- 1Department of Anesthesiology, Shengjing Hospital of China Medical University, Shenyang, China
- 2Department of Obstetrics and Gynecology, Shengjing Hospital of China Medical University, Shenyang, China
Background: Due to the absence of direct comparisons of different therapeutic drugs in preventing opioid-induced cough (OIC) during the induction of general anesthesia, clinicians often faced difficulties in choosing the optimal drug for these patients. Hence, this network meta-analysis was conducted to solve this problem.
Methods: Online databases, including Pubmed, Embase, Web of Science, Cochrane, and Google Scholar, were searched comprehensively to identify eligible randomized controlled trials (RCTs), up to March 15th, 2021. Within a Bayesian framework, network meta-analysis was performed by the “gemtc” version 0.8.2 package of R-3.4.0 software, and a pooled risk ratio (RR) associated with 95% credible interval (CrI) was calculated.
Results: A total of 20 RCTs were finally enrolled, and the overall heterogeneity for this study was low to moderate. Traditional pair-wise meta-analysis results indicated that all of the five drugs, namely, lidocaine, ketamine, dezocine, butorphanol, and dexmedetomidine could prevent OIC for four clinical outcomes, compared with the placebo (all p-values < 0.05). Moreover, dezocine had the best effect, compared with that of the other drugs (all p-values < 0.05). Network meta-analysis results suggested that the top three rank probabilities for four clinical outcomes from best to worst were dezocine, butorphanol, and ketamine based on individual/cumulative rank plots and surface under the cumulative ranking curve (SUCRA) probabilities. The node-splitting method indicated the consistency of the direct and indirect evidence.
Conclusions: Our results indicated that all of these five drugs could prevent OIC compared with the placebo. Moreover, the top three rank probabilities for four clinical outcomes from best to worst were dezocine, butorphanol, and ketamine. Our results were anticipated to provide references for guiding clinical research, and further high-quality RCTs were required to verify our findings.
Systematic Review Registration: [https://www.crd.york.ac.uk/prospero/], identifier [CRD42021243358].
Introduction
Due to the rapid onset, short duration, strong analgesia, and reduced cardiovascular response, opioids such as sufentanil, fentanyl, and remifentanil have been widely applied in the induction and maintenance of general anesthesia (Liu et al., 2014; Shuying et al., 2016). However, the complication of opioid-induced cough (OIC) is frequently encountered during the induction of anesthesia, with an incidence rate as high as 65% (Sun et al., 2014). Although most OIC is transient, light, and self-limiting, it is a risk factor for postoperative nausea and vomiting (Peringathara and Robinson, 2016) and is extremely dangerous for patients with comorbidities, such as brain hernia, increased intracranial pressure, increased ocular pressure, open eye injury, pneumothorax, and hypersensitive airway disease (Lin et al., 2005; Yu et al., 2007). Hence, there is an urgent need to take measures to prevent the occurrence of OIC during induction of general anesthesia.
Numerous pharmacological or non-pharmacological measures have been taken to prevent OIC. Therein, non-pharmacological measures are characterized by diluting drug concentration, slowing down injection rate, using the peripheral injection site, reducing the drug dose, instructing patients on performing the huffing maneuver, and verifying the proper administration sequence of the drug (Ambesh et al., 2010; Min et al., 2012; Shrestha et al., 2012; Xiong et al., 2020). Currently, pharmacological interventions have been widely used in the clinical setting, including lidocaine, dezocine, dexmedetomidine, ketamine, and butorphanol (Shuying et al., 2016; Zhang et al., 2018; Clivio et al., 2019). However, most of these articles were compared with the placebo, and direct comparisons of different pharmacological interventions were absent, along with the application of novel drugs. As a result, it was much harder for clinical physicians to choose the optimal therapeutic drug.
As is already known, network meta-analysis could overcome the limitations of traditional meta-analysis and gain evidence directly and indirectly (Lumley, 2002; Zhang et al., 2019). Hence, we applied a Bayesian network meta-analysis of randomized controlled trials (RCTs) to evaluate the efficacy of different pharmacological interventions for OIC. In this article, five different therapeutic drugs, namely, lidocaine, ketamine, dezocine, butorphanol, and dexmedetomidine were finally enrolled. Four clinical outcomes comprising incidence of OIC, mild severity of OIC, moderate severity of OIC, and severe severity of OIC were ultimately evaluated. Our results were anticipated to provide some references for guiding clinical practice.
Materials and Methods
Search Strategy
This network meta-analysis was carried out based on the Preferred Reporting Items for Systematic Reviews and Meta-Analysis (PRISMA) guidelines (Page et al., 2021). Online databases, including Pubmed, Embase, Web of Science, Cochrane, and Google Scholar, were searched comprehensively to identify eligible randomized controlled trials (RCTs) up to March 15th, 2021. Our search strategy was mainly comprised three parts utilizing the following keywords in combination with the following Medical Subject Heading (MeSH) terms and text words: “therapeutic drugs”, “lidocaine”, “ketamine”, “dezocine”, “butorphanol”, or “dexmedetomidine” and “opioid-induced cough”, “sufentanil-induced cough”, “fentanyl-induced cough”, or “remifentanil-induced cough” and (“randomized controlled trials”). Additional articles were manually screened from the reference lists of eligible studies to avoid omissions. This network meta-analysis was registered in PROSPERO (https://www.crd.york.ac.uk/prospero/) with the registration number “CRD42021243358”.
Inclusion and Exclusion Criteria
The inclusion criteria in this article were displayed as following: 1) English articles; 2) Randomized controlled trials; 3) At least two of six drugs (lidocaine, ketamine, dezocine, butorphanol, dexmedetomidine, and placebo) should be compared; 4) At least one of four clinical outcomes (incidence of OIC, mild severity of OIC, moderate severity of OIC, and severe severity of OIC) should be evaluated; and 5) Data could be extracted from articles; The exclusion criteria were detailed as follows: 1)Non-English articles; 2) Non-randomized controlled trials; 3) Articles that did not compare at least two of these six drugs; 4) Articles that did not evaluate at least one of four clinical outcomes; and 5) Data could not be extracted from articles;
Data Extraction
Two blind reviewers independently identified the data of eligible studies, based on our inclusion and exclusion criteria. When any discrepancy existed, we would discuss with a third reviewer to solve this problem. Moreover, we would record the following information for further analysis: the first author’s name of the study, publication year, American Society of Anesthesiologist (ASA) physical status, injection, study type, opioid, treatment, incidence of OIC, mild severity of OIC, moderate severity of OIC, and severe severity of OIC.
Quality Assessment
In this article, the potential source of bias of eligible RCTs would be evaluated based on the Cochrane Handbook (http://www.cochrane-handbook.org) (Higgins et al., 2019), containing the following seven aspects of bias: 1) Random sequence generation (selection bias); 2) Allocation concealment (selection bias); 3) Blinding of participants and personnel (performance bias); 4) Blinding of outcome assessment (detection bias); 5) Incomplete outcome data (attrition bias); 6) Selective reporting (reporting bias); and 7) Other bias. Finally, each aspect would be graded as a low, high, or unclear risk of bias.
Statistical Analysis
Within a Bayesian framework, network meta-analysis comprising different therapeutic drugs was performed by the version 0.8.2 “gemtc” package of R software (version 3.4.0; R Foundation, Vienna, Austria) (Lumley, 2002; Valkenhoef and Kuiper, 2016). A non-informative prior distribution was utilized in this Bayesian analysis, and posterior distribution was estimated by Gibbs sampling using the Markov chain Monte Carlo method (Ando et al., 2020; Cha et al., 2021). When three Markov chains run simultaneously, 10,000 simulations and 40,000 iterations were set by us for each chain to calculate the risk ratio (RR) with 95% credible interval (CrI) of model parameters by the mtc.run function. The Brooks–Gelman–Rubin plot, trace plot, and density plot mehods were utilized to assess the model convergence (Wu et al., 2013). Moreover, we would simultaneously obtain the matrix and the plot of rank probabilities, provided by the R package of “gemtc”. When a loop connecting three arms existed, the node-splitting method was utilized to access the inconsistency by reporting its Bayesian p-value (Dias et al., 2010; Wang et al., 2018). To evaluate the heterogeneity, the mtc. anohe command of the R package of “gemtc” was utilized by reporting the heterogeneity variance parameter I2. I2 > 50% was regarded as significant heterogeneity, and the random effects models would be utilized; otherwise the fixed effect models would be utilized (Ma et al., 2021; Zhou et al., 2021). Sensitivity analysis was also conducted to examine the robustness of our results. In summary, all p-values were adopted by a two-sided test, and p-value < 0.05 was considered to be statistically significant.
Results
Search Results and Study Characteristics
A total of 877 citations were yielded by searching five online databases (Pubmed, Embase, Web of Science, Cochrane, and Google Scholar) by our search strategy. Based on the inclusion and exclusion criteria, 20 RCTs were finally identified and considered eligible for this network meta-analysis (Figure 1) (Lin et al., 2004; Pandey et al., 2004; Pandey et al., 2005; Yeh et al., 2007; Kim et al., 2008; Kim et al., 2009; Bang et al., 2010; Guler et al., 2010; Sun et al., 2011; He et al., 2012; Yu et al., 2012; Gecaj-Gashi et al., 2013; Honarmand et al., 2013; Sun and Huang, 2013; Saleh et al., 2014; Liu et al., 2015; Cheng et al., 2016; Naldan et al., 2019; Yin and Zhang, 2019; Zhou et al., 2019). Moreover, Table 1 summarizes the detailed information of individual studies enrolled in this network meta-analysis. As for quality assessment, all of the 20 RCTs were evaluated based on the Cochrane Handbook (http://www.cochrane-handbook.org) and graded each potential source of bias as low, high, or unclear risk of bias (Supplement Supplementary Figures S1, S2). Moreover, the PRISMA 2020 checklist and PRISMA 2020 for abstract checklist are displayed in Supplementary Tables S1, S2, respectively.
Network Structure Diagrams
In this article, five different therapeutic drugs, namely, lidocaine, ketamine, dezocine, butorphanol, and dexmedetomidine were finally enrolled. Four clinical outcomes comprising incidence of OIC, mild severity of OIC, moderate severity of OIC, and severe severity of OIC were ultimately evaluated. As displayed in Figure 2, the network structure diagrams detailed the direct comparisons between different drugs in the four clinical outcomes, respectively. Besides, the numbers showed the number of direct comparisons. Line thicknesses were proportional to the number of direct comparisons. Circle diameters were proportional to the treatment numbers included in this network meta-analysis.
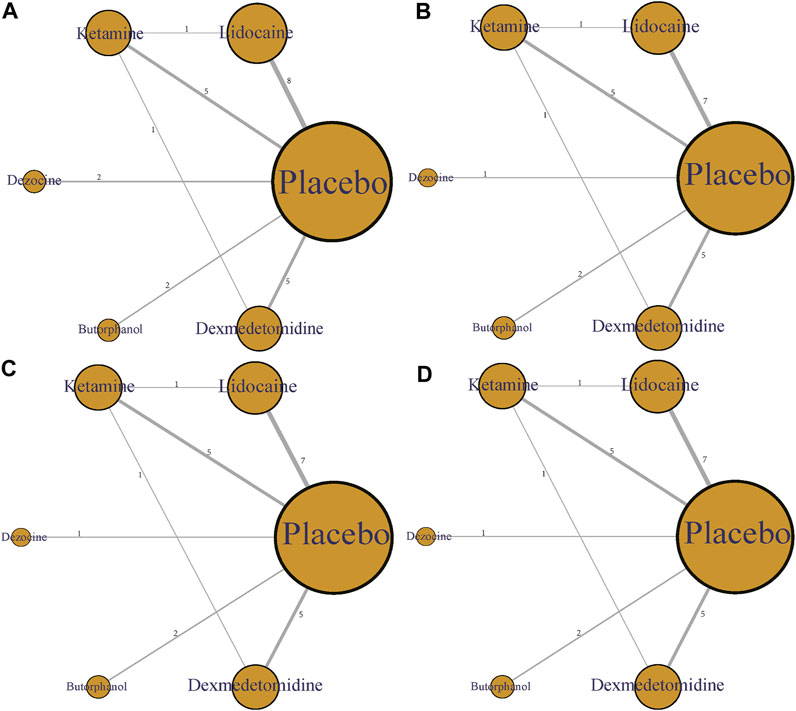
FIGURE 2. Network structure diagrams. (A) Incidence of OIC; (B) Mild severity of OIC; (C) Moderate severity of OIC; and (D) Severe severity of OIC. The numbers showed the number of direct comparisons. Line thicknesses were proportional to the number of direct comparisons. Circle diameters were proportional to the treatment numbers.
Incidence of OIC
A total of 20 RCTs, including six drugs (lidocaine, ketamine, dezocine, butorphanol, dexmedetomidine, and placebo) contributed to the clinical outcome of the incidence of OIC. As displayed in Figure 3A and Supplementary Figure S3A, it detailed the efficacy of different comparisons of drugs by RRs and corresponding 95% CrIs. We could easily find that all of the five drugs (lidocaine, ketamine, dezocine, butorphanol, and dexmedetomidine) could prevent the incidence of OIC, compared with the placebo (all p-values < 0.05). Moreover, dezocine had the best effect, compared with that of the other drugs (all-values p < 0.05). Figure 4A summarizes the heterogeneity between different comparisons of drugs. Individual and cumulative rank plots indicated that the rank probability for the incidence of OIC from best to worst was dezocine, butorphanol, ketamine, dexmedetomidine, lidocaine, and placebo (Figure 5A, Figure 6A). Moreover, their surface under the cumulative ranking curve (SUCRA) probabilities of six drugs for the incidence of OIC are also presented in Figure 7A; Table 2. Additionally, p- values of the node-splitting method between ketamine vs lidocaine were below 0.05, indicating the inconsistency of the direct and indirect evidence. P-values values of the node-splitting method between dexmedetomidine vs. ketamine were above 0.05, suggesting the consistency of the direct and indirect evidence (Figure 8A). Sensitivity analysis was also conducted as shown in Supplementary Figure S4A, indicating the robustness of our results.
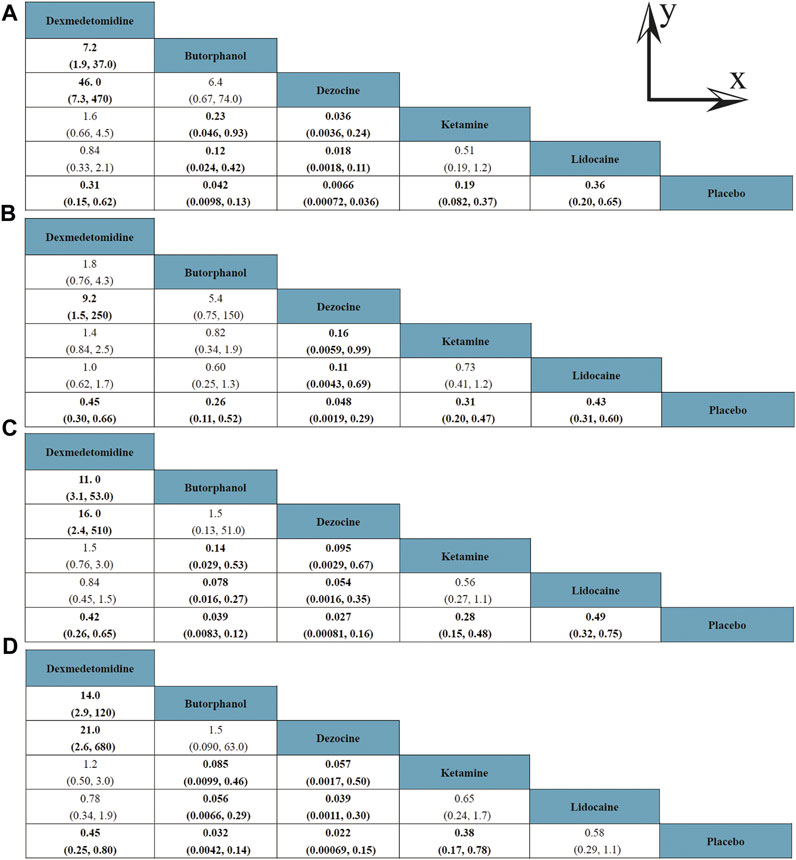
FIGURE 3. Efficacy of different comparisons of drugs by RRs and corresponding 95% CrIs; (A) Incidence of OIC; (B) Mild severity of OIC; (C) Moderate severity of OIC; and (D) Severe severity of OIC. All results were displayed as the ratio of the Y axis versus X axis. Bold fonts indicated p-value < 0.05.
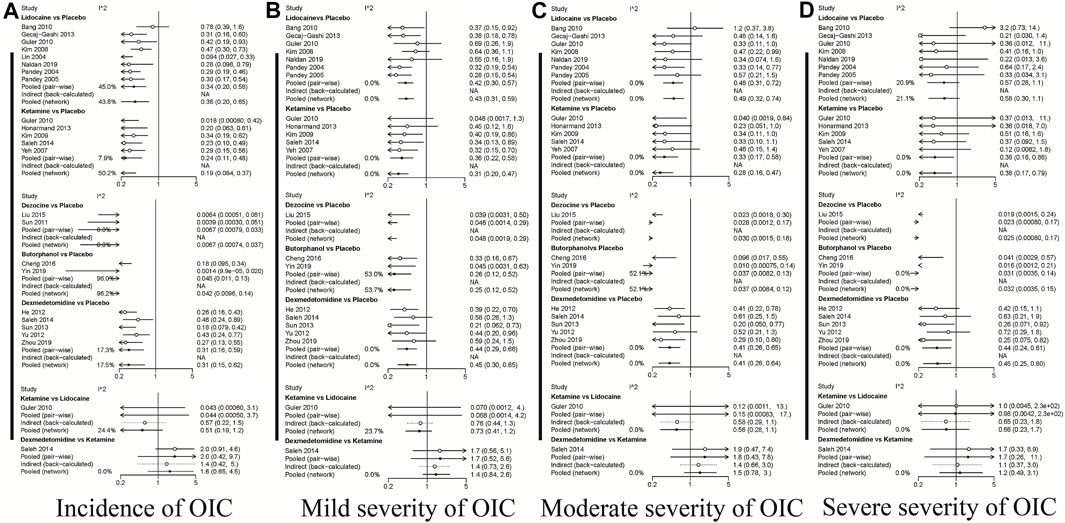
FIGURE 4. Heterogeneity between different comparisons of drugs. (A) Incidence of OIC; (B) Mild severity of OIC; (C) Moderate severity of OIC; and (D) Severe severity of OIC.
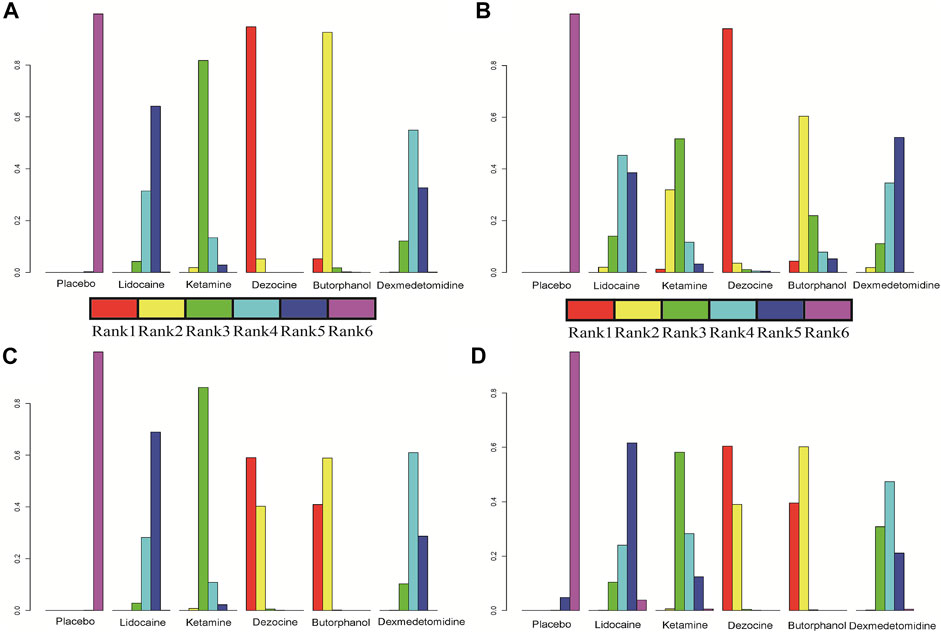
FIGURE 5. Individual rank plot for four clinical outcomes. (A) Incidence of OIC; (B) Mild severity of OIC; (C) Moderate severity of OIC; and (D) Severe severity of OIC.
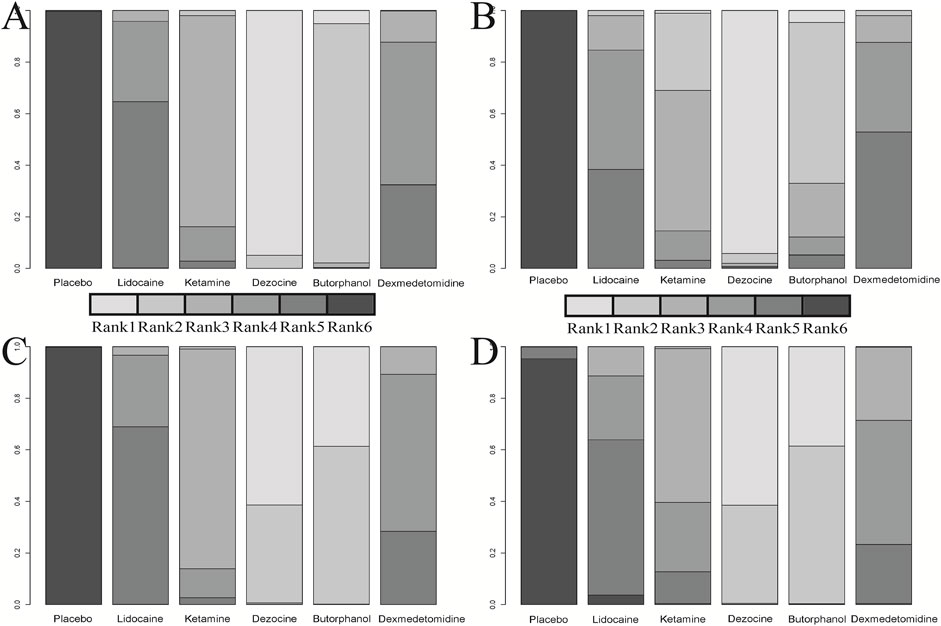
FIGURE 6. Cumulative rank plot for four clinical outcomes. (A) Incidence of OIC; (B) Mild severity of OIC; (C) Moderate severity of OIC; and (D) Severe severity of OIC.
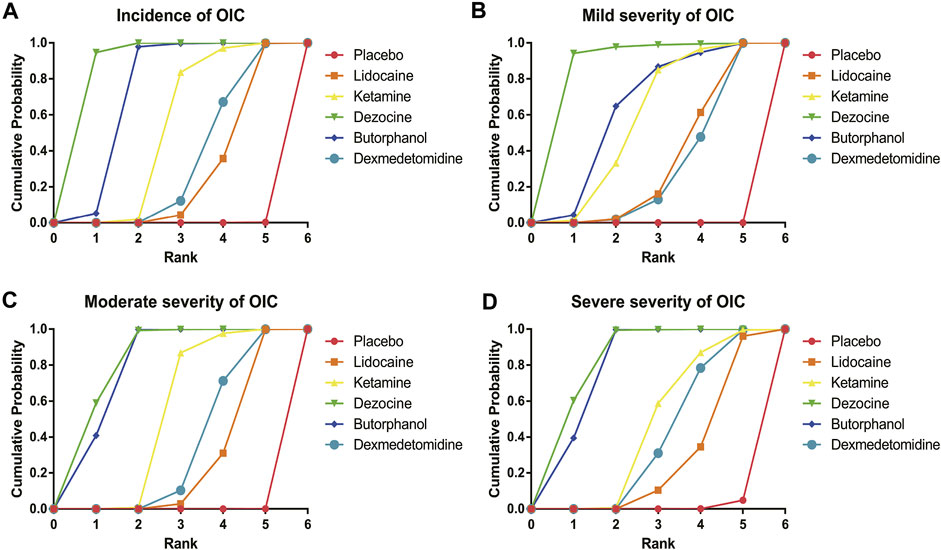
FIGURE 7. Surface under the cumulative ranking curve (SUCRA) probabilities of different drugs for four clinical outcomes. (A) Incidence of OIC; (B) Mild severity of OIC; (C) Moderate severity of OIC; and (D) Severe severity of OIC.

TABLE 2. Surface under the cumulative ranking curve (SUCRA) probabilities of different drugs for four clinical outcomes.
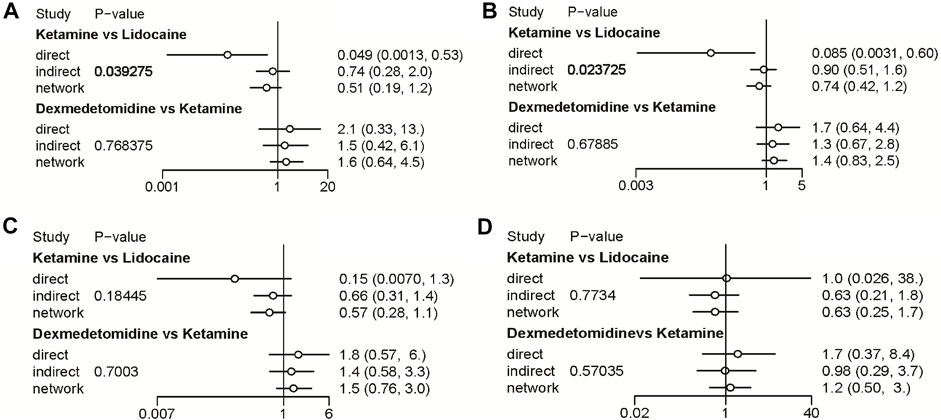
FIGURE 8. Node-splitting method in comparisons between direct and indirect evidence. (A) Incidence of OIC; (B) Mild severity of OIC; (C) Moderate severity of OIC; and (D) Severe severity of OIC.
Mild Severity of OIC
18 RCTs contributed to the analysis of mild severity of OIC, including six drugs, namely, lidocaine, ketamine, dezocine, butorphanol, dexmedetomidine, and placebo. Figure 3B; Supplementary Figure S3B details the efficacy of different comparisons of drugs by RRs and corresponding 95% CrIs. Similar to previous results, all of the five drugs (lidocaine, ketamine, dezocine, butorphanol, and dexmedetomidine) could reduce mild severity of OIC, compared with that of the placebo (all p-values < 0.05). Moreover, dezocine had the best effect compared with that of other drugs (all p-values < 0.05). Heterogeneity between different comparisons of drugs is summarized in Figure 4B. Individual and cumulative rank plots explained that the rank probability for mild severity of OIC from first to last was dezocine, butorphanol, ketamine, lidocaine, dexmedetomidine, and placebo (Figure 5B, Figure 6B). Moreover, their surface under the cumulative ranking curve (SUCRA) probabilities of the six drugs for mild severity of OIC are also shown in Figure 7B; Table 2. Besides, p-values of the node-splitting method between ketamine vs. lidocaine were below 0.05, indicating the inconsistency of the direct and indirect evidence. P-values of the node-splitting method between dexmedetomidine vs. ketamine were more than 0.05, suggesting the consistency of the direct and indirect evidence (Figure 8B). Sensitivity analysis was also conducted as shown in Supplementary Figure S4B, indicating the robustness of our results.
Moderate Severity of OIC
There were 18 RCTs contributing to the analysis of moderate severity of OIC, including six drugs (lidocaine, ketamine, dezocine, butorphanol, dexmedetomidine, and placebo). Figure 3C; Supplementary Figure S3C detailed the efficacy of different comparisons of drugs by RRs and corresponding 95% CrIs. As same as previous results, all of the five drugs (lidocaine, ketamine, dezocine, butorphanol, and dexmedetomidine) could inhibit the moderate severity of OIC, compared with that of the placebo (all p-values < 0.05). Moreover, dezocine had the best effect compared with that of other five drugs (all p-values < 0.05). Heterogeneity between different comparisons of drugs is shown in Figure 4C. Similar to the results of incidence of OIC, individual and cumulative rank plots explained that the rank probability for mild severity of OIC from best to worst was dezocine, butorphanol, ketamine, dexmedetomidine, lidocaine, and placebo (Figure 5C, Figure 6C). Furthermore, their surface under the cumulative ranking curve (SUCRA) probabilities of six drugs for moderate severity of OIC are also presented in Figure 7C; Table 2. In addition, p-values of the node-splitting method were all more than 0.05, indicating the consistency of the direct and indirect evidence (Figure 8C). Sensitivity analysis was also conducted as shown in Supplementary Figure S4C, indicating the robustness of our results.
Severe Severity of OIC
A total of 18 RCTs contributed to the analysis of severe severity of OIC, including six drugs (lidocaine, ketamine, dezocine, butorphanol, dexmedetomidine, and placebo). Figure 3D; Supplementary Figure S3D detailed the efficacy of different comparisons of drugs by RRs and corresponding 95% CrIs. As same as previous results, four drugs, namely, ketamine, dezocine, butorphanol, and dexmedetomidine could prevent the severe severity of OIC, compared with that of the placebo (all p-values < 0.05). Moreover, dezocine had the best effect compared with that of other five drugs (all p-values < 0.05). Figure 4D showed the heterogeneity between different comparisons of drugs. Similar to the results of the incidence of OIC, individual and cumulative rank plots explained that the rank probability for severe severity of OIC from first to last was dezocine, butorphanol, ketamine, dexmedetomidine, lidocaine, and placebo (Figure 5D, Figure 6D). Furthermore, the surface under the cumulative ranking curve (SUCRA) probabilities of six drugs for severe severity of OIC are also exhibited in Figure 7D; Table 2. Furthermore, p-values of the node-splitting method were all above 0.05, indicating the consistency of the direct and indirect evidence (Figure 8D). Sensitivity analysis was also conducted as shown in Supplementary Figure S4D, indicating the robustness of our results.
Discussion
Although OIC was a transient, light, and self-limiting disease, it is a well-known adverse effect encountered during opioid administration, and pharmacologically induced cough could even be severe enough to result in death, especially for patients with comorbidities (Tweed and Dakin, 2001; Clivio et al., 2019). Therefore, there was an urgent need to take effective measures for these patients. Currently, pharmacological interventions have been widely used in the clinical setting. Due to the absence of direct comparisons of different pharmacological interventions and the application of novel therapeutic drugs, we, as clinical physicians, often face difficulties in choosing the optimal therapeutic drug for patients for preventing OIC during administration of general anesthesia. Hence, this network meta-analysis of RCTs was conducted to provide a hierarchy of five different therapeutic drugs to provide some references for further clinical research.
In this article, a total of six drugs, namely, lidocaine, ketamine, dezocine, butorphanol, dexmedetomidine, and placebo were finally enrolled. A total of four clinical outcomes comprising incidence of OIC, mild severity of OIC, moderate severity of OIC, and severe severity of OIC were ultimately evaluated. The overall heterogeneity between different comparisons of drugs was low to moderate, except for butorphanol vs placebo. The results of traditional pair-wise meta-analyses indicated that all of the five drugs (lidocaine, ketamine, dezocine, butorphanol, and dexmedetomidine) could prevent OIC for four clinical outcomes, compared with that of the placebo. Moreover, dezocine had the best effect, compared with that of other drugs. Network meta-analysis results suggested that the rank probability for incidence of OIC, moderate severity of OIC, and severe severity of OIC from best to worst was dezocine, butorphanol, ketamine, dexmedetomidine, lidocaine, and placebo, and the rank probability for mild severity of OIC from first to last was dezocine, butorphanol, ketamine, lidocaine, dexmedetomidine, and placebo, according to individual rank plots, cumulative rank plots, and SUCRA probabilities.
Currently, the mechanisms of OIC still remain unclear. Previous studies revealed that two main mechanisms might be among the reasons for OIC. On the one hand, the activation of the parasympathetic nervous system after opioid administration, could result in cough and bronchoconstriction (Yasuda et al., 1978), and on the other hand, the pulmonary chemoreflex could be another possible mechanism, mediated by rapidly adapting receptors (irritant receptors) or vagal C-fiber receptors (juxtacapillary receptors) close to pulmonary vessels (Böhrer et al., 1990). As reported by previous research studies, dezocine, as a mixed agonist–antagonist opioid, could activate κ receptors and antagonize the μ receptors to reduce OIC with no obvious adverse effects (Liu et al., 2015). Butorphanol, also as an agonist–antagonist opioid, could not only antagonize opioid-activated μ receptors but also activate the C-fiber receptor to inhibit the cough reflex afferent pathway (Zhang et al., 2018). Lidocaine was found to be effective in reducing OIC by suppressing brain stem function or anesthetizing the peripheral cough receptors (Poulton and James, 1979). Ketamine was reported to inhibit OIC by having an antagonistic effect on N-methyl-D-aspartate (NMDA) receptors (Said et al., 1995). Dexmedetomidine, as a highly selective α2-adrenergic agonist, could also reduce OIC via activating α2-adrenergic receptors to reverse muscular rigidity or relax tracheal smooth muscle contraction induced by histamine (Groeben et al., 2004).
In consistence with previously published studies, our results shed light on the effectiveness of five therapeutic drugs (lidocaine, ketamine, dezocine, butorphanol, and dexmedetomidine) in preventing OIC. Meta-analysis of RCTs conducted by Xiong et al. reported that dezocine could significantly reduce sufentanil-induced cough during general anesthesia induction, with no significant effect on vital signs (Xiong et al., 2020). Meta-analysis of RCTs conducted by Zhang et al. explained that butorphanol could also effectively prevent the incidence and severity of OIC (Zhang et al., 2018). Meta-analysis of RCTs conducted by Sun et al. suggested the effectiveness of prophylactic intravenous lidocaine in decreasing OIC during general anesthesia induction (Sun et al., 2014). Meta-analysis of RCTs conducted by Li et al. showed that prophylactic intravenous drugs such as ketamine, lidocaine, priming of fentanyl, dexmedetomidine, dezocine, and propofol was successful in inhibiting OIC (Shuying et al., 2016). Although all of these five drugs were effective in preventing OIC, they were compared with the placebo, and direct comparisons of different pharmacological interventions were absent. In this article, we not only compared the effectiveness of five drugs but also took advantage of network meta-analysis of RCTs to provide a hierarchy of these drugs.
As for adverse effects, a high dose of lidocaine could result in arrhythmia and cardiovascular depression during general anesthesia induction (Schlimp and Wiedermann, 2005). Ketamine could lead to hallucinations and elevation of blood pressure, intraocular pressure, and intracranial pressure (Shuying et al., 2016). Dexmedetomidine had adverse effects such as hypotension and bradycardia (Ebert et al., 2000). Currently, no significant effect on vital signs had been found in administration of dezocine. Dezocine and butorphanol could mainly result in respiratory depression, postoperative nausea, and vomiting (Sun et al., 2011; Zhang et al., 2018). Interestingly, we noticed that the combination of different drugs could effectively enhance the effect of reducing OIC. Honarmand et al. explained that a combination of ketamine and dexamethasone could significantly reduce the incidence of OIC than their single use (Honarmand et al., 2013). Saleh et al. found that ketamine in combination with dexmedetomidine could also effectively suppress OIC and delay the cough onset time (Saleh et al., 2014). Yu et al. revealed similar results in the combination of dexmedetomidine and midazolam for suppressing fentanyl-induced cough (Yu et al., 2012). Subsequent research studies should pay more attention to different drug combinations and their adverse effects.
In terms of the effects of different drug doses on OIC, Cheng et al. suggested that 0.03 mg/kg butorphanol was as effective as 0.015 mg/kg butorphanol in clinical practice to suppress fentanyl-induced cough (Cheng et al., 2016). Xu et al. revealed that dezocine attenuated fentanyl-induced cough in a dose-dependent manner, and the optimal dose was 0.1 mg/kg (Xu et al., 2015). Pandey et al. identified that the minimal dose of intravenous lidocaine for suppressing OIC was 0.5 mg/kg and any increased dose could not further reduce OIC (Pandey et al., 2005). Kim et al. found that a low dose of ketamine (0.1 mg/kg) was also effective in decreasing remifentanil-induced cough without influencing its severity and onset time (Kim et al., 2009). Zhou et al. also identified that the optimal dose of dexmedetomidine in the suppression of fentanyl-induced cough was 0.6 mg/kg, with no side effects (Zhou et al., 2019). In summary, the optimal dose of different drugs for preventing OIC needs to be fully explored.
As far as we are aware, this is the first network meta-analysis comparing the effectiveness of five therapeutic drugs (lidocaine, ketamine, dezocine, butorphanol, and dexmedetomidine) in preventing OIC, based on RCTs, which shall have a clear impact on the group baseline features and provide enough statistical power. Moreover, we not only conducted the direct comparisons of the five drugs but also performed indirect comparisons by means of network meta-analysis to provide a hierarchy of these drugs. Our analysis was anticipated to provide some references for guiding further clinical research. There were several limitations in this article too. First, the overall heterogeneity between different comparisons of drugs was low to moderate, except for butorphanol vs. placebo. Second, p-values of the node-splitting method between ketamine vs. lidocaine for incidence of OIC and mild severity of OIC were all below 0.05, indicating the inconsistency of the direct and indirect evidence. Third, due to the limitation of the meta-analysis, we could only use limited data obtained from previously published articles, and thus could not specify patients’ baseline characteristics and demographics. Hence, we currently faced difficulties in performing network meta-regression analyses to adjust for those effect modifiers and confounders. In summary, our results were merely analyzed in consideration of effectiveness, without consideration of different doses, adverse effects, time point of drug administration, and cost-benefit analysis. Subsequent high-quality RCTs were required to pay more attention to these aspects.
Conclusion
Taken together, our results indicated that all of the five drugs, namely, lidocaine, ketamine, dezocine, butorphanol, and dexmedetomidine could prevent OIC for four clinical outcomes, compared with the placebo. Among them, dezocine had the best effect compared with that of other drugs. Moreover, the rank probability for the incidence of OIC, moderate severity of OIC, and severe severity of OIC from best to worst was dezocine, butorphanol, ketamine, dexmedetomidine, lidocaine, and placebo, and the rank probability for mild severity of OIC from first to last was dezocine, butorphanol, ketamine, lidocaine, dexmedetomidine, and placebo, based on the network meta-analysis results. Our analysis was anticipated to provide some references for guiding further clinical research, and subsequent high-quality RCTs were required to verify our results.
Data Availability Statement
The raw data supporting the conclusions of this article will be made available by the authors, without undue reservation.
Author Contributions
XC: Protocol/project development, data analysis, and article revision; YD: article writing/editing, data collection, or management.
Conflict of Interest
The authors declare that the research was conducted in the absence of any commercial or financial relationships that could be construed as a potential conflict of interest.
Publisher’s Note
All claims expressed in this article are solely those of the authors and do not necessarily represent those of their affiliated organizations, or those of the publisher, the editors, and the reviewers. Any product that may be evaluated in this article, or claim that may be made by its manufacturer, is not guaranteed or endorsed by the publisher.
Acknowledgments
We would like to thank the researchers and participants for their contributions.
Supplementary Material
The Supplementary Material for this article can be found online at: https://www.frontiersin.org/articles/10.3389/fphar.2021.684276/full#supplementary-material
References
Ambesh, S. P., Singh, N., Gupta, D., Singh, P. K., and Singh, U. (2010). A Huffing Manoeuvre, Immediately before Induction of Anaesthesia, Prevents Fentanyl-Induced Coughing: a Prospective, Randomized, and Controlled Study. Br. J. Anaesth. 104 (1), 40–43. doi:10.1093/bja/aep333
Ando, K., Akimoto, K., Sato, H., Manabe, R., Kishino, Y., Homma, T., et al. (2020). Brigatinib and Alectinib for ALK Rearrangement-Positive Advanced Non-small Cell Lung Cancer with or without Central Nervous System Metastasis: A cSystematic Review and Network Meta-Analysis. Cancers (Basel) 12 (4). doi:10.3390/cancers12040942
Bang, S. R., Ahn, H. J., Kim, H. J., Kim, G. H., Kim, J. A., Yang, M., et al. (2010). Comparison of the Effectiveness of Lidocaine and Salbutamol on Coughing Provoked by Intravenous Remifentanil during Anesthesia Induction. Korean J. Anesthesiol 59 (5), 319–322. doi:10.4097/kjae.2010.59.5.319
Böhrer, H., Fleischer, F., and Werning, P. (1990). Tussive Effect of a Fentanyl Bolus Administered through a central Venous Catheter. Anaesthesia 45 (1), 18–21. doi:10.1111/j.1365-2044.1990.tb14496.x
Cha, A. S., Chen, Y., Fazioli, K., Rivara, M. B., and Devine, E. B. (2021). Microvascular Benefits of New Antidiabetic Agents: A Systematic Review and Network Meta-Analysis of Kidney Outcomes. J. Clin. Endocrinol. Metab. 106 (4), 1225–1234. doi:10.1210/clinem/dgaa894
Cheng, X. Y., Lun, X. Q., Li, H. B., and Zhang, Z. J. (2016). Butorphanol Suppresses Fentanyl-Induced Cough during General Anesthesia Induction: A Randomized, Double-Blinded, Placebo-Controlled Clinical Trial. Medicine (Baltimore) 95 (26), e3911. doi:10.1097/MD.0000000000003911
Clivio, S., Putzu, A., and Tramèr, M. R. (2019). Intravenous Lidocaine for the Prevention of Cough: Systematic Review and Meta-Analysis of Randomized Controlled Trials. Anesth. Analg 129 (5), 1249–1255. doi:10.1213/ANE.0000000000003699
Dias, S., Welton, N. J., Caldwell, D. M., and Ades, A. E. (2010). Checking Consistency in Mixed Treatment Comparison Meta-Analysis. Stat. Med. 29 (8), 932–944. doi:10.1002/sim.3767
Ebert, T. J., Hall, J. E., Barney, J. A., Uhrich, T. D., and Colinco, M. D. (2000). The Effects of Increasing Plasma Concentrations of Dexmedetomidine in Humans. Anesthesiology 93 (2), 382–394. doi:10.1097/00000542-200008000-00016
Gecaj-Gashi, A., Nikolova-Todorova, Z., Ismaili-Jaha, V., and Gashi, M. (2013). Intravenous Lidocaine Suppresses Fentanyl-Induced Cough in Children. Cough 9, 20. doi:10.1186/1745-9974-9-20
Groeben, H., Mitzner, W., and Brown, R. H. (2004). Effects of the Alpha2-Adrenoceptor Agonist Dexmedetomidine on Bronchoconstriction in Dogs. Anesthesiology 100 (2), 359–363. doi:10.1097/00000542-200402000-00026
Guler, G., Aksu, R., Bicer, C., Tosun, Z., and Boyaci, A. (2010). Comparison of the Effects of Ketamine or Lidocaine on Fentanyl-Induced Cough in Patients Undergoing Surgery: A Prospective, Double-Blind, Randomized, Placebo-Controlled Study. Curr. Ther. Res. Clin. Exp. 71 (5), 289–297. doi:10.1016/j.curtheres.2010.10.004
He, L., Xu, J. M., and Dai, R. P. (2012). Dexmedetomidine Reduces the Incidence of Fentanyl-Induced Cough: a Double-Blind, Randomized, and Placebo-Controlled Study. Ups J. Med. Sci. 117 (1), 18–21. doi:10.3109/03009734.2011.629749
Higgins, J. P. T., Thomas, J., Chandler, J., Cumpston, M., Li, T., Page, M. J., et al. (Editors) (2019). Cochrane Handbook for Systematic Reviews of Interventions. 2nd Edition. Chichester, United Kingdom: John Wiley and Sons, S38.
Honarmand, A., Safavi, M., and Khalighinejad, F. (2013). A Comparison of the Effect of Pretreatment with Intravenous Dexamethasone, Intravenous Ketamine, and Their Combination, for Suppression of Remifentanil-Induced Cough: A Randomized, Double-Blind, Placebo-Controlled Clinical Trial. Adv. Biomed. Res. 2, 60. doi:10.4103/2277-9175.115808
Kim, J. Y., Kim, J. Y., Park, S. Y., Jung, W. S., and Kwak, H. J. (2009). Effect of Low Dose Ketamine to Prevent Remifentanil-Induced Cough: a Randomized, Double-Blind, Placebo Controlled Trial. Korean J. Anesthesiol 56 (6), 624–627. doi:10.4097/kjae.2009.56.6.624
Kim, J. Y., Park, K. S., Kim, J. S., Park, S. Y., and Kim, J. W. (2008). The Effect of Lidocaine on Remifentanil-Induced Cough. Anaesthesia 63 (5), 495–498. doi:10.1111/j.1365-2044.2007.05414.x
Lin, C. S., Sun, W. Z., Chan, W. H., Lin, C. J., Yeh, H. M., and Mok, M. S. (2004). Intravenous Lidocaine and Ephedrine, but Not Propofol, Suppress Fentanyl-Induced Cough. Can. J. Anaesth. 51 (7), 654–659. doi:10.1007/BF03018421
Lin, J. A., Yeh, C. C., Lee, M. S., Wu, C. T., Lin, S. L., and Wong, C. S. (2005). Prolonged Injection Time and Light Smoking Decrease the Incidence of Fentanyl-Induced Cough. Anesth. Analg 101 (3), 670–contents. doi:10.1213/01.ANE.0000159161.31276.DB
Liu, R., Huang, X. P., Yeliseev, A., Xi, J., and Roth, B. L. (2014). Novel Molecular Targets of Dezocine and Their Clinical Implications. Anesthesiology 120 (3), 714–723. doi:10.1097/ALN.0000000000000076
Liu, X. S., Xu, G. H., Shen, Q. Y., Zhao, Q., Cheng, X. Q., Zhang, J., et al. (2015). Dezocine Prevents Sufentanil-Induced Cough during General Anesthesia Induction: A Randomized Controlled Trial. Pharmacol. Rep. 67 (1), 52–55. doi:10.1016/j.pharep.2014.08.004
Lumley, T. (2002). Network Meta-Analysis for Indirect Treatment Comparisons. Stat. Med. 21 (16), 2313–2324. doi:10.1002/sim.1201
Ma, L., Jin, G., Yao, K., Yang, Y., Chang, R., Wang, W., et al. (2021). Safety and Efficacy of Anti-PD-1/pd-L1 Inhibitors Compared with Docetaxel for NSCLC: A Systematic Review and Meta-Analysis. Front. Pharmacol. 12, 699892. doi:10.3389/fphar.2021.699892
Min, S. K., Kim, D. H., Cho, H. B., Moon, B. K., and Kim, J. Y. (2012). Limited Maximal Flow Rate of Target-Controlled Remifentanil Infusion and Induced Cough. Anaesthesia 67 (2), 145–148. doi:10.1111/j.1365-2044.2011.06961.x
Naldan, M. E., Arslan, Z., Ay, A., and Yayık, A. M. (2019). Comparison of Lidocaine and Atropine on Fentanyl-Induced Cough: A Randomized Controlled Study. J. Invest. Surg. 32 (5), 428–432. doi:10.1080/08941939.2018.1424272
Page, M. J., McKenzie, J. E., Bossuyt, P. M., Boutron, I., Hoffmann, T. C., Mulrow, C. D., et al. (2021). The PRISMA 2020 Statement: an Updated Guideline for Reporting Systematic Reviews. Bmj 372, n71. doi:10.1136/bmj.n71
Pandey, C. K., Raza, M., Ranjan, R., Lakra, A., Agarwal, A., Singh, U., et al. (2004). Intravenous Lidocaine Suppresses Fentanyl-Induced Coughing: a Double-Blind, Prospective, Randomized Placebo-Controlled Study. Anesth. Analg 99 (6), 1696. doi:10.1213/01.ANE.0000136967.82197.82
Pandey, C. K., Raza, M., Ranjan, R., Singhal, V., Kumar, M., Lakra, A., et al. (2005). Intravenous Lidocaine 0.5 mg.Kg-1 Effectively Suppresses Fentanyl-Induced Cough. Can. J. Anaesth. 52 (2), 172–175. doi:10.1007/BF03027724
Peringathara, B., and Robinson, S. (2016). Fentanyl-induced Cough Is a Risk Factor for Postoperative Nausea and Vomiting. Br. J. Anaesth. 117 (2), 269. doi:10.1093/bja/aew207
Poulton, T. J., and James, F. M. (1979). Cough Suppression by Lidocaine. Anesthesiology 50 (5), 470–472. doi:10.1097/00000542-197905000-00018
Said, S. I., Berisha, H. I., and Pakbaz, H. (1995). N-methyl-D-aspartate Receptors outside the central Nervous System: Activation Causes Acute Lung Injury that Is Mediated by Nitric Oxide Synthesis and Prevented by Vasoactive Intestinal Peptide. Neuroscience 65 (4), 943–946. doi:10.1016/0306-4522(95)00021-a
Saleh, A. J., Zhang, L., Hadi, S. M., and Ouyang, W. (2014). A Priming Dose of Intravenous Ketamine-Dexmedetomidine Suppresses Fentanyl-Induced Coughing: a Double-Blind, Randomized, Controlled Study. Ups J. Med. Sci. 119 (4), 333–337. doi:10.3109/03009734.2014.968270
Schlimp, C. J., and Wiedermann, F. J. (2005). Does Fentanyl-Induced Cough Justify Pre-treatment with Iv Lidocaine 2 mg.Kg-1. Can. J. Anaesth. 52 (2), 207. doi:10.1007/BF03027731
Shrestha, S. K., Bhattarai, B., and Shah, R. S. (2012). Preemptive Use of Small Dose Fentanyl Suppresses Fentanyl Induced Cough. Kathmandu Univ. Med. J. (Kumj) 10 (40), 16–19. doi:10.3126/kumj.v10i4.10988
Shuying, L., Ping, L., Juan, N., and Dong, L. (2016). Different Interventions in Preventing Opioid-Induced Cough: a Meta-Analysis. J. Clin. Anesth. 34, 440–447. doi:10.1016/j.jclinane.2016.05.034
Sun, L., Guo, R., and Sun, L. (2014). The Impact of Prophylactic Intravenous Lidocaine on Opioid-Induced Cough: a Meta-Analysis of Randomized Controlled Trials. J. Anesth. 28 (3), 325–333. doi:10.1007/s00540-013-1732-3
Sun, S., and Huang, S. Q. (2013). Effects of Pretreatment with a Small Dose of Dexmedetomidine on Sufentanil-Induced Cough during Anesthetic Induction. J. Anesth. 27 (1), 25–28. doi:10.1007/s00540-012-1470-y
Sun, Z. T., Yang, C. Y., Cui, Z., Zhang, J., and Han, X. P. (2011). Effect of Intravenous Dezocine on Fentanyl-Induced Cough during General Anesthesia Induction: a Double-Blinded, Prospective, Randomized, Controlled Trial. J. Anesth. 25 (6), 860–863. doi:10.1007/s00540-011-1237-x
Tweed, W. A., and Dakin, D. (2001). Explosive Coughing after Bolus Fentanyl Injection. Anesth. Analg 92 (6), 1442–1443. doi:10.1097/00000539-200106000-00018
Valkenhoef, G. V., and Kuiper, J. (2016). Gemtc: Network Meta-Analysis Using Bayesian Methods. John Wiley & Sons, 76–93.
Wang, Y., Zhu, J., Qin, Z., Wang, Y., Chen, C., Wang, Y., et al. (2018). Optimal Biopsy Strategy for Prostate Cancer Detection by Performing a Bayesian Network Meta-Analysis of Randomized Controlled Trials. J. Cancer 9 (13), 2237–2248. doi:10.7150/jca.24690
Wu, H. Y., Huang, J. W., Lin, H. J., Liao, W. C., Peng, Y. S., Hung, K. Y., et al. (2013). Comparative Effectiveness of Renin-Angiotensin System Blockers and Other Antihypertensive Drugs in Patients with Diabetes: Systematic Review and Bayesian Network Meta-Analysis. Bmj 347 (2), f6008. doi:10.1136/bmj.f6008
Xiong, Z., Yi, P., Song, J., and Tan, M. (2020). Dezocine Prevents Sufentanil-Induced Cough during General Anesthesia Induction: a Meta-Analysis of Randomised Controlled Trials. BMC Anesthesiol 20 (1), 154. doi:10.1186/s12871-020-01076-w
Xu, Y., Zhu, Y., Wang, S., Ren, Y., and Miao, C. (2015). Dezocine Attenuates Fentanyl-Induced Cough in a Dose-dependent Manner-A Randomized Controlled Trial. Int. J. Clin. Exp. Med. 8 (4), 6091–6096.
Yasuda, I., Hirano, T., Yusa, T., and Satoh, M. (1978). Tracheal Constriction by Morphine and by Fentanyl in Man. Anesthesiology 49 (2), 117–119. doi:10.1097/00000542-197808000-00012
Yeh, C. C., Wu, C. T., Huh, B. K., Lee, M. S., Lin, S. L., J Sheen, M., et al. (2007). Premedication with Intravenous Low-Dose Ketamine Suppresses Fentanyl-Induced Cough. J. Clin. Anesth. 19 (1), 53–56. doi:10.1016/j.jclinane.2006.05.021
Yin, F., and Zhang, T. (2019). A Small Dose of Butorphanol Prevents Sufentanil-Induced Cough during General Anesthesia Induction. J. Craniofac. Surg. 30 (8), 2499–2501. doi:10.1097/SCS.0000000000005967
Yu, H., Yang, X. Y., Zhang, X., Li, Q., Zhu, T., Wang, Y., et al. (2007). The Effect of Dilution and Prolonged Injection Time on Fentanyl-Induced Coughing. Anaesthesia 62 (9), 919–922. doi:10.1111/j.1365-2044.2007.05147.x
Yu, J., Lu, Y., Dong, C., Zhu, H., and Xu, R. (2012). Premedication with Intravenous Dexmedetomidine-Midazolam Suppresses Fentanyl-Induced Cough. Ir J. Med. Sci. 181 (4), 517–520. doi:10.1007/s11845-012-0807-8
Zhang, J., Miao, S., Tu, Q., Shi, M., Zou, L., Liu, S., et al. (2018). Effect of Butorphanol on Opioid-Induced Cough: a Meta-Analysis of Randomized Controlled Trials. Drug Des. Devel Ther. 12, 3263–3268. doi:10.2147/DDDT.S180533
Zhang, Q., Cheng, H., Wang, Y., Tian, Y., Xia, J., Wang, Y., et al. (2019). Different Therapeutic Regimens in the Treatment of Metastatic Prostate Cancer by Performing a Bayesian Network Meta-Analysis. Int. J. Surg. 66, 28–36. doi:10.1016/j.ijsu.2019.04.006
Zhou, L., Shen, Y., Huang, T., Sun, Y., Alolga, R. N., Zhang, G., et al. (2021). The Prognostic Effect of Dexamethasone on Patients with Glioblastoma: A Systematic Review and Meta-Analysis. Front. Pharmacol. 12, 727707. doi:10.3389/fphar.2021.727707
Keywords: drugs, pharmacological interventions, network meta-analysis, opioid-induced cough, randomized controlled trials
Citation: Dong Y and Chang X (2021) Comparison of Five Prophylactically Intravenous Drugs in Preventing Opioid-Induced Cough: A Bayesian Network Meta-Analysis of Randomized Controlled Trials. Front. Pharmacol. 12:684276. doi: 10.3389/fphar.2021.684276
Received: 23 March 2021; Accepted: 25 October 2021;
Published: 17 November 2021.
Edited by:
Kenneth KC Lee, Monash University Malaysia, MalaysiaReviewed by:
Daniel Erku, Griffith University, AustraliaDavid Bin-Chia Wu, Monash University, Australia
Copyright © 2021 Dong and Chang. This is an open-access article distributed under the terms of the Creative Commons Attribution License (CC BY). The use, distribution or reproduction in other forums is permitted, provided the original author(s) and the copyright owner(s) are credited and that the original publication in this journal is cited, in accordance with accepted academic practice. No use, distribution or reproduction is permitted which does not comply with these terms.
*Correspondence: Xiaohan Chang, Y3hoMTg5NDAyNTg1MjhAMTI2LmNvbQ==.