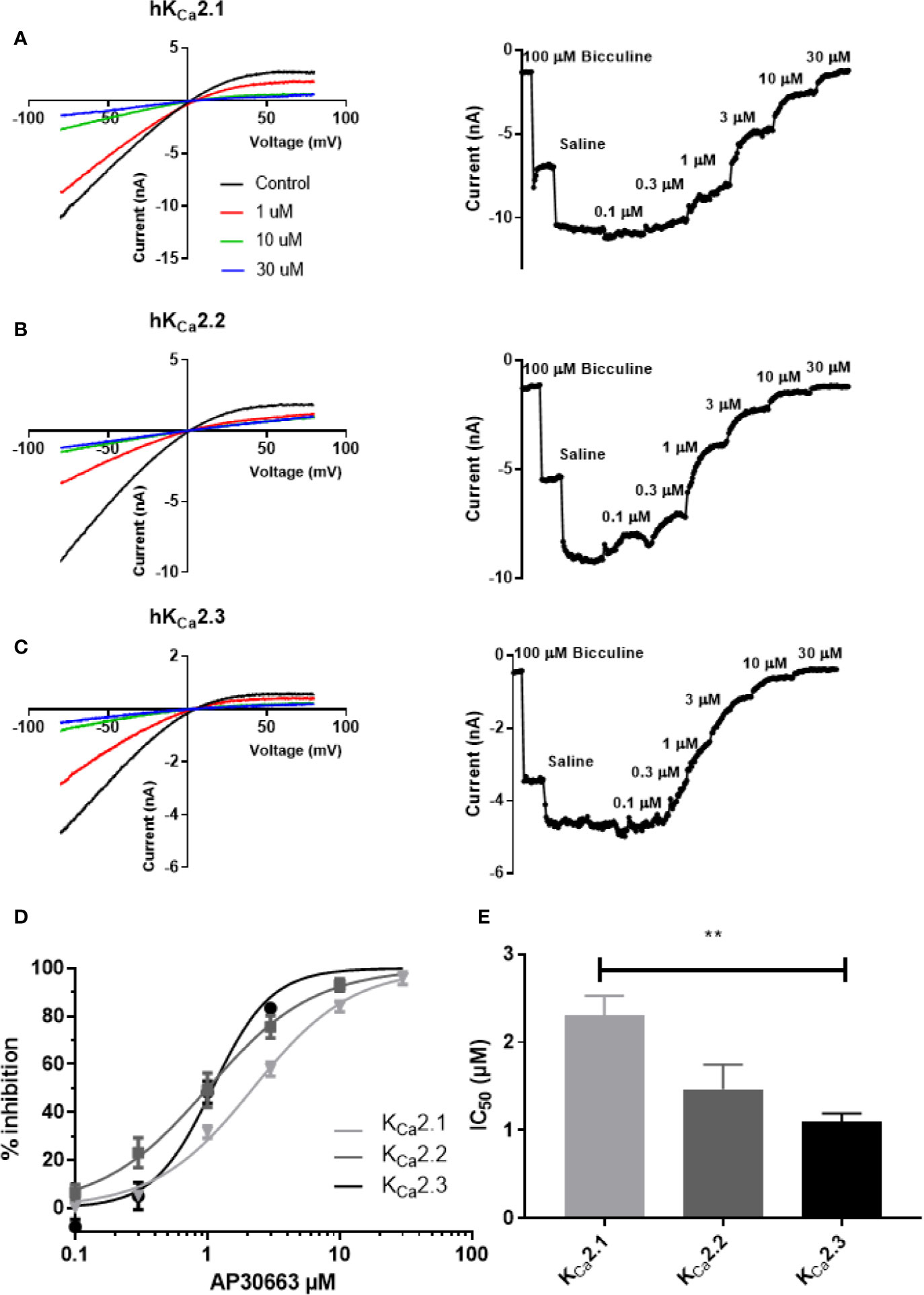
Figure 2 AP30663 inhibits hKCa2 channels. The effect of increasing concentrations of AP30663 was investigated by automated whole-cell patch-clamping in HEK cells stably expressing human KCa2 channels. Currents were elicited by applying a depolarizing voltage ramp protocol from −80 mV to +80 mV for 200 ms from a holding potential of 0 mV in symmetrical K+ solutions. Current–voltage recordings are depicted in the left panel for KCa2.1 (A), KCa2.2 (B) and KCa2.3 (C) before (black) and after application of 1 µM (red), 10 µM (green) and 30 µM (blue) AP30663. Current-time plots (right panel) showing the effect of increasing concentrations of AP30663 on the for KCa2.1 (A), KCa2.2 (B), and KCa2.3 (C) currents measured at a membrane potential of −80 mV. (D) Concentration–response curve of AP30663 on KCa2.1 (light gray), KCa2.2 (dark gray), and KCa2.3 (black). (E) Comparison of IC50 values for the 3 KCa2 channel subtypes. (KCa2.1, n = 9; KCa2.2, n = 19; and KCa2.3, n = 16). **p < 0.01.
To investigate the inhibitory mechanism of AP30663, we performed inside out patch-clamp experiments on HEK cells expressing KCa2.3 (Figure 3). In the absence of AP30663 the KCa2.3 channel had an EC50 for calcium activation of 0.33 ± 0.02 µM and was fully active at 3 µM. However, in the presence of 7 µM AP30663, 10 µM of calcium is needed to achieve full activation, and the EC50 for calcium activation is shifted to 1.50 ± 0.12 µM (EC50 baseline vs. AP30663: P < 0.0001). In addition to shifting the calcium activation curve, meaning that higher concentrations of calcium are needed to activate the channel, AP30663 also significantly decreased the Hill slope of the calcium activation curve from 4.4 ± 0.5 to 1.4 ± 0.1 (slope baseline vs. AP30663: P < 0.0001).
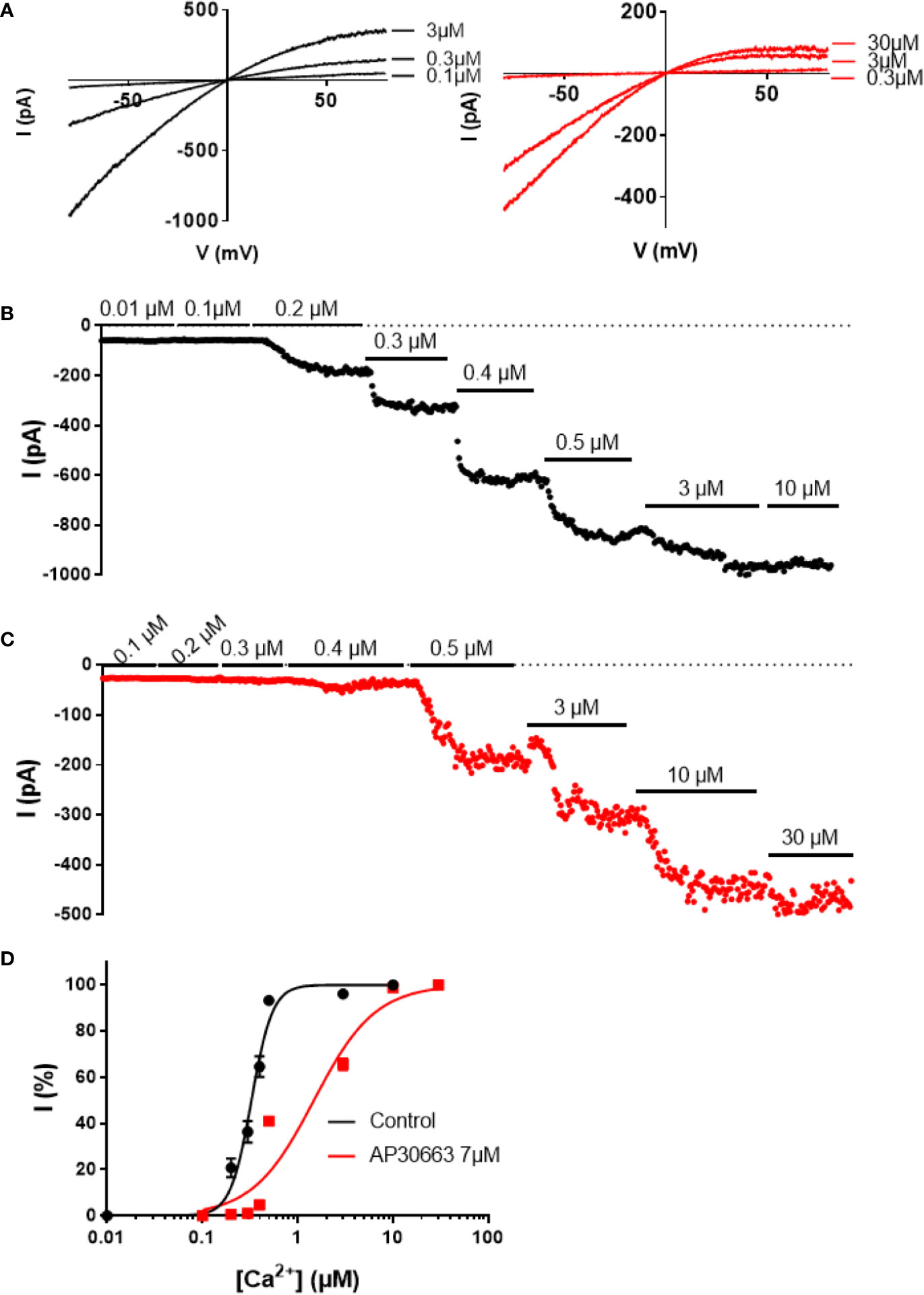
Figure 3 AP30663 shifts the calcium activation curve of hKCa2.3 channels. Current-voltage recordings (A) and corresponding current-time plots of KCa2.3 measured using the inside-out patch-clamp exposed to different free calcium concentrations, in the absence (B) (black) or presence of AP30663 7 µM (C) (red). (D) Calcium activation curves for KCa2.3 in the absence (black, n = 6) or presence of AP30663 7 µM (red, n = 6). Currents were measured at a membrane potential of −80 mV.
Ion Channel Selectivity Profile of AP30663
AP30663 was tested on a panel of cardiac ion channels to determine the functional selectivity profile of the compound. Automated whole-cell patch-clamp recordings of hKV11.1a channels revealed that AP30663 inhibited the IKr current with an IC50 value of 15.1 ± 2.1 µM (Figure 4A). In comparison when tested by manual patch clamp at 35°C, on HEK cells transfected with both cardiac isoforms KV11.1a and KV11.1b, AP30663 was estimated to have an IC50 of 4.0 ± 1.5 µM. 10 µM AP30663 did not significantly affect Kir3.1/Kir3.4 (IKACh), KV1.5 (IKur), KV7.1/KCNE1 (IKs), KV4.3/KChiP2 (Ito) and Kir2.1 (IK1) channels Figure 4B). AP30663 did not significantly inhibit the current mediated by CaV1.2 (ICa) (inhibition by 30 µM: 4±7 %). The effects on peak NaV1.5 channel current (INa) were investigated by automated patch-clamp experiments, and AP30663 in 10 µM did not affect INa (4±1% inhibition) (Figure 4B).
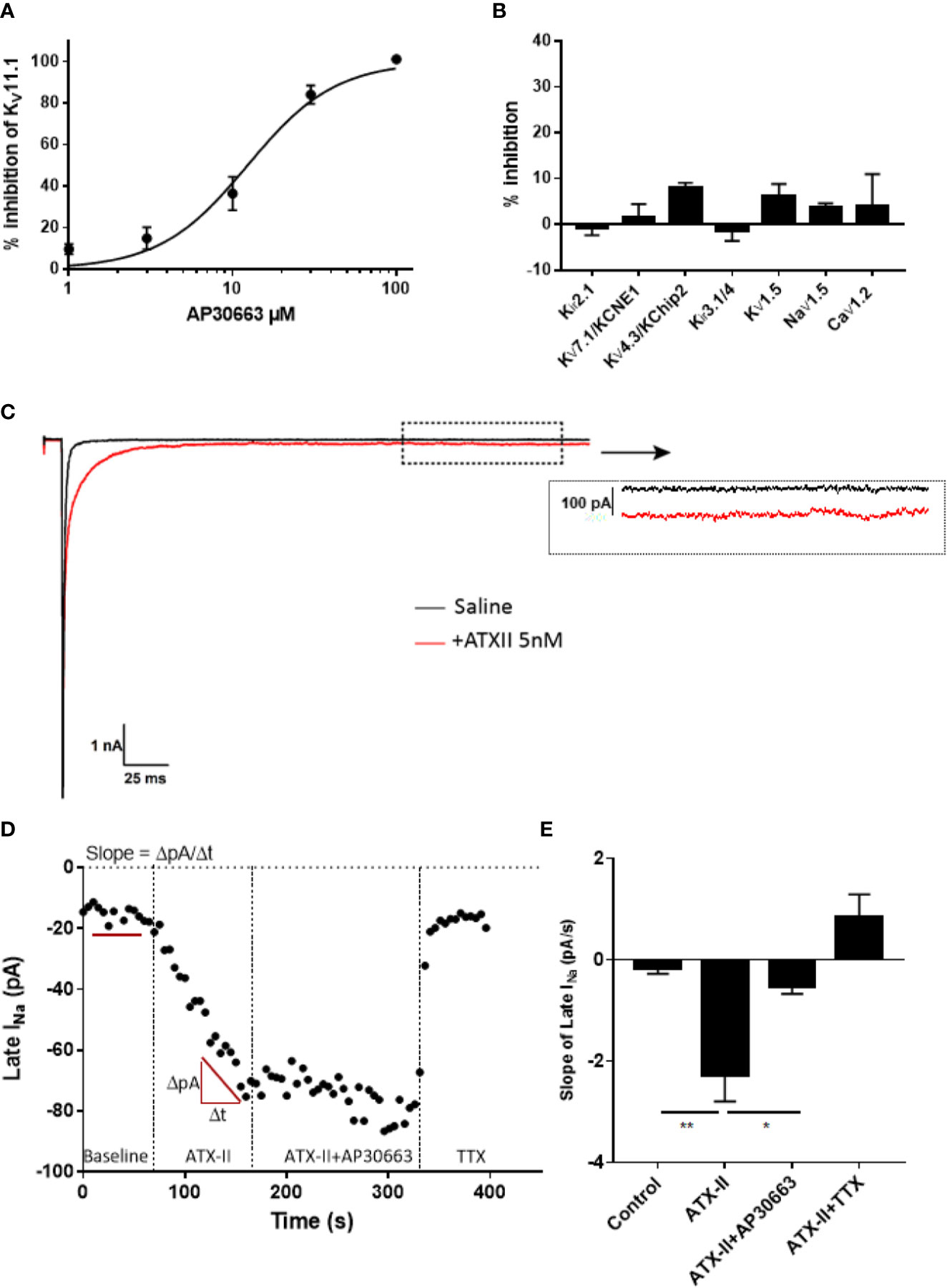
Figure 4 Ion channel selectivity profile of AP30663. (A) Concentration-response curve of the effect of increasing concentrations of AP30663 on KV11.1a recorded by automated whole-cell patch-clamp (n = 4). (B) % inhibition by 10 µM AP30663 on Kir3.1/Kir3.4 (IKACh, n = 6), KV1.5 (IKur, n = 5), KV7.1/KCNE1 (IKs, n = 5), KV4.3/KChiP2 (Ito, n = 5), and Kir2.1 (IK1, n = 5) using two-electrode voltage-clamp and NaV1.5 (INa, n = 11), CaV1.2 (ICa, n = 3; 30 µM) using automated whole-cell patch-clamping. (C) Current traces of the whole-cell Na+ current before and after application of ATXII recorded from HEK293 cells transiently transfected with hNaV1.5 (dotted box demonstrates where the late-sodium current was analyzed). (D) Late-sodium current amplitudes as a function of time. (E) Effect of compounds on the slope of the late-sodium current vs time plot. The slope was found by linear regression on the last 10 points of each liquid period (n = 14).
To determine if AP30663 had any effect on the late INa, we conducted a set of manual patch-clamp experiments on HEK cells transfected with the human NaV1.5 channel. Because of its small size, it is customary to co-apply the sea anemone toxin ATXII to augment late INa, and to use the sodium channel toxin tetrodotoxin (TTX) at the end of the experiment to inhibit residual late INa (Isenberg and Ravens, 1984; Maltsev et al., 1998). In Figure 4C, it can be seen how ATXII slows the inactivation of NaV1.5 and causes a small but relevant increase of the late current. From the time plot in Figure 4D, it can be appreciated that ATXII in our hands causes a continuous increase in the late INa amplitude. Hence, a steady state current is never achieved, and therefore judging the effect of AP30663 solely based on changes in late INa amplitude would be difficult. To circumvent this, we quantified the effect of 10 µM AP30663 on ATXII augmented late INa by comparing the changes in the slope of the late INa vs time plot. From the bar graph that summarizes the effect of AP30663 on the slope from 14 experiments it can be concluded that 10 µM AP30663 inhibits the late INa (Figure 4E).
AP30663 Prolongs the Atrial Refractory Period With Small Effects on Ventricular Repolarization—Functional Atrial Selectivity
A well-known anti-arrhythmic mechanism of rhythm control therapeutics is to prolong atrial refractoriness. After a baseline period the effects of increasing concentrations of AP30663 (1, 3, and 10 µM) on AERP in isolated female guinea pig hearts were investigated. From Figure 5, it can be appreciated that AP30663 increases AERP in a concentration-dependent manner, while QTcH is prolonged to a much lesser degree. To take into account baseline differences we evaluated the effect of AP30663 by comparing Δdrug-baseline between TMC and AP30663 groups for each concentration (Table 1). From this it can be observed that AP30663 significantly prolongs AERP at all concentrations, slows the heart rate in 3 and 10 µM, prolongs the QRS in 10 µM and prolongs the PQ-interval and QTcH-interval. The latter albeit to a much lesser extent (~8 ms) as compared to the effects of AP30663 on atrial refractoriness (10–50 ms). Similar experiments were conducted in male guinea pigs. No sex differences were observed (see Supplementary Figure 1 and Supplementary Table 1).
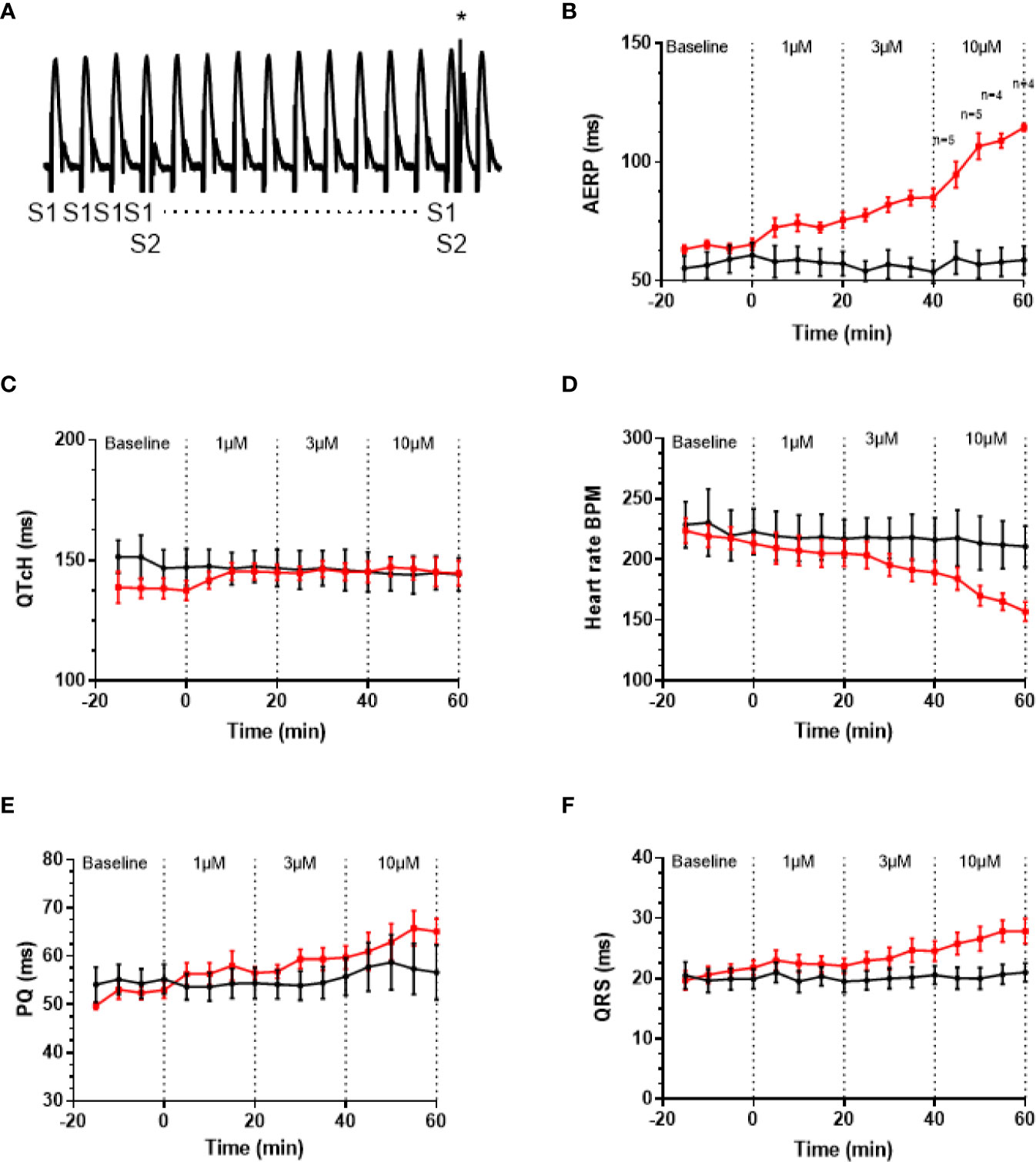
Figure 5 AP30663 prolongs the atrial refractory period in isolated perfused female guinea pig hearts. (A) Monophasic action potential recording recorded from the atrium of an isolated perfused guinea pig heart, during investigation of the atrial effective refractory period (AERP). Ten pacing stimuli (S1) are delivered at fixed basic cycle length of 200 ms, and for every 10th beat, an extra stimulus (S2 marked by * in the recording) is applied with 1 ms increments (for clarity not all S2 are shown). Notice how the first S2 does not result in an atrial action potential, whereas the second S2 (+1 ms) elicits an action potential. Graph demonstrating the effect of increasing concentrations of AP30663 on AERP (B), QTcH-interval (C), heart rate (D), PQ-interval (E), and QRS-duration (F) as compared with time matched control experiments (TMC) (AP30663, n = 6; TMC, n = 6).
AP30663 Prolongs Atrial Refractoriness In Vivo
To investigate the in vivo effects of AP30663, male rats were infused with increasing doses of AP30663 (5 and 10 mg/kg) or corresponding volumes of vehicle (1 and 2 ml/kg), while intracardiac recordings of AERP were performed. The AERP was significantly prolonged by 5 mg/kg and 10 mg/kg of AP30663 to 143% and 250% of TMC values (Figure 6).
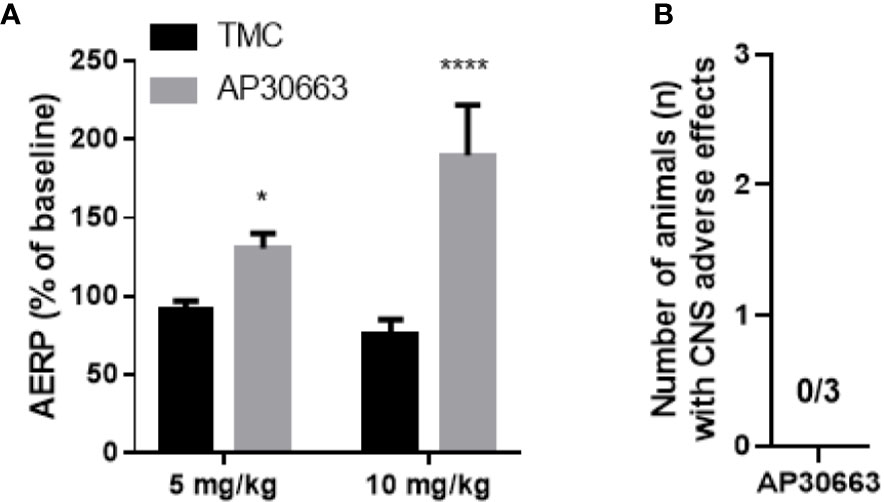
Figure 6 AP30663 prolongs the atrial refractory period in vivo in rats and does not induce CNS related adverse effects in mice. (A) The effect of i.v. injection of AP30663 or vehicle (time matched control, TMC) on AERP evaluated in anaesthetized rats using an intracardiac pacing catheter positioned in the right atria (TMC, n = 4; AP30663, n = 3). (B) Bar graph depicting the number of mice with CNS related adverse effects triggered by the i.v administration of AP30663 (10 mg/kg), n = 3. *0,0187, ****< 0,0001.
AP30663 Does Not Impair Coordination in Mice
KCa2 channels are expressed in the brain including cerebellum, and inhibition of these may lead to motor function impairment (Simó-Vicens et al., 2017). An observational Irwin test for assessing CNS exposure of AP30663 was performed in mice. Neither CNS related adverse effects nor other adverse effects were observed within 30 min of injection of 10 mg/kg i.v. AP30663 (n = 3, data not shown).
Discussion
KCa2 channels are widely expressed in the body. In the heart, KCa2 channels are functionally more important for repolarization of the atria as compared to the ventricles (Diness et al., 2010; Qi et al., 2014; Skibsbye et al., 2014; Zhang et al., 2014; Haugaard et al., 2015; Diness et al., 2017). Pharmacological experiments on mice, rats, guinea pigs, rabbits, pigs, goats, dogs and horses have demonstrated that KCa2 channel inhibitors can prolong atrial refractoriness, terminate AF and prevent reinduction of AF (Diness et al., 2010; Diness et al., 2011; Qi et al., 2014; Haugaard et al., 2015; Gatta et al., 2019). In addition, we previously demonstrated that KCa2 channel inhibition can cardiovert vernakalant-resistant AF in atrially tachypaced pigs (Diness et al.), and prolong atrial but not ventricular APD in human multicellular preparations (Skibsbye et al., 2014). Moreover, genome wide association studies have put the KCa2 channel (KCNN2 and KCNN3) on the list of genes that are highly associated with AF (Ellinor et al., 2010; Ellinor et al., 2012; Christophersen et al., 2017). KCa2 channels thus constitute an interesting novel drug target for treatment of AF.
The advances of genomics and transcriptomics allow us to study possible associations between genetic variants and their influence on mRNA expression levels (eQTL analyses), and hence guide drug discovery. Here we performed eQTL analyses and found that the AF-increasing variant of rs13376333 is associated with increased mRNA expression of KCNN3 in human atrial tissue. This suggests that increased KCa2 channel mRNA is associated with AF, and hence points towards the possibility of developing KCa2 channel inhibitors for the treatment of AF. To this end, we have developed AP30663. AP30663 is an inhibitor of KCa2 channels with no KCa2-subtype selectivity amongst KCa2.2 and KCa2.3 but a slightly lower potency on KCa2.1, which is the KCa2 subtype found to be least expressed in the human heart (Skibsbye et al., 2014). AP30663 inhibits the KCa2 channels when applied to both the intra- and extracellular side of the plasma membrane. From inside-out recordings we found that AP30663 significantly shifts the EC50 for calcium activation and lowers the Hill-slope, suggesting that AP30663 decreases the calcium sensitivity of KCa2 channels thereby acting as a negative allosteric modulator of the channel. This is similar to what has been reported for two other KCa2 inhibitors, NS8593 and AP14145 (Strøbaek et al., 2006; Simó-Vicens et al., 2017, 14145). Likewise, a changed Hill-slope was also observed for NS8593 and AP14145 and may indicate a loss of calcium cooperativity. AP30663 was functionally tested on a broad panel of other cardiac ion channels, and 10 µM AP30663 did not significantly affect the Kir3.1/Kir3.4 (IKACh), KV1.5 (IKur), KV7.1/KCNE1 (IKs), KV4.3/KChiP2 (Ito), Kir2.1 (IK1) and CaV2.1 (ICa,L) channels (the latter tested up to 30 µM). A concentration-dependent inhibition of KV11.1 by AP30663 was observed, with a calculated IC50 of 15 or 4 µM depending on assay type. The difference in potency can likely be explained by the differences in temperature, voltage protocols, and KV11.1 isoforms investigated (homomeric KV11.1a vs. heteromeric KV11.1a/KV11.b channels). Minor effects of 10 µM AP30663 on peak INa, but a significant inhibition of the late INa was observed. Selective inhibition of late INa is known to suppress ventricular arrhythmias, especially in settings of prolonged ventricular repolarization (Antzelevitch et al., 2004; Wu et al., 2004; Undrovinas and Maltsev, 2008; Antoons et al., 2010; Antzelevitch et al., 2011). Late INa has been found in atrial cardiomoycytes from patients with and without AF, although only at room temperature and not at physiological temperatures (Poulet et al., 2015). Ranolazine, which inhibits various ion channels in addition to also inhibiting the late INa, has demonstrated anti-AF efficacy in a number of studies (Scirica et al., 2007; Miles et al., 2011). Experimental evidence, however, indicates that ranolazine works against AF only at concentrations that also inhibit IKr and peak INa in atria (Burashnikov and Antzelevitch, 2013; Du et al., 2014). Late INa is reduced at higher heart rates and would not be expected to play a significant role during tachyarrhythmias such as AF (Burashnikov and Antzelevitch, 2013). Whether or not the inhibition of late INa seen by 10 µM AP30663 contributes to any antiarrhythmic activity of the compound is therefore unknown.
AP30663 consistently led to concentration- and dose-dependent prolongation of atrial refractoriness in ex vivo and in vivo experiments. Even though KCa2 channels are expressed in both atria and ventricles in guinea pigs (Kirchhoff et al., 2019), targeting KCa2 channel has previously been shown to be atrial selective in a wide range of species, including measurement on human heart tissue (Diness et al., 2010; Qi et al., 2014; Skibsbye et al., 2014; Zhang et al., 2014; Haugaard et al., 2015; Diness et al., 2017). Similarly, in the current study AP30663 was found to predominantly prolong the atrial refractoriness as compared to ventricular repolarization (QTcH). The QTcH prolongation (8 ms as compared to TMC) appeared to reach a plateau already at 1 µM. Considering the in vitro IC50 of AP30663 on KCa2 (~1 µM) and Kv11.1 (4–15 µM) and the mRNA expression of KCa2 and KV11.1 channels in guinea pig ventricles (Kirchhoff et al., 2019), the QTcH prolongation could be speculated to arise from combined contribution of ventricular KCa2 and Kv11.1 inhibition. The apparent observed plateau seen for QT prolongation could however also indicate that Kv11.1 inhibition has a minor impact. At this stage the exact contribution to the minor QT prolongation from KCa2 and Kv11.1 inhibition can only be speculative. We did not see any effects of AP30663 on QRS-duration, which correlates well with the lack of effects on peak NaV1.5 currents stimulated at 1 Hz. In addition to prolongation of AERP, the isolated guinea pig heart AP30663 also slows heart rate and prolongs the PQ-interval, suggesting effects on nodal tissue. This is in line with findings from mouse demonstrating that ablation of KCa2.2 reduces the firing of the AV node(Zhang et al., 2008) and pharmacological inhibition of KCa2 channels by apamin reduces the spontaneous firing of the sinus node(Torrente et al., 2017). Sex specific differences in the importance of SK channels for cardiac ventricular electrophysiology have been reported (Chen et al., 2018). However, we did not observe any differences in the response to AP30663 in male and female guinea pigs.
In the brain KCa2 channels contribute to neuronal action potential after hyperpolarization, and inhibition of the channel is known to disturb motor output from the cerebellum. Consequently, AP30663 was designed to have limited CNS exposure, and infusion of AP30663 to conscious mice confirmed this.
Conclusion
AP30663 was found to inhibit the KCa2 channel by decreasing the calcium sensitivity of the channel, while being selective over several relevant cardiac ion channels. Ex vivo and in vivo experiments confirmed the ability of the drug to prolong the AERP in a concentration-dependent fashion with minor effects on the QT interval. Based on this profile, AP30663 is an attractive compound for investigating if KCa2 channel inhibition can be used as a novel antiarrhythmic therapy.
Limitations
Although the calcium shift in calcium sensitivity was only investigated for KCa2.3 it is likely that the inhibitory mechanism of AP30663 on KCa2.1 and KCa2.2 is similar based on the high sequence homology between the three isoforms. The selectivity of the compound was investigated using overexpression systems and not on native cardiomyocytes. Hence, some of the complexity of native cardiac ion channel complexes, which potentially could impact the selectivity of the compounds, might have been missed. However, the obtained selectivity data on AP30663 combined with our ex vivo and in vivo ECG data supports that AP30663 does not have major impact on other cardiac ion channels. Moreover, for calcium recordings we only performed 3 experiments, which could limit the conclusion. However from our ex vivo and in vivo recordings we did not observe signs of calcium channel inhibition (e.g. PR interval prolongations and blood pressure drop).
Data Availability Statement
All datasets generated for this study are included in the article/Supplementary Material.
Ethics Statement
The animal experiments were performed under the license from the Danish Ministry of Justice (2018-15-0201-01430 & 2016-15-0201-00850) and in accordance with the Danish guidelines for animal experiments according to the European Commission Directive 86/609/EEC.
Author Contributions
SB, RS-V, LF, LA TS, and KM contributed with acquisition, interpretation and analysis of data. US, MG, and NE contributed with conception and design of the study. BB and JD wrote the first draft of the manuscript and interpreted and analysed the data, and contributed with conception and design of the study. All authors contributed to manuscript revision, read and approved the submitted version.
Funding
This work was supported by the Innovation Fund Denmark, Wellcome Trust (grant 100406/Z/12/Z), and the European Union’s Horizon 2020 research and innovation programme under the Marie Skłodowska-Curie grant agreement 675351.
Conflict of Interest
BB, RS-V, TS, NE, US, LA, SB, MG, and GD are employed by and/or have interests in Acesion Pharma and/or are inventors of Acesion Pharma patents within the field of SK channels.
The remaining authors declare that the research was conducted in the absence of any commercial or financial relationships that could be construed as a potential conflict of interest.
Acknowledgments
We thank Amer Mujezinovic for expert technical assistance.
Supplementary Material
The Supplementary Material for this article can be found online at: https://www.frontiersin.org/articles/10.3389/fphar.2020.00610/full#supplementary-material
References
Antoons, G., Oros, A., Beekman, J. D. M., Engelen, M. A., Houtman, M. J. C., Belardinelli, L., et al. (2010). Late na(+) current inhibition by ranolazine reduces torsades de pointes in the chronic atrioventricular block dog model. J. Am. Coll. Cardiol. 55, 801–809. doi: 10.1016/j.jacc.2009.10.033
Antzelevitch, C., Belardinelli, L., Wu, L., Fraser, H., Zygmunt, A. C., Burashnikov, A., et al. (2004). Electrophysiologic properties and antiarrhythmic actions of a novel antianginal agent. J. Cardiovasc. Pharmacol. Ther. 9 (Suppl 1), S65–S83. doi: 10.1177/107424840400900106
Antzelevitch, C., Burashnikov, A., Sicouri, S., Belardinelli, L. (2011). Electrophysiologic basis for the antiarrhythmic actions of ranolazine. Heart Rhythm. 8, 1281–1290. doi: 10.1016/j.hrthm.2011.03.045
Burashnikov, A., Antzelevitch, C. (2013). Role of late sodium channel current block in the management of atrial fibrillation. Cardiovasc. Drugs Ther. 27, 79–89. doi: 10.1007/s10557-012-6421-1
Carithers, L. J., Ardlie, K., Barcus, M., Branton, P. A., Britton, A., Buia, S. A., et al. (2015). A Novel Approach to High-Quality Postmortem Tissue Procurement: The GTEx Project. Biopreserv. Biobank. 13, 311–319. doi: 10.1089/bio.2015.0032
Chen, M., Yin, D., Guo, S., Xu, D.-Z., Wang, Z., Chen, Z., et al. (2018). Sex-specific activation of SK current by isoproterenol facilitates action potential triangulation and arrhythmogenesis in rabbit ventricles. J. Physiol. 596, 4299–4322. doi: 10.1113/JP275681
Christophersen, I. E., Rienstra, M., Roselli, C., Yin, X., Geelhoed, B., Barnard, J., et al. (2017). Large-scale analyses of common and rare variants identify 12 new loci associated with atrial fibrillation. Nat. Genet. 49, 946–952. doi: 10.1038/ng.3843
Diness, J. G., Sørensen, U. S., Nissen, J. D., Al-Shahib, B., Jespersen, T., Grunnet, M., et al. (2010). Inhibition of small-conductance Ca2+-activated K+ channels terminates and protects against atrial fibrillation. Circ. Arrhythm. Electrophysiol. 3, 380–390. doi: 10.1161/CIRCEP.110.957407
Diness, J. G., Skibsbye, L., Jespersen, T., Bartels, E. D., Sørensen, U. S., Hansen, R. S., et al. (2011). Effects on atrial fibrillation in aged hypertensive rats by Ca(2+)-activated K(+) channel inhibition. Hypertension 57, 1129–1135. doi: 10.1161/HYPERTENSIONAHA.111.170613
Diness, J. G., Skibsbye, L., Simó-Vicens, R., dos Santos, J., Lundegaard, P. R., Citerni, C., et al. (2017). Termination of Vernakalant-Resistant Atrial Fibrillation by Inhibition of Small Conductance Ca2+-Activated K+ Channels in pigs. Circ. Arrhythm. Electrophysiol. 10 (10). doi: 10.1161/CIRCEP.117.005125
Du, C., Zhang, Y., El Harchi, A., Dempsey, C. E., Hancox, J. C. (2014). Ranolazine inhibition of hERG potassium channels: drug-pore interactions and reduced potency against inactivation mutants. J. Mol. Cell. Cardiol. 74, 220–230. doi: 10.1016/j.yjmcc.2014.05.013
Ellinor, P. T., Lunetta, K. L., Glazer, N. L., Pfeufer, A., Alonso, A., Chung, M. K., et al. (2010). Common variants in KCNN3 are associated with lone atrial fibrillation. Nat. Genet. 42, 240–244. doi: 10.1038/ng.537
Ellinor, P. T., Lunetta, K. L., Albert, C. M., Glazer, N. L., Ritchie, M. D., Smith, A. V., et al. (2012). Meta-analysis identifies six new susceptibility loci for atrial fibrillation. Nat. Genet. 44, 670–675. doi: 10.1038/ng.2261
Folkersen, L., Wågsäter, D., Paloschi, V., Jackson, V., Petrini, J., Kurtovic, S., et al. (2011). Unraveling divergent gene expression profiles in bicuspid and tricuspid aortic valve patients with thoracic aortic dilatation: the ASAP study. Mol. Med. 17, 1365–1373. doi: 10.2119/molmed.2011.00286
Gatta, G., Sobota, V., SØRENSEN, U. S., DINESS, J. G., Verheule, S., Schotten, U., et al. (2019). Unraveling the Antiarrhythmic Action of SK Channel Block in a Goat Model of Stable Atrial Fibrillation. Heart Rhythm. (HRS 2019) 2019.
Haugaard, M. M., Hesselkilde, E. Z., Pehrson, S., Carstensen, H., Flethøj, M., Præstegaard, K. F., et al. (2015). Pharmacologic inhibition of small-conductance calcium-activated potassium (SK) channels by NS8593 reveals atrial antiarrhythmic potential in horses. Heart Rhythm. 12, 825–835. doi: 10.1016/j.hrthm.2014.12.028
Heijman, J., Dobrev, D. (2017). Inhibition of Small-Conductance Ca2+-Activated K+ Channels: The Long-Awaited Breakthrough for Antiarrhythmic Drug Therapy of Atrial Fibrillation? Circ. Arrhythm. Electrophysiol. 10 (10). doi: 10.1161/CIRCEP.117.005776
Holzgrefe, H., Ferber, G., Champeroux, P., Gill, M., Honda, M., Greiter-Wilke, A., et al. (2014). Preclinical QT safety assessment: Cross-species comparisons and human translation from an industry consortium. J. Pharmacol. Toxicol. Methods 69, 61–101. doi: 10.1016/j.vascn.2013.05.004
Isenberg, G., Ravens, U. (1984). The effects of the Anemonia sulcata toxin (ATX II) on membrane currents of isolated mammalian myocytes. J. Physiol. 357, 127–149. doi: 10.1113/jphysiol.1984.sp015493
Kirchhof, P., Benussi, S., Kotecha, D., Ahlsson, A., Atar, D., Casadei, B., et al. (2016). 2016 ESC Guidelines for the management of atrial fibrillation developed in collaboration with EACTS. Eur. Heart J. 37, 2893–2962. doi: 10.1093/eurheartj/ehw210
Kirchhoff, J. E., Skarsfeldt, M. A., Muthukumarasamy, K. M., Simó-Vicens, R., Bomholtz, S. H., Abildgaard, L., et al. (2019). The KCa2 Channel Inhibitor AP14145, But Not Dofetilide or Ondansetron, Provides Functional Atrial Selectivity in Guinea Pig Hearts. Front. Pharmacol. 10, 668. doi: 10.3389/fphar.2019.00668
Li, N., Timofeyev, V., Tuteja, D., Xu, D., Lu, L., Zhang, Q., et al. (2009). Ablation of a Ca2+-activated K+ channel (SK2 channel) results in action potential prolongation in atrial myocytes and atrial fibrillation. J. Physiol. (Lond.) 587, 1087–1100. doi: 10.1113/jphysiol.2008.167718
Maltsev, V. A., Sabbah Hani, N., Higgins Robert, S. D., Silverman, N., Lesch, M., Undrovinas, A. I. (1998). Novel, Ultraslow Inactivating Sodium Current in Human Ventricular Cardiomyocytes. Circulation 98, 2545–2552. doi: 10.1161/01.CIR.98.23.2545
Miles, R. H., Passman, R., Murdock, D. K. (2011). Comparison of effectiveness and safety of ranolazine versus amiodarone for preventing atrial fibrillation after coronary artery bypass grafting. Am. J. Cardiol. 108, 673–676. doi: 10.1016/j.amjcard.2011.04.017
Poulet, C., Wettwer, E., Grunnet, M., Jespersen, T., Fabritz, L., Matschke, K., et al. (2015). Late Sodium Current in Human Atrial Cardiomyocytes from Patients in Sinus Rhythm and Atrial Fibrillation. PloS One 10, e0131432. doi: 10.1371/journal.pone.0131432
Qi, X.-Y., Diness, J. G., Brundel, B. J. J. M., Zhou, X.-B., Naud, P., Wu, C.-T., et al. (2014). Role of small-conductance calcium-activated potassium channels in atrial electrophysiology and fibrillation in the dog. Circulation 129, 430–440. doi: 10.1161/CIRCULATIONAHA.113.003019
Scirica, B. M., Morrow, D. A., Hod, H., Murphy, S. A., Belardinelli, L., Hedgepeth, C. M., et al. (2007). Effect of ranolazine, an antianginal agent with novel electrophysiological properties, on the incidence of arrhythmias in patients with non ST-segment elevation acute coronary syndrome: results from the Metabolic Efficiency With Ranolazine for Less Ischemia in Non ST-Elevation Acute Coronary Syndrome Thrombolysis in Myocardial Infarction 36 (MERLIN-TIMI 36) randomized controlled trial. Circulation 116, 1647–1652. doi: 10.1161/CIRCULATIONAHA.107.724880
Simó-Vicens, R., Kirchhoff, J. E., Dolce, B., Jensen, L. A., Speerschneider, T., Sørensen, U. S., et al. (2017). A New Negative Allosteric Modulator AP14145 for the Study of Small Conductance Calcium-Activated Potassium Channels. Br. J. Pharmacol. 174 (23), 4396–4408. doi: 10.1111/bph.14043
Skibsbye, L., Poulet, C., Diness, J. G., Bentzen, B. H., Yuan, L., Kappert, U., et al. (2014). Small-conductance calcium-activated potassium (SK) channels contribute to action potential repolarization in human atria. Cardiovasc. Res. 103, 156–167. doi: 10.1093/cvr/cvu121
Strøbaek, D., Hougaard, C., Johansen, T. H., Sørensen, U. S., Nielsen, E.Ø., Nielsen, K. S., et al. (2006). Inhibitory gating modulation of small conductance Ca2+-activated K+ channels by the synthetic compound (R)-N-(benzimidazol-2-yl)-1,2,3,4-tetrahydro-1-naphtylamine (NS8593) reduces afterhyperpolarizing current in hippocampal CA1 neurons. Mol. Pharmacol. 70, 1771–1782. doi: 10.1124/mol.106.027110
Torrente, A. G., Zhang, R., Wang, H., Zaini, A., Kim, B., Yue, X., et al. (2017). Contribution of small conductance K+ channels to sinoatrial node pacemaker activity: insights from atrial-specific Na+/Ca2+ exchange knockout mice. J. Physiol. 595, 3847–3865. doi: 10.1113/JP274249
Tuteja, D., Xu, D., Timofeyev, V., Lu, L., Sharma, D., Zhang, Z., et al. (2005). Differential expression of small-conductance Ca2+-activated K+ channels SK1, SK2, and SK3 in mouse atrial and ventricular myocytes. Am. J. Physiol. Heart Circ. Physiol. 289, H2714–H2723. doi: 10.1152/ajpheart.00534.2005
Undrovinas, A., Maltsev, V. A. (2008). Late sodium current is a new therapeutic target to improve contractility and rhythm in failing heart. Cardiovasc. Hematol. Agents Med. Chem. 6, 348–359. doi: 10.2174/187152508785909447
Waks, J. W., Zimetbaum, P. (2016). Antiarrhythmic Drug Therapy for Rhythm Control in Atrial Fibrillation. J. Cardiovasc. Pharmacol. Ther. 22 (1), 3–19. doi: 10.1177/1074248416651722
Wu, L., Shryock, J. C., Song, Y., Li, Y., Antzelevitch, C., Belardinelli, L. (2004). Antiarrhythmic effects of ranolazine in a guinea pig in vitro model of long-QT syndrome. J. Pharmacol. Exp. Ther. 310, 599–605. doi: 10.1124/jpet.104.066100
Zhang, Q., Timofeyev, V., Lu, L., Li, N., Singapuri, A., Long, M. K., et al. (2008). Functional Roles of a Ca2+-Activated K+ Channel in Atrioventricular Nodes. Circ. Res. 102, 465–471. doi: 10.1161/CIRCRESAHA.107.161778
Keywords: atrial fibrillation, ion channels, anti-arrhythmic drugs, SK channels, KCa2
Citation: Bentzen BH, Bomholtz SH, Simó-Vicens R, Folkersen L, Abildgaard L, Speerschneider T, Muthukumarasamy KM, Edvardsson N, Sørensen US, Grunnet M and Diness JG (2020) Mechanisms of Action of the KCa2-Negative Modulator AP30663, a Novel Compound in Development for Treatment of Atrial Fibrillation in Man. Front. Pharmacol. 11:610. doi: 10.3389/fphar.2020.00610
Received: 16 December 2019; Accepted: 20 April 2020;
Published: 06 May 2020.
Edited by:
Heike Wulff, University of California, Davis, United StatesReviewed by:
Nipavan Chiamvimonvat, University of California, Davis, United StatesRicardo Gómez, University of La Laguna, Spain
Copyright © 2020 Bentzen, Bomholtz, Simó-Vicens, Folkersen, Abildgaard, Speerschneider, Muthukumarasamy, Edvardsson, Sørensen, Grunnet and Diness. This is an open-access article distributed under the terms of the Creative Commons Attribution License (CC BY). The use, distribution or reproduction in other forums is permitted, provided the original author(s) and the copyright owner(s) are credited and that the original publication in this journal is cited, in accordance with accepted academic practice. No use, distribution or reproduction is permitted which does not comply with these terms.
*Correspondence: Bo Hjorth Bentzen, Ym9iZUBzdW5kLmt1LmRr