- 1School of Basic Medical Sciences, Qingdao University, Qingdao, China
- 2State Key Laboratory of Quality Research in Chinese Medicine, Institute of Chinese Medical Sciences, University of Macau, Macao, China
- 3Department of Biomedical Engineering City University of Hong Kong, Hong Kong SAR, China
- 4Department of Pharmacology, School of Pharmacy, Qingdao University, Qingdao, China
- 5College of Chemistry and Pharmaceutical Sciences, Qingdao Agricultural University, Qingdao, China
- 6Qingdao Central Hospital, The Second Affiliated Hospital of Qingdao University, Qingdao, China
Traditional herb pair Salvia miltiorrhiza Bunge-Radix Puerariae (DG) owns various biological activities including anti-inflammatory and anti-oxidative stress. Oxidative stress is one high-risk factor for osteoporosis, then effect of DG on osteoporosis and underlying mechanisms was explored both in vivo and in vitro. Firstly, the predication from network pharmacology hinted that DG has the potential for ameliorating osteoporosis. Consistent with predication, DG significantly restored bone loss and deficiency of type II collagen, decreased TRAP and Cathepsin K positive areas in femur. Meanwhile it improved important characteristics of microarchitectural deterioration of tissue, reduced the numbers of NFATc1-positive osteoclast in the vertebra as well as decreased the serum osteoclast-specific cytokine RANKL and OPG release in OVX rats exhibiting its protective effect against osteoporosis. In vitro, DG noticeably decreased osteoclastic-special marker protein expressions of RANK, c-Fos and NFATc1. Furthermore, autophagy pathway p62/LC3B, ROS production and NF-κB were all activated by RANKL stimulation and blocked by DG pretreatment. Moreover, autophagy inhibitors, ROS scavenger, Ca2+ chelator and NF-κB inhibitor remarkably suppressed c-Fos and NFATc1 expressions. Taken together, DG may ameliorate osteoporosis by regulating osteoclast differentiation mediated by autophagy and oxidative stress. This study provided a mechanistic basis for DG treating osteoporosis and offered a safe dose for DG in preventing and improving bone diseases.
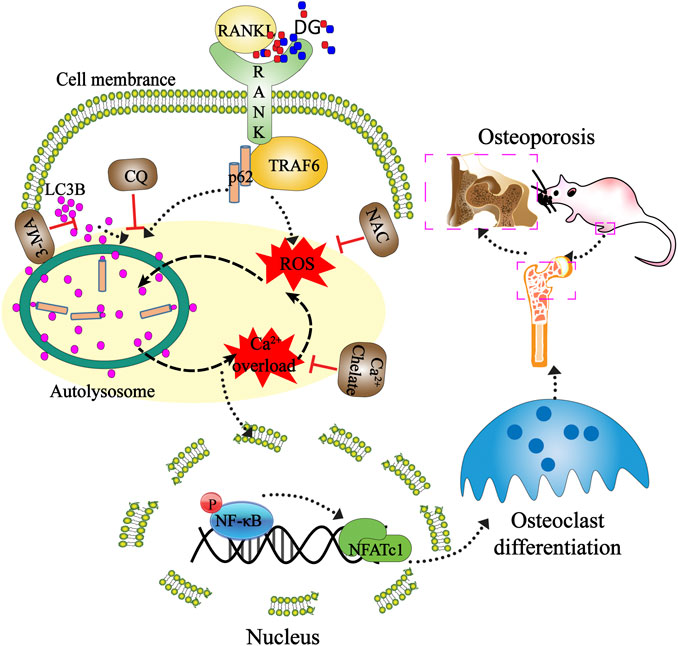
GRAPHICAL ABSTRACT. Mechanisms of DG ameliorating osteoporosis. DG improved osteoporosis by regulating osteoclast differentiation mediated by autophagy and oxidative stress. This study provided a mechanistic basis for the clinical application of DG in osteoporosis treatment.
Introduction
Osteoporosis is a progressive skeletal disorder characterized by increased bone resorption and reduced bone mineral density (Mirza and Canalis, 2015). Nowadays excessive bone resorption due to enhanced osteoclast formation is accepted the most important cellular cause of osteolytic disease (Appelman-Dijkstra and Papapoulos, 2015) and osteoclast is considered as an important target for treating osteoporosis (Cackowski et al., 2010).
Macrophage colony-stimulating factor (M-CSF) and the receptor activator of nuclear factor kappa B ligand (RANKL) are two indispensable hematopoietic factors for osteoclast proliferation and mutation (Roux and Briot, 2018). Among these, RANKL binding to its receptor activates a series of downstream signal pathways including AKT-PLCγ (Ma et al., 2018), MAPKs(Li et al., 2018; Du et al., 2020) and NF-κB (Thummuri et al., 2015; Tu et al., 2020).
Accumulated studies showed that autophagy and oxidative stress are involved in the process of osteoporosis (Schröder, 2015; Shen et al., 2018; Wang et al., 2019). Autophagy is activated in response to several pro-inflammatory cytokines such as IL-1β and TNF-α accompanied by a robust increase in osteoclast differentiation and bone resorption (Wehmeyer et al., 2016; Jiang et al., 2019). Autophagy-related proteins including atg5, atg7, atg4β, and LC3B are identified involved in the formation of ruffled border and facilitation of osteoclast polarization, ultimately results in bone resorption (Hu et al., 2020). Besides, ROS is a critical intracellular signal mediator which induces osteoblast apoptosis through multiple cellular signaling pathways and identifies the osteogenesis deterioration (Atashi et al., 2015).
Nowadays, clinical drugs for treating osteoporosis cause serious adverse effects specially kidney injury (Liu et al., 2018). Besides, they also have ineffective prognosis for long-term treatments (Roux and Briot, 2018). Considering the characteristic of chronic diseases, there is an urge for seeking potentially compounds with low toxicity for long-term treatment of osteoporosis.
Recently, traditional herbs are attracting more and more attention owing to their low toxicity, few side effects as well as high safety for long-term treatment (Adler et al., 2016; Kelly et al., 2019). Salvia miltiorrhiza Bunge, one common traditional Chinese medicine, is widely used to treat dementia and cardiovascular diseases (Chen et al., 2019). Pueraria montana var. lobata (Willd.) Maesen and S.M.Almeida ex Sanjappa and Predeep (Radix Puerariae), another important medicine, has been reported to improve liver function (Feng et al., 2019), enhance alcohol detoxification processes, regulate cardiac functions (Tan et al., 2017), as well as aid weight loss (Adler et al., 2016). Our previous study showed that herb pair Salvia miltiorrhiza Bunge-Radix Puerariae (DG) apparently improved vascular injury in diabetic mouse model by reducing oxidative stress (Zhao et al., 2019). In view of the fact that oxidative stress takes part in regulating activity and function of osteoblasts and osteoclasts cells (Forte et al., 2017), the effects of DG on osteoporosis and related mechanisms were explored both in vivo and in vitro.
Materials and Methods
Chemical Compounds and Reagents
Salvia miltiorrhiza Bunge and Radix Puerariae were purchased from Changda Prepared Chinese Medicinal Herbs Co. Ltd (Anguo, China). Healthy SD rats, SPF clean grade, female, 10 weeks old, weight 200–250 g, were purchased from Chinese Academy of Sciences; The ELISA kits for detecting blood urea nitrogen (BUN), creatinine, ALP, OPG and RANKL were purchased from Wuhan Huamei Biological co., LTD (Wuhan, China); DCFH2-DA, Fluo-3/AM, BAPTA-AM and Hoechst 33,258 were obtained from Molecular Probes (Eugene, OR); Chemicals used for DPI, TTRA, AA, ALL, NAC, NDGA, Rot were purchased from Sigma-Aldrich (St. Louis, MO). H&E and Masson dying kits were purchased from Nanjing Jiancheng Biological Engineering Research Institute (Nanjing, China); 3-methyladenine (3-MA), and monodansyl cadaverine (MDC) were obtained from MedChemExpress; TRAP staining kit was obtained from BIO-SCIENCE COMPANY LIMITED (Shanghai, China); Antibodies GAPDH, LC3B, Beclin-1, p62, p-p65 and NF-κBp65 were purchased from Sigma Company; Antibodies NFATc1 and c-Fos were purchased from Chengdu Zen Bio (Chengdu, China); Secondary antibodies were purchased from American CST Company; Hoechst 33,342 was purchased from Thermo Fisher Scientific; JYB1-1 calcium removal solution and protein extraction kit were purchased from Solarbio Company (Beijing, China); RANKL was purchased from Sino Biological Company (Beijing, China).
Preparation of DG
The dried Salvia miltiorrhiza Bunge and Radix Puerariae were crushed into powder and 500 g powder (1:1 w/w; 250 g each) was put into 6,000 ml water at 25°C for 30 min and then extracted under 100°C for 45 min. The extraction procedure was repeated. After freeze drying, the extract was produced into powder and stored at 4°C.
Analysis of DG Constitutes
The exact constitutes of DG was detected with HPLC. Detail experimental conditions and information are prepared as described previously (Zhao et al., 2019) (Supplementary Figure S2).
Animal Experiments and Ethical Statement
Forty specific pathogen-free SD rats were approved by the Animal Experimentation Ethics Committee of Chinese Medicine. All animal procedures followed the NIH guide for the Care and Use of Laboratory Animals (NIH Publications No. 80-23, revised 1978). One week after the rats arrived, 10 female rats in the same physiological condition underwent bilateral laparotomy (Sham), and the other 30 underwent bilateral ovariectomy (OVX). OVX female rats were further randomly assigned into three groups (n = 10/group): vehicle group, DG (50 mg/kg/d; n = 10), DG (200 mg/kg/d; n = 10) (with orally administrated DG treatment for 8 weeks). After finishing the trials, blood was collected from aorta abdominalis with 15 ml injector and centrifuged at 3,000 rpm for 10 min. Then supernatant was stored at 80°C for biochemical analyses. The left femur samples were removed by gauze and fixed in 4% paraformaldehyde overnight at 4°C, then stored in 70% ethanol for micro-CT scanning. The right femur samples and lumbar vertebras were obtained and fixed in 4% paraformaldehyde for histological detection. Then each group’s sections were taken for staining by H&E, Masson and Immunohistochemistry and the processes strictly followed the instructions of the kits.
Histomorphometric Analysis by Micro-CT
After mice were sacrificed, their left femurs were scanned and trabecular morphometric analysis were performed on a micro-CT imaging system (Skyscan 1,176, Belgium). The trabecular region of interest was selected and analyzed quantifying BMD, BMC and morphometric calculations. Related parameters including bone volumetric fraction (BV/TV, %), trabecular thickness (Tb.Th, mm), trabecular separation (Tb.Sp, mm), and trabecular number (Tb.N, mm−1) were measured.
ELISA Assay
The secretion levels of urea nitrogen (BUN), creatinine, osteoprotegerin (OPG), alkaline phosphatase (ALP) and RANKL in serum were determined using ELISA kits following the instructions of manufacture. Each specimen was repeated 3 times and averaged.
Cell Culture
RAW 264.7 cell line was obtained from American Type Culture Collection (ATCC, Rockville, United States). Cells were cultured in DMEM supplemented with 10% FBS, penicillin (100 μg/ml) and streptomycin (100 μg/ml) in a humidified incubator with 5% CO2 at 37°C.
TRAP Staining
In the experiment, on the 5th day, cells were fixed with 4% paraformaldehyde in PBS for 20 min at room temperature and then washed with PBS. After natural drying, TRAP fixation solution was fixed for 3 min at 4°C and slightly dried after washing. TRAP incubation solution was added and placed in an incubator avoiding light at 37°C for 60 min. Then plants were dyed with hematoxylin solution for 3–5 min, later washed and dried for microscopic examination (Olympus Corporation of the Americas, Waltham, MA, ix81). Finally, TRAP-positive multinucleated cells (purple) with at least five nuclei were counted as osteoclasts.
Network Pharmacology Predicts the Mechanism of DG in Treating Osteoporosis
Inputting DG into the symmap (http://www.symmap.org/) database, we got 615 symptoms and 60 targets. The symptoms and targets were screened by this network. In this network, we selected the symptoms of osteoporosis and targets involved in NF-κB inflammatory.
MDC Staining
MDC was used as a specific autophagolysosome marker to analyze the autophagic process. RAW264.7 cells were cultured on dishes at a density of 1×104 cells/mL overnight and then stimulated with RANKL (50 ng/ml) for 1–7 days, cells were incubated with 50 mM MDC at 37°C for 15 min and washed with 1 × PBS three times with 5 min interval. Finally, the cells were observed under the confocal laser scanning microscopy.
Western Blot Analysis
Treated RAW264.7 cells were lysed with RIPA buffer supplemented with phosphatase inhibitors. Cell lysates were separated using 8–12% SDS-PAGE and then transferred onto PVDF membranes. After membranes were blocked with 5% non-fat milk in TBST (20 mM Tris-HCl, 500 mM NaCl, and 0.1% Tween 20) at room temperature for 1 h, membranes were incubated with targeted primary antibodies and corresponding secondary antibodies. Lastly, chemiluminescence signals were detected with a ChemiDoc™ Imager.
Statistical Analysis
The data were expressed as the means ± SD. Statistical analyses were performed by one-way ANOVA followed by Tukey post-hoc test. Values of p < 0.05 were considered statistically significant.
Results
Effect of DG on OVX-Induced Osteoporosis in Rats
To identify the prediction from network pharmacologic analysis that DG has potential on improving osteoporosis (Figures 1A,B), effects of DG on osteoporosis were evaluated in vivo. Firstly, the rat model of OVX-induced osteoporosis was successfully established. Morphologically, bone loss (Figure 1C), deficiency of type II collagen (Figure 1D), TRAP-positive area (Figure 1E) and Cathepsin K-positive area (Figure 1F) were significantly observed in the femur of OVX group while these phenomena were remarkably restored with DG administration (Figures 1C–F). Secondly, data analysis from micro-CT revealed a significant decrease in bone mineral density (BMD), bone mineral content (BMC), bone volume fraction (BV/TV), trabecular separation (Tb.Sp), trabecular thickness (Tb.Th) and trabecular number (Tb.N) as well as an increase in trabecular separation (Tb.Sp), which were important characteristics of microarchitectural deterioration of tissue. As expected, these phenomena are remarkably reversed by DG administration (Figure 2). In all, current data indicated the potential of DG on ameliorating osteoporosis.
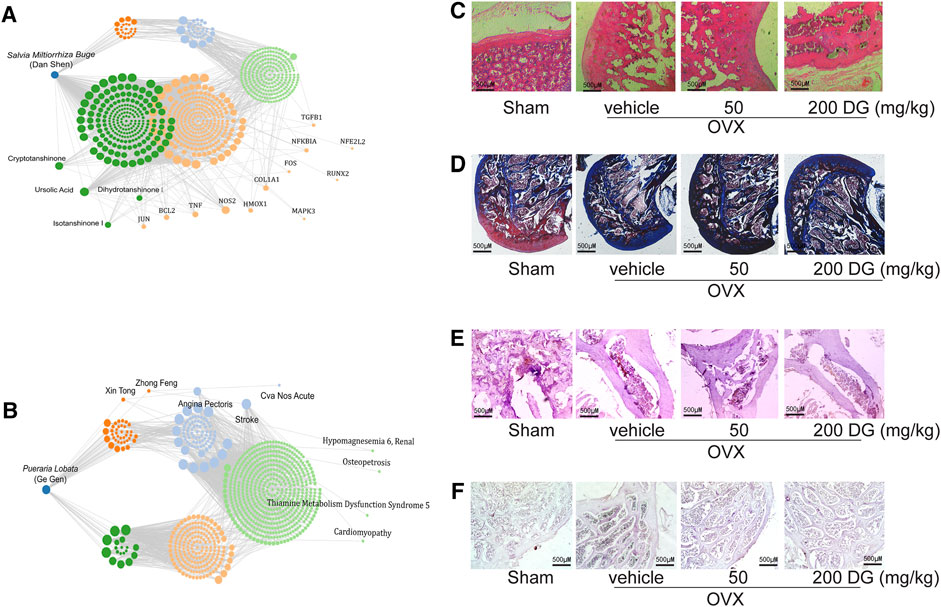
FIGURE 1. DG restored the bone loss and the deficiency of type II collagen in femur from osteoporosis rats (A and B). Data from network pharmacology analysis of Salvia miltiorrhiza Bunge-Radix Puerariae herb Rats were treated as described in MATERIALS AND METHODS section, (C) morphological changes of bone loss in femur were detected by HE staining (magnification × 40), (D) collagen content in femur was detected by Masson staining (magnification × 40), (E) TRAP-positive area was detected by TRAP staining (magnification × 40) and (F) Cathepsin K-positive area was detected by Immunohistochemistry (magnification × 40). DG, aqueous extract of Salvia miltiorrhiza Bunge-Radix Puerariae herb pair.
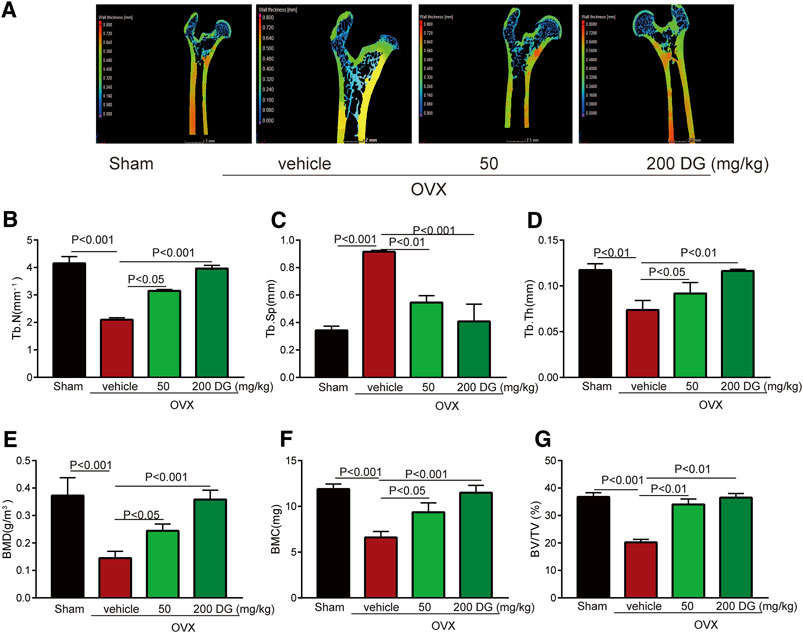
FIGURE 2. DG improved characteristics of microarchitectural deterioration of tissue in osteoporosis rats (A). After rats were treated, left femur samples were isolated for micro-CT scanning. (E) Characteristics of bone microarchitecture in femur including bone mineral density (BMD, g/m3), (F) bone mineral content (BMC, mg), (G) bone volume fraction (BV/TV, %), (C) trabecular separation (Tb.Sp, mm), (D) trabecular thickness (Tb.Th, mm) and (B) trabecular number (Tb.N, mm−1) were detected. DG, aqueous extract of Salvia miltiorrhiza Bunge-Radix Puerariae herb pair.
Mechanisms of DG on Protecting Against OVX-Induced Osteoporosis
The maintenance of bone homeostasis during bone remodeling and modeling was highly dependent on the stable modulation of osteoclast and osteoblast. In present study, data from serum ELISA tests revealed that DG apparently decreased the release of osteoclastogenesis markers RANKL and OPG instead of increasing osteogenesis marker ALP (Figures 3A–C) indicating that DG treated osteoporosis mainly by inhibiting osteoclastogenesis and this suppose was further identified by IHC staining of NFATc1 (Figure 3D). Currently, kidney injury is considered as one serious adverse effect of clinical drugs for treating osteoporosis. As shown in Figure 4, DG administration significantly ameliorated chronic kidney injury by improving glomerular atrophy, decreasing BUN and creatinine release levels in rats from OVX group demonstrating its advantage in the clinic.
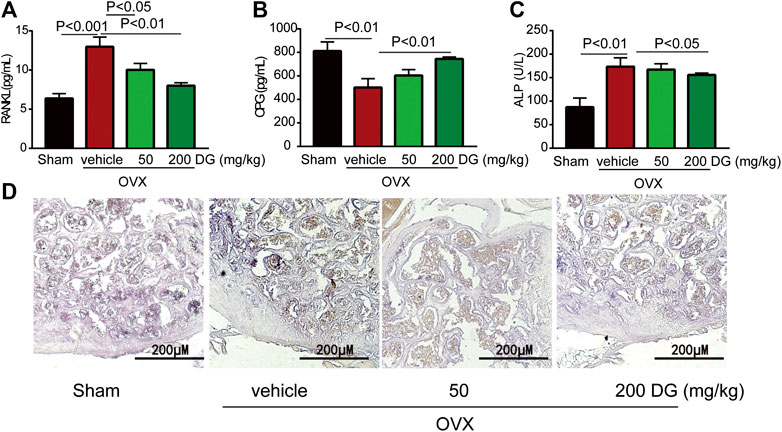
FIGURE 3. DG suppressed osteoclast-mediated bone loss. After rats were treated, serum was collected. (A) Serum RANKL (B) OPG and (C) ALP were detected by ELISA kits. Besides, (D) right femur samples were isolated for NFATc1 staining (magnification × 200). DG, aqueous extract of Salvia miltiorrhiza Bunge-Radix Puerariae herb pair.
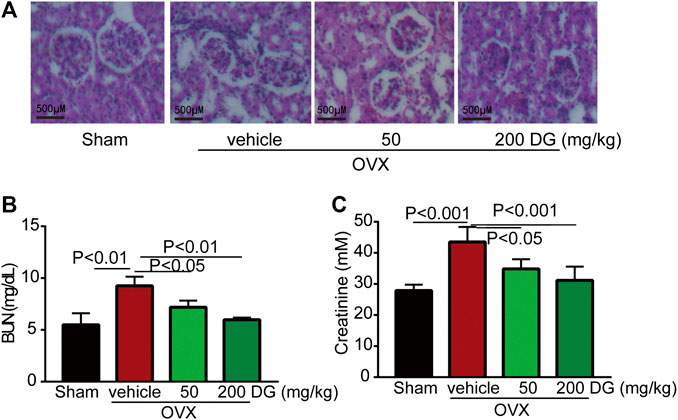
FIGURE 4. DG ameliorated kidney injury in rats with osteoporosis. After rats were treated, kidneys were completely isolated. (A) Morphological changes of glomerulus were assessed with HE staining, (B) kidney function indexes as BUN and (C) creatinine were also detected by ELISA kits. DG, aqueous extract of Salvia miltiorrhiza Bunge-Radix Puerariae herb pair.
Effect of DG on RANKL-Induced Osteoclast Differentiation in RAW264.7 Cell Line
To further investigate the mechanisms of DG on preventing against osteoporosis, in vitro studies have been done. Firstly, to avoid the damage of DG with high concentration on cells, we examined the cytotoxicity of DG in RAW264.7 cells using the MTT assay (Supplementary Figure S1). The data showed that DG had no cytotoxic effect at present concentration 50 μg/ml. Then, a standard osteoclast differentiation model was established in RAW264.7 cells with stimulation RANKL. Formation of TRAP-positive multinucleated osteoclasts were obviously observed in RANKL-treated group. Meanwhile DG pretreatment significantly suppressed above changes (Figure 5A). Furthermore, DG decreased the expression of osteoclast-specific proteins RANK, NFATc1 and c-Fos (Figures 5B,C).
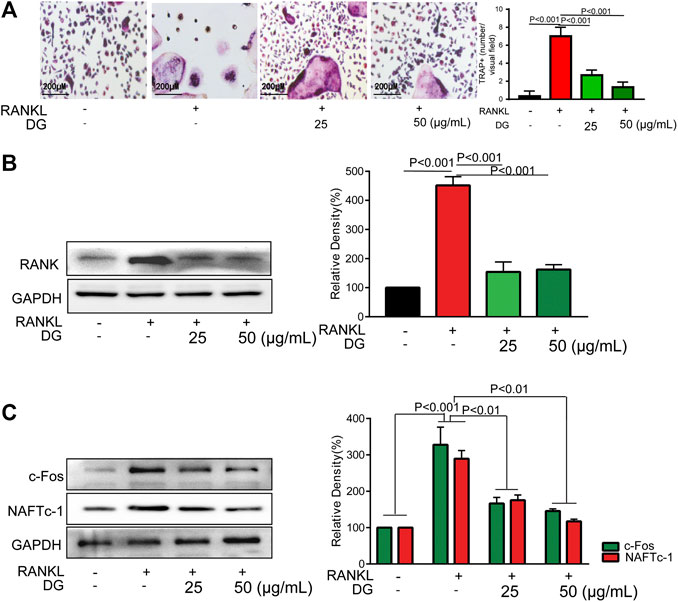
FIGURE 5. DG inhibited osteoclast differentiation in RAW264.7 cells. RAW264.7 cells were cultured for 5 days with RANKL (50 ng/ml) in the presence of DG (25, 50 μg/ml) for 1 h, (A) cells were stained for TRAP (magnification × 200) and (B) related proteins including RANK, (C) osteoclastic-special proteins c-Fos and NFATc1 were detected by western blotting. Representative images were acquired under a light microscope. DG, aqueous extract of Salvia miltiorrhiza Bunge-Radix Puerariae herb pair.
Participation of Autophagy, Oxidative Stress and NF-κB in the Process of Osteoclast Differentiation
Firstly, in our study, LC3B I/II ratio was significantly up-regulated in a time-dependent manner with RANKL stimulation and LC3B-II got the highest expression on fifth day (Figure 6A). Meanwhile autophagic vesicles dyed by MDC staining was markedly observed on fifth day (Figure 6B). Furthermore, autophagy signaling pathway including increased Beclin-1, LC3B and decreased p62/SQSTM1 were also activated in response to RANKL stimulation. However, DG pretreatment obviously reversed these phenomena (Figure 6C). Then lipoxygenases-derived ROS production and Ca2+ overload were obviously induced by RANKL stimulation and reduced by DG pretreatment (Figure 7). Besides, data from network pharmacology predicts that Salvia miltiorrhiza Bunge and Radix Puerariae suppress NF-κB activity (Figures 8A,B). Consistent with the predictability by software, DG significantly inhibited RANKL-stimulated NF-κBp65 phosphorylation (Figure 8C). Lastly, DG and specific inhibitors for ROS, autophagy, and NF-κB all significantly decreased osteoclast-specific proteins NFATc1 and c-Fos expressions (Figure 9). Generally, our study showed that DG protected against OVX-induced osteoporosis in rats by suppressing osteoclast differentiation mediated by autophagy, oxidative stress and NF-κB (Supplementary Figure S1).
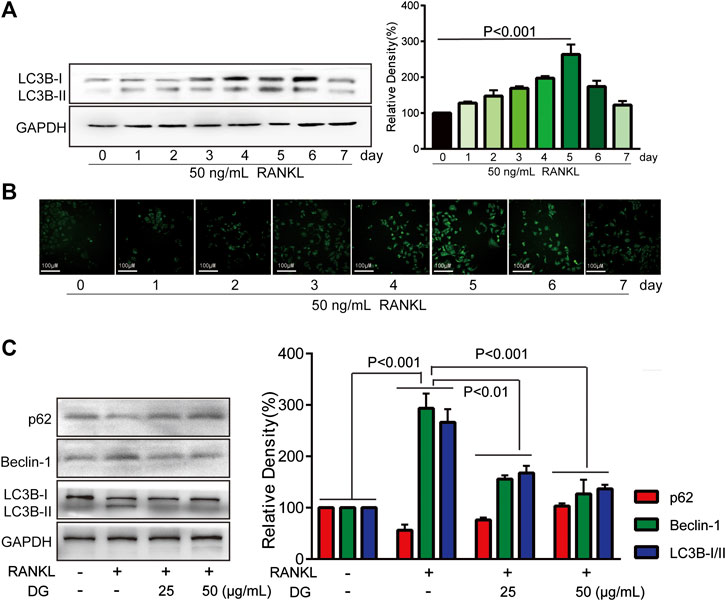
FIGURE 6. DG suppressed RANKL-induced autophagy signaling pathway. RAW264.7 cells were pretreated with RANKL (50 ng/ml) for 0, 1, 2, 3, 4, 5, 6, 7 days, (A) LC3BI/II expression was detected by western blotting and (B) autophagy vacuole was detected by MDC staining. (C) Then RAW264.7 cells were cultured for 5 days with RANKL (50 ng/ml) in the presence of DG (25, 50 μg/ml) for 1 h or not, autophagy-related proteins were detected by western blotting. DG, aqueous extract of Salvia miltiorrhiza Bunge-Radix Puerariae herb pair.
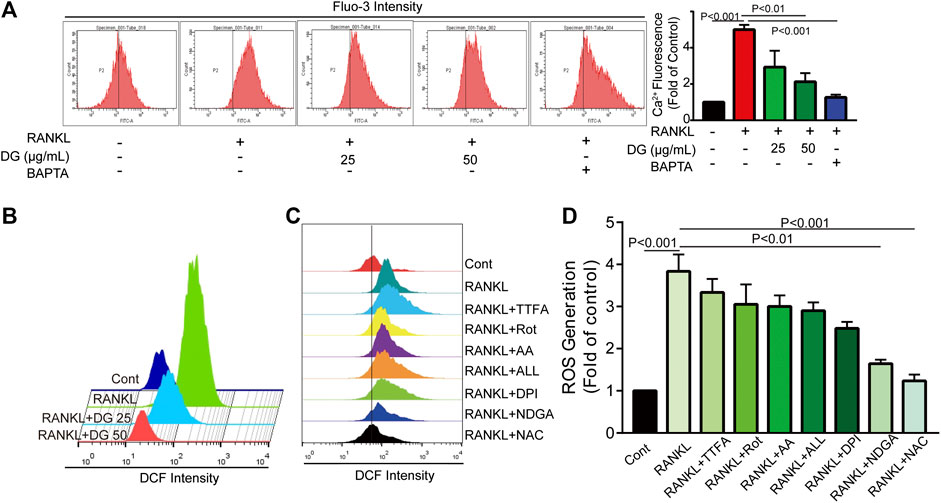
FIGURE 7. DG suppressed RANKL-induced ROS production and Ca2+ overload. The cells were pretreated with DG (50 μg/ml), BAPTA (10 μM), TTFA (10 μM), Rot (10 μM), AA (100 nM), ALL (5 μM), DPI (100 nM), NDGA (10 μM) and NAC (5 mM) separately for 1h and then stimulated with RANKL (50 ng/mL) for 5 days, (A) Ca2+ overload and (B-D) ROS production were determined by flow cytometry. AA, antimycin; ALL, allopurinol; BAPTA, BAPTA-AM; DG, aqueous extract of Salvia miltiorrhiza Bunge-Radix Puerariae herb pair; DPI, diphenyleneiodonium chloride; NAC, N-acetyl-L-cysteine; NDGA, nordihydroguaiaretic acid; Rot, rotenone; TTFA, 2-thenoyltrifluoroacetone.
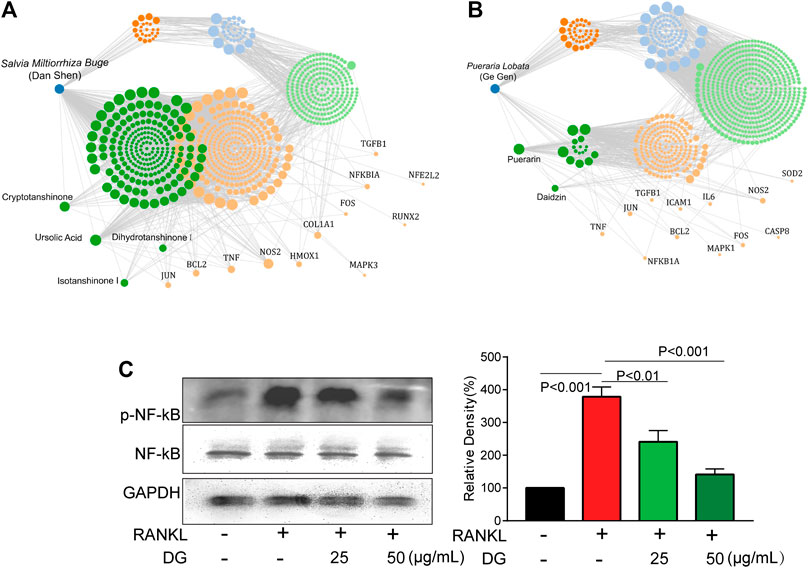
FIGURE 8. DG suppressed NF-κB pathway (A and B) Data from network pharmacology analysis of Salvia miltiorrhiza Bunge-Radix Puerariae herb. (C) RAW264.7 cells were pretreated with DG (25, 50 μg/ml) for 1h in response to RANKL (50 ng/ml) for 5 days, expressions of p-NF-κBp65 and NFκB were detected by western blotting. DG, aqueous extract of Salvia miltiorrhiza Bunge-Radix Puerariae herb pair.
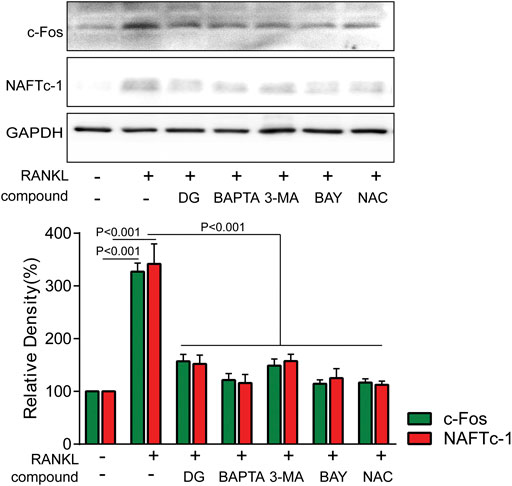
FIGURE 9. DG suppressed RANKL-induced osteoclast differentiation mediated by autophagy, oxidative stress and NF-κB RAW264.7 cells were pretreated with DG (50 μg/ml), BAPTA (10 μM), 3-MA (1 mM), BAY (10 μM) and NAC (5 mM) for 1 h in response to RANKL (50 ng/ml) for 5 days, osteoclastic-special proteins c-Fos and NFATc1 were detected by western blotting. BAPTA, BAPTA-AM; BAY , BAY11-7082; DG, aqueous extract of Salvia miltiorrhiza Bunge-Radix Puerariae herb pair; 3-MA, 3-methyladenine; NAC, N-acetyl-L-cysteine.
Discussion
Considering serious adverse reactions caused by clinical osteoporosis drugs (Su et al., 2019; Chen et al., 2020; Hu et al., 2020), seeking potential drugs with hypotoxicity is one novel therapeutic strategy. In recent years, a large number of studies have proved the beneficial effect of Chinese herbal medicine in the treatment for osteoporosis. Polysaccharides isolated from lotus leaves exert anti-osteoporotic effects by inhibiting osteoclastogenesis (Hwang et al., 2020). Catalpol, the primary active principle component of Rehmanniae Radix, promotes the osteogenic differentiation of bone marrow mesenchymal stem cells via the Wnt/β-catenin pathway (Zhu et al., 2019). Polysaccharides from the roots of Morinda officinalis induce bone formation by up-regulating the expression of runt-related transcription factor 2, osterix, osteopontin, and osteocalcin (Yan et al., 2019). Resveratrol promotes osteogenesis via activating SIRT1/FoxO1 pathway in osteoporosis mice (Hwang et al., 2019). Salvia miltiorrhiza Bunge (Danshen) and Radix Puerariae (Gegen) are two valued traditional Chinese medicine for multiple therapeutic remedies. A classical herbal pair contains both Danshen and Gegen has a history of usage in China for treating a series of diseases including cardiovascular disease, diabetes and aging-induced diseases (Lim et al., 2013; Guo et al., 2014). In this study, protective effects and mechanisms of this herb pair on osteoporosis were explored.
Firstly, in in vivo study, bone destruction, microarchitectural deterioration and decreased bone density of femur bone were occurred in OVX group while DG significantly reversed these changes hinting DG’ potential for improving osteoporosis. Furthermore, DG balanced the release levels of serum osteoclast-specific marker RANKL and OPG as well as decreased NFATc1-positive osteoclasts in lumbar vertebra in OVX group. These data hinted that possible mechanisms for DG ameliorating osteoporosis was to suppress osteoclast activities. Chronic kidney injury is accepted one serious adverse effect for mostly clinical drugs in treating bone disorder diseases. In our present study, we deliberately detected kidney function of rats in different groups. Intriguingly, compared with OVX group, DG apparently improved kidney function exhibiting its advantage for being ideal clinical application.
Clinically
Furthermore, in in vitro studies, RANKL-induced osteoclast formation accompanied by increased proteins expressions of osteoclastic-special marker c-Fos and NFATc1 were extensively suppressed with DG pretreatment. Then autophagy was occurred with RANKL stimulation evidenced by occurrence of autophagic vesicles and activation of autophagy related marker proteins while DG pretreatment significantly reversed these phenomena. Besides, DG evidently reduced RANK-induced ROS production. There are a variety of sources for ROS including NADPH oxidases, xanthine oxidases, arachidonic acid by lipoxygenases, and the mitochondrial respiratory chain (Mailloux et al., 2013; Aviello and Knaus, 2018; Mazat et al., 2020). In present study, NDAPH oxidase inhibitor diphenyleneiodonium chloride (DPI), xanthine oxidases inhibitor allopurinol (ALL), mitochondria respiratory electron-transport chain inhibitors antimycin (AA), rotenone (Rot) and 2-thenoyltrifluoroacetone (TTFA) had no significant scavenging effect response to RANK-induced ROS generation while lipoxygenases inhibitor nordihydroguaiaretic acid (NDGA) had similar scavenging capacity with DG hinting lipoxygenases was the main source of ROS generation induced by RANK. Besides, NF-κB is considered an activator of NFATc1 and c-Fos (Guo et al., 2019; Zhang et al., 2019). Data analysis from our network pharmacology showed that DG had a potential for inhibiting NF-κB. In practical experiments, DG did block RANKL-induced NF-κB/p65 activation. Finally, autophagy inhibitor, ROS scavenger, Ca2+ chelator and NF-κB inhibitor significantly suppressed osteoclast differentiation.
Conclusion
Our in vivo research reveals DG’s protective effect against osteoporosis in OVX rats. In vitro, DG inhibits osteoclast differentiation. All autophagy, ROS and NF-κB are all involved in this process and regulated by DG. Present study offers scientific support for DG’s widely application for treating age-ralated diseases as health care products or therapeutic drugs.
Data Availability Statement
The raw data supporting the conclusions of this article will be made available by the authors, without undue reservation, to any qualified researcher.
Ethics Statement
Animals study was approved by the Animal Experimentation Ethics Committee of Chinese Medicine. All animal procedures followed the NIH guide for the Care and Use of Laboratory Animals (NIH Publications No. 80-23, revised 1978).
Author Contributions
YH, YJ, WZ, HQ, and XP Chen take responsibility for the integrity of the data and the accuracy of the data analysis; Concept and design: WZ, HQ, XC, and YH; Acquisition, analysis, or interpretation of data: YJ, HZ, JJ, WZ, and QL; Critical revision of the manuscript for important intellectual content: HZ, WZ, HQ, and YH.
Funding
This study was supported by the National Natural Science Foundation of China (81803766), the Key Research and Discovery Program of Shandong Province (2019GSF107072), the Natural Science Foundation of Shandong Province (ZR2018BH036) and China Postdoctoral Science Foundation (2019M652336).
Conflict of Interest
The authors declare that the research was conducted in the absence of any commercial or financial relationships that could be construed as a potential conflict of interest.
Acknowledgments
We thank Haoyi Zheng for providing a model adopting network pharmacology to predict the active components’ pharmacology.
Supplementary Material
The Supplementary Material for this article can be found online at: https://www.frontiersin.org/articles/10.3389/fphar.2020.581049/full#supplementary-material.
Abbreviations
AA, antimycin; ALL, allopurinol; ALP, alkaline phosphatase; BAPTA, BAPTA-AM; BAY, BAY11–7,082; BUN, blood urea nitrogen; CQ, chloroquine; DG, aqueous extract of Salvia miltiorrhiza Bunge-Radix Puerariae herb pair; DPI, diphenyleneiodonium chloride; M-CSF, macrophage colony stimulating factor; MDC, monodansylca daverine; NAC, N-acetyl-l-cysteine; NDGA, nordihydroguaiaretic acid; NFATc1, nuclear factor of activated T-cells, cytoplasmic 1; OPG, osteoprotegerin; OVX, ovariectomy; RANKL, receptor activator of nuclear factor kappa-B ligand; Rot, rotenone; TRAP, tartrate-resistant acid phosphatase; TRAF6, TNF receptor associated factor 6; TTFA, 2-thenoyltrifluoroacetone; 3-MA, 3-methyladenine
References
Adler, R., El-Hajj Fuleihan, G., Bauer, D., Camacho, P., Clarke, B., Clines, G., et al. (2016). Managing osteoporosis in patients on long-term bisphosphonate treatment: report of a task force of the American society for bone and mineral research. J. Bone Miner. Res. 31 (1), 16–35. doi:10.1002/jbmr.2708
Appelman-Dijkstra, N., and Papapoulos, S. (2015). Modulating bone resorption and bone formation in opposite directions in the treatment of postmenopausal osteoporosis. Drugs. 75 (10), 1049–1058. doi:10.1007/s40265-015-0417-7
Atashi, F., Modarressi, A., and Pepper, M. S. (2015). The role of reactive oxygen species in mesenchymal stem cell adipogenic and osteogenic differentiation: a review. Stem Cell. Dev. 24 (10), 1150–1163. doi:10.1089/scd.2014.0484
Aviello, G., and Knaus, U. G. (2018). NADPH oxidases and ROS signaling in the gastrointestinal tract. Mucosal Immunol. 11 (4), 1011–1023. doi:10.1038/s41385-018-0021-8
Cackowski, F. C., Anderson, J. L., Patrene, K. D., Choksi, R. J., Shapiro, S. D., Windle, J. J., et al. (2010). Osteoclasts are important for bone angiogenesis. Blood. 115 (1), 140–149. doi:10.1182/blood-2009-08-237628
Chen, C., Chen, Y., Chu, M., Wang, C., Chung-Yee Hui, R., Lu, C., et al. (2020). The risk of anti-osteoporotic agent-induced severe cutaneous adverse drug reactions and their association with HLA. JEADV. [Epub ahead of print]. doi:10.1111/jdv.16924
Chen, X., Yu, J., Zhong, B., Lu, J., Lu, J., Li, S., et al. (2019). Pharmacological activities of dihydrotanshinone I, a natural product from Salvia miltiorrhiza Bunge. Pharmacol. Res. 145, 104254. doi:10.1016/j.phrs.2019.104254
Du, T., Yan, Z., Zhu, S., Chen, G., Wang, L., Ye, Z., et al. (2020). QKI deficiency leads to osteoporosis by promoting RANKL-induced osteoclastogenesis and disrupting bone metabolism. Cell Death and Disease. 11 (5), 330. doi:10.1038/s41419-020-2548-3
Feng, R., Chen, J., Liu, C., Xia, F., Xiao, Z., Zhang, X., et al. (2019). A combination of Pueraria lobata and Silybum marianum protects against alcoholic liver disease in mice. Phytomedicine. 58, 152824. doi:10.1016/j.phymed.2019.152824
Forte, L., Torricelli, P., Boanini, E., Rubini, K., Fini, M., and Bigi, A. (2017). Quercetin and alendronate multi-functionalized materials as tools to hinder oxidative stress damage. J. Biomed. Mater. Res. 105 (12), 3293–3303. doi:10.1002/jbm.a.36192
Guo, D., Hong, D., Wang, P., Wang, J., Chen, L., Zhao, W., et al. (2019). Histone deacetylase inhibitor CI-994 inhibits osteoclastogenesis via suppressing NF-κB and the downstream c-Fos/NFATc1 signaling pathways. Eur. J. Pharmacol. 848, 96–104. doi:10.1016/j.ejphar.2019.01.021
Guo, Y., Li, Y., Xue, L., Severino, R. P., Gao, S., Niu, J., et al. (2014). Salvia miltiorrhiza: an ancient Chinese herbal medicine as a source for anti-osteoporotic drugs. J. Ethnopharmacol. 155 (3), 1401–1416. doi:10.1016/j.jep.2014.07.058
Hu, Q., Long, C., Wu, D., You, X., Ran, L., Xu, J., et al. (2020). The efficacy and safety of ipriflavone in postmenopausal women with osteopenia or osteoporosis: a systematic review and meta-analysis. Pharmacol. Res. 159, 104860. doi:10.1016/j.phrs.2020.104860
Hwang, Y., Ha, H., Kim, R., Cho, C., Song, Y., Hong, H., et al. (2019). Protective effects of a polysaccharide BLE0 isolated from barley leaf on bone loss in ovariectomized mice. Int. J. Biol. Macromol. 123, 314–321. doi:10.1016/j.ijbiomac.2018.11.075
Hwang, Y., Jang, S., Lee, A., Cho, C., Song, Y., Hong, H., et al. (2020). Polysaccharides isolated from lotus leaves (LLEP) exert anti-osteoporotic effects by inhibiting osteoclastogenesis. Int. J. Biol. Macromol. 161, 449–456. doi:10.1016/j.ijbiomac.2020.06.059
Jiang, J., Pang, X., Liu, H., Yang, X., Zhang, Y., Xiang, X., et al. (2019). Reduced TIPE2 expression is inversely associated with proinflammatory cytokines and positively correlated with bone mineral density in patients with osteoporosis. Life Sci. 216, 227–232. doi:10.1016/j.lfs.2018.11.054
Kelly, R., McDonald, L., Jensen, N., Sidles, S., and LaRue, A. (2019). Impacts of psychological stress on osteoporosis: clinical implications and treatment interactions. Front. Psychiatr. 10, 200. doi:10.3389/fpsyt.2019.00200
Li, W., Yuan, L., Tong, G., He, Y., Meng, Y., Hao, S., et al. (2018). Phospholipase C signaling activated by parathyroid hormone mediates the rapid osteoclastogenesis in the fracture healing of orchiectomized mice. BMC Musculoskeletal Disorders. 19 (1), 311. doi:10.1186/s12891-018-2231-3
Lim, D. W., Kim, J. G., and Kim, Y. T. (2013). Effects of dietary isoflavones from Puerariae radix on lipid and bone metabolism in ovariectomized rats. Nutrients. 5 (7), 2734–2746. doi:10.3390/nu5072734
Liu, F., Tan, F., Tong, W., Fan, Q., Ye, S., Lu, S., et al. (2018). Effect of Zuoguiwan on osteoporosis in ovariectomized rats through RANKL/OPG pathway mediated by β2AR. Biomed. Pharmacother. 103, 1052–1060. doi:10.1016/j.biopha.2018.04.102
Ma, Q., Fang, L., Jiang, N., Zhang, L., Wang, Y., Zhang, Y., et al. (2018). Bone mesenchymal stem cell secretion of sRANKL/OPG/M-CSF in response to macrophage-mediated inflammatory response influences osteogenesis on nanostructured Ti surfaces. Biomaterials. 154, 234–247. doi:10.1016/j.biomaterials.2017.11.003
Mailloux, R. J., McBride, S. L., and Harper, M. E. (2013). Unearthing the secrets of mitochondrial ROS and glutathione in bioenergetics. Trends Biochem. Sci. 38 (12), 592–602. doi:10.1016/j.tibs.2013.09.001
Mazat, J. P., Devin, A., and Ransac, S. (2020). Modelling mitochondrial ROS production by the respiratory chain. Cell. Mol. Life Sci. 77 (3), 455–465. doi:10.1007/s00018-019-03381-1
Mirza, F., and Canalis, E. (2015). Management of endocrine disease: secondary osteoporosis: pathophysiology and management. Eur. J. Endocrinol. 173 (3), R131–R151. doi:10.1530/EJE-15-0118
Roux, C., and Briot, K. (2018). Osteoporosis in 2017: addressing the crisis in the treatment of osteoporosis. Nat. Rev. Rheumatol. 14 (2), 67–68. doi:10.1038/nrrheum.2017.218
Schröder, K. (2015). NADPH oxidases in bone homeostasis and osteoporosis. Cell. Mol. Life Sci. 72 (1), 25–38. doi:10.1007/s00018-014-1712-2
Shen, G., Ren, H., Shang, Q., Qiu, T., Yu, X., Zhang, Z., et al. (2018). Autophagy as a target for glucocorticoid-induced osteoporosis therapy. Cell. Mol. Life Sci. 75 (15), 2683–2693. doi:10.1007/s00018-018-2776-1
Su, S., He, N., Men, P., Song, C., and Zhai, S. (2019). The efficacy and safety of menatetrenone in the management of osteoporosis: a systematic review and meta-analysis of randomized controlled trials. Osteoporos. Int. 30 (6), 1175–1186. doi:10.1007/s00198-019-04853-7
Tan, C., Wang, A., Liu, C., Li, Y., Shi, Y., and Zhou, M. (2017). Puerarin improves vascular insulin resistance and cardiovascular remodeling in salt-sensitive hypertension. Am. J. Chin. Med. 45 (6), 1169–1184. doi:10.1142/s0192415x17500641
Thummuri, D., Jeengar, M., Shrivastava, S., Nemani, H., Ramavat, R., Chaudhari, P., and et al. Naidu, V. G. (2015). Thymoquinone prevents RANKL-induced osteoclastogenesis activation and osteolysis in an in vivo model of inflammation by suppressing NF-KB and MAPK Signalling. Pharmacol. Res. 99, 63–73. doi:10.1016/j.phrs.2015.05.006
Tu, Y., Wang, K., Tan, L., Han, B., Hu, Y., Ding, H., et al. (2020). Dolichosin A, a coumestan isolated from Glycine tabacina, inhibits IL-1β-induced inflammation in SW982 human synovial cells and suppresses RANKL-induced osteoclastogenesis: from network pharmacology to experimental pharmacology. J. Ethnopharmacol. 258, 112855. doi:10.1016/j.jep.2020.112855
Wang, L., Heckmann, B., Yang, X., and Long, H. (2019). Osteoblast autophagy in glucocorticoid-induced osteoporosis. J. Cell. Physiol. 234 (4), 3207–3215. doi:10.1002/jcp.27335
Wehmeyer, C., Frank, S., Beckmann, D., Böttcher, M., Cromme, C., König, U., et al. (2016). Sclerostin inhibition promotes TNF-dependent inflammatory joint destruction. Sci. Transl. Med. 8 (330), 330ra35. doi:10.1126/scitranslmed.aac4351
Yan, C., Huang, D., Shen, X., Qin, N., Jiang, K., Zhang, D., et al. (2019). Identification and characterization of a polysaccharide from the roots of Morinda officinalis, as an inducer of bone formation by up-regulation of target gene expression. Int. J. Biol. Macromol. 133, 446–456. doi:10.1016/j.ijbiomac.2019.04.084
Zhang, Y., Zou, B., Tan, Y., Su, J., Wang, Y., Xu, J., et al. (2019). Sinomenine inhibits osteolysis in breast cancer by reducing IL-8/CXCR1 and c-Fos/NFATc1 signaling. Pharmacol. Res. 142, 140–150. doi:10.1016/j.phrs.2019.02.015
Zhao, W., Yuan, Y., Zhao, H., Han, Y., and Chen, X. (2019). Aqueous extract of Salvia miltiorrhiza Bunge-Radix Puerariae herb pair ameliorates diabetic vascular injury by inhibiting oxidative stress in streptozotocin-induced diabetic rats. Food Chem. Toxicol. 129, 97–107. doi:10.1016/j.fct.2019.04.018
Keywords: osteoporosis, Salvia miltiorrhiza bunge-radix puerariae, autophagy, oxidative stress, osteoclast differentiation
Citation: Qin H, Zhao W, Jiao Y, Zheng H, Zhang H, Jin J, Li Q, Chen X, Gao X and Han Y (2021) Aqueous Extract of Salvia miltiorrhiza Bunge-Radix Puerariae Herb Pair Attenuates Osteoporosis in Ovariectomized Rats Through Suppressing Osteoclast Differentiation. Front. Pharmacol. 11:581049. doi: 10.3389/fphar.2020.581049
Received: 07 July 2020; Accepted: 11 December 2020;
Published: 21 January 2021.
Edited by:
Anna Karolina Kiss, Medical University of Warsaw, PolandReviewed by:
An Qin, Shanghai Ninth People’s Hospital, ChinaJen-Tsung Chen, National University of Kaohsiung, Taiwan
Copyright © 2021 Qin, Zhao, Jiao, Zheng, Zhang, Jin, Li, Chen, Gao and Han. This is an open-access article distributed under the terms of the Creative Commons Attribution License (CC BY). The use, distribution or reproduction in other forums is permitted, provided the original author(s) and the copyright owner(s) are credited and that the original publication in this journal is cited, in accordance with accepted academic practice. No use, distribution or reproduction is permitted which does not comply with these terms.
*Correspondence: Yantao Han, NDA4MzI3NjYxQHFxLmNvbQ==
†These authors have contributed equally to this work and share first authorship