- Department of Cardiology, The First Affiliated Hospital of Chongqing Medical University, Chongqing, China
Background: We performed a meta-analysis to evaluate the efficacy and safety of pulmonary vasodilators in pediatric pulmonary hypertension (PH) patients.
Methods: We searched electronic databases including PubMed, EMBASE, and the Cochrane Library up to May 2020, and conducted a subgroup analysis for pulmonary vasodilators or underlying disease.
Results: Fifteen studies with 719 pediatric PH patients were included in the meta-analysis. Adverse events did not differ (p = 0.11, I2 = 15%) between the pulmonary vasodilators group and the control group, neither in the subgroups. In total, compared with the control group treatment, pulmonary vasodilators significantly decreased the mortality (p = 0.002), mean pulmonary artery pressure (mPAP, p = 0.02), and mechanical ventilation duration (p = 0.03), also improved the oxygenation index (OI, p = 0.01). In the persistent pulmonary hypertension of the newborn (PPHN) subgroup, phosphodiesterase type 5 inhibitors (PDE5i) significantly reduced mortality (p = 0.03), OI (p = 0.007) and mechanical ventilation duration (p = 0.004). Administration of endothelin receptor antagonists (ERAs) improved OI (p = 0.04) and mechanical ventilation duration (p < 0.00001) in PPHN. We also found that in the pediatric pulmonary arterial hypertension (PPAH) subgroup, mPAP was pronouncedly declined with ERAs (p = 0.006). Systolic pulmonary artery pressure (sPAP, p < 0.0001) and pulmonary arterial/aortic pressure (PA/AO, p < 0.00001) were significantly relieved with PDE5i, partial pressure of arterial oxygen (PaO2) was improved with prostacyclin in postoperative PH (POPH) subgroup (p = 0.001). Compared with the control group, pulmonary vasodilators could significantly decrease PA/AO pressure (p < 0.00001) and OI (p < 0.00001) in the short-term (duration <7 days) follow-up subgroup, improve mPAP (p = 0.03) and PaO2 (p = 0.01) in the mid-term (7–30 days) follow-up subgroup, also decrease mortality, mPAP (p = 0.0001), PA/AO pressure (p = 0.0007), duration of mechanical ventilation (p = 0.004), and ICU stay (p < 0.00001) in the long-term follow subgroup (>30 days).
Conclusion: Pulmonary vasodilators decrease the mortality in pediatric PH patients, improve the respiratory and hemodynamic parameters, reduce the mechanical ventilation duration.
Introduction
Pediatric pulmonary hypertension (PH) is associated with severe morbidity and mortality (Berger et al., 2011; Frantz et al., 2013; Hoeper et al., 2013). Similar to adult PH, the definition of pediatric PH was updated in The Pediatric Task Force of the 6th World Symposium on Pulmonary Hypertension (WSPH) in Nice, France (2018): mean pulmonary artery pressure (mPAP) > 20 mmHg, normal pulmonary capillary wedge pressure (mPCWP) ≤ 15 mmHg, and pulmonary vascular resistance (PVR) > 3 WU (ten Harkel et al., 2019). According to the nationwide Netherlands PH Service (Berger et al., 2011) the yearly incidence rate for all PH diagnoses is 63.7 cases per million children. Persistent pulmonary hypertension of the newborn (PPHN) and cardiogenic PH had the highest incidence rates: 30.1 and 21.9 cases per million children, respectively. Progressive PH had an annual incidence rate of 3.0 cases per million children. For idiopathic pulmonary artery hypertension (iPAH) and PH associated with congenital heart disease (PH-CHD), the incidence was even lower: 0.7 and 2.2 cases per million. In addition, the subgroup of patients with postoperative PH (POPH) following CHD repair occurs in 21.9 cases per million and is one of the most common forms of PAH in children (Frantz et al., 2013; Hoeper et al., 2013).
There are three main options to treat PH in the clinic: endothelin, endothelin receptor antagonist, and prostacyclin (Galiè et al., 2015). Most treatment strategies for pediatric PH patients are based on adult clinical trial data and clinical experience. For example, Bosentan, as a dual endothelin receptor antagonist (ERA), which was approved by the FDA in September 2017 for pediatric use in patients over 3 years, exhibited positive efficacy in several pediatric PH trials (Gibbs and Ismail, 2012; PAN and KONG, 2016; Steinhorn et al., 2016). However, it has been reported that chronic use of bosentan may lead to elevated liver aminotransferases, also cause liver cirrhosis. Another drug, sildenafil can improve peak oxygen consumption, functional category, and hemodynamics in pediatric PH patients based on the double-blind, placebo-controlled Sildenafil in Treatment-naive Children, Aged 1–17 years, With Pulmonary Arterial Hypertension (STARTS-1) and Long-Term Survival With Oral Sildenafil Monotherapy in Treatment-Naive Pediatric Pulmonary Arterial Hypertension (STARTS-2) trials (Cornelisse et al., 2012; Szatmari et al., 2014). However, the STARTS-2 trial reported higher mortality in the high-dose sildenafil group (80 mg) (Szatmari et al., 2014). The FDA announced in 2014 that sildenafil could be considered when the benefits of this drug treatment may exceed its potential risk to each patient, but high-dose sildenafil needs to be warned (Layton and Bavdekar, 2015). The prostacyclin analog treprostinil was approved by FDA for adult PH patients in 2002, and it is also used in the clinic for pediatric patients who cannot be improved by other treatments and showed good efficacy (Rosenzweig et al., 1999). It is worth noting that the use of prostacyclin analogs may be associated with an increased risk of bleeding in patients (Wade et al., 2003). The choice of pulmonary vasodilators for different types of pediatric PH patients is currently controversial. Therefore, we conducted a meta-analysis to comprehensively evaluate the efficacy and safety of pulmonary vasodilators in pediatric patients with PH, and we also conducted a further subgroup analysis to compare the efficacy and safety of different subgroups of pulmonary vasodilators in the treatment of subgroups of pediatric PH.
Methods
Search Strategy and Data Collecting
This meta-analysis was performed in accordance with the Preferred Reporting Items for Systematic Reviews and Meta-Analyses (PRISMA) statement (Shamseer et al., 2015). The electronic databases included PubMed, EMBASE, and the Cochrane Library up to May of 2020, and the searches were conducted by Me-SH terms and keywords. The keywords were searched in various combinations using the Boolean operators “AND” and “OR”. Search expressions are shown in Supplementary Table S1. We manually searched clinical trial registries (clinicaltrials.gov) and the International Clinical Trials Registry Platform (www.whoint/ictrp/search/en/). We also searched the reference lists of all the selected articles and searched the studies published in peer-reviewed journals for additional related publications. Both the electronic search and manual search were performed independently by two authors (TT Shu and HQ Chen).
Study Inclusion and Exclusion Criteria
The inclusion criteria were as follows: 1) studies that were randomized controlled trials; 2) studies in which the participants comprised newborns, infants, and children (postnatal to 18 years) diagnosed with PPHN, POPH or PPAH (idiopathic PH, PH associated with connective tissue disease or congenital heart disease without repair surgery); 3) studies in which the diagnosis of PH was based on clinical findings with echocardiographic or right heart catheter confirmation; 4) studies comparison of mono-therapy with pulmonary vasodilators vs. placebo or no treatment; 5) the outcomes were reported in both pulmonary vasodilators group and control group. Animal therapy trials and preclinical trials were excluded. The duplicated studies were removed and the study with the largest sample size from the same institute was remained. Two independent researchers (TT Shu and HQ Chen) selected studies for inclusion by scanning the title, abstract, and full text.
Data Extraction and Quality Assessment
The following data were extracted: 1) study characteristics (first author, publication year, and participant sample size), 2) pharmacotherapy intervention, and 3) study results data. The review authors compared the results and resolved any discrepancies. One review author imported the extracted data into RevMan 5.3 software, and another author checked the imported data. If relevant articles were identified that provided insufficient data or did not provide relevant data for review, then the authors obtained the data from the authors of the relevant study by email.
The primary outcomes were defined as mortality and adverse events. Safety was evaluated by the adverse events. Adverse events were defined as headache, gastrointestinal upset (including nausea or vomiting), respiratory symptoms, abnormal hepatic function, hematological disorders (including anemia, red blood cell transfusion, thrombocytopenia, or epistaxis), and PH crisis. Secondary outcomes included the changes from baseline to follow-up: 1) respiratory and hemodynamic parameters, including oxygenation index (OI = fraction of inspired oxygen × mean airway pressure/arterial oxygen pressure), partial pressure of oxygen (PaO2), blood oxygen saturation (SpO2), mPAP, systolic PAP (sPAP), mean pulmonary arterial/aortic (PA/AO) pressures; 2) duration of mechanical ventilation; and 3) intensive care unit (ICU) stay (GalièGhersi et al., 2015).
Trials comparing the same type of pulmonary vasodilators but at different therapeutic doses (fixed or flexible-dose) and different treatment durations were grouped in the same node in the meta-analysis. The studies were classified into followed-up duration subgroups: short-term follow-up (<7 days), mid-term follow-up (7–30 days), long-term follow-up (>30 days). The included studies were analyzed using the RevMan 5.3 software, and we computed 95% confidence intervals (CIs) of mean differences (MDs) for the continuous outcome data and risk ratios (RRs) with a 95% CI for dichotomous outcome data. Values of p < 0.05 indicated statistical significance. The chi2 test was conducted on the research effect size to evaluate heterogeneity. We followed the CNRG recommendations by using the following criteria to describe the heterogeneity: < 25% no heterogeneity, 25–49% low heterogeneity, 50–74% moderate heterogeneity, and ≥75% high heterogeneity. When the research effect size was homogeneous, i.e., I2 greater than 50%, data were reanalyzed using a random-effects model, the Mantel-Haenszel method. If there was considerable bias, we explored the effect of bias by conducting a sensitivity analysis. The sensitivity analysis of results was performed by excluding low-quality studies or subgroups with different types of drugs or underlying diseases.
To select high-quality studies, we independently rated the quality of each retrieved study by the Cochrane Risk of Bias tool in the RevMan 5.3 software (Gibbs et al., 2011). The GRADE approach was utilized to assess the quality of evidence for the following outcomes: mortality, adverse events, respiratory and hemodynamic parameters (OI, PaO2, SpO2, mPAP, sPAP, PA/AO), mechanical ventilation duration and ICU stay. When the following five factors were included, the level of evidence was downgraded one level for serious or two levels for very serious limitations: risk of bias, consistency, directness, precision, and publication bias. According to the GRADE approach, the evidence was divided into four levels: high, moderate, low, and very low. The higher the level of evidence, the higher the confidence of this study in evaluating treatment effects.
Results
Study Characteristics
The search strategy revealed a total of 499 studies, of which fifteen studies with 719 pediatric PH patients met the inclusion criteria and were included in the analysis. The flow diagram of document selection for this analysis is shown in Supplementary Figure S2 . The follow-up duration ranged from 24 h to 15 months. The main characteristics of the included studies are shown in Table 1. Among the fifteen included studies, three had an ERA group and control group (Gibbs and Ismail, 2012; Pan and Kong, 2016; Steinhorn et al., 2016), nine had PDE5i and control groups (Juni et al., 2006; Wessel et al., 2006; Peiravian et al., 2007; Kolaee et al., 2009; Vargas-Origel et al., 2010; Fraisse et al., 2011; Uslu et al., 2011; Cornelisse et al., 2012; Sharma et al., 2015), and three had PGI2 treatment and control groups (Takahashi et al., 2003; Xu et al., 2015; Onan et al., 2016). Based on subgroups of pediatric PH patients, there were seven studies of PPHN (Juni et al., 2006; Wessel et al., 2006; Kolaee et al., 2009; Vargas-Origel et al., 2010; Uslu et al., 2011; Gibbs and Ismail, 2012; Steinhorn et al., 2016), two studies of PPAH (Cornelisse et al., 2012; PAN and KONG, 2016), and six studies of POPH (Takahashi et al., 2003; Peiravian et al., 2007; Fraisse et al., 2011; Sharma et al., 2015; Xu et al., 2015; Onan et al., 2016). A list of pulmonary vasodilator subgroups used in pediatric PH patients is shown in Table 2.
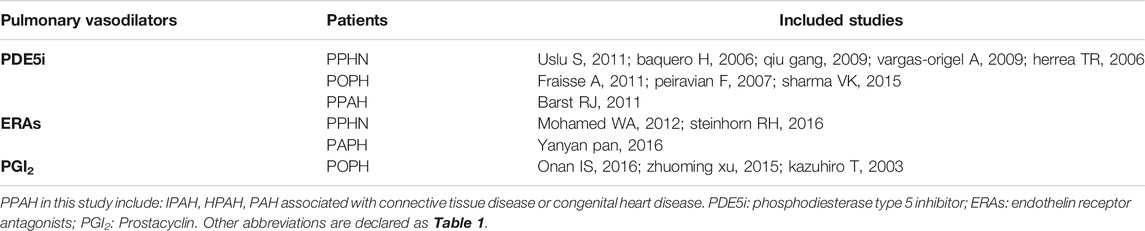
TABLE 2. List of studies on pulmonary vasodilators for the treatment of different types of pediatric pulmonary hypertension.
Risk of Bias in the Included Studies
After excluding low-quality studies, the included studies were multicenter, double-blind, placebo-controlled trials. In the evaluation of attrition bias, 7 studies reported high risks, which accounted for more than 25%. The high-risk proportions of the remaining various biases were all less than 25%. The included studies in the present study were high quality. The details and a summary of the risk of bias in the included studies are presented in Supplementary Figures S3,S4.
Overall Analysis of Pulmonary Vasodilators in Pediatric PH
Primary Outcomes
Fifteen studies with 703 patients evaluated pulmonary vasodilators and reported mortality. These studies showed that the mortality rate in the pulmonary vasodilator group (n = 423) was significantly lower (RR: 0.20; 95% CI: 0.07 to 0.56; p = 0.002; I2 = 0%; Figure 1) than that in the control group (n = 280), equating to an absolute risk difference of −0.03 (95% CI, −0.07 to 0.02). Eleven trials with 601 patients were evaluated adverse events and no significant difference was observed (RR: 0.63; 95% CI: 0.35 to 1.12; p = 0.11, I2 = 15%; Figure 1) between the pulmonary vasodilator group (n = 26) and the control group (n = 25), equating to an absolute risk difference of −0.02 (95% CI, −0.08 to 0.03). The incidence of headache (13.2 vs. 13.3%, p = 0.98, Figure 2), gastrointestinal upset (7.5 vs. 7.4%, p = 0.92), respiratory symptoms (10.6 vs. 6.3%, p = 0.37), abnormal hepatic function (0 vs. 0%, p = not applicable), hematological disorders (6.1 vs. 5.3%, p = 0.57), and PH crisis (11.4 vs. 11.8%, p = 0.90) in pulmonary vasodilator group was similar to the control group.
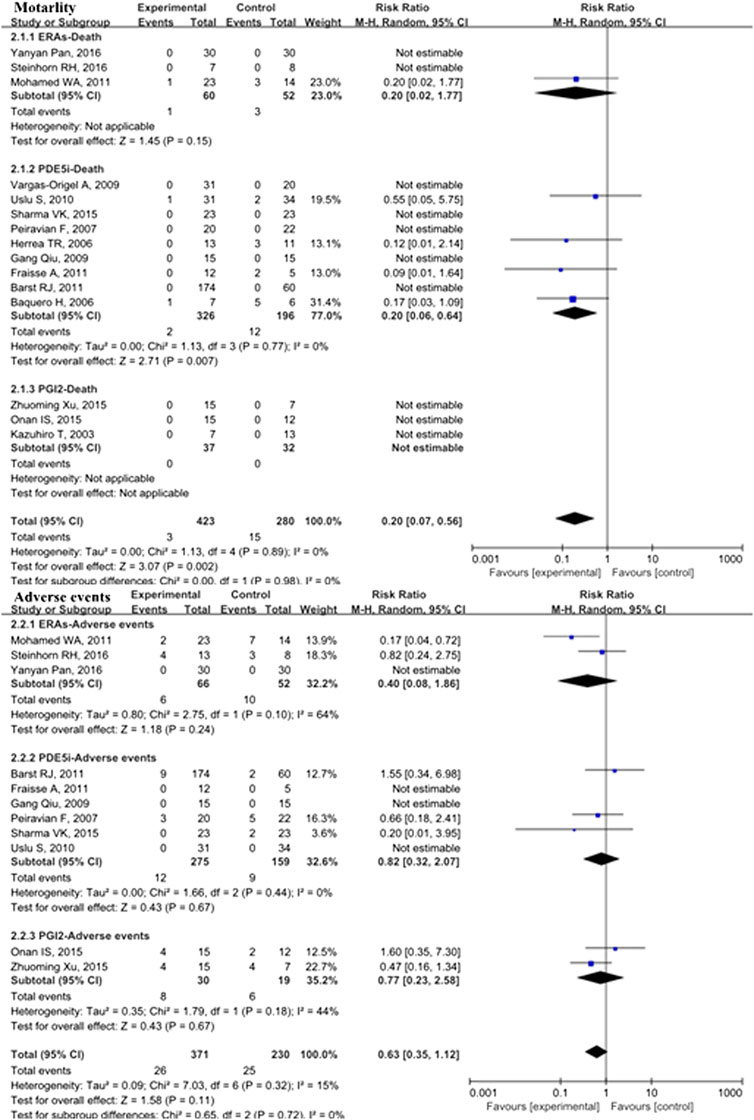
FIGURE 1. Mortality and adverse events in pediatric PH patients treated with pulmonary vasodilators. Abbreviations ERAs, endothelin receptor antagonists; PDE5i, phosphodiesterase type 5 inhibitors; PGI2, prostacyclins; PPHN, persistent pulmonary hypertension of the newborn; POPH, pediatric postoperative pulmonary hypertension; PPAH, pediatric pulmonary arterial hypertension.
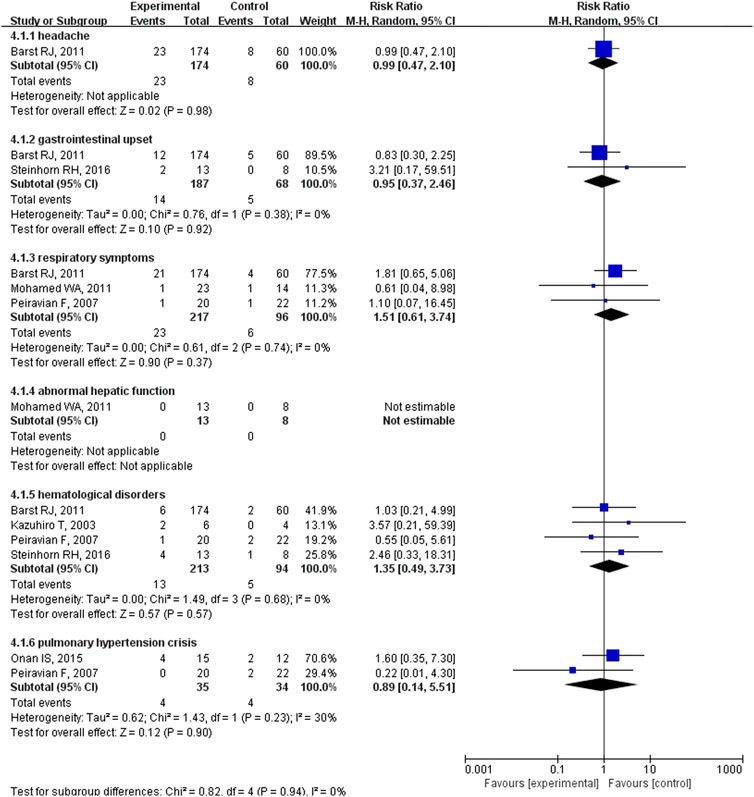
FIGURE 2. The incidence of adverse events in pediatric PH patients treated with pulmonary vasodilators comparing to the control group.
Secondary Outcomes
In the overall analysis of pediatric PH patients, OI (MD: −13.34; 95% CI: −23.89 to −2.80; p = 0.01; I2 = 98%; 5 trials with 176 patients; Figure 3), mPAP (MD: −3.35 mmHg; 95% CI: −6.24 to −0.47; p = 0.02; I2 = 58%; 8 trials with 462 patients; Figure 4), sPAP (MD: −11.19 mmHg; 95% CI: −16.50 to −5.88; p < 0.0001; I2 = 0%; 2 trials with 59 patients; Figure 4), PA/AO (MD: −0.11; 95% CI: −0.17 to −0.04; p < 0.0008; I2 = 53%; 4 trials with 137 patients; Figure 4), and mechanical ventilation duration (MD: −3.84 days; 95% CI: −7.28 to −0.41; p = 0.03; I2 = 99%; 7 trials with 295 patients; Figure 5) were significantly lower and PaO2 (MD: −8.42 mmHg; 95% CI: −11.88 to −4.96; p < 0.00001; I2 = 0%; 3 trials with 84 patients; Figure 2) was markedly higher in the pulmonary vasodilator group than in the control group. The analysis showed no significant differences in SpO2 change (p = 0.13; 4 trials with 105 patients; Figure 2) and ICU stay (p = 0.06; 4 trials with 132 patients; Figure 5) between the pulmonary vasodilator and control groups.
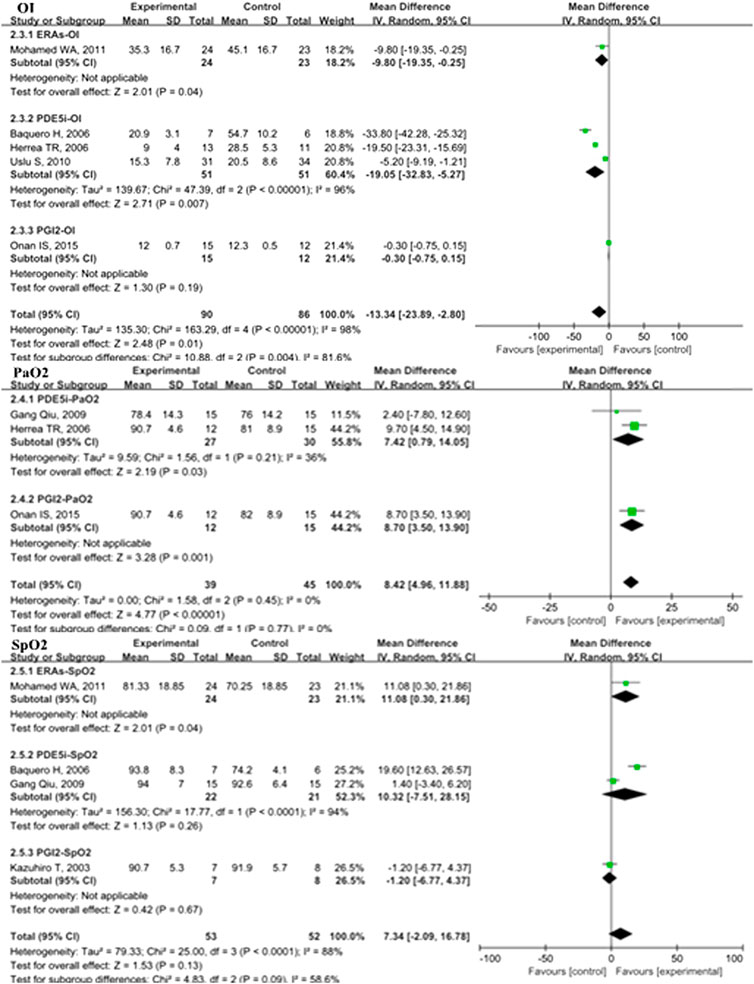
FIGURE 3. OI, PaO2, and SpO2 in pediatric PH patients treated with pulmonary vasodilators. Abbreviations: OI, oxygenation index; PaO2, partial pressure of arterial oxygen; SpO2, pulse oxygen saturation. Other abbreviations are defined in Figure 1.
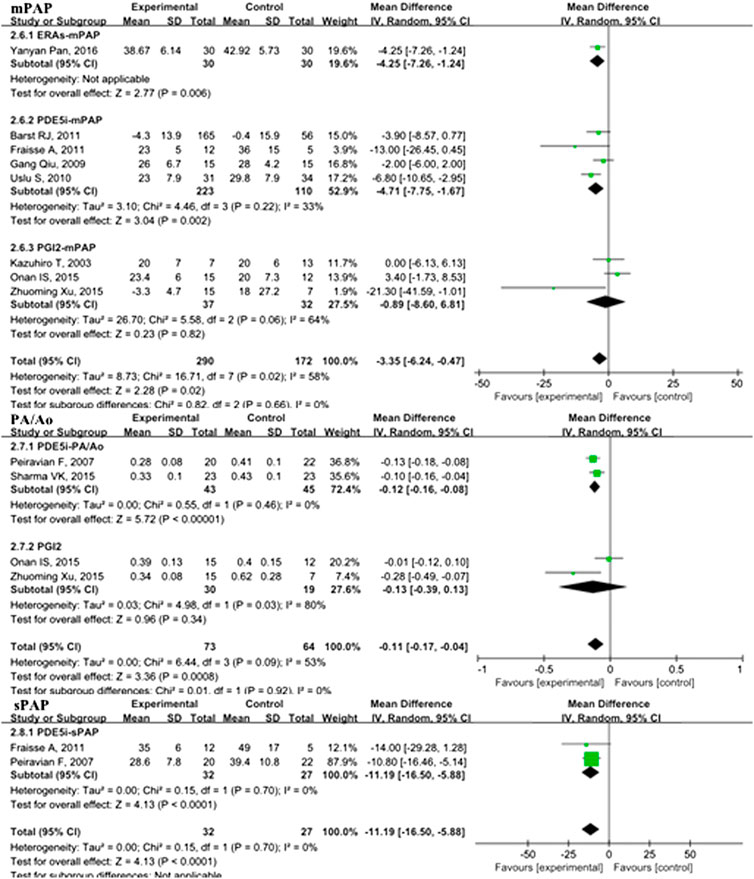
FIGURE 4. mPAP, PA/Ao, and sPAP in pediatric PH patients treated with pulmonary vasodilators. Abbreviations: mPAP, mean pulmonary artery pressure; PA, pulmonary artery; Ao, aorta; sPAP, systolic pulmonary artery pressure. Other abbreviations are defined in Figure 1.
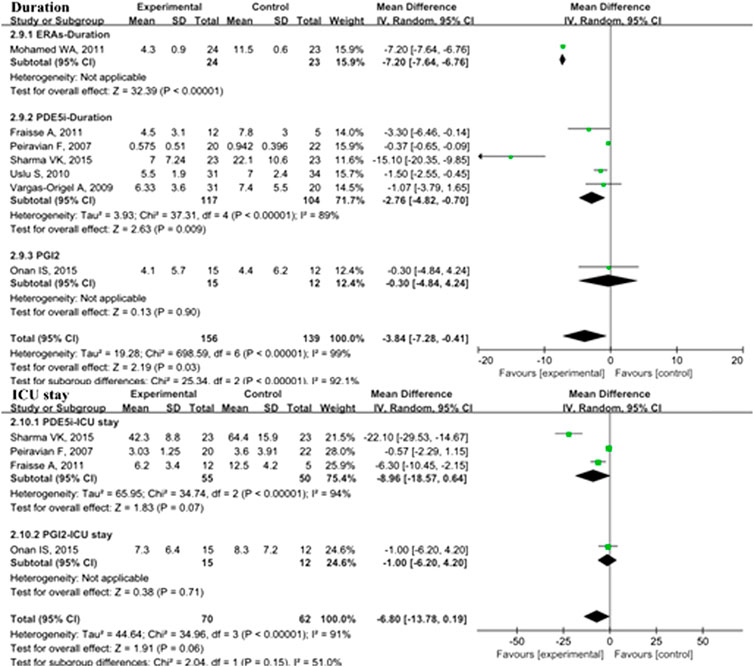
FIGURE 5. Duration of mechanical ventilation and ICU stay in pediatric PH patients treated with pulmonary vasodilators. Abbreviations: Duration, duration of mechanical ventilation; ICU, intensive care unit. Other abbreviations are defined in Figure 1.
This analysis showed no heterogeneity in mortality, adverse events, sPAP, and PaO2 (I2 < 25%); moderate heterogeneity in mPAP and PA/AO (50% < I2 < 75%); and high heterogeneity in OI and mechanical ventilation duration (I2 > 75%). Through sensitivity analysis, after removing the PGI2 subgroup, the heterogeneities of mPAP and PA/AO were reduced to the low level (MD: −4.49, p < 0.0001, I2 = 11%; MD: −0.12, p < 0.00001, I2 = 0%; respectively). After removing the PDE5i subgroup, the heterogeneity of OI was reduced to a moderate level but no statistical significance (MD: 0.38, p = 0.41, I2 = 74). The heterogeneity of mechanical ventilation duration was decreased to 87% after removing the ERAs subgroup but still a high level.
Subgroup Analysis of Pulmonary Vasodilators in Pediatric PH
Primary Outcomes
In the PDE5i subgroup, the incidence of mortality in pediatric PH patients treated with pulmonary vasodilators was significantly lower than that in the control group (RR: 0.20; 95% CI: 0.06 to 0.64; p = 0.007; n = 522; I2 = 0%; Figure 1), equating to an absolute risk difference of −0.05 (95% CI, −0.12 to 0.02). In the ERA subgroup, the mortality was not significantly different compared with the control group (n = 112). There were no deaths in the PGI2 subgroup (n = 69). Subgroup analyses comparing PDE5i, ERAs, and PGI2 with the control group treatment for pediatric PH showed no significant differences in adverse events.
Secondary Outcomes
In the subgroup analysis of the PDE5i group compared with the control, OI (MD: −19.05; 95% CI: −32.83 to −5.27; p = 0.007; I2 = 96%; n = 102; Figure 2), mPAP (MD: −4.71 mmHg; 95% CI: −7.75 to −1.67; p = 0.002; n = 333; I2 = 33%; Figure 4), sPAP (MD: −11.19 mmHg; 95% CI: −16.50 to −5.88; p < 0.0001; I2 = 0%; n = 59; Figure 4), PA/AO (p < 0.00001; n = 88; I2 = 0%; Figure 4), and mechanical ventilation duration (MD: −2.76 days; 95% CI: −4.82 to −0.70; p = 0.009; n = 221; I2 = 89%; Figure 5) were significantly decreased and PaO2 was increased (p = 0.03; I2 = 36%; n = 57; Figure 2). The ERA subgroup analysis showed that the OI (p = 0.04; n = 47; Figure 2), mPAP (p = 0.006; n = 60; Figure 4), and mechanical ventilation duration (p < 0.00001; n = 47; Figure 5) was significantly lower than in the control group. PaO2, sPAP, and PA/AO were not reported for the ERA group. In the PGI2 subgroup, compared with the control group, PaO2 was significantly improved (p = 0.001; n = 27; Figure 2) and there was no significant difference in OI (p = 0.19), mPAP (p = 0.82), PA/AO (p = 0.34), or mechanical ventilation duration (p = 0.90). Indicators of sPAP were not reported for the PGI2 subgroup. All subgroup analyses of the pulmonary vasodilators compared with the control group treatments showed no significant changes in SpO2 and ICU stay.
Subgroup Analysis of Pediatric PH With Different Pulmonary Vasodilators
Primary Outcomes
Five studies comparing PDE5i in PPHN patients (n = 183) showed significantly lower mortality in the vasodilator group (RR: 0.23; 95% CI: 0.06 to 0.83; p = 0.03; I2 = 0%; Supplementary Table S5 ) than in the control group, equating to an absolute risk difference of −0.11 (95% CI, −0.25 to 0.04). There was no death due to POPH (n = 69) in either the PGI2 group or the control group. Subgroup analyses of PPHN, POPH, and PPAH compared the treatment group with the control group, and none showed significant differences in adverse events.
Secondary Outcomes
The analysis of PPHN patient subgroups showed significantly lower OI with PDE5i (MD: −19.05; 95% CI: −32.83 to −5.27; p = 0.007; I2 = 96%; n = 102) and ERA treatment (p = 0.04; n = 47), shorter mechanical ventilation duration with PDE5i (MD: −1.44 days; 95% CI: −2.42 to −0.47; p = 0.004; I2 = 0%; n = 116) and ERA treatment (p < 0.00001; n = 47), and increased PaO2 with PDE5i treatment (p = 0.03; I2 = 36%; n = 57) than in the control treatment groups (S5 Table 2). Only one study with 60 PPAH patients showed that mPAP was significantly lower in the group treated with ERAs than in the control group (p = 0.006). In POPH patients, subgroup analyses showed that, compared with the placebo group, a significant improvement in PaO2 was observed with PGI2 treatment (p = 0.001; n = 27), sPAP with PDE5i (MD: −11.19; 95% CI: −16.50 to −5.88; p < 0.0001; n = 59), and PA/AO with PDE5i (p < 0.00001; I2 = 0%; n = 88).
Follow-Up Duration Subgroup Analysis
Primary Outcomes
There were four studies with 147 short-term follow-up patients, three studies with 66 mid-term follow-up patients, and eight studies with 506 patients with long-term follow-up duration. In the long-term subgroup analysis, the mortality of pediatric PH patients with pulmonary vasodilators was significantly decreased comparing with the control group (RR: 0.25; 95% CI: 0.07 to 0.82; p = 0.02; I2 = 0%; Supplementary Table S6), equating to an absolute risk difference of −0.03 (95% CI, −0.10 to 0.03). However, in short-term and mid-term subgroups, the difference in mortality was not significant between the pulmonary vasodilators group and the control group (p = 0.15, p = 0.10, respectively). The adverse events did not differ between the pulmonary vasodilators group and the control group in the follow-up duration subgroup analyses.
Secondary Outcomes
In the short-term subgroup, PA/AO pressure (p < 0.00001; S6 Table 3) and OI (p < 0.00001) were significantly decreased in the pulmonary vasodilators group. The improvements of mPAP (p = 0.03) and PaO2 (p = 0.01) were significant comparing the pulmonary vasodilators group with the control in the mid-term follow-up subgroup. The analysis in long-term subgroup showed that pulmonary vasodilators significantly decreased mPAP (p = 0.0001), PA/AO pressure (p = 0.0007), duration of mechanical ventilation (p = 0.004), and ICU stay (p < 0.00001).
Discussion
This is the first meta-analysis on the efficacy and safety of pulmonary vasodilators in pediatric PH with subgroups of pulmonary vasodilators and subgroups of patients. The present study demonstrates the clinical benefits of pulmonary vasodilators for the treatment of pediatric PH, including decreased mortality, improved hemodynamics (OI, PaO2, mPAP, sPAP, and PA/Ao), and shorter duration of mechanical ventilation compared with those of the control group.
Three pulmonary vasodilators were used to treat pediatric PH in the present study: PDE5i (sildenafil), ERAs (bosentan), and PGI2 (Iloprost or Beraprost). Sildenafil is a highly selective PDE5i that can enhance the concentration of cyclic guanosine monophosphate in pulmonary vascular smooth muscle cells by inhibiting PDE5 and promoting endogenous nitric oxide function (Archer and Michelakis, 2009). Sildenafil can also alleviate pulmonary vascular remodeling by inhibiting the proliferation of vascular smooth muscle cells (Layton and Bavdekar, 2015). Bosentan is a nonselective ERA with an affinity for ETA and ETB that can dilate pulmonary blood vessels (Barst et al., 2003). Iloprost and beraprost are analogs of PGI2, which can selectively dilate pulmonary blood vessels and inhibit proliferation and thrombotic effects (Cheng et al., 2002). All three categories of pulmonary vasodilators showed beneficial effects in pediatric PH patients. Sildenafil therapy showed a significant improvement in mortality in pediatric PH patients, but mortality in patients treated with ERAs and PGI2 was not significantly different from that in the control group. Besides, no deaths occurred in the PGI2 studies during follow-up, which also demonstrates the safety of PGI2. Compared with the previous studies in adults, adverse events in the present study for sildenafil were headache (34% in adult vs. 13% in pediatric patients), gastrointestinal upset (26% in adult vs. 11% in pediatric patients), respiratory symptoms (11% in adult vs. 12% in pediatric patients), and epistaxis (6% in adult vs. 3% in pediatric patients) (Peiravian et al., 2007; Cornelisse et al., 2012; Barnes et al., 2019); the adverse events for bosentan were headache (21% in adult vs. 0% in pediatric patients), respiratory symptoms (8% in adult vs. 9% in pediatric patients), abnormal hepatic function (9% in adult vs. 0% in pediatric patients), red blood cell transfusion (0% in adult vs. 31% in pediatric patients), and anemia (0% in adult vs. 23% in pediatric patients) (Rubin et al., 2002; Gibbs and Ismail, 2012; Steinhorn et al., 2016); and the adverse events for beraprost sodium and iloprost treatment were headache (53% in adult vs. 0% in pediatric patients), upper respiratory tract events (34% in adult vs. 0% in pediatric patients), nausea or vomiting (43% in adult vs. 0% in pediatric patients), PH crisis (6% in adult vs. 7% in pediatric patients), and thrombocytopenia (0% in adult vs. 27% in pediatric patients) (Xu et al., 2015; Onan et al., 2016; Pulido et al., 2019). All pediatric PH patients well tolerated the pulmonary vasodilators.
The pathological mechanisms of pediatric PH are shown in Figure 6. PPHN is a persistent increase in the PVR after birth, and the fetal-type circulation cannot transition normally to the adult-type circulation, resulting in unoxygenated blood flow shunting from the ovale or arterial catheter from the right to the left side of the heart, causing hypoxemia (Puthiyachirakkal and Mhanna, 2013; Teng and Wu, 2017). Lung parenchymal injury and dysplasia caused by meconium aspiration and lung infection are the most common causes of PPHN. The main manifestations of pulmonary vascular hypoplasia include abnormal conduction response (García-Cardeña et al., 1996), abnormal development, and microthrombosis (Rabinovitch et al., 1987) in the pulmonary vascular bed. Normal lung parenchyma with pulmonary vascular reconstruction is partially due to intrauterine factors, including chronic hypoxia of the fetus caused by oligohydramnios (Olley et al., 1981), vasoactive drugs crossing from the placental circulation to the fetal pulmonary circulation (Koren and Nordeng, 2012; 't Jong et al., 2012; Van Marter et al., 2013), and occlusion of the intrauterine artery (Pearson et al., 2001). Genetic factors are also involved in the development of PPHN. The pathological mechanisms of PH-CHD and POPH mainly include lack of blood perfusion, increased blood flow after the operation, acute pulmonary vasoconstriction, and altered PGI2 signaling (Wang et al., 2005; Scott, 2010; Taylor and Laussen, 2010). The present study found that PDE5i treatment improved mortality in patients with PPHN. Although ERA treatment did not significantly change the mortality of patients with PPHN, it significantly improved the respiratory and hemodynamic parameters in the patients. This may be related to the pathogenesis of PPHN. The pulmonary vessels of PPHN patients were not completely irreversibly changed. By dilating the pulmonary blood vessels, the vascular hypoxic state may be corrected. For patients with POPH, neither PDE5i nor PGI2 significantly reduced mortality, but they significantly improved respiratory and hemodynamic parameters. This result may be related to the reconstruction of pulmonary blood vessels in patients with POPH before surgery. The increased blood flow in the pulmonary circulation after the repair surgery may further harm pulmonary vessels, but the pulmonary vasodilators allow the blood to flow with less resistance, significantly improving clinical symptoms. The fact that the mortality rate does not decrease significantly may also be related to the short duration of follow-up time and insufficient patients in the included studies.
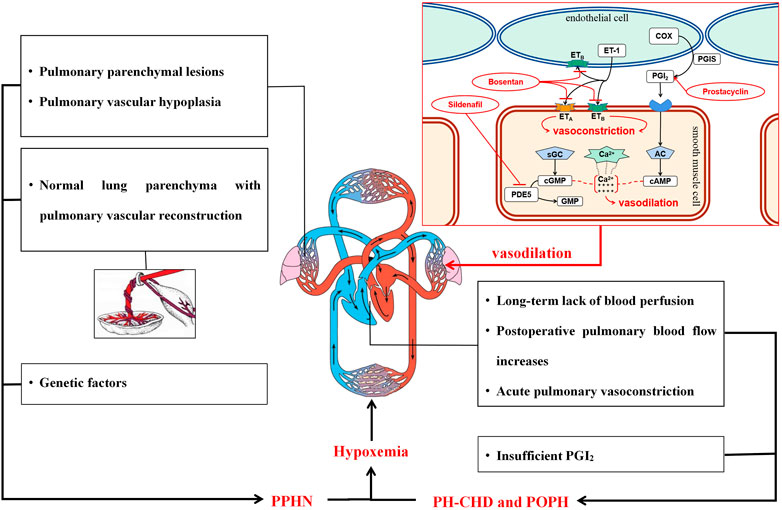
FIGURE 6. Pathological mechanisms of pediatric PH and pulmonary vasodilators. Abbreviations: PVR, pulmonary vascular resistance; SVR, systemic vascular resistance; ASD, atrial septal defect; VSD, ventricular septal defect; PDA, patent ductus arteriosus; ET-1, endothelin-1; ETA, endothelin receptor A; ETB, endothelin receptor B; PDE5, type 5 phosphodiesterase; COX, cyclooxygenase; PGIS, prostacyclin synthase; PGI2, prostacyclin; sGC, soluble guanylate cyclase; cGMP, cyclic guanosine monophosphate; GMP, guanosine monophosphate; AC, adenylate cyclase; cAMP, 3′-5′ cyclic adenosine monophosphate.
We used GRADEpro GDT software to score the quality of evidence (Supplementary Table S6). The research evidence is inaccurate due to the small number of studies included and the small number of participants. The substances used in the control groups were not completely uniform; some studies used distilled water, MgSO4, or tolazoline, and some studies did not explicitly describe the ingredients of the placebo. The measurement of some outcome indicators lacks a unified method and standard.
There were some limitations of the study. First, the meta-analysis included limited number of clinical trials and small sample sizes. Therefore, extra caution should be exercised when interpreting the treatment efficacy for the outcomes. Second, the studies in which the diagnosis of PH based on echocardiography were included. That is not the golden definition of PAH and may weaken the results. Third, some of the included studies have not evaluated the clinical endpoints, such as sPAP, mPAP, etc. Therefore, it may lead to limited and biased results. The original study lacked some key hemodynamic parameters such as PVR, CI, and CO. Most pediatric patients <15 years of age require conscious sedation or general anesthesia during cardiac catheterization which may lead to instability with induction and adverse effects, such as hypoxia and hypercapnia. During or after a cardiac catheterization, pediatrics with PH may experience acute deterioration. The possible reason may be that the parents of the pediatric patients might reject the invasive catheterization due to the potential risk. Therefore, these effect indicators are not included in the outcomes of our research. In addition, the potential effects of different ages on the response to pulmonary vasodilators in the cohort of patients with pediatric PAH may interfere with the statistical power of the conclusions. However, due to the limited number and size of included studies, an age subgroups analysis was difficult to be performed.
Conclusion
The present study shows that pulmonary vasodilators decrease mortality, improve respiratory and hemodynamic parameters, and shorten the duration of mechanical ventilation in pediatric PH patients. In PPHN, PDE5i significantly decrease mortality, and ERAs improve respiratory and hemodynamic parameters. In POPH, respiratory and hemodynamic indicators are markedly improved with PDE5i. The long-term benefits of pulmonary vasodilators for patients with pediatric PH are more clear. Overall, pulmonary vasodilators were well tolerated.
Data Availability Statement
The original contributions presented in the study are included in the article/Supplementary Material, further inquiries can be directed to the corresponding author.
Author Contributions
All authors have read and approved the final manuscript. WH is the corresponding author. TS, HC, and WH were responsible for the study screening, data extraction, data analysis and writing the manuscript. WW, LW, PF, RX, and LW were responsible for checking and reviewing the final manuscript.
Funding
This work was supported by the Chongqing municipal health and Health Committee (No. ZQNYXGDRCGZS2019001, No. 2019ZY3340, No. 2016HBRC001).
Conflict of Interest
The authors declare that the research was conducted in the absence of any commercial or financial relationships that could be construed as a potential conflict of interest.
Supplementary Material
The Supplementary Material for this article can be found online at: https://www.frontiersin.org/articles/10.3389/fphar.2021.668902/full#supplementary-material.
References
't Jong, G. W., Einarson, T., Koren, G., and Einarson, A. (2012). Antidepressant Use in Pregnancy and Persistent Pulmonary Hypertension of the Newborn (PPHN): a Systematic Review. Reprod. Toxicol. 34 (3), 293–297. doi:10.1016/j.reprotox.2012.04.015
Archer, S. L., and Michelakis, E. D. (2009). Phosphodiesterase Type 5 Inhibitors for Pulmonary Arterial Hypertension. N. Engl. J. Med. 361 (19), 1864–1871. doi:10.1056/nejmct0904473
Barnes, H., Brown, Z., Burns, A., and Williams, T. (2019). Phosphodiesterase 5 Inhibitors for Pulmonary Hypertension. Cochrane Database Syst. Rev. 1, CD012621. doi:10.1002/14651858.CD012621.pub2
Barst, R., Ivy, D., Dingemanse, J., Widlitz, A., Schmitt, K., Doran, A., et al. (2003). Pharmacokinetics, Safety, and Efficacy of Bosentan in Pediatric Patients with Pulmonary Arterial Hypertension. Clin. Pharmacol. Ther. 73 (4), 372–382. doi:10.1016/s0009-9236(03)00005-5
Berger, R. L. E., Roofthooft, M. T. R., Hillege, H. L., van Osch-Gevers, M., Delhaas, T., et al. (2011). Pediatric Pulmonary Hypertension in the Netherlands. Circulation 124(16): 1755–1764. doi:10.1161/circulationaha.110.969584
Cheng, Y., Austin, S. C., Rocca, B., Koller, BH., Coffman, TM., Grosser, T., et al. (2002). Role of Prostacyclin in the Cardiovascular Response to Thromboxane A2. Science (New York, NY) 296 (5567), 539–541. doi:10.1126/science.1068711
Cornelisse, R. J., Ivy, D. D., Gaitan, G., Rudzinski, A., Garcia, A. E., et al. (2012). A Randomized, Double-Blind, Placebo-Controlled, Dose-Ranging Study of Oral Sildenafil Citrate in Treatment-Naive Children with Pulmonary Arterial Hypertension. Circulation 125(2): 324–334. doi:10.1161/circulationaha.110.016667
Fraisse, A., Butrous, G., Taylor, M. B., Oakes, M., and Dilleen, M. (2011). Intravenous Sildenafil for Postoperative Pulmonary Hypertension in Children with Congenital Heart Disease. Intensive Care Med. 37 (3), 502–509. doi:10.1007/s00134-010-2065-420115028
Frantz, D. D., Abman, S. H., Barst, R. J., Bonnet, D., Fleming, T. R., et al. (2013). Pediatric Pulmonary Hypertension. J. Am. Coll. Cardiol. 62(25 Suppl. l): D117–D126. doi:10.1016/j.jacc.2013.10.028
Galiè, N., Humbert, M., Vachiery, J.-L., Lang, I., Torbicki, A., et al. (2015). 2015 ESC/ERS Guidelines for the Diagnosis and Treatment of Pulmonary Hypertension. Eur. Respir. J. 46(4): 903–975. doi:10.1183/13993003.01032-2015
GalièGhersi, N., Humbert, M., Vachiery, J.-L., Lang, I., Torbicki, A., et al. (2015). 2015 ESC/ERS Guidelines for the Diagnosis and Treatment of Pulmonary Hypertension. Eur. Heart J. 37(1): 67–119. doi:10.1093/eurheartj/ehv317
García-Cardeña, G., Oh, P., Liu, J., Schnitzer, J. E., and Sessa, W. C. (1996). Targeting of Nitric Oxide Synthase to Endothelial Cell Caveolae via Palmitoylation: Implications for Nitric Oxide Signaling. Proc. Natl. Acad. Sci. 93 (13), 6448–6453. doi:10.1073/pnas.93.13.6448
Gibbs, J. P. T., Altman, D. G., Gotzsche, P. C., Moher, D., Oxman, A. D., et al. (2011). The Cochrane Collaboration's Tool for Assessing Risk of Bias in Randomised Trials. Bmj 343, d5928. doi:10.1136/bmj.d5928
Gibbs, W. A., and Ismail, M. (2012). A Randomized, Double-Blind, Placebo-Controlled, Prospective Study of Bosentan for the Treatment of Persistent Pulmonary Hypertension of the Newborn. J. Perinatol 32 (8), 608–613. doi:10.1038/jp.2011.157
Hoeper, M. M., Bogaard, H. J., Condliffe, R., Khanna, D., Kurzyna, M., et al. (2013). Definitions and Diagnosis of Pulmonary Hypertension. J. Am. Coll. Cardiol. 62(25 Suppl. l): D42–D50. doi:10.1016/j.jacc.2013.10.032
Juni, H., Soliz, A., Neira, F., Venegas, M. E., and Sola, A. (2006). Oral Sildenafil in Infants with Persistent Pulmonary Hypertension of the Newborn: a Pilot Randomized Blinded Study. Pediatrics 117 (4), 1077–1083. doi:10.1542/peds.2005-0523
Kolaee, Z-c., Qi-wei, H. U. A. N. G., Rong, Qun-fang., Xiao-hui, G. O. N. G., and Huang, Min. (2009). A Comparison Study of Using Sildenafil on Persistent Pulmonary Hypertension of the Newborn. J. Clin. Pediatr. 27 (8), 4.
Koren, G., and Nordeng, H. (2012). Antidepressant Use during Pregnancy: the Benefit-Risk Ratio. Am. J. Obstet. Gynecol. 207 (3), 157–163. doi:10.1016/j.ajog.2012.02.009
Layton, A., and Bavdekar, S. (2015). Sildenafil in Pediatric Pulmonary Arterial Hypertension. J. Postgrad. Med. 61 (3), 181–192. doi:10.4103/0022-3859.159421
Olley, J. D., Rabinovitch, M., Goldstein, J. D., and Reid, L. M. (1981). The Structural Basis of Persistent Pulmonary Hypertension of the Newborn Infant. J. Pediatr. 98 (6), 962–967. doi:10.1016/s0022-3476(81)80605-1
Onan, I. S., Ozturk, E., Yildiz, O., Altin, H. F., Odemis, E., and Erek, E. (2016). The Effect of Intravenous Iloprost on Pulmonary Artery Hypertension after Paediatric Congenital Heart Surgery. Interact Cardiovasc. Thorac. Surg. 22 (2), 194–199. doi:10.1093/icvts/ivv325
Pan, Yanyan. S. Y. Z. C., and Kong, Qingyu. (2016). Clinical Efficacy of Bosentan in the Treatment of Babies with Congenital Heart Disease Complicated with Pulmonary Hypertension. J. Shandong Univ. (Health Sciences) 54 (2), 53–56.
Pearson, D. L., Dawling, S., Walsh, W. F., Haines, J. L., Christman, B. W., Bazyk, A., et al. Neonatal Pulmonary Hypertension. N. Engl. J. Med. 2001; 344(24): 1832–1838. doi:10.1056/nejm200106143442404
Peiravian, F., Amirghofran, A. A., Borzouee, M., Ajami, G. H., and Sabri, M. R. (2007). Sildenafil to Control Pulmonary Hypertension after Congenital Heart Surgery. Asian Cardiovasc. Thorac. Ann. 15(2): 113–117. doi:10.1177/021849230701500207
Pulido, H., Yeoh, H. L., Fothergill, T., Burns, A., Humbert, M., and Williams, T. (2019). Prostacyclin for Pulmonary Arterial Hypertension. Cochrane Database Syst. Rev. 5, CD012785. doi:10.1002/14651858.CD012785.pub2
Puthiyachirakkal, M., and Mhanna, M. J. (2013). Pathophysiology, Management, and Outcome of Persistent Pulmonary Hypertension of the Newborn: a Clinical Review. Front. Pediatr. 1, 23. doi:10.3389/fped.2013.00023
Rabinovitch, M., Andrew, M., Thom, H., Trusler, G. A., Williams, W. G., and Rowe, R. D. (1987). Abnormal Endothelial Factor VIII Associated with Pulmonary Hypertension and Congenital Heart Defects. Circulation 76(5): 1043–1052. doi:10.1161/01.cir.76.5.1043
Rosenzweig, E. B., Kerstein, D., and Barst, R. J. (1999). Long-term Prostacyclin for Pulmonary Hypertension with Associated Congenital Heart Defects. Circulation 99 (14), 1858–1865. doi:10.1161/01.cir.99.14.1858
Rubin, L. J., Badesch, D. B., Barst, R. J., Galiè, N., Black, C. M., Keogh, A., et al. (2002). Bosentan Therapy for Pulmonary Arterial Hypertension. N. Engl. J. Med. 346(12): 896–903. doi:10.1056/nejmoa012212
Scott, K. K. (2010). A Review of Prostaglandin Analogs in the Management of Patients with Pulmonary Arterial Hypertension. Respir. Med. 104 (1), 9–21.
Shamseer, L., Moher, D., Clarke, M., Liberati, A., Petticrew, M., et al. (2015). Preferred Reporting Items for Systematic Review and Meta-Analysis Protocols (PRISMA-P) 2015: Elaboration and Explanation. Bmj 349, g7647. doi:10.1136/bmj.g7647
Sharma, V., Joshi, S., Joshi, A., Kumar, G., Arora, H., and Garg, A. (2015). Does Intravenous Sildenafil Clinically Ameliorate Pulmonary Hypertension during Perioperative Management of Congenital Heart Diseases in Children? - a Prospective Randomized Study. Ann. Card. Anaesth. 18 (4), 510–516. doi:10.4103/0971-9784.166457
Steinhorn, R. H., Fineman, J., Kusic-Pajic, A., Cornelisse, P., Gehin, M., Nowbakht, P., et al. (2016). Bosentan as Adjunctive Therapy for Persistent Pulmonary Hypertension of the Newborn: Results of the Randomized Multicenter Placebo-Controlled Exploratory Trial. J. Pediatr. 177: 90–96. doi:10.1016/j.jpeds.2016.06.078
Szatmari, R. J., Beghetti, M., Pulido, T., Konourina, I., Zhang, M., et al. (2014) Starts-2. Circulation 129 (19), 1914–1923. doi:10.1161/circulationaha.113.005698
Takahashi, K., Mori, Y., Yamamura, H., Nakanishi, T., and Nakazawa, M. (2003). Effect of Beraprost Sodium on Pulmonaryvascular Resistance in Candidates for a Fontan Procedure: A Preliminarystudy. Pediatr. Int. 45 (6), 671–675. doi:10.1111/j.1442-200x.2003.01800.x
Taylor, M. B., and Laussen, P. C. (2010). Fundamentals of Management of Acute Postoperative Pulmonary Hypertension. Pediatr. Crit. Care Med. 11, S27–S29. doi:10.1097/pcc.0b013e3181c769a4
ten Harkel, E. B., Abman, S. H., Adatia, I., Beghetti, M., Bonnet, D., Haworth, S., et al. (2019). Paediatric Pulmonary Arterial Hypertension: Updates on Definition, Classification, Diagnostics and Management. Eur. Respir. J. 53 (1). doi:10.1183/13993003.01916-2018
Teng, R. J., and Wu, T. J. (2017). Persistent Pulmonary Hypertension of the Newborn. Semin. Fetal neonatal Med. 22 (4), 220–226.
Uslu, S., Kumtepe, S., Bulbul, A., Comert, S., Bolat, F., and Nuhoglu, A. (2011). A Comparison of Magnesium Sulphate and Sildenafil in the Treatment of the Newborns with Persistent Pulmonary Hypertension: a Randomized Controlled Trial. J. Trop. Pediatr. 57 (4), 245–250. doi:10.1093/tropej/fmq091
Van Marter, L. J., Hernandez-Diaz, S., Werler, M. M., Louik, C., and Mitchell, A. A. (2013). Nonsteroidal Antiinflammatory Drugs in Late Pregnancy and Persistent Pulmonary Hypertension of the Newborn. Pediatrics 131 (1), 79–87. doi:10.1542/peds.2012-0496
Vargas-Origel, A., Gómez-Rodríguez, G., Aldana-Valenzuela, C., Vela-Huerta, M. M., Alarcón-Santos, S. B., and Amador-Licona, N. (2010). The Use of Sildenafil in Persistent Pulmonary Hypertension of the Newborn. Amer J. Perinatol 27 (3), 225–230. doi:10.1055/s-0029-1239496
Wade, M., Hunt, T. L., and Lai, A. A. (2003). Effect of Continuous Subcutaneous Treprostinil Therapy on the Pharmacodynamics and Pharmacokinetics of Warfarin. J. Cardiovasc. Pharmacol. 41 (6), 908–915. doi:10.1097/00005344-200306000-00012
Wang, T., Li, S. X., Zhang, X. Q., Gu, X. H., Song, Y., Zhang, G., et al. (2005). Study on the Effect of Adrenomedulin and Urotensin-II on Pulmonary Hypertension of Patients with Congenital Heart Disease. Zhonghua yi xue za zhi. 85 (38), 2691–2695.
Wessel, T. R. C. G., Holberto, C. J., Loera, G. R. G., and Rodríguez, B. I. (2006). Oral sildenafifil as an alternative treatment in the persistent pulmonary hypertension in newborns[Sildenafifil oral como alternativa en el tratamiento de recien nacidos con hipertension pulmonar persistente]. Revista Mexicana de Pediatria 73, 4.
Keywords: pediatric pulmonary hypertension, efficacy, safety, meta-analysis, pulmonary vasodilators
Citation: Shu T, Chen H, Wang L, Wang W, Feng P, Xiang R, Wen L and Huang W (2021) The Efficacy and Safety of Pulmonary Vasodilators in Pediatric Pulmonary Hypertension (PH): A Systematic Review and Meta-analysis. Front. Pharmacol. 12:668902. doi: 10.3389/fphar.2021.668902
Received: 17 February 2021; Accepted: 12 April 2021;
Published: 23 April 2021.
Edited by:
Zhi-Cheng Jing, Peking Union Medical College Hospital, ChinaReviewed by:
Zhuang Tian, Peking Union Medical College Hospital (CAMS), ChinaHui Wang, Peking Union Medical College Hospital (CAMS), China
Jie Song, Central South University, China
Copyright © 2021 Shu, Chen, Wang, Wang, Feng, Xiang, Wen and Huang. This is an open-access article distributed under the terms of the Creative Commons Attribution License (CC BY). The use, distribution or reproduction in other forums is permitted, provided the original author(s) and the copyright owner(s) are credited and that the original publication in this journal is cited, in accordance with accepted academic practice. No use, distribution or reproduction is permitted which does not comply with these terms.
*Correspondence: Wei Huang, d2VpaHVhbmdjcUBnbWFpbC5jb20=