- 1Instituto de Farmacia, Facultad de Ciencias, Universidad Austral de Chile, Valdivia, Chile
- 2Laboratorio de Síntesis y Biotransformación de Productos Naturales, Departamento de Ciencias Básicas, Facultad de Ciencias, Universidad del Bío-Bío, Chillán, Chile
- 3Departamento de Química Orgánica y Fisicoquímica, Facultad de Ciencias Químicas y Farmacéuticas, Universidad de Chile, Santiago, Chile
- 4Departamento de Ciencias del Ambiente, Facultad de Química y Biología, Universidad de Santiago de Chile, Santiago, Chile
Weinmannia trichosperma Cav. (Cunoniaceae) (local name, tineo; Mapuche names, madén, mëdehue) is an endemic species of Chile and Argentina used in Mapuche traditional medicine in the treatment of chronic diarrhea, inflammation, and wound healing. This study focused on the isolation, analysis, and characterization of the biological activity of compounds and bark extracts from this plant for the first time. The infusion and tincture of the bark were characterized regarding antioxidant and important enzyme inhibitory activities, phenolics, and flavonoids content and UHPLC-ESI-OT-MS metabolite profiling. Twenty-five metabolites were detected in the medicinal infusion of W. trichosperma, three flavonols were isolated: isoastilbin, neoisoastilbin, and neoastilbin ((2R,3S)-, (2S,3R)-, and (2S,3S)-dihydroquercetin 3-O-alpha-L-rhamnoside) by countercurrent chromatography, and the isomers were quantified in the bark using a validated analytical HPLC methodology. The antioxidant properties were measured by ABTS, DPPH, FRAP, ORAC, and TEAC methods. The infusion displayed a strong DPPH and ABTS scavenging activity (IC50 = 20.58 and 3.070 µg ml−1, respectively) while a moderated effect was observed in the FRAP, ORAC, and ABTS assays. The infusion showed a content of phenolic and flavonoid compounds of 442.1 mg GAE g−1 and 15.54 mg QE g−1, respectively. Furthermore, the infusion showed a good and promissory inhibitory activity (33.80%, 33.12%, and 82.86% for AChE, BuChE, and 5-hLOX, respectively) and isoastilbin (51.70%, 50.10%, and 34.29–80.71% for AChE, BuChE, and 5-hLOX, respectively). The biomolecules identified in this study support the traditional uses of this bark and the potential industrial interest from this Valdivian plant species.
Introduction
Weinmannia trichosperma Cav. (Cunoniaceae) (local name: tineo, Mapuche name: madén, mëdehue, Figure 1) is an evergreen tree endemic of temperate rainforest of Chile and Argentina. In Chile, this plant grows in the regions of Maule, Biobío, Araucanía, Los Ríos, Los Lagos, Aysén, and Magallanes (Rodriguez et al., 2018). The local inhabitants use the bark infusion of W. trichosperma to treat chronic diarrhea, and it is used as poultice for application on wounds (Gusinde, 1936; Muñoz et al., 1981; Houghton and Manby, 1985). Previous pharmacological studies of W. trichosperma have demonstrated their antimicrobial potential (Mølgaard et al., 2011), and one quantitative phytochemical study showed that the composition of tannins and phenolic compounds of this bark were 19.98% and 4.81%, respectively (Holler et al., 2012). Extracts of natural products are highly complex mixtures of active compounds, and the purification is a challenging task (Skalicka-Woźniak and Garrard, 2014). Among the chemical methods for the study of plants, chromatographic analysis has a fundamental role because it involves numerous advantages, such as its specificity and the possibility of qualitative and quantitative analyses (Kowalska et al., 2007). The interest in endemic traditional plants is important since they represent a huge source very little explored of potential biomolecules that can become potential promising candidates for the study of new drugs of pharmacological interest and support the use of native species in functional foods or nutraceuticals. Ultra-high-performance chromatography (UHPLC) coupled to mass spectrometer (MS) hyphenated devices nowadays offer higher sample throughput with more accurate information per sample. In the case of orbitrap technology (OT), it offers the fast and reliable untargeted information of several different metabolites, such as phospholipids, glycans, phenolic compounds, peptides, pesticides, toxins, and other small bioactive molecules (Simirgiotis et al., 2016; Luna et al., 2018; Deng et al., 2019, Yang et al., 2018a). To our knowledge, there are no reports on chemical characterization, antioxidant, antiinflammatory, or enzyme inhibitory capacities of the phenolic-enriched bark obtained from W. trichosperma.
Centrifugal partition chromatography (CPC) (Ito, 2005) is a very versatile technique that has been used for decades as a fractionation technique, and nowadays, most of the applications are dedicated to the investigation of medicinal plants and isolation of natural products (Marston and Hostettmann, 2006; Friesen et al., 2015; Liu et al., 2015).
In this work, we have applied CPC for the fast isolation of the main phenolic constituents from bark of W. trichosperma. Additionally, an UHPLC-PDA-MS fingerprint was obtained, and the main compounds were quantified in the medicinal bark. Furthermore, the antioxidant, anti-inflammatory, and enzyme inhibitory effects complemented with the full polyphenolic profile are performed, supporting the reputed medicinal properties of this plant and showing the presence of molecules of pharmacological and industrial interest.
Materials and Methods
Chemical and Reagents
Gallic acid (purity > 98%), quercetin, (purity > 97%), 6-hydroxy-2,5,7,8-tetramethylchromane-2-carboxylic acid (Trolox) (purity > 97%), 2,2′-azinobis(3-ethylbenothiazoline-6-sulfonic acid) diammonium salt (ABTS), 2,2-diphenyl-1-picrylhydrazyl (DPPH), Folin-Ciocalteu reagent 2,4,6-tri(2-pyridyl)-s-triazine (TPTZ), aluminum chloride, iron (III) chloride hexahydrate, (4-(2-hydroxyethyl)-1-piperazineethanesulfonic acid (HEPES), ethylenediaminetetraacetic acid (EDTA), adenosine 5′-triphosphate (ATP) disodium salt, acetylcholinesterase from Electrophorus electricus (electric eel) and butyrylcholinesterase from equine serum were from Sigma-Aldrich® (Chile). The enzyme 5-lipoxygenase (human, recombinant) were from Cayman Chemical (Item No. 60402). Calcium chloride, sodium carbonate, sodium hydroxide, sodium nitrite, and potassium persulphate were obtained from Merck® (Chile). Acetone, galanthamine, zileuton, sodium acetate trihydrate, and glacial acetic acid were from Merck® (Chile). HPLC reagents, formic acid, methanol, ultrapure water, ethyl acetate, and n-hexane were form Merck® (Chile). Deionized water was used for all the experiments.
Plant Material
The bark of W. trichosperma was collected during the months of October and November of 2017 in Valdivia, XIV Region de Los Ríos, Chile, and deposited in our laboratory herbarium (voucher number Wt10012017). The plant was identified by the botanist Alicia Marticorena.
Extraction of Plant Material
Once the plant material was collected, it was cleaned, dried, and stored at room temperature for 7 days. Then, the bark was cut into pieces and ground to powder in a mill. To obtain the ethanolic extract, or tincture, three macerations were carried out with 540 g of bark powder, 2 L of absolute ethanol, and macerated for 24 h (three times) in darkness at room temperature. Then, the extract was filtered and concentrated in a rotary evaporator to dryness (at 40°C, at 2.7 Bar and 80 r.p.m.), finally, the extract was stored at −80°C in a 50-ml glass bottle. To obtain the edible infusion, 2.5 g of plant material were weighed then 250 ml of boiling deionized water was added, without stirring, to prepare a cup-of-tea infusion of W. trichosperma, then it was allowed to stand for 1 h at room temperature and filtered under vacuum, after that the infusion was frozen at −42°C, and finally lyophilized and stored at −80°C in a 1- to 5-ml glass bottle.
Centrifugal Partition Chromatography
Selection of the Solvent System
Centrifugal partition chromatography was applied for the rapid isolation of the main phenolic compounds present in the bark of W. trichosperma. The biphasic solvent system used to perform the isolation of the compounds was selected by measuring the partition coefficient (k) in different proportions of the hexane-ethyl acetate-methanol-water system (HEMWAT). The value of k was defined for the main phenolic compound of the extracts of W. trichosperma as k = Aupper/Alower, where Aupper corresponds to the area under the curve of the peak in the upper phase and Alower corresponds to the area under the curve of the peak in the lower phase of the solvent system, these areas were obtained by gradient elution (Table 1), using HPLC-PDA with detection wavelengths at 230, 290, 330, and 380 nm with a flow of 1 ml min−1 at 25°C. To obtain the k value, approximately 10 mg of ethanolic extract were weighed in a 2-ml vial, and 1 ml of upper phase and 1 ml of lower phase of the pre-equilibrated biphasic solvent system (HEMWAT 1: 9: 1: 9 v/v, 1: 3: 1: 3 v/v, 2: 5: 2: 5 v/v, and 4: 6: 4: 6 v/v) (Ito, 2005) were added, vortexed, and filtered using a 0.22-μm PTFE filter. Then, 10 ml of each phase was injected in the HPLC-DAD in triplicate to measure the area of each compound. Finally, the biphasic solvent system suitable for the separation of the phenolic compounds of W. trichosperma selected.
Separation and Purification of Phenolic Compounds
The biphasic system HEMWAT 1:9:1:9 v/v was used, and 11 g of ethanolic extract were weighed and dissolved in a mixture of 5 ml of upper phase and 5 ml of lower phase, sonicated for 5 min and vortexed for 5 min. The CPC equipment was purged for 1 min with a flow of 30 ml min−1, the equipment was filled with stationary phase in normal (or ascending) mode with a rotation of 500 rpm. Once the CPC equipment rotor had a speed of 1800 r.p.m. manual injection of the sample was performed using the injection loop. The mobile phase was supplied using two HPLC pumps with a flow rate of 10 ml min−1. The equipment had a PDA detector for the detection of the peaks, the wavelengths used were 280, 330, 350, and 254 nm, the eluent was collected for 1 h by means of an automatic fraction collector for test tubes (25 ml each). Finally, the extrusion was carried out for 15 min with a 100% stationary phase, and the fractions obtained from the majority compounds were lyophilized and subsequently stored in 15-ml glass bottles.
HPLC Quantitative Analysis
An HPLC-PDA (Thermo Scientific Dionex Ultimate 3000 Series RS, Thermo Fisher Scientific, Germany) was used to determine and quantify the major compounds in the extracts of W. trichosperma. A Purosphere Merck® C18-RP column, 25 cm long, particle size 2.5 μm with a precolumn was used, and an elution gradient of water with formic acid (1% v/v) and methanol with formic acid (1% v/v) was used at a flow rate of 1 ml min−1, injection volume of 20 μl, and UV detection wavelengths of 230, 290, 330, and 380 nm. Elution gradient of A (water with 1% v/v formic acid) and B (methanol with 1% v/v formic acid). The step gradient was as follows: 0 to 8 min linear increase from 10% to 32% B; then maintaining 32% B, from 8 to 25 min, then, linear increase to 70% B and coming back to initial conditions, then hold 15 min for the next injection. The quantification experiments were performed by HPLC-PDA and elution conditions of qualitative analysis (Supplementary Material, Figures S1 and S2). The content of isoastilbin compound and its derivatives were established with reference to calibration curves built with the pure compounds, (astilbin, neoastilbin, and neoisoastilbin) at 254 nm. A stock solution of 2 mg ml−1 of purified compounds was used, and the dilutions were prepared to perform calibration curves with the following concentration levels: 1, 5, 10, 30, 50 μg ml−1. These dilutions were prepared in triplicate and measured in triplicate (n = 9). With the obtained data, the linear regression curve was generated using the Chromeleon 7.2 software, the equation of the curve and the coefficient of determination were obtained (for neoastilbin y = 571.2372x–2.3871, R2 = 0.9998; for isoastilbin y = 571.6340x–2.4065, R2 = 0.9997, and for neoisoastilbin y = 571.0794x–2.3264, R2 = 0.9998). All injections were performed in triplicate, and the results were expressed as mean ± standard deviation (SD).
MS Conditions
Untargeted analysis of all compounds was performed using a Thermo Scientific Dionex Ultimate 3000 Series RS coupled to an Q-Exactive focus mass spectrometer (Thermo Fisher Scientific, Germany) operated both in positive and negative modes at 17.500 FWHM (m/z 200) using a scan range of m/z 130–1000 and injection time of 200 ms and automatic gain control at 3e6. Scan rate was set at 2 scans s−1, and a mass tolerance window was set to 5 ppm. The calibration was performed by positive and negative modes before each series of samples. In addition to the full scan mode, for confirmatory purposes, a targeted MS/MS analysis was performed using the mass inclusion list and expected retention times of the target analytes, with a 30-s time window. Data acquired were finally evaluated by the Browser Xcalibur 2.3 (Thermo Fisher) (Nwokocha et al., 2017).
Method Performance and Validation of the Analytical Methodology for HPLC-PDA
The validation of the analytical methodology was carried out according to the analytical performance parameters suggested by the ICH (International Council for Harmonization of Technical Requirements for Pharmaceuticals for Human Use) in the guide Q2 (R1) (Borman and Elder, 2018). The accuracy was determined in terms of percent recovery for three points of the calibration curve: 10, 50, and 500 μg ml−1. The accuracy was assured for the limit of quantification. In addition, the percentage of absolute recovery was determined.
Extraction with boiling water was carried out with 50.0 mg of powdered plant material, for that 0.3 mg (100%), 0.4 mg (130%), and 0.7 mg (150%) of isoastilbin were added. The repeatability (intraday precision) was determined in triplicate of six points of the calibration curve by the same analyst in the same working day. The accuracy under the repeatability conditions was expressed in relative standard deviation (RSD). The intermediate precision was determined by the same analyst in different days. Three points of the calibration curve were prepared, meaning low, medium, and high concentration in triplicate. The intermediate precision was expressed in RSD. The range of work chosen was between 0.01 (lower limit) and 0.5 mg ml−1 (upper limit) of isoastilbin and as intermediate concentrations 0.03, 0.05, 0.1, and 0.3 mg ml−1. The limit of detection (LOD) was determined based on the signal-to-noise ratio (3:1), and the limit of quantification (LOQ) was determined based on the signal-to-noise ratio (10:1). The method was validated; as seen in Supplementary Material, Tables S1–S6. According to the results all values were within acceptable criteria (Borman and Elder, 2018). The LOD and LOQ values were lower than 0.12 and 0.39 mg standard respectively (Supplementary Material, Table S1). Therefore, the method was concluded as suitable to quantify isoastilbin and related phenolic compounds in the plant extract. Over the selected range, peak areas linearly depended on concentrations for all phenolic compounds with high correlation coefficients (>0.9998, Table 1). The injection repeatability of the system was evaluated by seven consecutive injections of the standard solution of phenolic compounds. The RSD values of retention times of phenolic compounds were less than 0.60% (Supplementary Material, Table S6).
Determination of Total Phenolics and Flavonoids Content
The spectrophotometric quantification of total phenolic content (TPC) and total flavonoid content (TFC) of the lyophilized aqueous extract (infusion) and tincture of the bark of W. trichosperma was carried out following the previously reported Folin-Ciocalteu and AlCl3 methodology in a Synergy HTX microplate reader (Biotek, Winoosky, VT, USA) (Kuskoski et al., 2005; Bhaigyabati et al., 2017). Total phenolics using the Folin-Ciocalteu reagent, the results were derived from a calibration curve of gallic acid (y = 0.0319x + 0.138, R2 = 0.996). Also, for total flavonoids, the results were derived from the calibration curve of quercetin (y = 0.0136 x + 0.0414, R2 = 0.979). The bark was tested at 1 mg ml−1, Total phenolic content was expressed as milligrams of gallic acid equivalents (GAE) per gram of extracts (mg GAE g−1 extract). Flavonoids were expressed as milligrams of quercetin equivalents (QE) per gram of extracts (mg QE g−1 extract). The determinations were made in triplicate. The values from triplicates are reported as the mean ± SD.
In Vitro Antioxidant Activity of W. trichosperma
The antioxidant activity was evidenced by in vitro assays such as the oxygen radical absorbance capacity (ORAC), bleaching of the free radical 2,2-diphenyl-1-picrylhydracil hydrate (DPPH), Ferric Reducing Antioxidant Power (FRAP) and by the bleaching of 2,2′-azino-bis-(3-ethylbenzothiazoline-6-sulphonic acid (ABTS). These assays were performed for the extracts and the major compound isolated, isoastilbin. Data were recorded in triplicate and expressed as average value.
ABTS Assay
The ABTS free radical scavenging assay was employed to measure the free radical scavenging activity of extracts of W. trichosperma and for isoastilbin. We used the method of Re et al. (1999) with some modifications, a solution of 7 mM ABTS (2,2′-azino-bis(3-ethylbenzothiazoline-6-sulphonic acid)) and a solution 2.45 mM potassium persulfate in water was prepared. Both aqueous solutions were mixed in a 1:1 ratio (v/v) and incubated for 16 h in the dark for the formation of the radical at room temperature. After this period, using 96-well plates a volume of 275 µl of solution (absorbance 0.7) was mixed thoroughly with 25 µl of standard or the samples, the absorbance was immediately recorded at 734 nm using a microplate reader. Gallic acid was used as a reference standard (from 1 to 100 µg ml−1); a curve of extracts of W. trichosperma were made (from 19.53 to 625 µg ml−1) and a curve of isoastilbin (from 19.53 to 2500 µg ml−1) to calculate their IC50. The percentage of ABTS radical inhibition was calculated according to the following equation:
As, is the absorbance of the sample with ABTS; Abs, is the absorbance of the mixture of blank and sample; A: ABTS, is the absorbance of the ABTS stock solution. The IC50 was calculated using GraphPad Prism 7 software.
DPPH-Free Radical-Scavenging Activity Assay
DPPH free radical-scavenging activity was determined according to Brand-Williams et al. (1995) with some modifications. A solution of DPPH 400 µM was prepared and using a volume of 150 µl of this solution thoroughly mixed with 50 µl of standard or the samples, the absorbance was recorded at 515 nm using a microplate reader and 96-well plates 30 min later. Gallic acid was used as a reference standard (from 1 to 100 µg ml−1); curves of extracts of W. trichosperma (from 19.53 to 625 µg ml−1) and a curve of isoastilbin (from 19.53 to 2500 µg ml−1) were prepared. The percentage of DPPH radical inhibition was calculated according to the following equation:
As is the absorbance of the sample with DPPH, Abs is the absorbance of the mixture of blank and sample, A DPPH is the absorbance of the DPPH stock solution. The IC50 was calculated using GraphPad Prism 7 software.
Ferric Reducing/Antioxidant Power FRAP Assay
The method of Collins et al. (1959) with some modifications was used, briefly, a buffer solution CH3Na*3H2O 3.1%/CH3COOH (glacial) 16%, dissolved in water plus 20 mM FeCl3 in aqueous solution HCl 0.02 M and 10 mM TPTZ dissolved in absolute ethanol. The working solution corresponded to a mixture of one volume of buffer with one volume of FeCl3 and 11 volumes of ethanol. Trolox was used as a reference standard (from 10 to 250 µg ml−1), for each level of concentration, 290 µl of working solution were mixed with 10 µl of Trolox in a well of the microplate in triplicate, and then the absorbance was measured at 593 nm after 5 min, with the data obtained a linear regression equation was used to calculate the concentration of samples.
ORAC Assay
The assay was carried out on a plate reader, at 37°C as reported (Wu et al., 2004). Briefly, AAPH was used as peroxyl generator and Trolox as a standard. Using 48-well microplates, 40 µl of sample, blank, and Trolox calibration solutions were mixed in triplicate. The fluorescence of fluorescein disodium (FL) of each cycle was recorded. Parameters for the plate reader were: orbital shaking (4 mm shake width), cycle time, 210 s; position delay, 0.3 s; cycle number, 35; shaking mode, 8 s before each cycle; injection speed, 420 µl s−1. Values were calculated by using a quadratic regression equation between the Trolox or sample concentration and net area under the FL decay curve. Data are expressed as micromoles of Trolox equivalents (TE) per gram of sample (µmol of TE g−1). The area under curve (AUC) was calculated as
where f3 = fluorescence reading at cycle 3; fn= fluorescence reading at cycle n; and CT, cycle time in minutes.
Enzyme Inhibitory Activity
Cholinesterases (ChE) Inhibition
The inhibitory activity of these important enzymes was measured thorough the Ellman’s method, as stated previously (Mocan et al., 2017). Briefly, DTNB was dissolved in buffer Tris-HCl at pH 8.0 containing 0.1 M NaCl and 0.02 M MgCl2. Then, a filtered sample solution dissolved in deionized water (50 ml, 2 mg ml−1) was mixed with 125 ml of 5-dithio-bis(2-nitrobenzoic) acid (DTNB), acetylcholinesterase (AChE), or butyrylcholinesterase (BChE) solution (25 ml) dissolved in Tris-HCl buffer at pH 8.0 in a 96-well microplate and incubated for 15 min at 25°C. Initiation of reaction was performed by the addition of acetyl-thiocholine iodide (ATCI) or butyryl-thiocholine chloride (BTCl) (25 ml). In addition, a blank was prepared by adding the solution sample to all reagents without the enzyme(s) (AChE or BChE) solutions. The sample and blank absorbances were then read at 405 nm after 10 min of incubation at 25°C. The absorbance of the sample was subtracted from that of the blank and the cholinesterase inhibitory capacity was expressed as IC50 (µg ml−1, concentration range 0.05 to 25 µg ml−1). Galantamine was used as positive control. All data were collected in triplicate.
Human 5-lipoxygenase (5-hLOX) Inhibition
The screening inhibitory activity of 5-hLOX was performed fluorescence-based assay is performed using microplates. For this, a 50-mM HEPES buffer was prepared at pH 7.5, which contains 2 mM EDTA, 2 mM CaCl2, and 10 μM ATP. A dilution of the human 5-lipoxygenase (5-hLOX) enzyme (1:500) in the reaction buffer was used together with 10μM 2,7-dichlorofluorescein diacetate (H2DCFDA dye, fluorimetric probe). The mixture was allowed to incubate in the assay microplate for 15 min at room temperature. Subsequently, 33.3 μl of the buffer was added to each well of the cell culture plate to be used. To each well, 3.3 μl of inhibitor was added and incubated for 30 min. Finally, the reaction was started by the addition of a suitable concentration of arachidonic acid substrate (3.3 µl, 0.5 μM), and fluorescence was read in a Synergy™ HT Multi-Mode Microplate Reader (Biotek Instruments, Inc, Winooksi, VT, USA) at 480 nm excitation/520 nm emission after a reaction that had proceeded for 1 h at room temperature for determine the values of percentage inhibition. Nordihydroguaiaretic acid (NDGA) was used as a positive control. All data were collected in triplicate.
Docking Studies
The geometries and partial charges of astilbin, isoastilbin, neoastilbin, and neoisoastilbin contained in the W. trichosperma aqueous extract, as well as the known cholinesterases (TcAChE- hBuChE) inhibitor galantamine and the 5-hLOX inhibitor zileuton were fully optimized using the DFT method with the standard basis set PBE0/6-311+g* (Adamo and Barone, 1999, Petersson et al., 1988). All calculations were performed in Gaussian 09W software.
Statistical Analysis
Results were expressed by means of values ± standard error of nine separate determinations (GraphPad Prism 8). Comparison of means was performed by one-way analysis of variance (ANOVA) (p < 0.05) followed by post hoc Tukey honestly significant difference (HSD) test (p < 0.01).
Results and Discussion
Centrifugal Partition Chromatography
The major phenolic compounds present in the extracts, isoastilbin, neoisoastilbin, and neoastilbin ((2R,3S)-dihydroquercetin 3-O-alpha-L-rhamnoside, (2S,3R)-dihydroquercetin 3-O-alpha-L-rhamnoside, (2S,3S)-dihydroquercetin 3-O-alpha-L-rhamnoside) were isolated using a countercurrent chromatography (CCC) machine, and a biphasic solvent system HEMWAT 1:9:1:9. The partition coefficient is the parameter that reflects the distribution of the solute between the two mutually balanced solvent phases. The ideal partition coefficient, for optimum CCC performance, must be a k value between 0.4 and 2.5 (Friesen and Pauli, 2005; Kumar, 2015). It is necessary to point out that to obtain an efficient separation and an adequate execution time the value k should be close to 1.0 (Kumar, 2015). On the one hand, in the event that the partition coefficient is much smaller than 1.0, the loss of the maximum resolution results as the solutes would be close to each other on the solvent front (Kumar, 2015). On the other hand, in the case that the value of k is much greater than 1, elution of the solutes in excessively wide peaks (band widening) would occur, which can lead to a prolonged elution time (Kumar, 2015). For all the above, the biphasic solvent system 1:9:1:9 (v/v) with a k value equal to 1.55 ± 009 was chosen (Table 1). This solvent system allowed to separate in a step the main compound present in the ethanolic extract of W. trichosperma.
HPLC-PDA-OT-MS
In this study, through HPLC-PDA-OT-MS analysis, twenty-five compounds were tentatively identified in the ethanolic extract of W. trichosperma bark (Figure 2). The structures of the compounds were proposed based on UV absorption and MS fragmentation patterns (Table 2). The detailed analysis is explained below.
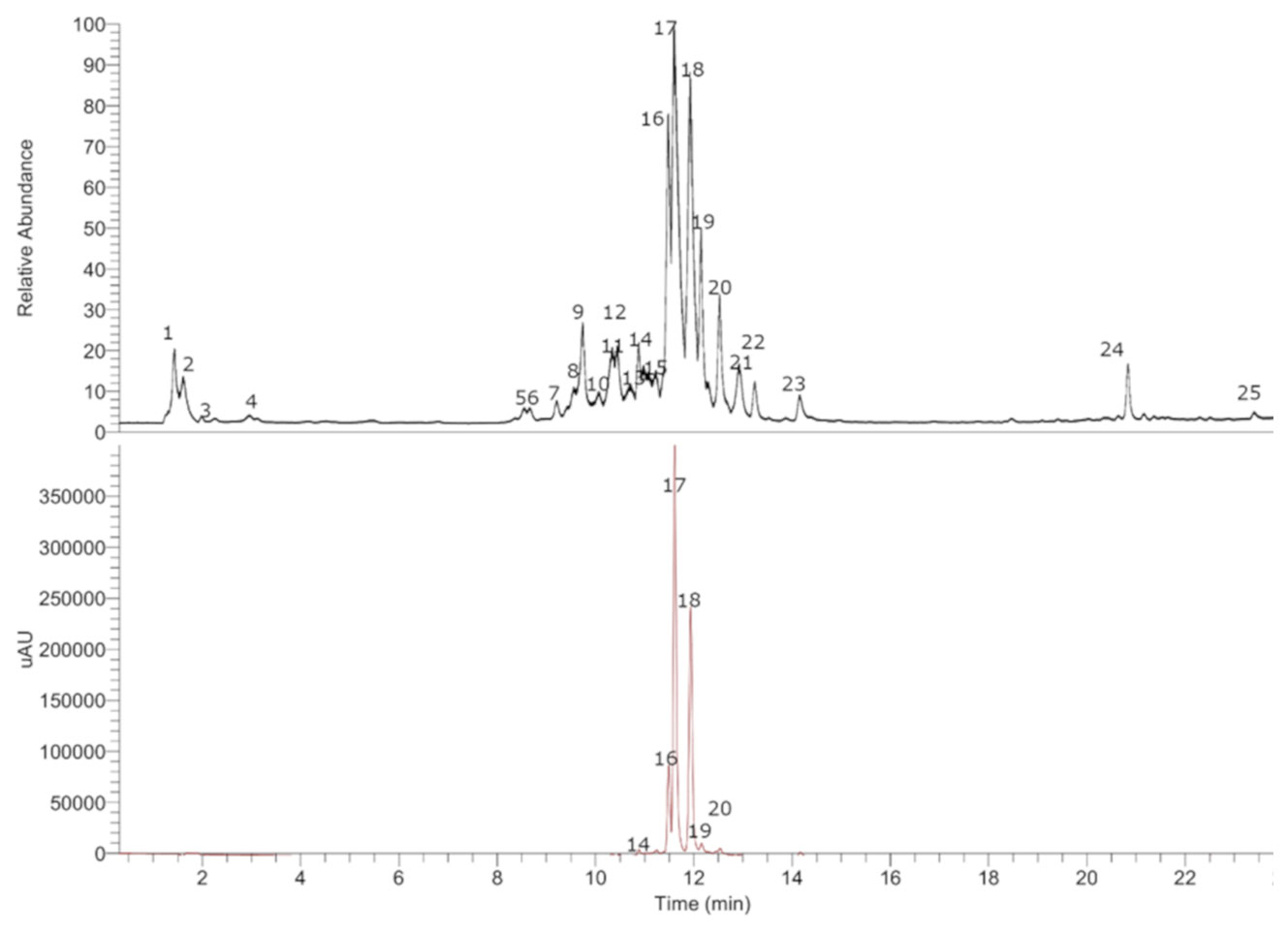
Figure 2 HPLC-ESI-MS/MS profile (black: TIC, red: UV at 280 nm) of the infusion of bark from W. trichosperma.
Phenolic Acids
Peak 1 was tentatively identified as bergapten (215.03389, C12H7O4-), (Acharya et al., 2019) peaks 2 and 3 as the isomers galloylglucose (331.06597, C15H13O6-) (Salminen et al., 2014), and galloylgalactose (C15H13O6-), respectively. Peak 4 with a M-H- ion at m/z: 169.01315 as gallic acid (C15H13O6-), Peak 5 as phlorigidoside B (463.14462 C19H27O13-) (Takeda et al., 2001), and finally peak 6 as verbasoside (461.16535 C20H29O12-) (Quirantes-Piné et al., 2009), while peak 24 was identified as pentyl benzoate, (435.09219, C20H19O11-).
Procyanidins
Peak 7 with a pseudomolecular ion at m/z: 577.13405 producing a catechol moiety at m/z: 285.06122 was identified as procyanidin B2 (C30H25O12−) (Glavnik and Vovk, 2019), while peak 9 as epicatequin (289.07199).
Iridoids
Peak 8 was identified as tudoside (417.10275, C17H22O12-)(Venditti et al., 2015), peak 11 as hedanthroside E. (483.11450, C21H23O13-)
Chalcones
Peak 10 with a M-H ion at m/z: 435.12936 (C21H23O10-) was identified as phlorizine (Liu et al., 2003)
Flavonoids
Peak 12 was identified as the flavanonol taxifolin-3-O-glucoside (C21H21O12−) (Sakushima et al., 2002) and peak 13 as its isomer helicioside A (Jia et al., 2018) and peak 15 as naringenin-7-O-pentoside (C20H19O11-). Peaks 20, 21, and 22 as naringenin-4′-O-glucoside, eriodictyol 7-(6´´-galloylglucoside) (C28H25O15-) and naringenin-7-O-glucoside (prunin, C28H25O15-) (Ali et al., 2019), respectively. Finally, Peak 24 was identified as kaempferol (C15H10O6-).
Flavanones
Peaks 16 to 19 were identified as neoastilbin, isoastilbin, neoisoastilbin, and astilbin (Figure 3). (C21H21O11-) (Zhang et al., 2013), respectively, using spiking experiments with standard compounds. The structural identification of isoastilbin was confirmed by NMR analysis (Supplementary Material, Figures S3–S9).
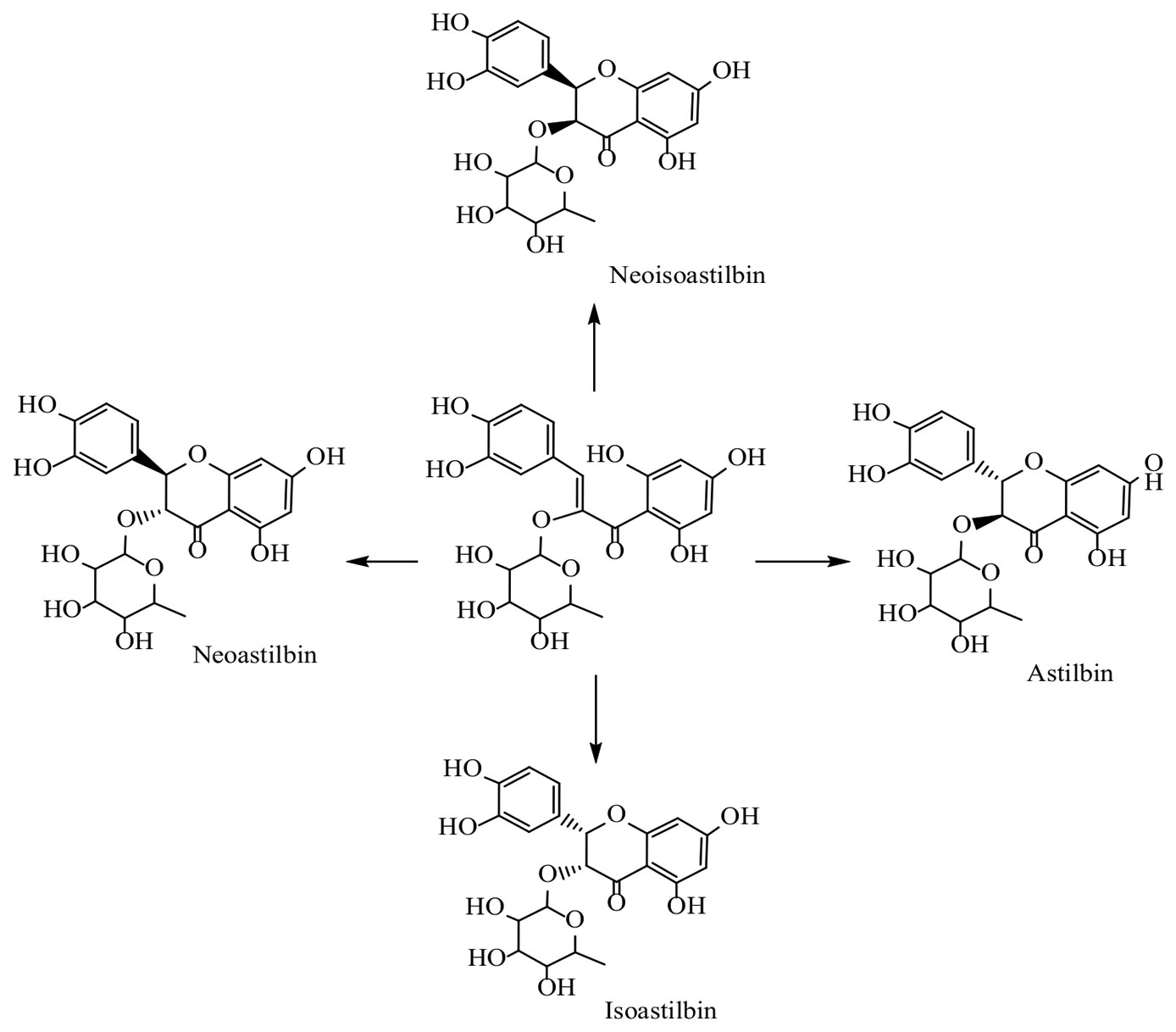
Figure 3 Biosynthetic relationship of the isomers of isoastilbin detected in the bark of W. trichosperma.
Coumarins
Peak 14 was identified as esculin (339.07239, C15H15O9-) (Qazi et al., 2018)
Sesquiterpenes
Peak 25 was regarded as polygodial (Barrosa et al., 2016).
HPLC-PDA Quantitation
Isomers of astilbin were quantified by HPLC-PDA and the methodology validated; (as seen in Supplementary Material, Tables S1–S6), the method was suitable to quantify isoastilbin and related phenolic compounds in the bark extract. In the infusion, four isomers were found but only three mayor isolated ones quantified, the highest amount found was for isoastilbin, followed by neoisoastilbin, and neoastilbin (141.7366 ± 1.18, 99.59 ± 0.83, and 53.1795 ± 0.31 mg/g extract, respectively), this makes the bark a good source for those bioactive isomer glycoside compounds.
Total Phenolic Content, Total Flavonoid Content, and Antioxidant Activity
Total phenolic content (TPC) and total flavonoid content (TFC) was measured of the aqueous extract of W. trichosperma to determine if the antioxidant activity was directly related to the content of these metabolites. The content of phenolic compounds in aqueous extracts of W. trichosperma was 442.1 ± 21.1 mg GAE g−1 dry plant (Table 3). The flavonoid content in aqueous extract was 1.554 ± 0.111 μg of quercetin equivalents per gram of dry plant. On the other hand, we used the ABTS•+ and DPPH assays to perform the assessment of the bleaching capacities of the aqueous extract of W. trichosperma and the major compound isolated, isoastilbin, the two assays showed a concentration-dependent increases in radical scavenging activity both the extract and isoastilbin, and we compared the data with the IC50 value of standard gallic acid for each method, as presented in Table 3, for ABTS assay the IC50 value for gallic acid was 0.09855 ± 0.00026 μg of gallic acid per milliliter and for DPPH assay was 0.4622 ± 0.0015 μg of gallic acid per milliliter. Also, for FRAP assay, the results were derived from a calibration curve of Trolox (y = 0.0019x + 0.0178, R2 = 0.997), for the aqueous extract this was 2.700 ± 0.554 mmol Trolox equivalent per gram of dry plant and for isoastilbin this was 0.1494 ± 0.0166 mmol Trolox equivalent per milligram of isoastilbin. For ORAC assay, the results support the hypothesis that the antioxidant activity is related with the content of the phenolic compounds present in the extract of the bark of W. trichosperma.
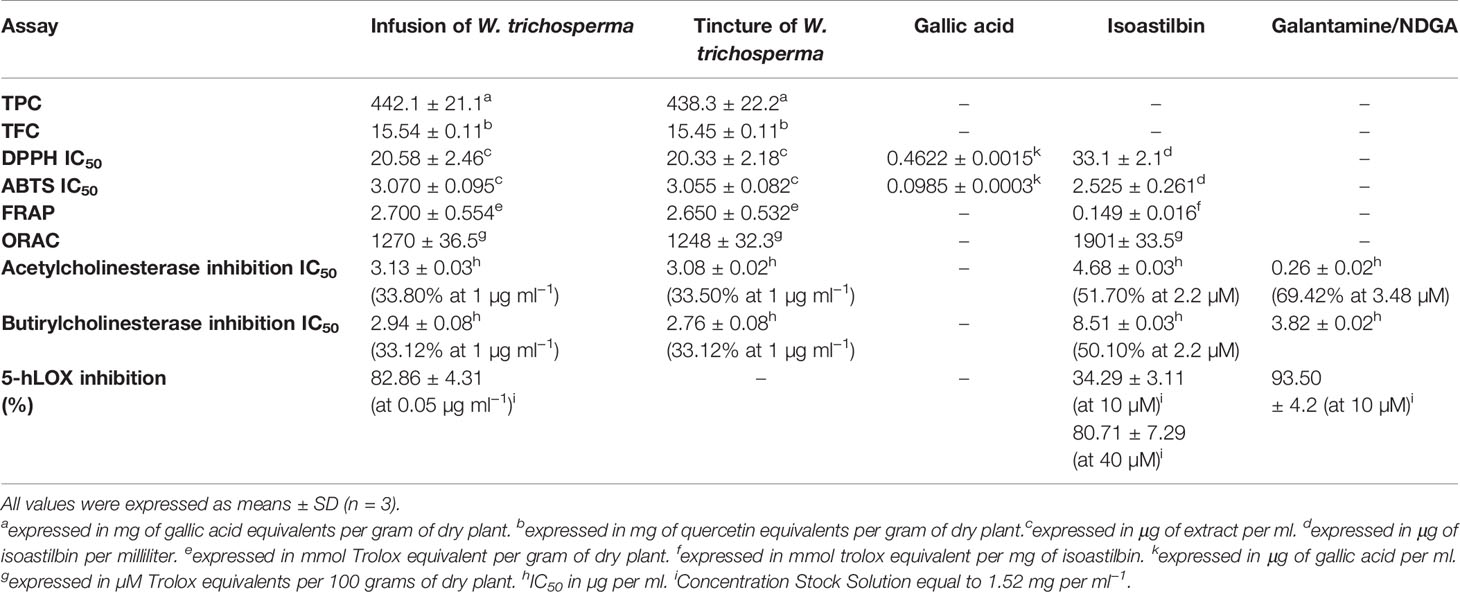
Table 3 Total phenolics, flavonoids, antioxidant, and inhibition of cholinesterase and 5-hLOX for infusion and tincture of W. trichosperma and the main isolated compound, isoastilbin.
In Vitro 5-hLOX and Cholinesterase Inhibitory Enzyme Assay
The application of enzyme inhibition therapeutic approaches is considered effective to control several health degenerative diseases, including Alzheimer’s disease, arthritis, and arthrosis. LOXs are a class of enzymes that catalyze the oxygenation of poly-unsaturated fatty acids in cis,cis-1,4-pentadiene structures, which are classified in order to their arachidonic acid oxidation specificity as 5, 8, 12, and 15-LOX. Indeed, the enzymes cyclooxygenase (COX), lipoxygenase (LOX), and matrix Metalloproteinase (MMP) are closely related to inflammatory responses, key factors in joint and arterial health. They are important key factors in immunity responses and pathological processes, such as wound healing, arthrosis, rheumatoid arthritis, pain, cancer, fever, and inflammatory reactions. On the other hand, the first therapeutic line for the treatment of degenerative Alzheimer’s disease are cholinesterase inhibitors (Howes et al., 2003). In this study, an in vitro inhibitory effect of the aqueous extract of W. trichosperma, on 5-hLOX, acetylcholinesterase, and butyrilcholinesterase enzymes was investigated.
The aqueous extract of W. trichosperma showed an interesting activity as 5-hLOX inhibitor of 82.86% (at 0.05 μg ml−1), while isolated isoastilbin was 34.29% (at 10 µM) and 80.71% (at 40 µM) (Table 3). Within the identified compounds, neoastilbin, isoastilbin, neoisoastilbin, and astilbin have shown anti-inflammatory activity through a mechanism that related to lipopolysaccharide-stimulated PGE(2) release, and Tumour Necrosis Factor alpha (TNF-α) and nitric oxide (NO) production from RAW 264.7 cells (Qureshi et al., 2011). In addition, Bergapten exhibited significant inhibition of the production of pro-inflammatory cytokines, namely, TNF-α and interleukin-6 (IL-6) by human peripheral blood mononuclear cells (PBMCs) stimulated with lipopolysaccharide in a concentration-dependent manner (Bose et al., 2011), and also exhibit an anti-inflammatory activity by inhibiting reactive oxygen species (ROS) and NO accumulation in the tail-cutting-induced inflammation zebrafish model (Yang et al., 2018b). Gallic acid suppresses the activation of nuclear factor kappa-light-chain-enhancer of activated B cells (NF-kB), COX-1, COX-2, p65 acetylation, Histone acetyltransferases (HAT), Intercellular adhesion molecule-1 (ICAM-1), Vascular cell adhesion molecule 1 (VCAM-1), TNF-α, interleukin-1β (IL-1β), IL-6, and inducible nitric oxide synthase (iNOS) (Kroes et al., 1992; Locatelli et al., 2013). Procyanidin B2 exerts anti-inflammatory activity and molecular mechanisms targeted include the modulation of various mediators of inflammation, including eicosanoids, cytokines, and NO, NLR Family Pyrin Domain Containing 3 (NLRP3) inflammasome, as well as the NF-κB and mitogen-activated protein kinase (MAPK) pathways (Martinez-Micaelo et al., 2012; Jiang et al., 2018). Epicatechin effectively attenuates the production of inflammatory mediators including NO, PGE2, TNF-α, IL-1β, and IL-6 in the LPS-induced macrophages through inactivation of NF-κB, MAPKs (ERK, JNK, and p38) and JAK2/STAT3 pathways (Yang et al., 2015). Esculin has potent anti-inflammatory activities in vivo and in vitro, which may involve the inhibition of the MAPK pathway (Niu et al., 2015). Kaempferol showed potent effect in the inhibition of inflammatory cell function as well as inhibition in the expression of pro-inflammatory cytokines and chemokines (Devi et al., 2015). Polygodial inhibited significantly the mouse paw edema induced by prostaglandin E2, bradykinin (BK), substance P (SP), dextran, platelet activating factor (PAF) or carrageenan, and also inhibited arachidonic acid-, capsaicin- and croton oil-induced ear edema in mice (da Cunha et al., 2001). Flavanones were regarded previously as anti-inflammatory showing inhibitory effects on lipopolysaccharide-stimulated PGE(2) release, and TNF-α and NO production from RAW 264.7 cells (Qureshi et al., 2011).
The inhibition of both the AChE and BChE cholinesterase enzymes is believed to be beneficial over inhibiting only one of the enzymes. The results of W. trichosperma inhibitory potencies of choline esterase (AChE and BChE) inhibitory activities are shown in Table 3 and expressed as IC50, particularly, AChE inhibition IC50 was 3.13 ± 0.03 µg ml−1 (33.8% at 1 µg ml−1) and BChE inhibition IC50 was 2.94 ± 0.08 µg ml−1 (33.12% at 1 µg ml−1) while its component isomer isoastilbin its IC50 was 4.68 ± 0.03 µg ml−1 (51.70% at 2.2 µM) and 8.51 ± 0.03 µg ml−1 (50.10% at 2.2 µM) for AChE and BChE, respectively.
Docking Studies
The isomers astilbin, isoastilbin, neoastilbin, and neoisoastilbin (Supplementary Material, Figure S10) contained in W. trichosperma as well as the known inhibitors of acetylcholinesterase (TcAChE), butyrylcholinesterase (hBuChE) and 5-lipoxygenase (5-hLOX), galantamine, and zileuton, were subjected to docking assays into their catalytic sites. The best docking binding energies expressed in kcal/mol of each compound are shown in Table 4. By the analysis of docking poses in this enzyme, astilbin and isoastilbin are arranged in similar manners with a slightly tilt between their molecular structures. Indeed, the 5,7-dihydroxychroman-4-one, dihydroxyphenyl, and glycoside portions are quite overlapped, which could explain the similarities in their binding energy values (−8.92 and −8.59 kcal mol−1, respectively). The relative superposition between astilbin and isoastilbin mentioned above results in that both isomers interacts with some same amino acids of the acetylcholinesterase catalytic site (Supplementary Material, Figures S11A, B). In this sense, the hydroxyl groups (–OH) at position 7- of the 5,7-dihydroxychroman-4-one frameworks, one of the hydroxyl groups (–OH) of the glycosides moieties and one of the hydroxyl groups (–OH) of the dihydroxyphenyl cores of both astilbin and isoastilbin molecules showed hydrogen bond interactions with the amino acids Glu199, Tyr121, and Asn85, respectively. The only difference between the aforementioned interactions of these two molecules is specifically in the Hydrogen bond interaction performed by the hydroxyl group (–OH) of the glycoside core in astilbin, which take place between the oxygen atom of the saccharide and the hydrogen atom of Tyr121, whereas in isoastilbin occurs exactly the opposite. Figure 4 shows the Hydrogen bond interactions in a two-dimensional diagram of isoastilbin binding mode into every catalytic site of the three studied enzymes as a pure and sole inhibitor in experimental inhibition assays. Binding energy data of flavonoids astilbin, isoastilbin, and neoastilbin obtained from docking assays over acetylcholinesterase shown in Table 4, exhibited similar values. This suggest that all these isomers possess similar ability to inhibit this enzyme. The similar binding energy values of these three compounds could explain why the experimental IC50 of the W. trichosperma aqueous extract and the IC50 of the isolated isoastilbin showed such close values (4.68 ± 0.03 and 3.13 ± 0.32 µg ml−1, respectively). On the other hand, neoisoastilbin presented the best binding energy profile, which could lead to a higher inhibitory potency if this compound were isolated and tested as a single inhibitor. Therefore, since the W. trichosperma aqueous extract contain the four isomers (astilbin, isoastilbin, neoastilbin, and neoisoastilbin) the eventual higher potency of neoisoastilbin compared to the other three isomers, it cannot be detected due the existing competition among all flavonoids for the acetylcholinesterase catalytic site.

Table 4 Binding energies obtained from docking experiments of astilbin, isoastilbin, neoastilbin, neoisoastilbin, and the respective known enzyme inhibitors galantamine and zileuton over acetylcholinesterase (TcAChE) (Supplementary Material, Figure S11), butyrylcholinesterase (hBuChE) (Supplementary Material, Figure S12) and 5-lipoxygenase (5- hLOX) (Supplementary Material, Figure S13).
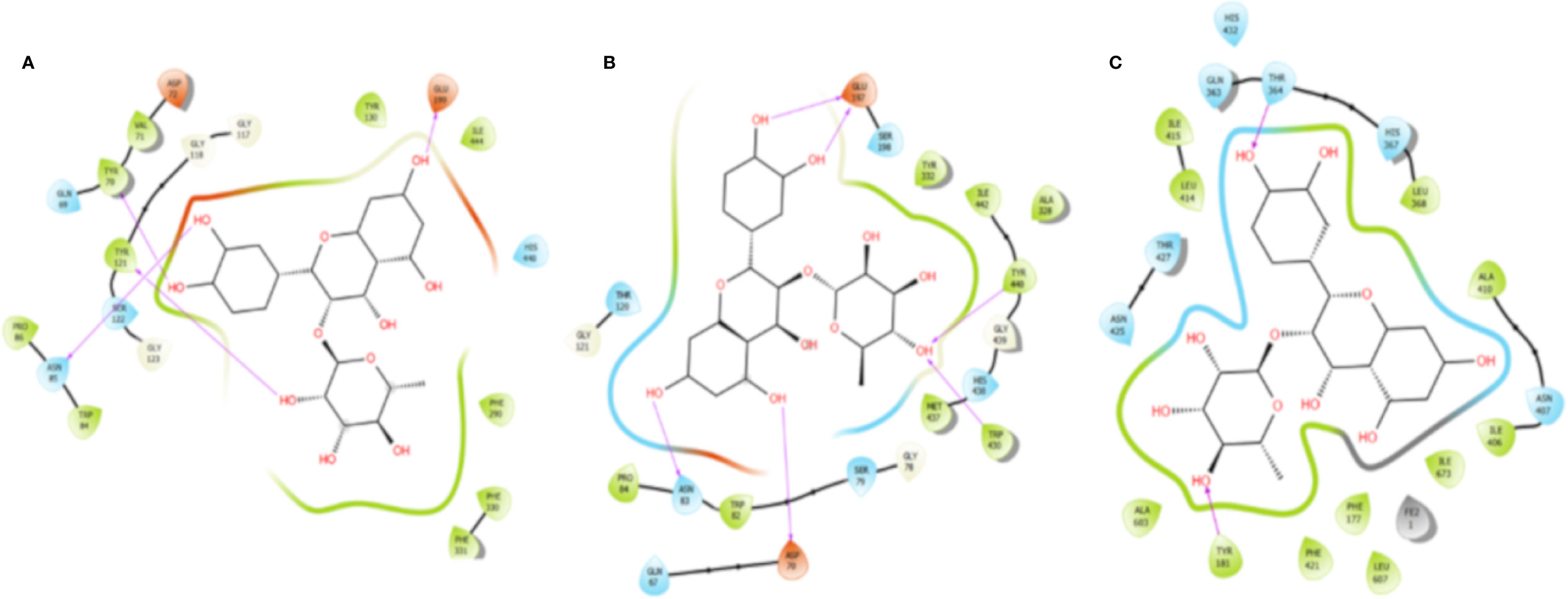
Figure 4 Hydrogen bond interactions showed by isoastilbin into the catalytic site of the three studied enzymes. (A) Isoastilbin into acetylcholinesterase (TcAChE) catalytic site (B) Isoastilbin into butyrylcholinesterase (hBuChE) catalytic site. (C) Isoastilbin into 5-lipoxygenase (5- hLOX) catalytic site. Magenta arrows represent H-bondings with the different amino acids.
Conclusions
This study deals with the antioxidant, and enzyme inhibitory effects of Weinmannia trichosperma, in order to find new endemic and underutilized sources of natural products for potential applications in human health. This study makes new contributions to the chemistry of this plant, not previously described. The CPC machine was useful for the isolation of the major compounds while the HPLC-MS allowed the identification of several phenolic constituents. In this study, the assessment of antioxidant activity indicates that the bark could be an important source of natural antioxidants and inhibitory compounds for potential use in neurodegenerative diseases. Hence, W. trichosperma bark could be recommended for the formulation of nutraceuticals or antioxidant-rich therapeutic diets and for its enzyme inhibitory properties it can have potential applications in drug industry for the develop of treatment for Alzheimer’s disease, as well as to alleviate inflammatory processes involved in other pathologies and diseases. However, more detailed in vivo studies are needed to evaluate the safety and efficacy of the bark.
Data Availability Statement
All datasets generated for this study are included in the article/Supplementary Material.
Author Contributions
RB and EP performed isolation by CPC. MS and CF-G performed LC-MS experiments and analysis. RB and SA performed quantitation by HPLC-PDA. JE performed the NMR analysis. SA and CF-G performed the enzymatic experiments and analysis. JR-P performed the docking studies. MS, JE, and EP wrote the manuscript.
Funding
This research received funds from FONDECYT regular 1180059, Postdoctorado grants 3190794, and 3190572, and doctorado grant CONICYT PFCHA/beca doctorado nacional/2019-21191978. JE gratefully acknowledges funding from CONICYT(PAI/ACADEMIA N° 79160109).
Conflict of Interest
The authors declare that the research was conducted in the absence of any commercial or financial relationships that could be construed as a potential conflict of interest.
Acknowledgments
We acknowledge Milena Rios for her help in in vitro microplate assays.
Supplementary Material
The Supplementary Material for this article can be found online at: https://www.frontiersin.org/articles/10.3389/fphar.2020.00780/full#supplementary-material
Abbreviations
AAPH, 2,2′-Azobis(2-amidinopropane) dihydrochloride; ABTS, 2,2′-Azino-bis(3-ethylbenzothiazoline-6-sulfonic acid); AChE, Acetylcholinesterase; ANOVA, One-way analysis of variance; ATCI, Acetyl-thiocholine iodide; AUC, Area under curve; BChE, Butyrylcholinesterase; BK, Bradykinin; BTC1, Butyryl-thiocholine chloride; CCC, Counter-current chromatography; COX, Cyclooxygenase; CPC, Centrifugal partition chromatography; CT, Cycle time; DPPH, 2,2,1-diphenyl-1-picrylhydrazyl; DTNB, 5-dithio-bis(2-nitrobenzoic) acid; ESI, Electrospray ionization; f3, Fluorescence reading at cycle 3; FL, Fluorescein disodium; Fn, Fluorescence reading at cycle n; FRAP, Ferric Reducing Antioxidant Power; FWHM, Full width at half maximum; GAE, Gallic acid equivalent; h5-LOX, Human 5-lipoxygenase; HAT, Histone acetyltransferases; HEPES, (4-(2-hydroxyethyl)-1-piperazineethanesulfonic acid); HEMWAT, Hexane-ethyl acetate-methanol-water system; HSD, Honestly significant difference; IC50, Half maximal inhibitory concentration; ICAM-1, Intercellular adhesion molecule-1; ICH, International Council for Harmonization of Technical Requirements for Pharmaceuticals for Human Use; IL-1β, Interleukin-1β; IL-6, Interleukin-6; iNOS, Inducible Nitric Oxide Synthase; k, Partition coefficient; LOD, Limit of detection; LOQ, Limit of quantification; MAPK, mitogen-activated protein kinase; MMP, Matrix Metalloproteinase; MS, mass spectrometry; NF-kB, Nuclear factor kappa-light-chain-enhancer of activated B cells; NLRP3, NLR Family Pyrin Domain Containing 3; NO, Nitric oxide; ORAC, Oxygen radical absorbance capacity; OT, Orbitrap technology; PAF, Platelet activating factor; PBMC, Human peripheral blood mononuclear cells; PDA, Photodiode Array Detection; PTFE, Polytetrafluoroethylene; QE, Quercetin equivalent; ROS, Reactive oxygen species; RP, Reverse phase; RSD, Relative standard deviation; SD, Standard deviation; SP, Substance P; TE, Trolox equivalents; TEAC, Trolox equivalent antioxidant capacity; TFC, Total Flavonoid Content; TIC, Total Ion Chromatogram; TNF-α, Tumour Necrosis Factor alpha; TPC, Total Phenolic Content; TPTZ, 2,4,6-tri(2-pyridyl)-s-triazine; Trolox, 6-hydroxy-2,5,7,8-tetramethylchromane-2-carboxylic acid; UV, Ultraviolet; UHPLC, Ultra high performance chromatography; VCAM-1, Vascular cell adhesion molecule 1.
References
Acharya, R., Chacko, S., Bose, P., Lapenna, A., Pattanayak, S. P. (2019). Structure Based Multitargeted Molecular Docking Analysis of Selected Furanocoumarins against Breast Cancer. Sci. Rep. 9, 15743. doi: 10.1038/s41598-019-52162-0
Adamo, C., Barone, V. (1999). Toward reliable density functional methods without adjustable parameters: The PBE0 model Seeking for parameter-free double-hybrid functionals: The PBE0-DH model Accurate excitation energies from time-dependent density functional theory: Assessing the PBE0 model Toward reliable density functional methods without adjustable parameters: The PBE0 model. Cit. J. Chem. Phys. 110, 2889. doi: 10.1063/1.478522
Ali, M. Y., Jannat, S., Edraki, N., Das, S., Chang, W. K., Kim, H. C., et al. (2019). Flavanone glycosides inhibit β-site amyloid precursor protein cleaving enzyme 1 and cholinesterase and reduce Aβ aggregation in the amyloidogenic pathway. Chem. Biol. Interact. 309, 108707. doi: 10.1016/j.cbi.2019.06.020
Barrosa, K. H., Mecchi, M. C., Rando, D. G., Ferreira, A. J. S., Sartorelli, P., Valle, M. M. R., et al. (2016). Polygodial, a sesquiterpene isolated from Drimys brasiliensis (Winteraceae), triggers glucocorticoid-like effects on pancreatic β-cells. Chem. Biol. Interact. 258, 245–256. doi: 10.1016/j.cbi.2016.09.013
Bhaigyabati, T., Devi, P. G., Devi, N. R., Bag, G. C. (2017). Antioxidant activity, total phenolic and total flavonoid content of Oenanthe javanica Blume (DC) collected from Imphal West District. Int. Reseach J. Pharm. 8, 63–68. doi: 10.7897/2230-8407.08698
Borman, P., Elder, D. (2018). “Q2(R1) Validation of Analytical Procedures: Text and Methodology,” in ICH Quality Guidelines: An Implementation Guide. Eds. Teasdale, A., Elder, D., Nims, R., John Wiley & Sons, Inc. 127–166.
Bose, S. K., Dewanjee, S., Sahu, R., Dey, S. P. (2011). Effect of bergapten from Heracleum nepalense root on production of proinflammatory cytokines. Nat. Prod. Res. 25, 1444–1449. doi: 10.1080/14786410902800665
Brand-Williams, W., Cuvelier, M. E., Berset, C. (1995). Use of a free radical method to evaluate antioxidant activity. LWT - Food Sci. Technol. 28, 25–30. doi: 10.1016/S0023-6438(95)80008-5
Collins, P., Diehl, H., Smith, F. (1959). 2,4,6-Tripyridyl-s-triazine as a Reagent for Iron Determination of Iron in Limestone, Silicates, and Refractories. Anal. Chem. 31, 1862–1867. doi: 10.1021/ac60155a056
da Cunha, F. M., Fröde, T. S., Mendes, G. L., Malheiros, A., Cechinel Filho, V., Yunes, R. A., et al. (2001). Additional evidence for the anti-inflammatory and anti-allergic properties of the sesquiterpene polygodial. Life Sci. 70, 159–169. doi: 10.1016/S0024-3205(01)01387-X
Deng, H., Wang, H., Liang, M., Su, X. (2019). A novel approach based on supramolecular solvent microextraction and UPLC-Q-Orbitrap HRMS for simultaneous analysis of perfluorinated compounds and fluorine-containing pesticides in drinking and environmental water. Microchem. J. 151, 104250. doi: 10.1016/j.microc.2019.104250
Devi, K. P., Malar, D. S., Nabavi, S. F., Sureda, A., Xiao, J., Nabavi, S. M., et al. (2015). Kaempferol and inflammation: From chemistry to medicine. Pharmacol. Res. 99, 1–10. doi: 10.1016/j.phrs.2015.05.002
Friesen, J. B., Pauli, G. F. (2005). G.U.E.S.S. - A generally useful estimate of solvent systems in CCC. J. Liq. Chromatogr. Relat. Technol. 28, 2777–2806. doi: 10.1080/10826070500225234
Friesen, J. B., McAlpine, J. B., Chen, S. N., Pauli, G. F. (2015). Countercurrent Separation of Natural Products: An Update. J. Nat. Prod. 78, 1765–1796. doi: 10.1021/np501065h
Glavnik, V., Vovk, I. (2019). High performance thin-layer chromatography–mass spectrometry methods on diol stationary phase for the analyses of flavan-3-ols and proanthocyanidins in invasive Japanese knotweed. J. Chromatogr. A 1598, 196–208. doi: 10.1016/j.chroma.2019.03.050
Gusinde, M. (1936). Plantas medicinales que los indios Araucanos recomiendan.(Fin). Anthropos, 850–873.
Holler, J. G., Søndergaard, K., Slotved, H.-C., Gúzman, A., Mølgaard, P. (2012). Evaluation of the antibacterial activity of Chilean plants traditionally used for wound healing therapy against multidrug-resistant Staphylococcus aureus. Planta Med. 78, 200–205. doi: 10.1055/s-0031-1280316
Houghton, P., Manby, J. (1985). Medicinal plants of the mapuche. J. Ethnopharmacol. 13, 89–103. doi: 10.1016/0378-8741(85)90063-7
Howes, M.-J. R., Perry, N. S. L., Houghton, P. J. (2003). Plants with traditional uses and activities, relevant to the management of Alzheimer’s disease and other cognitive disorders. Phyther. Res. 17, 1–18. doi: 10.1002/ptr.1280
Ito, Y. (2005). Golden rules and pitfalls in selecting optimum conditions for high-speed counter-current chromatography. J. Chromatogr. A. (2), 145–168. doi: 10.1016/j.chroma.2004.12.044
Jia, X., Xie, H., Jiang, Y., Wei, X. (2018). Flavonoids isolated from the fresh sweet fruit of Averrhoa carambola, commonly known as star fruit. Phytochemistry 153, 156–162. doi: 10.1016/j.phytochem.2018.06.007
Jiang, Y., Yang, W., Gui, S. (2018). Procyanidin B2 protects rats from paraquat-induced acute lung injury by inhibiting NLRP3 inflammasome activation. Immunobiology 223, 555–561. doi: 10.1016/j.imbio.2018.07.001
Kowalska, T., Sherma, J., Waksmundzka-Hajnos, M. (2007). ““Overview of the Field of TLC in Phytochemistry and the Structure of the Book,”,” in Thin Layer Chromatography in Phytochemistry. Eds. Wkamundzka-Hajnos, M., Sherma, J., Kowalska, T. (Boca Raton, FL:CRC Press), 3–14. doi: 10.1201/9781420046786.pt1
Kroes, B. H., Van den Berg, A. J. J., Van Ufford, H. C. Q., Van Dijk, H., Labadie, R. P. (1992). Anti-inflammatory activity of gallic acid. Planta Med. 58, 499–504. doi: 10.1055/s-2006-961535
Kumar, S. (2015). Analytical Techniques for Natural Product Research. Ed. Nicki, D. (Boston, MA: CABI).
Kuskoski, E. M., Asuero, A. G., Troncoso, A. M., Mancini-Filho, J., Fett, R. (2005). Aplicación de diversos métodos químicos para determinar actividad antioxidante en pulpa de frutos. Ciência e Tecnol. Aliment. 25 (4), 726–732. doi: 10.1590/S0101-20612005000400016
Liu, H., Qu, G., Yao, X. (2003). The polyphenolic constituents of Engelhardtia serrata stem bark. Pharm. Biol. 41, 305–307. doi: 10.1076/phbi.41.4.305.15675
Liu, Y., Friesen, J., McAlpine, J., Pauli, G. (2015). Solvent System Selection Strategies in Countercurrent Separation. Planta Med. 81, 1582–1591. doi: 10.1055/s-0035-1546246
Locatelli, C., Filippin-Monteiro, F. B., Centa, A., Creczinsky-Pasa, T. B. (2013). Antioxidant, antitumoral and anti-inflammatory activities of gallic acid. Handb. Gall. Acid Nat. Occur. Antioxid. Prop. Heal. Implic. Ed.). Nov. Publ., 1–23.
Luna, L., Simirgiotis, M. J., Lima, B., Bórquez, J., Feresin, G. E., Tapia, A. (2018). UHPLC-MS metabolome fingerprinting: The isolation of main compounds and antioxidant activity of the andean species Tetraglochin ameghinoi (Speg.) Speg. Molecules 23, 793. doi: 10.3390/molecules23040793
Marston, A., Hostettmann, K. (2006). Developments in the application of counter-current chromatography to plant analysis. J. Chromatogr. A 1112, 181–194. doi: 10.1016/j.chroma.2005.10.018
Martinez-Micaelo, N., González-Abuín, N., Ardèvol, A., Pinent, M., Blay, M. T. (2012). Procyanidins and inflammation: Molecular targets and health implications. Biofactors 38, 257–265. doi: 10.1002/biof.1019
Mocan, A., Zengin, G., Simirgiotis, M., Schafberg, M., Mollica, A., Vodnar, D. C., et al. (2017). Functional constituents of wild and cultivated Goji ( L. barbarum L.) leaves: phytochemical characterization, biological profile, and computational studies. J. Enzyme Inhib. Med. Chem. 32, 153–168. doi: 10.1080/14756366.2016.1243535
Mølgaard, P., Holler, J. G., Asar, B., Liberna, I., Rosenbæk, L. B., Jebjerg, C. P., et al. (2011). Antimicrobial evaluation of Huilliche plant medicine used to treat wounds. J. Ethnopharmacol. 138, 219–227. doi: 10.1016/j.jep.2011.09.006
Muñoz, M., Barrera, E., Meza, I. (1981). El uso medicinal y alimenticio de plantas nativas y naturalizadas en Chile. Mus. Nac. Hist. Nat. 33, 91.
Niu, X., Wang, Y., Li, W., Zhang, H., Wang, X., Mu, Q., et al. (2015). Esculin exhibited anti-inflammatory activities in vivo and regulated TNF-α and IL-6 production in LPS-stimulated mouse peritoneal macrophages in vitro through MAPK pathway. Int. Immunopharmacol. 29, 779–786. doi: 10.1016/j.intimp.2015.08.041
Nwokocha, C., Palacios, J., Simirgiotis, M. J., Thomas, J., Nwokocha, M., Young, L., et al. (2017). Aqueous extract from leaf of Artocarpus altilis provides cardio-protection from isoproterenol induced myocardial damage in rats: Negative chronotropic and inotropic effects. J. Ethnopharmacol. 203, 163–170. doi: 10.1016/j.jep.2017.03.037
Petersson, G. A., Bennett, A., Tensfeldt, T. G., Al-Laham, M. A., Shirley, W. A., Mantzaris, J. (1988). A complete basis set model chemistry. I. The total energies of closed-shell atoms and hydrides of the first-row elements. J. Chem. Phys. 89, 2193–2218. doi: 10.1063/1.455064
Qazi, S. S., Lombardo, D. A., Abou-Zaid, M. M. (2018). A metabolomic and HPLC-MS/MS analysis of the foliar phenolics, flavonoids and coumarins of the Fraxinus species resistant and susceptible to emerald ash borer. Molecules 23, 2734. doi: 10.3390/molecules23112734
Quirantes-Piné, R., Funes, L., Micol, V., Segura-Carretero, A., Fernández-Gutiérrez, A. (2009). High-performance liquid chromatography with diode array detection coupled to electrospray time-of-flight and ion-trap tandem mass spectrometry to identify phenolic compounds from a lemon verbena extract. J. Chromatogr. A 1216, 5391–5397. doi: 10.1016/j.chroma.2009.05.038
Qureshi, A. A., Tan, X., Reis, J. C., Badr, M. Z., Papasian, C. J., Morrison, D. C., et al. (2011). Inhibition of nitric oxide in LPS-stimulated macrophages of young and senescent mice by δ-tocotrienol and quercetin. Lipids Health Dis. 10, 239. doi: 10.1186/1476-511X-10-239
Re, R., Pellegrini, N., Proteggente, A., Pannala, A., Yang, M., Rice-Evans, C. (1999). Antioxidant activity applying an improved ABTS radical cation decolorization assay. Free Radic. Biol. Med. 26, 1231–1237. doi: 10.1016/S0891-5849(98)00315-3
Rodriguez, R., Marticorena, C., Alarcón, D., Baeza, C., Cavieres, L., Finot, V. L., et al. (2018). Catálogo de las plantas vasculares de Chile. Gayana. Botánica 75, 1–430. doi: 10.4067/S0717-66432018000100001
Sakushima, A., Ohno, K., Coskun, M., Seki, K. I., Ohkura, K. (2002). Separation and identification of taxifolin 3-O-glucoside isomers from Chamaecyparis obtusa (Cupressaceae). Nat. Prod. Lett. 16, 383–387. doi: 10.1080/10575630290033141
Salminen, J. P., Shikov, A. N., Karonen, M., Pozharitskaya, O. N., Kim, J., Makarov, V. G., et al. (2014). Rapid profiling of phenolic compounds of green and fermented Bergenia crassifolia L. leaves by UPLC-DAD-QqQ-MS and HPLC-DAD-ESI-QTOF-MS. Nat. Prod. Res. 28, 1530–1533. doi: 10.1080/14786419.2014.923999
Simirgiotis, M. J., Quispe, C., Bórquez, J., Mocan, A., Sepúlveda, B. (2016). High resolution metabolite fingerprinting of the resin of Baccharis tola Phil. from the Atacama Desert and its antioxidant capacities. Ind. Crops Prod. 94, 368–375. doi: 10.1016/j.indcrop.2016.08.037
Skalicka-Woźniak, K., Garrard, I. (2014). Counter-current chromatography for the separation of terpenoids: A comprehensive review with respect to the solvent systems employed. Phytochem. Rev. 13, 547–572. doi: 10.1007/s11101-014-9348-2
Takeda, Y., Isai, N., Masuda, T., Honda, G., Takaishi, Y., Ito, M., et al. (2001). Phlomisflavosides A and B, new flavonol bisglycosides from Phlomis spinidens. Chem. Pharm. Bull. 49, 1039–1041. doi: 10.1248/cpb.49.1039
Venditti, A., Guarcini, L., Ballero, M., Bianco, A. (2015). Iridoid glucosides from Pentas lanceolata (Forssk.) Deflers growing on the Island of Sardinia. Plant Syst. Evol. 301, 685–690. doi: 10.1007/s00606-014-1106-9
Wu, X., Beecher, G. R., Holden, J. M., Haytowitz, D. B., Gebhardt, S. E., Prior, R. L. (2004). Lipophilic and Hydrophilic Antioxidant Capacities of Common Foods in the United States. J. Agric. Food Chem. 52, 4026–4037. doi: 10.1021/jf049696w
Yang, D., Liu, S., Chen, Y., Hsu, S., Chang, Y., Lin, J. (2015). Three pathways assess anti-inflammatory response of epicatechin with lipopolysaccharide-mediated macrophage RAW 264.7 Cells. J. Food Biochem. 39, 334–343. doi: 10.1111/jfbc.12134
Yang, H., Yang, C., Sun, T. (2018a). Characterization of glycopeptides using a stepped higher-energy C-trap dissociation approach on a hybrid quadrupole orbitrap. Rapid Commun. Mass Spectrom. 32, 1353–1362. doi: 10.1002/rcm.8191
Yang, Y., Zheng, K., Mei, W., Wang, Y., Yu, C., Yu, B., et al. (2018b). Anti-inflammatory and proresolution activities of bergapten isolated from the roots of Ficus hirta in an in vivo zebrafish model. Biochem. Biophys. Res. Commun. 496, 763–769. doi: 10.1016/j.bbrc.2018.01.071
Keywords: Weinmannia trichosperma, tineo, astilbin, traditional medicine, HPLC-MS, centrifugal partition chromatography
Citation: Barrientos R, Fernández-Galleguillos C, Pastene E, Simirgiotis M, Romero-Parra J, Ahmed S and Echeverría J (2020) Metabolomic Analysis, Fast Isolation of Phenolic Compounds, and Evaluation of Biological Activities of the Bark From Weinmannia trichosperma Cav. (Cunoniaceae). Front. Pharmacol. 11:780. doi: 10.3389/fphar.2020.00780
Received: 13 March 2020; Accepted: 11 May 2020;
Published: 27 May 2020.
Edited by:
Andrei Mocan, Iuliu Hațieganu University of Medicine and Pharmacy, RomaniaReviewed by:
Víctor López, Universidad San Jorge, SpainLuigi Menghini, University “G. d’Annunzio” of Chieti-Pescara, Italy
Copyright © 2020 Barrientos, Fernández-Galleguillos, Pastene, Simirgiotis, Romero-Parra, Ahmed and Echeverría. This is an open-access article distributed under the terms of the Creative Commons Attribution License (CC BY). The use, distribution or reproduction in other forums is permitted, provided the original author(s) and the copyright owner(s) are credited and that the original publication in this journal is cited, in accordance with accepted academic practice. No use, distribution or reproduction is permitted which does not comply with these terms.
*Correspondence: Mario Simirgiotis, bWFyaW8uc2ltaXJnaW90aXNAdWFjaC5jbA==; Javier Echeverría, amF2aWVyLmVjaGV2ZXJyaWFtQHVzYWNoLmNs