- 1College of Life Sciences and Medicine, Zhejiang Sci-Tech University, Hangzhou, China
- 2Zhejiang Provincial Key Laboratory of Silkworm Bioreactor and Biomedicine, Zhejiang Sci-Tech University, Hangzhou, China
Gastrodia elata Blume (G. elata) is a valuable traditional Chinese medicine with neuroprotection, anti-inflammatory, and immune regulatory functions. MicroRNAs (miRNA) is a kind of endogenous noncoding small RNAs that plays distinctly important roles for gene regulation of organisms. So far, the research on G. elata is mainly focused on the pharmacological functions of the natural chemical ingredients, and the function of G. elata miRNA remains unknown. In this study, 5,718 known miRNAs and 38 novel miRNAs were identified by high-throughput sequencing from G. elata. Based on GO and KEGG analysis, we found that the human genes possibly regulated by G. elata miRNAs were related to the cell cycle, immune regulation, intercellular communication, etc. Furthermore, two novel miRNAs as Gas-miR01 and Gas-miR02 have stable and high expression in the medicinal tissues of G. elata. Further bioinformatics prediction showed that both Gas-miR01 and Gas-miR02 could target Homo sapiens A20 gene, furthermore, the dual-luciferase reporter gene assay and Western Blotting verified the interaction of Gas-miR01 or Gas-miR02 with A20. These evidences suggested that G. elata-unique miRNAs might be involved in certain physiological processes. The animal experiment showed that Gas-miR01 and Gas-miR02 could be detected in some tissues of mice by intragastric administration; meanwhile, the A20 expression in some tissues of mice was downregulated. These results supported for the functional study of G. elata miRNAs.
Introduction
Gastrodia elata (G. elata) Blume is a precious Chinese herbal medicine in China (Yuan et al., 2018). Many kinds of active ingredients in G. elata play an important role in the treatment of diseases. Since the 1950s, 81 compounds have been isolated from G. elata, including phenols, polysaccharides, and various sterols (Duan et al., 2013). Gastrodin is the main biologically active ingredient of G. elata (Liu et al., 2018).
In ancient China, G. elata was widely used to treat headache, dizziness, paralysis, epilepsy, joint pain, and numbness of limbs (Zhan et al., 2016). Some studies on its pharmacological properties have shown that G. elata has neuroprotective and antioxidant functions and could be used as an anti-inflammatory and antipsychotic drug (Kumar et al., 2013; Hu et al., 2014; Du et al., 2016; Wang et al., 2016). The pharmacological mechanisms of potential anticonvulsant activity of arrow G. elata have been extensively studied, which is similar to the mechanisms of known antiepileptic drugs (Wu et al., 2017). G. elata has also been found to treat anxiety, regulate the circulatory system, and improve memory (Niu et al., 2004). Additionally, G. elata could achieve its anti-aging effect by regulating certain signaling pathways (Song et al., 2016). Except for these, some chemical constituents such as anti-fungal protein GAFP- 1 in G. elata also play an important role. Certain phenols in G. elata can regulate the apoptosis-related signaling pathway by upregulating Bcl-2 and protect the nerves of mice (Wu et al., 1996). Recent studies have shown that phenolic glycoside gastrodin, the main component of G. elata, showed potential neuroprotective effects by inhibiting neurotoxic proinflammatory mediators (Li et al., 2018). G. elata has a high potential medicinal value. However, research on G. elata in China and abroad mainly focuses on the pharmacological functions and product development of natural chemical constituents of G. elata. To date, there is no research report on the miRNA and its function in G. elata.
MicroRNAs (miRNAs) are conserved small RNA molecules, which can negatively regulate target genes at the transcriptional and posttranscriptional level through near-perfect complementarity to target mRNA (Nam et al., 2011; Razaviyan et al., 2018). Over the past decade, more and more reports have paid attention to their cross-kingdom regulation (Wang et al., 2014; Oghbaei et al., 2017; Zhou et al., 2017). The regulation of human target genes by rice MIR168a was first discovered in 2012 (Zhang et al., 2012). In 2013, Yang et al. demonstrated that plant-derived small RNAs are present in animal serum and urine through two independent studies (Yang et al., 2015a; Yang et al., 2015b). In 2014, Zhang et al. found that honeysuckle had little degradation of miR2911 after the decoction, and further experiments in vitro and in vivo showed that honeysuckle could target influenza A virus (IAV), thereby inhibiting its replication and reducing mouse mortality (Zhou et al., 2015). Furthermore, these studies demonstrate that storage, processing, and cooking do not eliminate plant miRNAs in food sources and that plant-derived miRNAs could survive in the simulated digestive system for at least 75 min (Philip et al., 2015). Additionally, miRNAs detected in human plasma were found to resist to ribonuclease A, suggesting that miRNA molecules may be accompanied by certain proteins, lipids, or other particles that protect them from degradation (Wang et al., 2012). To further explore the properties of small RNA through the placenta, Li et al. found that the level of small interfering RNA (siRNA) in mouse embryos increased significantly after feeding siRNA, and the level of alpha-fetoprotein (AFP) targeted by siRNA was downregulated (Wong et al., 2015). In addition, the factors like ultrasonic treatment, extreme heat, especially RNase treatment that could cause miRNA degradation, while being associated with plant molecules such as proteins and exosomes might be an effective way to protect miRNAs from degradation (Xie and Melzig, 2018). These studies confirmed the stability of plant-derived miRNAs during herb preparations, suggesting the possibility of medicinal plant miRNAs in mammals with complete function. The therapeutic potential for plant miRNAs has been confirmed by two different laboratories. A study reported that a mixture of three tumor suppressor miRNAs (simulated plant miRNAs) reduced colon tumor burden by oral administration in a mouse model of colon cancer (Mlotshwa et al., 2015). Another study found that plant miR159 was present in female serum, and its level was inversely correlated with breast cancer incidence and progression (Chin et al., 2016). Most of the identified miR159 are abundant in extracellular vesicles (EV), and in vitro experiments have shown that synthetic miR159 could reduce the proliferation of breast cancer cells (Chin et al., 2016). Furthermore, oral administration of the miR159 mimics significantly inhibited the growth of venous angiographic breast tumors in mice (Chin et al., 2016). Currently, many studies have provided evidence that exogenous miRNAs can play an important biological role in animals through oral administration.
In this study, using high-throughput sequencing, we comprehensively identified 5718 known miRNAs and 38 novel miRNAs from G. elata for the first time. The relative levels of these miRNAs in various stages of G. elata were further verified by qRT-PCR. The bioinformatics prediction showed these miRNAs could target the Homo sapiens genes, the dual-luciferase reporter gene assay and Western blotting indicated that both Gas-miR01 and Gas-miR02 could interact with the A20 gene in 293T cell. As a zinc finger protein in the NF-κB signaling pathway, A20 is involved in cytokine-mediated immune and inflammatory responses, which has been proved to play a complex role impacting tumor development and therapeutic response according to some reports (Ma and Malynn, 2012; Santolla et al., 2018). Then, animal experiments also confirmed that Gas-miR01 and Gas-miR02 could be detected in some tissues of mice by intragastric administration with fresh G. elata total RNAs, Gastrodia decoction, and Gastrodia powder. Moreover, the A20 expression in some tissues of mice was downregulated in some tissues of mice. Our research provides a theoretical basis for the functional study of G. elata.
Materials and Methods
Sample Collection
The life cycle of G. elata includes G. elata seeds, protocorms, white G. elata, arrow G. elata, and bolted G. elata, which is shown as Figure 1. The sample of fresh arrow G. elata, numbered as CL20151008, which was authenticated by Professor Zongsuo Liang in Zhejiang Provincial Key Laboratory of Plant Secondary Metabolism and Regulation, was collected at coordinate 115°93′12.5″E 31°27′50.0″N in Dabie Mountain, Anhui Province in China and stored at -80°C until total RNA was extracted. The voucher specimen is deposited at Herbarium of Northwest A&F University (WUK). After collecting the sample, G. elata seeds from fresh arrow G. elata were cultured. Protocorms, white G. elata and bolted G. elata were collected separately according to their growing stages.
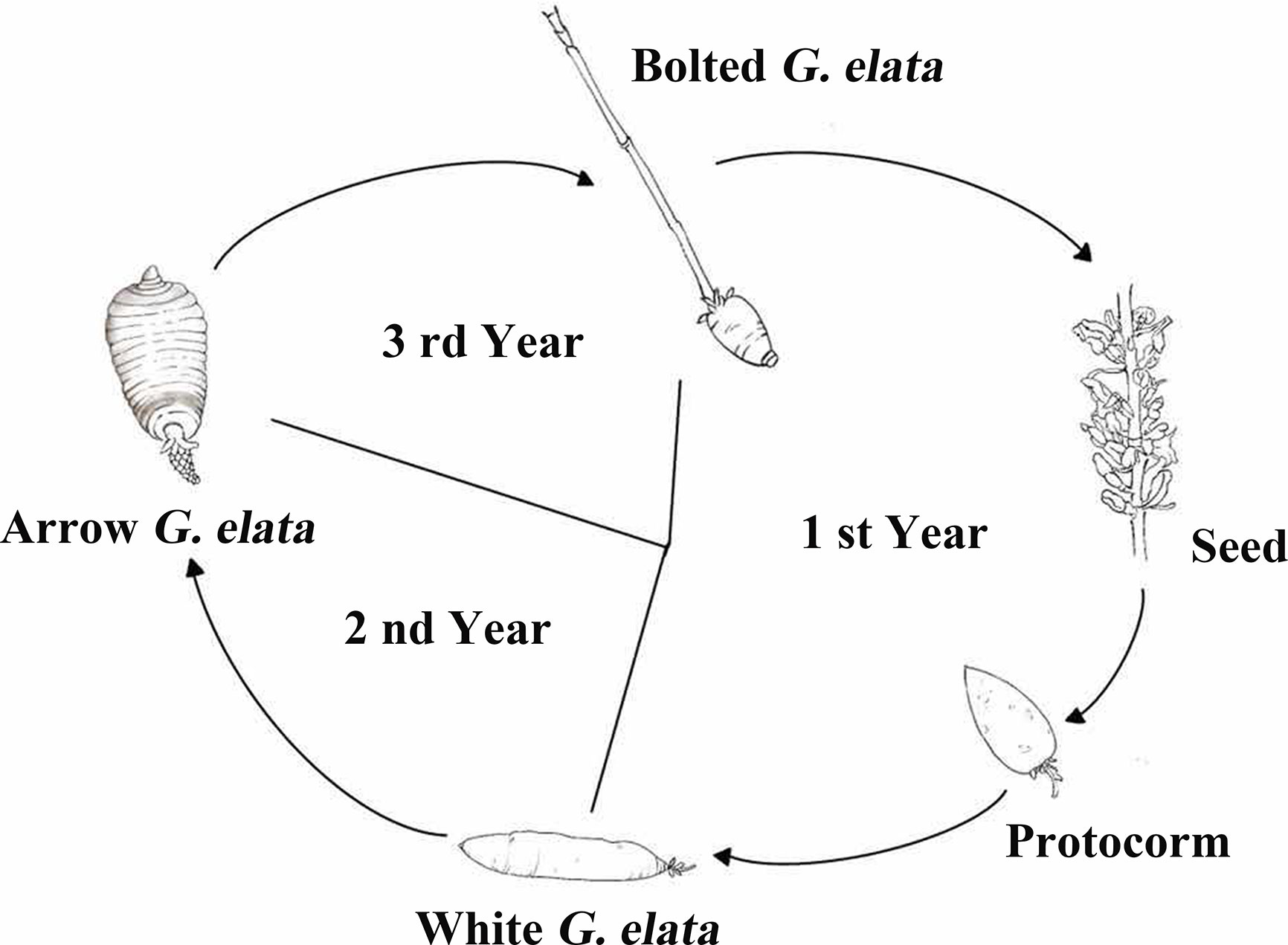
Figure 1 The life cycle of G. elata (trace diagram). The life cycle of G. elata has five stages, including G. elata seeds, protocorms, white G. elata, arrow G. elata, and bolted G. elata.
In animal experiment, total RNAs of fresh G. elata, Gastrodia decoction and Gastrodia powder were used. Gastrodia superfine powder purchased from Yunnan Panlong Yunhai Ltd. Total RNA was extracted from fresh arrow G. elata. Gastrodia decoction was made by putting 150 mg of fresh arrow G. elata in 90 mL ddH2O, with cutting into pieces, soaking at room temperature for 30 min, simmering over low heat for 20 min to simulate traditional Chinese herbal decoction, finally getting 30 mL of Gastrodia decoction. Gastrodia powder was made as the follows: put 30 mg (the moisture content of arrow G. elata is 80%) of Gastrodia superfine powder in 30 mL ddH2O, and then blend to get a mixture called Gastrodia powder.
RNA Extraction and qRT-PCR
Total RNAs extracted from different developmental stages from G. elata were reverse transcribed into the cDNAs using the Transcriptor First-Strand cDNA Synthesis Kit (Roche). The following program was used for the qRT-PCR using Fast Start Universal SYBR Green Master (Rox) (Roche): 95°C for 10 min, followed by 40 cycles of 95°C for 15 s and 60°C for 1 min, 95°C for 15 s, 60°C for 1 min, and 95°C for 15 s. Likewise, stem-loop qRT-PCR was used to detect miRNA (Specific RT-primer) using 5.8S rRNA as a normal control (Li et al., 2016). Likewise, total RNAs of mouse tissues in animal experiments were extracted. Then, the expression of Gas-miR01, Gas-miR02, and A20 were also detected by qRT-PCR using Fast Start Universal SYBR Green Master (Rox) (Roche). Primers for the quantitative real-time PCR and reverse transcription (Supplementary materials Table 1) were synthesized by Sangon Biotech (Shanghai). The data analysis was performed using ABI Prism 7500 SDS Software (Applied Biosystems, USA).
Bioinformatics Analysis
We used the two-phase model (TPM) of Gastrodia to normalize the expression level. Normalized expression = (read count × 1,000,000)/libsize, where libsize refers to the number of reads on a miRNA compared to a sample (Zhou et al., 2010). We use DEGSeq for differential analysis (Wang et al., 2010) and count its Q-value (or adjust p-value) (Pounds and Cheng, 2004). The predicted target genes were subjected aligned against to GO and KEGG database, and the specific processes and pathways were emphatically analyzed (Nie et al., 2018). The GO enrichment analysis method refers to GOseq, and we counted the number of genes belonging to a certain GO number in the whole genome background and differentially expressed genes. Then, according to Fisher’s test, chi-squared test, and binominal test, the enrichment of GO entries in differentially expressed genes was determined relative to the background. RNAhybrid and miRanda softwares were used to predict differential miRNA target genes.
Transfection With miRNA Mimics
Gas-miR01, Gas-miR02, and negative control were synthetized by Gene Pharma (Shanghai, China). 293T cells were cultured in six-well plates overnight in 37°C at 5% CO2, and Gas-miR01 and Gas-miR02 were overexpressed in 293T cells by transfection of the mimics for 20 pmol/1 × 105 cells. The NC mimics was used as the negative control. Cells were collected 48 h post transfection for real-time qPCR analysis.
Dual Luciferase Reporter Assay
The 293 T cells were cultured in a 24-well plate. And after 24 hours, the cells were transfected with pGL3-basic-A20 vector and co-transfected with Gas-miR01/Gas-miR02 mimics or negative control by Lipofectamine 3000TM transfection reagent (Thermo Scientific, MA, USA). The luciferase vectors were constructed by General Biol (Anhui, China). The sequence of Gas-miR01 and Gas-miR02 were as follows: Gas-miR01: 5’-GUUCAGGAAUGCUGUGGGAAG-3’; Gas-miR02: 5’-UUCAAUAAAGCUGUGGGAAA-3’. After 48 hours of transfection, the luciferase activity was detected by Dual-Luciferase Reporter Assay System (Promega, USA, E1910) according to the manufacturer’s protocol.
Western Blotting
The 293T cells transfected with Gas-miR01/Gas-miR02/NC mimics were collected, and then the total proteins were extracted using the Cell Lysis Reagent (Roche). Total proteins were separated by 12% SDS-PAGE and transferred onto a polyvinylidene difluoride (PVDF) membrane (Millipore, USA) by electroblotting. After blocking with 5% skim milk in tris-buffered saline with Tween (TBST) (pH 7.4) for 2 h at room temperature, the protein was detected with an anti-A20 antibody (Cell Signaling Technology, USA) overnight at 4°C. The membrane was washed with TBST, and HRP-labeled (horseradish peroxidase-labeled) mouse anti-rabbit antibody was used as the secondary antibody (Cell Signaling Technology, USA). The signals were detected using the ECL Detection Kit (Advansta).
Animal Experiments
Twenty-four female ICR mice were purchased from a pathogen-free animal facility at Zhejiang Chinese Medical University. The Institutional Review Board of Zhejiang Chinese Medical University approved all housing and surgical procedures. Animals were housed in cages (three to five mice per cage) and given free access to rodent chow and water ad libitum in a room maintained at 24°C and a 12-h/12-h light-dark cycle. At 7 weeks of age, each ICR mouse was fed with fresh G. elata total RNAs (30 μg/kg/2 h), Gastrodia decoction (3 mg/kg/2 h), Gastrodia powder (3 mg/kg/2 h), or double distilled H2O by gavage after fasting overnight. The mice were treated with total RNAs of fresh G. elata (30 μg/kg), Gastrodia decoction (3 mg/kg) and Gastrodia powder (3 mg/kg) by feeding a time per 2 h with six times during 12 h. After the sixth gavage for 0.5 h, tissues were collected, and total RNAs were extracted. The flow chart of animal experiments is shown in Figure 6A. All procedures used in this study were approved by the Zhejiang Sci-Tech University Animal Experimental Ethics Committee.
Statistical Analysis
All data are the average of three separate experiments presented as the mean ± SEM. Data were analyzed by one-way ANOVA and LSD test with SPSS statistical analysis software.
Results
Length Distribution of G. elata Small RNA and Expression Profiles of miRNAs
In the clean total reads, the length distribution show higher peaks at 24 nt and 35 nt; in the clean unique reads, the highest peak of the length distribution is at 24 nt (Figures 2A, B). The length of miRNA and siRNA is approximately 24 nt, suggesting that the majority of G. elata small RNAs (sRNAs) were miRNAs or siRNAs, which may play an important role in regulating gene expression. Then, the expression of miRNAs from different G. elata tissues was explored. We firstly drew a hierarchical clustering map of 38 miRNAs with high expression and good reproducibility. The results are shown in Figure 2C. The arrow G. elata bud and bloted G. elata tuber showed a relative higher expression level of miRNAs. Eight known miRNAs (Gas-miR159, Gas-miR6478, Gas-miR148a-3p, Gas-miR99, Gas-miR143-3p, Gas-miR319f, Gas-miR396e, and Gas-miR26a) and two new miRNAs (Gas-miR01 and Gas-miR02) were selected to detect for expression levels (Supplementary materials Table 2). In traditional medicinal tissue of G. elata (arrow G. elata tuber), Gas-miR6478, Gas-miR148a-3p, Gas-miR99, Gas-miR143-3p, and Gas-miR26a had higher expression. Furthermore, the expression levels of 10 G. elata miRNAs were verified by qRT-PCR, and the qRT-PCR results were generally consistent with the sequencing results. It is worth mentioning that the two G. elata-unique miRNAs (Gas-miR01 and Gas-miR02) had relatively high expression levels in G. elata tissues (Figure 2D).
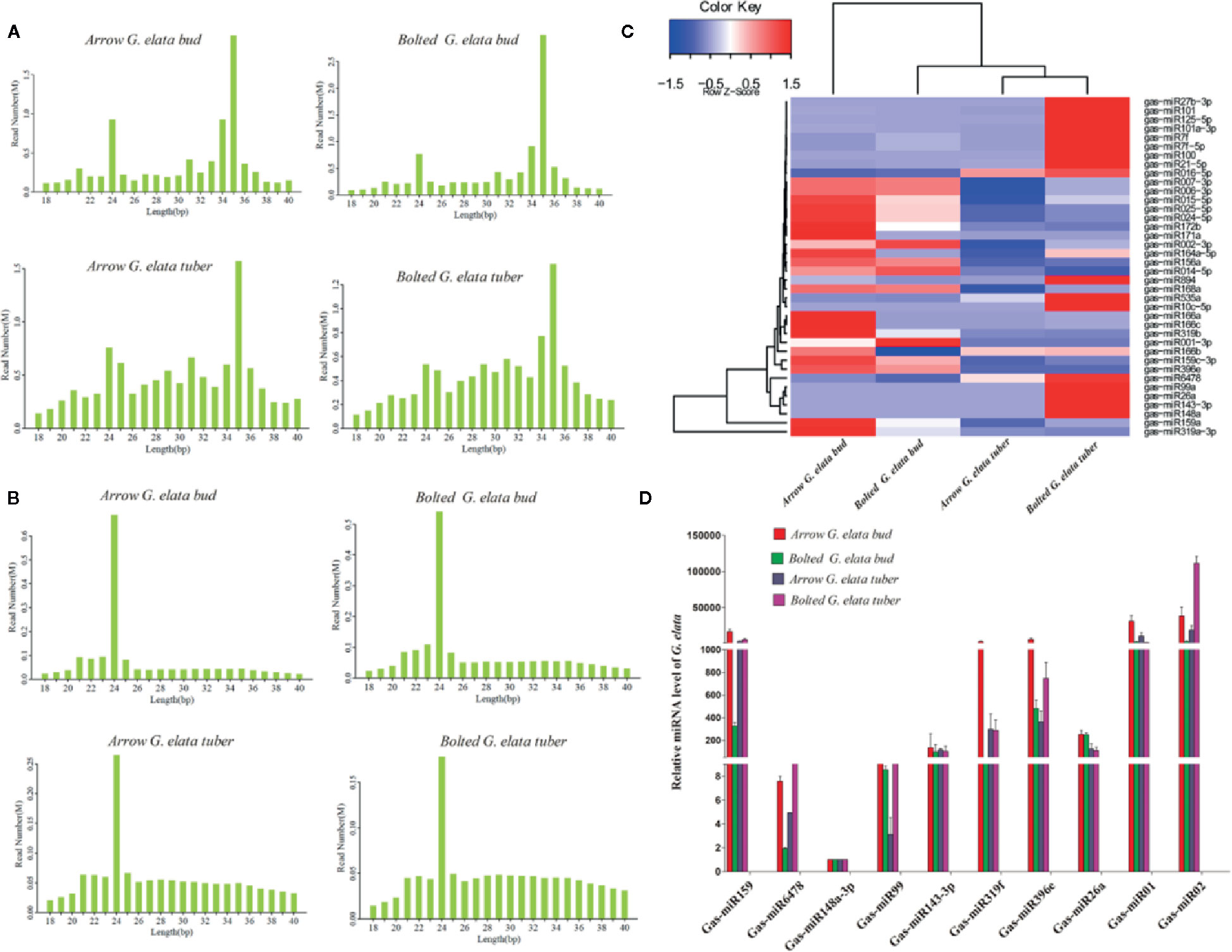
Figure 2 Length distribution of G. elata sRNA and expression profiles of 10 G. elata miRNAs in different tissues. Length distributions of clean total reads (A) and clean unique reads (B). (C) Clustering map of difference miRNAs. (D) Relative expression levels of 10 G. elata miRNAs in different tissues.
The relative G. elata miRNA expression levels in different growth stages were also determined by qRT-PCR. The results showed that all the detected 10 miRNAs showed a different expression levels in different growth stages (Figure 3). On the whole, the Gas-miR01, Gas-miR319f and Gas-miR396e had relative high expression levels in certain growth stages. The G. elata-unique Gas-miR01 has lower expression levels in protocorms and white G. elata but higher expression levels in G. elata seeds and arrow G. elata. Compared with G. elata seeds, white G. elata and arrow G. elata, Gas-miR02 had the highest expression level in protocorms (Figure 3).
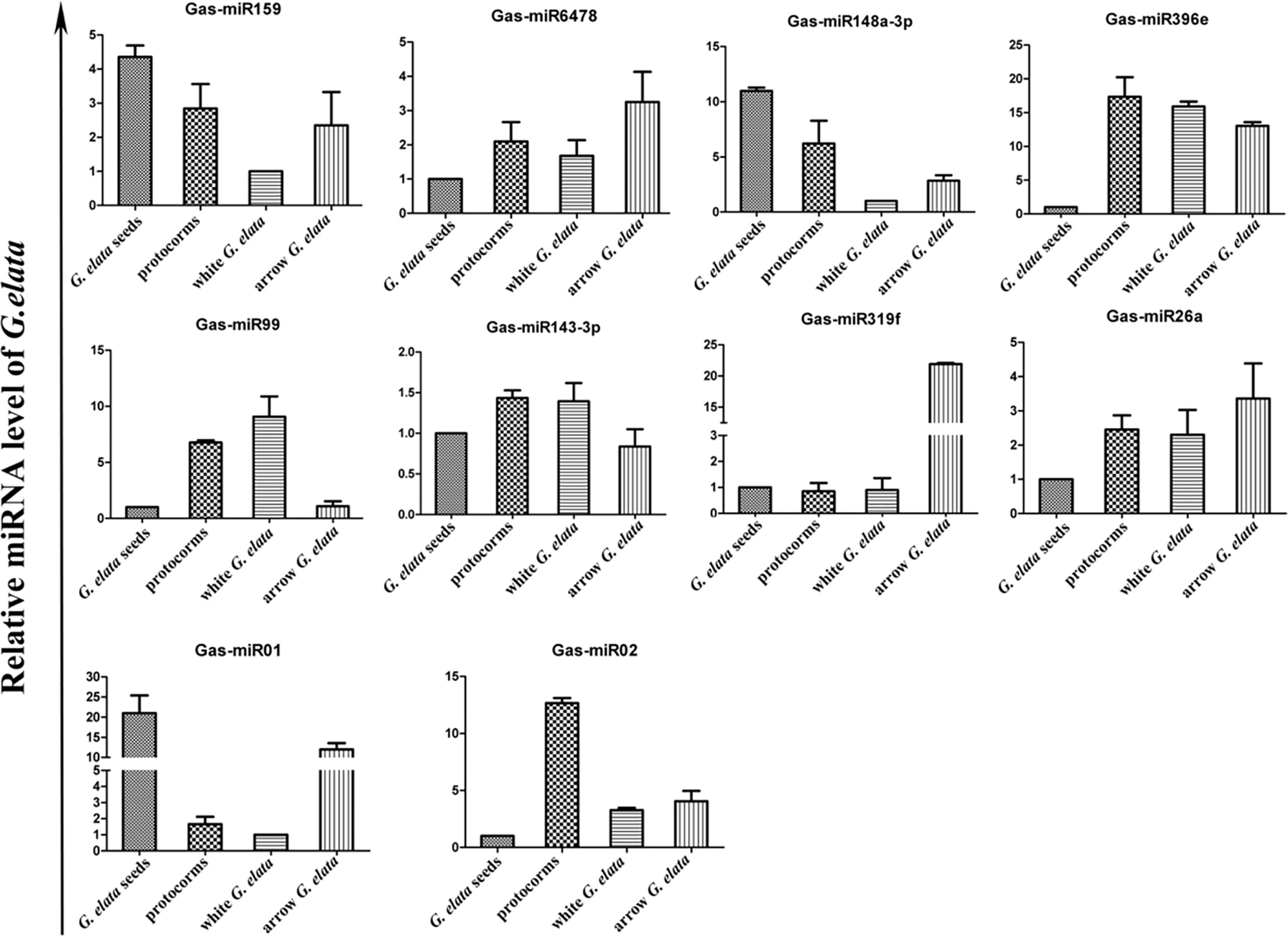
Figure 3 Expression profiles of 10 G. elata miRNAs in different growth stages. The relative G. elata miRNA expression levels in different growth stages were determined by qRT-PCR. The vertical coordinate means fold change.
GO and KEGG Enrichment Analysis of Differential miRNA Target Genes
To explore the potential functions of some specific miRNAs in G. elata, the potential Homo sapiens target genes of G. elata miRNAs were obtained by prediction using RNAhybrid and miRanda softwares. The candidate target genes were further analyzed by Gene Ontology analysis. In the medicinal tissue of G. elata (arrow G. elata tuber), the enrichment GO annotation of miRNA target genes involves biological regulation, immune system regulation, intercellular communication, and nucleic acid binding in three aspects: biological processes, cellular components, and molecular functions (Figure 4A). The KEGG pathway enrichment analysis indicated that the target genes annotation of the G. elata miRNAs is mainly enriched in the cell cycle, intestinal immune network, tumor transcriptional regulation, and olfactory signal transduction pathway (Figure 4B).
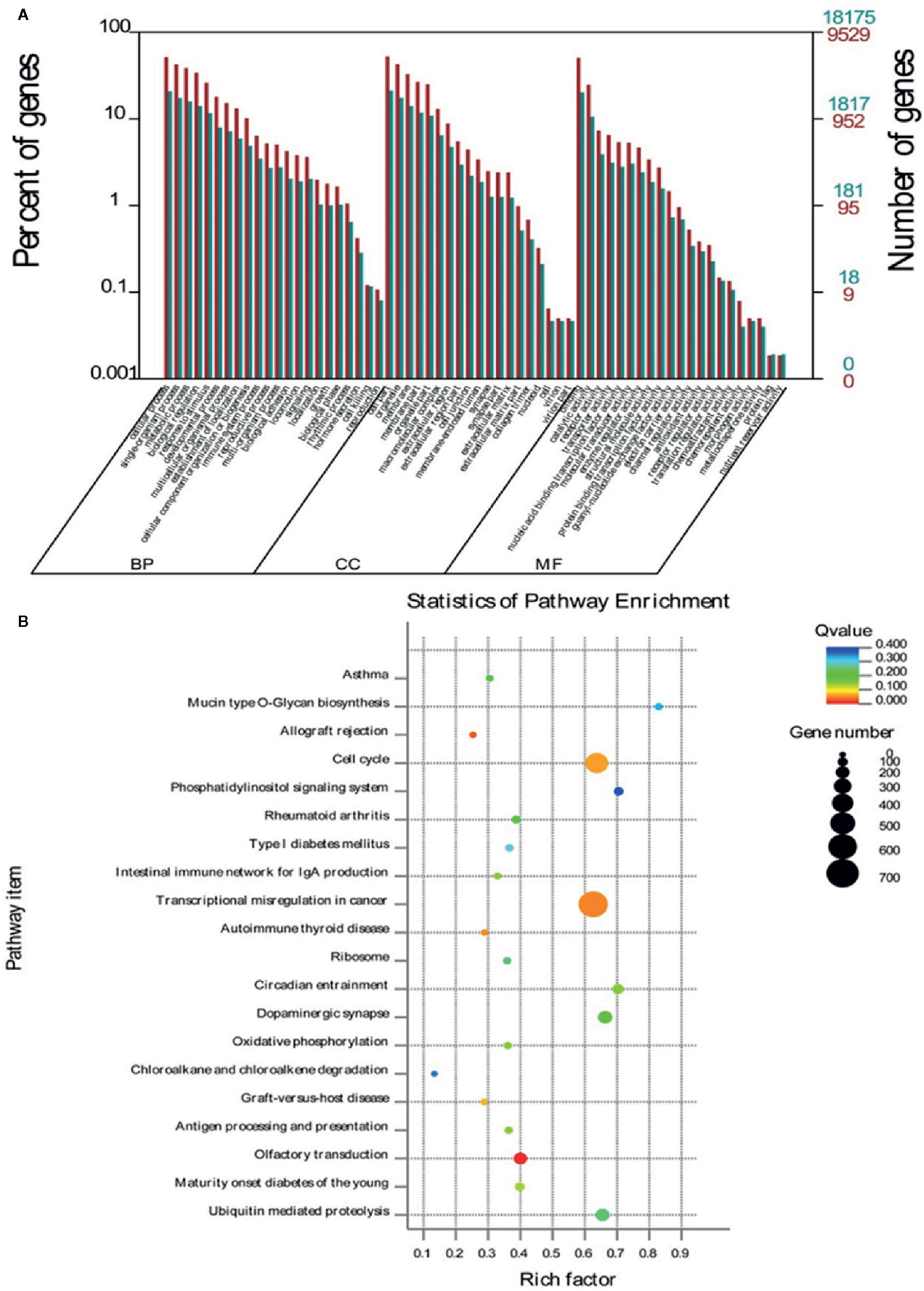
Figure 4 GO and KEGG enrichment analysis of differential miRNA target genes. (A) The target genes of miRNA involve BP (biological processes), CC (cellular components), and MF (molecular functions). (B) The closer the Q-value is to zero, the more significant the particular GO term associated with the group of genes; the closer the Q-value is to one, the opposite. The closer the Q-value is to 0, the more significant the particular GO term associated with the group of genes; the closer the Q-value is to one, the opposite.
The G. elata-Unique Gas-miR01 and Gas-miR02 Targeted the Homo sapiens A20 Gene
The bioinformatics analysis found that the seed sequences of the 5’ end of G. elata-unique miRNA Gas-miR01 and Gas-miR02 could perfectly match the targeted sites in the 3’ UTR region of the Homo sapiens A20 gene (Figure 5A). Subsequently, the relative luciferase activity of pGL3-basic-A20 vector was significantly reduced when transfected with Gas-miR01 mimic or Gas-miR02 mimic, and this indicated that Gas-miR01 and Gas-miR02 could suppress the translation of A20 by interacting with the A20 gene (Figures 5B, C). The Gas-miR01 and Gas-miR02 mimics were then transfected into 293T cells successfully (Figure 5D), and the down-regulated expression level of A20 protein was detected by Western blotting (Figure 5E). The result showed that both Gas-miR01 and Gas-miR02 could significantly downregulate the expression of the protein A20 in vitro.
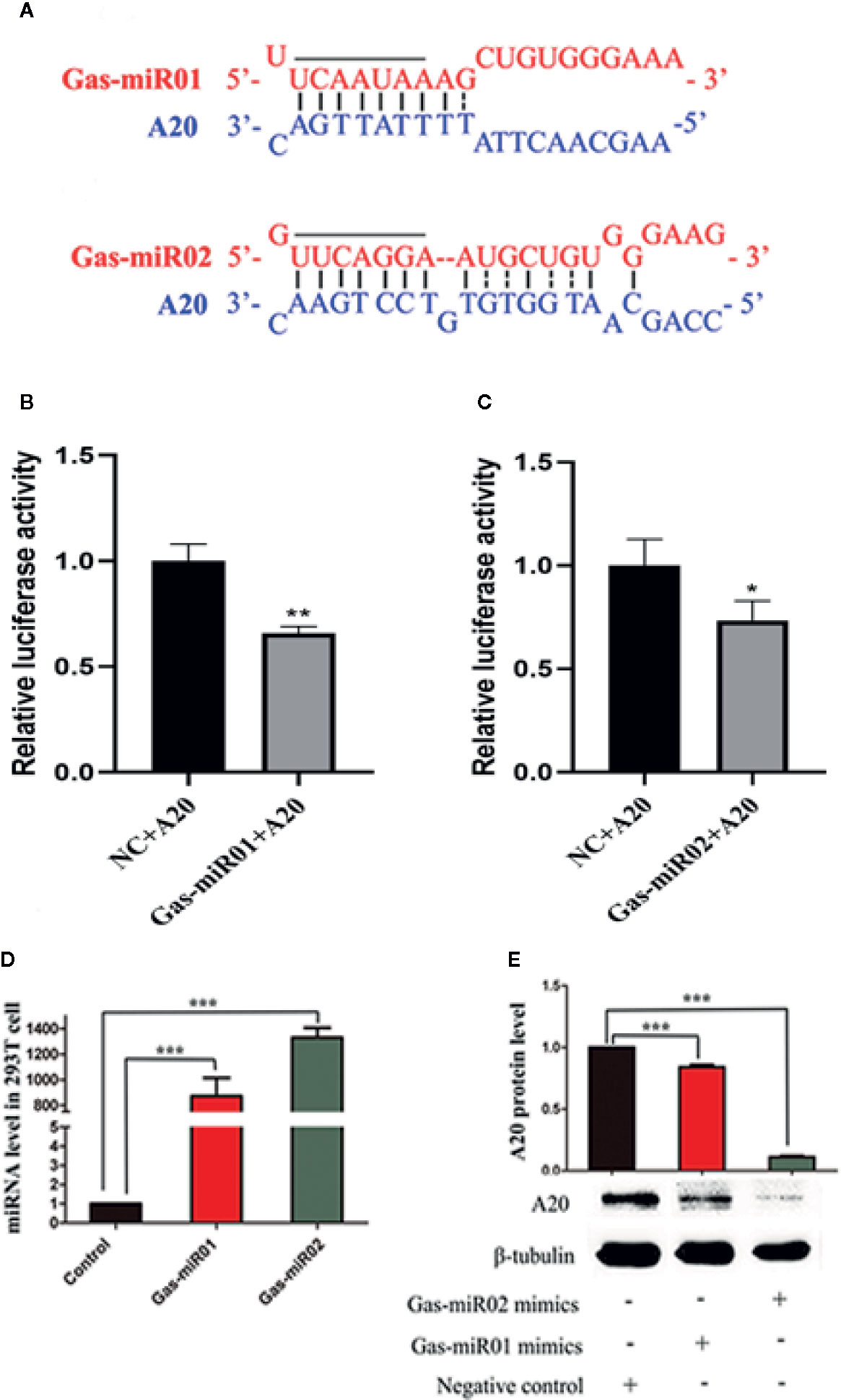
Figure 5 The down-regulation of A20 by Gas-miR01 and Gas-miR02. (A) Matching between Gas-miR01, Gas-miR02 and their target sites in A20 gene. (B) The relative luciferase activity down-regulated by Gas-miR01. (C) The relative luciferase activity down-regulated by Gas-miR02. (D) Levels of Gas-miR01 and Gas-miR02 in 293T cells after transfected with corresponding mimics. (E) The expression level of A20 protein after transfected with Gas-miR01 and Gas-miR02 mimics. *p < 0.05; **p < 0.01; ***p < 0.001. Values are the mean ± SEM.
Gas-miR01 and Gas-miR02 Were Detected in Some Tissues of Mice by Intragastric Administration
The total RNAs were extracted from fresh G. elata tuber, Gastrodia decoction, and Gastrodia powder, respectively, and both Gas-miR01 and Gas-miR02 were all detected in them by using qPCR (Figures 6B, C). To further determine the cross-kingdom regulation of G. elata miRNAs, the fresh G. elata total RNAs, Gastrodia decoction Gastrodia powder, and double distilled H2O were gavaged. The levels of Gas-miR01 and Gas-miR02 in various tissues of mice were detected by qRT-PCR (Figures 6D–I). Compared with the control, the levels of Gas-miR01 and Gas-miR02 in the brain, cerebellum, hypothalamus, heart, kidney, and spleen of mice were significantly increased after total RNAs were gavaged, especially in the kidneys. After the Gastrodia powder and Gastrodia decoction were gavaged, the levels of Gas-miR01 and Gas-miR02 in the brain, cerebellum, and spleen tissues of mice also increased significantly. These results indicated that Gas-miR01 and Gas-miR02 could be into the mouse internal environment through the gastrointestinal tract and could be detected in some tissues of mice. Furthermore, qRT-PCR showed the A20 expression was also downregulated in some tissues of mice (Figures 7A–F).
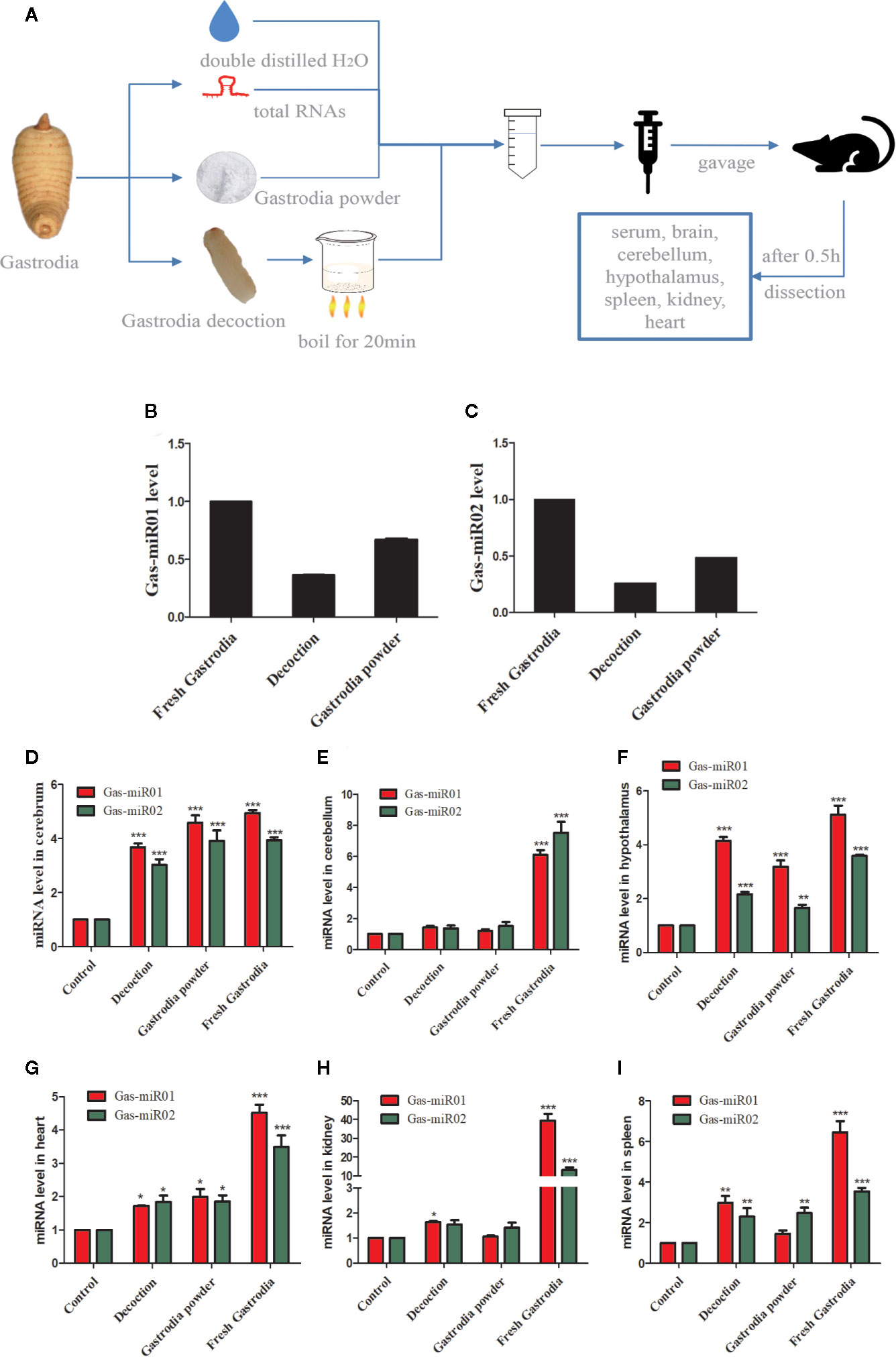
Figure 6 Gas-miR01 and Gas-miR02 were detected in some tissues of mice by intragastric administration. (A) Flow chart of animal experiments. The levels of Gas-miR01 (B) and Gas-miR02 (C) in fresh G. elata tuber, Gastrodia decoction and Gastrodia powder. The fresh G. elata total RNAs, Gastrodia decoction and Gastrodia powder were gavaged and the levels of Gas-miR01 and Gas-miR02 in various tissues of mice were detected by qRT-PCR. The levels of Gas-miR01 and Gas-miR02 in mouse tissues. (D) cerebrum; (E) cerebellum; (F) hypothalamus; (G) heart; (H) kidney; (I) spleen. *p < 0.05; **p < 0.01; ***p < 0.001 compared with the control group, n = 6. Values are the mean ± SEM.
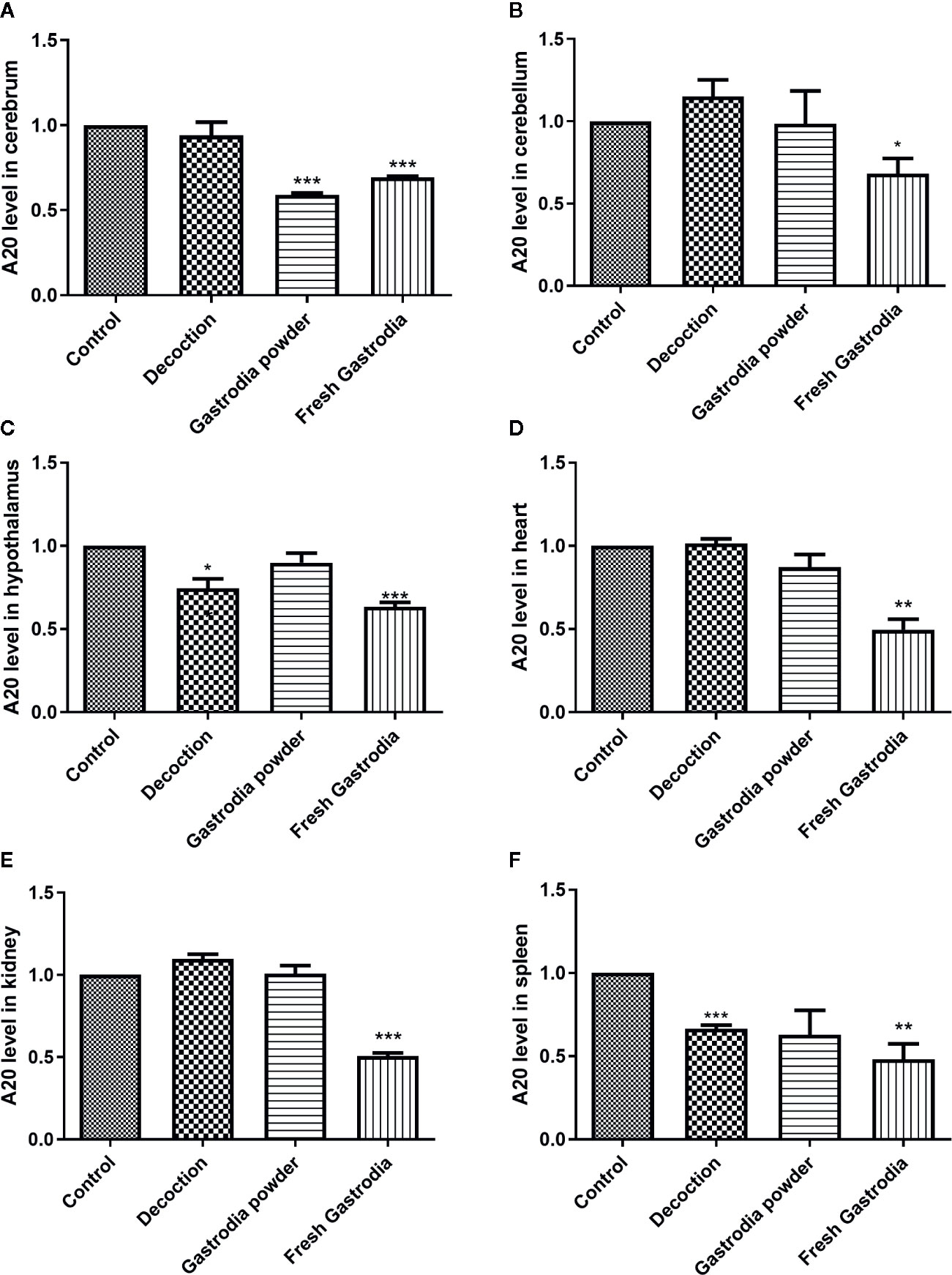
Figure 7 The A20 expression was downregulated in many tissues of mouse. The result of qRT-PCR showed the mRNA level of A20 in various tissues of mice. (A) Cerebrum. (B) Cerebellum. (C) Hypothalamus. (D) Heart. (E) Kidney. (F) Spleen. *p < 0.05; **p < 0.01; ***p < 0.001 compared with the control group, n = 6. Values are the mean ± SEM.
Discussion
miRNA, a small endogenous RNA, plays an important role by targeting specific mRNAs in plants, animals and humans (Sala-Cirtog et al., 2015). Studies have shown that many plant miRNAs are indispensable in cell proliferation and the secondary process of plant secondary metabolism. miRNA detection analysis displayed that Gas-miR319f had slightly different expression levels in G. elata seeds, protocorms, and white G. elata, but it would rise sharply 20 times to the original in arrow G. elata, which is the main medicinal tissue of G. elata. Therefore, we inferred that the sharp increase in the level of Gas-miR319f during this stage might be related to the large amount of synthetic medicinal components, such as gastrodin.
In China, the traditional medicinal herbs have been used for thousands of years and are usually decocted and orally administrated. A growing body of research suggests that the molecular mechanisms of medicinal herbs have been revealed. Since the discovery of plant miRNAs in human tissues and serum after ingestion, the relationship between the two kingdoms has been presented in a new perspective (Han and Luan, 2015). For example, miRNA therapy might be the next best step in determining new treatment options for medicinal plants (Thakur et al., 2014; Bai et al., 2018). Plant miRNAs could be detected in the sera and tissues of various animals, and these exogenous miRNAs were primarily acquired orally through food intake (Zhang et al., 2012). In addition to the findings on transfer of miRNAs and their therapeutic applications, similar studies could provide evidences for further research that might influence modern definitions of herbs. A recent study showed that synthetic MIR2911, extracted RNA from honeysuckle decoction, and honeysuckle decoction all significantly inhibited H1N1 viral replication and rescued viral infection-induced mouse weight loss. The results indicated that MIR2911 is an active component identified in traditional Chinese medicine to directly target various IAVs and may represent a novel type of natural product that effectively suppresses viral infection (Zhou et al., 2015).
In our research, the results suggested that the human genes related to immune system regulation could be regulated by G. elata miRNAs, and in vitro experiment evidenced that both Gas-miR01 and Gas-miR02 mimics could downregulate the expression of the Homo sapiens A20, the key protein in the NF-κB signaling pathway. NF-κB is present in many eukaryotic cells, widely involved in many physiological processes in humans, and related to many diseases such as Parkinson’s disease, Alzheimer’s disease and fatty liver disease (Lee and Ambros, 2001; Gommans and Berezikov, 2012; Ge et al., 2016; Bazazzadegan et al., 2017). And according to some reports, A20 expression is increased in a number of solid human tumors and cancers, which likely contributes to both carcinogenesis and response to chemotherapy. These evidences uncovered the complexities of the mechanisms involved in A20’s impact on tumor development and response to treatment (Hjelmeland et al., 2010; Bellail et al., 2012; Dong et al., 2012; Qiao et al., 2015). Animal experiments showed that the G. elata-unique miRNAs as Gas-miR01 and Gas-miR02 could be detected in both Gastrodia decoction and Gastrodia powder, suggesting these two G. elata-unique miRNAs could still remain stable after decoction and long-time storage. Furthermore, Gas-miR01 and Gas-miR02 could be detected in some tissues of mice by intragastric administration of the total RNAs of fresh G. elata tuber, Gastrodia decoction, and Gastrodia powder; meanwhile, the A20 expression in some tissues of mice was also downregulated. These evidences suggest that G. elata miRNAs could be into the mouse internal environment and conductcross-kingdom regulation. The above results provided a new theoretical basis for the further study on the cross-kingdom regulation of G. elata miRNAs. Our findings may provide data support for the functional study of miRNAs and the medicinal ingredients in G. elata in the future.
Conclusion
In this study, we firstly obtained 5,718 known miRNAs and 38 G. elata–specific miRNAs by high-throughput sequencing. The expression levels of the miRNAs in various stages and tissues of G. elata were different by qRT-PCR, which was found to be high expression in the medicinal tissue of G. elata (arrow G. elata tuber). Bioinfomatics analysis indicated that G. elata miRNAs might be involved in the cross-kingdom regulation of Homo sapiens genes. It was confirmed for the first time that the two G. elata-unique miRNAs Gas-miR01 and Gas-miR02 could downregulate the expression of the Homo sapiens A20 gene in vitro. Furthermore, G. elata-unique miRNAs Gas-miR01 and Gas-miR02 could be detected in some tissues of mice through the gastrointestinal tract, and A20 expression was downregulated. These findings lay a foundation for the study of the cross-kingdom regulation of G. elata miRNAs.
Data Availability Statement
All datasets generated for this study are included in the article/supplementary material.
Ethics Statement
The animal study was reviewed and approved by Zhejiang Sci-Tech University Animal Experimental Ethics Committee.
Author Contributions
QS designed the project and instructed all the process. CX and HZ designed and performed the main experiments and wrote the article under the guidance of QS. XX and TJ undertook part of the experiments and analyzed the data. SL participated in the research and helped to write the article. DW and ZN helped to design the project and revise articles.
Funding
This work was supported by grants from Project of Public Welfare Technology Research in Zhejiang Province (grant numbers LGF18H250004 and LGF19H250002).
Conflict of Interest
The authors declare that the research was conducted in the absence of any commercial or financial relationships that could be construed as a potential conflict of interest
Acknowledgments
We thank Wenbing Ou for critical comments on the article.
Supplementary Material
The Supplementary Material for this article can be found online at: https://www.frontiersin.org/articles/10.3389/fphar.2020.542405/full#supplementary-material
References
Bai, Q., Wang, X., Chen, X., Shi, G., Liu, Z., Guo, C., et al. (2018). Wheat miRNA TaemiR408 Acts as an Essential Mediator in Plant Tolerance to Pi Deprivation and Salt Stress via Modulating Stress-Associated Physiological Processes. Front. Plant Sci. 9, 499. doi: 10.3389/fpls.2018.00499
Bazazzadegan, N., Dehghan Shasaltaneh, M., Saliminejad, K., Kamali, K., Banan, M., Khorram Khorshid, H. R. (2017). The Effects of Melilotus officinalis Extract on Expression of Daxx, Nfkb and Vegf Genes in the Streptozotocin-Induced Rat Model of Sporadic Alzheimer’s Disease. Avicenna J. Med. Biotechnol. 9 (3), 133–137.
Bellail, A. C., Olson, J. J., Yang, X., Chen, Z. J., Hao, C. (2012). A20 ubiquitin ligase-mediated polyubiquitination of RIP1 inhibits caspase-8 cleavage and TRAIL-induced apoptosis in glioblastoma. Cancer Discovery 2 (2), 140–155. doi: 10.1158/2159-8290.CD-11-0172
Chin, A. R., Fong, M. Y., Somlo, G., Wu, J., Swiderski, P., Wu, X., et al. (2016). Cross-kingdom inhibition of breast cancer growth by plant miR159. Cell Res. 26 (2), 217–228. doi: 10.1038/cr.2016.13
Dong, B., Lv, G., Wang, Q., Wei, F., Bellail, A. C., Hao, C., et al. (2012). Targeting A20 enhances TRAIL-induced apoptosis in hepatocellular carcinoma cells. Biochem. Biophys. Res. Commun. 418 (2), 433–438. doi: 10.1016/j.bbrc.2012.01.056
Du, F., Wang, X., Shang, B., Fang, J., Xi, Y., Li, A., et al. (2016). Gastrodin ameliorates spinal cord injury via antioxidant and anti-inflammatory effects. Acta Biochim. Pol. 63 (3), 589–593. doi: 10.18388/abp.2016_1272
Duan, X. H., Li, Z. L., Yang, D. S., Zhang, F. L., Lin, Q., Dai, R. (2013). [Study on the chemical constituents of Gastrodia elata]. Zhong Yao Cai 36 (10), 1608–1611.
Ge, J. F., Walewski, J. L., Anglade, D., Berk, P. D. (2016). Regulation of Hepatocellular Fatty Acid Uptake in Mouse Models of Fatty Liver Disease with and without Functional Leptin Signaling: Roles of NfKB and SREBP-1C and the Effects of Spexin. Semin. Liver Dis. 36 (4), 360–372. doi: 10.1055/s-0036-1597248
Gommans, W. M., Berezikov, E. (2012). Controlling miRNA regulation in disease. Methods Mol. Biol. 822, 1–18. doi: 10.1007/978-1-61779-427-8_1
Han, L., Luan, Y.-S. (2015). Horizontal Transfer of Small RNAs to and from Plants. Front. Plant Sci. 6, 1113. doi: 10.3389/fpls.2015.01113
Hjelmeland, A. B., Wu, Q., Wickman, S., Eyler, C., Heddleston, J., Shi, Q., et al. (2010). Targeting A20 decreases glioma stem cell survival and tumor growth. PloS Biol. 8 (2), e1000319. doi: 10.1371/journal.pbio.1000319
Hu, Y., Li, C., Shen, W. (2014). Gastrodin alleviates memory deficits and reduces neuropathology in a mouse model of Alzheimer’s disease. Neuropathology 34 (4), 370–377. doi: 10.1111/neup.12115
Kumar, H., Kim, I. S., More, S. V., Kim, B. W., Bahk, Y. Y., Choi, D. K. (2013). Gastrodin protects apoptotic dopaminergic neurons in a toxin-induced Parkinson’s disease model. Evid. Based Complement Alternat. Med. 2013, 514095. doi: 10.1155/2013/514095
Lee, R. C., Ambros, V. (2001). An extensive class of small RNAs in Caenorhabditis elegans. Science 294 (5543), 862–864. doi: 10.1126/science.1065329
Li, B., Cheng, X., Zhang, T., Liu, L., Nie, Z., Sheng, Q. (2016). The identification of microRNAs in Ganoderma lingzhi sporocarp. Mycoscience 57 (4), 271–278. doi: 10.1016/j.myc.2016.03.004
Li, J. J., Liu, S. J., Liu, X. Y., Ling, E. A. (2018). Herbal compounds with special reference to gastrodin as potential therapeutic agents for microglia mediated neuroinflammation. Curr. Med. Chem. 25 (42), 5958–5974. doi: 10.2174/0929867325666180214123929
Liu, Y., Gao, J., Peng, M., Meng, H., Ma, H., Cai, P., et al. (2018). A Review on Central Nervous System Effects of Gastrodin. Front. Pharmacol. 9, 24. doi: 10.3389/fphar.2018.00024
Ma, A., Malynn, B. A. (2012). A20: linking a complex regulator of ubiquitylation to immunity and human disease. Nat. Rev. Immunol. 12 (11), 774–785. doi: 10.1038/nri3313
Mlotshwa, S., Pruss, G. J., MacArthur, J. L., Endres, M. W., Davis, C., Hofseth, L. J., et al. (2015). A novel chemopreventive strategy based on therapeutic microRNAs produced in plants. Cell Res. 25 (4), 521–524. doi: 10.1038/cr.2015.25
Nam, Y., Chen, C., Gregory, R. I., Chou, J. J., Sliz, P. (2011). Molecular basis for interaction of let-7 microRNAs with Lin28. Cell 147 (5), 1080–1091. doi: 10.1016/j.cell.2011.10.020
Nie, H., Wang, Y., Su, Y., Hua, J. (2018). Exploration of miRNAs and target genes of cytoplasmic male sterility line in cotton during flower bud development. Funct. Integr. Genomics 18 (4), 457–476. doi: 10.1007/s10142-018-0606-z
Niu, Q., Niu, P., He, S. (2004). Effect of gastrodia elata on learning and memory impairment induced by aluminum in rats]. Wei Sheng Yan Jiu 33 (1), 45–48.
Oghbaei, H., Ahmadi Asl, N., Sheikhzadeh, F. (2017). Can regular moderate exercise lead to changes in miRNA-146a and its adapter proteins in the kidney of streptozotocin-induced diabetic male rats? Endocr. Regul. 51 (3), 145–152. doi: 10.1515/enr-2017-0015
Philip, A., Ferro, V. A., Tate, R. J. (2015). Determination of the potential bioavailability of plant microRNAs using a simulated human digestion process. Mol. Nutr. Food Res. 59 (10), 1962–1972. doi: 10.1002/mnfr.201500137
Pounds, S., Cheng, C. (2004). Improving false discovery rate estimation. Bioinformatics 20 (11), 1737–1745. doi: 10.1093/bioinformatics/bth160
Qiao, P., Gao, X. M., Yu, X. X., Zhang, X. F., Dong, Q., Qin, L.-X. (2015). Expression of A20 gene in hepatocellular carcinoma patients and its prognostic significance. Tumor 35, 639–645. doi: 10.3781/j.issn.1000-7431.2015.33.100
Razaviyan, J., Hadavi, R., Tavakoli, R., Kamani, F., Paknejad, M., Mohammadi-Yeganeh, S. (2018). Expression of miRNAs Targeting mTOR and S6K1 Genes of mTOR Signaling Pathway Including miR-96, miR-557, and miR-3182 in Triple-Negative Breast Cancer. Appl. Biochem. Biotechnol. 186 (4), 1074–1089. doi: 10.1007/s12010-018-2773-8
Sala-Cirtog, M., Marian, C., Anghel, A. (2015). New insights of medicinal plant therapeutic activity-The miRNA transfer. BioMed. Pharmacother. 74, 228–232. doi: 10.1016/j.biopha.2015.08.016
Santolla, M. F., Lappano, R., Cirillo, F., Rigiracciolo, D. C., Sebastiani, A., Abonante, S., et al. (2018). miR-221 stimulates breast cancer cells and cancer-associated fibroblasts (CAFs) through selective interference with the A20/c-Rel/CTGF signaling. J. Exp. Clin. Cancer Res. CR 37 (1), 94. doi: 10.1186/s13046-018-0767-6
Song, E., Chung, H., Shim, E., Jeong, J. K., Han, B. K., Choi, H. J., et al. (2016). Gastrodia elata Blume Extract Modulates Antioxidant Activity and Ultraviolet A-Irradiated Skin Aging in Human Dermal Fibroblast Cells. J. Med. Food 19 (11), 1057–1064. doi: 10.1089/jmf.2016.3722
Thakur, V. S., Deb, G., Babcook, M. A., Gupta, S. (2014). Plant phytochemicals as epigenetic modulators: role in cancer chemoprevention. AAPS J. 16 (1), 151–163. doi: 10.1208/s12248-013-9548-5
Wang, L., Feng, Z., Wang, X., Wang, X., Zhang, X. (2010). DEGseq: an R package for identifying differentially expressed genes from RNA-seq data. Bioinformatics 26 (1), 136–138. doi: 10.1093/bioinformatics/btp612
Wang, K., Li, H., Yuan, Y., Etheridge, A., Zhou, Y., Huang, D., et al. (2012). The complex exogenous RNA spectra in human plasma: an interface with human gut biota? PloS One 7 (12), e51009. doi: 10.1371/journal.pone.0051009
Wang, C., Ji, B., Cheng, B., Chen, J., Bai, B. (2014). Neuroprotection of microRNA in neurological disorders (Review). BioMed. Rep. 2 (5), 611–619. doi: 10.3892/br.2014.297
Wang, X. S., Tian, Z., Zhang, N., Han, J., Guo, H. L., Zhao, M. G., et al. (2016). Protective Effects of Gastrodin Against Autophagy-Mediated Astrocyte Death. Phytother. Res. 30 (3), 386–396. doi: 10.1002/ptr.5538
Wong, K. H., Razmovski-Naumovski, V., Li, K. M., Li, G. Q., Chan, K. (2015). Comparing morphological, chemical and anti-diabetic characteristics of Puerariae Lobatae Radix and Puerariae Thomsonii Radix. J. Ethnopharmacol. 164, 53–63. doi: 10.1016/j.jep.2014.12.050
Wu, C. R., Hsieh, M. T., Liao, J. (1996). p-Hydroxybenzyl alcohol attenuates learning deficits in the inhibitory avoidance task: involvement of serotonergic and dopaminergic systems. Chin. J. Physiol. 39 (4), 265–273.
Wu, J., Wu, B., Tang, C., Zhao, J. (2017). Analytical Techniques and Pharmacokinetics of Gastrodia elata Blume and Its Constituents. Molecules 22 (7), 1137. doi: 10.3390/molecules22071137
Xie, W., Melzig, M. F. (2018). The Stability of Medicinal Plant microRNAs in the Herb Preparation Process. Molecules 23 (4), 919. doi: 10.3390/molecules23040919
Yang, J., Farmer, L. M., Agyekum, A. A., Elbaz-Younes, I., Hirschi, K. D. (2015a). Detection of an Abundant Plant-Based Small RNA in Healthy Consumers. PloS One 10 (9), e0137516. doi: 10.1371/journal.pone.0137516
Yang, J., Farmer, L. M., Agyekum, A. A., Hirschi, K. D. (2015b). Detection of dietary plant-based small RNAs in animals. Cell Res. 25 (4), 517–520. doi: 10.1038/cr.2015.26
Yuan, Y., Jin, X., Liu, J., Zhao, X., Zhou, J., Wang, X., et al. (2018). The Gastrodia elata genome provides insights into plant adaptation to heterotrophy. Nat. Commun. 9 (1), 1615. doi: 10.1038/s41467-018-03423-5
Zhan, H. D., Zhou, H. Y., Sui, Y. P., Du, X. L., Wang, W. H., Dai, L., et al. (2016). The rhizome of Gastrodia elata Blume - An ethnopharmacological review. J. Ethnopharmacol. 189, 361–385. doi: 10.1016/j.jep.2016.06.057
Zhang, L., Hou, D., Chen, X., Li, D., Zhu, L., Zhang, Y., et al. (2012). Exogenous plant MIR168a specifically targets mammalian LDLRAP1: evidence of cross-kingdom regulation by microRNA. Cell Res. 22 (1), 107–126. doi: 10.1038/cr.2011.158
Zhou, L., Chen, J., Li, Z., Li, X., Hu, X., Huang, Y., et al. (2010). Integrated profiling of microRNAs and mRNAs: microRNAs located on Xq27.3 associate with clear cell renal cell carcinoma. PloS One 5 (12), e15224. doi: 10.1371/journal.pone.0015224
Zhou, Z., Li, X., Liu, J., Dong, L., Chen, Q., Liu, J., et al. (2015). Honeysuckle-encoded atypical microRNA2911 directly targets influenza A viruses. Cell Res. 25 (1), 39–49. doi: 10.1038/cr.2014.130
Keywords: Gastrodia elata Blume, microRNA, high-throughput sequencing, expression profiles, A20, functional study
Citation: Xia C, Zhou H, Xu X, Jiang T, Li S, Wang D, Nie Z and Sheng Q (2020) Identification and Investigation of miRNAs From Gastrodia elata Blume and Their Potential Function. Front. Pharmacol. 11:542405. doi: 10.3389/fphar.2020.542405
Received: 17 March 2020; Accepted: 28 August 2020;
Published: 25 September 2020.
Edited by:
Jia-bo Wang, Fifth Medical Center of the PLA General Hospital, ChinaReviewed by:
Bin Geng, Chinese Academy of Medical Sciences and Peking Union Medical College, ChinaXiwen Li, China Academy of Chinese Medical Sciences, China
Copyright © 2020 Xia, Zhou, Xu, Jiang, Li, Wang, Nie and Sheng. This is an open-access article distributed under the terms of the Creative Commons Attribution License (CC BY). The use, distribution or reproduction in other forums is permitted, provided the original author(s) and the copyright owner(s) are credited and that the original publication in this journal is cited, in accordance with accepted academic practice. No use, distribution or reproduction is permitted which does not comply with these terms.
*Correspondence: Qing Sheng, Y3NoZW5nQHpzdHUuZWR1LmNu
†These authors share first authorship