- 1The Center for New Drug Safety Evaluation and Research, Inner Mongolia Medical University, Hohhot, China
- 2The Center for New Drug Screening Engineering and Research of Inner Mongolia Autonomous Region, Inner Mongolia Medical University, Hohhot, China
- 3College of Pharmacy, Inner Mongolia Medical University, Hohhot, China
Background and Aim: QingXiaoWuWei Decoction (QXWWD) is a traditional Chinese medicine that is commonly used in clinical settings to treat inflammatory and bacterial diseases. However, there is still a lot to learn about its molecular mechanism. A network pharmacology approach was applied to investigate the pharmacological mechanisms of QXWWD in inflammation treatment.
Methods: The basic mechanisms involved in the anti-inflammatory and antibacterial potentials of QXWWD were identified using network pharmacology and molecular docking. The principal components of QXWWD were identified by the HPLC-Q-Exactive-MS method. The antibacterial bioactivity of QXWWD was further investigated using the Kirby-Bauer disc diffusion method and the determination of the minimum inhibitory concentration. The anti-inflammatory activity of QXWWD was evaluated using mice ear swelling test, RAW264.7 cell culture, and pro-inflammatory cytokines measurement. Skin irritation and HE staining were employed to evaluate the safety of QXWWD topical use and to depict the drug’s potential therapeutic function. The hub genes and signaling pathways associated with inflammatory and bacterial diseases were validated by western blot in addition to biochemical and pathological markers.
Results: Our findings revealed that the ethanolic extract of QXWWD had a strong inhibitory effect against Staphylococcus aureus, Enterococcus faecalis, and Streptococcus pneumoniae. Meanwhile, QXWWD was potentially effective in suppressing ear swelling, elevated white blood cell counts, and the TNF-α, IL-1, and IL-6 levels. According to skin irritation, QXWWD was found to be safe when tested for topical application. The results of HE staining showed that the possible therapeutic role of QXWWD was related to the change in skin microstructure. Also, the network pharmacology, molecular docking as well as Q-Exactive-MS and HPLC analysis suggested that the synergistic effect of quercetin, luteolin and other ingredients could serve as main contributor of QXWWD for its anti-inflammatory and antibacterial activities. Moreover, the JUN, MAPK1, RELA, NFKBIA, MYC, and AKT1 were the potential identified key targets, and MAPK/PI3K/Akt was among the possibly involved signaling pathways in the anti-inflammatory and antibacterial activities of QXWWD.
Conclusions: From a therapeutic standpoint, QXWWD may be a promising antibacterial and anti-inflammatory agent for the treatment of bacterial, acute, and chronic dermatitis.
Introduction
Inflammation is one of the most common and complex reactions, which mostly occurs due to the cell damage or death caused by certain chemicals or bacterial infection, resulting in the release of inflammatory cytokines like IL-1, IL-6, and TNF-α from the macrophages and leukocytes (Zhao Y et al., 2014; Wang et al., 2015; Paolucci et al., 2018; Ahmad et al., 2020; Diaz et al., 2021). For the management of such inflammatory events, NSAIDs (Non-steroidal anti-inflammatory drugs) are the widely used therapeutics, and they play a prominent role among anti-inflammatory drugs due to their strong anti-inflammatory potentials (Bacchi et al., 2012; Dona et al., 2016; Marquez-Lara et al., 2016; Bindu et al., 2020; Zappavigna et al., 2020). Among them, ibuprofen and aspirin have emerged as effective anti-inflammatory drugs that could potentially inhibit prostaglandin biosynthesis by inhibiting the function of the cyclooxygenase (COX) enzyme (Harris et al., 2005; Renda et al., 2006; Harris et al., 2012). Long-term use of NSAIDs, on the other hand, is linked to a variety of side effects, including bleeding, coagulopathy, interstitial nephritis, gastrointestinal mucosa damage, and allergic reactions due to very low prostaglandin levels (Harirforoosh et al., 2013; Marjoribanks et al., 2015). Traditional Chinese herbal medicines have a broad antibacterial range and low drug resistance, which makes them ideal for treating inflammation (Zhao CQ et al., 2014; Cheng and Merz, 2016). In one study carried by (Zhang L et al., 2013), 58 kinds of traditional Chinese herbal medicines were screened out based on their traditional usage and the existing literature. The antimicrobial effects of their ethanol extracts were assessed against yeast (Candida albicans), mold (Aspergillus fumigatus), Gram-positive bacteria (Staphylococcus aureus) as well as Gram-negative bacteria (Acinetobacter baumanniiand and Pseudomonas aeruginosa). The results indicated that 15 extracts showed an anti-fungal effect while 23 extracts exhibited an antibacterial effect. Further, eight extracts exhibited both anti-fungal and antibacterial effects. For example, Polygonum cuspidatum, Eucommia ulmoides, Uncaria rhyncophylla, and Poria cocos showed effects against both fungal and bacterial strains, indicating their broad spectrum of activities. On the other hand, due to the multi-component characteristics, many small molecules with good binding affinity were identified from the Traditional Chinese Medicine database as potential candidates. Herbal therapies are thus useful in combating fungal and bacterial resistance because they have low drug resistance, high effectiveness, and are widely available. For the reasons mentioned above, they have attracted the interest of researchers looking into the use of herbal medicines to treat a variety of diseases. When combined with the broad antibacterial spectrum, traditional Chinese herbal medicines are expected to be formulated as therapeutic drugs for the treatment of inflammatory and bacterial diseases.
QingXiaoWuWei Decoction (QXWWD) is traditional Chinese medicine and is composed of Sophorae flavescentis radix (dried radix of Sophora flavescens Aiton), Fructus cnidii (dried and ripe fructus of Cnidium monnieri (L.) Cusson), Rhei Radix et Rhizoma (dried rhizomia of Rheum palmatum L), Artemisia Argyi Folium (dried folium of Artemisia argyi H. Lév. and Vaniot), and Borneolum (extraction of branch and folium of Cinnamomum camphora (L.) J. Presl). To prepare a typical traditional decoction, a mixture of Sophorae Flavescentis Radix, Fructus Cnidii, Rhei Radix et Rhizoma, and Artemisia Argyi Folium (3, 3, 3, 3 g) is soaked in water (usually with adding less strong liquor) for ∼0.5–1 h before a first boiling step follows. After filtration, the herbal material is reextracted twice with less water and the combined extracts are collected. The full extract is then added with 0.15 g Borneolum to yield the final QXWWD preparation for one-day use.
In Traditional Chinese Medicine, Sophorae Flavescentis Radix, Fructus Cnidii, Rhei Radix et Rhizoma, Artemisia Argyi Folium, and Borneolum can be used alone or in combination for the treatment of inflammatory diseases. For instance, the dried radix of Sophorae Flavescentis Radix has anti-inflammatory, antibacterial, antiviral, anticancer, and antifibrotic properties, and can also be used to treat various immunological diseases (Ma et al., 2018). The predominant compounds in Fructus Cnidii and Rhei Radix et Rhizoma have antibacterial, anti-inflammatory, antifibrotic, anticancer, and antioxidant activities (Zhong et al., 2017; Espinosa et al., 2020). Artemisia Argyi Folium is traditionally used in South East Asia for the treatment of various inflammatory diseases, such as dermatitis, arthritis, and bronchitis (Chen et al., 2017). Borneolum, a widely used food, and cosmetics additive, has antibacterial, analgesic, anti-inflammatory and penetration promoting effects (Dai et al., 2009). In summary, the clinical application and existing literature demonstrate that QXWWD therapy is both internally and externally safe and effective.
In China, QXWWD has long been used to treat inflammatory conditions such as acute and chronic dermatitis. However, there is still a lot to learn about its molecular mechanism. The whole review and systematic characteristics of network pharmacology are of great significance for assessing traditional Chinese medicines, the theory related to syndrome differentiation and synergy in the Chinese medicines developed among various components, channels and targets as well. This, in turn, provides new insights into the fundamental studies of complex traditional Chinese medicine systems. Therefore, the compounds in QXWWD, their anti-inflammatory potentials, and the underlying molecular mechanism of such effects could easily be evaluated by adopting network pharmacology and molecular docking. Considering the therapeutic effects of QXWWD, the anti-inflammatory and antibacterial effects and related mechanisms were verified by in vitro experiments using bacteria, cells, and animal models. The workflow of the study is shown in Figure 1.
Materials and Methods
Chemicals and Materials
Sophorae Flavescentis Radix (“Kushen” in Chinese, KS, batch number 190601), dried radix of Sophora flavescens Aiton, Fructus Cnidii (“Shechuangzi” in Chinese, SCZ, batch number 191001), dried and ripe fructus of Cnidium monnieri (L.) Cusson, Rhei Radix et Rhizoma (“Dahuang” in Chinese, DH, batch number 190901), dried rhizomia of Rheum palmatum L, Artemisia Argyi Folium (“Aiye” in Chinese, AY, batch number 190501), dried folium of Artemisia argyi H. Lév. and Vaniot, Borneolum (“Bingpian” in Chinese, BP, batch number 191001), extraction of branch and folium of Cinnamomum camphora (L.) J. Presl were all procured from the Anguo medicine market (Baoding, China). The whole plant materials of the five herbal materials were obtained from their original source and the botanic identification was carried out by Professor Bi Qu (Inner Mongolia Medical University, Hohhot, China). The specimens were deposited at the herbarium of medicinal plants at College of Pharmacy, Inner Mongolia Medical University, until further processing.
The KM mice (male and female half, 5–6 weeks old, weight range: 18–22 g) were procured from Beijing Weitonglihua Laboratory Animal Co, Ltd. (production license number: SCXK (Jing) 2012–0,001, Beijing, China). Twelve rabbits (male and female half, 2 months old, weight range: 1.8–2.2 kg) were obtained from Suzhou Huqiao Biotechnology Co, Ltd. (production license number: SCXK (Su) 2015–0,002, Suzhou, China). Three strains of Staphylococcus aureus with different drug resistance (ATCC29213, ATCC25923, ATCC43300), Enterococcus faecalis (ATCC29212), Streptococcus pneumoniae (ATCC49619), Pseudomonas aeruginosa (ATCC27853), Streptococcus pyogenes (ATCC19615), Klebsiella pneumoniae (ATCC700603), Escherichia coli (ATCC25922), Enterobacter cloacae (ATCC700323) and RAW264.7 cells were procured from ATCC (Rockville, MD, United States). The DMEM and Fetal bovine serum (FBS) were acquired from GIBCO (Grand Island, NY, United States). MH agar plates were obtained from Qingdao Haibo Biotechnology Co, Ltd. (Qingdao, China). ELISA kits and lipopolysaccharide (LPS) were procured from Soleibao Technology Co, Ltd. (Beijing, China). CCK8 kits were procured from Meilun Biotechnology Co, Ltd. (Dalian, China). Aspirin was purchased from Langtze Biomedical Technology (Nanjing, China). The thin layer silica gel plates were procured from Qingdao Ocean Chemical Co, Ltd. (Qingdao, China). All of the solvents used in the experiments were of an analytical quality (Tianjin Fuyu Fine Chemical Co, Ltd, Tianjin, China). Methanol and formic acid (HPLC-grade) were procured from Fisher Chemicals (Fisher, United States). All the animal studies comply with the 3 R (Heinrich M et al., 2020) of animal research and were approved by the Animal Ethics Committee of Inner Mongolia Medical University (YKD202002060).
Identification and Screening of Main Chemical Compounds and Protein Targets of QXWWD
The database and analysis Platform (TCMSP, https://tcmspw.com/tcmsp.php) of Traditional Chinese Medicine Systems Pharmacology was used for the authentication of main chemical compounds and the protein targets linked with these compounds of the five herbs in QXWWD. The main chemical compounds of QXWWD were opted according to the established criterion of both OB ≥ 30% and DL ≥ 0.18. To further evaluate the network pharmacology, the protein targets such as gene ID and gene names were standardized by employing UniProtKB (http://www.uniprot.org).
Predicting the Targets of the Disease
The keywords “inflammation and bacteria” were utilized for screening the inflammation and bacteria-associated targets while searching the GeneCards database (https://www.genecards.org/), DrugBank database (https://go.drugbank.com/), TTD database (http://db.idrblab.net/ttd//), PharmGkb database (https://www.pharmgkb.org/), and OMIM database (https://omim.org/). The most frequent targets of QXWWD and disease were then assembled using the R platform.
Construction and Analysis of the Interaction Network
The common targets of QXWWD and disease were introduced into the STRING database (https://string-db.org/cgi/input.pl) to construct the PPI (protein-protein interaction) network. The species was selected as “Homo sapiens,” the lowest interaction score was greater than “0.900” and the PPI network was exported after hiding the free points. Cytoscape3.8.0 was employed to build and image the PPI network. CytoNCA, a network plug-in in Cytoscape, was utilized for screening the hub genes of the network by experimental data available for protein interaction networks, such as Closeness (CC), Betweenness (BC), Eigenvector (EC), Degree (DC), and Local Average Connectivity-based method (LAC). The level of these five parameters represented the topological significance of a node in a network, i.e., the higher the value, the more significant the node is. The targets with a degree greater than the median were chosen as the hub genes and were considered for further molecular docking.
GO and KEGG Pathway Enrichment Analysis
To deeply explore the pathways and biological process of the network, GO (Gene Ontology) and KEGG (Kyoto Encyclopedia of Genes and Genomes) pathway analyses were conducted by employing the cluster profiler package in the R platform. GO enrichment analysis encompassed cellular components (CC), biological processes (BP), and molecular functions (MF). The statistical significance threshold of enrichment analysis was established at p ≤ 0.05.
Molecular Docking
PubChem database (https://pubchem.ncbi.nlm.nih.gov/) was used for acquiring the 2D structures of the basic compounds. Then, Chem3D was used to process and transfer the 2D structure of the main compounds into a 3D structure, which was saved as ligands in PDBQT format. The 3D structure of the desired molecules was retrieved from the PDB database (https://www.rcsb.org/). PyMol was utilized for eliminating the water molecules, added the nonpolar hydrogen for the structure, and saved it as a PDBQT file. Autodock Vina 1.1.2 was employed to dock ligands with target molecules, respectively. The files after molecular docking were visualized by DiscoveryStudio2020 software.
Preparation of QXWWD
Equivalent weight (18 g) of Sophorae Flavescentis Radix, Fructus Cnidii, Rhei Radix et Rhizoma, and Artemisia Argyi Folium were soaked in 75% ethanol (w/v = 1: 10) for 30 min, then refluxed twice (1 h each time), and finally filtered. The resultant filtrate was collected and concentrated to prepare the final concentration equivalent to 1 g raw herb/mL. Borneolum (0.9 g) was added to the filtrate for the Q Exactive MS analysis and the antibacterial activity test. The filtrate was concentrated in a rotary evaporator at 40°C, freeze-dried, and preserved for xylene-induced ear swelling test, cell culture viability assay, inflammatory factors measurement, western blot assay and skin irritation test.
Thin Layer Chromatography
Following the method of Chinese Pharmacopoeia 2015, the identification of Sophorae Flavescentis Radix, Fructus Cnidii, Rhei Radix et Rhizoma, Artemisia Argyi Folium, and Borneolum was conducted by employing the thin layer chromatography method.
Identification of the Main Chemical Components in QXWWD by Chromatograph and Q Exactive MS.
The extract of QXWWD was centrifuged at 1 × 104 rpm for 15 min, and the supernatant was diluted with 50% methanol to appropriate concentrations. The resultant solution was filtered through a 0.22 μm filter for the HPLC-Q-Exactive-MS analysis.
HPLC conditions: Analyses were conducted on the Thermo U3000 system (Thermo Fisher Scientific, United States) with a YMC-Pack HPLC column (ODS-A C18 250 mm × 4.6 mm, 5 μm) and the column temperature was maintained at 40°C. The mobile phase consisted of methanol (A) and 0.1% formic acid (B). The injection volume was 20 μL, the flow rate of the mobile phase was 1 ml/min, and the UV and MS split ratio was 7:3. Analytes were eluted from the column in a gradient. The gradient procedure was carried out as follows: the initial composition of B was 93%, and after 40–50 min, it was reduced to 0%.
MS conditions: Analyses were conducted on a Thermo Q Exative spectrometer (Thermo Fisher Scientific, United States) in a positive mode combined with an electrospray ionization (ESI) source. The MS parameters were as follows: the sheath gas flow rate and aux gas flow rate were 40 L/h and 2 L/h, respectively. The capillary temperature and the aux gas heater temperature were set at 350 and 150°C, respectively. The spray voltage was set at 3.5 kV. Data were collected over the range of m/z 100–1,100 with a full ms dd ms2 mode.
Xylene-Induced Ear Swelling Test
Six groups of 10 KM mice (male and female half) were treated with 0.5% CMC-Na (carboxymethyl cellulose sodium), QXWWD (680, 340, and 170 mg/kg), or aspirin (200 mg/kg). The QXWWD group’s dosage was calculated using its clinical dose (Heinrich M et al., 2020). The high, medium, and low dose groups of QXWWD were all dissolved in 0.5% CMC-Na. The drugs were given to the mice once a day for five days with an oral dose of 0.1 ml/10 g. On the sixth day, xylene (0.02 ml) was added to the posterior and anterior surfaces of the right ear. After 30 min, 0.5 ml of blood was collected from the orbit for white blood cell counts measurement. The mice were subjected to euthanasia while a 6-mm hole was punched out of both the treated and control ears. The ear swelling rate was expressed as the weight difference between the xylene treated ear (right ear, MR) and non-treated ear (left ear, ML). The formula is showed as following: (MR-ML)/ML.
Antibacterial Activity Test
For antibacterial activity, the Kirby-Bauer (K-B) disc diffusion method was carried out on the agar plates. For control strains, three strains of Staphylococcus aureus (ATCC29213, ATCC25923, ATCC43300) with different drug resistance, Enterococcus faecalis (ATCC29212), Streptococcus pneumoniae (ATCC49619), Pseudomonas aeruginosa (ATCC27853), Streptococcus pyogenes (ATCC19615), Klebsiella pneumoniae (ATCC700603), Escherichia coli (ATCC25922), Enterobacter cloacae (ATCC700323) were used. A 20 μL sample solution was used for the impregnation of the filter discs of 6 mm. Also, a 6 mm diameter disc prepared with 20 μL normal saline was used as negative control while antimicrobials (Vancomycin: VA 30 μg, Imipenem: IPM 10 µg) were used as the positive controls. Antimicrobial activity was measured as a diameter of the inhibition zone around the disc after the incubation period of 24 h at 37°C, and the results were compared with negative and positive controls.
Minimum Inhibitory Concentration (MIC) Determination
The samples were diluted into a series of concentration gradients (500, 250, 125, 62.5, 31.25, 15.625, 7.8125, and 3.90625 μg/ml) by the double dilution method. The samples were then added to the test tubes containing a 10 ml medium. Then, 50 μL of bacteria solution (1 × 105 CFU/ml) was added to each test tube. All the tubes were cultured at 37°C for 24 h. The MIC was the lowest concentration of observable turbidity in the test tube that could be seen through the naked eye.
Cell Culture and Viability Assay
The DMEM high glucose medium augmented with 10% (v/v) FBS, was employed for the growth of RAW 264.7 cells at 37°C and 5% CO2. For the evaluation of QXWWD's effect on cell viability, RAW264.7 cells were sown in 96-well plates for 24 h at a density of 5 × 103 cells/well. For the treatment of these cells, different concentrations of QXWWD in the 0–500 μg/ml range were used for 24 h. Then, the culture medium was added with 10 µL of CCK-8 solution (100 µL/well) and was incubated at 37°C for 3 h. The absorbance was read with the help of a Multiskan MK3 microplate reader (Thermo Fisher Scientific, United States), at 450 nm and the IC50 (the half-maximal inhibitory concentration) was calculated using GraphPad Prism 8.0.
Measurement of Pro-inflammatory Cytokines
RAW264.7 cells were transferred to the 96-well plates at a density of 5×103 cells/well and incubated for 24 h. The cells were treated with different concentrations (80 μg/ml, 40 μg/ml, 20 μg/ml) of QXWWD for 24 h in the presence and absence of LPS (1 μg/ml). Murine TNF-α, IL-1, and IL-6 ELISA kits were used to measure pro-inflammatory cytokines TNF-α, IL-1, and IL-6 in cell-free supernatants according to the manufacturer’s instructions.
Western Blot Analysis
RAW264.7 cells were cultured in high-glucose DMEM containing 10% FBS at 37°C in a incubator containing 5% CO2. To see how QXWWD affects these cells’ signaling pathways, cells were plated in 6-well plates (5 × 103/well) and treated for 24 h with a variety of QXWWD concentrations (80 μg/ml, 40 μg/ml, 20 μg/ml), in the presence as well as in the absence of LPS (1 μg/ml). The cells were collected from the 6-well plates after being washed with ice-cold PBS followed by the addition of lysis buffer (Beyotime, Shanghai, China). The recovered lysate was incubated on ice for 30 min and centrifuged at 14,000 × g for 20 min at 4°C. Thereafter, the concentrations of protein were measured with a BCA Protein Assay Kit (Beyotime, Shanghai, China). An equal amount of protein (30 µg) was separated by 12% SDS-PAGE and transferred to the polyvinylidene difluoride (PVDF) membranes (Beyotime, Shanghai, China). The PVDF membranes were blocked with 5% fat-free milk and incubated with different primary antibodies against P38 (1:1,000 dilution), JNK (1:500 dilution), ERK (1:1,000 dilution), Akt (1:1,000 dilution), PI3K (1:1,000 dilution), P65 (1:1,000 dilution), p-P38 (1:1,000 dilution), p-JNK (1:1,000 dilution), p-ERK (1:1,000 dilution), p-Akt (1:1,000 dilution), p-PI3K (1:1,000 dilution), p-P65 (1:1,000 dilution), and β-actin/GAPDH (1:1,500 dilution) respectively for 18–24 h at 4°C and followed by washing twice. After incubation with the horseradish peroxidase-conjugated antibodies (1:1,000 dilution) for 0.5–1 h at room temperature, the target protein bands were detected with enhanced chemiluminescence substrate (Beyotime, Shanghai, China). Each WB analysis was performed three times. Semiquantitative analysis was performed using ImageJ software.
Evaluation of Skin Irritation
Twelve rabbits were divided into two groups (normal skin group and damaged skin group), with six rabbits in each group. Before the experiment, hairs were removed from both sides of the experimental animals’ spines, with a hair-free area of 4 cm × 4 cm and an application area of 3 cm × 3 cm. To retain a little exudative in the skin, a sterilized surgical scalpel was used to gently scrape the damaged skin model. Then, 680 mg/kg of QXWWD was dissolved in 0.5% CMC-Na. For 7 days, 2 ml of QXWWD was applied topically on one side of the skin while 0.5% CMC-Na (2 ml) was applied topically on the other side. On daily basis, the medication site was observed for the presence of edema and erythema, which was noted 1, 24, 48, and 72 h after the last time drug, scored using skin irritation criteria, and assessed the stimulation intensity (Table 1).
Hematoxylin and Eosin (HE) Staining
The skin of the rabbit was peeled off about 1 cm2 in each experimental zone and was kept in paraformaldehyde (4%) for a duration of 1 h at 4°C. After dipping the tissues in 0.01 mol/L PBS for 5 min, they were dehydrated in an ethanol gradient. Following that, the tissues were vitrified with xylene and fixed in wax. The Leica RM2235 rotary microtome (Leica, Germany) was employed for the preparation of 5 μm thick sections. The slices were HE stained and were examined under a Leica DM2000 microscope (Leica, Germany).
Statistical Analysis
All data were depicted as mean and standard deviations (SD) and compared the control and treated groups by one-way analysis of variance (ANOVA) or nonparametric Wilcoxon rank-sum test with Bonferroni corrections while using SPSS20.0, p-value <0.05 was considered statistically significant.
Results
Chemical Ingredients of QXWWD
In total, 1,058 chemical compounds were obtained from the TCMSP database, they were obtained from Sophorae Flavescentis Radix (490 compounds), Fructus Cnidii (222 compounds), Artemisia Argyi Folium (236 compounds), Rhei Radix et Rhizoma (110 compounds), and Borneolum (0 compounds).
Network Construction and Analysis
A total of 2,352 potential targets of disease were examined through “inflammation and bacteria”. These targets were added to the 1,058 targets of QXWWD to get a total of 136 core targets. The core targets were inputted into the network visualization software Cytoscape 3.8.0 to build the following network (Figure 2) which had 47 nodes. The larger node was of great significance. With regard to the degree analysis, the top ten compounds were quercetin, beta-sitosterol, stigmasterol, luteolin, formononetin, 8-Isopentenyl-kaempferol, aloe-emodin, phaseolin, o-Isovalerylcolum bianetin, and wighteone with 308-degree, 114-degree, 62-degree, 57-degree, 39-degree, 30-degree, 24-degree, 24-degree, 20-degree, and 19-degree respectively. Further details of these compounds have been depicted in Table 2. The targets (RELA, NFKBIA, MYC, MAPK1, MAPK14, ESR1, NR3C1, AKT1, TP53, JUN, and FOS) with a degree greater than the median were opted as the hub genes (Figure 3) and were considered for further molecular docking.
Biological Function and Pathway Analysis
To deeply explore the underlying mechanism of drug treatment, GO and KEGG enrichment analyses were conducted with the R platform on 136 core targets. A total of 2088 terminologies related to biological events were chosen, specifically included inflammatory response, response to bacteria, response to nutritional level, response to lipopolysaccharide, response to oxidative stress, etc. (Figure 4A). Concerning the enrichment analysis of cellular components, the targets were comprised of the cell membrane. Concurrently, the molecular function terms primarily comprised steroid hormone receptor activity, ligand−activated transcription factor activity, nuclear receptor activity, and so on. The main effects linked to the QXWWD were grouped using the KEGG pathway enrichment analysis. A sum of 30 top-ordered pathways (Figure 4B) were screened out (p < 0.05). Among them, the most important pathways included the MAPK signaling pathway, TNF signaling pathway, PI3K-Akt signaling pathway, AGE-RAGE signaling pathway, and other pathways associated with immunity and inflammation.
Molecular Docking of Main Chemical Compounds Binding to Hub Genes
In this study, the most expected interaction activity between 11 hub genes and their corresponding compounds of QXWWD was assessed by using molecular docking verification (Figure 5). However, the main chemical compounds, as well as the targets, were filtered using AutoDock Vina’s docking affinity values. Thus, the larger the absolute value of the docking affinity, the greater the binding capability between the active sites of targets and compounds. For concreteness, quercetin and luteolin were selected for molecular docking with the hub genes (Figure 6).
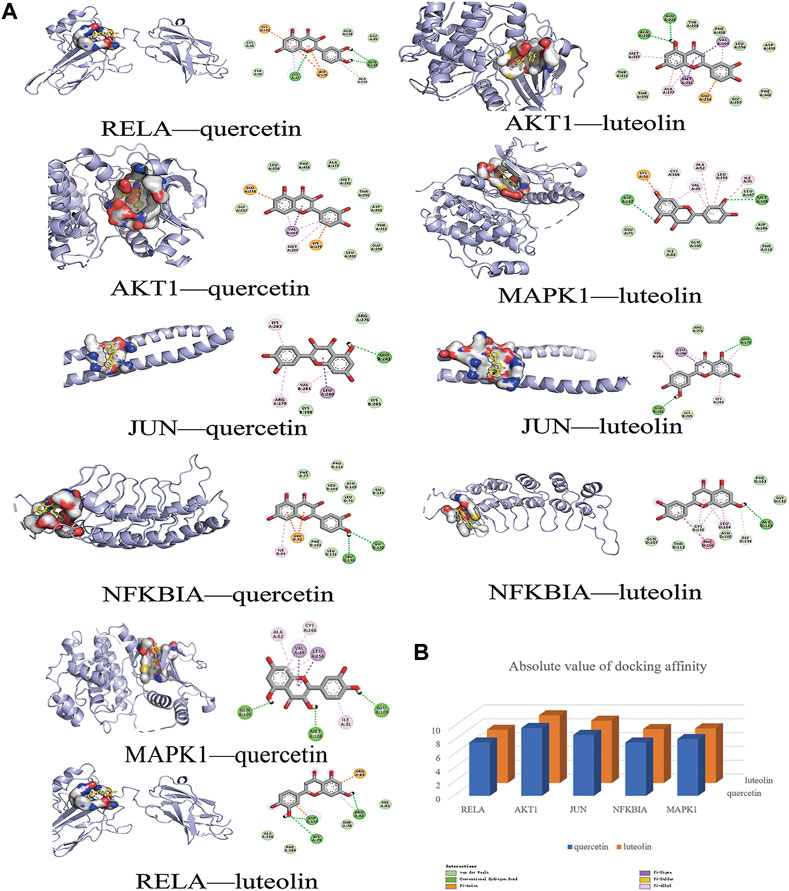
FIGURE 6. Molecular docking models of main chemical compounds binding to potential targets. (A) Molecular docking models of main chemical compounds, (B) Absolute value of docking affinity.
Thin Layer Chromatography
Within the chromatogram of the sample solution, the same color spots were represented in corresponding positions with standard herb or control herb solution (Figure 7).
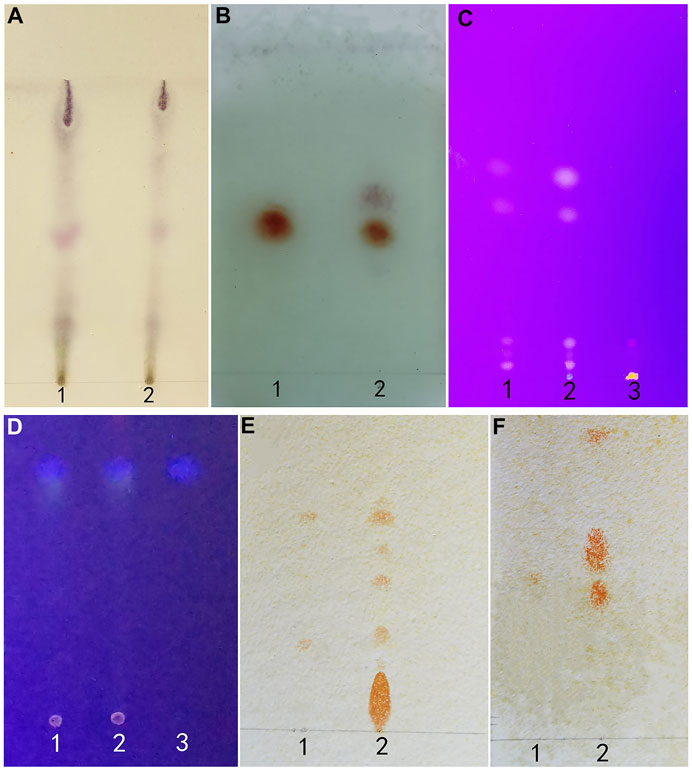
FIGURE 7. (A) (Artemisia Argyi Folium): 1 test herb 2 control herb. (B) (Borneolum): standard herb 2 test herb. (C) (Rhei Radix et Rhizoma): 1 control herb 2 test herb 3 standard herb. (D) (Fructus Cnidii): 1 standard herb 2 test herb 3 control herb. (E) (Sophorae Flavescentis Radix): 1 matrine and sophoridine 2 test herb. (F) (Sophorae Flavescentis Radix): 1 oxymatrine 2 test herb.
The Main Chemical Components in QXWWD
According to the degree analysis and HPLC-Q-Exactive-MS, from the QXWWD, among the top ten compounds (Table 2), nine compounds including quercetin, beta-sitosterol, luteolin, formononetin, 8-Isopentenyl-kaempferol, aloe-emodin, phaseolin, o-Isovalerylcolum bianetin and wighteone were obtained. The peaks in the Q Exative chromatogram (Figure 8 and Supplementary Figures S1–S9) confirmed the presence of these compounds.
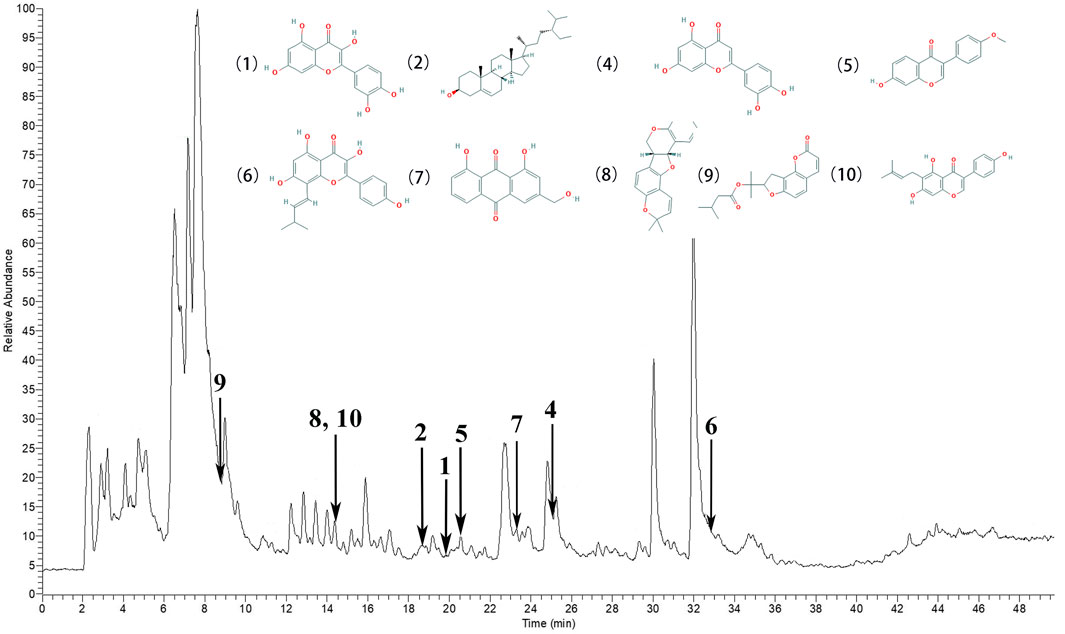
FIGURE 8. The peaks in the Q Exative chromatogram. The Q Exactive MS of the QXWWD (1) quercetin, (2) beta-sitosterol, (4) luteolin, (5) formononetin, (6) 8-Isopentenyl-kaempferol, (7) aloe-emodin, (8) phaseolin, (9) o-Isovalerylcolum bianetin, (10) wighteone.
Anti-Inflammatory Effects of QXWWD in the Animal Model
The ear swelling and the white blood cell (WBC) counts of the model group were considerably greater (**p < 0.01) in contrast with the control group. In contrast with the model group, each dose level group of QXWWD depicted spectacular suppressing effects on xylene-driven ear swelling and the white blood cell counts in a concentration-dependent manner (##p < 0.01 compared with the model group). The results are presented in Figure 9.
Antibacterial Activities of QXWWD
QXWWD was tested for its antibacterial activity. As shown in Figure 10, position 1 in the agar plates was the negative control, positions 2, 3, 4, and 5 were the drug groups, and position 6 was a positive control. In contrast with the negative control group, QXWWD had a significant inhibitory effect on Enterococcus faecalis (ATCC29212), Staphylococcus aureus (ATCC29213, ATCC25923, ATCC43300), and Streptococcus pneumoniae (ATCC49619) but had no obvious inhibitory effect on Streptococcus pyogenes (ATCC19615), Enterobacter cloacae (ATCC700323), Escherichia coli (ATCC25922), Pseudomonas aeruginosa (ATCC27853), and Klebsiella pneumoniae (ATCC700603). (**p < 0.01 in contrast with the positive control group, ##p < 0.01 in contrast with the negative control group). Besides, MICs of QXWWD were found to be 7.8125 μg/ml, 7.8125 μg/ml, 7.8125 μg/ml, 15.625 μg/ml and 250 μg/ml against Staphylococcus aureus (ATCC29213, ATCC25923, ATCC43300), Streptococcus pneumoniae (ATCC49619) and Enterococcus faecalis (ATCC29212) respectively (Supplementary Figures S10–S14).
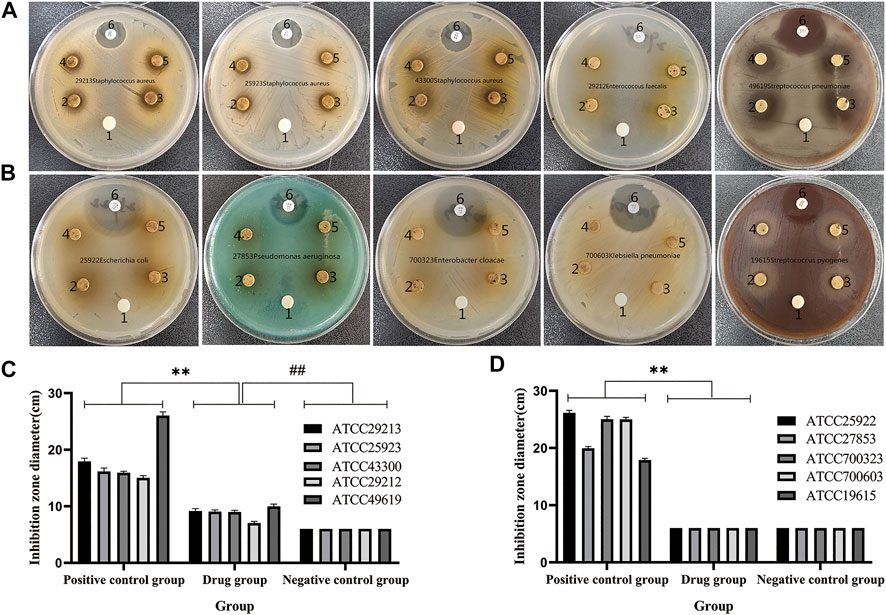
FIGURE 10. Antibacterial activity test. (A and C) QXWWD had a significant inhibitory effect on these bacteria, (B and D) QXWWD had no obvious inhibitory effect on these bacteria.
QXWWD Inhibited the Expression of Multiple Inflammatory Cytokines in RAW264.7 Cells
As shown in Figure 11A, IC50 was found to be 228.1 μg/ml when using the Graph Pad Prism 8.0. Compared with the control group, LPS could significantly induce RAW 274.7 cells to secrete inflammatory cytokines, such as TNF-α, IL-1 and IL-6 (**p < 0.01 compared with the control group). In comparison to the model group, 20–80 μg/ml of QXWWD in LPS-effectuated RAW 264.7 cells greatly suppressed the release of inflammatory mediators such as IL-1, IL-6 and TNF-α (#p< 0.05, ##p< 0.01 compared with the model group) in a dose-dependent manner (Figures 11B, C, D).
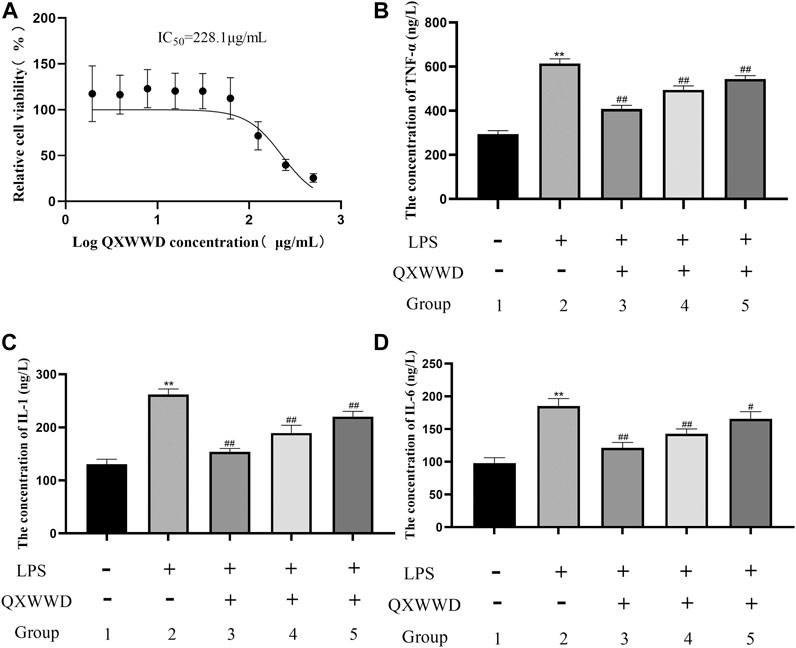
FIGURE 11. Cell viability assay and measurement of pro-inflammatory cytokines 1: control group; 2: model group (1 μg/ml LPS); 3: high dose group (80 μg/ml QXWWD+1 μg/ml LPS); 4: medium dose group (40 μg/ml QXWWD+1 μg/ml LPS); 5: low dose group (20 μg/ml QXWWD+1 μg/ml LPS). (A) Relative cell viability, (B) The concentration of TNF-α in cell-free supernatants, (C) The concentration of IL-1 in cell-free supernatants, (D) The concentration of IL-6 in cell-free supernatants.
QXWWD Regulated the PI3K-Akt and MAPK Signaling Pathways in RAW264.7 Cells
Based on the importance of “top 10” KEGG pathways analyzed and the hub genes of the network, PI3K-Akt, MAPK signaling pathways as well as nuclear factor NF-κB P65 were selected for experimental validation as major bacterial and inflammation-related signaling pathways. As shown in Figure 12A, B, after 24 h treatment with different concentrations of QXWWD (80 μg/ml, 40 μg/ml, 20 μg/ml), the phosphorylated protein levels of P38, JNK, ERK, Akt, PI3K, and P65 were significantly decreased in a dose-dependent manner (**p < 0.01 compared with the control group and #p < 0.05, ##p < 0.01 compared with the model group). Taken together with the results of pro-inflammatory cytokines measurement, the anti-inflammatory and antibacterial activities of QXWWD seem to be mediated by inhibition of the exaggerated release of inflammatory cytokines including IL-1, IL-6, TNF-α as well as regulation of P65, MAPK and PI3K-Akt signaling pathways (Figure 12C).
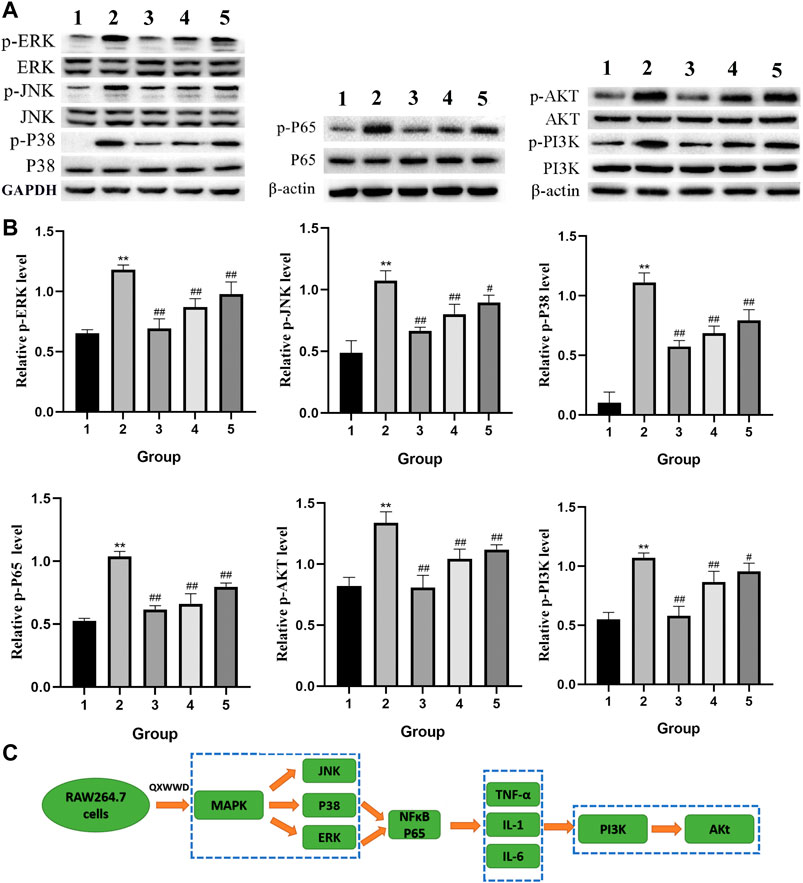
FIGURE 12. QXWWD treatment regulates the expression of key nodes in PI3K-Akt and MAPK signaling pathways activated in RAW264.7 cells 1: control group; 2: model group (1 μg/ml LPS); 3: high dose group (80 μg/ml QXWWD+1 μg/ml LPS); 4: medium dose group (40 μg/ml QXWWD+1 μg/ml LPS); 5: low dose group (20 μg/ml QXWWD+1 μg/ml LPS). (A) Representative bands of protein levels detected by WB, (B) The relative protein levels, (C) QXWWD regulated the PI3K-Akt and MAPK signaling pathways in RAW264.7 cells.
The Possible Therapeutic Role of External Usage of QXWWD
QXWWD did not irritate healthy skin, but it did irritate damaged skin mildly (Supplementary Tables S7–S11). During the microscopic examination, HE staining revealed that the skin structure of control groups (Figure 13) was normal and the epidermal layers were firmly and neatly arranged with clear stratification. The stratum corneum cells were loosely organized after using QXWWD, and the intercellular space in the spinous layer increased. The results revealed that QXWWD’s increased permeability was linked to changes in skin microstructure. For the damaged skin drug group, the decreased inflammatory cells under the stratum spinosum also demonstrated the therapeutic effects of QXWWD relative to the damaged skin control group.
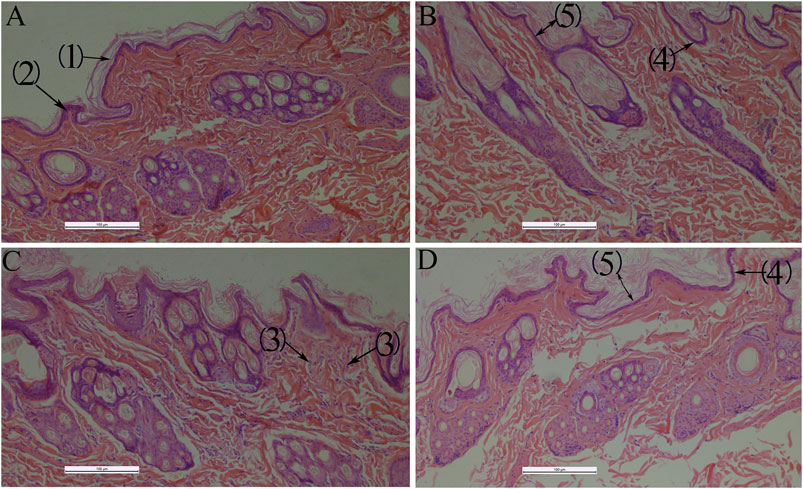
FIGURE 13. Histological photomicrographs of a frozen section taken from rabbit treated with several applications 100×, (A): Normal skin-control (B): Normal skin-drug, (C): Damaged skin-control, (D): Damaged skin-drug; (1): Stratum corneum, (2): Stratum spinosum, (3): Inflammatory cells, (4): Stratum basale, (5): Epidermis.
Discussion
QXWWD is a Chinese folk prescription for the treatment of inflammatory and bacterial diseases that has a long history of clinical practice. QXWWD has a wide range of applications, including the prevention and treatment of traumatic infections, as well as the clinical treatment of acute and chronic dermatitis and other common skin diseases. Our findings revealed that QXWWD ethanolic extract has good antibacterial (inhibition of Enterococcus faecalis, Staphylococcus aureus, and Streptococcus pneumoniae) and anti-inflammatory (suppressing the ear swelling, elevated white blood cell counts, and the levels of TNF-α, IL-1, and IL-6) effects in vitro. The transcutaneous mechanism of QXWWD, which was tested using skin irritation and HE staining, revealed the drug's potential therapeutic function by disrupting the cuticle's microstructure. Based on the network pharmacology and molecular docking, JUN, MAPK1, RELA, NFKBIA, MYC, and AKT1 were found to be the potential identified key targets. The effects of QXWWD on these targets were further verified by western blot. The MAPK and PI3K-Akt signaling pathways were found to be among the top 10 KEGG pathways, with the genes encoding them serving as treatment targets. Both the PI3K-Akt and MAPK signaling pathways were chosen for experimental validation due to lower p values and more key targets identified through KEGG analysis. For the PI3K-Akt signaling pathway, it mediates the proliferation, apoptosis, differentiation, and migration in various cells. Our findings show that QXWWD can reduce the levels of phosphorylated Akt protein in RAW264.7 cells, indicating that it can play a role in the treatment of inflammatory and bacterial diseases by controlling relevant signaling pathways. MAPK signaling pathway also plays a crucial role in inflammation and infection. Particularly, NF-κB is a key factor in regulating inflammatory mediators during the process of inflammation. Drug stimulation of nuclear factor NF-κB increased the levels of pro-inflammatory cytokines such as TNF-α, IL-1, and IL-6. Phosphorylated protein expressions of key targets in the MAPK pathway were significantly decreased after QXWWD treatment. Besides, protein expression of NF-κB p-P65 was also decreased. Current data elucidated that QXWWD could exert better anti-inflammatory and antibacterial effects via blocking the MAPK pathway.
According to network pharmacological analysis, we examined ten important chemical components of QXWWD: quercetin, beta-sitosterol, stigmasterol, luteolin, formononetin, 8-Isopentenyl-kaempferol, aloe-emodin, phaseolin, o-Isovalerylcolum bianetin, and wighteone. Among the top ten compounds, nine compounds including quercetin, beta-sitosterol, luteolin, formononetin, 8-Isopentenyl-kaempferol, aloe-emodin, phaseolin, o-Isovalerylcolum bianetin, and wighteone were obtained by the HPLC-Q-Exactive-MS. Previously, the anti-inflammatory, as well as the immunomodulation properties of quercetin and luteolin, have been extensively implicated in various inflammatory incidents, suggesting that QXWWD may probably play a significant role in the suppression of inflammatory diseased conditions characterized by the immunoinflammatory response. In many investigations, the researchers have found that luteolin arrested the association between p65 (RELA) and transcriptional coactivator and also suppressed the NF-κB transcriptional activity in Rat-1 fibroblast stimulated with LPS (Che et al., 2020). Also, luteolin was found to affect the MAPK pathway and thereby inhibited the IL-1β-induced c-Jun N-terminal kinase (JNK) and p38 kinase stimulation in SW982 cells (Choi and Lee, 2010). Quercetin, one of the main components of QXWWD, was found to inhibit the LPS activated inflammatory responses in mononuclear cells by arresting the TLR2/NF-κB signaling pathways that serve as a connecting bridge between specific and non-specific immunity and could be a useful candidate for the management of inflammatory conditions involving factors like PI3K, NF-κB and TNFα (Zhang et al., 2016). Considering the importance of the two compounds, based on the authentic compound standards (≥99% purity), quercetin and luteolin were identified in our QXWWD. The HPLC chromtograms of quercetin and luteolin were presented in Supplementary Figure S15. Furthermore, to deeply understand the underlying mechanism of QXWWD in the cure of inflammatory disease, the 136 targets were examined for 11 hub genes in the PPI network. Unexpectedly, the PPI network contained six targets (e.g., RELA, AKT1, NFKBIA, MYC, JUN, and MAPK1), which are considered to be the most important genes involved in the 11 hub genes, reconfirming QXWWD might possess good effects against inflammatory disease. Also, we conducted the simulation of molecular docking between six hub genes and two main chemical compounds to complement main targets. The obtained results indicated good docking affinities for all of the pairs of target compounds. Moreover, the pro-inflammatory genes such as RELA, AKT1, JUN, NFKBIA, MYC, and MAPK1 have confirmed the involvement in the onset of inflammatory diseases, particularly, in terms of immune inflammation. The commensal organisms such as anaerobic gut bacteria have been found to regulate the shuttling of RELA and PPAR-gamma between cytoplasm and nucleus and thus play a key role in an inflammatory condition (Kelly et al., 2004). The study reports that AKT1/NF-κB signaling pathways are involved in the production of IL-6, which is widely implicated in the pathogenesis of autoimmune disorders (Zhang et al., 2013). The elevated levels of serum TNF during the inflammatory response were mediated by AKT1/NF-κB signaling pathways, as previously stated (Zhang et al., 2013). Recently, studies demonstrated that c-Jun N-terminal kinase 2 (c-JUN) knockout mice with passive murine collagen lead to Joint damage and inflammation (Han et al., 2002). Besides this, Gene variants in NFKBIA facilitate inflammation and display a crucial role in the event of atherosclerosis and coronary artery disorders (Coto et al., 2019). A study showed that c-Myc is considerably associated with several cellular events including cell proliferation, differentiation, and growth. The c-Myc was found to upregulate the TNF-α, TGF-β, IL-8, and IL-10 whereas, TNF-α, TGF-β, IL-1, IL-2, and IL-4 enhanced c-Myc expression (Nakashima et al., 2005). These interactions depicted a key role in different inflammatory conditions. MAPK1 is involved in several signaling pathways that control a variety of cellular events such as apoptosis and proliferation (Zabihula et al., 2019). As a result, the study's findings revealed that RELA, AKT1, JUN, NFKBIA, MYC, and MAPK1 play an important role in apoptosis, proliferation, and immune inflammation responses and that inhibiting these processes may be an effective therapeutic target for QXWWD against inflammatory disease. The results were further verified by molecular docking. The results revealed that luteolin displayed a strong affinity toward RELA, AKT1, JUN, NFKBIA, and MAPK1, while luteolin had a strong affinity for RELA, AKT1, JUN, NFKBIA, and MYC. As a result, quercetin and luteolin can play a key role in apoptosis, antioxidant activity, angiogenesis, cell cycle, and inflammation. The KEGG enrichment analysis revealed that the main targets were abundantly located in certain signaling pathways like PI3K/AKT, MAPK, TNF, and NF-κB signaling pathways. The findings of the PPI network indicated that RELA, NFKBIA, MYC, MAPK1, MAPK14, ESR1, NR3C1, AKT1, TP53, JUN, and FOS were the possible hub genes. In light of these findings, we hypothesize that QXWWD may regulate inflammatory disease via the following pathways. The PI3K/AKT signaling pathway is mainly attributed to regulate the stimulation of inflammatory response cells and also the release of inflammatory transmitters to perform an important role during the chronic inflammatory response (Zhang et al., 2018). Likewise, the MAPK signaling pathway is responsible for transmitting signals from the cellular membrane toward the nucleus in response to various stimuli. This pathway regulates a broader spectrum of cellular events such as growth, stress responses, and inflammation, thus, it is a broadly implicated therapeutic target for tumor and peripheral inflammatory conditions (Yuan et al., 2017). TNF is a well-known pleiotropic cytokine and has been demonstrated with several important roles in disease pathogenesis as well as homeostasis. The TNF receptor signaling model has also been expanded to include the development of specific signaling complexes, which are commonly linked to functional outcomes such as necroptosis, apoptosis, and inflammation (Borghi et al., 2016). It is worthwhile to mention the role of transcriptional factors belonging to the NF-κB family, which play significant roles in innate immunity and inflammation as well (Sun, 2017). Also, NF-κB is a widely acknowledged key player in several steps linked with the onset and subsequent progression of inflammation (Karin and Greten, 2005). The NF-κB has also been found to facilitate several other signaling pathways and molecules during such a process.
In many instances, inflammation has been appeared to be one of the most complex processes, responsible for several events including tissue breakdown and repair, enzyme activation, cell migration, pro-inflammatory cytokines release, and extravasation of fluid (Castello et al., 2017). As a result, when conducting pharmacological studies, the use of specific experimental models becomes important. Acute inflammation is characterized by the formation of redness, proliferative cells, swelling, heat, and pain (Habtezion, 2015). Therefore, swelling and exudation of fluid are important manifestations of acute inflammation (Ruiz et al., 2019). Xylene can cause ear swelling in mice which leads to fluid accumulation, elevated white blood cell counts and triggers an acute inflammatory response (Zeng et al., 2020). In contrast, chronic inflammation is characterized by the formation of proliferative cells, which occurs when the acute response fails to kill proinflammatory agents (Zhao et al., 2016). In our study, QXWWD was found to significantly decrease ear swelling and white blood cell counts in mice. Thus, the suppression of the said response is quite indicative of the antiphlogistic effect. LPS is one of the key mediators responsible for tissue injuries, which is frequently originating from the infection of Gram-negative bacterial consortia (Cheng et al., 2018). TNF-α is a classic inflammation indicator, which can initiate the production of various inflammatory cytokines including IL-1and IL-6 (Kauppinen et al., 2013). Compared with the model group, 20–80 μg/ml of QXWWD in LPS-induced RAW 264.7 cells greatly inhibited the release of inflammatory mediators, mainly of IL-1, Il-6, and TNF-α in a dose-dependent manner, suggesting that QXWWD was effective in inflammatory conditions.
Bacterial infection is one of the most important causes of inflammation. Recently, knockout mouse models were used to reveal the consequences of pro-inflammatory and tumorigenic properties of the colon’s normal bacterial flora (Yang and Pei., 2006). Therefore, we conducted an antibacterial experiment to evaluate the antibacterial properties of QXWWD. Antibacterial experiments found that QXWWD had inhibitory effects on pathogenic bacteria of Enterococcus faecalis (ATCC29212) (MIC = 250 μg/ml), Staphylococcus aureus (ATCC29213, ATCC25923, ATCC43300) (MIC = 7.8125 μg/ml), Streptococcus pneumoniae (ATCC49619) (MIC = 15.625 μg/ml) but had no obvious inhibitory effect on Streptococcus pyogenes (ATCC19615), Enterobacter cloacae (ATCC700323), Klebsiella pneumoniae (ATCC700603), Escherichia coli (ATCC25922), and Pseudomonas aeruginosa (ATCC27853). Staphylococcus aureus is most frequently isolated from the skin microbiota of dermatitis patients (Geoghegan et al., 2018). Due to Staphylococcus aureus infection, the diversity of the microorganisms is reduced as well as the normal microbiota on the skin is disrupted (Geoghegan et al., 2018). Thus, drugs that inhibiting Staphylococcus aureus with a low MIC can play a good role in the treatment of acute and chronic dermatitis. These results were consistent with the good therapeutic effects of QXWWD in our previous clinical applications. Enterococcus faecalis, one of the most frequently isolated bacterial species in animal wounds, is an anaerobic Gram-positive bacterial that normally commences in the human oral cavity, gastrointestinal tract, and vagina because it has demonstrated good adaptation to such environments with rich nutrient and low oxygen levels (Chong et al., 2017; Alghamdi and Shakir, 2020). Enterococcus faecalis is the most frequently detected bacteria in cases of post-endodontic therapy pain and infection, with prevalence values as high as 90% (Alghamdi and Shakir, 2020). Streptococcus pneumoniae colonizes the human nasopharynx and can cause a variety of diseases, including otitis media, pneumonia, bacteraemia, and meningitis (Mitchell and Mitchell, 2010). The results of antibacterial experiments directly demonstrated that the potential mechanism of the Chinese herbal formula QXWWD in the treatment of bacterial, acute, and chronic dermatitis and provide additional evidence for the promotion of the wide use of QXWWD in the clinic for the treatment of diseases such as periodontitis and bacterial pneumonia.
The comprehensiveness, systematicness and integrity of network pharmacology are in line with the characteristics of multi compound, multi-target and multi-channel of Chinese herbal medicine, and it is expected to become an important tool in the research of traditional Chinese medicine. Despite its broad application prospects, network pharmacology still has some limitations. Firstly, the incomplete existing database will lead to the lack of analysis data. Secondly, the current network pharmacology technology is difficult to achieve the goal of quantification. Finally, most studies based on network pharmacology are still static network analysis. Therefore, a large number of in vivo or in vitro experiments are needed. In the future, with the popularization of network pharmacology technology, an ultra high throughput, fast and non-destructive method can be found to effectively solve the above limitations.
Conclusions
Based on the network pharmacology and molecular docking analysis, the underlying mechanism of QXWWD in dermatitis therapy involves the regulation of pathways and targets in multiple biological processes. The antibacterial and anti-inflammatory effects of QXWWD in bacteria, cells, and animal models seem to be mediated by inhibition of the release of pro-inflammatory cytokines including TNF-α, IL-1, IL-6, and regulation of NF-κB, ERK, P38 MAPK, and PI3K-Akt signaling pathways. Overall, the obtained results suggested that QXWWD might be used as a promising therapeutic agent for bacterial, acute, and chronic dermatitis and provide additional evidence for the promotion of the wide use of QXWWD in the clinic for the treatment of diseases such as periodontitis and bacterial pneumonia.
Data Availability Statement
The raw data supporting the conclusions of this article will be made available by the authors, without undue reservation, to any qualified researcher.
Ethics Statement
The animal study was reviewed and approved by Ethical Committee of Inner Mongolia Medical University.
Author Contributions
QZ carried out antibacterial activity test, RAW264.7 cell culture, measurement of pro-inflammatory cytokines and western blot analysis, searched data in electronic databases and wrote the manuscript. XL carried out thin layer chromatography, searched data in electronic databases, processed the data and analyses. JunL carried out preparation of QXWWD, qualitative analysis of QXWWD extracts by using HPLC and Q Exactive MS. YH searched data in electronic databases, processed the data and analyses. JL and WZ searched data in electronic databases and conducted the network pharmacology and molecular docking analysis. FW carried out animal expriments and HE staining, processed the data and analyses. FC designed the experiment and supervised the project. All authors have read and approved the manuscript.
Funding
This study was financially supported by the Natural Science Foundation of Inner Mongolia (No. 2021MS08087), the Higher Education Scientific Research Project of Inner Mongolia Autonomous Region (No.NJZY21632), the Natural Science Foundation of China (No. 81760676) and the Science and Technology Million Fund of Inner Mongolia Medical University (No. YKD2018JKBW011, YKD2020JKBW020).
Conflict of Interest
The authors declare that the research was conducted in the absence of any commercial or financial relationships that could be construed as a potential conflict of interest.
Supplementary Material
The Supplementary Material for this article can be found online at: https://www.frontiersin.org/articles/10.3389/fphar.2021.678685/full#supplementary-material
References
Ahmad, A., Wali, A. F., Rehman, M. U., Khan, A., Raish, M., Kazi, M., et al. (2020). Therapeutic Potential of Rhododendron Arboreum Polysaccharides in an Animal Model of Lipopolysaccharide-Inflicted Oxidative Stress and Systemic Inflammation. Molecules 25 (24), 6045. doi:10.3390/molecules25246045
Alghamdi, F., and Shakir, M. (2020). The Influence of Enterococcus faecalis as a Dental Root Canal Pathogen on Endodontic Treatment: A Systematic Review. Cureus 12 (3), e7257. doi:10.10.7759/cureus.7257
Bacchi, S., Palumbo, P., Sponta, A., and Coppolino, M. F. (2012). Clinical Pharmacology of Non-steroidal Anti-inflammatory Drugs: a Review. Aiaamc 11 (1), 52–64. doi:10.2174/187152312803476255
Bindu, S., Mazumder, S., and Bandyopadhyay, U. (2020). Non-steroidal Anti-inflammatory Drugs (NSAIDs) and Organ Damage: A Current Perspective. Biochem. Pharmacol. 180, 114147. doi:10.1016/j.bcp.2020.114147
Borghi, A., Verstrepen, L., and Beyaert, R. (2016). TRAF2 Multitasking in TNF Receptor-Induced Signaling to NF-Κb, MAP Kinases and Cell Death. Biochem. Pharmacol. 116, 1–10. doi:10.1016/j.bcp.2016.03.009
Castello, L. M., Raineri, D., Salmi, L., Clemente, N., Vaschetto, R., Quaglia, M., et al. (2017). Osteopontin at the Crossroads of Inflammation and Tumor Progression. Mediators Inflamm. 2017, 1–22. doi:10.1155/2017/4049098
Che, D. N., Cho, B. O., Kim, J.-s., Shin, J. Y., Kang, H. J., and Jang, S. I. (2020). Luteolin and Apigenin Attenuate LPS-Induced Astrocyte Activation and Cytokine Production by Targeting MAPK, STAT3, and NF-Κb Signaling Pathways. Inflammation 43 (5), 1716–1728. doi:10.1007/s10753-020-01245-6
Chen, L.-L., Zhang, H.-J., Chao, J., and Liu, J.-F. (2017). Essential Oil of Artemisia Argyi Suppresses Inflammatory Responses by Inhibiting JAK/STATs Activation. J. Ethnopharmacology 204, 107–117. doi:10.1016/j.jep.2017.04.017
Cheng, N., Liang, Y., Du, X., and Ye, R. D. (2018). Serum Amyloid A Promotes LPS Clearance and Suppresses LPS ‐induced Inflammation and Tissue Injury. EMBO Rep. 19 (10), e45517. doi:10.15252/embr.201745517
Cheng, X., and Merz, K.-H. (2016). The Role of Indirubins in Inflammation and Associated Tumorigenesis. Adv. Exp. Med. Biol. 929, 269–290. doi:10.1007/978-3-319-41342-6_12
Choi, E. M., and Lee, Y. S. (2010). Luteolin Suppresses IL-1β-induced Cytokines and MMPs Production via P38 MAPK, JNK, NF-kappaB and AP-1 Activation in Human Synovial Sarcoma Cell Line, SW982. Food Chem. Toxicol. 48 (10), 2607–2611. doi:10.1016/j.fct.2010.06.029
Chong, K. K. L., Tay, W. H., Janela, B., Yong, A. M. H., Liew, T. H., Madden, L., et al. (2017). Enterococcus faecalis Modulates Immune Activation and Slows Healing during Wound Infection. J. Infect. Dis. 216 (12), 1644–1654. doi:10.1093/infdis/jix541
Coto, E., Reguero, J. R., Avanzas, P., Pascual, I., Martín, M., Hevia, S., et al. (2019). Gene Variants in the NF-KB Pathway (NFKB1, NFKBIA, NFKBIZ) and Risk for Early-Onset Coronary Artery Disease. Immunol. Lett. 208, 39–43. doi:10.1016/j.imlet.2019.02.007
Dai, J.-P., Chen, J., Bei, Y.-F., Han, B.-X., and Wang, S. (2009). Influence of Borneol on Primary Mice Oral Fibroblasts: a Penetration Enhancer May Be Used in Oral Submucous Fibrosis. J. Oral Pathol. Med. 38 (3), 276–281. doi:10.1111/j.1600-0714.2008.00738.x
Diaz, D., Lopez-Dolado, E., Haro, S., Monserrat, J., Martinez-Alonso, C., Balomeros, D., et al. (2021). Systemic Inflammation and the Breakdown of Intestinal Homeostasis Are Key Events in Chronic Spinal Cord Injury Patients. Ijms 22 (2), 744. doi:10.3390/ijms22020744
Dona, I., Salas, M., Perkins, J., Barrionuevo, E., Gaeta, F., Cornejo-Garcia, J., et al. (2017). Hypersensitivity Reactions to Non-steroidal Anti-inflammatory Drugs. Cpd 22 (45), 6784–6802. doi:10.2174/1381612822666160928142814
Espinosa, A., Paz-y-Miño-C, G., Santos, Y., Ma, H., Nadeau, M., Seeram, N. P., et al. (2020). Anti-amebic Effects of Chinese Rhubarb (Rheum Palmatum) Leaves' Extract, the Anthraquinone Rhein and Related Compounds. Heliyon 6 (4), e03693, e03693. doi:10.1016/j.heliyon.2020.e03693
Geoghegan, J. A., Irvine, A. D., and Foster, T. J. (2018). Staphylococcus aureus and Atopic Dermatitis: A Complex and Evolving Relationship. Trends Microbiol. 26 (6), 484–497. doi:10.1016/j.tim.2017.11.008
Habtezion, A. (2015). Inflammation in Acute and Chronic Pancreatitis. Curr. Opin. Gastroenterol. 31 (5), 395–399. doi:10.1097/MOG.0000000000000195
Han, Z., Chang, L., Yamanishi, Y., Karin, M., and Firestein, G. S. (2002). Joint Damage and Inflammation in C-Jun N-Terminal Kinase 2 Knockout Mice with Passive Murine Collagen-Induced Arthritis. Arthritis Rheum. 46 (3), 818–823. doi:10.1002/art.10104
Harirforoosh, S., Asghar, W., and Jamali, F. (2013). Adverse Effects of Nonsteroidal Antiinflammatory Drugs: an Update of Gastrointestinal, Cardiovascular and Renal Complications. J. Pharm. Pharm. Sci. 16 (5), 821–847. doi:10.18433/j3vw2f
Harris, R., Beebe, J., and Alshafie, G. A. (2012). Reduction in Cancer Risk by Selective and Nonselective Cyclooxygenase-2 (COX-2) Inhibitors. Jep 4, 91–96. doi:10.2147/JEP.S23826
Harris, R. E., Beebe-Donk, J., Doss, H., and Burr Doss, D. (2005). Aspirin, Ibuprofen, and Other Non-steroidal Anti-inflammatory Drugs in Cancer Prevention: a Critical Review of Non-selective COX-2 Blockade (Review). Oncol. Rep. 13 (4), 559–583.
Heinrich, M., Appendino, G., Efferth, T., Fürst, R., Izzo, A. A., Kayser, O., et al. (2020). Best Practice in Research - Overcoming Common Challenges in Phytopharmacological Research. J. Ethnopharmacology 246, 112230. doi:10.1016/j.jep.2019.112230
Karin, M., and Greten, F. R. (2005). NF-κB: Linking Inflammation and Immunity to Cancer Development and Progression. Nat. Rev. Immunol. 5 (10), 749–759. doi:10.1038/nri1703
Kauppinen, A., Suuronen, T., Ojala, J., Kaarniranta, K., and Salminen, A. (2013). Antagonistic Crosstalk between NF-Κb and SIRT1 in the Regulation of Inflammation and Metabolic Disorders. Cell Signal. 25 (10), 1939–1948. doi:10.1016/j.cellsig.2013.06.007
Kelly, D., Campbell, J. I., King, T. P., Grant, G., Jansson, E. A., Coutts, A. G. P., et al. (2004). Commensal Anaerobic Gut Bacteria Attenuate Inflammation by Regulating Nuclear-Cytoplasmic Shuttling of PPAR-γ and RelA. Nat. Immunol. 5 (1), 104–112. doi:10.1038/ni1018
Ma, H., Huang, Q., Qu, W., Li, L., Wang, M., Li, S., et al. (2018). In Vivo and In Vitro Anti-inflammatory Effects of Sophora Flavescens Residues. J. Ethnopharmacology 224, 497–503. doi:10.1016/j.jep.2018.06.019
Marjoribanks, J., Ayeleke, R. O., Farquhar, C., and Proctor, M. (2015). Nonsteroidal Anti-inflammatory Drugs for Dysmenorrhoea. Cochrane Database Syst. Rev. 2015 (7), CD001751. doi:10.1002/14651858.CD001751.pub3
Marquez-Lara, A., Hutchinson, I. D., Nuñez, F., Smith, T. L., and Miller, A. N. (2016). Nonsteroidal Anti-inflammatory Drugs and Bone-Healing. JBJS Rev. 4 (3), 01874474–201603000-00005. doi:10.2106/JBJS.RVW.O.00055
Mitchell, A. M., and Mitchell, T. J. (2010). Streptococcus Pneumoniae: Virulence Factors and Variation. Clin. Microbiol. Infect. 16 (5), 411–418. doi:10.1111/j.1469-0691.2010.03183.x
Nakashima, A., Kumakura, S., Mishima, S., Ishikura, H., and Kobayashi, S. (2005). IFN-alpha Enhances TNF-Alpha-Induced Apoptosis through Down-Regulation of C-Myc Protein Expression in HL-60 Cells. J. Exp. Clin. Cancer Res. 24 (3), 447–456.
Paolucci, E. M., Loukov, D., Bowdish, D. M. E., and Heisz, J. J. (2018). Exercise Reduces Depression and Inflammation but Intensity Matters. Biol. Psychol. 133, 79–84. doi:10.1016/j.biopsycho.2018.01.015
Renda, G., Tacconelli, S., Capone, M., Sacchetta, D., Santarelli, F., Sciulli, M., et al. (2006). Celecoxib, Ibuprofen, and the Antiplatelet Effect of Aspirin in Patients with Osteoarthritis and Ischemic Heart Disease. Clin. Pharmacol. Ther. 80 (3), 264–274. doi:10.1016/j.clpt.2006.05.004
Ruiz, F., Vigne, S., and Pot, C. (2019). Resolution of Inflammation during Multiple Sclerosis. Semin. Immunopathol. 41 (6), 711–726. doi:10.1007/s00281-019-00765-0
Sun, S.-C. (2017). The Non-canonical NF-Κb Pathway in Immunity and Inflammation. Nat. Rev. Immunol. 17 (9), 545–558. doi:10.1038/nri.2017.52
Wang, H. T., Fang, Y. Q., Bao, X. C., Yuan, H. R., Ma, J., Wang, F. F., et al. (2015). Expression Changes of TNF-α, IL-1β and IL-6 in the Rat Lung of Decompression Sickness Induced by Fast Buoyancy Ascent Escape. Undersea Hyperb. Med. 42 (1), 23–31.
Yang, L., and Pei, Z. (2006). Bacteria, Inflammation, and colon Cancer. Wjg 12 (42), 6741–6746. doi:10.3748/wjg.v12.i42.6741
Yuan, P., Zheng, X., Li, M., Ke, Y., Fu, Y., Zhang, Q., et al. (2017). Two sulfur glycoside compounds isolated from Lepidium apetalum willd protect NRK52e cells against hypertonic-induced adhesion and inflammation by suppressing the MAPK signaling pathway and RAAS. Molecules 22 (11), 1956. doi:10.3390/molecules22111956
Zabihula, B., Yiliyasi, M., Lu, Y., and Salai, A. (2019). MicroRNA-490-3p I-nhibits P-roliferation and S-timulates A-poptosis of ESCC C-ells via MAPK1 D-ownregulation. Oncol. Lett. 18 (3), 3170–3176. doi:10.3892/ol.2019.10636
Zappavigna, S., Cossu, A. M., Grimaldi, A., Bocchetti, M., Ferraro, G. A., Nicoletti, G. F., et al. (2020). Anti-inflammatory Drugs as Anticancer Agents. Ijms 21 (7), 2605. doi:10.3390/ijms21072605
Zeng, A. Q., Hua, H., Chen, C. R., Liu, L., Zhang, M., Luo, Y., et al. (2020). [Comparative Study on Anti-inflammatory Effect of Lonicerae Japonicae Flos and Lonicerae Flos]. Zhongguo Zhong Yao Za Zhi 45 (16), 3938–3944. doi:10.19540/j.cnki.cjcmm.20200520.401
Zhang, H., Chen, J., Liu, X., Awar, L., McMickle, A., Bai, F., et al. (2013). IL-17 Induces Expression of Vascular Cell Adhesion Molecule through Signalling Pathway of NF-Κb, but Not Akt1 and TAK1 in Vascular Smooth Muscle Cells. Scand. J. Immunol. 77 (4), 230–237. doi:10.1111/sji.12030
Zhang, H. X., Yang, J. J., Zhang, S. A., Zhang, S. M., Wang, J. X., Xu, Z. Y., et al. (2018). HIF-1α Promotes Inflammatory Response of Chronic Obstructive Pulmonary Disease by Activating EGFR/PI3K/AKT Pathway. Eur. Rev. Med. Pharmacol. Sci. 22 (18), 6077–6084. doi:10.26355/eurrev_201809_15946
Zhang, L., Ravipati, A. S., Koyyalamudi, S. R., Jeong, S. C., Reddy, N., Bartlett, J., et al. (2013). Anti-fungal and Anti-bacterial Activities of Ethanol Extracts of Selected Traditional Chinese Medicinal Herbs. Asian Pac. J. Trop. Med. 6 (9), 673–681. doi:10.1016/S1995-7645(13)60117-0
Zhang, M., Lin, J. M., Li, X. S., and Li, J. (2016). Quercetin Ameliorates LPS-Induced Inflammation in Human Peripheral Blood Mononuclear Cells by Inhibition of the TLR2-NF-Κb Pathway. Genet. Mol. Res. 15 (2), gmr8279. doi:10.4238/gmr.15028297
Zhao, C.-q., Zhou, Y., Ping, J., and Xu, L.-m. (2014). Traditional Chinese Medicine for Treatment of Liver Diseases: Progress, Challenges and Opportunities. J. Integr. Med. 12 (5), 401–408. doi:10.1016/S2095-4964(14)60039-X
Zhao, R., Liang, H., Clarke, E., Jackson, C., and Xue, M. (2016). Inflammation in Chronic Wounds. Ijms 17 (12), 2085. doi:10.3390/ijms17122085
Zhao, Y., Xiao, X., Frank, S. J., Lin, H. Y., and Xia, Y. (2014). Distinct Mechanisms of Induction of Hepatic Growth Hormone Resistance by Endogenous IL-6, TNF-α, and IL-1β. Am. J. Physiology-Endocrinology Metab. 307 (2), E186–E198. doi:10.1152/ajpendo.00652.2013
Keywords: QingXiaoWuWei Decoction, anti-inflammatory, antibacterial, network pharmacology, molecular docking, HPLC-Q-Exactive-MS
Citation: Zhang Q, Li X, Li J, Hu Y, Liu J, Wang F, Zhang W and Chang F (2021) Mechanism of Anti-Inflammatory and Antibacterial Effects of QingXiaoWuWei Decoction Based on Network Pharmacology, Molecular Docking and In Vitro Experiments. Front. Pharmacol. 12:678685. doi: 10.3389/fphar.2021.678685
Received: 10 March 2021; Accepted: 21 June 2021;
Published: 15 July 2021.
Edited by:
Fang-Rong Chang, Kaohsiung Medical University, TaiwanReviewed by:
Christian Agyare, Kwame Nkrumah University of Science and Technology, GhanaJinfan Tian, Capital Medical University, China
Copyright © 2021 Zhang, Li, Li, Hu, Liu, Wang, Zhang and Chang. This is an open-access article distributed under the terms of the Creative Commons Attribution License (CC BY). The use, distribution or reproduction in other forums is permitted, provided the original author(s) and the copyright owner(s) are credited and that the original publication in this journal is cited, in accordance with accepted academic practice. No use, distribution or reproduction is permitted which does not comply with these terms.
*Correspondence: Fuhou Chang, Y2hhbmdmdWhvdUAxMjYuY29t
†These authors have contributed equally to this work and share first authorship