- 1Department of Paediatrics and Child Health, Desmond Tutu TB Centre, Faculty of Medicine and Health Sciences, Stellenbosch University, Cape Town, South Africa
- 2Department of Medicine, Division of Clinical Pharmacology, University of Cape Town, Cape Town, South Africa
- 3Department of Pediatrics, Divisions of General Pediatrics and Adolescent Medicine and Global Pediatrics, University of Wisconsin School of Medicine and Public Health, Madison, WI, United States
Given the high prevalence of multidrug-resistant (MDR)-TB in high HIV burden settings, it is important to identify potential drug-drug interactions between MDR-TB treatment and widely used nucleoside reverse transcriptase inhibitors (NRTIs) in HIV-positive children. Population pharmacokinetic models were developed for lamivudine (n = 54) and abacavir (n = 50) in 54 HIV-positive children established on NRTIs; 27 with MDR-TB (combinations of high-dose isoniazid, pyrazinamide, ethambutol, ethionamide, terizidone, fluoroquinolones, and amikacin), and 27 controls without TB. Two-compartment models with first-order elimination and transit compartment absorption described both lamivudine and abacavir pharmacokinetics, respectively. Allometric scaling with body weight adjusted for the effect of body size. Clearance was predicted to reach half its mature value
Principal Investigator Statement
The authors confirm that the PI’s for this paper are Prof A C Hesseling and Prof H S Schaaf. They had direct clinical responsibility for the patients.
What is Already Known About the Study?
• The burden of multidrug-resistant (MDR)-TB (Mycobacterium tuberculosis resistant to rifampicin and isoniazid) in children is increasing. In high HIV-burden settings, a substantial proportion (20–53.9%) of these children are HIV co-infected.
• The World Health Organization (WHO) recommends lamivudine and abacavir as preferred dual nucleoside reverse transcriptase inhibitors for initial antiretroviral treatment in HIV-positive children older than 3 months of age.
• There is no data on the potential effect of MDR-TB drugs on lamivudine and abacavir in children.
What this Study Adds:
• The study describes the pharmacokinetics of lamivudine and abacavir in HIV-positive children with and without MDR-TB treatment and could detect no significant drug-drug interactions of MDR-TB treatment on lamivudine and abacavir.
• Despite modest numbers, our results are reassuring for the antituberculosis drugs used commonly in children including terizidone, ethambutol, ethionamide, high-dose isoniazid, pyrazinamide, amikacin and fluoroquinolones as a group. For individual drugs, such as moxifloxacin, levofloxacin, linezolid and para-aminosalicylic acid (PAS), definitive conclusions were not possible given our small numbers and no patients were on bedaquiline and delamanid.
Introduction
The burden of multidrug-resistant (MDR)-TB (Mycobacterium tuberculosis resistant to rifampicin and isoniazid) is increasing, with modelled estimates of 25,000−32,000 incident cases annually in children (Jenkins and Yuen, 2018). In high HIV-burden settings, a substantial proportion (20–53.9%) of these children are HIV co-infected (Fairlie et al., 2011; Seddon et al., 2011; Hesseling et al., 2012). HIV co-infection is associated with higher morbidity and mortality in children with MDR-TB (Seddon et al., 2011; Seddon et al., 2014). Optimizing both HIV and TB treatment in children, is critically important.
Data regarding the impact of MDR-TB drugs on the pharmacokinetics of antiretroviral drugs (ARVs) are limited, especially in children. A recent report found no significant effect of older MDR-TB drugs on lopinavir-ritonavir exposures in HIV-positive children (Van der Laan et al., 2018). The effect of MDR-TB drugs on nucleoside reverse transcriptase inhibitors (NRTIs) in children has not yet been studied.
Data on the potential effect of MDR-TB drugs on lamivudine and abacavir, the World Health Organization (WHO)-recommended preferred dual NRTI backbone for initial antiretroviral treatment (ART) in HIV-positive children older than 3 months of age, is particularly relevant (World Health Organization, 2018).
Lamivudine is widely distributed into total body fluid which may be partly related to its low protein binding (generally <36%) (Perry and Faulds, 1997; Johnson et al., 1999). Protein binding for abacavir is around 50% and it is suggested that the drug is distributed to extravascular spaces (Yuen et al., 2008). Lamivudine undergoes minimal metabolism and is primarily renally eliminated (approximately 70%) (Heald et al., 1996). Abacavir is extensively metabolised by the liver with less than 2% excreted unchanged in urine (Chittick et al., 1999; McDowell et al., 1999); it is primarily metabolised via two pathways, urine diphosphate glucuronyltransferase and alcohol dehydrogenase (Yuen et al., 2008). Lamivudine induces p-glycoprotein (Weiss et al., 2008), whereas abacavir is a substrate and possible inhibitor (Shaik et al., 2007; Storch et al., 2007; Namanja et al., 2012). These are all sites for possible DDIs with drugs used to treat MDR-TB.
There are a number of potential interactions with MDR-TB drugs included in this study. Clofazimine is metabolised by, and a weak inhibitor of the CYP P450 enzyme system (Cholo et al., 2012; Sangana et al., 2018). Moxifloxacin undergoes partial hepatic metabolism (Lehmann, 1969; Clofazimine, 2008; Sy et al., 2015) and primarily renal mechanisms account for the elimination of PAS, linezolid, levofloxacin, amikacin, and terizidone (Slatter et al., 2001; Garcia-Prats Donald et al., 2013). As substrates of p-glycoprotein, linezolid and moxifloxacin (Escribano et al., 2007; Brillault et al., 2009; Bolhuis et al., 2013) might interact with lamivudine and abacavir through efflux pump transport mechanisms.
Previous population pharmacokinetic models for lamivudine and abacavir in children have included one (Tremoulet et al., 2007; Piana et al., 2013) (Jullien et al., 2005) and two (Bouazza et al., 2011; Zhang et al., 2012) (Zhao et al., 2013; Rabie et al., 2020) compartment models, with first-order elimination, and either first-order absorption or transit compartments (Zhang et al., 2012; Rabie et al., 2020). These former models were used to inform our final population pharmacokinetic models with the aim to characterize the effect of routine antituberculosis drugs used for MDR-TB treatment on the pharmacokinetics of lopinavir and abacavir in HIV-positive children.
Methods
The study was conducted in Cape Town, South Africa, as part of the MDR-PK1 study (R01 HD069169-01). The study was approved by Stellenbosch University (N11/03/059) and the University of Cape Town (397/2011) Health Research Ethics Committees. Written informed consent was obtained from parents/legal guardians and assent was obtained from participants where appropriate.
HIV-positive children (ages 0 to <15 years) routinely treated for MDR-TB were consecutively enrolled (MDR-TB group). A control group of HIV-positive children without TB was matched roughly to the MDR-TB group for age and according to use of lopinavir/ritonavir or efavirenz-based combination ART. Children were established on at least 2 weeks of lamivudine, abacavir and MDR-TB treatment prior to enrolment.
Lamivudine was given as a 4 mg/kg twice-daily or 8 mg/kg once-daily dose. Abacavir was given as an 8 mg/kg twice-daily or 16 mg/kg once-daily dose. Both drugs were given as either a suspension or tablet (whole or crushed with water). Brands included Aspen, Aurobindo, Adcock Ingram, Sonke, Mylan and Cipla. Some children (<2 years), that refused to swallow, were dosed using a nasogastric tube. All antituberculosis drugs were administered after a minimum of 4 h fast; ARVs including lamivudine and abacavir were dosed 1 h later and afterwards a breakfast was offered.
Since the study was nested in a larger study on MDR-TB drugs, the sampling schedule was optimised around the time of the dose of antituberculosis drugs. Blood samples were drawn at six time points: two samples before dosing ARVs [1 h (time −1 h, just before administration of the antituberculosis drugs) and immediately before (time 0) the ARV dose] and at 1, 3, 7 and either 5 or 10 h after observed dosing. For participants who remained on once-daily dosing, samples were obtained the following day, at 10, 11, 13, 15, and 17 h after the evening ARV dose.
The assays for lamivudine and abacavir were developed at the Division of Clinical Pharmacology, University of Cape Town (Archary et al., 2019). Validation was done according to the US Food and Drug Administration (Author Anonymous, 2011) and European Medicines Agency (European Medicines Agency, 2012) guidelines.
Population pharmacokinetic analyses were completed using NONMEM version 7.4.3 (Icon Development Solutions, Ellicott City, MD, United States of America). All statistical analyses, including summary statistics and visual displays, were generated in R (http://www.R-project.org/) while Perl-speaks-NONMEM (PsN), Pirana and the R package Xpose4 (http://xpose.sourceforge.net) were used in the model building process for data exploration, visualization and creation of diagnostics (Keizer et al., 2013).
Several structural models were tested for lamivudine and abacavir: one- and two-compartment disposition with first-order elimination, and first-order absorption, with and without absorption lag time or transit compartments (Savic et al., 2007).
The pharmacokinetic samples collected pre-dose were treated as a separate occasion in the model, to allow estimation of both inter-individual (IIV) and inter-occasion variability (IOV). A lognormal distribution was assumed for these random effects and correlation between them was investigated both at IIV and IOV level. The relative bioavailability was fixed to one for a typical patient to investigate the presence of IIV and/or IOV on this parameter. The residual unexplained variability (RUV) was evaluated using a combined additive and proportional model. Samples with concentrations below the limit of quantitation (BLQ) were handled by the M6 method from Beal (Beal, 2001). This means that they were replaced with half the lower limit of quantitation (LLOQ), except for consecutive values in a series, for which the trailing BLQ values were ignored for the fit but included in the diagnostic plots. The additive error was inflated by half the LLOQ value for the imputed BLQ values (i.e., by LLOQ/2) to allow for extra uncertainty due to the imputation (and proportionally to the size of the LLOQ for that specific assay) (Francis et al., 2021). Finally, the additive error for all samples obtained from a specific assay was bound to be at least 20% of the LLOQ of that assay.
Allometric scaling was applied to oral clearance, intercompartmental clearance, volume of distribution and peripheral volume of distribution to account for differences in body size (Anderson and Holford, 2008). Besides total body weight, fat-free mass (Al-Sallami et al., 2015) was also tested as alternative descriptor of body size.
After the inclusion of allometric scaling, covariate selection was performed by first narrowing down the search to factors that were either known or physiologically plausible to affect a certain pharmacokinetic parameter. Then, the plots of individual random effects (Empirical Bayes Estimates) (Lavielle and Ribba, 2016) versus covariates were used to identify possibly significant trends in the data. Finally the candidate covariate effect were tested and included in the model using a step-wise procedure with forward inclusion (p < 0.05 based on drop in −2 log-likelihood (-2LL)) and backward elimination (p < 0.01) (Wählby et al., 2015). Additionally, the improvement in goodness of fit including visual predictive checks, reduction in unexplained variability, and stability of the model parameter estimates were considered to retain the effects in the model.
Age was tested using a sigmoidal maturation model (Anderson and Holford, 2008; Holford et al., 2013), as shown in the equation below
where MAT is the fraction of the adult value of clearance, PMAGE is postmenstrual age (post-natal plus gestational age), with
MDR-TB treatment, as a single combined variable or selected single MDR-TB drugs, was used as a categorical covariate to test whether parameter estimates (oral clearance, bioavailability, and the absorption parameters including mean transit time) were different between lamivudine and abacavir in the MDR-TB group and the control group.
Other covariates tested for significance in the model were sex, method of drug administration on the sampling day (nasogastric tube vs oral, crushing tablets), drug formulation (suspension/whole tablets) and other ART treatment (lopinavir/ritonavir and efavirenz).
Model development was guided by changes in the −2LL (with drops of more than 6.64 points considered significant at p < 0.01 for the inclusion of one additional parameter in the model), precision in parameter estimates, graphical analysis of goodness of fit plots including visual predictive checks, and scientific plausibility (Jonsson and Karlsson, 1998; Karlsson and Holford, 2008; Kiang et al., 2012). Parameter uncertainty of the estimated from the final model was assessed using Sampling Importance Resampling (Dosne et al., 2016).
Statistical Power Calculations
This analysis was nested in a larger study of children with MDR-TB, and with no clear expectations on the size of expected drug-drug interactions and the number of children that would have been on each specific MDR-TB drug, so no formal calculation of statistical power was prospectively performed. We however assessed a posteriori the statistical power of our data to detect significant differences in ART exposure between HIV-positive children with MDR-TB and controls, based on simulation and re-estimation (Lee, 2001), using the stochastic simulation and estimation (SSE) tool of Perl-speaks-NONMEM (Lindbom et al., 2004). Briefly, the final pharmacokinetic model was used to re-simulate (n = 200) the current trial (thus assuming the same patient covariates, doses, and sampling times), but with postulation of a known difference in clearance or bioavailability between the MDR-TB and control groups. Then, alternative models with or without this MDR-TB covariate effect were fitted to each simulated data set and compared to evaluate whether the effect was statistically significant in terms of improvement of the −2LL value. The percentage of simulated data in which the effect could be detected as significant, provided the statistical power.
Results
A summary of participant characteristics is presented in Table 1. Fifty-four HIV-positive children (27 MDR-TB and 27 control cases) were included. All children were on both NRTIs, lamivudine and abacavir, except for two controls on zidovudine and two MDR-TB cases on stavudine as substitute for abacavir.
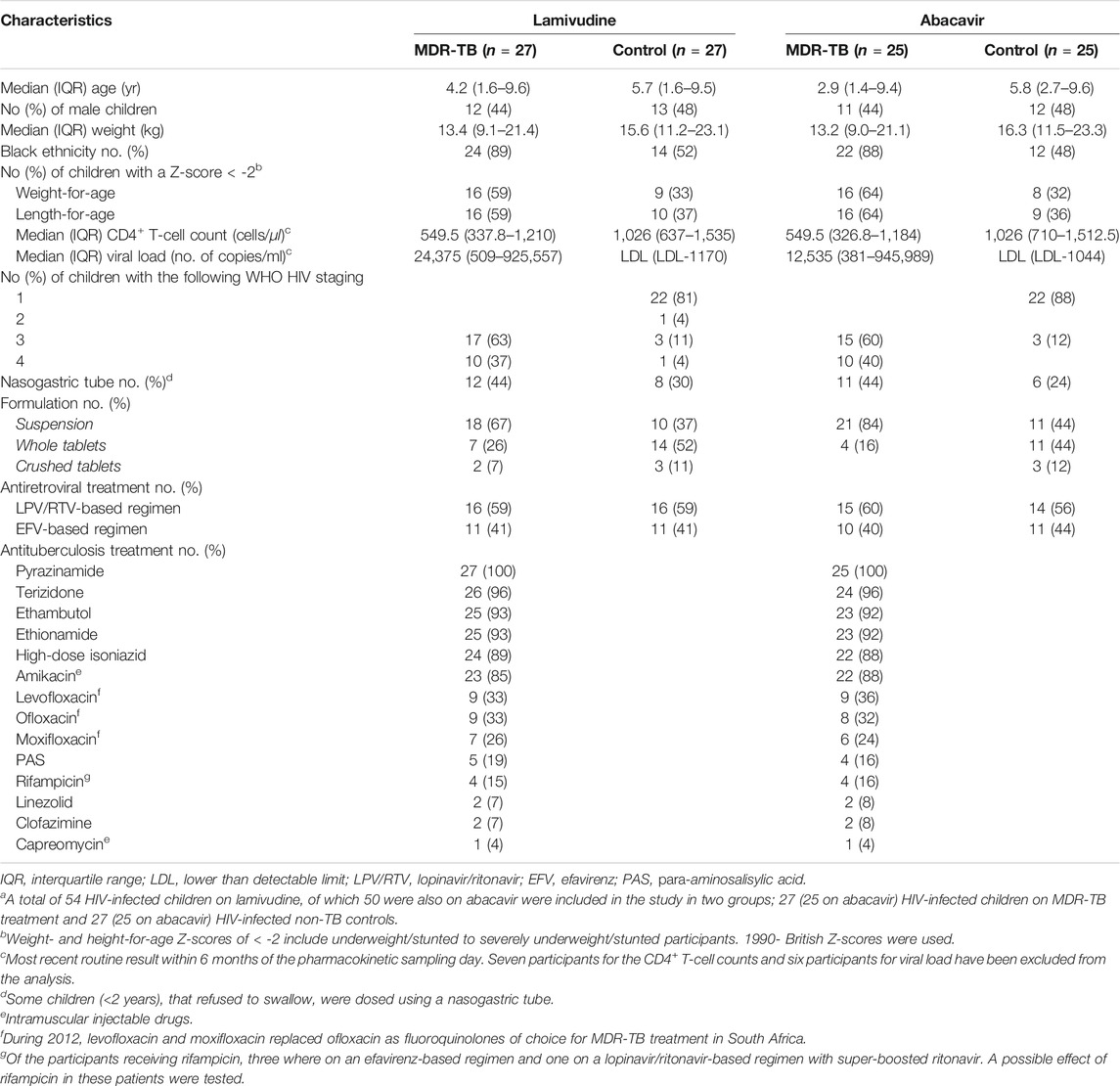
TABLE 1. Summary of characteristics of HIV-positive children with and without MDR-TB on a lamivudine and abacavir–containing antiretroviral regimena.
A total of 322 samples for lamivudine and 299 samples for abacavir were collected (2 lamivudine and one abacavir pharmacokinetic profile had only one pre-dose sample). Seven and 36 samples were below the LLOQ, for lamivudine and abacavir, respectively, and most of these (Zhang et al., 2012; World Health Organization, 2018) were the two predose samples. In 4 participants (3 of whom were out-patients) both drugs had LLOQ at all predose concentrations available (7 samples), while the pharmacokinetic profile observed after the supervised dose did not indicate low concentrations compatible with the undetectable trough concentration observed. For these patients, a missed evening dose was assumed. Another three samples were excluded due to values incompatible to the dose times of both drugs.
Antituberculosis drugs are listed in Table 1. Four children received rifampicin on the sampling day because of inconclusive or pending rifampicin susceptibility results; three were on an efavirenz- and one on a lopinavir/ritonavir-based regimen with super-boosted ritonavir. In addition, one participant, received rifampicin up to 3 days before the sampling day, and was on lopinavir/ritonavir-based regimen with super-boosted ritonavir. A possible delayed effect of rifampicin for this patient along with the other rifampicin patients were retained.
Creatinine levels were routinely obtained in 25/27 (93%) of the children in the MDR-TB group, and these all had values in the normal range. Creatinine levels for the HIV group were not done as it is not part of routine standard of care.
A two-compartment model with first-order elimination and transit compartment absorption was found to suitably describe the pharmacokinetics of both lamivudine and abacavir. The final parameter estimates are presented in Table 2. A Visual Predictive Check plot stratified by MDR-TB treatment status is displayed in Figure 1. Goodness of fit plots for both models are presented in Supplementary Figure S1 (Jonsson and Karlsson, 1998).
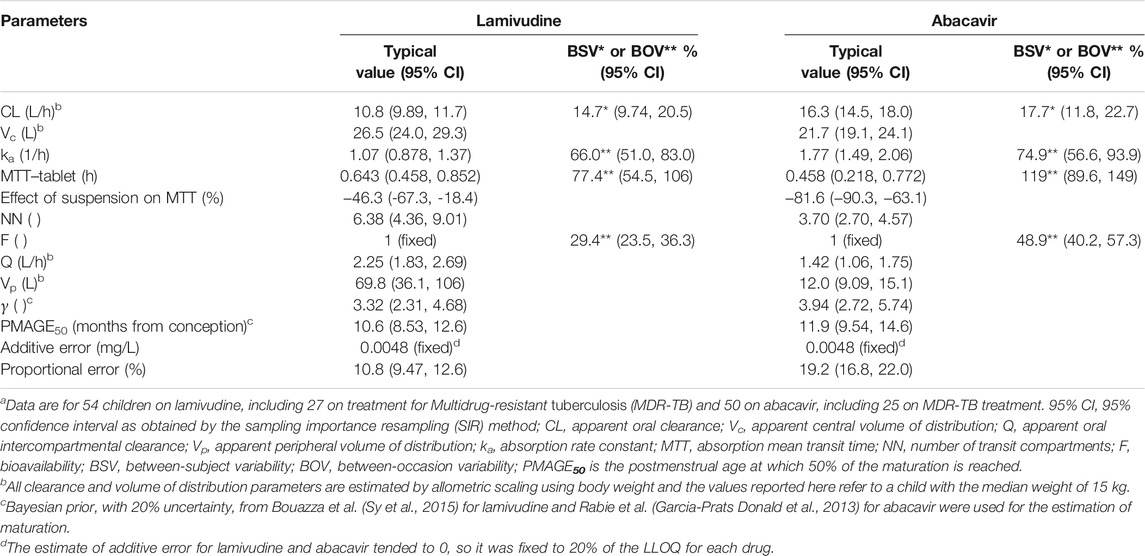
TABLE 2. Parameter estimates of the final model for lamivudine and abacavir pharmacokinetics in HIV-positive childrena.
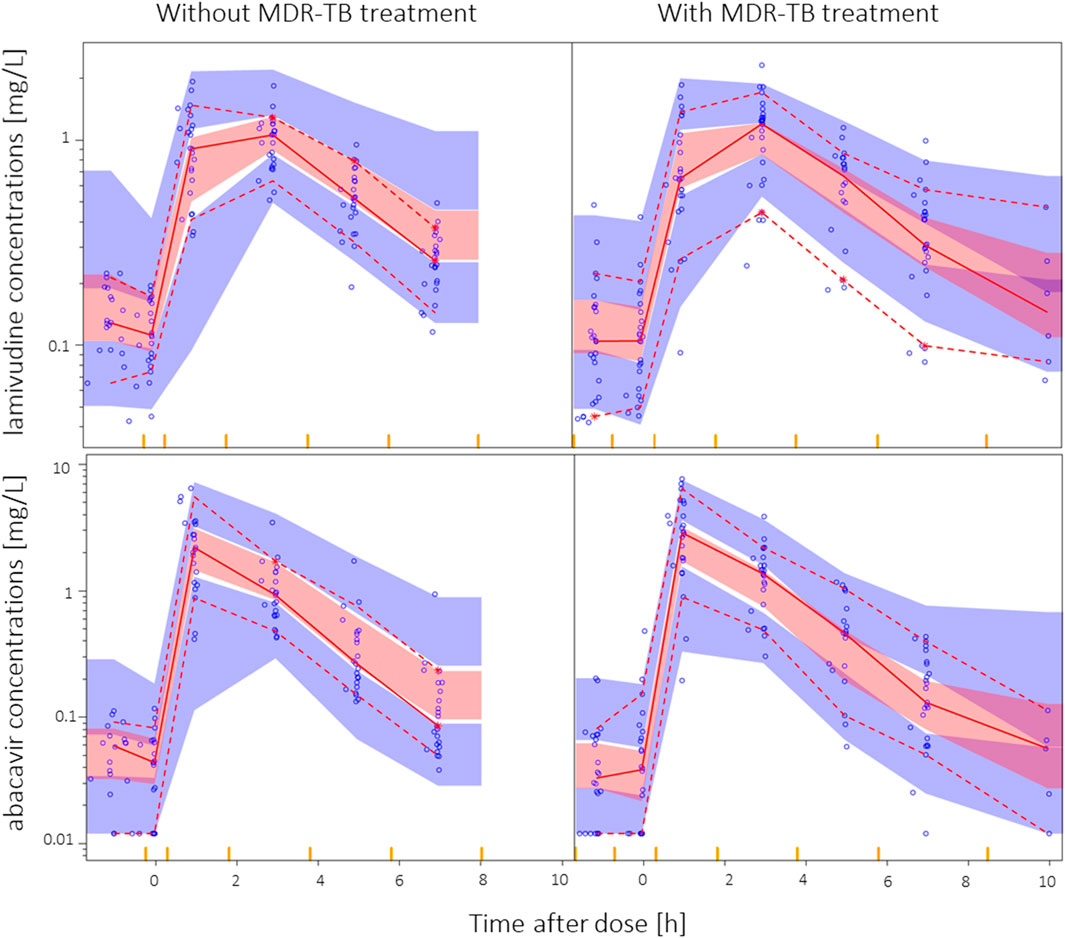
FIGURE 1. Visual predictive check for lamivudine and abacavir in HIV-positive children stratified by MDR-TB vs controls, using 1,000 simulations. The solid and dashed lines represent the fifth, 50th, and 95th percentiles of the observed data, while the shaded areas (pink and blue) are the model-predicted 95% confidence intervals for the same percentiles. Observed data are displayed as blue circles. Two children on once-daily dosing, sampled at later time points, were removed for the visual predictive check for display purposes.
The estimate of additive error for both lamivudine and abacavir was small and not robust and was therefore conservatively fixed to 20% of the LLOQ for each drug.
Allometric scaling was used to account for size differences and improved the model fit for both drugs (77 and 45 points decrease in −2LL for lamivudine and abacavir, respectively). Using fat-free mass instead of body weight did not provide any further benefit in terms of model fit. Maturation could be identified; clearance was predicted to reach half its mature value
The mean absorption transit time for the suspension formulation of both drugs were significantly faster than tablets (21 vs 39 min for lamivudine and 5 vs 27 min for abacavir), but no difference was found for bioavailability.
No significant differences were detected due to lopinavir/ritonavir-, ritonavir superboosted-vs efavirenz-containing ART, sex, or the use of a nasogastric tube. Exclusion of the participants on rifampicin also did not affect our findings.
MDR-TB treatment was not found to significantly affect the pharmacokinetics of lamivudine or abacavir. Terizidone, ethambutol, ethionamide, high-dose isoniazid, pyrazinamide and amikacin were tested individually with similar results. None of the models including effects on clearance, bioavailability, or absorption rate constant achieved a significant improvement in −2LL. The visual predictive checks stratified by MDR-TB group vs controls are shown in Figure 1, showing that a model assuming no effect of MDR-TB treatment was suitable for both datasets.
Two participants in the control group remained on once-daily lamivudine and abacavir dosing. The pre-sampling dose was therefore not observed. As precaution, we excluded these participants in the model development process and when evaluating TB treatment as covariate, but this made no difference, so the patients were retained in the final model.
The a posteriori power calculations predicted that, at a significance level of p < 0.01, our study design was expected to be able to detect with 80% power a 20% decrease in lamivudine exposure (i.e. 20% increase in clearance) in the MDR-TB group. For abacavir, at the same power and significance level, a 25% decrease in exposure (i.e. 25% increase in clearance) would be detected. Therefore, if a difference greater than these effects were present in the data, our model would have an 80% chance of detecting it at p < 0.01.
Discussion
We describe the pharmacokinetics of lamivudine and abacavir in HIV-positive children with and without MDR-TB treatment and could detect no significant DDIs of MDR-TB treatment on lamivudine and abacavir. The newer antituberculosis drugs, such as bedaquiline and delamanid were not yet available for the treatment of MDR-TB in children during the time of the study, and were therefore not included. Despite modest numbers, our results are reassuring for the antituberculosis drugs used commonly in children including terizidone, ethambutol, ethionamide, high-dose isoniazid, pyrazinamide, amikacin and fluoroquinolones as a group. For other single drugs, such as moxifloxacin, levofloxacin, linezolid and PAS, definitive conclusions were not possible given our small numbers.
The estimated value for lamivudine oral clearance of 10.8 L/h was similar to previously published reports in HIV-positive children (infants to 18 years) (Tremoulet et al., 2007; Bouazza et al., 2011; Zhang et al., 2012; Piana et al., 2013). The median allometrically scaled lamivudine clearance ranged from 7.2–16.5 L/h in four studies (n = 752) with similar populations. For abacavir, the estimated value for oral clearance of 16.3 L/h was similar to previously published reports in HIV-positive children (infants to 16 years) (Jullien et al., 2006; Zhao et al., 2013; Rabie et al., 2020). The median allometrically scaled abacavir clearance ranged from 14.7–17.8 L/h in three studies (n = 261) with similar populations. Overall, findings from these studies on both drugs were compatible with our data.
Abacavir’s bioavailability has been shown to decrease during co-treatment with rifampicin and lopinavir/ritonavir (Rabie et al., 2020). Our study did not find this likely due to the small number of children receiving rifampicin, and due to the rest of the children being on an efavirenz-containing regimen.
Our study has several limitations, the main one being the observational nature, where children were on individualized MDR-TB treatment regimens with varying combinations of antituberculosis drugs, which increased the possibility of unknown confounders.
Despite our modest sample size, we believe our findings to remain valuable, since large clinically relevant effects would have been detected. A cross-over design may have increased the power of the analysis, but this was not feasible in our study.
Another limitation is that actual routine creatinine clearance samples were not available for all participants, and therefore we were unable to test renal function as covariate. This would have been an important covariate in the model for lamivudine since it is primarily renally eliminated. However, all children in the MDR-TB group, who are generally sicker than the controls, had normal renal function.
In conclusion, we found no significant effect on key pharmacokinetic parameters (clearance, bioavailability and absorption) of lamivudine and abacavir when co-administered with antituberculosis drugs commonly used for MDR-TB treatment in HIV-positive children. While a modest-sized study, our findings are reassuring. Optimal and safe dosing of both ARVs and MDR-TB treatment in HIV-co-infected children is essential. Additional research is needed to evaluate DDIs between ARVs and increasingly used TB drugs including moxifloxacin, levofloxacin, clofazimine, linezolid, bedaquiline and delamanid.
Data Availability Statement
The data that support the findings of this study are available from the corresponding author upon reasonable request.
Ethics Statement
The studies involving human participants were reviewed and approved by Stellenbosch University HREC. Written informed consent to participate in this study was provided by the participants’ legal guardian/next of kin.
Author Contributions
LL, AG-P, and AH, contributed to conceptualization, data collection, data interpretation, writing of the original draft, review and editing of the manuscript. LL and PD contributed to conceptualization of the study, formal analysis, data interpretation, writing of the original draft, review and editing of the manuscript. JW, HS contributed to data collection, data interpretation, writing—review and editing of the manuscript. HD contributed to data curation, formal analysis, writing of the original draft, review and editing of the manuscript. LW and JN contributed to the methodology, investigation, data interpretation, writing—review and editing of the manuscript. HM contributed to the conceptualization, data interpretation, writing—review, editing of the manuscript. All authors approved the final manuscript. LL and PD verified the underlying data. AH and PD contributed equally.
Funding
NIH R01 grant (Eunice Kennedy Shriver National Institute of Child Health and Human Development (NICHD-069169-01: PI: AH)). South African National Research Foundation SArCHI Chair (AH). National Research Foundation, grant for rated researchers 90,729 (HM), 109056 (PD). UCT pharmacokinetic laboratory: drug assays were supported in part by the NIAID (UM1 AI068634, UM1 AI068636 and UM1AI106701, U01 AI068632), the NICHD, and the National Institute of Mental Health (AI068632). L. van der Laan was supported by the Swedish Foundation for International Cooperation in Research and Higher Education (STINT) jointly with the South African National Research Council, National Research Foundation (NRF) (NRF grant number: 101575).
Conflict of Interest
The authors declare that the research was conducted in the absence of any commercial or financial relationships that could be construed as a potential conflict of interest.
Publisher’s Note
All claims expressed in this article are solely those of the authors and do not necessarily represent those of their affiliated organizations, or those of the publisher, the editors and the reviewers. Any product that may be evaluated in this article, or claim that may be made by its manufacturer, is not guaranteed or endorsed by the publisher.
Acknowledgments
The authors would like to thank the children and parents for participating in this study. We also thank the clinical team including Marianne Willemse, Adelaide Carelse, Primrose Puling, Zibelezenkosi Hlebani, Nickey Jass, Klassina Zimri, and Zingiwe Mramba for their assistance in study implementation. A special recognition to the University of Cape Town ICTS High Performance Computing team (http://hpc.uct.ac.za) for the use of their facilities. The laboratory subscribes to the National Institute of Allergies and Infectious Diseases Division (NIAID, DAIDS) of the AIDS Clinical Pharmacology Quality Assurance Antiretroviral Proficiency Testing Program.
Supplementary Material
The Supplementary Material for this article can be found online at: https://www.frontiersin.org/articles/10.3389/fphar.2021.722204/full#supplementary-material
References
Al-Sallami, H. S., Goulding, A., Grant, A., Taylor, R., Holford, N., and Duffull, S. B. (2015). Prediction of Fat-free Mass in Children. Clin. Pharmacokinet. 54, 1169–1178. doi:10.1007/s40262-015-0277-z
Anderson, B. J., and Holford, N. H. (2008). Mechanism-based Concepts of Size and Maturity in Pharmacokinetics. Annu. Rev. Pharmacol. Toxicol. 48, 303–332. doi:10.1146/annurev.pharmtox.48.113006.094708
Archary, M., Mcllleron, H., Bobat, R., LaRussa, P., Sibaya, T., Wiesner, L., et al. (2019). Population Pharmacokinetics of Abacavir and Lamivudine in Severely Malnourished Human Immunodeficiency Virus-Infected Children in Relation to Treatment Outcomes. Br. J. Clin. Pharmacol. 85, 2066–2075. doi:10.1111/bcp.13998
Author Anonymous, (2011). FDA Guidance for Industry: Process Validation: General Principles and Practices.
Beal, S. L. (2001). Ways to Fit a PK Model with Some Data below the Quantification Limit. J. Pharmacokinet. Pharmacodyn. 28, 481–504. doi:10.1023/a:1012299115260
Bolhuis, M. S., van Altena, R., van Soolingen, D., de Lange, W. C., Uges, D. R., van der Werf, T. S., et al. (2013). Clarithromycin Increases Linezolid Exposure in Multidrug-Resistant Tuberculosis Patients. Eur. Respir. J. 42, 1614–1621. doi:10.1183/09031936.00001913
Bouazza, N., Hirt, D., Blanche, S., Frange, P., Rey, E., Tréluyer, J. M., et al. (2011). Developmental Pharmacokinetics of Lamivudine in 580 Pediatric Patients Ranging from Neonates to Adolescents. Antimicrob. Agents Chemother. 55, 3498–3504. doi:10.1128/AAC.01622-10
Brillault, J., De Castro, W. V., Harnois, T., Kitzis, A., Olivier, J. C., and Couet, W. (2009). P-glycoprotein-mediated Transport of Moxifloxacin in a Calu-3 Lung Epithelial Cell Model. Antimicrob. Agents Chemother. 53, 1457–1462. doi:10.1128/AAC.01253-08
Chittick, G. E., Gillotin, C., McDowell, J. A., Lou, Y., Edwards, K. D., Prince, W. T., et al. (1999). Abacavir: Absolute Bioavailability, Bioequivalence of Three Oral Formulations, and Effect of Food. Pharmacotherapy 19, 932–942. doi:10.1592/phco.19.11.932.31568
Cholo, M. C., Steel, H. C., Fourie, P. B., Germishuizen, W. A., and Anderson, R. (2012). Clofazimine: Current Status and Future Prospects. J. Antimicrob. Chemother. 67, 290–298. doi:10.1093/jac/dkr444
Dosne, A. G., Bergstrand, M., Harling, K., and Karlsson, M. O. (2016). Improving the Estimation of Parameter Uncertainty Distributions in Nonlinear Mixed Effects Models Using Sampling Importance Resampling. J. Pharmacokinet. Pharmacodyn. 43, 583–596. doi:10.1007/s10928-016-9487-8
Escribano, I., Rodríguez, J. C., Llorca, B., García-Pachon, E., Ruiz, M., and Royo, G. (2007). Importance of the Efflux Pump Systems in the Resistance of Mycobacterium tuberculosis to Fluoroquinolones and Linezolid. Chemotherapy 53, 397–401. doi:10.1159/000109769
European Medicines Agency (2012). Guideline on Bioanalytical Method Validation. London: European Medicines Agency
Fairlie, L., Beylis, N. C., Reubenson, G., Moore, D. P., and Madhi, S. A. (2011). High Prevalence of Childhood Multi-Drug Resistant Tuberculosis in Johannesburg, South Africa: a Cross Sectional Study. BMC Infect. Dis. 11, 28. doi:10.1186/1471-2334-11-28
Francis, J., Mngqibisa, R., McIlleron, H., Kendall, M. A., Wu, X., Dooley, K. E., et al. (2021). A Semimechanistic Pharmacokinetic Model for Depot Medroxyprogesterone Acetate and Drug-Drug Interactions with Antiretroviral and Antituberculosis Treatment. Clin. Pharmacol. Ther. 110, 1057–1065. doi:10.1002/cpt.2324
Garcia-Prats Donald, P. R., Hesseling, A. C., and Schaaf, H. S. A. J. (2013). Second-line Antituberculosis Drugs in Children: A Commissioned Review for the World Health Organization 19th Expert Committee on the Selection and Use of Essential Medicines. Switzerland, Geneva: World Health Organization.
Gisleskog, P. O., Karlsson, M. O., and Beal, S. L. (2002). Use of Prior Information to Stabilize a Population Data Analysis. J. Pharmacokinet. Pharmacodyn. 29, 473–505. doi:10.1023/a:1022972420004
Heald, A. E., Hsyu, P. H., Yuen, G. J., Robinson, P., Mydlow, P., and Bartlett, J. A. (1996). Pharmacokinetics of Lamivudine in Human Immunodeficiency Virus-Infected Patients with Renal Dysfunction. Antimicrob. Agents Chemother. 40, 1514–1519. doi:10.1128/AAC.40.6.1514
Hesseling, A. C., Kim, S., Madhi, S., Nachman, S., Schaaf, H. S., Violari, A., et al. (2012). High Prevalence of Drug Resistance Amongst HIV-Exposed and -infected Children in a Tuberculosis Prevention Trial. Int. J. Tuberc. Lung Dis. 16, 192–195. doi:10.5588/ijtld.10.0795
Holford, N., Heo, Y. A., and Anderson, B. (2013). A Pharmacokinetic Standard for Babies and Adults. J. Pharm. Sci. 102, 2941–2952. doi:10.1002/jps.23574
Jenkins, H. E., and Yuen, C. M. (2018). The burden of Multidrug-Resistant Tuberculosis in Children. Int. J. Tuberc. Lung Dis. 22, 3–6. doi:10.5588/ijtld.17.0357
Johnson, M. A., Moore, K. H., Yuen, G. J., Bye, A., and Pakes, G. E. (1999). Clinical Pharmacokinetics of Lamivudine. Clin. Pharmacokinet. 36, 41–66. doi:10.2165/00003088-199936010-00004
Jonsson, E. N., and Karlsson, M. O. (1998). Xpose--an S-PLUS Based Population Pharmacokinetic/pharmacodynamic Model Building Aid for NONMEM. Comput. Methods Programs Biomed. 58, 51–64. doi:10.1016/s0169-2607(98)00067-4
Jullien, V., Urien, S., Chappuy, H., Dimet, J., Rey, E., Pons, G., et al. (2005). Abacavir Pharmacokinetics in Human Immunodeficiency Virus-Infected Children Ranging in Age from 1 Month to 16 years: A Population Analysis. J. Clin. Pharmacol. 45, 257–264. doi:10.1177/0091270004272215
Jullien, V., Urien, S., Hirt, D., Delaugerre, C., Rey, E., Teglas, J. P., et al. (2006). Population Analysis of Weight-, Age-, and Sex-Related Differences in the Pharmacokinetics of Lopinavir in Children from Birth to 18 Years. Antimicrob. Agents Chemother. 50, 3548–3555. doi:10.1128/AAC.00943-05
Karlsson, M. O., and Holford, N. (2008). A Tutorial on Visual Predictive Checks. Annu. Meet. Popul. Approach Gr Eur. 17.
Keizer, R. J., Karlsson, M. O., and Hooker, A. (2013). Modeling and Simulation Workbench for NONMEM: Tutorial on Pirana, PsN, and Xpose. CPT Pharmacometrics Syst. Pharmacol. 2, e50. doi:10.1038/psp.2013.24
Kiang, T. K., Sherwin, C. M., Spigarelli, M. G., and Ensom, M. H. (2012). Fundamentals of Population Pharmacokinetic Modelling : Modelling and Software. Clin. Pharmacokinet. 51 (8), 515–525. doi:10.1007/BF03261928
Lavielle, M., and Ribba, B. (2016). Enhanced Method for Diagnosing Pharmacometric Models: Random Sampling from Conditional Distributions. Pharm. Res. 33, 2979–2988. doi:10.1007/s11095-016-2020-3
Lee, P. I. (2001). Design and Power of a Population Pharmacokinetic Study. Pharm. Res. 18, 75–82. doi:10.1023/a:1011030827847
Lehmann, J. (1969). The Role of the Metabolism of P-Aminosalicylic Acid (PAS) in the Treatment of Tuberculosis. Interaction with the Metabolism of Isonicotinic Acid Hydrazide (INH) and the Synthesis of Cholesterol. Scand. J. Respir. Dis. 50, 169–185.
Lindbom, L., Ribbing, J., and Jonsson, E. N. (2004). Perl-speaks-NONMEM (PsN)-Aa Perl Module for NONMEM Related Programming. Comput. Methods Programs Biomed. 75, 85–94. doi:10.1016/j.cmpb.2003.11.003
McDowell, J. A., Chittick, G. E., Ravitch, J. R., Polk, R. E., Kerkering, T. M., Stein, D. S., et al. (1999). Pharmacokinetics of [(14)C]abacavir, a Human Immunodeficiency Virus Type 1 (HIV-1) Reverse Transcriptase Inhibitor, Administered in a Single Oral Dose to HIV-1-Infected Adults: a Mass Balance Study. Antimicrob. Agents Chemother. 43, 2855–2861. doi:10.1128/AAC.43.12.2855
Namanja, H. A., Emmert, D., Davis, D. A., Campos, C., Miller, D. S., Hrycyna, C. A., et al. (2012). Toward Eradicating HIV Reservoirs in the Brain: Inhibiting P-Glycoprotein at the Blood-Brain Barrier with Prodrug Abacavir Dimers. J. Am. Chem. Soc. 134, 2976–2980. doi:10.1021/ja206867t
Perry, C. M., and Faulds, D. (1997). Lamivudine. Drugs 53, 657–680. doi:10.2165/00003495-199753040-00008
Piana, C., Zhao, W., Adkison, K., Burger, D., Jacqz-Aigrain, E., Danhof, M., et al. (2013). Covariate Effects and Population Pharmacokinetics of Lamivudine in HIV-Infected Children. Br. J. Clin. Pharmacol. 77, 861–872. doi:10.1111/bcp.12247
Rabie, H., Tikiso, T., Lee, J., Fairlie, L., Strehlau, R., Bobat, R., et al. (2020). Abacavir Exposure in Children Cotreated for Tuberculosis with Rifampin and Superboosted Lopinavir-Ritonavir. Antimicrob. Agents Chemother. 64, e01923. doi:10.1128/AAC.01923-19
Sangana, R., Gu, H., Chun, D. Y., and Einolf, H. J. (2018). Evaluation of Clinical Drug Interaction Potential of Clofazimine Using Static and Dynamic Modeling Approaches. Drug Metab. Dispos. 46, 26–32. doi:10.1124/dmd.117.077834
Savic, R. M., Jonker, D. M., Kerbusch, T., and Karlsson, M. O. (2007). Implementation of a Transit Compartment Model for Describing Drug Absorption in Pharmacokinetic Studies. J. Pharmacokinet. Pharmacodyn. 34, 711–726. doi:10.1007/s10928-007-9066-0
Seddon, J. A., Hesseling, A. C., Godfrey-Faussett, P., and Schaaf, H. S. (2014). High Treatment success in Children Treated for Multidrug-Resistant Tuberculosis: an Observational Cohort Study. Thorax 69, 458–464. doi:10.1136/thoraxjnl-2013-203900
Seddon, J. A., Hesseling, A. C., Willemse, M., Donald, P. R., and Schaaf, H. S. (2011). Culture-confirmed Multidrug-Resistant Tuberculosis in Children: Clinical Features, Treatment, and Outcome. Clin. Infect. Dis. 54, 157–166. doi:10.1093/cid/cir772
Shaik, N., Giri, N., Pan, G., and Elmquist, W. F. (2007). P-Glycoprotein-Mediated Active Efflux of the Anti-HIV1 Nucleoside Abacavir Limits Cellular Accumulation and Brain Distribution. Drug Metab. Dispos 35, 2076–2085. doi:10.1124/dmd.107.017723
Slatter, J., Stalker, D., Feenstra, K., and Welshman, I. (2001). Pharmacokinetics, Metabolism, and Excretion of Linezolid Following an Oral Dose of Linezolid to Healthy Human Subjects. Drug Metab 29, 1136–1145.
Storch, C. H., Theile, D., Lindenmaier, H., Haefeli, W. E., and Weiss, J. (2007). Comparison of the Inhibitory Activity of Anti-HIV Drugs on P-Glycoprotein. Biochem. Pharmacol. 73, 1573–1581. doi:10.1016/j.bcp.2007.01.027
Sy, S. K., de Kock, L., Diacon, A. H., Werely, C. J., Xia, H., Rosenkranz, B., et al. (2015). N-acetyltransferase Genotypes and the Pharmacokinetics and Tolerability of Para-Aminosalicylic Acid in Patients with Drug-Resistant Pulmonary Tuberculosis. Antimicrob. Agents Chemother. 59, 4129–4138. doi:10.1128/AAC.04049-14
Tremoulet, A. H., Capparelli, E. V., Patel, P., Acosta, E. P., Luzuriaga, K., Bryson, Y., et al. (2007). Population Pharmacokinetics of Lamivudine in Human Immunodeficiency Virus-Exposed and -Infected Infants. Antimicrob. Agents Chemother. 51, 4297–4302. doi:10.1128/AAC.00332-07
Van der Laan, L. E., Garcia-Prats, A. J., Schaaf, H. S., Tikiso, T., Wiesner, L., De Kock, M., et al. (2018). Pharmacokinetics and Drug-Drug Interactions of Lopinavir-Ritonavir Administered with First- and Second-Line Antituberculosis Drugs in HIV-Infected Children Treated for Multidrug-Resistant Tuberculosis. Antimicrob. Agents Chemother. 62, e00420. doi:10.1128/AAC.00420-17
Wählby, U., Jonsson, E. N., and Karlsson, M. O. (2015). Comparison of Stepwise Covariate Model Building Strategies in Population Pharmacokinetic-Pharmacodynamic Analysis. Aaps J. 4 (4), 68–79. doi:10.1208/ps040427
Weiss, J., Weis, N., Ketabi-Kiyanvash, N., Storch, C. H., and Haefeli, W. E. (2008). Comparison of the Induction of P-Glycoprotein Activity by Nucleotide, Nucleoside, and Non-nucleoside Reverse Transcriptase Inhibitors. Eur. J. Pharmacol. 579, 104–109. doi:10.1016/j.ejphar.2007.11.007
World Health Organization, (2018). Updated Recommendations on First-Line and Second-Line Antiretroviral Regimens and post-exposure Prophylaxis and Recommendations on Early Infant Diagnosis of HIV: Interim guidelines.Supplement to the 2016 Consolidated Guidelines on the Use of Antiretroviral Drugs for Treating and Preventing HIV Infection. Geneva: World Health Organization. WHO/CDS/HIV/18.51. Licence: CC BY-NC-SA 3.0 IGO. Geneva.
Yuen, G. J., Weller, S., and Pakes, G. E. (2008). A Review of the Pharmacokinetics of Abacavir. Clin. Pharmacokinet. 47, 351–371. doi:10.2165/00003088-200847060-00001
Zhang, C., Denti, P., van der Walt, J. S., Ren, Y., Smith, P., Karlsson, M. O., et al. (2012). Population Pharmacokinetic Model for Adherence Evaluation Using Lamivudine Concentration Monitoring. Ther. Drug Monit. 34, 481–484. doi:10.1097/FTD.0b013e31825c6067
Keywords: tuberculosis, paediatrics (drugs and medicines), HIV, drug drug interactions, pharmacometric modeling
Citation: van der Laan LE, Garcia-Prats AJ, Schaaf HS, Winckler JL, Draper H, Norman J, Wiesner L, McIlleron H, Denti P and Hesseling AC (2021) Pharmacokinetics and Drug-Drug Interactions of Abacavir and Lamuvudine Co-administered With Antituberculosis Drugs in HIV-Positive Children Treated for Multidrug-Resistant Tuberculosis. Front. Pharmacol. 12:722204. doi: 10.3389/fphar.2021.722204
Received: 08 June 2021; Accepted: 22 September 2021;
Published: 08 October 2021.
Edited by:
Linda Akoth Chaba, Strathmore University, KenyaReviewed by:
Muhammad Usman, University of Veterinary and Animal Sciences, PakistanEne Ette, Anoixis Corporation, United States
Copyright © 2021 van der Laan, Garcia-Prats, Schaaf, Winckler, Draper, Norman, Wiesner, McIlleron, Denti and Hesseling. This is an open-access article distributed under the terms of the Creative Commons Attribution License (CC BY). The use, distribution or reproduction in other forums is permitted, provided the original author(s) and the copyright owner(s) are credited and that the original publication in this journal is cited, in accordance with accepted academic practice. No use, distribution or reproduction is permitted which does not comply with these terms.
*Correspondence: Louvina E. van der Laan, dmRsYWFuQHN1bi5hYy56YQ==
†These authors share senior authorship