- 1Department of Medical Chemistry and Biochemistry, Faculty of Medicine and Dentistry, Palacky University, Olomouc, Czechia
- 2Department of Pharmacology, Faculty of Medicine and Dentistry, Palacky University, Olomouc, Czechia
- 3Department of Medical Biophysics, Faculty of Medicine and Dentistry, Palacky University, Olomouc, Czechia
- 4Institute of Microbiology, Czech Academy of Sciences, Novy Hradek, Czechia
Sexual differences and the composition/function of the gut microbiome are not considered the most important players in the drug metabolism field; however, from the recent data it is obvious that they may significantly affect the response of the patient to therapy. Here, we evaluated the effect of microbial colonization and sex differences on mRNA expression and the enzymatic activity of hepatic cytochromes P450 (CYPs) in germ-free (GF) mice, lacking the intestinal flora, and control specific-pathogen-free (SPF) mice. We observed a significant increase in the expression of Cyp3a11 in female SPF mice compared to the male group. However, the sex differences were erased in GF mice, and the expression of Cyp3a11 was about the same in both sexes. We have also found higher Cyp2c38 gene expression in female mice compared to male mice in both the SPF and GF groups. Moreover, these changes were confirmed at the level of enzymatic activity, where the female mice exhibit higher levels of functional CYP2C than males in both groups. Interestingly, we observed the same trend as with CYP3A enzymes: a diminished difference between the sexes in GF mice. The presented data indicate that the mouse gut microbiome plays an important role in sustaining sexual dimorphism in terms of hepatic gene expression and metabolism.
Introduction
The gut microbiome, an aggregate genome of trillions of microorganisms, provides a wide range of beneficial functions for the host and has an immense effect on the host’s health status and predisposition to disease (Kinross et al., 2011). This ecosystem of bacteria, archaea, viruses, and unicellular eukaryotes is mostly stable in the long run, but transiently they may be affected by many factors (Schlomann and Parthasarathy, 2019). Among the wide range of factors that influence the composition of the gut microbiota, diet seems to be the most potent (Wilson et al., 2020). Although the effect of sex appears to be less influential, some studies highlight sex-based differences in the gut microbiome (Markle et al., 2013; Haro et al., 2016; Org et al., 2016). Also, the interactions between the diet and gut microbiota tended to be sex-dependent (Bolnick et al., 2014). It is now clear that without this bacterial community, the immune system and other physiological processes would never reach their full potential (Tlaskalová-Hogenová et al., 1983), and an imbalance in its composition leads to a broad spectrum of pathological conditions. Moreover, studies have shown that the gut microbiota influence the metabolism and toxicity of xenobiotics (Sousa et al., 2008; Jourova et al., 2016; Wilson and Nicholson, 2017) with an impact on their oral bioavailability and pharmacokinetics (Yoo et al., 2014; Jourova et al., 2019).
Cytochromes P450 (CYPs) are key enzymes involved in the initial metabolism of drugs in humans, including 70–80% of all drugs in clinical use (Anzenbacher and Anzenbacherová, 2001). The expression of these enzymes is under the control of specific nuclear receptors (Hakkola et al., 2018), and their regulation is influenced by many factors such as genetic polymorphisms, regulation by cytokines, pathological states, age, etc. (Zanger and Schwab, 2013). In addition, the gut microbiome mentioned above has been shown to play an important role in this, and its effect on the expression of CYP enzymes has been published recently (Matuskova et al., 2010; Selwyn et al., 2015; Selwyn et al., 2016; Jourova et al., 2017). Further, sex influences a number of important pharmacokinetic parameters including the expression of drug-metabolizing enzymes and transporters (Yang et al., 2012). It has been also found that sex differences together with circadian rhythmicity influence the CYPs and nuclear receptor expression in the mouse liver (Lu et al., 2013).
Sexual differences and the composition/function of the gut microbiome are not considered the most important players in the drug metabolism field; however, from the recent data it is obvious that they may significantly affect the patient’s response to therapy. Deeper knowledge of their combined effect on the function of CYPs may be helpful for predicting pharmacokinetics and drug response in a particular patient and avoiding undesirable side effects, which is especially important for drugs with a narrow therapeutic index.
Here, we evaluated the effect of microbial colonization and sex differences on the mRNA expression and enzymatic activity of hepatic cytochromes P450 (CYPs) in germ-free (GF) mice, lacking the intestinal flora, and control specific-pathogen-free (SPF) mice.
Materials and Methods
Chemicals
Protease inhibitor cocktail tablets (EDTA free Complete Protease Inhibitor Cocktail Tablets) were supplied by Roche (Prague, Roche, Czech Republic). Hydrochloric acid (p.a., 37%) was supplied by Penta (Prague, Czech Republic), and sodium chloride and dimethyl sulfoxide were obtained from Lach-Ner (Neratovice, Czech Republic). Acetonitrile was supplied by VWR International (Prague, Czech Republic) and was obtained in the highest purity available.
Substrates of orthologous human CYP forms for the determination of murine CYP activities (ethoxyresorufin, diclofenac, diazepam, bufuralol) and their respective metabolites were supplied by Sigma-Aldrich CZ (Prague, Czech Republic). Midazolam was purchased from Abcam (Cambridge, UK). Other chemicals were purchased from Sigma-Aldrich CZ (Prague, Czech Republic). All chemicals were of the highest purity available.
Animals and Experimental Design
Male and female germ-free (GF) and specific-pathogen-free (SPF) inbred BALB/c two-month-old mice were used for the experiment (5 animals per group). GF mice were born and housed under sterile conditions in Trexler-type plastic isolators and fed a 50 kGy irradiated sterile pellet diet of Altromin 1410 (Altromin, Lage, Germany) and sterile water ad libitum. Axenicity was assessed every two weeks by confirming the absence of bacteria, moulds, and yeast by aerobic and anaerobic cultivation of mouse feces and swabs from the isolators in VL (Viande-Levure), Sabouraud-dextrose, and meat-peptone broth and subsequent plating on blood, Sabouraud, and VL agar plates. SPF mice were kept in IVC cages (Tecniplast, Italy) and fed with the same sterile diet as their gnotobiotic counterparts. SPF mice were regularly checked for the absence of potential pathogens according to an internationally established standard (FELASA). Animals were kept in a room with a 12 h light-dark cycle at 22oC. Two-month-old mice were used for our experiments.
The mice were euthanized at the age of 8–12 weeks by cervical dislocation and exsanguination. The livers were aseptically removed, weighed, frozen in liquid nitrogen, and subsequently stored at -70°C until further processing.
Preparation of Subcellular Fractions
Microsomal fractions were obtained from the liquid nitrogen-frozen liver of mice. Microsomes were prepared by differential centrifugation according to established protocols (Lake, 1987). The buffer for homogenization was supplemented with protease inhibitor cocktail tablets (Roche CZ, Prague). Microsomal fractions were stored at −80°C. Protein concentrations in the microsomal fractions were assayed using a bicinchonic acid assay according to the established method (Smith et al., 1985). The concentration of CYP enzymes in liver microsomes was determined using difference spectroscopy (Zhang et al., 2010).
RNA Isolation and Quantitative Real-Time PCR (qPCR)
Total RNA was isolated from tissue samples stored in RNAlater (Qiagen, Dynex, Czech Republic) using an RNeasy Plus Mini Kit (Qiagen). RNA concentration and purity was determined spectrophotometrically, and the RNA integrity was verified by gel electrophoresis. First strand cDNA was synthesized from total RNA with a Transcriptor High-Fidelity cDNA synthesis kit (Roche, Prague, Czech Republic). Real-time PCR for CYPs quantification was performed in a LightCycler 1536 Instrument (Roche, Prague, Czech Republic) using specific TaqMan Gene Expression Assays (Applied Biosystems, Life Technologies, Prague, Czech Republic). The 1536-well plates were pipetted using an Automate Labcyte Echo (Dublin, Ireland).
The calculations were based on the “Delta-Delta Ct method” (Livak and Schmittgen, 2001). The data was expressed as the fold change in the treatment groups relative to the control, and HPRT1 and 18S RNA were used as an internal control.
Enzyme Assays
Enzyme activities were assayed in the microsomal fractions from the homogenate of mouse liver. The amount of organic solvents in the final reaction mixtures did not exceed 1% (v/v). The enzyme activities of selected CYP enzymes were measured according to the established method (Kronbach et al., 1989; Phillips and Shephard, 2006). For the determination of murine CYP activities, substrates of orthologous human CYP forms were used: CYP1A1/2, ethoxyresorufin (murine CYP1A2); CYP2C9, diclofenac (murine 2C subfamily); CYP2C19, diazepam (murine 2C subfamily); CYP2D6, bufuralol (murine 2D22) and CYP3A4, midazolam (murine CYP3A11 and 3A13). All activities were measured using a Shimadzu LC-20 HPLC system (Shimadzu, Kyoto, Japan) with UV or fluorescence detection being used for the determination of metabolites. The measurements were performed in a LiChrospher RP-18 column or a Chromolith® High Resolution RP-18 endcapped column (determination of midazolam substrate) (Merck, Germany).
Statistics
The normal distribution of data was tested using Shapiro-Wilk test. The statistical significance of gene expression was determined by two-way ANOVA using software IBM SPSS Statistics for Windows, Version 23.0 (Armonk, NY: IBM Corp.). Differences were regarded as statistically significant when the p-value was lower than 0.05. Software GraphPad Prism 8 (GraphPad Software Inc., California, USA) was used to create the graphs. Due to the scarcity of material, the statistical significance of activity assays could not be determined.
Results
We investigated the role of sex and presence/absence of the gut microbiome on the gene expression and enzymatic activity of CYPs in the liver using germ-free (GF) mice, lacking the intestinal flora and specific-pathogen-free (SPF) mice. SPF male data were used as a control group.
The Cyp3a11 mRNA was significantly increased (21 times) in female SPF mice compared to their male counterparts. Interestingly, these phenomena were not observed in GF mice, where the GF female mice have shown rather decreasing tendency compared to other groups (Figure 1).
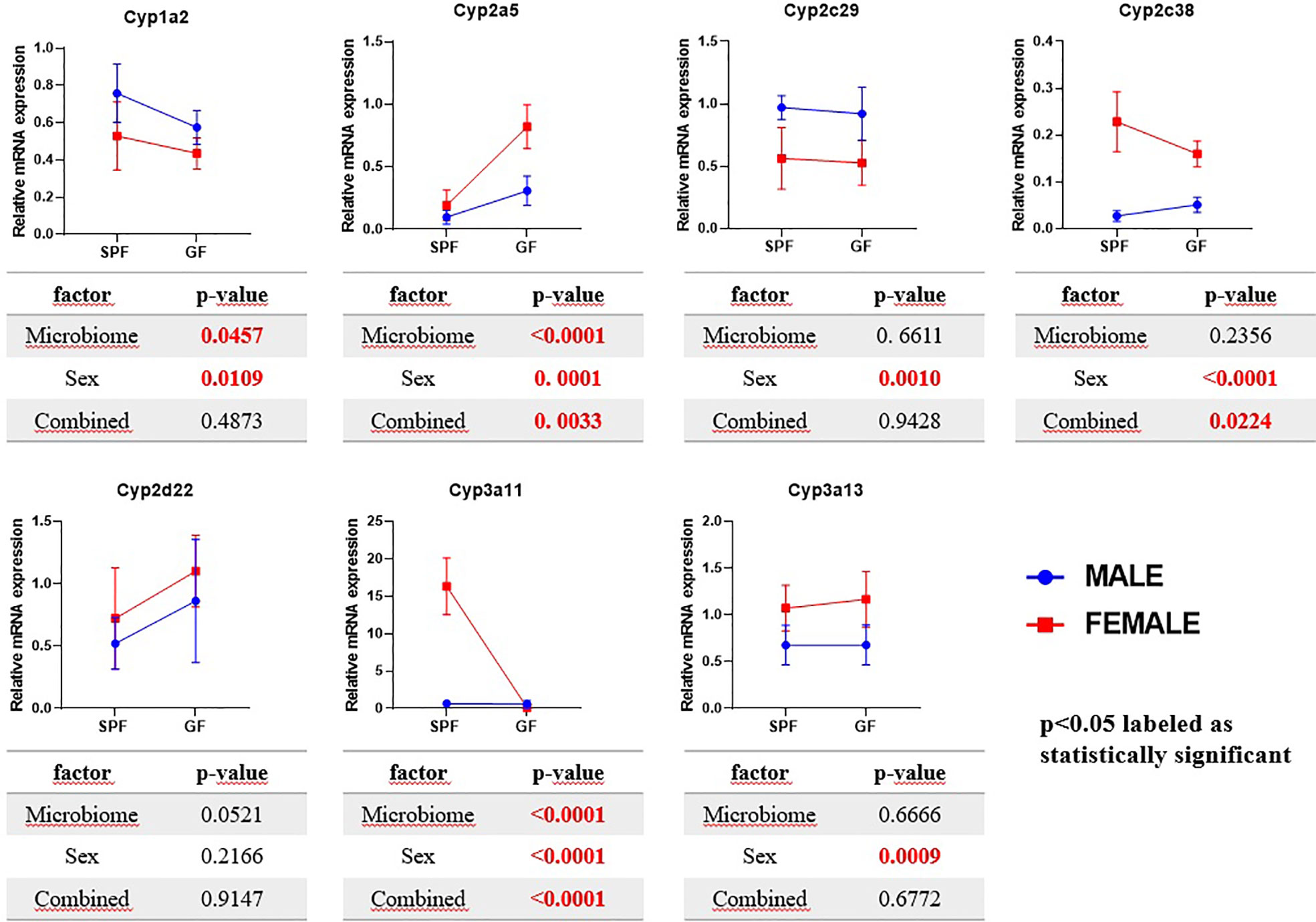
Figure 1 Comparison of estimated marginal mean values of mRNA expression of selected Cyps in relation with microbiome presence and sex in mice liver. The data represent the mean ±SD from 4–5 individual animals. The statistical significance was determined using two-way ANOVA and p-values for the effect of microbiome (i.e., difference between SPF and GF groups), sex and combined effect of the both are shown in the tables below the respective graphs.
The expression of Cyp3a13 was slightly increased in the females of both the group SPF and GF mice. The Cyp2a5 mRNA expression was significantly increased in GF female mice, but not the SPF female group, compared to control. Moreover, the expression of the Cyp2c38 gene was significantly increased in the females in both groups, GF and SPF, but to a larger extent in SPF mice. In the case of the Cyp1a2 and Cyp2c29, the mRNA expression was significantly decreased in SPF and GF female mice compared to the control (Figure 1).
The females of both groups (GF and SPF) exhibited a much greater enzymatic activity of 2C (using diclofenac as substrate) than the male groups (Table 1). The effect of gut microbiome was also observed as the GF male and female have shown increased 2C enzymatic activity compared to their SPF counterparts (Table 1). The enzymatic activity of CYP3A was significantly decreased in GF mice compared to SPF mice. In the female groups, the activity of CYP3A did not differ significantly from the male groups but had a tendency to decrease (Table 1). The other selected CYP activities were not significantly changed by the gut microbiome presence or sex (Table 1).
Discussion
Recent studies clearly show that the gut microbiota plays an indispensable role in the equation leading to interindividual variation in response to therapy. For this reason, to reach the desired goal of “personalized medicine,” in addition to other factors, we have to take into account our 100 trillion bacterial inhabitants and their genomes with substantial metabolic potential.
The effect of the gut microbiota on the hepatic gene expression of some CYPs in mice has been previously studied (Claus et al., 2011; Selwyn et al., 2015; Selwyn et al., 2016; Jourova et al., 2017). Most of these experiments, however, were only done with male mice and did not consider sex-specific differences. However, sex differences were among the main variables influencing the hepatic drug metabolism in rodents (Corton et al., 2012). Moreover, some animal and human studies pointed out sex/gender-related differences in gut microbiota composition (Markle et al., 2013; Haro et al., 2016; Org et al., 2016). Little is known about the combined effect of both factors. Thus, we assumed not only the effect of the microbiome but also sex differences in the presence/absence of gut microbiota on the regulation of liver biotransformation enzymes.
In rodents, sex differences are one of the most important factors influencing drug metabolism in the liver (Corton et al., 2012). Further, mice females have been shown to have a higher expression of most Cyp genes (Renaud et al., 2011; Lu et al., 2013). A higher expression of Cyp3a11 in female mice than males was reported earlier (Hernandez et al., 2009; Renaud et al., 2011). Even though in humans the differences are subtler, it is known that the CYP3A4 activity features apparent sexual dimorphism with higher activity in women due to differences in growth hormone patterns (Waxman and O’Connor, 2006). In line with these data, we observed a significant increase in the expression of Cyp3a11 in female SPF mice compared to the male group. Interestingly, the sex differences were erased in GF mice, and the expression of Cyp3a11 was about the same in both sexes. In other words, there is significant interaction between the gender and presence of the gut microbiome, which together influence the Cyp3a11 mRNA expression. A recent paper has proposed a possible explanation, showing that the mouse microbiome is required for sex-specific diurnal rhythms of gene expression and metabolism (Weger et al., 2019). The enzymatic activity of CYP3A (using midazolam as a substrate) in both the male and female mice lacking the microbiota was significantly decreased compared to their SPF counterparts. These results are in line with our previous study on the GF and SPF male mice (Jourova et al., 2017). Further we have found that the activity of CYP3A did not differ significantly in female groups from the male groups, but had a tendency to decrease. This data suggests that the down-regulation of CYP3A enzymes may not be sex-specific and, above all, that the gut microbiome is crucial in the synthesis of CYP3A in both sexes.
Moreover, we have found a higher Cyp2c38 mRNA expression in female mice compared to male mice in both SPF and GF groups. Moreover, these changes were confirmed at the level of enzymatic activity (using diclofenac as substrate), when female mice exhibit higher levels of functional CYP2C than males in both mice groups. Interestingly, we observed the same trend as with CYP3A enzymes: diminishing the changes between sexes in GF mice. The increase in Cyp2c38 expression in the female group compared to the male was almost two times lower in GF mice than in SPF mice (2 times in the case of enzymatic activity of CYP2C). In other words, sex differences in Cyp2c38 expression and the enzymatic activity of CYP2C were less pronounced in GF mice. Therefore, not only the effect of sex but also the combined effect of gut microbiome and sex was found significant in regulation of Cyp2c38 mRNA expression in the liver of mice. In humans, CYP2C and CYP3A enzymes are abundantly present in the liver and, completely or partially, metabolize a large fraction of all prescribed drugs (Anzenbacher and Anzenbacherová, 2001). The presented data thus highlight the possible modulation of the response to a broad spectrum of clinically used drugs by the interaction of gut microbiota and the sex-based hormonal level.
As mentioned in the Introduction, the regulation of the CYP expression is very complex and many factors play a role here (Zanger and Schwab, 2013), and among them, the gut microbiome is gaining the increasing attention of scientists. After all, the gastrointestinal tract, with 100 trillion microbes, is a rich resource of microbial metabolites that may be responsible for the indirect effect of gut microbiome on whole-body metabolism (Lee and Hase, 2014). Moreover, novel potential mechanism of action of the gut microbiome in regulation of host metabolism was defined recently by Weger et al. (2019). They have found that the absence of the microbiome reduces liver sexual dimorphism. In other words, microbial metabolites contribute significantly to maintaining the sex differences in gene expression and metabolism by promoting proper sexual development and growth hormone secretion (Weger et al., 2019). These effects are often associated with activation of nuclear receptors such as aryl hydrocarbon receptor (AhR) and pregnane X receptor (PXR), which participate in regulation of CYPs expression (Zhang et al., 2010; Huang et al., 2016). CYP enzymes, however, are not only involved in detoxification of xenobiotics, but they are involved as well in the synthesis of steroid hormones, eicosanoids, prostaglandins, and thromboxanes (Nebert and Russell, 2002). CYP3A4 contributes to the oxidation of many steroids (Niwa et al., 2015), and its activity in humans is sexually dimorphic (Lamba et al., 2010). The exact mechanism for sex differences in CYP3A4 (and other CYPs) remains elusive but may include altered growth hormone signalling (Waxman and Holloway, 2009). All data mentioned above open the possibility of an important involvement of microbiome-derived metabolites in the signalling pathways regulating hepatic drug metabolism and sexual dimorphism in gene expression.
In conclusion, the current data and the literature indicate that the gut microbiome plays an important role in sustaining the sexual dimorphism in hepatic gene expression and metabolism. As the concept of modulating the gut microbiome to improve health may provide promising therapeutic effects, further studies are needed to explain in more detail the role of the gut microbiota, along with other factors, in the pathways that participate in the regulation of CYP synthesis and drug metabolism. Better understanding the ways gut microbiota may influence drug metabolism could make a significant contribution to the improvement of pharmacotherapy (e.g., in better efficacy and in avoiding the undesirable side effects).
Data Availability Statement
The raw data supporting the conclusions of this article will be made available by the authors, without reservation, to any qualified researcher.
Ethics Statement
The animal study was reviewed and approved by The experiment was carried out in accordance with Czech Act No. 359/2012 Coll. for the protection of animals against abuse. All procedures with animals were approved by the Ethics Committee, Ministry of Education of the Czech Republic. Experiments were approved by the Committee for the Protection and Use of Experimental Animals of the Institute of Microbiology. v.v.i., Academy of Sciences of the Czech Republic (approval ID: 50/2011).
Author Contributions
LJ measured and analyzed the data and, with the help and supervision of EA and PA, wrote the paper. MV measured the enzymatic activity. NZ measured the gene expression. KL performed the statistical analysis. PH and TH performed the experiment with germ-free mice. All authors contributed to the article and approved the submitted version.
Funding
This work was supported by The Czech Science Foundation (grant no. 19-08294S) and by project Toxicology CZ.02.2.69/0.0/0.0/16_018/0002311.
Conflict of Interest
The authors declare that the research was conducted in the absence of any commercial or financial relationships that could be construed as a potential conflict of interest.
References
Anzenbacher, P., Anzenbacherová, E. (2001). Cytochromes P450 and metabolism of xenobiotics. Cell. Mol. Life Sci. CMLS. 58, 737–747. doi: 10.1007/pl00000897
Bolnick, D., II, Snowberg, L. K., Hirsch, P. E., Lauber, C. L., Org, E., Parks, B., et al. (2014). Individual diet has sex-dependent effects on vertebrate gut microbiota. Nat. Communications 5, 4500. doi: 10.1038/ncomms5500
Claus, S. P., Ellero, S. L., Berger, B., Krause, L., Bruttin, A., Molina, J., et al. (2011). Colonization-induced host-gut microbial metabolic interaction. mBio 2, e00271–e00210. doi: 10.1128/mBio.00271-10
Corton, J. C., Bushel, P. R., Fostel, J., O’Lone, R. B. (2012). Sources of variance in baseline gene expression in the rodent liver. Mutat. Res. 746, 104–112. doi: 10.1016/j.mrgentox.2011.12.017
Hakkola, J., Bernasconi, C., Coecke, S., Richert, L., Andersson, T. B., Pelkonen, O. (2018). Cytochrome P450 Induction and Xeno-Sensing Receptors Pregnane X Receptor, Constitutive Androstane Receptor, Aryl Hydrocarbon Receptor and Peroxisome Proliferator-Activated Receptor alpha at the Crossroads of Toxicokinetics and Toxicodynamics. Basic Clin. Pharmacol. Toxicol. 123 Suppl 5, 42–50. doi: 10.1111/bcpt.13004
Haro, C., Rangel-Zúñiga, O. A., Alcalá-Díaz, J. F., Gómez-Delgado, F., Pérez-Martínez, P., Delgado-Lista, J., et al. (2016). Intestinal Microbiota Is Influenced by Gender and Body Mass Index. PloS One 11, e0154090. doi: 10.1371/journal.pone.0154090
Hernandez, J. P., Mota, L. C., Huang, W., Moore, D. D., Baldwin, W. S. (2009). Sexually dimorphic regulation and induction of P450s by the constitutive androstane receptor (CAR). Toxicology 256, 53–64. doi: 10.1016/j.tox.2008.11.002
Huang, B., Butler, R., Miao, Y., Dai, Y., Wu, W., Su, W., et al. (2016). Dysregulation of Notch and ERα signaling in AhR–/– male mice. Proc. Natl. Acad. Sci. 113, 11883–11888. doi: 10.1073/pnas.1613269113
Jourova, L., Anzenbacher, P., Anzenbacherova, E. (2016). Human gut microbiota plays a role in the metabolism of drugs. BioMed. Pap. Med. Fac. Univ. Palacky Olomouc Czech Repub. 160, 317–326. doi: 10.5507/bp.2016.039
Jourova, L., Anzenbacher, P., Liskova, B., Matuskova, Z., Hermanova, P., Hudcovic, T., et al. (2017). Colonization by non-pathogenic bacteria alters mRNA expression of cytochromes P450 in originally germ-free mice. Folia Microbiol. (Praha) 62, 463–469. doi: 10.1007/s12223-017-0517-8
Jourova, L., Anzenbacher, P., Matuskova, Z., Vecera, R., Strojil, J., Kolar, M., et al. (2019). Gut microbiota metabolizes nabumetone in vitro: Consequences for its bioavailability in vivo in the rodents with altered gut microbiome. Xenobiotica; fate foreign compounds Biol. Syst. 49, 1296–1302. doi: 10.1080/00498254.2018.1558310
Kinross, J. M., Darzi, A. W., Nicholson, J. K. (2011). Gut microbiome-host interactions in health and disease. Genome Med. 3, 14. doi: 10.1186/gm228
Kronbach, T., Mathys, D., Umeno, M., Gonzalez, F. J., Meyer, U. A. (1989). Oxidation of midazolam and triazolam by human liver cytochrome P450IIIA4. Mol. Pharmacol. 36, 89–96.
Lamba, V., Panetta, J. C., Strom, S., Schuetz, E. G. (2010). Genetic predictors of interindividual variability in hepatic CYP3A4 expression. J. Pharmacol. Exp. Ther. 332, 1088–1099. doi: 10.1124/jpet.109.160804
Lee, W. J., Hase, K. (2014). Gut microbiota-generated metabolites in animal health and disease. Nat. Chem. Biol. 10, 416–424. doi: 10.1038/nchembio.1535
Livak, K. J., Schmittgen, T. D. (2001). Analysis of relative gene expression data using real-time quantitative PCR and the 2(-Delta Delta C(T)) Method. Methods (San Diego Calif.) 25, 402–408. doi: 10.1006/meth.2001.1262
Lu, Y. F., Jin, T., Xu, Y., Zhang, D., Wu, Q., Zhang, Y. K., et al. (2013). Sex differences in the circadian variation of cytochrome p450 genes and corresponding nuclear receptors in mouse liver. Chronobiol. Int. 30, 1135–1143. doi: 10.3109/07420528.2013.805762
Markle, J. G., Frank, D. N., Mortin-Toth, S., Robertson, C. E., Feazel, L. M., Rolle-Kampczyk, U., et al. (2013). Sex differences in the gut microbiome drive hormone-dependent regulation of autoimmunity. Sci. (New York NY) 339, 1084–1088. doi: 10.1126/science.1233521
Matuskova, Z., Tunkova, A., Anzenbacherova, E., Vecera, R., Siller, M., Tlaskalova-Hogenova, H., et al. (2010). Effects of probiotic Escherichia coli Nissle 1917 on expression of cytochromes P450 along the gastrointestinal tract of male rats. Neuro Endocrinol. Lett. 31 Suppl 2, 46–50.
Nebert, D. W., Russell, D. W. (2002). Clinical importance of the cytochromes P450. Lancet (London Engl.) 360, 1155–1162. doi: 10.1016/s0140-6736(02)11203-7
Niwa, T., Murayama, N., Imagawa, Y., Yamazaki, H. (2015). Regioselective hydroxylation of steroid hormones by human cytochromes P450. Drug Metab. Rev. 47, 89–110. doi: 10.3109/03602532.2015.1011658
Org, E., Mehrabian, M., Parks, B. W., Shipkova, P., Liu, X., Drake, T. A., et al. (2016). Sex differences and hormonal effects on gut microbiota composition in mice. Gut Microbes 7, 313–322. doi: 10.1080/19490976.2016.1203502
Renaud, H. J., Cui, J. Y., Khan, M., Klaassen, C. D. (2011). Tissue distribution and gender-divergent expression of 78 cytochrome P450 mRNAs in mice. Toxicol. Sci. an Off. J. Soc. Toxicol. 124, 261–277. doi: 10.1093/toxsci/kfr240
Schlomann, B. H., Parthasarathy, R. (2019). Timescales of gut microbiome dynamics. Curr. Opin. Microbiol. 50, 56–63. doi: 10.1016/j.mib.2019.09.011
Selwyn, F. P., Cui, J. Y., Klaassen, C. D. (2015). RNA-Seq Quantification of Hepatic Drug Processing Genes in Germ-Free Mice. Drug Metab. Dispos. 43, 1572–1580. doi: 10.1124/dmd.115.063545
Selwyn, F. P., Cheng, S. L., Klaassen, C. D., Cui, J. Y. (2016). Regulation of Hepatic Drug-Metabolizing Enzymes in Germ-Free Mice by Conventionalization and Probiotics. Drug Metab. Dispos. 44, 262–274. doi: 10.1124/dmd.115.067504
Smith, P. K., Krohn, R., II, Hermanson, G. T., Mallia, A. K., Gartner, F. H., Provenzano, M. D., et al. (1985). Measurement of protein using bicinchoninic acid. Anal. Biochem. 150, 76–85. doi: 10.1016/0003-2697(85)90442-7
Sousa, T., Paterson, R., Moore, V., Carlsson, A., Abrahamsson, B., Basit, A. W. (2008). The gastrointestinal microbiota as a site for the biotransformation of drugs. Int. J. Pharm. 363, 1–25. doi: 10.1016/j.ijpharm.2008.07.009
Tlaskalová-Hogenová, H., Sterzl, J., Stĕpánkova, R., Dlabac, V., Vĕ, V., Rossmann, P., et al. (1983). Development of immunological capacity under germfree and conventional conditions. Ann. New York Acad. Sci. 409, 96–113. doi: 10.1111/j.1749-6632.1983.tb26862.x
Waxman, D. J., Holloway, M. G. (2009). Sex differences in the expression of hepatic drug metabolizing enzymes. Mol. Pharmacol. 76, 215–228. doi: 10.1124/mol.109.056705
Waxman, D. J., O’Connor, C. (2006). Growth hormone regulation of sex-dependent liver gene expression. Mol. Endocrinol. (Baltimore Md). 20, 2613–2629. doi: 10.1210/me.2006-0007
Weger, B. D., Gobet, C., Yeung, J., Martin, E., Jimenez, S., Betrisey, B., et al. (2019). The Mouse Microbiome Is Required for Sex-Specific Diurnal Rhythms of Gene Expression and Metabolism. Cell Metab. 29, 362–382 e368. doi: 10.1016/j.cmet.2018.09.023
Wilson, I. D., Nicholson, J. K. (2017). Gut microbiome interactions with drug metabolism, efficacy, and toxicity. Trans. Res. J. Lab. Clin. Med. 179, 204–222. doi: 10.1016/j.trsl.2016.08.002
Wilson, A. S., Koller, K. R., Ramaboli, M. C., Nesengani, L. T., Ocvirk, S., Chen, C., et al. (2020). Diet and the Human Gut Microbiome: An International Review. Digest. Dis. Sci. 65, 723–740. doi: 10.1007/s10620-020-06112-w
Yang, L., Li, Y., Hong, H., Chang, C. W., Guo, L. W., Lyn-Cook, B., et al. (2012). Sex Differences in the Expression of Drug-Metabolizing and Transporter Genes in Human Liver. J. Drug Metab. Toxicol. 3:1000119. doi: 10.4172/2157-7609.1000119
Yoo, D. H., Kim, I. S., Van Le, T. K., Jung, I. H., Yoo, H. H., Kim, D. H. (2014). Gut microbiota-mediated drug interactions between lovastatin and antibiotics. Drug Metab. Dispos. 42, 1508–1513. doi: 10.1124/dmd.114.058354
Zanger, U. M., Schwab, M. (2013). Cytochrome P450 enzymes in drug metabolism: Regulation of gene expression, enzyme activities, and impact of genetic variation. Pharmacol. Ther. 138, 103–141. doi: 10.1016/j.pharmthera.2012.12.007
Keywords: liver cytochromes P450, gut microbiome, sex difference, germ-free mice, metabolism of drugs
Citation: Jourová L, Vavreckova M, Zemanova N, Anzenbacher P, Langova K, Hermanova P, Hudcovic T and Anzenbacherova E (2020) Gut Microbiome Alters the Activity of Liver Cytochromes P450 in Mice With Sex-Dependent Differences. Front. Pharmacol. 11:01303. doi: 10.3389/fphar.2020.01303
Received: 03 April 2020; Accepted: 06 August 2020;
Published: 02 October 2020.
Edited by:
Ganna Tolstanova, Taras Shevchenko National University of Kyiv, UkraineReviewed by:
Martin Roderfeld, University of Giessen, GermanyBeshay Zordoky, University of Minnesota, United States
Copyright © 2020 Jourová, Vavreckova, Zemanova, Anzenbacher, Langova, Hermanova, Hudcovic and Anzenbacherova. This is an open-access article distributed under the terms of the Creative Commons Attribution License (CC BY). The use, distribution or reproduction in other forums is permitted, provided the original author(s) and the copyright owner(s) are credited and that the original publication in this journal is cited, in accordance with accepted academic practice. No use, distribution or reproduction is permitted which does not comply with these terms.
*Correspondence: Lenka Jourová, bGVua2Euam91cm92YUB1cG9sLmN6