- Fujian Institute of Hematology, Fujian Medical Center of Hematology, Fujian Provincial Key Laboratory of Hematology, Fujian Medical University Union Hospital, Fuzhou, China
Background: It has been verified that deficiency of Qi, a fundamental substance supporting daily activities according to the Traditional Chinese Medicine theory, is an important symptom of cancer. Qi-invigorating herbs can inhibit cancer development through promoting apoptosis and improving cancer microenvironment. In this study, we explored the potential mechanisms of Qi-invigorating herbs in diffuse large B cell lymphoma (DLBCL) through network pharmacology and in vitro experiment.
Methods: Active ingredients of Qi-invigorating herbs were predicted from the Traditional Chinese Medicine Systems Pharmacology Database. Potential targets were obtained via the SwissTargetPrediction and STITCH databases. Target genes of DLBCL were obtained through the PubMed, the gene-disease associations and the Malacards databases. Overlapping genes between DLBCL and each Qi-invigorating herb were collected. Hub genes were subsequently screened via Cytoscape. The Gene Ontology and pathway enrichment analyses were performed using the DAVID database. Molecular docking was performed among active ingredients and hub genes. Hub genes linked with survival and tumor microenvironment were analyzed through the GEPIA 2.0 and TIMER 2.0 databases, respectively. Additionally, in vitro experiment was performed to verify the roles of common hub genes.
Results: Through data mining, 14, 4, 22, 22, 35, 2, 36 genes were filtered as targets of Ginseng Radix et Rhizoma, Panacis Quinquefolii Radix, Codonopsis Radix, Pseudostellariae Radix, Astragali Radix, Dioscoreae Rhizoma, Glycyrrhizae Radix et Rhizoma for DLBCL treatment, respectively. Then besides Panacis Quinquefolii Radix and Dioscoreae Rhizoma, 1,14, 10, 14,13 hub genes were selected, respectively. Molecular docking studies indicated that active ingredients could stably bind to the pockets of hub proteins. CASP3, CDK1, AKT1 and MAPK3 were predicted as common hub genes. However, through experimental verification, only CASP3 was considered as the common target of Qi-invigorating herbs on DLBCL apoptosis. Furthermore, the TIMER2.0 database showed that Qi-invigorating herbs might act on DLBCL microenvironment through their target genes. Tumor-associated neutrophils may be main target cells of DLBCL treated by Qi-invigorating herbs.
Conclusion: Our results support the effects of Qi-invigorating herbs on DLBCL. Hub genes and immune infiltrating cells provided the molecular basis for each Qi-invigorating herb acting on DLBCL.
Introduction
Diffuse large B cell lymphoma (DLBCL) is an aggressive lymphoma and the most common subtype of lymphoma, accounting for more than 30% of the lymphoma cases (Siegel et al., 2021). Although 60–70% of DLBCL patients reach long-term remission in response to a combination of rituximab, cyclophosphamide, doxorubicin, and prednisone (R-CHOP) immunochemotherapy, tumor recurrent, coupled with therapeutic resistant figures remain high.
In recent years, several agents constitute the new landscape of treatments for DLBCL, including brentuximab vedotin (Svoboda et al., 2021), ibrutinib (Younes et al., 2019) and polatuzumab vedotin (Sehn et al., 2020). Besides, targeting tumor microenvironment (TME) is one promising option for increasing evidence strongly highlights that lymphomagenesis and DLBCL progression mainly rely on its microenvironment (Scott and Gascoyne, 2014).
Immune cells are main components of TME surrounding DLBCL cells, including T lymphocytes, macrophages and natural killer (NK) cells. It has been identified that a heterogeneous DLBCL microenvironment differs significantly in the subtype of tumor-infiltrating immune cells. For instance, a high proportion of programmed cell death protein 1 (PD1) + CD8+ T cells and programmed cell death-ligand 1 (PD-L1) + T cells in the TME was found to predict poor survival in DLBCL, whereas high expression of immune checkpoint cytotoxic T-lymphocyte-associated protein 4 (CTLA4) on T cells might be associated with favorable outcome (Xu-Monette et al., 2019). A recent study indicated that high proportions of T-cell immunoglobulin, mucin-domain containing 3 (TIM3) + T cells and TIM3+CD4+ cells have an independent adverse impact on survival (Autio et al., 2021). However, the above targeted therapy is not always feasible in DLBCL (Ansell et al., 2019; Barrueto et al., 2020; Kline et al., 2020). Much is still unknown concerning DLBCL microenvironment. Therefore, there is an urgent need to develop alternative or complementary therapeutics for DLBCL.
Traditional Chinese medicine (TCM) is gradually being accepted as a therapeutic option of cancer for they have provided much promise in vivo experiment and clinical trials (Parekh et al., 2009; Xiang et al., 2019). Based on TCM theory, the human body is recognized as an organic entity with Yin-Yang balance, which is a philosophical concept that represent a unity of opposing forces in the Universe. Generally speaking, Yin refers to stable or negative factors, while Yang represents positive and active features. if the Yin-Yang balance in a single individual is broken, the human body becomes sick. This theory is somewhat similar to TME. Qi is one of the most important products derived from the interaction between Yin and Yang, which stimulates the flow of blood throughout the body, and promotes the absorption and utility of the nutrients of food. The deprivation of Qi can be caused by many factors including malnutrition, fatigue, surgery and chronic diseases, leading to symptoms such as cough, short breath, muscle weakness, and immune-deficiency. Previous studies reported qi deficiency was an important syndrome of cancer (Hou et al., 2021; Qi et al., 2021). Qi-invigorating herbs, including Ginseng Radix et Rhizoma, Astragali Radix, and Glycyrrhizae Radix et Rhizoma, have been verified for their positive roles in cancer immune regulation (Jiang et al., 2019; Wang et al., 2020a). Several Qi-invigorating decoctions can inhibit cancer development through altering cancer immune microenvironment in vitro and vivo (Du et al., 2016; Wu et al., 2019; Xu et al., 2020). Furthermore, some Qi-invigorating herbs could directly promote the apoptosis of tumor cells in vivo and vitro (Xiong et al., 2018; Wang et al., 2020b). In lymphoma, TCMs have been verified to enhance physical function, reduce adverse effects of chemotherapy, and improve long-term survival (Hijikata et al., 1995; Ben-Arye et al., 2010; Guo et al., 2015; Kim et al., 2018). However, the underlying mechanisms and potential targets of Qi-invigorating herbs in DLBCL remain unclear. It is this idea that is the starting point of this article hoping to provide a theoretical basis for the application of Qi-invigorating herbs in DLBCL.
In this study, we conducted a network pharmacology strategy for Qi-invigorating herbs. The so-called network pharmacology is a method which combines systems biology with multidirectional pharmacology based on high-throughput omics data analysis and network database retrieval (Hopkins, 2008; Li and Zhang, 2013). Through this discipline and experimental validation, the interaction between Qi-invigorating herbs and DLBCL would be discussed for the first time, which is helpful to elucidate the impact of Qi-invigorating herbs on DLBCL and provide guidance for herb selection, prescription formation, or even new drug design. The detailed flowchart of this study was shown in Figure 1.
Materials and Methods
Screening Active Constituents of Qi-Invigorating Herbs
Eight common Qi-invigorating herbs were discussed in this study, including Ginseng Radix et Rhizoma (RenShen), Panacis Quinquefolii Radix (XiYangShen), Codonopsis Radix (DangShen), Pseudostellariae Radix (TaiZiShen), Astragali Radix (HuangQi), Glycyrrhizae Radix et Rhizoma (GanCao), Atractylodis Macrocephalae Rhizoma (BaiZhu) and Dioscoreae Rhizoma (ShanYao). All chemical constituents of the above herbs were obtained from the Traditional Chinese Medicine Systems Pharmacology (TCMSP) database (https://tcmsp-e.com/) (Ru et al., 2014). The potential active ingredients of the above Qi-invigorating herbs were selected according to the oral bioavailability (OB)≥30% (Xu et al., 2012), the Drug-Likeness (DL) Index≥0.18 and the drug half-life (HL)≥4 h (Jin et al., 2021). Chemical structures of the active ingredients were then collected from the PubChem database (https://pubchem.ncbi.nlm.nih.gov/) (Kim et al., 2016).
Screening Drug Targets
The target genes of the active ingredients were obtained from the SwissTargetPrediction (http://www.swisstargetprediction.ch/) and STITCH (https://stitch.embl.de/) databases (Kuhn et al., 2008; Daina et al., 2019). By uploading chemical structures (SMILE files) to these databases and selecting “Homo sapiens” for the species, results were outputted. In the STITCH database, a target with combined-score ≥ 0.7 was selected, while in the SwissTargetPrediction database, probability ≥ 0.7 was assigned as the criteria for screening target genes of the active ingredients. The gene names of the targets were obtained from the UniProt database (https://www.uniprot.org/) (Consortium, 2019). All the target genes of each active ingredient were obtained after combining the two databases and excluding duplicates. The target genes of each Qi-invigorating herb were collected after combining target genes of its active ingredients and excluding the repetitive targets.
Obtaining Potential Target Genes for Diffuse Large B Cell Lymphoma
We used “diffuse large B cell lymphoma,” “diffuse large-B cell lymphoma” and “DLBCL” as keywords to identify DLBCL-related genes from the PubMed database (update time: September 15, 2021, https://www.ncbi.nlm.nih.gov/gene), the gene-disease associations database (DisGeNET, https://www.disgenet.org/) and the Malacards (https://www.malacards.org/) database (Piñero et al., 2017; Rappaport et al., 2017; Piñero et al., 2020). Only those genes from “Homo sapiens” were included. In the end, a total of 1174 target genes of DLBCL were obtained after removing duplicates.
Constructing Protein-Protein Interaction Networks and Screening Key Targets
Venn diagrams via R software 4.1.0 were conducted to show overlapping targets between DLBCL and each Qi-invigorating herb. These targets were considered as potential targets for Qi-invigorating herbs acting on DLBCL.
To illustrate the enrichment of candidate targets in terms of the gene function, the study applied the Database for Annotation Visualization and Integrated Discovery (DAVID, https://david.ncifcrf.gov/) 6.8 to perform Gene Ontology (GO) and Kyoto Encyclopedia of Genes and Genome (KEGG) analyses (Huang et al., 2007). GO contains molecular function (MF), cellular component (CC), and biological process (BP) analyses. p-value < 0.05 were set as the criteria for screening (Huang et al., 2009).
PPI networks of candidate targets were constructed using the STRING database (https://string-db.org/) (Szklarczyk et al., 2021). We also used the Cytoscape 3.7.2 software to construct PPI networks based on the STRING results (Shannon et al., 2003). The criteria for considering significant was interactions with a combined score ≥ 0.7. Genes with degrees ≥ 5 were considered as hub genes. The hub genes were also input into the DAVID 6.8 for GO and KEGG enrichment analyses. These hub genes were subsequently prepared for molecular docking.
Molecular Docking
The crystal structures of the above hub genes were downloaded from the Protein Data Bank (https://www.pdb.org/) (Berman et al., 2000). PyMol (version 2.3.2) was used to process proteins, including removing water and ligands. The 2D structures of the active ingredients were obtained from the PubChem database (https://pubchem.ncbi.nlm.nih.gov/) and considered as ligands. AutoDockTools 1.5.6 was run for molecular docking (Trott and Olson, 2010). During the docking process, hydrogen atoms were added to all proteins, and partial atomic charges were calculated. Target proteins were held as rigid and ligands allowed traveling freely. After the flexible-ligand docking, the grid box size was set to 40 × 40 × 40 points with a spacing of 0.375 Å. The Lamarckian Genetic Algorithm was used to search for populations of 150 individuals with a mutation rate of 0.02 over 10 generations. The results were sorted on the basis of the binding energy. The smaller the binding energy value, the more stable the structure after binding.
Analyzing Genes Acting on Diffuse Large B Cell Lymphoma Survival
The GEPIA2 database (http://gepia2.cancer-pku.cn/) was used for validation of hub gene expression in DLBCL and survival analysis (Tang et al., 2019).
Screening Common Hub Genes and Active Ingredients
A Venn diagram was constructed to select common hub genes and ingredients. Hub genes and targeted active ingredients existed in at least three groups were considered as common hub genes and ingredients, respectively.
Performing Experimental Verification
Preparation of Qi-Invigorating Herbs
All the Qi-invigorating herbs, including Ginseng Radix et Rhizoma, Codonopsis Radix, Pseudostellariae Radix, Astragali Radix, Glycyrrhizae Radix et Rhizoma, were purchased from the TCM pharmacy of Fujian Medical University Union Hospital, Fujian, China. Each herb (10 g) was placed in a beaker, then boiled water (100 ml) was added and the solution was kept boiling for 3 hours (Chen et al., 2019; Miao et al., 2019). The decoctions were concentrated to 0.2 g/ml. The supernatant was collected as research objects after centrifugation, filtration, and sterilization (Chen et al., 2019; Miao et al., 2019).
Cell Cultivation
Human DLBCL cell lines SU-DHL-2 (activated B-cell-like lymphoma) and SU-DHL-6 (germinal center B-cell-like lymphoma) were obtained from the American Type Culture Collection (ATCC, Manassas, VA, United States) and cultured in Roswell Park Memorial Institute-1640 (RPMI-1640) medium (HyClone, United States) supplemented with 10% fetal bovine serum (FBS) (CellBox, China) and 1% penicillin-streptomycin. All the cell lines were authenticated using short tandem repeat analysis. Cells were maintained at 37°C in standard culture conditions.
Cell Viability Assay
1 × 104 SU-DHL-2 or SU-DHL-6 cells were incubated into the 96‐well plates in 100 μL serum medium per well. A series of two-fold dilutions of decoctions were carried out to give concentrations of 10, 5, 2.5, 1.25, 0.625 and 0.313 mg/ml. Untreated tumor cells and complete medium (blank control) were left as control groups. After 48 h incubation, 10 µL of CCK8 (AbMole Bioscience) was added into all wells and the plates were further incubated for 2 h. Then, the plates were read at 450 and 630 nm as reference wavelength. After background subtraction, half maximal inhibitory concentration (IC50) was calculated using the SPSS 20.0 (IBM, United States). The experiments were repeated in triplicate for each inhibitor concentration.
Cell Proliferation Analysis
Proliferative ability of DLBCL cells was analyzed by CCK-8. Briefly, 1 × 104 cells were incubated into the 96‐well plates in 100 μL serum medium. Subsequently, the cells were left negative control and experimental groups for 0, 24, 48, 72 h. The plates were read at 450 and 630 nm as reference wavelength. Each experiment was repeated in triplicate.
Flow Cytometry
The Annexin V-APC/propidium iodide (PI) apoptosis detection kit (KeyGEN, China) was used to detect apoptosis by flow cytometry. DLBCL cells were cultured in vitro and divided into nine groups, including untreated (blank control), negative control, Astragali Radix group, Glycyrrhizae Radix et Rhizoma group, Ginseng Radix et Rhizoma group, Pseudostellariae Radix group and Codonopsis Radix group. After cultivation for 48 h, cells were washed with PBS, resuspended in 100 µL binding buffer at a concentration of 2 × 106 cells/ml, and anti-Annexin V APC-conjugated antibody and PI were added. The mixtures were incubated for 15 min at room temperature, supplemented with binding buffer to 500 µL and processed by BD Accuri C6 Plus Flow Cytometry (BD Biosciences, United States). Data were analyzed through flow cytometry (BD PharmingenTM, United States).
Quantitative Real-Time Polymerase Chain Reaction
Total RNA of cells was extracted by TRIzol (CWBIO, China) and sequentially purified via the chloroform, isopropanol, and 70% ethanol. Reverse transcription for mRNA relied on a kit named Hifair III 1st Strand cDNA Synthesis Supermix for qPCR (YEASEN, China). qRT-PCR was carried out on Applied Biosystems 7500/7500 Fast Real-time PCR System (Thermo Fisher Scientific, United States) and QuantStudio™ 5 Real-Time PCR Instrument (384-well block, Thermo Fisher Scientific, United States). The GADPH was used to normalize mRNA samples. Relative quantification of target primers was calculated by the 2-ΔΔCT method. The experiment was repeated three times. Primers used for qRT-PCR analysis are listed as follow: GADPH Forward (5′-3′) TTGGTATCGTGGAAGGACTCA, Reverse (5′-3′) AGTAGAGGCAGGGATGATGTT; CASP3 Forward (5′-3′) CATGGAAGCGAATCAATGGACT, Reverse (5′-3′) CTGTACCAGACCGAGATGTCA; CDK1 Forward (5′-3′) GTCAGTCTTCAGGATGTGCT, Reverse (5′-3′) ATGTACTGACCAGGAGGGAT; AKT1 Forward (5′-3′) GTCATCGAACGCACCTTCCAT, Reverse (5′-3′) AGCTTCAGGTACTCAAACTCGT; MAPK3 Forward (5′-3′) GCTTCCGCCATGAGAATGTC; Reverse (5′-3′) GGCGGAGTGGATGTACTTGA.
Analyzing Genes Acting on Diffuse Large B Cell Lymphoma Tumor Microenvironment
The TIMER database (http://timer.cistrome.org/) is a database for comprehensive analysis of immune infiltrating cells across multiple cancer types. We applied TIMER2.0 to study the relationship between hub genes and tumor-infiltrating immune cells [B cells, CD4+ T cells, CD8+ T cells, neutrophils, macrophages, and myeloid dendritic cells (DCs), myeloid derived suppressor cells (MDSC)] in DLBCL (Li et al., 2020).
Statistical Analysis
All data were analyzed by the SPSS 20.0 (IBM, United States) or GraphPad Prism 7 (GraphPad Software Inc., United States) software. p < 0.05 was considered as statistical significance. p < 0.01 was considered as obvious statistical significance.
Results
Filtering of Active Constituents of Qi-Invigorating Herbs
Among Qi-invigorating herbs, 20 active ingredients were identified from Ginseng Radix et Rhizoma, 9 from Panacis Quinquefolii Radix, 19 from Codonopsis Radix, 6 from Pseudostellariae Radix, 16 from Astragali Radix, 5 from Atractylodis Macrocephalae Rhizoma, 16 from Dioscoreae Rhizoma and 76 from Glycyrrhizae Radix et Rhizoma. The details of active ingredients were summarized in Supplementary Table S1.
Screening of Drug Targets
Through the SwissTargetPrediction and Stitch platforms, 63 genes were selected as candidate targets of Ginseng Radix et Rhizoma, 27 genes as candidate targets of Panacis Quinquefolii Radix, 62 genes as candidate targets of Codonopsis Radix, 63 genes as candidate targets of Pseudostellariae Radix, 121 genes as candidate targets of Astragali Radix, 0 genes as candidate targets of Atractylodis Macrocephalae Rhizoma, 23 genes as candidate targets of Dioscoreae Rhizoma, and 136 genes as candidate targets of Glycyrrhizae Radix et Rhizoma. The drug-active compounds-targets network diagrams were shown in Figure 2 and the target names of Qi-invigorating herbs were summarized in Supplementary Table S2.
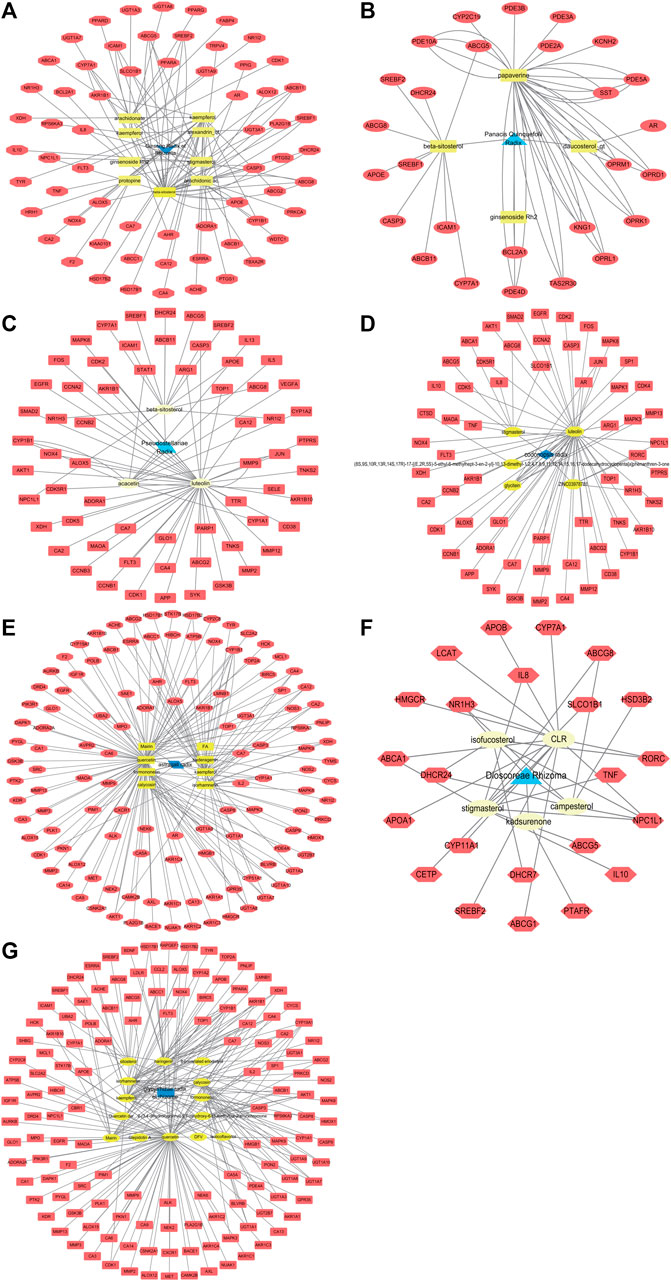
FIGURE 2. Network diagrams of drug-active ingredients-targets. The red nodes represent target genes, the yellow nodes represent active ingredients, while the blue nodes mean herb. (A). The network diagram of Ginseng Radix et Rhizoma-active ingredients-targets; (B). The network diagram of Panacis Quinquefolii Radix-active ingredients-targets; (C). The network diagram of Pseudostellariae Radix-active ingredients-targets; (D). The network diagram of Codonopsis Radix-active ingredients-targets; (E). The network diagram of Astragali Radix-active ingredients-targets; (F). The network diagram of Dioscoreae Rhizoma-active ingredients-targets; (G). The network diagram of Glycyrrhizae Radix et Rhizoma-active ingredients-targets.
Screening of Common Targets Between Qi-Invigorating Herbs and Diffuse Large B Cell Lymphoma
A total of 1174 target genes associated with DLBCL were retrieved from the PubMed, DisGeNET, and Malacards databases. Common targets of DLBCL and each Qi-invigorating herb were considered as interacting targets between herbs and DLBCL. As shown in Table 1, there existed 14 interacting targets between Ginseng Radix et Rhizoma and DLBCL, 4 targets between Panacis Quinquefolii Radix and DLBCL, 22 targets between Codonopsis Radix and DLBCL, 22 targets between Pseudostellariae Radix and DLBCL, 35 targets between Astragali Radix and DLBCL, 2 targets between Dioscoreae Rhizoma and DLBCL, and 36 targets between Glycyrrhizae Radix et Rhizoma and DLBCL.
Gene Ontology and Pathway Functional Enrichment Analyses of Interacting Targets
To further explore functions of the above interacting targets, GO and pathway functional enrichment analyses were performed with DAVID Bioinformatics Resources 6.8. It was demonstrated that there were some similarities as well as differences among Qi-invigorating herbs. According to p-values, the top 5 BP, MF, CC and KEGG pathways of interacting targets were shown in Table 2. For instance, interacting targets between Ginseng Radix et Rhizoma and DLBCL had main KEGG pathways in African trypanosomiasis, NF-kappa B signaling pathway, amoebiasis, TNF signaling pathway, natural killer cell mediated cytotoxicity; while interacting targets between Panacis Quinquefolii Radix and DLBCL had main pathways in viral myocarditis, NF-kappa B signaling pathway, TNF signaling pathway; interacting targets between Codonopsis Radix and DLBCL had main pathways in hepatitis B, T cell receptor signaling pathway, pertussis, viral carcinogenesis, progesterone-mediated oocyte maturation; interacting targets between Pseudostellariae Radix and DLBCL had main pathways in hepatitis B, Epstein-Barr virus infection, TNF signaling pathway, pathways in cancer, osteoclast differentiation; interacting targets between Astragali Radix and DLBCL had main pathways in pathways in cancer, progesterone-mediated oocyte maturation, toxoplasmosis, oocyte meiosis, colorectal cancer; interacting targets between Dioscoreae Rhizoma and DLBCL had main pathways in asthma, African trypanosomiasis, allograft rejection, malaria, inflammatory bowel disease; interacting targets between Glycyrrhizae Radix et Rhizoma and DLBCL had main pathways in pathways in cancer, progesterone-mediated oocyte maturation, chagas disease, TNF signaling pathway, toxoplasmosis.
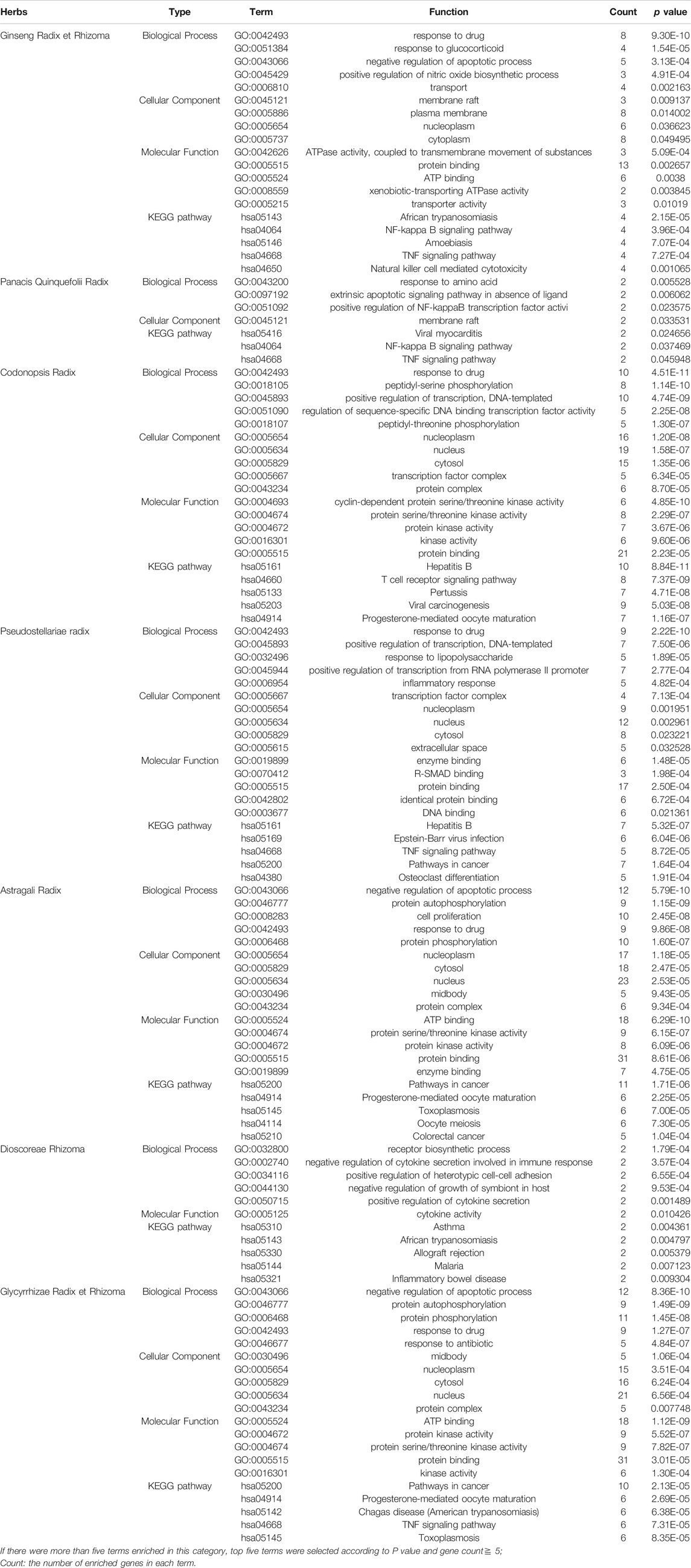
TABLE 2. GO and signaling pathway analysis of common targets between Qi-invigorating herbs and DLBCL.
Construction of PPI Networks and Screening of Hub Genes
Using the STRING online database and Cytoscape software, interacting targets between Ginseng Radix et Rhizoma and DLBCL were filtered into the PPI network complex, containing 14 nodes and 38 edges. Among the 14 nodes, 11 nodes were considered significant, with a combined score ≧ 0.7. CASP3 was identified as the hub gene with the filtering of degree ≧ 5. Similarly, 20 nodes were considered significant among 22 interacting targets between Codonopsis Radix and DLBCL. 14 genes were identified as hub genes with the filtering of degree ≥ 5 criteria, including JUN, CASP3, CDK2, AKT1, MAPK3, MAPK1, CDK5, CCNB1, CDK1, CDK4, CCNA2, FOS, TNF, APP.19 nodes were considered significant among 22 interacting targets between Pseudostellariae Radix and DLBCL. 10 hub genes were identified with the filtering of degree ≥ 5 criteria, including JUN, CASP3, AKT1, CDK5, CDK2, CCNB1, CCNA2, STAT1, VEGFA, CDK1. Besides, 31 nodes were considered significant among 35 interacting targets between Astragali Radix and DLBCL. 14 hub genes were identified with the filtering of degree ≥ 5 criteria, including CASP3, MAPK3, CDK1, AKT1, BIRC5, TOP2A, AURKB, MCL1, PLK1, NEK2, AR, CASP9, CASP8, TYMS. Furthermore, 32 nodes were considered significant among 36 interacting targets between Glycyrrhizae Radix et Rhizoma and DLBCL. 13 genes were identified as hub genes with the filtering of degree ≥ 5 criteria, including CASP3, MAPK3, AKT1, BIRC5, CDK1, TOP2A, MCL1, AURKB, NEK2, IL2, CASP9, BDNF, PLK1. No hub genes were found in Panacis Quinquefolii Radix and Dioscoreae Rhizoma.
Gene Ontology and Pathway Functional Enrichment Analyses of Hub Genes
We also performed GO and KEGG pathway enrichment analyses on the hub genes. Bar charts of GO (BP, CC, MF) and bubble charts of KEGG pathways were obtained. As shown in Figure 3, GO and KEGG enrichment analyses predicted hub genes in Codonopsis Radix mainly involved in response to reactive oxygen species, serine/threonine protein kinase complex, cyclin-dependent protein serine/threonine kinase activity, progesterone-mediated oocyte maturation and so forth; hub genes in Pseudostellariae Radix mainly involved in response to reactive oxygen species, serine/threonine protein kinase complex, histone kinase activity, progesterone-mediated oocyte maturation and so forth; hub genes in Astragali Radix mainly involved in execution phase of apoptosis, spindle, cysteine-type endopeptidase activity involved in apoptotic signaling pathway, colorectal cancer and so forth; while hub genes in Glycyrrhizae Radix et Rhizoma mainly involved in signal transduction in absence of ligand, spindle, protein serine/threonine kinase activity, protein serine/threonine kinase complex, colorectal cancer and so forth. It is shown that these hub genes mainly enriched in cancer-related pathways.
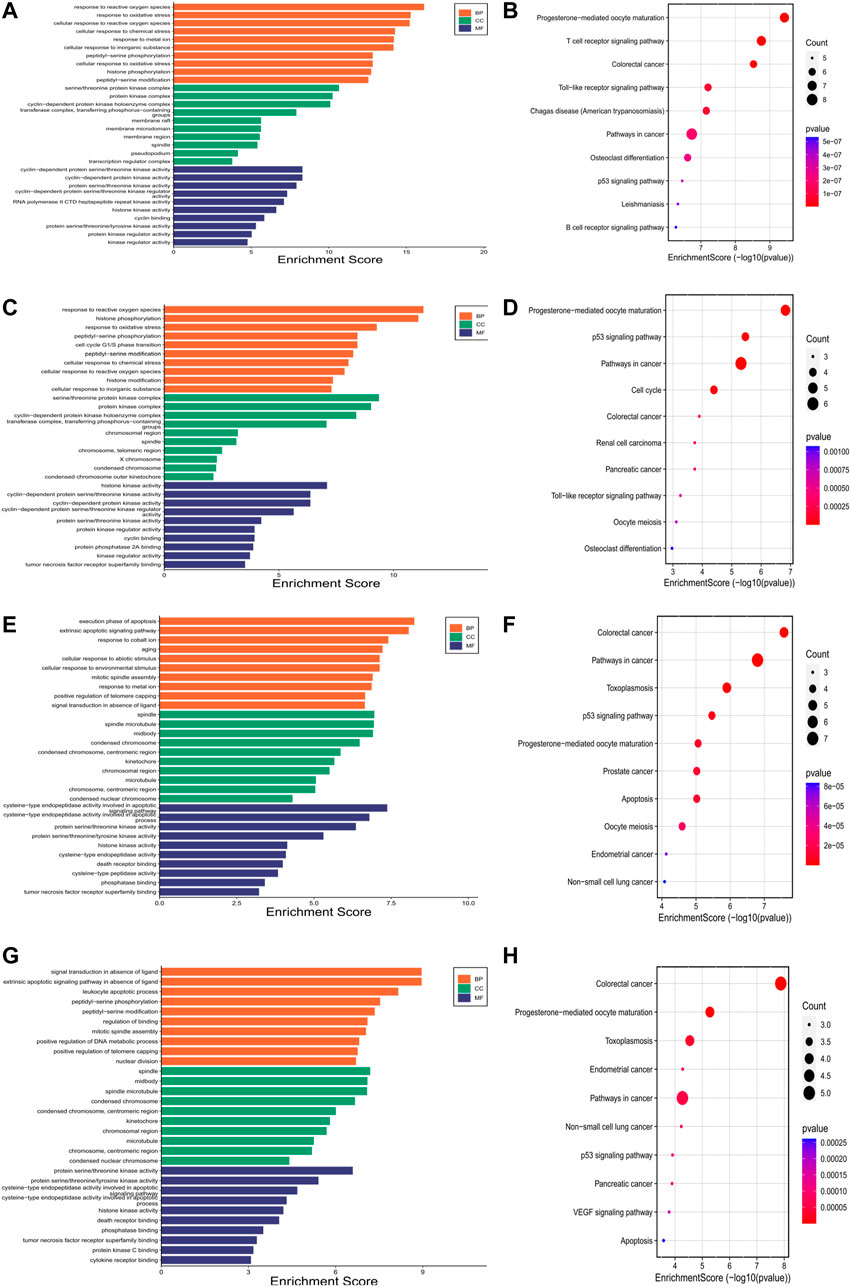
FIGURE 3. Gene Ontology and Kyoto Encyclopedia of Genes and Genomes analyses of hub genes. Each figure contains top ten pathways of Gene Ontology or Kyoto Encyclopedia of Genes and Genomes. In Gene Ontology enrichment analysis, Y-axis is biological process (BP), cellular component (CC) and molecular function (MF), X-axis is enrichment score. In Kyoto Encyclopedia of Genes and Genomes analysis, Y-axis is pathway name, X-axis is enrichment score. Bubble size represents gene numbers involved in the pathway enrichment. Color represents p-value, the redder the color, the smaller the p-value. (A). Gene Ontology analysis of hub genes for Codonopsis Radix; (B). Pathway enrichment analysis of hub genes for Codonopsis Radix; (C). Gene Ontology analysis of hub genes for Pseudostellariae Radix; (D). Pathway enrichment analysis of hub genes for Pseudostellariae Radix; (E). Gene Ontology analysis of hub genes for Astragali Radix; (F). Pathway enrichment analysis of hub genes for Astragali Radix; (G). Gene Ontology analysis of hub genes for Glycyrrhizae Radix et Rhizoma; (H). Pathway enrichment analysis of hub genes for Glycyrrhizae Radix et Rhizoma.
From the top ten KEGG pathway results, the significantly enriched hub genes were CASP3, CDK2, AKT1, MAPK3, MAPK1, CCNB1, CDK1, CCNA2, JUN, CDK4, FOS, TNF in Codonopsis Radix; CASP3, AKT1, CDK2, CCNB1, CCNA2, CDK1, JUN, STAT1, VEGFA in Pseudostellariae Radix; CASP3, MAPK3, AKT1, BIRC5, CASP9, AR, CASP8, PLK1, CDK1 in Astragali Radix; CASP3, MAPK3, AKT1, BIRC5, CASP9, CDK1, PLK1 in Glycyrrhizae Radix et Rhizoma.
Molecular Docking Verification
Through AutoDock Vina, all the hub genes and their related active ingredients were run for molecular docking. As shown in Figure 4, 16 active ingredients and 28 hub genes were run via AutoDock Vina for molecular docking. The spatial coordinates and the lowest binding affinity are shown in Table 3. Results in Figure 4 showed that in Ginseng Radix et Rhizoma, beta-sitosterol had a good affinity to CASP3 through hydrogen bonding. In Codonopsis Radix, luteolin might suppress cancer cell growth through hydrogen-bonding with AKT1, APP, CASP3, CCNA2, CCNB1, CDK1, CDK2, CDK5, FOS, JUN; glycitein could hydrogen-bond with CDK4, JUN, MAPK1, MAPK3; while stigmasterol only bound with TNF by van der Waals forces. In Pseudostellariae Radix, good affinities were also detected between luteolin and hub genes, including AKT1, CASP3, CCNA2, CCNB1, CDK1, CDK2, CDK5, JUN. Besides, acacetin and JUN, STAT1, VEGFA were bound together by hydrogen bonds. Beta-sitosterol took effect through CASP3. In Astragali Radix, quercetin might hold together with AKT1, AUKRB, CDK1, NEK2, PLK1 through hydrogen bonds, with MCL1 by van der Waals forces; mairin was bound with AKT1, BIRC5, TOP2A by hydrogen bonds, with CASP3 by van der Waals forces. Formononetin might induce cancer apoptosis through hydrogen-bonding with CASP3, CASP8, CASP9. Isorhamnetin, kaempferol, FA, calycosin, hederagenin might play an anti-tumor role through targeting AKT1, CDK1 TYMS, MAPK3, AR, respectively. As for Glycyrrhizae Radix et Rhizoma, similar results were found in quercetin, formononetin, isorhamnetin, kaempferol, calycosin and mairin. Meanwhile, CASP3, AKT1, IL2, BDNF could also bond with sitosterol, formononetin, naringenin, 2-(3,4-dihydroxyphenyl)-5,7-dihydroxy-6-(3-methylbut-2-enyl) chromone, respectively.
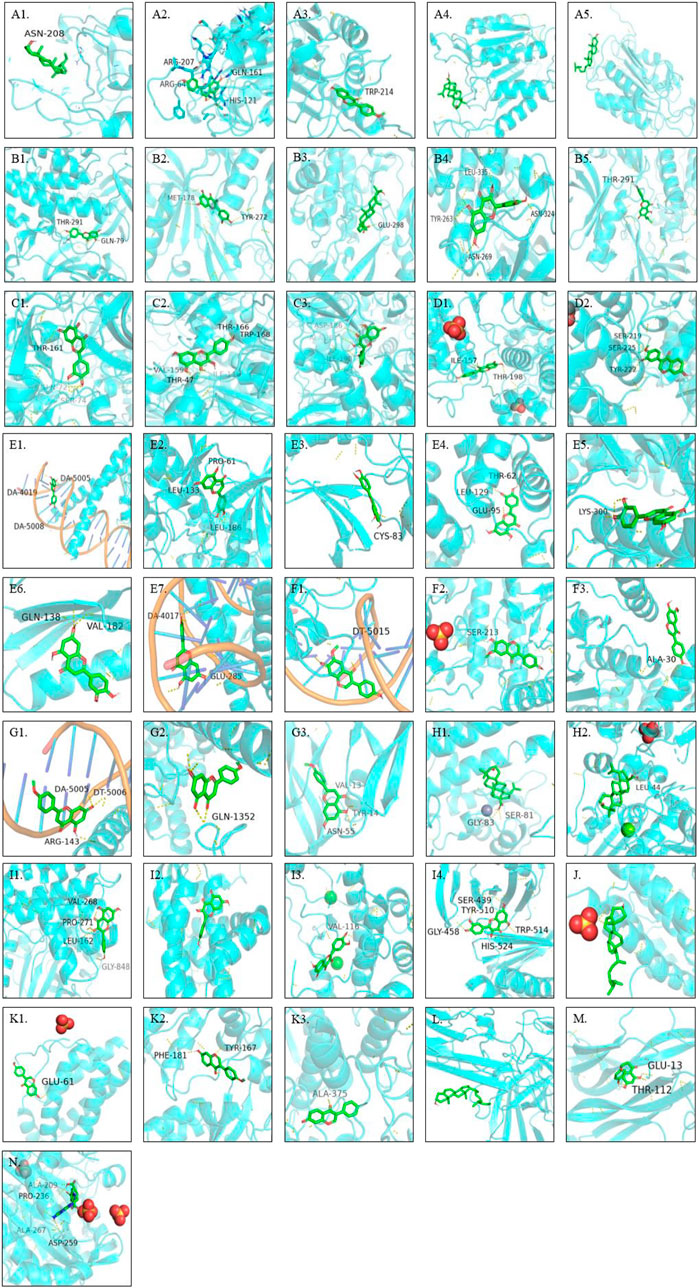
FIGURE 4. Molecular docking results: A1-A5. CASP3 and beta-sitosterol, luteolin, formononetin, mairin, sitosterol, respectively; B1-B5. AKT1 and luteolin, isorhamnetin, mairin, quercetin,2-(3,4-dihydroxyphenyl)-5,7-dihydroxy-6-(3-methylbut-2-enyl)chromone, respectively; C1-C3. CDK1 and luteolin, kaempferol, quercetin, respectively; D1-D2. MAPK3 and glycitein, calycosin, respectively; E1-E7. luteolin and JUN, CDK2, CDK5, CCNB1, CCNA2, APP, FOS, respectively; F1-F3. glycitein and JUN, MAPK1, CDK4, respectively; G1-G3. acacetin and JUN, STAT1, VEGFA, respectively; H1-H2. mairin and BIRC5, TOP2A, respectively; I1-I4. quercetin and AUKRB, MCL1, NEK2, PLK1, respectively; J. hederagenin and AR; K1-K3. formononetin and IL2, CASP8, CASP9, respectively; L. stigmasterol and TNF; M. naringenin and BDNF; N. FA and TYMS.
Correlation Between Hub Genes and Survival Time
In order to discover whether hub genes had correlations with DLBCL prognosis, hub genes were input into the GEPIA 2.0 database. Firstly, we tested their transcription levels in datasets concerning DLBCL vs non-tumor tissues. As shown in Figure 5A, all the hub genes were differently expressed in DLBCL as compared to those in non-tumor tissues. Then comparison of each gene’s survival curves was made and p values were calculated by log-rank. Results showed that only alteration in CASP3 and BDNF correlated with disease-free survival, for their p-values of log-rank were less than or close to 0.05, when group cutoff was set to 50% (Figures 5B,C). In view of the small number of samples, a retrospective study with larger sample is expected to confirm the conclusion.
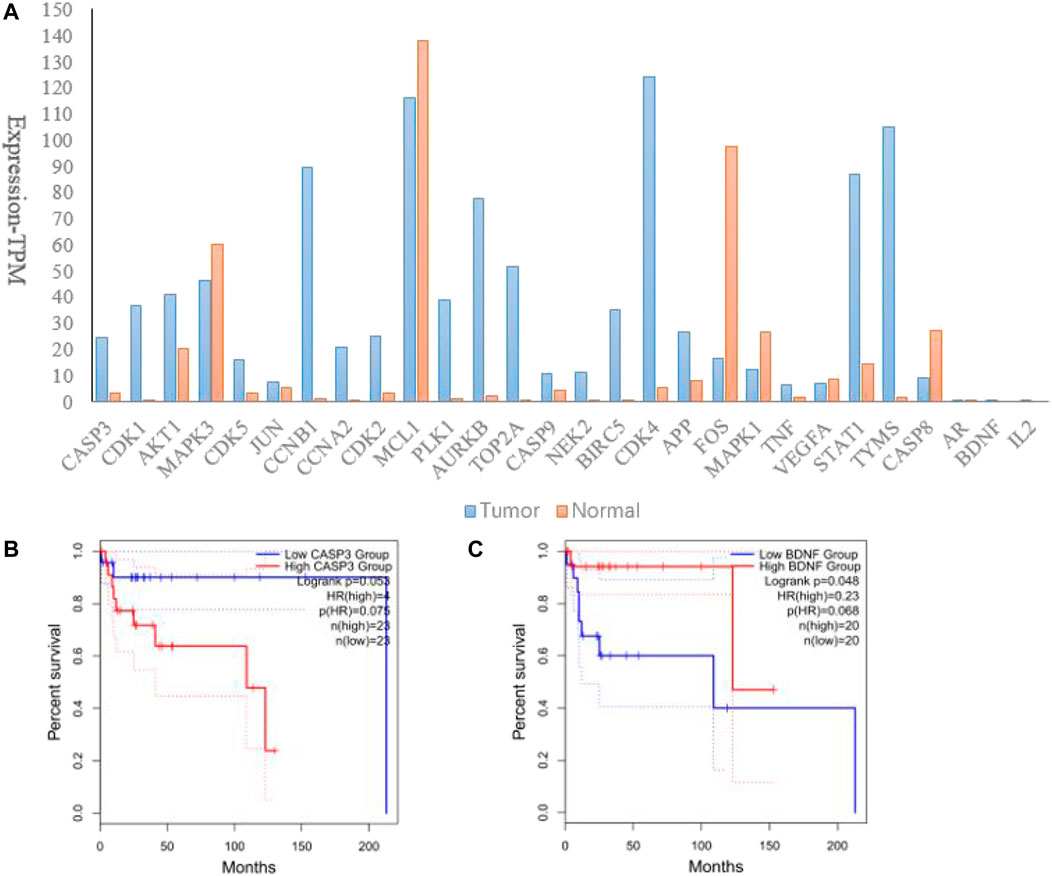
FIGURE 5. Verification of hub genes in the GEPIA 2.0 database. (A). Expression of hub genes in DLBCL as compared to those in non-tumor tissues; (B). Correlation between CASP3 and disease-free survival in DLBCL; (C). Correlation between BDNF and disease-free survival in DLBCL.
Common Target Genes and Active Ingredients of Different Qi-Invigorating Herbs
It is noteworthy that different Qi-invigorating herbs exist same active ingredients, i.e. different Qi-invigorating herbs may target same genes in DLBCL. Thus, we first took the intersection of hub gene related ingredients. However, no common hub gene related ingredients were found. Subsequently, additional analysis was performed on hub genes. Through R software 4.1.0, it was detected that four hub genes (CASP3, CDK1, AKT1, MAPK3) corresponded to at least three of five Qi-invigorating herbs (Figure 6). Therefore, it is suggested that CASP3, CDK1, AKT1 and MAPK3 may be common target genes for different Qi-invigorating herbs acting on DLBCL.
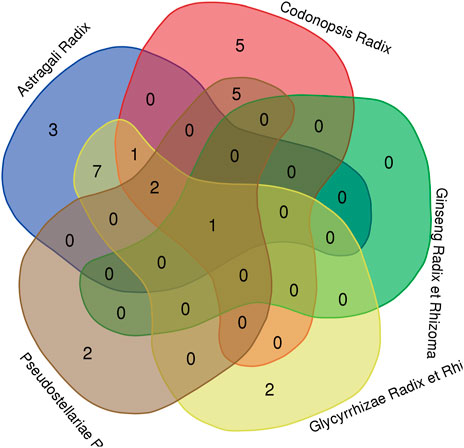
FIGURE 6. A Venn diagram for common hub genes. The red part represents Codonopsis Radix; the green part represents Ginseng Radix et Rhizoma; the yellow part represents Glycyrrhizae Radix et Rhizoma; the brown part represents Pseudostellariae Radix; the blue part represents Astragali Radix.
Experimental Verification
Cytotoxicity Study of Qi-Invigorating Herbs in Diffuse Large B Cell Lymphoma Cells
In order to verify the effect of common hub genes in Qi-invigorating herbs, an in vitro experiment was carried out. Firstly, we evaluated the cytotoxic efficacy of Qi-invigorating herbs in two DLBCL cell lines at concentrations between 3 and 10 mg/ml. Results showed that the IC50 values were 3.91 mg/ml, 4.01 mg/ml for Astragali Radix; 2.4 mg/ml, 1.94 mg/ml for Glycyrrhizae Radix et Rhizoma; 2.05 mg/ml, 3.2 mg/ml for Ginseng Radix et Rhizoma; 1.73 mg/ml, 4.11 mg/ml for Pseudostellariae Radix; 3.23 mg/ml, 4.1 mg/ml for Codonopsis Radix in SUDHL2 and SUDHL6 cells, respectively. Considering the cytotoxicity of all Qi-invigorating herbs, 5 mg/ml concentration of Qi-invigorating herbs was selected as a suitable intervention concentration for subsequent experiments.
The Role of Qi-Invigorating Herbs in the Proliferation of Diffuse Large B Cell Lymphoma Cells
CCK-8 assays were applied to determine the effects of Astragali Radix, Glycyrrhizae Radix et Rhizoma, Ginseng Radix et Rhizoma, Pseudostellariae Radix and Codonopsis Radix on DLBCL cell proliferation. Results showed that the proliferation ability of SU-DHL-2 and SU-DHL-6 cells was decreased via incubating with Qi-invigorating herbs (Figures 7A,B).
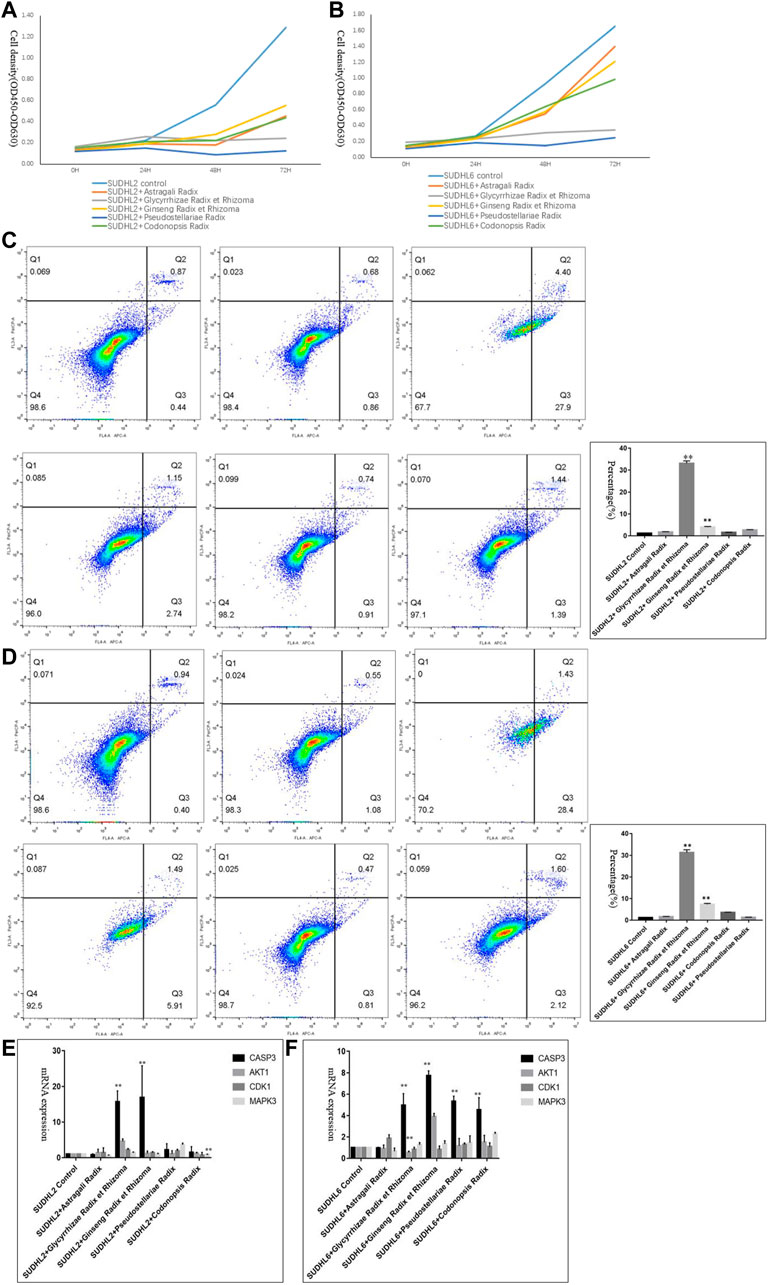
FIGURE 7. Experimental validation in vitro. (A). The proliferative ability of SUDHL2 cells incubating with different Qi-invigorating herbs; (B). The proliferative ability of SUDHL6 cells incubating with different Qi-invigorating herbs; (C). The cell apoptosis of SU-DHL-2 cells incubating with different Qi-invigorating herbs was measured through flow cytometry analysis (From upper left to right: Negative control, SUDHL2 cells treated with Astragali Radix, SUDHL2 cells treated with Glycyrrhizae Radix et Rhizoma; From lower left to right: SUDHL2 cells treated with Ginseng Radix et Rhizoma, SUDHL2 cells treated with Pseudostellariae Radix, SUDHL2 cells treated with Codonopsis Radix); (D). The cell apoptosis of SU-DHL-6 cells incubating with different Qi-invigorating herbs was measured through flow cytometry analysis (From upper left to right: Negative control, SUDHL6 cells treated with Astragali Radix, SUDHL6 cells treated with Glycyrrhizae Radix et Rhizoma; from lower left to right: SUDHL6 cells treated with Ginseng Radix et Rhizoma, SUDHL6 cells treated with Pseudostellariae Radix, SUDHL6 cells treated with Codonopsis Radix); (E). mRNA expression of CASP3, AKT1, CDK1, MAPK3 in SUDHL2 cells incubating with different Qi-invigorating herbs; (F). mRNA expression of CASP3, AKT1, CDK1, MAPK3 in SUDHL6 cells incubating with different Qi-invigorating herbs. *p < 0.05 and **p < 0.01.
The Role of Qi-Invigorating Herbs in the Apoptosis of Diffuse Large B Cell Lymphoma Cells
To measure the induction of apoptosis, cells were treated with 5 mg/ml of Astragali Radix, Glycyrrhizae Radix et Rhizoma, Ginseng Radix et Rhizoma, Pseudostellariae Radix and Codonopsis Radix decoctions for 48 h, respectively. Flow cytometry analysis exhibited that incubation with Glycyrrhizae Radix et Rhizoma resulted in a remarkable rise in cell apoptosis [(33.09 ± 0.99)% in SUDHL2 and (31.3 ± 1.3)% in SUDHL6], comparing to negative control groups (Figures 7C,D). Meanwhile cell apoptosis in DLBCL cells treated with Ginseng Radix et Rhizoma was slightly elevated (Figures 7C,D). Thus it is suggested that some Qi-invigorating herbs participated in the apoptosis of DLBCL cells.
The Role of Common Hub Genes in Diffuse Large B Cell Lymphoma Cells Treated With Qi-Invigorating Herbs
In order to further elaborate the roles of common hub genes, we tested CASP3, CDK1, AKT1 and MAPK3 expression in DLBCL cells incubated with Qi-invigorating herbs, respectively. As shown in Figures 7E,F, DLBCL cells treated with Glycyrrhizae Radix et Rhizoma or Ginseng Radix et Rhizoma had an effectively increase in CASP3 at mRNA levels compared with negative control groups, suggesting that Qi-invigorating herbs regulated DLBCL cell apoptosis mainly through targeting CASP3. It was noteworthy that most CDK1, AKT1, MAPK3 expression did not change significantly in DLBCL cells treated with Qi-invigorating herbs (Figures 7E,F). Therefore, it is concluded that CDK1, AKT1 and MAPK3 are not common hub genes in DLBCL cells treated with Qi-invigorating herbs.
Tumor Microenvironment Analysis
To further explore the mechanisms of Qi-invigorating herbs acting on DLBCL TME, we conducted the analysis of the relationship between hub genes and tumor-infiltrating immune cells (B cells, CD4+ T cells, CD8+ T cells, neutrophils, macrophages, myeloid DCs, MDSCs) from the TIMER 2.0 database. As shown in Figure 8, the expression of AKT1 was negatively associated with tumor purity (r = −0.386, p = 1.15e-02), positively correlated with CD8+ T cells (r = 0.523, p = 4.50e-04) and neutrophils (r = 0.583, p = 6.44e-05) in infiltrating levels. Similar relationships were found in CASP8, JUN, APP and MCL1 (p < 0.05). CASP3, CDK2, MAPK1, TOP2A, MAPK3 only had positive significant correlation with CD8+ T cells and neutrophils (r > 0, p < 0.01). CDK1, CCNA2, FOS had statistical correlation with CD4+ T cells and neutrophils (p < 0.05), while BIRC5 and CCNB1 correlated with both neutrophils and MDSCs (p < 0.05). PLK1, VEGFA, AR only correlated with neutrophils (p < 0.05), TYMS, AURKB, TNF mainly correlated with MDSCs (p < 0.05). The expression of STAT1 was negatively associated with tumor purity (r = −0.451, p = 2.69e-03), B cells (r = −0.364, p = 1.94e-02), macrophage (r = −0.499, p = 8.89e-04) and MDSCs (r = −0.531, p = 3.57e-04) in infiltrating levels, but positively correlated with CD8+ T cells (r = 0.641, p = 6.20e-06), myeloid dendritic cells (r = 0.62, p = 1.55e-05) and neutrophils (r = 0.809, p = 1.55e-10) in infiltrating levels. However, no correlation was found among BDNF, CDK5, IL2 and tumor-infiltrating immune cells in DLBCL. Since most hub genes were associated with neutrophils in infiltrating levels, we speculated that Qi-invigorating herbs mainly affects DLBCL microenvironment through acting on neutrophils.
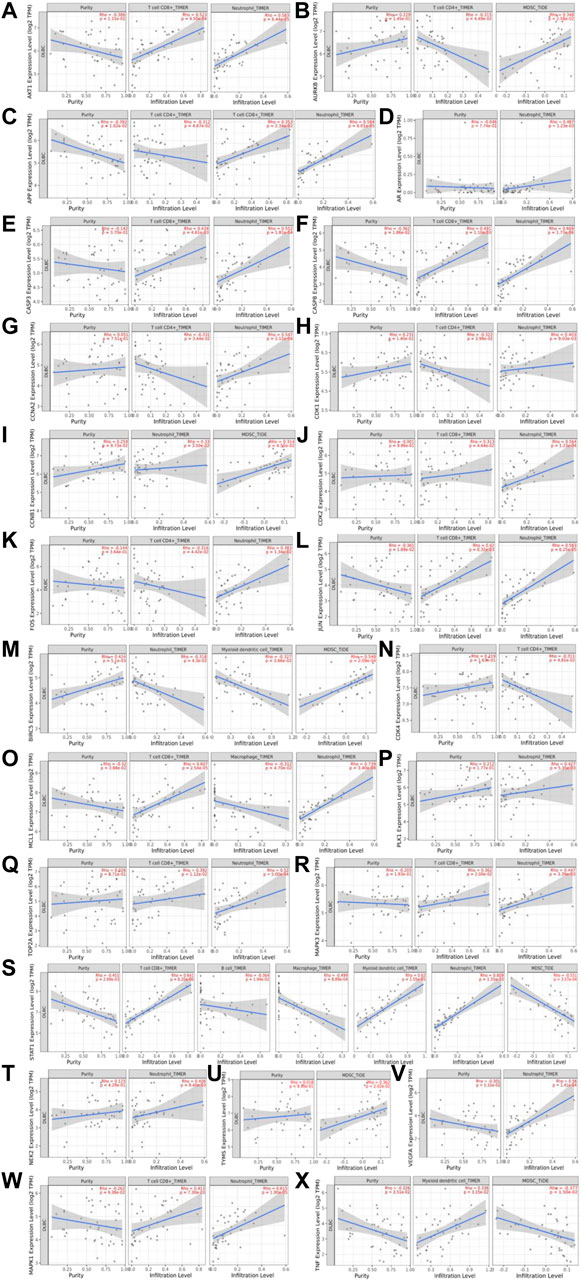
FIGURE 8. The relationship between hub genes and tumor-infiltrating immune cells. (A). The relationship between AKT1 and tumor-infiltrating immune cells (CD8+ T cells and neutrophils); (B). The relationship between AURKB and tumor-infiltrating immune cells (CD4+ T cells and MDSCs); (C). The relationship between APP and tumor-infiltrating immune cells (CD4+ T cells, CD8+ T cells and neutrophils); (D). The relationship between AR and neutrophils; (E). The relationship between CASP3 and tumor-infiltrating immune cells (CD8+ T cells and neutrophils); (F). The relationship between CASP8 and tumor-infiltrating immune cells (CD8+ T cells and neutrophils); (G). The relationship between CCNA2 and tumor-infiltrating immune cells (CD4+ T cells and neutrophils); (H). The relationship between CDK1 and tumor-infiltrating immune cells (CD4+ T cells and neutrophils); (I). The relationship between CCNB1 and tumor-infiltrating immune cells (MDSCs and neutrophils); (J). The relationship between CDK2 and tumor-infiltrating immune cells (CD8+ T cells and neutrophils); (K). The relationship between FOS and tumor-infiltrating immune cells (CD4+ T cells and neutrophils); (L). The relationship between JUN and tumor-infiltrating immune cells (CD8+ T cells and neutrophils); (M). The relationship between BIRC5 and tumor-infiltrating immune cells (neutrophils, myeloid dendritic cells and MDSCs); (N). The relationship between CDK4 and CD4+ T cells; (O). The relationship between MCL1 and neutrophils, CD8+ T cells, macrophage; (P). The relationship between PLK1 and neutrophils; (Q). TOP2A and tumor-infiltrating immune cells (CD8+ T cells and neutrophils); (R). The relationship between MAPK3 and tumor-infiltrating immune cells (CD8+ T cells and neutrophils); (S). The relationship between STAT1 and tumor-infiltrating immune cells (B cells, CD8+ T cells, macrophage, myeloid dendritic cells, neutrophils, MDSCs); (T). The relationship between NEK2 and neutrophils; (U). The relationship between TYMS and MDSCs; (V). The relationship between VEGFA and neutrophils; (W). MAPK1 and tumor-infiltrating immune cells (CD8+ T cells and neutrophils); (X). The relationship between TNF and myeloid dendritic cells, MDSCs. Myeloid derived suppressor cells: MDSC.
Discussion
TCM is a very important cancer treatment strategy in China. It has been widely reported that TCM herbs can improve DLBCL survival and provide a complementary therapy for cases that are refractory or have relapsed. According to the TCM theory, disturbance of Yin-Yang is one of the main reasons for carcinogenesis. To alleviate symptoms of disease, TCM aims to restore the balance of Yin and Yang in addition to eliminate pathogenic factors (“Quxie”), which is somewhat similar to TME from the view of theory. Based on preclinical studies, Qi-invigorating herbs have been proved to have immune enhancement and multi-target regulation. Therefore, a systematic study of Qi-invigorating herbs in DLBCL would elucidate their mechanisms and provide guidance for herb selection, or even personalized therapy.
In this study, eight commonly used Qi-invigorating herbs were included, which have been widely employed in herbal prescriptions for cancer treatment. Active ingredients were first selected from the TCMSP database. Then potential targets were output via the SwissTargetPrediction and STITCH databases. By intersection with target genes of DLBCL, it was found that besides Atractylodis Macrocephalae Rhizoma, the other seven Qi-invigorating herbs could act on DLBCL. GO and KEGG pathway enrichment results revealed that there were some similarities as well as differences among Qi-invigorating herbs.
Through PPI construction networks, hub genes were output, which implicated Ginseng Radix et Rhizoma, Codonopsis Radix, Pseudostellariae Radix, Astragali Radix, Glycyrrhizae Radix et Rhizoma might be more appropriate for herbal prescriptions in treating DLBCL. The suppressed proliferation by these Qi-invigorating herbs in vitro verified this view. Excellent molecular docking results for hub genes and their corresponding ingredients further confirmed this view.
Interestingly, CASP3, CDK1, AKT1 and MAPK3 were considered as common target genes. Among them, CASP3 is one important member of caspases, characterized by programmed cell death (Galluzzi et al., 2016). It is often used as a marker for efficacy of cancer therapy. In TME, it has been indicated that CASP3 activation could trigger pyroptosis, a form of cell death that is critical for immunity by cleaving gasdermin E (GSDME) in GSDME-expressing tumors (Wang et al., 2017; Zhang et al., 2020). Similar finding was observed in T-cell lymphoma, where immunogenic cell death depended on CASP3 activity, with reduced antitumor immunity generated by CASP3-deficient EL4 cells (Jaime-Sanchez et al., 2020). Our study detected that DLBCL cells treated with Glycyrrhizae Radix et Rhizoma or Ginseng Radix et Rhizoma promoted their apoptosis accompanied by up-regulated CASP3. Thus it is concluded that Qi-invigorating herbs regulated DLBCL cell apoptosis mainly through targeting CASP3. In view of the correlation between CASP3 and DLBCL prognosis, it is of great significance to further explore the mechanisms of Glycyrrhizae Radix et Rhizoma and Ginseng Radix et Rhizoma acing on CASP3 in DLBCL.
Although CDK1, AKT1 and MAPK3 were considered as other common hub genes for Qi-invigorating herbs, most CDK1, AKT1, MAPK3 expression did not change significantly in vitro. Therefore, it is concluded that CDK1, AKT1 and MAPK3 are not common hub genes in DLBCL cells treated with Qi-invigorating herbs. In this study we have well described the effect of common hub genes by wet lab experiments. The effects and mechanisms of other hub genes required further verification in the future. The detailed mechanisms obtained by experiments may provide a guidance for herb selection, prescription formation, new drug design, or even personalized therapy.
Molecular docking results revealed that one common hub gene exhibited good binding energy for multiple active ingredients and one ingredient targeted more than one hub gene. Based on binding affinity and specific ligand-receptor residue interactions, there are six active ingredients (luteolin, glycitein, acacetin, quercetin, mairin, formononetin) targeting more than three hub genes in each Qi-invigorating herb, indicating they have potential as DLBCL drugs. So far, some of these ingredients have been well described for their anti-lymphoma effects in experimental studies. luteolin, an active ingredient extracted from Codonopsis Radix and Pseudostellariae Radix, has been tested to inhibit the proliferation of classical Hodgkin’s lymphoma cells via caspase activation (Gharbaran et al., 2020). Quercetin, an active ingredient of Astragali Radix and Glycyrrhizae Radix et Rhizoma, has been detected to inhibit cancer cell growth by modulating AKT and NF-κB pathways in lymphoma models (Soofiyani et al., 2021). The experimental results also displayed the prospect of Qi-invigorating herbs in DLBCL treatment. Thus, more pharmacodynamic studies are required to examine their effects in DLBCL models.
Besides directly targeting cancer cells, previous evidence suggested that Qi-invigorating herbs could exert antitumor effects by upregulating immune responses even in immunosuppressive TME. Therefore, to explore the mechanisms of Qi-invigorating herbs for DLBCL treatment in depth, we conduct a comprehensive TME analysis for hub genes. In DLBCL microenvironment, although much is still unknown concerning the composition of immune cells, CD4+ T cells, CD8+ T cells, neutrophils, macrophages, myeloid DCs and MDSCs have been widely tested as independent predictors of DLBCL outcome (Azzaoui et al., 2016; Chen et al., 2016; Judd et al., 2017; Kusano et al., 2017; Ciavarella et al., 2018; Staiger et al., 2020; Merdan et al., 2021; Xu et al., 2021). Manfroi et al. (Manfroi et al., 2017) further demonstrated an association between neutrophils and A Proliferation-Inducing TNF Ligand (APRIL). They reported that APRIL produced by DLBCL-related neutrophils increased tumor aggressiveness and affected disease prognosis (Manfroi et al., 2017). In our study, the relationship between hub genes and tumor-infiltrating immune cells (B cells, CD4+ T cells, CD8+ T cells, neutrophils, macrophages, myeloid DCs and MDSCs) was tested via the TIMER 2.0 database. Since most hub genes were found to be associated with neutrophils in infiltrating levels, it was reasonable to speculate that Qi-invigorating herbs mainly affected DLBCL TME through acting on tumor-associated neutrophils. Further research is needed to validate our findings.
In a word, this study systematically explored the active ingredients, potential targets, hub genes and the impact of immune cells in Qi-invigorating herbs for DLBCL treatment. Hub genes and immune infiltrating cells provided the molecular basis for each Qi-invigorating herb acting on DLBCL. Study on common mechanisms revealed that CASP3 might be the common target of Qi-invigorating herbs on DLBCL apoptosis. Tumor-associated neutrophils may be main target cells of DLBCL treated by Qi-invigorating herbs. Although this study provided preliminary predictions, it was important for the modernization of TCM. Such researches would undoubtedly increase our understanding of DLBCL pathogenesis. However, the conclusions still need to be verified with subsequent wet lab experiments.
Conclusion
Through network pharmacology analysis and experimental validation, Qi-invigorating herbs were proved to be appropriate for DLBCL treatment. Potential targets, hub genes and targeted immune cells of Qi-invigorating herbs helped us to understand the mechanisms. Study on common mechanisms revealed that CASP3 might be the common target of Qi-invigorating herbs on DLBCL apoptosis. Tumor-associated neutrophils may be main target cells of DLBCL treated by Qi-invigorating herbs. However, the mechanisms of hub genes still required verification, which may provide a guidance for herb selection, prescription formation, new drug design, or even personalized therapy.
In all, our findings may provide a theoretical basis for the application of Qi-invigorating herbs in DLBCL. This article may provide a reference and clinical transformation value for TCM.
Data Availability Statement
The original contributions presented in the study are included in the article/Supplementary Materials, further inquiries can be directed to the corresponding author.
Author Contributions
QH: Data collection, data mining; Drafting the article; experimental validation; JL: Data collection and qRT-PCR; SH: Data collection and and qRT-PCR; JS: Conception and design of the work; Critical revision of the article; Final approval of the version to be published.
Funding
This study was supported by Hospital project of Fujian Medical University Union Hospital, Fujian, P.R.China (Grant No. 2020XH017); Projects of Industry, Education and Research of Fujian Science and Technology Department, Fujian, P.R.China (Grant No. 2018Y4004); Fujian Science and Technology Innovation Joint Fund Project, Fujian, P.R.China (Grant No. 2018Y9028); Grant-in-Aid for the National Natural Science Foundation of China (81300428, 81800167); Joints Funds for the Innovation of Science and Technology, Fujian Province (2018Y9010, 2018Y9205); Construction Project of Fujian Medical Center of Hematology, Fujian, P.R.China (Grant No. Min201704), National and Fujian Provincial Key Clinical Specialty Discipline Construction Program, P.R.China (Grant No. 2010301). On behalf of all authors, I declare all sources of funding received for the research have been submitted.
Conflict of Interest
The authors declare that the research was conducted in the absence of any commercial or financial relationships that could be construed as a potential conflict of interest.
Publisher’s Note
All claims expressed in this article are solely those of the authors and do not necessarily represent those of their affiliated organizations, or those of the publisher, the editors and the reviewers. Any product that may be evaluated in this article, or claim that may be made by its manufacturer, is not guaranteed or endorsed by the publisher.
Acknowledgments
This work was supported through funds provided by four projects. The authors thank all reviewers for their time, comments, and constructive criticism.
Supplementary Material
The Supplementary Material for this article can be found online at: https://www.frontiersin.org/articles/10.3389/fphar.2021.787816/full#supplementary-material
References
Ansell, S. M., Minnema, M. C., Johnson, P., Timmerman, J. M., Armand, P., Shipp, M. A., et al. (2019). Nivolumab for Relapsed/Refractory Diffuse Large B-Cell Lymphoma in Patients Ineligible for or Having Failed Autologous Transplantation: A Single-Arm, Phase II Study. J. Clin. Oncol. 37 (6), 481–489. doi:10.1200/JCO.18.00766
Autio, M., Leivonen, S. K., Brück, O., Mustjoki, S., Mészáros Jørgensen, J., Karjalainen-Lindsberg, M. L., et al. (2021). Immune Cell Constitution in the Tumor Microenvironment Predicts the Outcome in Diffuse Large B-Cell Lymphoma. Haematologica 106 (3), 718–729. doi:10.3324/haematol.2019.243626
Azzaoui, I., Uhel, F., Rossille, D., Pangault, C., Dulong, J., Le Priol, J., et al. (2016). T-cell Defect in Diffuse Large B-Cell Lymphomas Involves Expansion of Myeloid-Derived Suppressor Cells. Blood 128, 1081–1092. doi:10.1182/blood-2015-08-662783
Barrueto, L., Caminero, F., Cash, L., Makris, C., Lamichhane, P., and Deshmukh, R. R. (2020). Resistance to Checkpoint Inhibition in Cancer Immunotherapy. Transl. Oncol. 13 (3), 100738. doi:10.1016/j.tranon.2019.12.010
Ben-Arye, E., Attias, S., Tadmor, T., and Schiff, E. (2010). Herbs in Hemato-Oncological Care: an Evidence-Based Review of Data on Efficacy, Safety, and Drug Interactions. Leuk. Lymphoma 51 (8), 1414–1423. doi:10.3109/10428194.2010.487622
Berman, H. M., Westbrook, J., Feng, Z., Gilliland, G., Bhat, T. N., Weissig, H., et al. (2000). The Protein Data Bank. Nucleic Acids Res. 28 (1), 235–242. doi:10.1093/nar/28.1.235
Chen, Y., Bi, L., Luo, H., Jiang, Y., Chen, F., Wang, Y., et al. (2019). Water Extract of Ginseng and astragalus Regulates Macrophage Polarization and Synergistically Enhances DDP's Anticancer Effect. J. Ethnopharmacol. 232, 11–20. doi:10.1016/j.jep.2018.12.003
Chen, Y., Neelapu, S., Feng, L., Bi, W., Yang, T. H., Wang, M., et al. (2016). Prognostic Significance of Baseline Peripheral Absolute Neutrophil, Monocyte and Serum β2-microglobulin Level in Patients with Diffuse Large B-Cell Lymphoma: a New Prognostic Model. Br. J. Haematol. 175 (2), 290–299. doi:10.1111/bjh.14237
Ciavarella, S., Vegliante, M. C., Fabbri, M., De Summa, S., Melle, F., Motta, G., et al. (2018). Dissection of DLBCL Microenvironment Provides a Gene Expression-Based Predictor of Survival Applicable to Formalin-Fixed Paraffin-Embedded Tissue. Ann. Oncol. 29 (12), 2363–2370. doi:10.1093/annonc/mdy4510.1093/annonc/mdy450
Consortium, Uni. Prot. (2019). UniProt: a Worldwide Hub of Protein Knowledge. Nucleic Acids Res. 47 (D1), D506–D515. doi:10.1093/nar/gky1049
Daina, A., Michielin, O., and Zoete, V. (2019). SwissTargetPrediction: Updated Data and New Features for Efficient Prediction of Protein Targets of Small Molecules. Nucleic Acids Res. 47 (W1), W357–W364. doi:10.1093/nar/gkz382
Du, J., Cheng, B. C., Fu, X. Q., Su, T., Li, T., Guo, H., et al. (2016). In Vitro assays Suggest Shenqi Fuzheng Injection Has the Potential to Alter Melanoma Immune Microenvironment. J. Ethnopharmacol. 194, 15–19. doi:10.1016/j.jep.2016.08.038
Galluzzi, L., López-Soto, A., Kumar, S., and Kroemer, G. (2016). Caspases Connect Cell-Death Signaling to Organismal Homeostasis. Immunity 44 (2), 221–231. doi:10.1016/j.immuni.2016.01.020
Gharbaran, R., Shang, E., Onwumere, O., Codrington, N., Sarpong, E. D., and Redenti, S. (2020). Luteolin Induces Cytotoxicity in Mix Cellularity Classical Hodgkin's Lymphoma via Caspase Activated-Cell Death. Anticancer. Res. 40 (9), 4907–4912. doi:10.21873/anticanres.14493
Guo, Q., Lin, J., Liu, R., Gao, Y., He, S., Xu, X., et al. (2015). Review on the Applications and Molecular Mechanisms of Xihuang Pill in Tumor Treatment. Evid. Based Complement. Alternat. Med. 2015, 854307. doi:10.1155/2015/854307
Hijikata, Y., Kaneko, J., Xi, L., Nasu, M., and Yamashita, S. (1995). Traditional Chinese Medicines Improve the Course of Refractory Leukemic Lymphoblastic Lymphoma and Acute Lymphocytic Leukemia: Two Case Reports. Am. J. Chin. Med. 23 (2), 195–211. doi:10.1142/S0192415X95000250
Hopkins, A. L. (2008). Network Pharmacology: the Next Paradigm in Drug Discovery. Nat. Chem. Biol. 4 (11), 682–690. doi:10.1038/nchembio.118
Hou, Z., Liu, S., Song, F., Pi, Z., and Liu, Z. (2021). Comprehensive Physiopathology and Serum Metabolomics for the Evaluation of the Influence Mechanism of Qi Deficiency on Xenograft Mouse Models of Liver Cancer. J. Sep. Sci. 44, 3789–3798. doi:10.1002/jssc.202100260
Huang, da. W., Sherman, B. T., and Lempicki, R. A. (2009). Systematic and Integrative Analysis of Large Gene Lists Using DAVID Bioinformatics Resources. Nat. Protoc. 4 (1), 44–57. doi:10.1038/nprot.2008.211
Huang, D. W., Sherman, B. T., Tan, Q., Kir, J., Liu, D., Bryant, D., et al. (2007). DAVID Bioinformatics Resources: Expanded Annotation Database and Novel Algorithms to Better Extract Biology from Large Gene Lists. Nucleic Acids Res. 35, W169–W175. doi:10.1093/nar/gkm415
Jaime-Sanchez, P., Uranga-Murillo, I., Aguilo, N., Khouili, S. C., Arias, M. A., Sancho, D., et al. (2020). Cell Death Induced by Cytotoxic CD8+ T Cells Is Immunogenic and Primes Caspase-3-dependent Spread Immunity against Endogenous Tumor Antigens. J. Immunother. Cancer 8 (1), e000528. doi:10.1136/jitc-2020-000528
Jiang, Y. X., Chen, Y., Yang, Y., Chen, X. X., and Zhang, D. D. (2019). Screening Five Qi-Tonifying Herbs on M2 Phenotype Macrophages. Evid. Based Complement. Alternat. Med. 2019, 9549315. doi:10.1155/2019/9549315
Jin, D., Zhang, J., Zhang, Y., An, X., Zhao, S., Duan, L., et al. (2021). Network Pharmacology-Based and Molecular Docking Prediction of the Active Ingredients and Mechanism of ZaoRenDiHuang Capsules for Application in Insomnia Treatment. Comput. Biol. Med. 135, 104562. doi:10.1016/j.compbiomed.202110.1016/j.compbiomed.2021.104562
Judd, J., Dulaimi, E., Li, T., Millenson, M. M., Borghaei, H., Smith, M. R., et al. (2017). Low Level of Blood CD4+ T Cells Is an Independent Predictor of Inferior Progression-free Survival in Diffuse Large B-Cell Lymphoma. Clin. Lymphoma Myeloma. Leuk. 17 (2), 83–88. doi:10.1016/j.clml.2016.11.005
Kim, K., Kim, S. H., Ok, O. N., Kim, I. R., Lee, S., Kim, S. H., et al. (2018). Use of Complementary and Alternative Medicine by Lymphoma Survivors in South Korea. Eur. J. Oncol. Nurs. 33, 91–96. doi:10.1016/j.ejon.2018.01.012
Kim, S., Thiessen, P. A., Bolton, E. E., Chen, J., Fu, G., Gindulyte, A., et al. (2016). PubChem Substance and Compound Databases. Nucleic Acids Res. 44 (D1), D1202–D1213. doi:10.1093/nar/gkv951
Kline, J., Godfrey, J., and Ansell, S. M. (2020). The Immune Landscape and Response to Immune Checkpoint Blockade Therapy in Lymphoma. Blood 135 (8), 523–533. doi:10.1182/blood.2019000847
Kuhn, M., von Mering, C., Campillos, M., Jensen, L. J., and Bork, P. (2008). STITCH: Interaction Networks of Chemicals and Proteins. Nucleic Acids Res. 36 (Database issue), D684–D688. doi:10.1093/nar/gkm795
Kusano, Y., Yokoyama, M., Terui, Y., Nishimura, N., Mishima, Y., Ueda, K., et al. (2017). Low Absolute Peripheral Blood CD4+ T-Cell Count Predicts Poor Prognosis in R-CHOP-Treated Patients with Diffuse Large B-Cell Lymphoma. Blood Cancer J. 7 (4), e558. doi:10.1038/bcj.2017.37
Li, S., and Zhang, B. (2013). Traditional Chinese Medicine Network Pharmacology: Theory, Methodology and Application. Chin. J. Nat. Med. 11 (2), 110–120. doi:10.1016/S1875-5364(13)60037-0
Li, T., Fu, J., Zeng, Z., Cohen, D., Li, J., Chen, Q., et al. (2020). TIMER2.0 for Analysis of Tumor-Infiltrating Immune Cells. Nucleic Acids Res. 48 (W1), W509–W514. doi:10.1093/nar/gkaa407
Manfroi, B., McKee, T., Mayol, J. F., Tabruyn, S., Moret, S., Villiers, C., et al. (2017). CXCL-8/IL8 Produced by Diffuse Large B-Cell Lymphomas Recruits Neutrophils Expressing a Proliferation-Inducing Ligand APRIL. Cancer Res. 77 (5), 1097–1107. doi:10.1158/0008-5472.CAN-16-0786
Merdan, S., Subramanian, K., Ayer, T., Van Weyenbergh, J., Chang, A., Koff, J. L., et al. (2021). Gene Expression Profiling-Based Risk Prediction and Profiles of Immune Infiltration in Diffuse Large B-Cell Lymphoma. Blood Cancer J. 11 (1), 2. doi:10.1038/s41408-020-00404-0
Miao, W. G., Tang, C., Ye, Y., Quinn, R. J., and Feng, Y. (2019). Traditional Chinese Medicine Extraction Method by Ethanol Delivers Drug-like Molecules. Chin. J. Nat. Med. 17 (9), 713–720. doi:10.1016/S1875-5364(19)30086-X
Parekh, H. S., Liu, G., and Wei, M. Q. (2009). A New Dawn for the Use of Traditional Chinese Medicine in Cancer Therapy. Mol. Cancer 8, 21. doi:10.1186/1476-4598-8-21
Piñero, J., Bravo, À., Queralt-Rosinach, N., Gutiérrez-Sacristán, A., Deu-Pons, J., Centeno, E., et al. (2017). DisGeNET: a Comprehensive Platform Integrating Information on Human Disease-Associated Genes and Variants. Nucleic Acids Res. 45 (D1), D833–D839. doi:10.1093/nar/gkw943
Piñero, J., Ramírez-Anguita, J. M., Saüch-Pitarch, J., Ronzano, F., Centeno, E., Sanz, F., et al. (2020). The DisGeNET Knowledge Platform for Disease Genomics: 2019 Update. Nucleic Acids Res. 48 (D1), D845–D855. doi:10.1093/nar/gkz1021
Qi, X., Guo, Z., Chen, Q., Lan, W., Chen, Z., Chen, W., et al. (2021). A Data Mining-Based Analysis of Core Herbs on Different Patterns (Zheng) of Non-small Cell Lung Cancer. Evid. Based Complement. Alternat. Med. 2021, 3621677. doi:10.1155/2021/3621677
Rappaport, N., Twik, M., Plaschkes, I., Nudel, R., Iny Stein, T., Levitt, J., et al. (2017). MalaCards: an Amalgamated Human Disease Compendium with Diverse Clinical and Genetic Annotation and Structured Search. Nucleic Acids Res. 45 (D1), D877–D887. doi:10.1093/nar/gkw1012
Ru, J., Li, P., Wang, J., Zhou, W., Li, B., Huang, C., et al. (2014). TCMSP: a Database of Systems Pharmacology for Drug Discovery from Herbal Medicines. J. Cheminform. 6, 13. doi:10.1186/1758-2946-6-13
Scott, D. W., and Gascoyne, R. D. (2014). The Tumour Microenvironment in B Cell Lymphomas. Nat. Rev. Cancer 14, 517–534. doi:10.1038/nrc3774
Sehn, L. H., Herrera, A. F., Flowers, C. R., Kamdar, M. K., McMillan, A., Hertzberg, M., et al. (2020). Polatuzumab Vedotin in Relapsed or Refractory Diffuse Large B-Cell Lymphoma. J. Clin. Oncol. 38 (2), 155–165. doi:10.1200/JCO.19.00172
Shannon, P., Markiel, A., Ozier, O., Baliga, N. S., Wang, J. T., Ramage, D., et al. (2003). Cytoscape: a Software Environment for Integrated Models of Biomolecular Interaction Networks. Genome Res. 13 (11), 2498–2504. doi:10.1101/gr.1239303
Siegel, R. L., Miller, K. D., Fuchs, H. E., and Jemal, A. (2021). Cancer Statistics, 2021. CA Cancer J. Clin. 71 (1), 7–33. doi:10.3322/caac.21654
Soofiyani, S. R., Hosseini, K., Forouhandeh, H., Ghasemnejad, T., Tarhriz, V., Asgharian, P., et al. (2021). Quercetin as a Novel Therapeutic Approach for Lymphoma. Oxid. Med. Cel. Longev. 2021, 3157867. doi:10.1155/2021/3157867
Staiger, A. M., Altenbuchinger, M., Ziepert, M., Kohler, C., Horn, H., Huttner, M., et al. (2020). A Novel Lymphoma-Associated Macrophage Interaction Signature (LAMIS) Provides Robust Risk Prognostication in Diffuse Large B-Cell Lymphoma Clinical Trial Cohorts of the DSHNHL. Leukemia 34 (2), 543–552. doi:10.1038/s41375-019-0573-y
Svoboda, J., Bair, S. M., Landsburg, D. J., Dwivedy Nasta, S., Nagle, S. J., Barta, S. K., et al. (2021). Brentuximab Vedotin in Combination with Rituximab, Cyclophosphamide, Doxorubicin, and Prednisone as Frontline Treatment for Patients with CD30-Positive B-Cell Lymphomas. Haematologica 106 (6), 1705–1713. doi:10.3324/haematol.2019.238675
Szklarczyk, D., Gable, A. L., Nastou, K. C., Lyon, D., Kirsch, R., Pyysalo, S., et al. (2021). The STRING Database in 2021: Customizable Protein-Protein Networks, and Functional Characterization of User-Uploaded Gene/measurement Sets. Nucleic Acids Res. 49 (D1), D605–D612. doi:10.1093/nar/gkaa1074
Tang, Z., Kang, B., Li, C., Chen, T., and Zhang, Z. (2019). GEPIA2: an Enhanced Web Server for Large-Scale Expression Profiling and Interactive Analysis. Nucleic Acids Res. 47 (W1), W556–W560. doi:10.1093/nar/gkz430
Trott, O., and Olson, A. J. (2010). AutoDock Vina: Improving the Speed and Accuracy of Docking with a New Scoring Function, Efficient Optimization, and Multithreading. J. Comput. Chem. 31 (2), 455–461. doi:10.1002/jcc.21334
Wang, C. Z., Hou, L., Wan, J. Y., Yao, H., Yuan, J., Zeng, J., et al. (2020). Ginseng berry Polysaccharides on Inflammation-Associated colon Cancer: Inhibiting T-Cell Differentiation, Promoting Apoptosis, and Enhancing the Effects of 5-fluorouracil. J. Ginseng Res. 44 (2), 282–290. doi:10.1016/j.jgr.2018.12.010
Wang, S., Long, S., Deng, Z., and Wu, W. (2020). Positive Role of Chinese Herbal Medicine in Cancer Immune Regulation. Am. J. Chin. Med. 48 (7), 1577–1592. doi:10.1142/S0192415X20500780
Wang, Y., Gao, W., Shi, X., Ding, J., Liu, W., He, H., et al. (2017). Chemotherapy Drugs Induce Pyroptosis through Caspase-3 Cleavage of a Gasdermin. Nature 547 (7661), 99–103. doi:10.1038/nature22393
Wu, J., Zhang, X. X., Zou, X., Wang, M., Wang, H. X., Wang, Y. H., et al. (2019). The Effect of Jianpi Yangzheng Xiaozheng Decoction and its Components on Gastric Cancer. J. Ethnopharmacol. 235, 56–64. doi:10.1016/j.jep.2019.02.003
Xiang, Y., Guo, Z., Zhu, P., Chen, J., and Huang, Y. (2019). Traditional Chinese Medicine as a Cancer Treatment: Modern Perspectives of Ancient but Advanced Science. Cancer Med. 8 (5), 1958–1975. doi:10.1002/cam4.2108
Xiong, Y., Zhao, Q., Gu, L., Liu, C., and Wang, C. (20182018). Shenqi Fuzheng Injection Reverses Cisplatin Resistance through Mitofusin-2-Mediated Cell Cycle Arrest and Apoptosis in A549/DDP Cells. Evid. Based. Complement. Alternat. Med. 2018, 8258246. doi:10.1155/2018/8258246
Xu, R., Wu, J., Zhang, X., Zou, X., Li, C., Wang, H., et al. (2020). Modified Bu-Zhong-Yi-Qi Decoction Synergies with 5 Fluorouracile to Inhibits Gastric Cancer Progress via PD-1/PD- L1-dependent T Cell Immunization. Pharmacol. Res. 152, 104623. doi:10.1016/j.phrs.2019.104623
Xu, T., Chai, J., Wang, K., Jia, Q., Liu, Y., Wang, Y., et al. (2021). Tumor Immune Microenvironment Components and Checkpoint Molecules in Anaplastic Variant of Diffuse Large B-Cell Lymphoma. Front. Oncol. 11, 638154. doi:10.3389/fonc.2021.638154
Xu, X., Zhang, W., Huang, C., Li, Y., Yu, H., Wang, Y., et al. (2012). A Novel Chemometric Method for the Prediction of Human Oral Bioavailability. Int. J. Mol. Sci. 13 (6), 6964–6982. doi:10.3390/ijms13066964
Xu-Monette, Z. Y., Xiao, M., Au, Q., Padmanabhan, R., Xu, B., Hoe, N., et al. (2019). Immune Profiling and Quantitative Analysis Decipher the Clinical Role of Immune-Checkpoint Expression in the Tumor Immune Microenvironment of DLBCL. Cancer Immunol. Res. 7 (4), 644–657. doi:10.1158/2326-606610.1158/2326-6066.CIR-18-0439
Younes, A., Sehn, L. H., Johnson, P., Zinzani, P. L., Hong, X., Zhu, J., et al. (2019). Randomized Phase III Trial of Ibrutinib and Rituximab Plus Cyclophosphamide, Doxorubicin, Vincristine, and Prednisone in Non-germinal center B-Cell Diffuse Large B-Cell Lymphoma. J. Clin. Oncol. 37 (15), 1285–1295. doi:10.1200/JCO.18.02403
Keywords: qi-invigorating herbs, traditional Chinese medicine, diffuse large B cell lymphoma, network pharmacology, tumor microenvironment
Citation: Huang Q, Lin J, Huang S and Shen J (2021) Impact of Qi-Invigorating Traditional Chinese Medicines on Diffuse Large B Cell Lymphoma Based on Network Pharmacology and Experimental Validation. Front. Pharmacol. 12:787816. doi: 10.3389/fphar.2021.787816
Received: 01 October 2021; Accepted: 15 November 2021;
Published: 09 December 2021.
Edited by:
Gautam Sethi, National University of Singapore, SingaporeReviewed by:
Chenyue Liu, Beijing University of Chinese Medicine, ChinaAndrew Hung, RMIT University, Australia
Copyright © 2021 Huang, Lin, Huang and Shen. This is an open-access article distributed under the terms of the Creative Commons Attribution License (CC BY). The use, distribution or reproduction in other forums is permitted, provided the original author(s) and the copyright owner(s) are credited and that the original publication in this journal is cited, in accordance with accepted academic practice. No use, distribution or reproduction is permitted which does not comply with these terms.
*Correspondence: Jianzhen Shen, c2p6aGVuQGFsaXl1bi5jb20=