- 1Department of Toxicology, School of Public Health, Qingdao University, Qingdao, China
- 2Department of Toxicology, School of Public Health, Sun Yat-sen University, Guangzhou, China
- 3Department of Pharmacology, School of Pharmacy, Qingdao University, Qingdao, China
- 4Department of Occupational Diseases, Occupational Disease Center, Qingdao Central Hospital, Qingdao, China
Particulate matter poses health risk to developing organisms. To investigate particulate matters with a diameter smaller than 2.5 um (PM2.5)-induced developmental cardiotoxicity, fertile chicken eggs were exposed to PM2.5 via air cell injection at doses of 0.05, 0.2, 0.5, 2, and 5 mg/egg kg. Morphological changes in the embryonic day four (ED4) and hatchling hearts were assessed with histological techniques. Heart rates of hatchling chickens were measured with electrocardiography. The protein expression levels of nuclear factor kappa-light-chain-enhancer of activated B cells p65 (NF-kb p65), inducible nitric oxide synthase (iNOS), and matrix metallopeptidase 9 (MMP9) were assessed with immunohistochemistry or western blotting in hatchling hearts. PM2.5 exposure elevated areas of heart in ED4 embryo, increased heart rate, and thickened right ventricular wall thickness in hatchling chickens. Immunohistochemistry revealed enhanced NF-kb p65 expression in hatchling hearts. Western blotting results indicated that both iNOS and MMP9 expression were enhanced by lower doses of PM2.5 exposure (0.2 and 0.5 mg/kg) but not 2 mg/kg. In summary, developmental exposure to PM2.5 induced developmental cardiotoxicity in chicken embryo and hatchling chickens, which is associated with NF-kb p65, iNOS, and MMP9.
Introduction
Air pollution, especially particulate matter (PM) pollution, is a significant health risk factor for human beings (Yu G. et al., 2019). Generated from multiple resources: coal combustions, diesel exhausts, biomass burning, etc. (Cho et al., 2018; Leepe et al., 2019), PM pollution affects large area across the world (Giannadaki et al., 2016; Wang et al., 2019a). Some areas have relatively high levels, far beyond the healthy levels determined by the world health organization, posing a significant threat to human health (Giannadaki et al., 2016).
PM may be categorized according to the size of the particles. Among them, PM2.5 (particulate matters with a diameter smaller than 2.5 μm) attracts most attentions, since the small size of PM2.5 allows inhalation into deeper, even into systemic circulation, thus may induce detrimental health effects to the cardiovascular system, pulmonary system, etc. (Wilson and Suh, 1997; Xing et al., 2016; Chen et al., 2017). Among the negative outcomes associated with PM2.5 exposure, developmental toxicity is an important one, yet received relatively less attention. PM2.5 has been demonstrated to be capable of passing the placenta barrier (Teng et al., 2016) and expose developing embryo/fetus. Epidemiological data has associated in utero exposure to PM2.5 with developmental cardiotoxicity (Chen S. et al., 2019) and neurotoxicity (Lubczynska et al., 2017). However, a precise mode of toxicity and molecular mechanism for the developmental toxicity induced by PM2.5 in utero exposure is not yet available. Further investigation is urgently needed for better understanding of PM2.5-induced developmental toxicity and risk assessments.
Chicken embryo has been used as a development model for over 200 years (Vergara and Canto-Soler, 2012), which allows direct observation and manipulation of developing embryo. Moreover, chicken embryo is a closed system, allowing relatively accurate exposure to toxicants without the interference of maternal effects. In the current study, chicken embryo model was utilized to assess the potential developmental toxicities induced by PM2.5 exposure. PM2.5 was exposed to the chicken embryos via air cell injection, by which the PM2.5 was injected onto the air cell membrane without direct penetration into the egg. This method has been demonstrated to elicit comparable results to real-world exposure to environmental contaminants (Hoffman et al., 1996). Early (embryonic day four, ED4) and late development stages (hatchling chickens) were both investigated for a more comprehensive understanding of the developmental toxicity at different stages of development. For early development assessment, ED4 embryos were used, since the embryos are undergoing organogenesis at this stage, in which the vital organs such as brain, heart, and eyes are visible, but the organogenesis is still going on, and defects may be visible in response to extragenous disruptions (Vergara and Canto-Soler, 2012; Vancamp and Darras, 2017; Wittig and Munsterberg, 2019). For late development assessment, hatchling chickens were selected, since most organ systems finished development at this stage.
One major proposed mechanism of toxicity for the PM2.5 is the inflammatory response, which may contribute to the negative effects of PM2.5 in the cardiovascular system, pulmonary system, and nervous system (Zhao et al., 2013). Among the signaling molecules regulating inflammatory response, nuclear factor kappa-light-chain-enhancer of activated B cells (NF-kb) is a major one, which plays role in the production of cytokines, chemokines, and growth factors regulating the expression of genes involved in the immune and inflammatory responses (Frode-Saleh and Calixto, 2000). Its downstream signaling molecules include inducible nitric oxide synthase (iNOS) and matrix metallopeptidase 9 (MMP9). iNOS plays roles in the production of excessive amounts of nitric oxide (NO), while MMP9 participates in the degradation of the extracellular matrix during tissue remodeling (Lee et al., 2012; Wilmes et al., 2020). PM2.5 exposure has been associated with the stimulation of inflammatory reaction, but specific mechanism had not been fully elucidated yet. In the current study, expression levels of NF-kb, iNOS, and MMP9 were investigated in PM2.5-exposed hatchling chicken heart tissues, further revealing the roles of these molecules in PM2.5-induced developmental toxicity. This study adds to the knowledge base of PM2.5-exposure induced developmental toxicities and provided information about the potential molecular mechanisms.
Materials and Methods
Animals
Fertile chicken eggs were purchased from Linwen Trade Corp (Jining, China). Prior to incubation, the eggs were cleaned with 20% povidone iodine and carefully dipped dry with paper towel. Eggs were then candled in a dark room to mark the air cell with a pencil. The eggs were then weighed, numbered, and assigned evenly into treatment groups (vehicle control, 0.05, 0.2, 0.5, 2, and 5 mg/kg) according to the egg weight. Three to five alive embryonic day four (ED4) embryos per group were collected. Two batches of hatchling chickens were used, with a total of 8, 4, 8, 6, 8, and 4 hatchlings included for control, 0.05, 0.2, 0.5, 2, and 5 mg/kg, respectively (one more animal from 0.05 mg/kg died during anesthesia thus was only counted in hatchability). Three to five viable hatchling chickens were included for the hatchling cardiac morphological/functional assessments and immunohistochemistry. Three independent samples per group (excluding 0.05 or 5 mg/kg groups) were included for western blotting.
Materials
PM2.5 from the 2016–2017 winter air of Beijing was acquired from a previous study (Chen S. et al., 2019). Briefly, the 24 h PM2.5 samples were collected with high-volume air samplers (Thermo, Waltham, MA, US) at a flow rate of 1.05 m3/min. Primary antibody against NF-kb p65 was purchased from Abcam (ab16502, Shanghai, China). Antibodies against iNOS and MMP9 were purchased from Bioss (bs-0162R for iNOS and bs-4593R for MMP9, Beijing, China). Antibody against GAPDH was purchased from ZSGB-BIO (TA-08, Beijing, China). Hematoxylin-eosin staining kit was purchased from Beyotime (C0105, Beijing, China). Immunohistochemistry kit was purchased from ZSGB-BIO (SP-9001, Beijing, China). Other general laboratory supplies are of the highest grade obtainable.
Air-Cell Injection
Air-cell injection was performed on fertile chicken eggs prior to development (embryonic day zero, ED0) as described in Jiang et al. (2012) with slight modifications. The original method was used for the injection of perfluorooctanoic acid, which is adopted for PM2.5 in the current study. Briefly, the PM2.5 particles were thoroughly mixed with sunflower oil by vortexing vigorously for 30 seconds. The concentrations for the dosing suspension were: 0.5, 2, 5, 20, and 50 mg/ml PM2.5 in sunflower oil for final doses of 0.05, 0.2, 0.5, 2, and 5 mg/egg kg PM2.5, respectively. Control animals received vehicle injection (sunflower oil). It had been proven that the sunflower oil had no remarkable effects regarding to the cardiovascular development in chicken embryos (Jiang et al., 2012).The doses of PM2.5 is comparable to previous similar studies performed in rodents (Wang Z. et al., 2019). However, to the best of our knowledge, no similar avian studies were reported previously. Yang et al. mentioned that the ambient levels of particulate matters in chicken houses located at Shandong, China were 114 to 230 μg/m3, from which the estimated real-life daily exposure to particulate matter would be approximately 0.1–0.2 mg/kg/day in chickens at Shandong, China (Yang W. et al., 2018). Such doses were covered in the current study. Regarding to the environmental levels, it had been reported that the particulate matter levels ranged from 80–90 ug/m3 in the 2017 winter at Shijiazhuang, China (Li D. et al., 2019), while a mean level of 60.64 ug/m3 was observed in China from 2005–2016 (He et al., 2017). Although it is difficult to accurately estimate the total exposure burden per body weight from just the ambient concentrations, the actual exposure levels were likely at the lower ends of the doses used in the current study. The air cell area was cleaned with 75% ethanol briefly, a hole with diameter of approximately 1 mm was drilled with a metal probe, the dosing suspension was then injected into the air cell area at 0.1 μl/g (egg weight). The dosing suspension was vortexed for at least ten seconds immediately prior to every injection. After the injection, the hole was sealed with melted paraffin.
Egg Incubation
Injected eggs were incubated in a Keyu incubator (Dezhou, China) as previously described (Lv et al., 2018). The incubation temperature and humidity were automatically controlled by the incubator. The incubation temperature was set at 37.9 degree Celsius at the beginning of incubation and gradually decreased to 37.1 degree Celsius as incubation continued, while humidity increased from 50 to 70%. Eggs were turned every three hours until ED19. Prior to hatch, externally pipped eggs were moved to individual hatch boxes, and hatched chickens were kept in a warm box with water supplied until the experiments were performed. This work has received approval for research ethics from the Institutional Animal Care and Use Committee (IACUC) of Qingdao University.
Electrocardiography
Within 24 h post hatch, hatchling chickens were anesthetized with 33 mg/kg pentobarbital via intraperitoneal injection and then subjected to electrocardiography as previously described (Lv et al., 2018). Briefly, two electrodes were embedded on both sides of the chest subcutaneously, and then connected to a BL-420E+ instrument (Taimeng, Sichuan, China). Electrocardiography were recorded and the heart rates were calculated with R-R intervals with the following equation: Heart rate (beats per minute) = 60/R-R interval (second).
Histological Assessments
For the ED4 chicken embryo, the whole embryos were removed from the eggs and directly visualized under a dissection microscope (Olympus SXZ9, Tokyo, Japan). The areas of heart of the embryos was quantified with ImageJ (NIH, US). Please refer to Supplementary Figure 1 for a demonstration of the measurements.
For the hatchling chickens, animals were anesthetized and quickly decapitated. Heart tissues were carefully dissected out, rinsed in cold phosphate buffered saline (PBS), fixed in PBS-buffered 4% formaldehyde for 24 h, and then embedded with paraffin. Embedded tissues were then sectioned with a Leica RM2016 microtome at six μm thickness. Sections were then subjected to hematoxylin and eosin (HE) staining following instructions from manufacturer (Beyotime, Beijing, China), or immunohistochemistry (ZSGB-Bio SP-9001, Beijing, China) as previously described (Lv et al., 2018). Briefly, the sections were firstly deparaffinized with xylene, antigen-retrieved with citrate buffer, quenched with hydrogen peroxide, blocked with goat serum, probed with primary antibodies (1:1,000) or PBS as negative control, and then visualized with HRP-conjugated secondary antibody (Goat anti rabbit), streptavidin working solution and DAB working solution (Solarbio, Beijing, China). Resulting sections were briefly counterstained with hematoxylin and visualized with a dissection microscope (Phoenix SMZ180, Jiangxi, China). HE stained heart pictures were quantified as described in Jiang et al. for the right ventricular wall thickness. Immunohistochemistry pictures were quantified with ImageJ for the positive staining area ratio.
Western Blotting
Hatchling chickens were anesthetized and quickly decapitated. Heart tissues were carefully dissected out and then homogenized in radio immunoprecipitation assay (RIPA) buffer (Beyotime, Beijing, China) with 1 mM PMSF (Solarbio, Beijing, China) and 2 μg/ml aproteinin (Solarbio, Beijing China) added. Western blotting was performed as described previously (Jiang et al., 2020). The protein concentrations of resulting samples were determined with BCA assays (Beyotime, Beijing, China) and adjusted with PBS. The samples were then subjected to electrophoresis, transferred to polyvinyldene fluoride (PVDF) membranes, blocked with non-fat milk, probed with iNOS, MMP9 or GAPDH primary antibodies (1:1,000 for iNOS/MMP9 and 1:5,000 for GAPDH) and corresponding secondary antibodies (1:5,000 HRP conjugated Anti-rabbit or mouse antibodies), visualized with a Fusion Solo S (Vilber Lourmat, Collégien, France) and quantified with ImageJ (NIH, US) software. Three independent samples were assessed per group.
Lactate Dehydrogenase Activities Assay
The lactate dehydrogenase (LDH) activities in hatchling chicken serum were assessed with a commercially available kit (A020-2-2, Jiancheng, Nanjing, China) following manufacturer’s instructions.
Superoxide Dismutase Activities Assay
The superoxide dismutase (SOD) activities in hatchling chicken serum were assessed with a commercially available kit (A001-3-2, Jiancheng, Nanjing, China) following manufactuer’s instructions.
Statistical Analysis
Data were represented as mean ± standard derivation. Statistical analysis was performed with SPSS 17.0. One-way analysis of variance (ANOVA) was used to assess differences among groups. When ANOVA detected significant results, post-hoc tests (least significant difference test) were used to compare differences between groups. Results were considered statistically significant when P < 0.05.
Results
Particulate Matter 2.5 Component Analysis
To confirm the component of the PM2.5 used in the current study, component analysis was performed. The collection and identification method of the PM2.5 was as described in Chen S. et al. (2019). The polycyclic aromatic hydrocarbons (PAHs) was estimated at 67.273 ng/m3, and the alkyl PAHs was estimated at 16.406 ng/m3 (Chen S. et al., 2019). Additionally, the water extraction of the PM2.5 was analyzed with inductively coupled plasma mass spectrometry (ICP-MS; ELEMENT2;221 Thermo Finnigan, San Jose, CA, USA), and the results was reported in Table 1.
Areas of ED4 Chicken Embryo Heart
To investigate whether developmental exposure to PM2.5 may affect early heart development in chicken embryos, the areas of heart in ED4 chicken embryo were measured and analyzed. Significantly increased areas of heart were observed in 0.2, 2, and 5 mg/kg PM2.5-exposed ED4 chicken embryos, resulting in 39.9, 82.5, and 70.2% increase relative to control embryo hearts, respectively (Figure 1).
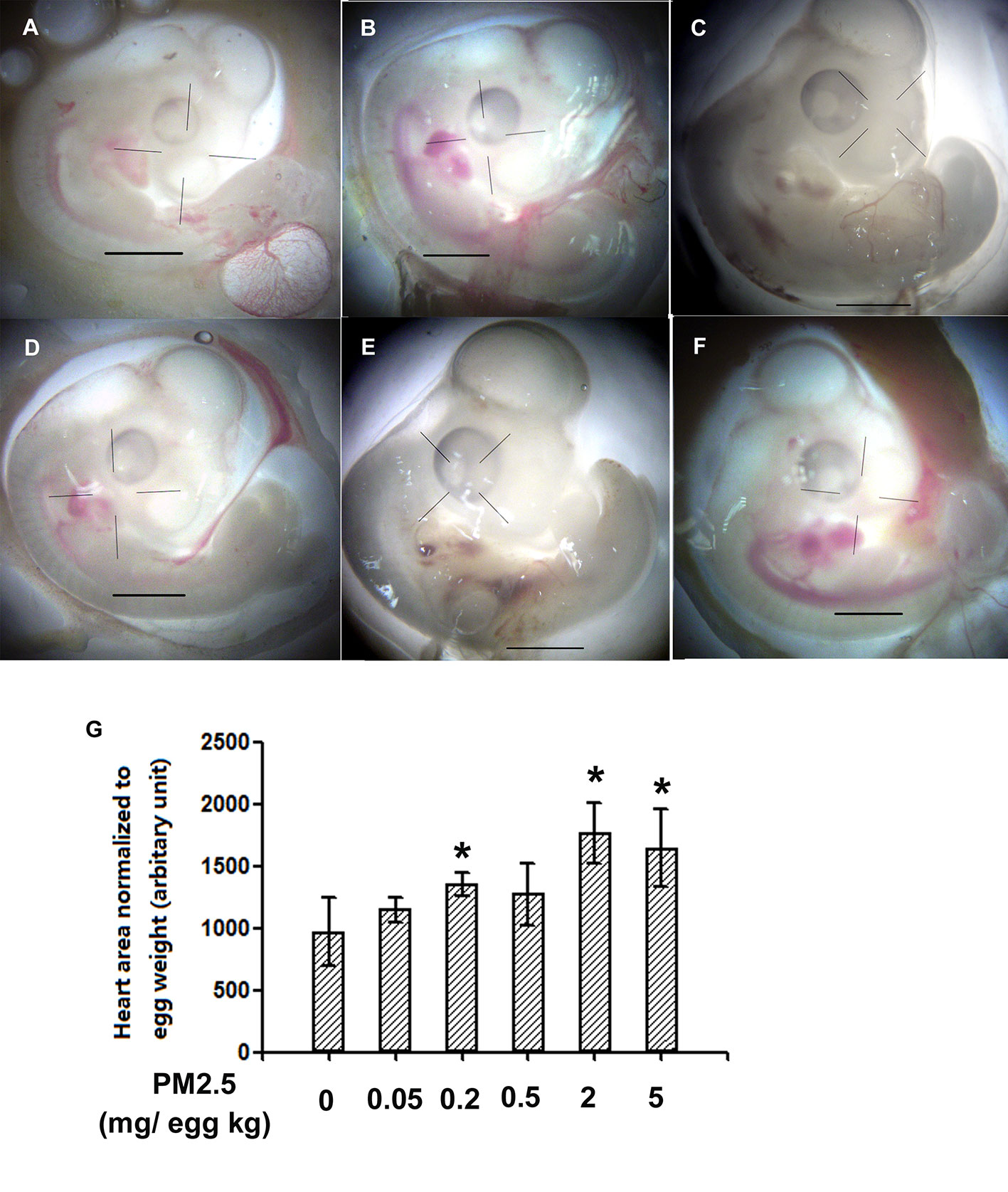
Figure 1 Morphological assessment of areas of embryonic day 4 chicken embryo heart. Fertile chicken eggs were air-cell injected with 0, 0.05, 0.2, 0.5, 2, and 5 mg/kg PM2.5 in sunflower oil, and incubated until embryonic day 4. Embryos were then taken out of the shell and directly visualized with a microscope (Olympus SXZ9, Tokyo, Japan). ImageJ was used to quantify the area of the hearts. N=3–5 per group. *: statistically different from control (P < 0.05). Scale bars represent 1,000 μm. (A) Representative photo of control ED4 embryo. (B) Representative photo of ED4 embryos exposed to 0.05 mg/kg PM2.5. (C) Representative photo of ED4 embryos exposed to 0.2 mg/kg PM2.5. (D) Representative photo of ED4 embryos exposed to 0. 5 mg/kg PM2.5. (E) Representative photo of ED4 embryos exposed to 2 mg/kg PM2.5. (F) Representative photo of ED4 embryos exposed to 5 mg/kg PM2.5. (G) Quantification of the areas of heart.
General Parameters of Hatchling Chickens
To confirm whether overt toxicities were present following developmental exposure to PM2.5, general parameters were analyzed and reported in Figure 2. Decreased slim body weight (body weight minus yolk weight) was observed in the hatchling chickens developmentally exposed to 0.05 and 0.5 mg/kg PM2.5 (18.1 and 16.0%, respectively). No statistically changes were observed in other endpoints, including the heart index (heart weight normalized to slim body weight for the specific cardiac effects), liver index (liver weight normalized to slim body weight), and hatchability (number of successful hatches divided by total number of embryos reached hatchling stage) (Figure 2).
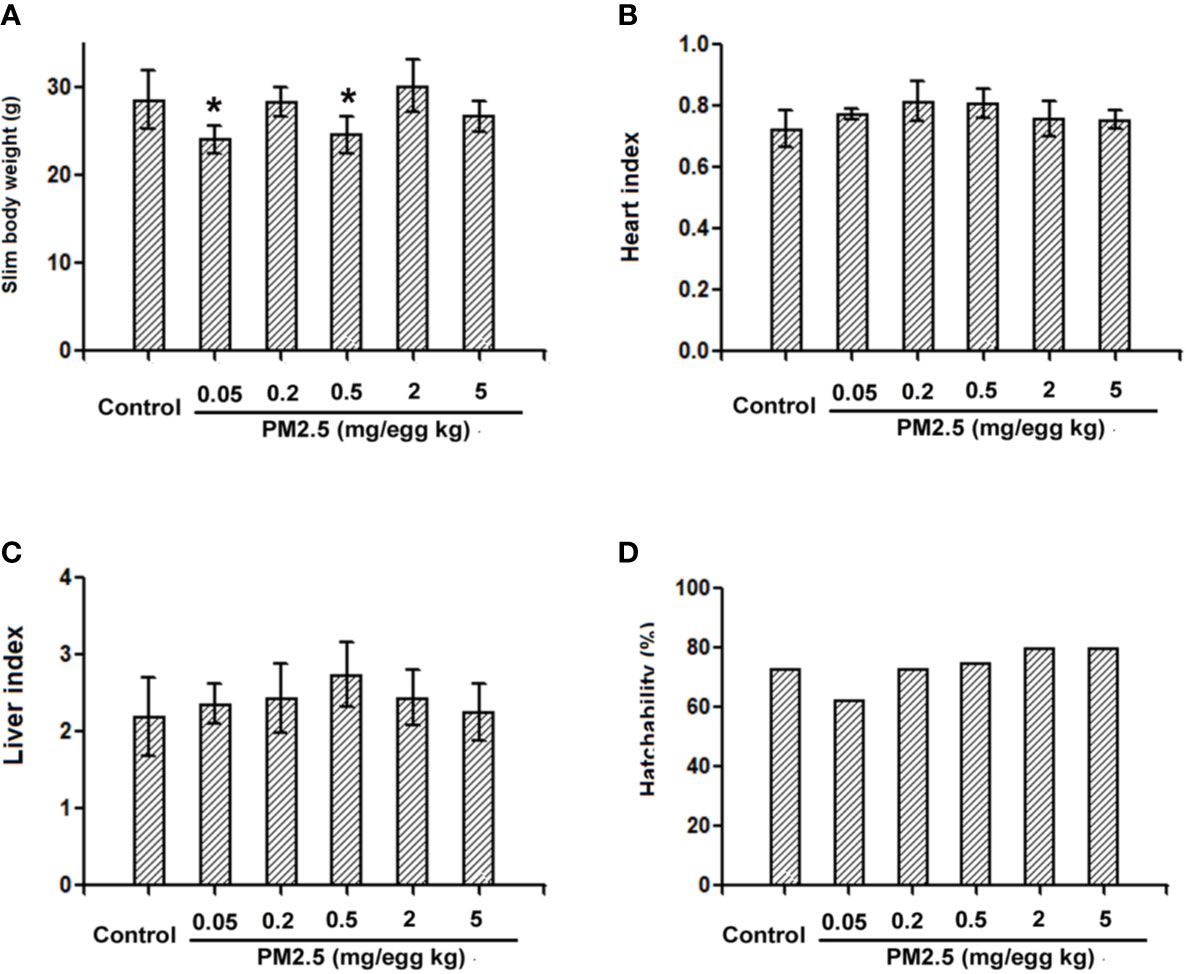
Figure 2 General parameters of hatchling chickens. Fertile chicken eggs were air-cell injected with 0, 0.05, 0.2, 0.5, 2, and 5 mg/kg PM2.5 in sunflower oil, and incubated until hatch. Within 24 h post hatch, hatchling chickens were anaesthetized with 33 mg/kg pentobarbital via intraperitoneal injection, weighed and sacrificed, yolks, hearts and livers were collected and weighed, slim body weight, heart index, liver index and hatchability were calculated. N=4–8 per group. *: statistically different from control group (P < 0.05). (A) Slim body weight of the hatchling chickens [(Body weight – yolk weight)*100%]. (B) Heart index of the hatchling chickens [(heart weight/slim body weight)*100%]. (C) Liver index of the hatchling chickens [(liver weight/slim body weight)*100%]. (D) Hatchability of the hatchling chickens (number of successful hatchlings/total number of embryos reached hatching stage). Note: one animal from 0.05 mg/kg group died during anesthesia thus was not counted in slim body weight, heart index or liver index.
Hatchling Chicken Heart Rates
To assess the potential cardiac functional changes following developmental exposure to PM2.5, electrocardiography was used to assess the heart rates of hatchling chickens. At the higher doses tested (0.2, 2, and 5 mg/kg), PM2.5 developmental exposures led to significantly elevated heart rates relative to control, resulting in 11.1, 17.4, and 16.6% increase, respectively (Figure 3).
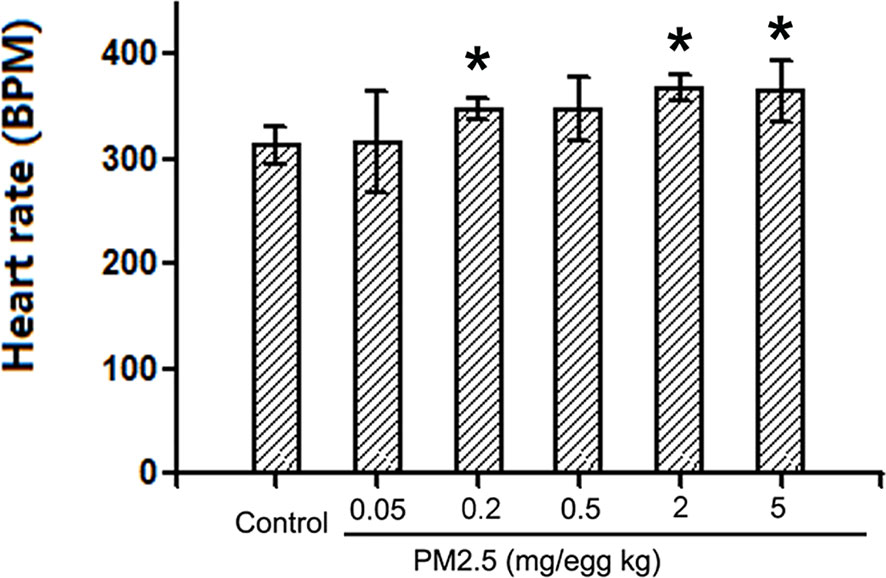
Figure 3 Heart rates of hatchling chickens. Fertile chicken eggs were air-cell injected with 0, 0.05, 0.2, 0.5, 2, and 5 mg/kg PM2.5 in sunflower oil, and incubated until hatch. Hatchling chickens were anaesthetized with 33 mg/kg pentobarbital via intraperitoneal injection, and subjected to electrocardiography with the BL-420E+ (Taimeng, Chengdu, China). Heart rates were calculated with the equation: 60/R-R interval. N=3–5 per group. *: statistically different from control (P < 0.05).
Histological Assessment of Hatchling Chicken Heart Right Ventricular Wall Thickness
To assess the potential cardiac morphological changes following developmental exposure to PM2.5, the right ventricular wall thickness was assessed with histological methods. While no significant changes were observed following 0.05 or 0.2 mg/kg PM2.5 developmental exposure, 0.5 and 2 mg/kg PM2.5 exposure led to significantly elevated right ventricular wall thickness (46.2 and 38.6% comparing to control, respectively). Interestingly, the highest dose tested (5 mg/kg) resulted in remarkably decreased right ventricular wall thickness (26.2% relative to control) (Figure 4).
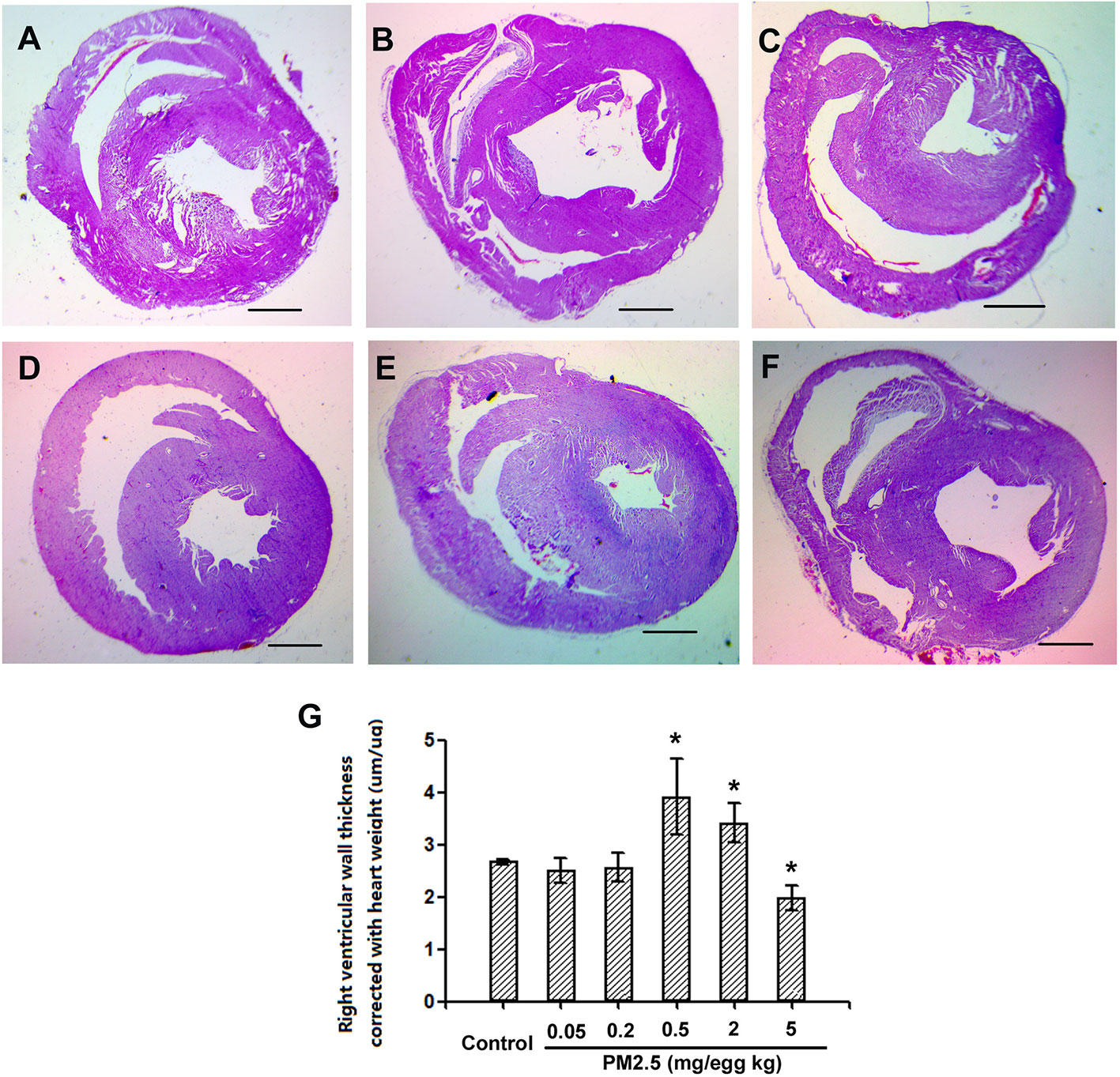
Figure 4 Histological assessment of hatchling chicken hearts. Fertile chicken eggs were air-cell injected with 0, 0.05, 0.2, 0.5, 2, and 5 mg/kg PM2.5 in sunflower oil, and incubated until hatch. Animals were sacrificed and hearts were collected, fixed in 4% formaldehyde for 24 h, and embedded in paraffin. Six μm sections were made and stained with hematoxylin and eosin, resulting slides were visualized with a microscope (Olympus SXZ9, Tokyo, Japan), and measured for the right ventricular wall thickness. N=3 per group. *: statistically different from control (P < 0.05). Scale bars represent 1,000 μm. (A) Representative picture of hearts from control animals. (B) Representative picture of hearts from animals exposed to 0.05 mg/kg PM2.5. (C) Representative picture of hearts from animals exposed to 0.2 mg/kg PM2.5. (D) Representative picture of hearts from animals exposed to 0.5 mg/kg PM2.5. (E) Representative picture of hearts from animals exposed to 2 mg/kg PM2.5. (F) Representative picture of hearts from animals exposed to 5 mg/kg PM2.5. (G) Quantification of right ventricular wall thickness.
Immunohistochemistry for NF-kb p65 in Hatchling Chicken Hearts
To confirm whether NF-kb p65 is involved in PM2.5-exposure induced developmental cardiotoxicity in chicken embryo, immunohistochemistry for NF-kb p65 was performed on the hatchling heart sections. While no significant changes were observed in 0.2 mg/kg, significantly higher levels of positively stained cells were present in tissues from 0.5 and 2 mg/kg PM2.5-exposed animals. Quantification revealed a relative change of 278.8 and 375.9%, respectively (Figure 5).
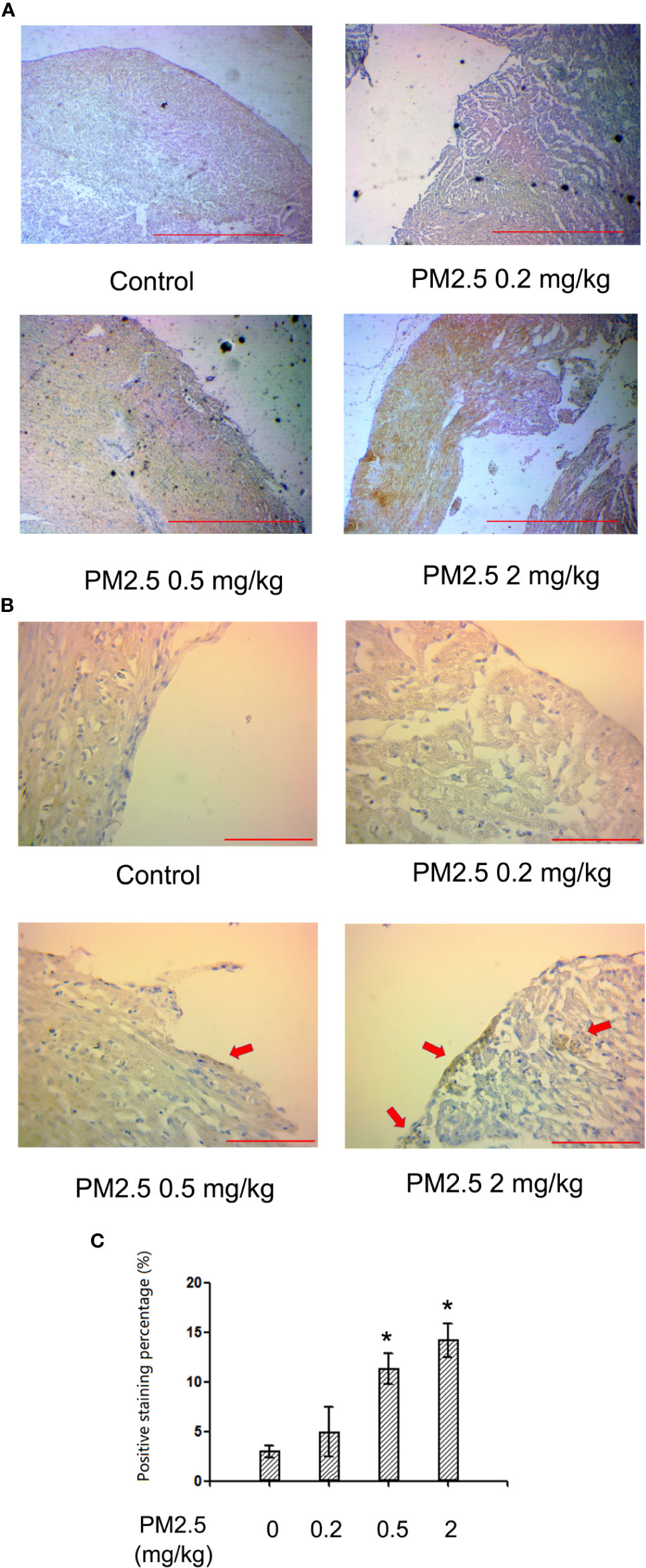
Figure 5 Immunohistochemistry for NF-kb p65 in hatchling chicken hearts. Fertile chicken eggs were air-cell injected with 0, 0.2, 0.5, and 2 mg/kg PM2.5 in sunflower oil, and incubated until hatch. Animals were sacrificed and hearts were collected, fixed in 4% formaldehyde for 24 h, and embedded in paraffin. Six μm sections were made and subjected to immunohistochemistry for NF-kb p65. (A) Representative low magnitude pictures. Scale bars represent 600 μm. (B) Representative high magnitude pictures. Arrows indicate the positive staining areas. Scale bars represent 70 μm. (C) Quantification results from the high magnitude pictures. N=3 per group. *: statistically different from control (P < 0.05).
Western Blotting for iNOS and MMP9 Expression Levels in Hatchling Chicken Hearts
To assess the potential changes of major inflammation/cardiac remodeling mediators, iNOS and MMP9, western blotting was performed with heart protein extracts. The results indicated similar results of iNOS and MMP9 expression levels in hatchling chicken heart: significantly elevated expression levels in 0.2 and 0.5 mg/kg groups, but no remarkable changes in 2 mg/kg groups. For iNOS, the percentage changes in 0.2 and 0.5 mg/kg groups were 13.2 and 45.5%, respectively. For MMP9, the percentage changes in 0.2 and 0.5 mg/kg groups were 36.2 and 67.5%, respectively (Figure 6).
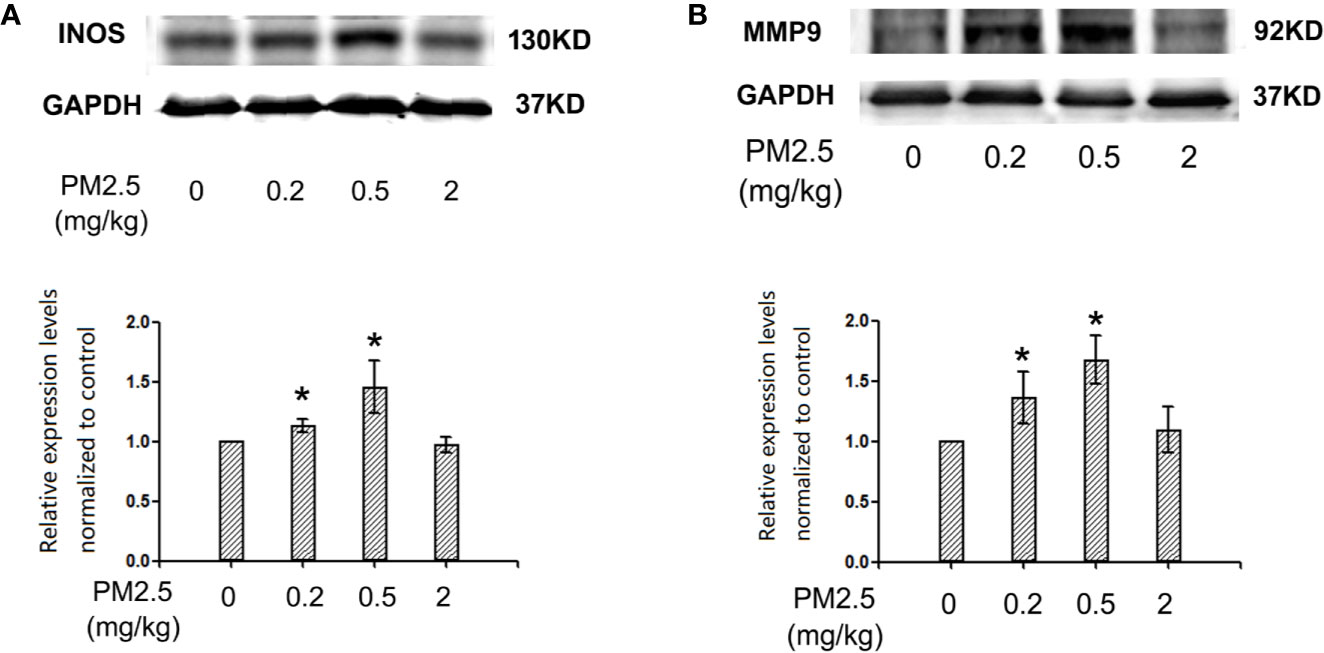
Figure 6 Western blotting for iNOS and MMP9 in hatchling chicken hearts. Fertile chicken eggs were air-cell injected with 0, 0.2, 0.5, and 2 mg/kg PM2.5 in sunflower oil, and incubated until hatch. Animals were sacrificed and hearts were collected, protein samples were extracted and subjected to western blotting. Results were semi-quantified with ImageJ by firstly normalizing to the internal controls (GAPDH), then normalizing to the control sample on the same gel. N=3 per group. *: statistically different from control (P < 0.05). (A) Representative images and semi-quantifications for iNOS. (B) Representative images and semi-quantifications for MMP9.
Lactate Dehydrogenase and Superoxidase Dismutase Activities in Hatchling Chicken Serum
To assess the potential direct myocardium damage and oxidative stress, LDH activities and SOD activities were assessed in hatchling chicken serum, respectively. The results indicated that LDH activities were not affected in hatchling chicken serum following 0.05, 0.2, or 2 mg/kg PM 2.5 exposure, but significantly increased following 5 mg/kg PM2.5 exposure (30.4% relative to control, Figure 7A). On the other hand, no remarkable changes were observed in SOD activities following 0.05, 0.2, or 2 mg/kg PM2.5 exposure, but significantly increased SOD activities were observed in serums of hatchling chickens exposed to 0.5 mg/kg PM2.5 (41.5% relative to control), and remarkably decreased SOD activities were observed in those exposed to 5 mg/kg PM2.5 (24.1% relative to control, Figure 7B).
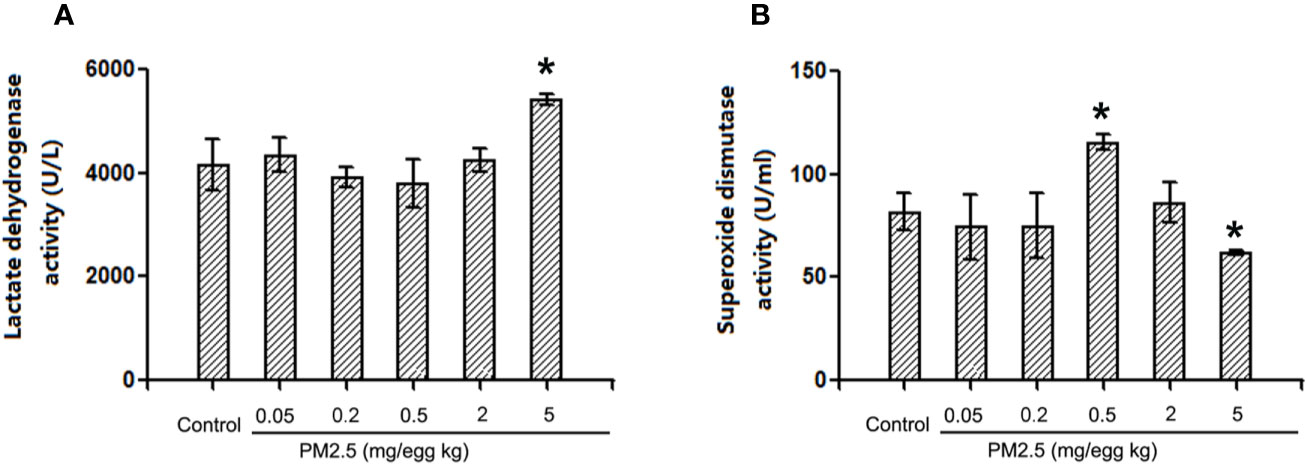
Figure 7 Lactate dehydrogenase and superoxide dismutase activities in hatchling chicken serum. Fertile chicken eggs were air-cell injected with 0, 0.2, 0.5, and 2 mg/kg PM2.5 in sunflower oil, and incubated until hatch. Animals were sacrificed, serum were collected and subjected to LDH and SOD activities assays. N=3 per group. *: statistically different from control (P < 0.05). (A) Quantification of lactate dehydrogenase activities. (B) Quantification of superoxide dismutase activities.
Discussion
PM2.5 exposure affects a large population among the world. While its negative health effects are currently being extensively investigated, the developmental toxicities received relatively less attention. Moreover, the developmental cardiotoxicity was even less investigated, while heart development is one of the most important early events in embryo development (Astrof et al., 2007) and potentially affects cardiovascular health after birth and even in adulthood (Klattenhoff et al., 2013). The current study utilized the classical chicken embryo model to investigate the potential developmental cardiotoxicity induced by PM2.5 exposure, revealing potential effects exerted by developmental exposure to PM2.5 and the underlying molecular mechanism. While many still remain to be elucidated, our data suggests that developmental exposure to PM2.5 has the potential to interfere with heart development, and is associated with inflammatory responses.
The real-world PM2.5 exposure occurs by inhalation, while in utero exposure is possible since PMs may pass the placenta barrier (Teng et al., 2016). In laboratory, it is generally difficult to expose animals with accurate doses according to the ambient concentrations, and alternative exposure methods, such as intratracheal instillation or direct exposure are commonly used. One study performed in rats used 9 and 24 mg/kg PM exposure via intratracheal instillation for seven weeks (Wei et al., 2018). Another study exposed zebrafish embryos with up to 900 μg/ml PM2.5 in water for 120 h (Zhang Y. et al., 2018). In the current study, 0, 0.05, 0.2, 0.5, 2, and 5 mg/kg PM2.5 were exposed to fertile chicken eggs as a one-time air cell injection, which is comparable to previous studies. From He et al. (2017), the average particulate matter level in China from 2005–2016 was 60.64 ug/m3. The lowest dose (0.05 mg/kg) used in the study may be roughly translated to approximately 175 hours’ exposure at that level, while the highest dose used (5 mg/kg) roughly equals 2 years’ exposure. Since chicken eggs are closed systems, the injected PM2.5 stays in the shell for the whole embryonic development period (21 days). The lowest and highest doses (0.05 and 5 mg/kg) were not included in the immunohistochemistry and western blotting experiments, since 0.05 mg/kg induced little effects, while 5 mg/kg seemed to start to induce overt toxicity from the histological data.
PM2.5 exposure occurs almost ubiquitously in areas with air pollution, resulting in definite exposure to developing embryo/fetus in these areas, thus the potential developmental toxicity is a concern. Many toxic components, such as the heavy metals and PAHs are present in PM, which are capable of exerting developmental toxicities (Shankar et al., 2019; Zhao et al., 2020). Epidemiological studies revealed that developmental exposure to PM resulted in increased risk of asthma (Kravitz-Wirtz et al., 2018), decreased airway antimicrobial activity (Zhang et al., 2019), low birth weight (Wang et al., 2019b), and pregnancy loss (Xue et al., 2019).
In the current study, lower body weight was observed in hatchling chickens, which is consistent with the epidemiological results. Regarding to the heart, although many studies focused on the cardiovascular effects following PM exposure in general population (Hamanaka and Mutlu, 2018), no epidemiological reports have associated cardiovascular effects with developmental exposure to PM to the best of our knowledge. Nevertheless, several laboratory studies reported developmental cardiotoxicity in zebra fish model, and associated the mechanism with AhR signaling (Yue et al., 2017; Chen T. et al., 2019). In these studies, abnormal heart structure was observed following PM exposure, suggesting that PM2.5 has the potential to interference with cardiac morphology, which may be explained by the fact that both metals and PAHs in PM are capable of inducing developmental cardiotoxicity (Jayasundara et al., 2015; Liu et al., 2018). In the current study, morphological changes in chicken embryo/hatchling chicken hearts were observed following PM2.5 developmental exposure, confirming the previous studies performed with zebra fish embryos. While the heart index did not change in hatchling chickens, suggesting that exposure to PM2.5 did not directly retard heart development, thickened right ventricular wall thickness was observed, which was a typical change following pulmonary damage (Vonk Noordegraaf et al., 2017). Since right ventricle was typically associated with cardiopulmonary effects, observed changes suggested that PM exposure may target pulmonary system and right ventricle. Notably, different doses of PM exposure resulted in different responses. While doses equal or lower than 0.2 mg/kg did not induce significant changes, 0.5 and 2 mg/kg exposure to PM resulted in remarkably thicker right ventricular walls. Interestingly, 5 mg/kg exposure did not further increase the right ventricular wall thickness, instead, the thickness significantly decreased, even thinner than those of the control animals. Such effects may be the results of overt toxicity at higher doses, as demonstrated by histological results. Additionally, elevated heart rate was observed with electrocardiography, which had been associated with myocardial injury and cardiac dysfunction (Abbott et al., 2018; Grande et al., 2018). It was also worth noting that the altered heart morphology and function are consistent with the teratogenic effects reported by Ejaz, S. and Massarsky, A., suggesting that the particulate matter used in the current study has teratogenic potential at the doses tested (Ejaz et al., 2009; Massarsky et al., 2015).
NF-kb signaling pathway is involved in tissue damage, especially inflammatory responses (Zhang et al., 2017; Song et al., 2018). It had been reported that NF-kb participates in PM2.5-induced toxicities, including atherosclerosis (Geng et al., 2019), pulmonary toxicity (Shi et al., 2019), and general inflammation (Fukatsu et al., 2018; Ryu et al., 2019). Inflammation plays important role in general cardiac hypertrophy (Li H. et al., 2019b; Li Q. et al., 2019) as well as particulate matter-induced cardiotoxicity (Luo et al., 2019). In the current study, elevated NF-kb expression levels were observed in exposed animal hearts, indicating the involvement of NF-kb and potentially inflammation in PM2.5-induced developmental cardiotoxicity. Interestingly, cumulated positive stains in clusters were observed in immunohistochemistry, suggesting that the inflammation tend to occur in relatively localized locations rather than diffused throughout the whole organ. This phenomenon will be further investigated.
Oxidative stress plays important roles in particulate matter-induced cardiotoxicities (Rao et al., 2018). Several studies associated oxidative stress with cardiac dysfunction (Qin et al., 2018), cardiac hypertrophy (Yue et al., 2019), and cardiac fibrosis (Gao et al., 2020). One important marker and potentially contributor of oxidative stress is iNOS, which is an inducible enzyme, producing excessive amounts of NO in case of inflammation or oxidative stress elevation. On the other hand, MMP9 participates in the degradation of the extracellular matrix during tissue remodeling (Lee et al., 2012; Wilmes et al., 2020). Both iNOS and MMP9 had been associated with PM2.5-induced toxicities frequently (Li et al., 2015; Wang et al., 2016; Yang B. et al., 2018; Zhang H. et al., 2018). iNOS is typically induced in response to inflammation, thus might participate in the developmental cardiotoxicity observed in the current study. MMP9 is an important factor in cardiac remodeling (Jenke et al., 2017), and might partially explain the morphological changes in the hearts following PM2.5 exposure. Moreover, iNOS and MMP9 are closely related to each other, often involved in toxicities together and had been both reported to be regulated by NF-kb (Yu J. et al., 2019). In the current study, enhanced expression of both iNOS and MMP9 were observed following PM exposure in hatchling chicken hearts, suggesting involvement of iNOS and MMP9 in the observed developmental cardiotoxicity, and further confirms the involvement of inflammation/oxidative stress. Direct measurement of LDH and SOD activities in serum also provided evidence that higher doses of PM exposure induced myocardium damage and oxidative stress. Similar expression patterns indicated that these two are likely to be regulated together. Interestingly, the highest dose tested for iNOS and MMP9 (2 mg/kg) did not have significant impact, while the two lower doses did (0.2 and 0.5 mg/kg). Since similar pattern (higher doses generate different responses from lower ones) were also observed in slim body weight and histopathology data, the effect is unlikely caused by chance. Actually, many toxicants featured such non-linear dose-response curves, sometimes are known as hormesis (Teeguarden et al., 2000), usually resulting from low-dose exposure-induced defense/compensation. It is also worth noting that the above-mentioned immunohistochemistry results indicated no such trends for NF-kb expression in heart tissues. A possible explanation is that some other unknown regulatory/defensive factors started to take effect at higher doses, suppressing the expression of iNOS and MMP9, while the relatively up-stream NF-kb was not covered by those unknown factors. Whether the lower doses of effect can be interpreted as protective/hormesis remain to be elucidated. In summary, the NF-kb-iNOS/MMP9 pathway is associated with PM2.5-induced developmental cardiotoxicity in chicken embryo. To further elucidate the mechanisms, further investigations on this specific pathway is guaranteed.
Conclusions
In the current study, developmental exposure to PM2.5 particles induced morphological and functional changes in the hearts of developing chicken embryo and hatchling chickens, suggesting developmental cardiotoxicity. Mechanistic investigations associated NF-kb p65, iNOS, and MMP9 with the observed endpoints. Further investigations regarding to such effects is necessary.
Data Availability Statement
The materials described in the article, including all relevant raw data, will be freely available to any scientist wishing to use them for non-commercial purposes, and the data used in the current study can be obtained from the corresponding author on a reasonable request.
Ethics Statement
The animal study was reviewed and approved by Institutional Animal Care and Use Committee (IACUC) of Qingdao University.
Author Contributions
YZ and QJ designed the study. QJ and CZ performed the data analysis and drafted the manuscript. SC and DL assisted the manuscript writing. QJ, SC, and LC performed the statistical analysis. SC and DL performed the particulate matter 2.5 content analysis. QJ, CZ, LS, and NL performed animals’ examinations and collected data. QJ and YC performed the histopathological assessments. LS and NL performed western blotting experiments. YZ reviewed and edited the manuscript. All authors read and approved the final manuscript.
Funding
This work was supported by National Natural Science Foundation of China (Grant No. 91643203, 91543208, 81872591, 81502835).
Conflict of Interest
The authors declare that the research was conducted in the absence of any commercial or financial relationships that could be construed as a potential conflict of interest.
Supplementary Material
The Supplementary Material for this article can be found online at: https://www.frontiersin.org/articles/10.3389/fphar.2020.00841/full#supplementary-material
Supplementary Figure 1 | Measurement method for the areas of heart in embryonic day four (ED4) chicken embryos. The blue circle represents the area of heart, whose area was then measured with ImageJ (NIH, US).
References
Abbott, T. E. F., Pearse, R. M., Archbold, R. A., Ahmad, T., Niebrzegowska, E., Wragg, A., et al. (2018). A Prospective International Multicentre Cohort Study of Intraoperative Heart Rate and Systolic Blood Pressure and Myocardial Injury After Noncardiac Surgery: Results of the VISION Study. Anesth Analg 126, 1936–1945. doi: 10.1213/ANE.0000000000002560
Astrof, S., Kirby, A., Lindblad-Toh, K., Daly, M., Hynes, R. O. (2007). Heart development in fibronectin-null mice is governed by a genetic modifier on chromosome four. Mech. Dev. 124, 551–558. doi: 10.1016/j.mod.2007.05.004
Chen, H., Chen, X., Hong, X., Liu, C., Huang, H., Wang, Q., et al. (2017). Maternal exposure to ambient PM2.5 exaggerates fetal cardiovascular maldevelopment induced by homocysteine in rats. Environ. Toxicol. 32, 877–889. doi: 10.1002/tox.22287
Chen, S., Li, D., Zhang, H., Yu, D., Chen, R., Zhang, B., et al. (2019). The development of a cell-based model for the assessment of carcinogenic potential upon long-term PM2.5 exposure. Environ. Int. 131, 104943. doi: 10.1016/j.envint.2019.104943
Chen, T., Jin, H., Wang, H., Yao, Y., Aniagu, S., Tong, J., et al. (2019). Aryl hydrocarbon receptor mediates the cardiac developmental toxicity of EOM from PM2.5 in P19 embryonic carcinoma cells. Chemosphere 216, 372–378. doi: 10.1016/j.chemosphere.2018.10.160
Cho, C. C., Hsieh, W. Y., Tsai, C. H., Chen, C. Y., Chang, H. F., Lin, C. S. (2018). In Vitro and In Vivo Experimental Studies of PM2.5 on Disease Progression. Int. J. Environ. Res. Public Health 15, 1380. doi: 10.3390/ijerph15071380
Ejaz, S., Ashraf, M., Nawaz, M., Lim, C. W., Kim, B. (2009). Anti-angiogenic and teratological activities associated with exposure to total particulate matter from commercial cigarettes. Food Chem. Toxicol. 47, 368–376. doi: 10.1016/j.fct.2008.11.029
Frode-Saleh, T. S., Calixto, J. B. (2000). Synergistic antiinflammatory effect of NF-kappaB inhibitors and steroidal or non steroidal antiinflammatory drugs in the pleural inflammation induced by carrageenan in mice. Inflammation Res. 49, 330–337. doi: 10.1007/PL00000213
Fukatsu, H., Koide, N., Tada-Oikawa, S., Izuoka, K., Ikegami, A., Ichihara, S., et al. (2018). NFkappaB inhibitor DHMEQ inhibits titanium dioxide nanoparticleinduced interleukin1beta production: Inhibition of the PM2.5induced inflammation model. Mol. Med. Rep. 18, 5279–5285. doi: 10.1016/j.redox.2019.101345
Gao, J., Yuan, J., Wang, Q., Lei, T., Shen, X., Cui, B., et al. (2020). Metformin protects against PM2.5-induced lung injury and cardiac dysfunction independent of AMP-activated protein kinase alpha2. Redox Biol. 28, 101345.
Geng, J., Liu, H., Ge, P., Hu, T., Zhang, Y., Zhang, X., et al. (2019). PM2.5 promotes plaque vulnerability at different stages of atherosclerosis and the formation of foam cells via TLR4/MyD88/NFkappaB pathway. Ecotoxicol Environ. Saf. 176, 76–84. doi: 10.1016/j.ecoenv.2019.03.068
Giannadaki, D., Lelieveld, J., Pozzer, A. (2016). Implementing the US air quality standard for PM2.5 worldwide can prevent millions of premature deaths per year. Environ. Health 15, 88. doi: 10.1186/s12940-016-0170-8
Grande, D., Iacoviello, M., Aspromonte, N. (2018). The effects of heart rate control in chronic heart failure with reduced ejection fraction. Heart Fail Rev. 23, 527–535. doi: 10.1007/s10741-018-9704-1
Hamanaka, R. B., Mutlu, G. M. (2018). Particulate Matter Air Pollution: Effects on the Cardiovascular System. Front. Endocrinol. (Lausanne) 9, 680. doi: 10.3389/fendo.2018.00680
He, M. Z., Zeng, X., Zhang, K., Kinney, P. L. (2017). Fine Particulate Matter Concentrations in Urban Chinese Cities 2005-2016: A Systematic Review. Int. J. Environ. Res. Public Health 14, 191. doi: 10.3390/ijerph14020191
Hoffman, D. J., Rice, C. P., Kubiak, T. J. (1996). "PCBs and dioxins in birds," in Environmental Contaminants in Wildlife: Interpreting Tissue Concentrations. Eds. Beyer, W. N., Heinz, G. H., Redmon-Norwood, A. W. (Boca Raton, Florida, USA: CRC Press), 165–208.
Jayasundara, N., Van Tiem Garner, L., Meyer, J. N., Erwin, K. N., Di Giulio, R. T. (2015). AHR2-Mediated transcriptomic responses underlying the synergistic cardiac developmental toxicity of PAHs. Toxicol. Sci. 143, 469–481. doi: 10.1093/toxsci/kfu245
Jenke, A., Schur, R., Roger, C., Karadeniz, Z., Gruger, M., Holzhauser, L., et al. (2017). Adiponectin attenuates profibrotic extracellular matrix remodeling following cardiac injury by up-regulating matrix metalloproteinase 9 expression in mice. Physiol. Rep. 5, e13523. doi: 10.14814/phy2.13523
Jiang, Q., Lust, R. M., Strynar, M. J., Dagnino, S., DeWitt, J. C. (2012). Perflurooctanoic acid induces developmental cardiotoxicity in chicken embryos and hatchlings. Toxicology 293, 97–106. doi: 10.1016/j.tox.2012.01.005
Jiang, Q., Xu, X., Zhang, C., Luo, J., Lv, N., Shi, L., et al. (2020). In ovo very early-in-life exposure to diesel exhaust induced cardiopulmonary toxicity in a hatchling chick model. Environ. Pollut. 264, 114718. doi: 10.1016/j.envpol.2020.114718
Klattenhoff, C. A., Scheuermann, J. C., Surface, L. E., Bradley, R. K., Fields, P. A., Steinhauser, M. L., et al. (2013). Braveheart, a long noncoding RNA required for cardiovascular lineage commitment. Cell 152, 570–583. doi: 10.1016/j.cell.2013.01.003
Kravitz-Wirtz, N., Teixeira, S., Hajat, A., Woo, B., Crowder, K., Takeuchi, D. (2018). Early-Life Air Pollution Exposure, Neighborhood Poverty, and Childhood Asthma in the United States 1990(-)2014. Int. J. Environ. Res. Public Health 15, 1114. doi: 10.3390/ijerph15061114
Lee, M. Y., Shin, I. S., Lim, H. S., Shin, H. K. (2012). A water extract of Samchulkunbi-tang attenuates airway inflammation by inhibiting inos and MMP-9 activities in an ovalbumin-induced murine asthma model. BMC Complement Altern. Med. 12, 257. doi: 10.1186/1472-6882-12-257
Leepe, K. A., Li, M., Fang, X., Hiyoshi, A., Cao, Y. (2019). Acute effect of daily fine particulate matter pollution on cerebrovascular mortality in Shanghai, China: a population-based time series study. Environ. Sci. Pollut. Res. Int. 26, 25491–25499. doi: 10.1007/s11356-019-05689-8
Li, R., Kou, X., Geng, H., Xie, J., Tian, J., Cai, Z., et al. (2015). Mitochondrial damage: an important mechanism of ambient PM2.5 exposure-induced acute heart injury in rats. J. Hazard Mater 287, 392–401. doi: 10.1016/j.jhazmat.2015.02.006
Li, D., Zhang, R., Cui, L., Chu, C., Zhang, H., Sun, H., et al. (2019). Multiple organ injury in male C57BL/6J mice exposed to ambient particulate matter in a real-ambient PM exposure system in Shijiazhuang, China. Environ. Pollut. 248, 874–887. doi: 10.1016/j.envpol.2019.02.097
Li, H., Shi, Y., Wang, X., Li, P., Zhang, S., Wu, T., et al. (2019). Piceatannol alleviates inflammation and oxidative stress via modulation of the Nrf2/HO-1 and NF-kappaB pathways in diabetic cardiomyopathy. Chem. Biol. Interact. 310, 108754. doi: 10.1016/j.cbi.2019.108754
Li, Q., Wei, G., Tao, T. (2019). Leukocyte immunoglobulin-like receptor B4 (LILRB4) negatively mediates the pathological cardiac hypertrophy by suppressing fibrosis, inflammation and apoptosis via the activation of NF-kappaB signaling. Biochem. Biophys. Res. Commun. 509, 16–23. doi: 10.1016/j.bbrc.2018.11.137
Liu, Z., He, C., Chen, M., Yang, S., Li, J., Lin, Y., et al. (2018). The effects of lead and aluminum exposure on congenital heart disease and the mechanism of oxidative stress. Reprod. Toxicol. 81, 93–98. doi: 10.1016/j.reprotox.2018.07.081
Lubczynska, M. J., Sunyer, J., Tiemeier, H., Porta, D., Kasper-Sonnenberg, M., Jaddoe, V. W. V., et al. (2017). Exposure to elemental composition of outdoor PM2.5 at birth and cognitive and psychomotor function in childhood in four European birth cohorts. Environ. Int. 109, 170–180. doi: 10.1016/j.envint.2017.09.015
Luo, C. M., Feng, J., Zhang, J., Gao, C., Cao, J. Y., Zhou, G. L., et al. (2019). 1,25-Vitamin D3 protects against cooking oil fumes-derived PM2.5-induced cell damage through its anti-inflammatory effects in cardiomyocytes. Ecotoxicol Environ. Saf. 179, 249–256. doi: 10.1016/j.ecoenv.2019.04.064
Lv, N., Zhao, M., Han, Y., Cui, L., Zhong, W., Wang, C., et al. (2018). The roles of bone morphogenetic protein 2 in perfluorooctanoic acid induced developmental cardiotoxicity and l-carnitine mediated protection. Toxicol. Appl. Pharmacol. 352:, 68–76. doi: 10.1016/j.taap.2018.05.028
Massarsky, A., Jayasundara, N., Bailey, J. M., Oliveri, A. N., Levin, E. D., Prasad, G. L., et al. (2015). Teratogenic, bioenergetic, and behavioral effects of exposure to total particulate matter on early development of zebrafish (Danio rerio) are not mimicked by nicotine. Neurotoxicol Teratol 51, 77–88. doi: 10.1016/j.ntt.2015.09.006
Qin, G., Xia, J., Zhang, Y., Guo, L., Chen, R., Sang, N. (2018). Ambient fine particulate matter exposure induces reversible cardiac dysfunction and fibrosis in juvenile and older female mice. Part Fibre Toxicol. 15, 27. doi: 10.1186/s12989-018-0264-2
Rao, X., Zhong, J., Brook, R. D., Rajagopalan, S. (2018). Effect of Particulate Matter Air Pollution on Cardiovascular Oxidative Stress Pathways. Antioxid Redox Signal 28, 797–818. doi: 10.1089/ars.2017.7394
Ryu, Y. S., Kang, K. A., Piao, M. J., Ahn, M. J., Yi, J. M., Hyun, Y. M., et al. (2019). Particulate matter induces inflammatory cytokine production via activation of NFkappaB by TLR5-NOX4-ROS signaling in human skin keratinocyte and mouse skin. Redox Biol. 21, 101080. doi: 10.1016/j.redox.2018.101080
Shankar, P., Geier, M. C., Truong, L., McClure, R. S., Pande, P., Waters, K. M., et al. (2019). Coupling Genome-wide Transcriptomics and Developmental Toxicity Profiles in Zebrafish to Characterize Polycyclic Aromatic Hydrocarbon (PAH) Hazard. Int. J. Mol. Sci. 20, 2570. doi: 10.3390/ijms20102570
Shi, Q., Wang, W., Chen, M., Zhang, H., Xu, S. (2019). Ammonia induces Treg/Th1 imbalance with triggered NF-kappaB pathway leading to chicken respiratory inflammation response. Sci. Total Environ. 659, 354–362. doi: 10.1016/j.scitotenv.2018.12.375
Song, I. H., Jung, K. J., Lee, T. J., Kim, J. Y., Sung, E. G., Bae, Y. C., et al. (2018). Mesenchymal stem cells attenuate adriamycin-induced nephropathy by diminishing oxidative stress and inflammation via downregulation of the NF-kB. Nephrol. (Carlton) 23, 483–492. doi: 10.1111/nep.13047
Teeguarden, J. G., Dragan, Y., Pitot, H. C. (2000). Hazard assessment of chemical carcinogens: the impact of hormesis. J. Appl. Toxicol. 20, 113–120. doi: 10.1002/(SICI)1099-1263(200003/04)20:2<113::AID-JAT641>3.0.CO;2-9
Teng, C., Wang, Z., Yan, B. (2016). Fine particle-induced birth defects: Impacts of size, payload, and beyond. Birth Defects Res. C Embryo Today 108, 196–206. doi: 10.1002/bdrc.21136
Vancamp, P., Darras, V. M. (2017). Dissecting the role of regulators of thyroid hormone availability in early brain development: Merits and potential of the chicken embryo model. Mol. Cell Endocrinol. 459, 71–78. doi: 10.1016/j.mce.2017.01.045
Vergara, M. N., Canto-Soler, M. V. (2012). Rediscovering the chick embryo as a model to study retinal development. Neural Dev. 7, 22. doi: 10.1186/1749-8104-7-22
Vonk Noordegraaf, A., Westerhof, B. E., Westerhof, N. (2017). The Relationship Between the Right Ventricle and its Load in Pulmonary Hypertension. J. Am. Coll. Cardiol. 69, 236–243. doi: 10.1016/j.jacc.2016.10.047
Wang, H., Guo, Y., Liu, L., Guan, L., Wang, T., Zhang, L., et al. (2016). DDAH1 plays dual roles in PM2.5 induced cell death in A549 cells. Biochim. Biophys. Acta 1860, 2793–2801. doi: 10.1016/j.bbagen.2016.03.022
Wang, Z., Gao, S., Xie, J., Li, R. (2019). Identification of multiple dysregulated metabolic pathways by GC-MS-based profiling of lung tissue in mice with PM2.5-induced asthma. Chemosphere 220, 1–10. doi: 10.1016/j.chemosphere.2018.12.092
Wang, Q., Kwan, M. P., Zhou, K., Fan, J., Wang, Y., Zhan, D. (2019a). The impacts of urbanization on fine particulate matter (PM2.5) concentrations: Empirical evidence from 135 countries worldwide. Environ. Pollut. 247, 989–998. doi: 10.1016/j.envpol.2019.01.086
Wang, Q., Liang, Q., Li, C., Ren, M., Lin, S., Knibbs, L. D., et al. (2019b). Interaction of Air Pollutants and Meteorological Factors on Birth Weight in Shenzhen, China. Epidemiology 30 Suppl 1, S57–S66. doi: 10.1097/EDE.0000000000000999
Wei, Y., Cao, X. N., Tang, X. L., Shen, L. J., Lin, T., He, D. W., et al. (2018). Urban fine particulate matter (PM2.5) exposure destroys blood-testis barrier (BTB) integrity through excessive ROS-mediated autophagy. Toxicol. Mech. Methods 28, 302–319. doi: 10.1080/15376516.2017.1410743
Wilmes, V., Scheiper, S., Roehr, W., Niess, C., Kippenberger, S., Steinhorst, K., et al. (2020). Increased inducible nitric oxide synthase (iNOS) expression in human myocardial infarction. Int. J. Legal Med. 134, 575–581. doi: 10.1007/s00414-019-02051-y
Wilson, W. E., Suh, H. H. (1997). Fine particles and coarse particles: concentration relationships relevant to epidemiologic studies. J. Air Waste Manag Assoc. 47, 1238–1249. doi: 10.1080/10473289.1997.10464074
Wittig, J. G., Munsterberg, A. (2019). The Chicken as a Model Organism to Study Heart Development. Cold Spring Harb Perspect. Biol. a037218. doi: 10.1101/cshperspect.a037218
Xing, Y. F., Xu, Y. H., Shi, M. H., Lian, Y. X. (2016). The impact of PM2.5 on the human respiratory system. J. Thorac. Dis. 8, E69– 74. doi: 10.3978/j.issn.2072-1439.2016.01.19
Xue, T., Zhu, T., Geng, G., Zhang, Q. (2019). Association between pregnancy loss and ambient PM2.5 using survey data in Africa: a longitudinal case-control study 1998-2016. Lancet Planet Health 3, e219–ee225. doi: 10.1016/S2542-5196(19)30047-6
Yang, B., Guo, J., Xiao, C. (2018). Effect of PM2.5 environmental pollution on rat lung. Environ. Sci. Pollut. Res. Int. 25, 36136–36146. doi: 10.1007/s11356-018-3492-y
Yang, W., Guo, M., Liu, G., Yu, G., Wang, P., Wang, H., et al. (2018). Detection and analysis of fine particulate matter and microbial aerosol in chicken houses in Shandong Province, China. Poult Sci. 97, 995–1005. doi: 10.3382/ps/pex388
Yu, G., Wang, F., Hu, J., Liao, Y., Liu, X. (2019). Value Assessment of Health Losses Caused by PM2.5 in Changsha City, China. Int. J. Environ. Res. Public Health 16, 2063. doi: 10.3390/ijerph16112063
Yu, J., Chen, L. H., Zhang, B., Zheng, Q. M. (2019). . The modulation of endometriosis by lncRNA MALAT1 via NF-kappaB/iNOS. Eur. Rev. Med. Pharmacol. Sci. 23, 4073–4080. doi: 10.26355/eurrev_201905_17908
Yue, C., Ji, C., Zhang, H., Zhang, L. W., Tong, J., Jiang, Y., et al. (2017). Protective effects of folic acid on PM2.5-induced cardiac developmental toxicity in zebrafish embryos by targeting AhR and Wnt/beta-catenin signal pathways. Environ. Toxicol. 32, 2316–2322. doi: 10.1002/tox.22448
Yue, W., Tong, L., Liu, X., Weng, X., Chen, X., Wang, D., et al. (2019). Short term Pm2.5 exposure caused a robust lung inflammation, vascular remodeling, and exacerbated transition from left ventricular failure to right ventricular hypertrophy. Redox Biol. 22, 101161. doi: 10.1016/j.redox.2019.101161
Zhang, H., Zhao, Z., Pang, X., Yang, J., Yu, H., Zhang, Y., et al. (2017). Genistein Protects Against Ox-LDL-Induced Inflammation Through MicroRNA-155/SOCS1-Mediated Repression of NF-kB Signaling Pathway in HUVECs. Inflammation 40, 1450–1459. doi: 10.1007/s10753-017-0588-3
Zhang, H., Xue, L., Li, B., Tian, H., Zhang, Z., Tao, S. (2018). Therapeutic potential of bixin in PM2.5 particles-induced lung injury in an Nrf2-dependent manner. Free Radic. Biol. Med. 126, 166–176. doi: 10.1016/j.freeradbiomed.2018.08.015
Zhang, Y., Li, S., Li, J., Han, L., He, Q., Wang, R., et al. (2018). Developmental toxicity induced by PM2.5 through endoplasmic reticulum stress and autophagy pathway in zebrafish embryos. Chemosphere 197, 611–621. doi: 10.1016/j.chemosphere.2018.01.092
Zhang, S., Huo, X., Zhang, Y., Huang, Y., Zheng, X., Xu, X. (2019). Ambient fine particulate matter inhibits innate airway antimicrobial activity in preschool children in e-waste areas. Environ. Int. 123, 535–542. doi: 10.1016/j.envint.2018.12.061
Zhao, J., Gao, Z., Tian, Z., Xie, Y., Xin, F., Jiang, R., et al. (2013). The biological effects of individual-level PM(2.5) exposure on systemic immunity and inflammatory response in traffic policemen. Occup. Environ. Med. 70, 426–431. doi: 10.1136/oemed-2012-100864
Keywords: particulate matter 2.5, developmental toxicity, chicken embryo, nuclear factor kappa-light-chain-enhancer of activated B cells, inducible nitric oxide synthase, matrix metallopeptidase 9
Citation: Jiang Q, Zhang C, Chen S, Shi L, Li DC, Lv N, Cui L, Chen Y and Zheng Y (2020) Particulate Matter 2.5 Induced Developmental Cardiotoxicity in Chicken Embryo and Hatchling. Front. Pharmacol. 11:841. doi: 10.3389/fphar.2020.00841
Received: 01 March 2020; Accepted: 21 May 2020;
Published: 05 June 2020.
Edited by:
Eleonore Fröhlich, Medical University of Graz, AustriaReviewed by:
Ekhtear Hossain, Louisiana State University, United StatesWei Zi Zhang, Universidade do Grande Rio Professor José de Souza Herdy, Brazil
Copyright © 2020 Jiang, Zhang, Chen, Shi, Li, Lv, Cui, Chen and Zheng. This is an open-access article distributed under the terms of the Creative Commons Attribution License (CC BY). The use, distribution or reproduction in other forums is permitted, provided the original author(s) and the copyright owner(s) are credited and that the original publication in this journal is cited, in accordance with accepted academic practice. No use, distribution or reproduction is permitted which does not comply with these terms.
*Correspondence: Yuxin Zheng, eXh6aGVuZ0BxZHUuZWR1LmNu