- 1Department of Clinical Pharmacology and Toxicology, Anam Hospital, Korea University College of Medicine, Seoul, Korea
- 2Department of Clinical Pharmacology and Toxicology, Guro Hospital, Korea University College of Medicine, Seoul, Korea
Imatinib is transported extracellularly by ABCB1 and ABCG2 efflux transporters and bound to alpha-1-acid glycoprotein (AGP) in the bloodstream. However, the clinical and pharmacokinetic effects of ABCB1 and ABCG2 on imatinib were inconsistent in the previous literature and have not been confirmed. Therefore, in the present study, we explored the effects of the ABCG2 and ABCB1 genetic polymorphisms on imatinib pharmacokinetics in association with plasma AGP levels in healthy subjects. Twenty-seven healthy individuals were recruited, genotyped for ABCG2 and ABCB1, and given a single oral dose of 400 mg imatinib. Plasma imatinib concentrations were measured and its pharmacokinetics was assessed with respect to ABCG2 (c.421C>A and c.34G>A) and ABCB1 (c.1236C>T, c.2677C>T/A, and c.3435C>T) genotypes, and plasma AGP levels. AGP levels showed a strong positive correlation with imatinib pharmacokinetics. ABCG2 c.421C>A single nucleotide polymorphism showed a statistically significant effect on imatinib pharmacokinetics in low plasma AGP levels groups (<80 mg/dl); subjects with high plasma AGP levels (n = 5, ≥80 mg/dl) were excluded. The results indicate that plasma AGP levels and ABCG2 polymorphisms modulated imatinib pharmacokinetics; however, the effects of the ABCG2 transporter was masked at high plasma AGP levels.
Introduction
Imatinib mesylate (Gleevec, formerly STI-571) is an approved drug for chronic myeloid leukemia (CML) (Capdeville et al., 2002) and gastrointestinal stromal tumors (GISTs) that work by selectively inhibiting BCR-ABL and tyrosine kinase (Demetri et al., 2002). It has been reported that patients with poor imatinib treatment response, generally have lower systemic levels of imatinib than patients who respond well (Picard et al., 2007; Larson et al., 2008). Furthermore, plasma levels of imatinib correlate with clinical response and survival rates (Picard et al., 2007; Demetri et al., 2009). Considerable inter-individual differences have been observed in imatinib pharmacokinetics (Peng et al., 2005) and therefore variable response.
Imatinib is a biopharmaceutical classification system (BCS) Class 1 compound with high permeability and solubility (Bhattacharya, 2020). It is well absorbed with an absolute bioavailability of 98%, and it reaches Cmax in 2–4 h (Peng et al., 2004). Imatinib is metabolized in the liver, predominantly by cytochrome P450 isoforms CYP3A4 and CYP3A5 and to a lesser extent CYP1A2, CYP2D6, CYP2C8, CYP2C9, and CYP2C19 (Gschwind et al., 2005; Nebot et al., 2010). Imatinib is also a substrate of drug efflux ATP-Binding Cassette (ABC) transporters, including ABCB1 (MDR, P-glycoprotein) and ABCG2 (BCRP, breast cancer resistance protein) (Burger et al., 2004; Oostendorp et al., 2009; Eechoute et al., 2011). These transporters are substantially involved in the absorption, distribution, and elimination of drugs through efflux.
The two drug transporters are polymorphic and their polymorphisms are known to alter substrates’ blood levels and therefore clinical effects (i.e., loss of function of the transporters) (Kim et al., 2007; Haufroid, 2011; Kim et al., 2011a; Silverton et al., 2011). Although previous investigations produced contrasting results, a review of the literature indicated that the polymorphisms of either ABCB1 (c.1236C>T, c.2677C>T/A, and c.3435C>T) or ABCG2 (c.421C>A and c.34G>A) possibly influenced the plasma levels and clearance of imatinib, as well as patients’ clinical outcomes (Eechoute et al., 2011). Additionally, imatinib primarily binds to plasma alpha-1-acid glycoprotein (AGP) (approximate mean free fraction: 4%), which may significantly alter drug distribution and elimination; it has been revealed that plasma imatinib pharmacokinetics is affected by AGP levels (Gambacorti-Passerini et al., 2003). Since AGP levels are known to be elevated in various physiologic (e.g., age, pregnancy, obesity) and disease conditions (e.g., inflammation, cancer) (Kim et al., 2015), the pharmacokinetics of imatinib is prone to effects of diverse underlying conditions.
Despite various studies reporting the involvement of ABCB1 and ABCG2 transporters in the disposition of imatinib (Burger and Nooter, 2004), their roles remain controversial with limited information on their effects on imatinib pharmacokinetics. In addition, to date, no study has evaluated the effects of both the AGP levels and polymorphisms of ABCB1 and ABCG2 transporters on imatinib pharmacokinetics. In the present study, we investigated the effects of both polymorphic ABCG2 and ABCB1 genotypes, and plasma AGP levels on imatinib pharmacokinetics in a controlled study of healthy subjects. We hypothesized that the effect of ABCG2 and ABCB1 single nucleotide polymorphism on the imatinib pharmacokinetics was dependent on AGP levels and that in low AGP levels, ABCG2 and ABCB1 transporters increased the free fraction of imatinib. We aimed at providing insight, and a clear understanding of their effects on imatinib pharmacokinetics and their relationship with the genetic contribution to interindividual variation of imatinib exposure and response.
Material and Methods
Subjects
Twenty-seven healthy male participants were enrolled with a mean (±S.D.) age of 24.6 ± 1.9 years (range, 23–30 years), mean (±S.D.) weight of 69.4 ± 7.6 kg (range, 54–68 kg), and mean (±S.D.) height of 174.8 ± 4.7 cm (range, 164–174 cm). All subjects were confirmed to be healthy by a physician through a detailed physical examination; 12-lead electrocardiography, serum biochemistry, hematology, and urinalysis. Exclusion criteria were: a history of, or evidence of, a hepatic, renal, gastrointestinal, or hematologic abnormality, any other acute or chronic disease, or an allergy to any drug. All subjects were nonsmokers and were not taking any medication, and provided written informed consent. The study protocol was approved by the Institutional Review Board (IRB) of Anam Hospital, Korea University, Korea (clinicaltrial.gov; NCT01270984).
ABCG2 and ABCB1 Genotyping
To determine the ABCG2 and ABCB1 genotypes, a blood sample was obtained from each of the participants and stored at −20°C awaiting DNA extraction. Genomic DNA was isolated from peripheral leukocytes. All individuals were genotyped for the c.421C>A and c.34G>A alleles of ABCG2 polymorphisms and for the c.1236C>T, c.2677C>T(A), and c.3435C>T alleles of ABCB1 polymorphisms through pyrosequencing methods using a pyrosequencer (Biotage, Uppsala, Sweden), to evaluate the SNPs rapidly, precisely, and cost-effectively as described previously (Kim et al., 2010; Kim et al., 2015).
Study Design
After overnight fasting, the subjects were given a single oral dose of 400 mg imatinib (Gleevec; Novartis Korea, Seoul, Korea) with 240 ml of water. Blood samples were collected in heparinized tubes (Vacutainer; Becton Dickinson, Franklin Lakes, NJ) immediately before drug administration (baseline) and at 0.5, 1, 1.5, 2, 2.5, 3, 3.5, 4, 5, 6, 7, 8, 10, 12, 24, 48, and 72 h after drug administration. Plasma was separated by centrifugation (1,977 g, 4°C) for 15 min, and the samples were stored at −70°C awaiting analysis.
Determination of Imatinib Concentration in Plasma Samples
The plasma concentrations of total imatinib (both protein-bound and unbound form of imatinib) were determined using a previously validated LC-MS/MS method with slight modifications (Kim et al., 2011b). Liquid-liquid extraction (LLE) technique was used to separate the imatinib from the plasma sample. Plasma sample (0.2 ml) was added to a glass tube containing the internal standard amount of imatinib (20 μL of imatinib-d8 5 μg/ml). The tube was shaken for 10 s, after which 1.5 ml methyl t-butyl ether was added to the mixture, which was then vortexed for 20 min. The organic phase was then transferred to a clean glass tube and evaporated to dryness under a flow of nitrogen gas. After reconstitution of the dry residue with 1 ml acetonitrile (50%), a 2 μl aliquot of this solution was injected onto the LC-MS/MS system, equipped with an Imtakt Unison C8 column (3 μm, 75 mm × 2.0 mm, Imtakt Corp., Kyoto, Japan). The mobile phase was composed of 10 mM ammonium formate: acetonitrile: formic acid (60:40:0.1, vol/vol/vol) and used at a constant flow rate of 0.2 ml/min. Quantification was performed in multiple reaction monitoring modes, with the transitions of m/z 494.4→217.1 for imatinib and m/z 502.4→225.1 for the internal standard. A linear calibration curve within the range of 5–5000 ng/ml was established for imatinib. The regression correlation coefficients of the calibration curves were >0.9999. Intra-day and inter-day coefficient of variations (CVs) was below 5%.
Pharmacokinetic Analysis
The pharmacokinetic parameters for imatinib were determined by non-compartmental analysis using WinNonlin software (version 8.1, Certara, NJ, United States). The maximum plasma concentration (Cmax) and the time to reach Cmax (Tmax) were estimated directly from the raw data. The total area under the plasma concentration-time curve (AUCall) was calculated using the linear trapezoidal rule, with extrapolation to infinity (AUCinf) by the division of the last measured concentration by the elimination constant (Ke). The value of Ke was obtained from the slope of the linear regression of the log-linear part of the raw data. The t1/2 (half-life) was equal to ln2/Ke and the oral clearance (CL/F) of imatinib was estimated by dose/AUCinf.
Determination of AGP Levels in Plasma
Plasma AGP concentration was measured in samples obtained immediately before imatinib treatment. AGP concentration was determined using a commercially available ELISA kit (Abcam Inc., Cambridge, MA) and the intra-assay and inter-assay CV values were 4.4% and 7.0%, respectively.
Statistical Analysis
The data were expressed as the mean ± SD in the text and tables and, for clarity, as the mean ± SEM in figures. The statistical comparisons between the ABCG2/ABCB1 genotype groups were made with one-way analysis of variance (ANOVA) or Kruskal-Wallis one-way ANOVA by rank, with multiple post hoc comparisons performed after the normality test. Before the ANOVA test, the genotype groups were compared by the performance of an analysis of covariance with an effective term for both ABCG2 and ABCB1 genotypes and with demographic data including age, body weight, and height as covariates. The possible correlation between AGP levels and imatinib pharmacokinetics parameters was assessed using parametric Pearson’s correlation coefficient. The plasma AGP levels were illustrated by a probability plot and were examined for normality of the distribution by the normality test. The data analysis was computed with the statistical program SAS 9.2 for Windows. p values of < 0.05 were considered to indicate statistically significant differences.
Results
Demographic Data
A total of twenty-seven healthy subjects were enrolled in this study, they were genotyped for ABCG2 genotype, and ABCB1. The observed frequencies of ABCG2 and ABCB1 genetic polymorphisms in the subjects and their demographic data are presented in Table 1. The genotype groups were compared by the performance of an analysis of covariance with an effective term for both ABCG2 and ABCB1 genotypes and with demographic data including age, body weight, and height as covariates. However, the interactions between genotype and each of the covariates were not statistically significant.
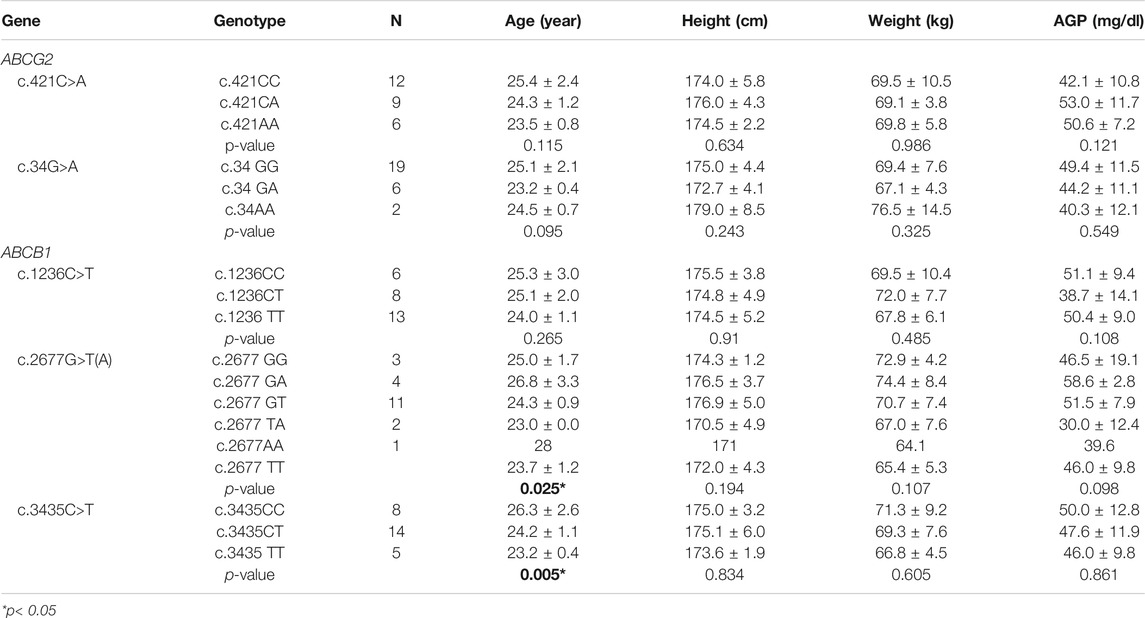
TABLE 1. Genotype frequencies of ABCG2 and ABCB1 genetic polymorphisms in 27 Korean subjects and demographic data.
Effects of Polymorphic ABCG2 and ABCB1 Genotypes on Imatinib Pharmacokinetics
Based on our study results ABCG2 genotypes, c.421C>A and c.34C>T polymorphism did not influence imatinib pharmacokinetics. ABCG2 c.421C>A, exhibited a gene-dose dependent trend on imatinib pharmacokinetics marginally (CL/F, p = 0.049, Table 2). Similarly, ABCB1 genotypes, c.1236C>T, c.2677G>T/A, and c.3435C>T polymorphisms did not show influence on the pharmacokinetic parameters of imatinib in this study population (Table 2).
Effects of AGP Levels on Imatinib Pharmacokinetics
Plasma AGP levels exhibited a strong correlation with the pharmacokinetic parameters of imatinib. In particular, plasma AGP levels exhibited a significant positive correlation with Cmax (Pearson r = 0.764, p < 0.0001) and AUCinf (Pearson r = 0.872, p < 0.0001) and a significant and negative correlation with CL/F (Pearson r = −0.667, p < 0.001) (Figure 1; Table 3). No association was found with Tmax or half-life.
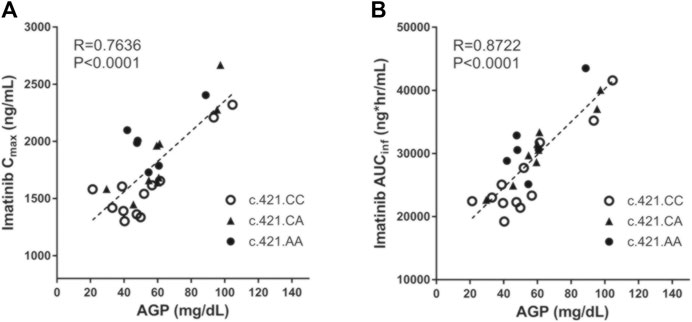
FIGURE 1. The correlation between individuals’ AGP level and their pharmacokinetic parameters (Cmax and AUCinf) with respect to ABCG2 c.421C>A genotype.
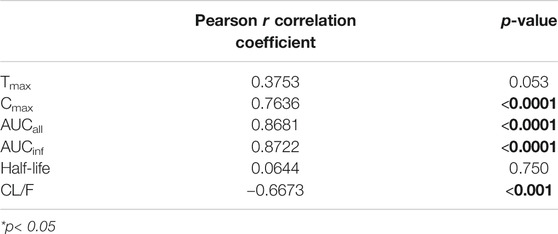
TABLE 3. Correlation between individual alpha-1-acid glycoprotein (AGP) level and the respective pharmacokinetic parameters of imatinib.
Effects of Polymorphic ABCB1 and ABCG2 Genotypes Based on the Stratified AGP Levels
An evaluation of the plasma AGP distribution revealed a bimodal distribution with an antimode at 80 mg/dl (Figure 2). We, therefore, re-evaluated the imatinib pharmacokinetics with respect to the ABCB1 and ABCG2 polymorphisms after the exclusion of five subjects with AGP values above the antimode (≥80 mg/dl, n = 5). After this adjustment, ABCG2 c.421C>A substantially influenced imatinib pharmacokinetics in this population: the ABCG2 c.421C>A genotype increased the imatinib Cmax values to 14,801.7 ng/ml for c.421CC, 1708.1 ng/ml for c.421CA, and 1921.4 ng/ml for c.421AA (p < 0.001). In addition, the average AUCinf value was 23,835.6 ng h/ml for c.421CC, 28,764.3 ng h/ml for c.421CA, and 29,605.3 ng h/ml for c.421AA (p = 0.008) and their average CL/F values were 17.1 L/h, 14.1 L/h, and 13.6 L/h, respectively (p = 0.007), while no association was found in Tmax, and CL/F (Table 4; Figure 3, Supplementary Figure S1). We could not find any meaningful results in ABCG2 c.34G>A.
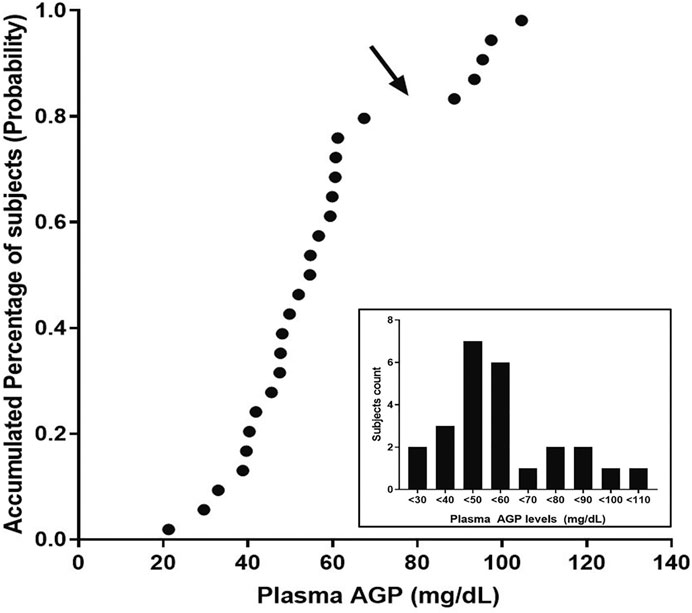
FIGURE 2. Probability plot for the plasma AGP levels observed in this study. The arrow points at the antimode, which determines normal and higher AGP levels in this population. The inset shows the distribution of the observed AGP levels in the population.
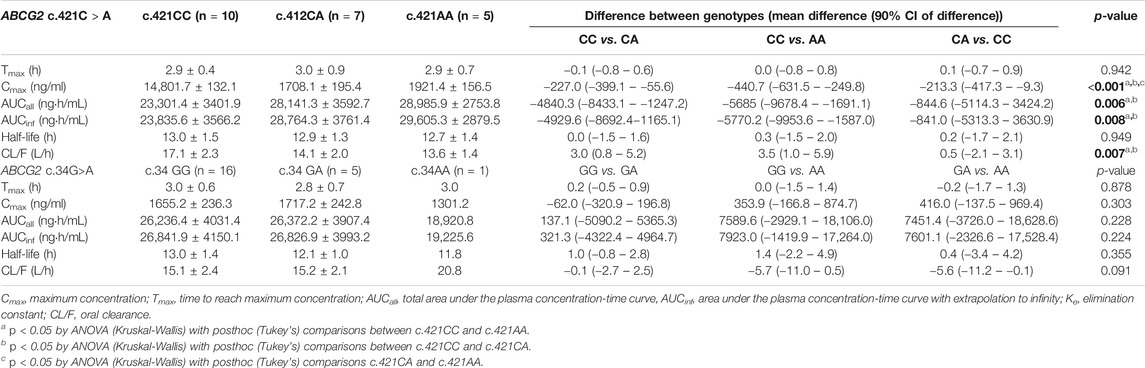
TABLE 4. Comparison of pharmacokinetic parameters of imatinib by ABCG2 genotypes in lower AGP levels (<80 mg/dl, n = 22).
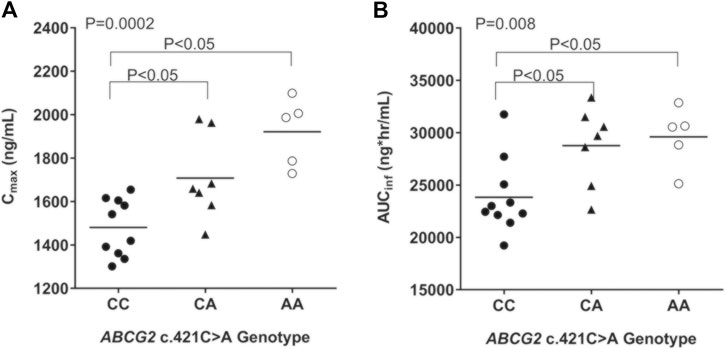
FIGURE 3. Comparison of pharmacokinetic parameters [Cmax (A) and AUCinf (B)] by ABCG2 c.421C>A genotype in subjects with plasma AGP less than 80 mg/dl. ANOVA (Kruskal-Wallis test) with posthoc comparisons (Tukey's) were performed.
Discussion
The results of the present study indicated that ABCG2 c.421C>A single nucleotide polymorphism significantly influenced imatinib pharmacokinetics in relatively low AGP plasma levels. However, neither the ABCG2 nor the ABCB1 polymorphism independently affected imatinib pharmacokinetics suggesting that the plasma AGP level was an important factor in the interindividual variability of imatinib pharmacokinetics.
The results of the present study showed a strong correlation between plasma AGP levels, and imatinib exposure and disposition, this was in agreement with previous literature (Widmer et al., 2006; Petain et al., 2008; Haouala et al., 2013), suggesting that the plasma AGP level was an important factor in the imatinib pharmacokinetics (Huang and Ung, 2013).
Based on our present study results, ABCG2 c.34G>A polymorphism did not affect imatinib pharmacokinetics, whereas the ABCG2 c.421C>A genotype significantly influenced the imatinib CL/F (p = 0.049), and showed a gene-dose dependent effect on the imatinib Cmax and AUC values in the low AGP plasma levels group. Moreover, the participants who had more than one SNPs causing loss of function of ABCG2 and/or ABCB1 (not including ABCG2 c.421C>A) did not show any tendency to affect imatinib pharmacokinetics, while the participants who only had ABCG2 c.421C>A mutation with no other additional SNPs were shown to have a more discriminative effect (data not shown). These results suggest that ABCG2 c.421C>A is a moderate factor in the modulation of imatinib pharmacokinetics. Although previous studies showed similar results regarding the effect of ABCG2 c.421C>A on the imatinib pharmacokinetics (Breedveld et al., 2005), some studies showed a significant difference in imatinib pharmacokinetics only in the dominant model (i.e., c.421 CC + CA vs. AA) or a gene-dose dependent effect on imatinib pharmacokinetics with no statistical significance (Petain et al., 2008; Takahashi et al., 2010; Seong et al., 2013). Given the discrepancies between the results of previous studies and those of the present study, we can postulate, a priori, that other factors may mask the effect of the ABCG2, including plasma AGP levels and demographic characteristics (Takahashi and Miura, 2011). However, in this study, the effects of age, body weight, and BMI on imatinib pharmacokinetics were unlikely to play a substantial role. Similarly, previous studies did not show any relationship with demography (Larson et al., 2008; Takahashi and Miura, 2011). When we analyzed plasma AGP distribution, the probability plot showed a bimodal distribution. Considering the strong positive correlation between imatinib pharmacokinetics and plasma AGP levels, we hypothesized that higher plasma AGP levels concealed the genetic effects on imatinib pharmacokinetics. Interestingly, ABCG2 c.421C>A genotypes substantially influenced imatinib pharmacokinetics in subjects with plasma AGP levels below 80 mg/dl, while other genotypes did not. This phenomenon suggests that plasma AGP levels and ABCG2 simultaneously influence imatinib pharmacokinetics, but that the role of ABCG2 might be masked in subjects with higher plasma AGP levels. We supposed that a low concentration of AGP contributed to a relatively increased fraction of free plasma imatinib levels, and thereby more easily affected by the functional ABCG2 polymorphism (Bohnert and Gan, 2013).
It has been suggested that imatinib is a substrate of P-glycoprotein expressed by ABCB1 (Gurney et al., 2007; Vivona et al., 2012). ABCB1 genetic polymorphisms, including c.1236C>T, c.2677G>T/A, and c.3435C>T, influence its disposition and clinical response to various ABCB1 substrates; in addition, it has been suggested that these polymorphisms modulate the plasma levels of imatinib as a substrate of P-glycoprotein (Burger and Nooter, 2004; Gurney et al., 2007; Vivona et al., 2012). However, a previous meta-analysis showed that neither ABCB1 c.2677G>T(A) nor c.3435C>T was a risk factor for poor clinical response of imatinib treatment in Asian CML patients, whereas c.1236C>T was a risk factor in Asian but not Caucasian CML patients (Zu et al., 2014; Zheng et al., 2015). In the present study evaluation of the effects of polymorphisms on imatinib pharmacokinetics showed no statistically significant effects on imatinib disposition. Similarly, other studies concluded that none of these polymorphisms influenced imatinib pharmacokinetics (Petain et al., 2008; Seong et al., 2013); Dickens et al. revealed that the SNPs did not affect the transporter activity of human P-glycoprotein in vitro (Dickens et al., 2013). We, therefore, suggested that ABCB1 polymorphisms might play a minor role in the disposition of imatinib.
Our study has some limitations. First, we assessed the pharmacokinetics of imatinib in healthy subjects with various ABCG2 and ABCB1 genotypes after a single dose of imatinib, even though imatinib is a long-term anticancer drug. However, to assess the pharmacogenetic effects only, we recruited a relatively homogenous group without other confounding factors that could affect imatinib pharmacokinetics (Takahashi and Miura, 2011). Secondly, while it has been suggested that various cytochrome P450 isoforms (CYP3A4/5, CYP1A2, CYP2C9, and CYP2C19) are involved in imatinib disposition (Weiner et al., 2007), in this study, we only assessed the pharmacogenetic effects of ABCG2 and ABCB1 polymorphisms. Although we did not assess the effect of polymorphisms of these genes, recently published findings have demonstrated their minor contributions (Gardner et al., 2006; Gurney et al., 2007). Another limitation of this study is the low sample size, which was inevitable to perform a thorough pharmacokinetic study design.
In conclusion, we observed that plasma AGP levels were strongly correlated with imatinib pharmacokinetics, and ABCB1 polymorphisms did not influence imatinib pharmacokinetics. However, the ABCG2 c.421C>A polymorphism plays a substantial role in imatinib pharmacokinetics in subjects with low plasma AGP levels; this implied that its effect on the imatinib pharmacogenetics was masked at higher AGP plasma levels.
Data Availability Statement
The data analyzed in this study is subject to the following licenses/restrictions: The datasets presented in this article are not readily available to protect participants’ privacy. Requests to access the datasets should be directed to JP. Requests to access these datasets should be directed to JP, anlwYXJrMjFAa29yZWEuYWMua3I=.
Ethics Statement
The studies involving human participants were reviewed and approved by the Institutional Review Board (IRB) of Anam Hospital, Korea University, Korea. The patients/participants provided their written informed consent to participate in this study.
Author Contributions
J-WP and HC equally contributed to this work as first authors. K-AK and SL designed research. J-MK and I-HP wrote the manuscript. J-YP is a corresponding author.
Conflict of Interest
The authors declare that the research was conducted in the absence of any commercial or financial relationships that could be construed as a potential conflict of interest.
Supplementary Material
The Supplementary Material for this article can be found online at: https://www.frontiersin.org/articles/10.3389/fphar.2021.658039/full#supplementary-material.
References
Bhattacharya, S. (2020). Fabrication and Characterization of Chitosan-Based Polymeric Nanoparticles of Imatinib for Colorectal Cancer Targeting Application. Int. J. Biol. Macromolecules 151, 104–115. doi:10.1016/j.ijbiomac.2020.02.151
Bohnert, T., and Gan, L.-S. (2013). Plasma Protein Binding: from Discovery to Development. J. Pharm. Sci. 102, 2953–2994. doi:10.1002/jps.23614
Breedveld, P., Pluim, D., Cipriani, G., Wielinga, P., Van Tellingen, O., Schinkel, A. H., et al. (2005). The Effect of Bcrp1 (Abcg2) on the In Vivo Pharmacokinetics and Brain Penetration of Imatinib Mesylate (Gleevec): Implications for the Use of Breast Cancer Resistance Protein and P-Glycoprotein Inhibitors to Enable the Brain Penetration of Imatinib in Patients. Cancer Res. 65, 2577–2582. doi:10.1158/0008-5472.can-04-2416
Burger, H., Van Tol, H., Boersma, A. W. M., Brok, M., Wiemer, E. A. C., Stoter, G., et al. (2004). Imatinib Mesylate (STI571) Is a Substrate for the Breast Cancer Resistance Protein (BCRP)/ABCG2 Drug Pump. Blood 104, 2940–2942. doi:10.1182/blood-2004-04-1398
Burger, K., and Nooter, K. (2004). Pharmacokinetic Resistance to Imatinib Mesylate: Role of the ABC Drug Pumps ABCG2 (BCRP) and ABCB1 (MDR1) in the Oral Bioavailability of Imatinib. Cell Cycle 3, 1502–1505. doi:10.4161/cc.3.12.1331
Capdeville, R., Buchdunger, E., Zimmermann, J., and Matter, A. (2002). Glivec (STI571, Imatinib), a Rationally Developed, Targeted Anticancer Drug. Nat. Rev. Drug Discov. 1, 493–502. doi:10.1038/nrd839
Demetri, G. D., Von Mehren, M., Blanke, C. D., Van Den Abbeele, A. D., Eisenberg, B., Roberts, P. J., et al. (2002). Efficacy and Safety of Imatinib Mesylate in Advanced Gastrointestinal Stromal Tumors. N. Engl. J. Med. 347, 472–480. doi:10.1056/nejmoa020461
Demetri, G. D., Wang, Y., Wehrle, E., Racine, A., Nikolova, Z., Blanke, C. D., et al. (2009). Imatinib Plasma Levels Are Correlated with Clinical Benefit in Patients with Unresectable/metastatic Gastrointestinal Stromal Tumors. Jco 27, 3141–3147. doi:10.1200/jco.2008.20.4818
Dickens, D., Owen, A., Alfirevic, A., and Pirmohamed, M. (2013). ABCB1 Single Nucleotide Polymorphisms (1236C>T, 2677G>T, and 3435C>T) Do Not Affect Transport Activity of Human P-Glycoprotein. Pharmacogenet Genomics 23, 314–323. doi:10.1097/fpc.0b013e328360d10c
Eechoute, K., Sparreboom, A., Burger, H., Franke, R. M., Schiavon, G., Verweij, J., et al. (2011). Drug Transporters and Imatinib Treatment: Implications for Clinical Practice. Clin. Cancer Res. 17, 406–415. doi:10.1158/1078-0432.ccr-10-2250
Gambacorti-Passerini, C., Zucchetti, M., Russo, D., Frapolli, R., Verga, M., Bungaro, S., et al. (2003). Alpha1 Acid Glycoprotein Binds to Imatinib (STI571) and Substantially Alters its Pharmacokinetics in Chronic Myeloid Leukemia Patients. Clin. Cancer Res. 9, 625–632.
Gardner, E., Burger, H., Vanschaik, R., Vanoosterom, A., Debruijn, E., Guetens, G., et al. (2006). Association of Enzyme and Transporter Genotypes with the Pharmacokinetics of Imatinib. Clin. Pharmacol. Ther. 80, 192–201. doi:10.1016/j.clpt.2006.05.003
Gschwind, H.-P., Pfaar, U., Waldmeier, F., Zollinger, M., Sayer, C., Zbinden, P., et al. (2005). Metabolism and Disposition of Imatinib Mesylate in Healthy Volunteers. Drug Metab. Dispos 33, 1503–1512. doi:10.1124/dmd.105.004283
Gurney, H., Wong, M., Balleine, R. L., Rivory, L. P., Mclachlan, A. J., Hoskins, J. M., et al. (2007). Imatinib Disposition and ABCB1 (MDR1, P-Glycoprotein) Genotype. Clin. Pharmacol. Ther. 82, 33–40. doi:10.1038/sj.clpt.6100201
Haouala, A., Widmer, N., Guidi, M., Montemurro, M., Leyvraz, S., Buclin, T., et al. (2013). Prediction of Free Imatinib Concentrations Based on Total Plasma Concentrations in Patients with Gastrointestinal Stromal Tumours. Br. J. Clin. Pharmacol. 75, 1007–1018. doi:10.1111/j.1365-2125.2012.04422.x
Haufroid, V. (2011). Genetic Polymorphisms of ATP-Binding Cassette Transporters ABCB1 and ABCC2 and Their Impact on Drug Disposition. Cdt 12, 631–646. doi:10.2174/138945011795378487
Huang, Z., and Ung, T. (2013). Effect of Alpha-1-Acid Glycoprotein Binding on Pharmacokinetics and Pharmacodynamics. Curr. Drug Metab. 14, 226–238. doi:10.2174/138920013804870709
Kim, K.-A., Cha, Y.-J., Lee, H.-M., Joo, H.-J., and Park, J.-Y. (2015). Effects of the ABCG2 and ABCB1 Drug Transporter Polymorphisms on the Pharmacokinetics of Bicalutamide in Humans. Clinica Chim. Acta 438, 7–11. doi:10.1016/j.cca.2014.08.006
Kim, K.-A., Joo, H.-J., and Park, J.-Y. (2010). ABCG2 Polymorphisms, 34G>A and 421C>A in a Korean Population: Analysis and a Comprehensive Comparison with Other Populations. J. Clin. Pharm. Ther. 35, 705–712. doi:10.1111/j.1365-2710.2009.01127.x
Kim, K.-A., Joo, H.-J., and Park, J.-Y. (2011a). Effect of ABCG2 Genotypes on the Pharmacokinetics of A771726, an Active Metabolite of Prodrug Leflunomide, and Association of A771726 Exposure with Serum Uric Acid Level. Eur. J. Clin. Pharmacol. 67, 129–134. doi:10.1007/s00228-010-0916-0
Kim, K.-A., Lim, J. L., Kim, C., and Park, J.-Y. (2011b). Pharmacokinetic Comparison of Orally Disintegrating and Conventional Donepezil Formulations in Healthy Korean Male Subjects: a Single-Dose, Randomized, Open-Label, 2-sequence, 2-period Crossover Study. Clin. Ther. 33, 965–972. doi:10.1016/j.clinthera.2011.06.003
Kim, K.-A., Park, P.-W., and Park, J.-Y. (2007). Effect of ABCB1 (MDR1) Haplotypes Derived from G2677T/C3435T on the Pharmacokinetics of Amlodipine in Healthy Subjects. Br. J. Clin. Pharmacol. 63, 53–58. doi:10.1111/j.1365-2125.2006.02733.x
Larson, R. A., Druker, B. J., Guilhot, F., O'brien, S. G., Riviere, G. J., Krahnke, T., et al. (2008). Imatinib Pharmacokinetics and its Correlation with Response and Safety in Chronic-phase Chronic Myeloid Leukemia: a Subanalysis of the IRIS Study. Blood 111, 4022–4028. doi:10.1182/blood-2007-10-116475
Nebot, N., Crettol, S., D'esposito, F., Tattam, B., Hibbs, D. E., and Murray, M. (2010). Participation of CYP2C8 and CYP3A4 in the N-Demethylation of Imatinib in Human Hepatic Microsomes. Br. J. Pharmacol. 161, 1059–1069. doi:10.1111/j.1476-5381.2010.00946.x
Oostendorp, R. L., Buckle, T., Beijnen, J. H., Van Tellingen, O., and Schellens, J. H. M. (2009). The Effect of P-Gp (Mdr1a/1b), BCRP (Bcrp1) and P-Gp/BCRP Inhibitors on the In Vivo Absorption, Distribution, Metabolism and Excretion of Imatinib. Invest. New Drugs 27, 31–40. doi:10.1007/s10637-008-9138-z
Peng, B., Dutreix, C., Mehring, G., Hayes, M. J., Ben-Am, M., Seiberling, M., et al. (2004). Absolute Bioavailability of Imatinib (Glivec) Orally versus Intravenous Infusion. J. Clin. Pharmacol. 44, 158–162. doi:10.1177/0091270003262101
Peng, B., Lloyd, P., and Schran, H. (2005). Clinical Pharmacokinetics of Imatinib. Clin. Pharmacokinet. 44, 879–894. doi:10.2165/00003088-200544090-00001
Petain, A., Kattygnarath, D., Azard, J., Chatelut, E., Delbaldo, C., Geoerger, B., et al. (2008). Innovative Therapies with Children with Cancer European, C.Population Pharmacokinetics and Pharmacogenetics of Imatinib in Children and Adults. Clin. Cancer Res. 14, 7102–7109. doi:10.1158/1078-0432.ccr-08-0950
Picard, S., Titier, K., Etienne, G., Teilhet, E., Ducint, D., Bernard, M.-A., et al. (2007). Trough Imatinib Plasma Levels Are Associated with Both Cytogenetic and Molecular Responses to Standard-Dose Imatinib in Chronic Myeloid Leukemia. Blood 109, 3496–3499. doi:10.1182/blood-2006-07-036012
Seong, S. J., Lim, M., Sohn, S. K., Moon, J. H., Oh, S.-J., Kim, B. S., et al. (2013). Influence of Enzyme and Transporter Polymorphisms on Trough Imatinib Concentration and Clinical Response in Chronic Myeloid Leukemia Patients. Ann. Oncol. 24, 756–760. doi:10.1093/annonc/mds532
Silverton, L., Dean, M., and Moitra, K. (2011). Variation and Evolution of the ABC Transporter Genes ABCB1, ABCC1, ABCG2, ABCG5 and ABCG8: Implication for Pharmacogenetics and Disease. Drug Metabol Drug Interact 26, 169–179. doi:10.1515/dmdi.2011.027
Takahashi, N., Miura, M., Scott, S. A., Kagaya, H., Kameoka, Y., Tagawa, H., et al. (2010). Influence of CYP3A5 and Drug Transporter Polymorphisms on Imatinib Trough Concentration and Clinical Response Among Patients with Chronic Phase Chronic Myeloid Leukemia. J. Hum. Genet. 55, 731–737. doi:10.1038/jhg.2010.98
Takahashi, N., and Miura, M. (2011). Therapeutic Drug Monitoring of Imatinib for Chronic Myeloid Leukemia Patients in the Chronic Phase. Pharmacology 87, 241–248. doi:10.1159/000324900
Vivona, D., Bueno, C. T., Lima, L. T., Hirata, R. D. C., Hirata, M. H., Luchessi, A. D., et al. (2012). ABCB1 Haplotype Is Associated with Major Molecular Response in Chronic Myeloid Leukemia Patients Treated with Standard-Dose of Imatinib. Blood Cell Mol. Dis. 48, 132–136. doi:10.1016/j.bcmd.2011.11.001
Weiner, M., Burman, W., Luo, C.-C., Peloquin, C. A., Engle, M., Goldberg, S., et al. (2007). Effects of Rifampin and Multidrug Resistance Gene Polymorphism on Concentrations of Moxifloxacin. Aac 51, 2861–2866. doi:10.1128/aac.01621-06
Widmer, N., Decosterd, L. A., Csajka, C., Leyvraz, S., Duchosal, M. A., Rosselet, A., et al. (2006). Population Pharmacokinetics of Imatinib and the Role of Alpha1-Acid Glycoprotein. Br. J. Clin. Pharmacol. 62, 97–112. doi:10.1111/j.1365-2125.2006.02719.x
Zheng, Q., Wu, H., Yu, Q., Kim, D. H., Lipton, J. H., Angelini, S., et al. (2015). ABCB1 Polymorphisms Predict Imatinib Response in Chronic Myeloid Leukemia Patients: a Systematic Review and Meta-Analysis. Pharmacogenomics J. 15, 127–134. doi:10.1038/tpj.2014.54
Keywords: imatinib (gleevec), glivec, STI-571, alpha-1 acid glycoprotein, ABCG2 (BCRP), genetic polymorphism
Citation: Park J-W, Chung H, Kim K-A, Kim J-M, Park I-H, Lee S and Park J-Y (2021) ABCG2 Single Nucleotide Polymorphism Affects Imatinib Pharmacokinetics in Lower Alpha-1-Acid Glycoprotein Levels in Humans. Front. Pharmacol. 12:658039. doi: 10.3389/fphar.2021.658039
Received: 25 January 2021; Accepted: 06 April 2021;
Published: 29 April 2021.
Edited by:
Sabina Passamonti, University of Trieste, ItalyCopyright © 2021 Park, Chung, Kim, Kim, Park, Lee and Park. This is an open-access article distributed under the terms of the Creative Commons Attribution License (CC BY). The use, distribution or reproduction in other forums is permitted, provided the original author(s) and the copyright owner(s) are credited and that the original publication in this journal is cited, in accordance with accepted academic practice. No use, distribution or reproduction is permitted which does not comply with these terms.
*Correspondence: Ji-Young Park, anlwYXJrMjFAa29yZWEuYWMua3I=
†These authors have contributed equally to this work and share first authorship