- 1Neuro-Otolaryngology Unit, EuroEspes Biomedical Research Center, Institute of Medical Science and Genomic Medicine, Bergondo, Corunna, Spain
- 2Department of Neuroscience, International Center of Neuroscience and Genomic Medicine, EuroEspes Biomedical Research Center, Bergondo, Corunna, Spain
- 3Genomic Medicine, EuroEspes Biomedical Research Center, Institute of Medical Science and Genomic Medicine, Bergondo, Corunna, Spain
The growing interest in the development of drugs that target the endocannabinoid system has extended to conditions that affect the audiovestibular pathway. The expression of cannabinoid (CB) receptors in that pathway has been widely demonstrated, indicating a therapeutic potential for drug development at this level. These medications may be beneficial for conditions such as noise-induced hearing loss, ototoxicity, or various forms of vertigo of central or peripheral origin. The therapeutic targets of interest include natural or synthetic compounds that act as CB1/CB2 receptor agonists/antagonists, and inhibitors of the endocannabinoid-degrading enzymes FAAH and MAGL. Furthermore, genetic variations implicated in the response to treatment and the development of related disorders such as epilepsy or migraine have been identified. Direct methods of administering these medications should be examined beyond the systemic strategy.
1 Introduction
Auditory and vestibular disorders comprise a heterogeneous group of conditions that have different pathophysiological mechanisms. Depending on the disease, they may affect different structures of the inner ear, cochleovestibular nerve, central signaling or processing nuclei. At the same time, these disorders can either increase or decrease sensory and neural excitability. For example, increased sensory and neural excitability occurs during an acute vestibular crisis (e.g., vestibular neuritis, migraine, and labyrinthitis) or during tinnitus. By contrast, in other disorders such as chronic vestibular hypofunction or age-related hearing loss, there is a deterioration of sensory and neural activity (Peelle and Wingfield, 2016). The neurochemistry of these audiovestibular disorders includes diverse neurotransmitters with excitatory (glutamate, acetylcholine), inhibitory (GABA, glycine), modulatory (histamine, noradrenaline) and other actions (enkephalin, motilin, somatostatin, dopamine and serotonin) (Guerra and Cacabelos, 2019). As a result, and depending on the case, therapeutic approaches differ, with depressive drugs administered in cases of increased neuronal excitability, and stimulants prescribed in cases of decreased neuronal activity.
Regardless of their etiology, acute vestibular disorders are treated with vestibular sedatives. Most of the medications used to treat audiovestibular syndromes are also used to treat central nervous system (CNS) disorders. Anticholinergics, antihistamines, benzodiazepines, calcium-channel blockers and dopamine receptor antagonists minimize nystagmus and vegetative symptoms that include tachycardia, sweating, nausea, and vomiting. To avoid delaying vestibular compensation, prescribing those drugs that have sedative effects should be used in the short term (Hain and Uddin, 2003). Drugs that decrease oxidative stress and inflammation and increase cochlear vascularization can be used to slow hearing loss. Antioxidants, neurotrophic factors, calcium-channel blockers, vasodilators, antiglutamatergic, steroid, and other anti-inflammatory and antiapoptotic agents are also commonly used to treat auditory disorders. However, the majority of these drugs are only protective and there is weak evidence of their efficacy (Sha and Schacht, 2016).
Knowledge of the patient’s pharmacogenetic profile is essential for reducing the risk of adverse effects and for optimizing treatment response; this prescription of pharmacogenetic profile-based strategies improves the therapeutic response. Single nucleotide polymorphisms (SNPs) in genes encoding drug-metabolizing enzymes, drug transporters and drug targets are primarily responsible for the variations in individual drug response. One of the potential consequences of using pharmacogenomics in clinical trials and molecular therapeutics is that a given disease may be treated based on genomic and biological markers, selecting drugs optimized for individual patients or for groups of patients with similar genomic profiles. This aspect has been little explored in the scientific literature (Guerra and Cacabelos, 2019).
The endocannabinoid (EC) system is distributed widely in the brain and is also expressed in the audiovestibular pathway (Gong et al., 2006; Chi and Kandler, 2012; Trattner, Berner, Grothe and Kunz, 2013). There has been increased interest in the development of drugs that act either as an agonist or antagonist on the EC system. This review summarizes current understanding on the role of the EC system for the future use and development of natural- or synthetically-derived compounds that act on the audiovestibular pathway. To pursue those objectives, it is necessary to fully understand the properties of the EC system and the pharmacogenetic profiles of drugs that act on the EC system.
2 The endocannabinoid system
CB1 (encoded by CNR1 gene, locus 6q15) and CB2 (encoded by CNR2 gene, locus 1p36.11) are the 2 G protein-coupled cannabinoid (CB) receptors. Activation of the Gi/Goα subunits reduces intracellular cAMP and increases MAPK levels. CB1 can occasionally activate the G-protein Gs thereby enhancing cAMP levels and the CB2 Gβγ subunits thus activating the MAPK-ERK signaling pathway. Most of the CB receptors in the CNS are CB1 receptors whose function is primarily neuromodulatory through inhibiting GABAergic and glutamatergic neurotransmission. However, CB2 receptors are functionally anti-inflammatory. Other clinically cannabinoid orphan receptors of interest in this review are GPR18 (13q32.3), GPR55 (2q37.1 locus) and GPR119 (Xq26.1 locus), that also encode G protein-coupled receptors (Lu and Mackie, 2016; Zou and Kumar, 2018; Kilaru and Chapman, 2020). Regarding endogenous cannabinoids (CBs), six ligands that act as neurotransmitters have been identified: arachidonoylethanolamine (anandamide/AEA), 2-arachidonoylglycerol (2-AG), 2-arachidonoylglyceryl ether (noladin ether) N-arachidonoyl-dopamine (NADA), Virodhamine, and lysophosphatidylinositol (LPI) (Gallelli et al., 2018). Some of these molecules have anti-inflammatory, sedative, or analgesic properties that are of interest in several audiovestibular disorders, with AEA and 2-AG being the most clinically relevant (Zogopoulos, Vasileiou, Patsouris and Theocharis, 2013). Fatty acid amide hydrolase (FAAH, encoded by FAAH gene, locus 1p33) and monoacylglycerol lipase (MAGL, encoded by MGLL gene, locus 3q21.3) are the enzymes responsible for the degradation of the endocannabinoids (Lu and Mackie, 2016; Zou and Kumar, 2018; Kilaru and Chapman, 2020).
3 Cannabinoids: Targets, effects and pharmacogenomics
More than one hundred CBs derived from the Cannabis sativa plant have been identified. Of these, tetrahydrocannabinol (THC), cannabidiol (CBD) and cannabinol (CBN) are the most studied and for which the most clinical data are available (Chandra et al., 2020).
In addition to the commercialization of cannabinoid-containing medications and supplements, several clinical studies are currently investigating the effects of 1) synthetic cannabinoid-derived drugs (CB1/CB2 receptor agonists/antagonists), and 2) non-cannabinoid-derived compounds (MAGL/FAAH inhibitors) that belong to a part of a different class of biologically active molecules, that regulate the EC system. These compounds have been used in clinical trials and observational studies to manage treatment-resistant epilepsy (Devinsky et al., 2016), ALS (amyotrophic lateral sclerosis) (Weber et al., 2010), MS (multiple sclerosis) (Collin, Davies, Mutiboko and Ratcliffe, 2007; Zajicek et al., 2012), Alzheimer’s disease (Herrmann et al., 2019), schizophrenia (Leweke et al., 2012; McGuire et al., 2018), acute and chronic pain (van de Donk et al., 2018; Weizman et al., 2018), and several other conditions (Kogan and Mechoulam, 2007; Perin et al., 2020; Khalsa et al., 2022). Since many of the above-mentioned and other nervous system disorders also affect the auditory and vestibular pathway, and are associated with dizziness, nystagmus and auditory symptoms, these molecules may help address several types of audiovestibular disorders. Moreover, symptoms that show these neurological diseases appear in peripheral audiovestibular pathology, such as nystagmus, tinnitus or hearing loss (Baguley et al., 2013; Zwergal and Dieterich, 2020).
THC and its synthetic derivative dronabinol are CB1/CB2 receptor agonists. They mimick the effects of ECs in the CNS and modulate the excitatory effect of other neurotransmitters including glutamate. These agents may adversely enhance the tachycardic response of anticholinergics and sympathomimetics and can cause tinnitus, ataxia, and seizures as side effects. Dronabinol’s half-life is 25–36 h, while its metabolites can last up to 59 h. Nabilone, another synthetic cannabinoid that mimics THC, has a more variable half-life, ranging from 2 to 35 h (Cacabelos, 2012). The analogous use of THC and its synthetic derived drugs with opioid derived drugs, antidepressants and anticonvulsants requires a dose reduction due to greater analgesic efficacy and/or higher drug toxicity caused by down-regulation of transporter efflux or enzymatic inhibition (Vázquez et al., 2020). The pharmacogenetic profile of cannabinoid derivatives is still under development, especially with regard to new synthetic derivatives. Dronabinol’s mechanistic genes are CNR1 and CNR2, and it acts as an agonist. Its metabolic genes are CYP3A4, CYP2C9, CYP2C19, CYP1A2, CYP2B6, CYP2D6, CYP1A1, CYP2J2, CYP3A5, CYP3A7, CYP1B1, CYP2A6, CES1 and PTGS1, and the transporter genes include ABCG2, ABCB1 and ABCC1. Nabilone displays the same mechanistic genes as Dronabinol, but it binds partially to its receptors; CYP2C9, CYP3A4, CYP2C8, CYP2E1 and CYP2J2 are its metabolic genes.
Cannabidiol, however, is a non-competitive CB1/CB2 receptor antagonist. It reduces neuronal hyperexcitability by modulating Ca2+ entry via GPR55 and TRPV1 receptors, and by inhibiting ENT-1-receptor-mediated adenosine reuptake (Ibeas Bih et al., 2015). It has a half-life of 18–32 h and causes somnolence, sedation, diarrhea, and changes in appetite as its side effects. It may reduce the adverse effects caused by THC and its derivatives. Drug-drug interactions include regular medications such as anticonvulsants, statins or common analgesics (Vázquez et al., 2020; Davis et al., 2021). The mechanistic genes of cannabidiol are much broader, with diverse targets, as seen in Table 1. CYP3A4, CYP2C19, CYP1A2, CYP2B6, CYP2C9, UGT1A7, UGT1A9, UGT2B7, UGT2B17, CYP1A1, CYP1B1, CYP2C8, CYP2D6, CYP2E1, CYP3A5, CYP2A6, CYP3A7, CYP2J2, CES1, AANAT, FAAH, ALOX5, ALOX15, and CRYZ are its metabolic genes. Transporter genes associated with cannabidiol include ABCC1, ABCG2, SLC29A1, and ABCB1 (Cacabelos, 2012; Wishart et al., 2017).
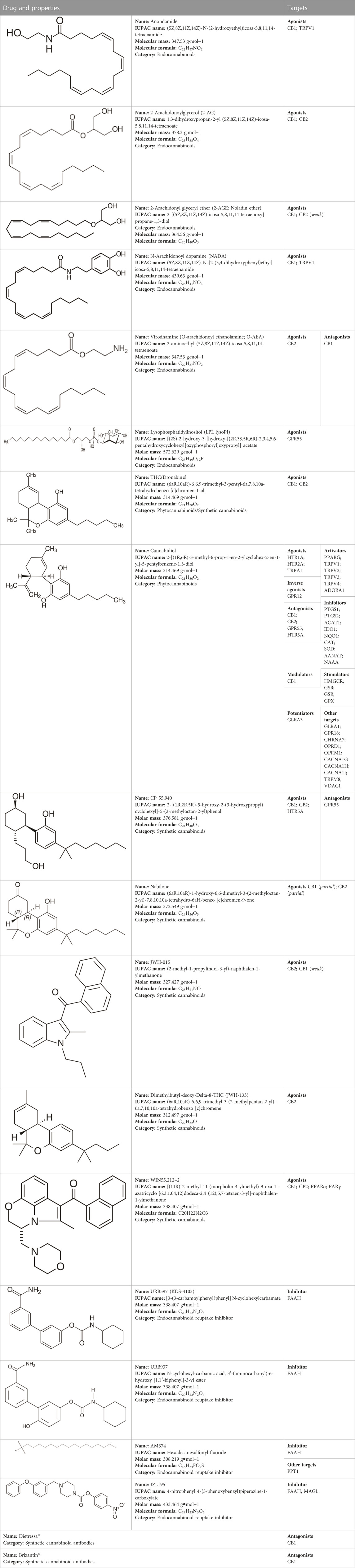
TABLE 1. Profile of the different cannabinoids and drugs that act on the cannabinoid system that may be effective in the treatment of audiovestibular disorders.
CNR1 and CNR2 variants show mixed results in terms of cannabis dependency. CNR1, -64 + 1046T>G and c.-63-9c.1359G>A variants show an association with drug dependency, while 597T>C -64 + 1046T>G c.*3475A>G are also linked to drug dependency in single studies, although with no replication of results. The CNR2 188A>G and 946C>T variants alter its CB2 receptor function in human embryonic kidney-293 cells. Because the COMT rs4680 472A>G Val158Met variant is linked to memory impairment, alterations in executive function, and psychosis, the use of cannabis derivatives should be approached with caution with corresponding adjustments in prescribed doses. The FAAH 385C>APro129Thr variant may be involved in excessive consumption of cannabis, although other authors did not find any association between FAAH variants. The ABCB1 rs10456423455C>T Ile1145Ile variant is associated with cannabis dependency (Hryhorowicz et al., 2018).
CB1 antagonists such as rimonabant, surinabant and other diarylpyrazole derivatives have been experimentally and clinically evaluated for treating conditions unrelated to audiovestibular symptoms such as obesity or addiction disorders. However, their use has raised safety concerns due to CNS or cardiac side effects (Christopoulou and Kiortsis, 2011; Klumpers et al., 2013). Therefore, its role in treating the conditions described in this review is not defined.
Unfortunately, little is known regarding the half-life, adverse effects and pharmacogenetic profiles of other drugs of interest described in this review. One of the primary reasons is that those drugs have been used solely for research purposes. These include CB1/CB2 agonists (CP 55,940, JWH-015 and WIN55,212–2) CB1 agonists (JWH-133), CB1 antagonists (Dietressa© and Brizantin©), FAAH inhibitors (URB597, URB937, and AM374) and FAAH/MAGL inhibitors (JZL195). The targets of these compounds are listed in Table 1 (Cacabelos, 2012; Wishart et al., 2017).
4 The cannabinoid system and the vestibular pathway
The association of the EC system in the vestibular pathway has been previously demonstrated by early reports that indicated that the audiovestibular pathway expresses CB1 receptors. The density of these receptors in this pathway was initially reported as modest, but functionally significant (Newsham-West et al., 1998). Furthermore, CB2 receptors are expressed in the vestibular and cochlear nuclei (Gong et al., 2006; Baek et al., 2008), with lower distribution and relevance than CB1 receptors. The EC system interacts with the different vestibular pathways such as the vestibulocerebellar tract (Suárez et al., 2008). Optogenetically-induced plasticity and motor adaptation in the cerebellum are dependent on EC signaling. When vestibular stimuli are paired with relatively small amplitude Purkinje cell calcium responses, this Purkinje cell activation induces decreased vestibulo-ocular reflex gain. By contrast, pairing with large magnitude calcium responses promotes vestibulo-ocular reflex gain. This indicates that EC signaling acts downstream of Purkinje cell calcium elevation (Bonnan et al., 2021). CB1 receptors modulate the vestibular reflex, as indicated by their activation following unilateral vestibular deafferentiation in rats. However, their expression does not change during vestibular compensation (Ashton et al., 2004). In rats, bilateral vestibular deafferentation causes lifelong spatial memory impairments and hippocampal dysfunction (Baek et al., 2010), that is associated with CB1 receptor down-regulation in the CA3 area of the rat hippocampus (Baek et al., 2011).
4.1 Cannabinoids and vestibular disorders
Despite the abundance of data indicating the presence and participation of the EC system in the vestibular pathway, there are currently only a few publications (Schon et al., 1999; Pradeep et al., 2008) and just one clinical trial (Barchukov et al., 2015), which limits their clinical impact.
In animal models, the administration of the potent non-selective CB receptor agonist CP-55,940 affects the vestibular pathway, comparable to the action of certain metabotropic glutamate receptor agonists, generating dizziness. These effects are sustained and involve neurons in the medial vestibular nucleus (Newsham-West et al., 1998). TRPV1 pathways, which are targets of CBD, are associated with motion sickness processes as observed in Trpv1-null mice (Inprasit et al., 2018). Anecdotal case-reports indicate a reduction or suppression of congenital, and pendular nystagmus associated with multiple sclerosis in cannabis users (Schon et al., 1999; Pradeep et al., 2008). However, the use of nabilone has no effect on nystagmus, indicating that the use of THC agonists alone may not be sufficient to suppress it (Schon et al., 1999). The inhibitory action of CB agonists, and other compounds found in Cannabis Sativa, may suppress nystagmus; however, the lack of evidence pointing to the dosages used in the case reports limits these conclusions (Schon et al., 1999; Pradeep et al., 2008). A clinical trial evaluated the role of active-release preparations containing CB receptor antagonists (marketed in Russia under the Dietressa© and Brizantin© brands); these preparations incorporated antibodies against the CB1 receptor. In that study, patients were evaluated over 1 h after the test dose (1 tablet) of the preparation/placebo. In the follow-up period, the preparation/placebo (1 tablet) was given four times at 30-min intervals. The tolerance to accelerations in a model of vertigo with healthy subjects was increased, with no change in nystagmus duration or recovery time (Barchukov et al., 2015). In theory, cannabinoid antagonists may be advantageous during vestibular compensation. However, there are concerns about these products, related to the utilization of ultra-low doses of the active substance and limited information regarding their molecular properties.
Potential uses of CBs for other symptoms associated with vestibular disorders are described below.
4.1.1 Seizures
Vestibular epilepsy is a rare condition that affects areas in the temporal cortex responsible for motor coordination. Its key characteristics are the presence of rapidly-discharging EEG activity and a favorable response to antiepileptic therapy (Hewett and Bartolomei, 2013). On the other hand, several anticonvulsants including diazepam, gabapentin and carbamazepine are used to treat several vestibular disorder subtypes, even those unrelated to seizures (Casani et al., 2021). CBD is a proposed drug indicated for treating epilepsy. Its brand-labelled product (Epidyolex©) is currently used to treat severe resistant epilepsies such as severe myoclonic epilepsy of infancy (Dravet syndrome; SCN1A gene, locus 2q24.3) (Scheffer et al., 2021). The anti-epileptic action of CBD can be explained by: 1) generating anti-glutamatergic activity and lowering neuronal excitability via GPR55 receptor antagonism and adenosine tone control; 2) activating 5HT1A receptors; and 3) modifying intracellular calcium levels. Other CB1 receptor agonists (WIN55,212–2) also have an anticonvulsant effect, similar to the anti-epileptic action of CBD (Colangeli et al., 2017). Inhibiting EC degradation has also been considered as a potential therapy for epilepsy. FAAH inhibitors (URB597, AM374) suppress hippocampal discharges and improve short- and long-term cognitive performance in rats by increasing blood-brain levels of anandamide (Karanian et al., 2007; Colangeli et al., 2017). Long-term usage of compounds (e.g., palmitoylethanolamide) that target the atypical CB receptors GPR55 and GPR11 has neuroprotective and neuromodulatory advantages in preventing epileptic convulsions in mice when combined with FAAH inhibitors (Post et al., 2018). Several antiepileptic drugs, however, are used to treat vestibular problems such as vestibular paroxysmia or central nystagmus (Strupp et al., 2011). Non-etheless, administering CBD or other CBs in these cases remains to be evaluated (Morano et al., 2020). Variants in the genes that encode aldehyde oxygenase (AOX1 rs6729738 CC) and diamine oxidase (ABP1 rs12539) increase the likelihood of an antiepileptic response. Since CBD produces an antioxidant effect, this could explain that finding. By contrast, SLC15A1 rs1339067 TT carriers exhibit a decreased antiepileptic response, indicating that this transporter limits CBD activity. EC receptor GPR18 expression in white matter is reduced by rs1339067, and hippocampal HTR3E serotonergic receptor expression is decreased by rs3749442, thus modulating the drug response in treatment-resistant epilepsy (Davis et al., 2021).
4.1.2 Headache
Many patients with migraine describe neuro-otological symptoms such as phonophobia and vertigo. Vestibular migraine is a distinct entity whose diagnostic criteria include at least five episodes of migraine with/without aura and vestibular symptoms of moderate or severe intensity, lasting between 5 min and 72 h. In addition, at least half of the episodes must be associated with at least one of the following three migrainous features: 1) headache with at least two of the following four characteristics: 1) unilateral location; 2) pulsating quality; 3) moderate or severe intensity; 4) aggravation by routine physical activity; 2) photophobia and phonophobia; 3) visual aura; and 3) not better accounted for by another vestibular disorder (Lempert et al., 2012). Cannabis and its derivatives are efficacious for controlling pain and are viable agents for treating migraine and other headaches. The EC system acts via CB1 and CB2 receptors to alleviate pain, whereas pain stimulation occurs through TRPV1 receptors. MAGL and FAAH regulates EC levels; this analgesia affects the meninges and brainstem (Leimuranta et al., 2018). Central EC deficiency (as observed in the cerebrospinal fluid), specifically AEA and 2-AG, is a potential cause of migraine (Russo, 2016). Cannabis consumption reduces the intensity of migraine attacks but its long-term use produces tolerance (Cuttler, Spradlin, Cleveland and Craft, 2020). Moreover, a better prophylactic control was shown after treatment with medical cannabis, although with sparse evidence (Poudel et al., 2021). Both THC and CBD are responsible for this anti-migraine effect, but with the ideal ratio of these compounds yet to be determined (Mechtler et al., 2021). In preclinical studies, FAAH inhibitors used for the preventive control of migraine increased anandamide and palmitoylethanolamide levels, but has no effect in response to an acute migraine attack (Greco et al., 2020). The use of the FAAH inhibitor URB937 reduces hyperalgesia and c-Fos expression in the trigeminal caudal nucleus (TNC) and locus coeruleus in rodents. This response was accompanied by decreased neuronal gene expression of nitric oxide synthase, calcitonin gene-related peptide and cytokines (Greco et al., 2020; Greco et al., 2021a); using dual inhibitors of FAAH and MAGL produce similar effects (Greco et al., 2021b). Several rare, non-sense mutations in CNR1 increase the predisposition to developing migraine (Smith et al., 2017). CNR1 carriers with the HT6 haplotypic variant, for example, are most susceptible with an increased likelihood of headache together with nausea and photophobia (Juhasz et al., 2009). CNR2 and FAAH variants do not appear to be involved in migraine (Smith et al., 2017).
4.1.3 Vomiting
Vomiting is one of the symptoms associated with a vestibular crisis; despite the limited data, THC and other agonists appear to be effective in decreasing chemotherapy-induced vomiting (Whiting et al., 2015). This central antiemetic action occurs via the activation of CB1 receptors, and possibly TRPV1 in the dorsal vagal complex (Darmani, 2010). But contrary to popular belief, one side effect of frequent cannabis use is vomiting (Castaneto et al., 2014). Moreover, the suggested best treatment for this hyperemesis, which is associated with THC and its plasma high levels (Chu and Cascella, 2022), is discontinuing THC consumption (Sorensen et al., 2016). THC may cause desensitizing, pharmacogenetic, or tolerance effects following its binding to CB1 receptors, as well as antagonizing the peripheral emetic effect versus the central antiemetic effect. However, its pathophysiology remains unclear (Sorensen et al., 2016). Although AEA alone does not diminish reduce vomiting in animal models, URB597 attenuates and even suppresses vomiting caused by chemotherapy and nicotine (Parker et al., 2009).
5 The cannabinoid system and the auditory pathway
EC signaling modulates neurotransmission within auditory circuits and contributes to their development (Chi and Kandler, 2012; Trattner, et al., 2013). CB1-receptor knockout mice have lower auditory thresholds at hearing frequencies greater than 8 kHz in their audiograms, than wild type mice. In terms of central auditory processing, these knockout mice outperform wild-type mice in identifying gaps in low-pass noise bursts (Toal et al., 2016). CB2 receptors are found in several areas of the inner ear, including the Organ of Corti, stria vascularis, spiral ligament, and spiral ganglion cells (Ghosh et al., 2018). CB2 receptor activation is involved in protecting against drug-induced hearing loss (Martín-Saldaña et al., 2016; Dhukhwa et al., 2021).
5.1 Cannabinoids and hearing disorders
There are few publications on auditory pathology than on the effect of cannabis on hearing disorders. Considering the use of CB agonists, in animal models, JWH-015, a CB2 agonist that is also a weak CB1 agonist, is otoprotective against cisplatin exposure. CB2 antagonists reverse this effect (Ghosh et al., 2018). Furthermore, another pharmacological agonist (JWH-133) activates CB2 receptors, produces an anti-inflammatory effect in the inner ear, and increases microcirculation following exposure to bacterial endotoxins (Weiss et al., 2021). Although these results appear promising, they were performed in animal models, and therefore the translational implications for humans are quite limited given the absence of studies in this regard.
As mentioned previously, CBD modulates the TRPV1 receptor. TRPV1 and other TRPs are up-regulated when exposed to an ototoxic agent (Kitahara et al., 2005) and can exert dual effects on hearing function, depending on which drug acts on TRP channels. For example, capsaicin would be protective while an aminoglycoside would exacerbate the inflammatory response (Ramkumar et al., 2022). TRPV1 activity begins after the activation of NOX3 NADPH oxidase and STAT1 and STAT3 transcription factors that mediate oxidative stress, which cause inflammation and cochlear apoptosis (Ramkumar et al., 2022). CBD may theoretically be an otoprotective drug, but no reports to date have demonstrated its efficacy in this regard.
Concerning tinnitus, several reviews have demonstrated a detrimental effect of cannabis and its derivatives on the development of tinnitus. Based on data obtained from animal models, null or even negative effects of CB1 receptor agonists on tinnitus have been reported in response to noise exposure and the use of ototoxic drugs (Zheng et al., 2010; Zheng et al., 2015; Berger et al., 2017). In humans, these findings have also been observed according to the results of surveys and clinical trials; paradoxically, a patient with intracranial hypertension showed tinnitus relief following dronabinol consumption (Narwani et al., 2020). Auditory excitability extends to the auditory cortex and may account for the auditory hallucinations reported in some subjects (Nestoros et al., 2017). It is unknown whether the use of cannabinoid antagonists may be effective for controlling tinnitus.
6 Conclusion
The present review shows that the EC system regulates audiovestibular function. The few but significant references to the use of CB agonists/antagonists, and MAGL/FAAH inhibitors that act on the EC system indicate that they may be effective for treating audiovestibular disorders. The neuromodulatory effects of those compounds would act on symptoms as diverse as dizziness, nystagmus, nausea, vomiting, tinnitus or headache across different vestibular syndromes of central and peripheral origin (linked or not to neurological pathology), in some cases with inhibitory effects, particularly CB agonists, with sedative action and excitatory effects in others, and with compensatory or protective functions, as is typical in the case of CB antagonists. A critical aspect concerning the use of drugs that act on the EC system is the absence and/or variability of doses used in all studies, even in those studies performed in animal models. This severely limits the application of EC system-targeting drugs and standardization in humans. A further issue that requires discussion is the lack of pharmacogenetic profiles for most cannabinoid derivatives, which affects all conditions that may benefit from their use. However, an understanding of the pharmacogenetics of the cannabinoid derivatives dronabinol, nabilone and cannabidiol is undoubtedly invaluable. Genes related to the metabolism and/or to the mechanisms of action of these drugs contribute to problems with therapeutic efficacy and safety of those agents. A pharmacogenomic strategy to optimize the use of drugs that interact with the EC system is required for successful, personalized therapy. Finally, whereas systemic drug administration allows for wider drug distribution to exert central and peripheral effects, the effects of local delivery of these agents to the inner ear via more invasive direct approaches are unknown. This application would be particularly appealing for those drugs with peripheral action or those with minimal CNS effects.
Author contributions
Made substantial contributions to conception and design of the review and interpretation, JG, read, adjusted and approved the final manuscript, VN and RC.
Acknowledgments
JG wants to acknowledge Adria Pijuan Monroy and Iria Rodriguez Lopez for being the inspiration to prepare this article.
Conflict of interest
The authors declare that the research was conducted in the absence of any commercial or financial relationships that could be construed as a potential conflict of interest.
Publisher’s note
All claims expressed in this article are solely those of the authors and do not necessarily represent those of their affiliated organizations, or those of the publisher, the editors and the reviewers. Any product that may be evaluated in this article, or claim that may be made by its manufacturer, is not guaranteed or endorsed by the publisher.
References
Ashton, J., Zheng, Y., Liu, P., Darlington, C., and Smith, P. (2004). Immunohistochemical characterisation and localisation of cannabinoid CB1 receptor protein in the rat vestibular nucleus complex and the effects of unilateral vestibular deafferentation. Brain Res. 1021 (2), 264–271. doi:10.1016/j.brainres.2004.06.065
Baek, J., Zheng, Y., Darlington, C., and Smith, P. (2011). Cannabinoid CB1 receptor expression and affinity in the rat hippocampus following bilateral vestibular deafferentation. Neurosci. Lett. 487 (3), 330–334. doi:10.1016/j.neulet.2010.10.050
Baek, J., Zheng, Y., Darlington, C., and Smith, P. (2008). Cannabinoid CB2 receptor expression in the rat brainstem cochlear and vestibular nuclei. Acta Otolaryngol. 128 (9), 961–967. doi:10.1080/00016480701796944
Baek, J., Zheng, Y., Darlington, C., and Smith, P. (2010). Evidence that spatial memory deficits following bilateral vestibular deafferentation in rats are probably permanent. Neurobiol. Learn. Mem. 94 (3), 402–413. doi:10.1016/j.nlm.2010.08.007
Baguley, D., McFerran, D., and Hall, D. (2013). Tinnitus. Lancet 382 (9904), 1600–1607. doi:10.1016/S0140-6736(13)60142-7
Barchukov, V., Zhavbert, E., Dugina, Y., and Epstein, O. (2015). The use of release-active antibody-based preparations for vertigo prevention in adults. Bull. Exp. Biol. Med. 160 (1), 61–63. doi:10.1007/s10517-015-3098-z
Berger, J., Coomber, B., Hill, S., Alexander, S., Owen, W., Palmer, A., et al. (2017). Effects of the cannabinoid CB 1 agonist ACEA on salicylate ototoxicity, hyperacusis and tinnitus in Guinea pigs. Hear. Res. 356, 51–62. doi:10.1016/j.heares.2017.10.012
Bonnan, A., Rowan, M., Baker, C., Bolton, M., and Christie, J. (2021). Autonomous Purkinje cell activation instructs bidirectional motor learning through evoked dendritic calcium signaling. Nat. Commun. 12 (1), 2153. doi:10.1038/s41467-021-22405-8
R. Cacabelos (Editor) (2012). World guide for drug use and pharmacogenomics (Spain: EuroEspes Publishing; A Coruña).
Casani, A., Gufoni, M., and Capobianco, S. (2021). Current insights into treating vertigo in older adults. Drugs Aging 38 (8), 655–670. doi:10.1007/s40266-021-00877-z
Castaneto, M., Gorelick, D., Desrosiers, N., Hartman, R., Pirard, S., and Huestis, M. (2014). Synthetic cannabinoids: Epidemiology, pharmacodynamics, and clinical implications. Drug Alcohol Depend. 144, 12–41. doi:10.1016/j.drugalcdep.2014.08.005
Chandra, S., Lata, H., and ElSohly, M. (2020). Propagation of cannabis for clinical research: An approach towards a modern herbal medicinal products development. Front. Plant Sci. 11, 958. doi:10.3389/fpls.2020.00958
Chi, D., and Kandler, K. (2012). Cannabinoid receptor expression at the MNTB-LSO synapse in developing rats. Neurosci. Lett. 509 (2), 96–100. doi:10.1016/j.neulet.2011.12.047
Christopoulou, F., and Kiortsis, D. (2011). An overview of the metabolic effects of rimonabant in randomized controlled trials: Potential for other cannabinoid 1 receptor blockers in obesity. J. Clin. Pharm. Ther. 36 (1), 10–18. doi:10.1111/j.1365-2710.2010.01164.x
Colangeli, R., Pierucci, M., Benigno, A., Campiani, G., Butini, S., and Di Giovanni, G. (2017). The FAAH inhibitor URB597 suppresses hippocampal maximal dentate afterdischarges and restores seizure-induced impairment of short and long-term synaptic plasticity. Sci. Rep. 7 (1), 11152. doi:10.1038/s41598-017-11606-1
Collin, C., Davies, P., Mutiboko, I., and Ratcliffe, S. (2007). Randomized controlled trial of cannabis-based medicine in spasticity caused by multiple sclerosis. Eur. J. Neurol. 14 (3), 290–296. doi:10.1111/j.1468-1331.2006.01639.x
Cuttler, C., Spradlin, A., Cleveland, M., and Craft, R. (2020). Short- and long-term effects of cannabis on headache and migraine. J. Pain 21 (5-6), 722–730. doi:10.1016/j.jpain.2019.11.001
Darmani, N. (2010). Mechanisms of broad-spectrum antiemetic efficacy of cannabinoids against chemotherapy-induced acute and delayed vomiting. Pharmaceuticals 3 (9), 2930–2955. doi:10.3390/ph3092930
Davis, B., Beasley, T., Amaral, M., Szaflarski, J., Gaston, T., Perry Grayson, L., et al. (2021). Pharmacogenetic predictors of cannabidiol response and tolerability in treatment-resistant epilepsy. Clin. Pharmacol. Ther. 110 (5), 1368–1380. doi:10.1002/cpt.2408
Devinsky, O., Marsh, E., Friedman, D., Thiele, E., Laux, L., Sullivan, J., et al. (2016). Cannabidiol in patients with treatment-resistant epilepsy: An open-label interventional trial. Lancet. Neurol. 15 (3), 270–278. doi:10.1016/S1474-4422(15)00379-8
Dhukhwa, A., Al Aameri, R., Sheth, S., Mukherjea, D., Rybak, L., and Ramkumar, V. (2021). Regulator of G protein signaling 17 represents a novel target for treating cisplatin induced hearing loss. Sci. Rep. 11 (1), 8116. doi:10.1038/s41598-021-87387-5
Gallelli, C., Calcagnini, S., Romano, A., Koczwara, J., de Ceglia, M., Dante, D., et al. (2018). Modulation of the oxidative stress and lipid peroxidation by endocannabinoids and their lipid analogues. Antioxidants 7 (7), 93. doi:10.3390/antiox7070093
Ghosh, S., Sheth, S., Sheehan, K., Mukherjea, D., Dhukhwa, A., Borse, V., et al. (2018). The endocannabinoid/cannabinoid receptor 2 system protects against cisplatin-induced hearing loss. Front. Cell. Neurosci. 12, 271. doi:10.3389/fncel.2018.00271
Gong, J., Onaivi, E., Ishiguro, H., Liu, Q., Tagliaferro, P., Brusco, A., et al. (2006). Cannabinoid CB2 receptors: Immunohistochemical localization in rat brain. Brain Res. 1071 (1), 10–23. doi:10.1016/j.brainres.2005.11.035
Greco, R., Demartini, C., Francavilla, M., Zanaboni, A., and Tassorelli, C. (2021b). Dual inhibition of FAAH and MAGL counteracts migraine-like pain and behavior in an animal model of migraine. Cells 10 (10), 2543. doi:10.3390/cells10102543
Greco, R., Demartini, C., Zanaboni, A., Casini, I., De Icco, R., Reggiani, A., et al. (2021a). Characterization of the peripheral FAAH inhibitor, URB937, in animal models of acute and chronic migraine. Neurobiol. Dis. 147, 105157. doi:10.1016/j.nbd.2020.105157
Greco, R., Demartini, C., Zanaboni, A., Tumelero, E., Reggiani, A., Misto, A., et al. (2020). FAAH inhibition as a preventive treatment for migraine: A pre-clinical study. Neurobiol. Dis. 134, 104624. doi:10.1016/j.nbd.2019.104624
Guerra, J., and Cacabelos, R. (2019). Pharmacoepigenetics of vertigo and related vestibular syndromes. Pharmacoepigenetics 10, 755–779. doi:10.1016/B978-0-12-813939-4.00028-0
Hain, T., and Uddin, M. (2003). Pharmacological treatment of vertigo. CNS Drugs 17 (2), 85–100. doi:10.2165/00023210-200317020-00002
Herrmann, N., Ruthirakuhan, M., Gallagher, D., Verhoeff, N., Kiss, A., Black, S., et al. (2019). Randomized placebo-controlled trial of nabilone for agitation in Alzheimer's disease. Am. J. Geriatr. Psychiatry 27 (11), 1161–1173. doi:10.1016/j.jagp.2019.05.002
Hewett, R., and Bartolomei, F. (2013). Epilepsy and the cortical vestibular system: Tales of dizziness and recent concepts. Front. Integr. Neurosci. 7, 73. doi:10.3389/fnint.2013.00073
Hryhorowicz, S., Walczak, M., Zakerska-Banaszak, O., Slomski, R., and Skrzypczak-Zielinska, M. (2018). Pharmacogenetics of cannabinoids. Eur. J. Drug Metab. Pharmacokinet. 43, 1–12. doi:10.1007/s13318-017-0416-z
Ibeas Bih, C., Chen, T., Nunn, A., Bazelot, M., Dallas, M., and Whalley, B. (2015). Molecular targets of cannabidiol in neurological disorders. Neurotherapeutics 12 (4), 699–730. doi:10.1007/s13311-015-0377-3
Inprasit, C., Lin, Y., Huang, C., Wu, S., and Hsieh, C. (2018). Targeting TRPV1 to relieve motion sickness symptoms in mice by electroacupuncture and gene deletion. Sci. Rep. 8 (1), 10365. doi:10.1038/s41598-018-23793-6
Juhasz, G., Lazary, J., Chase, D., Pegg, E., Downey, D., Toth, Z., et al. (2009). Variations in the cannabinoid receptor 1 gene predispose to migraine. Neurosci. Lett. 461 (2), 116–120. doi:10.1016/j.neulet.2009.06.021
Karanian, D., Karim, S., Wood, J., Williams, J., Lin, S., Makriyannis, A., et al. (2007). Endocannabinoid enhancement protects against kainic acid-induced seizures and associated brain damage. J. Pharmacol. Exp. Ther. 322 (3), 1059–1066. doi:10.1124/jpet.107.120147
Khalsa, J., Bunt, G., Blum, K., Maggirwar, S., Galanter, M., and Potenza, M. (2022). Review: Cannabinoids as medicinals. Curr. Addict. Rep. 7, 1–17. doi:10.1007/s40429-022-00438-3
Kilaru, A., and Chapman, K. (2020). The endocannabinoid system. Essays Biochem. 64 (3), 485–499. doi:10.1042/EBC20190086
Kitahara, T., Li, H., and Balaban, C. (2005). Changes in transient receptor potential cation channel superfamily V (TRPV) mRNA expression in the mouse inner ear ganglia after kanamycin challenge. Hear. Res. 201 (1-2), 132–144. doi:10.1016/j.heares.2004.09.007
Klumpers, L., Roy, C., Ferron, G., Turpault, S., Poitiers, F., Pinquier, J., et al. (2013). Surinabant, a selective cannabinoid receptor type 1 antagonist, inhibits Δ9-tetrahydrocannabinol-induced central nervous system and heart rate effects in humans. Br. J. Clin. Pharmacol. 76 (1), 65–77. doi:10.1111/bcp.12071
Kogan, N., and Mechoulam, R. (2007). Cannabinoids in health and disease. Dialogues Clin. Neurosci. 9 (4), 413–430. doi:10.31887/DCNS.2007.9.4/nkogan
Leimuranta, P., Khiroug, L., and Giniatullin, R. (2018). Emerging role of (Endo)Cannabinoids in migraine. Front. Pharmacol. 9, 420. doi:10.3389/fphar.2018.00420
Lempert, T., Olesen, J., Furman, J., Waterston, J., Seemungal, B., Carey, J., et al. (2012). Vestibular migraine: Diagnostic criteria. J. Vestib. Res. 22 (4), 167–172. doi:10.3233/VES-2012-0453
Leweke, F., Piomelli, D., Pahlisch, F., Muhl, D., Gerth, C., Hoyer, C., et al. (2012). Cannabidiol enhances anandamide signaling and alleviates psychotic symptoms of schizophrenia. Transl. Psychiatry 2 (3), e94. doi:10.1038/tp.2012.15
Lu, H., and Mackie, K. (2016). An introduction to the endogenous cannabinoid system. Biol. Psychiatry 79 (7), 516–525. doi:10.1016/j.biopsych.2015.07.028
Martín-Saldaña, S., Trinidad, A., Ramil, E., Sánchez-López, A., Coronado, M., Martínez-Martínez, E., et al. (2016). Spontaneous cannabinoid receptor 2 (CB2) expression in the cochlea of adult albino rat and its up-regulation after cisplatin treatment. PLOS ONE 11 (8), e0161954. doi:10.1371/journal.pone.0161954
McGuire, P., Robson, P., Cubala, W., Vasile, D., Morrison, P., Barron, R., et al. (2018). Cannabidiol (CBD) as an adjunctive therapy in schizophrenia: A multicenter randomized controlled trial. Am. J. Psychiatry 175 (3), 225–231. doi:10.1176/appi.ajp.2017.17030325
Mechtler, L., Gengo, F., and Bargnes, V. (2021). Cannabis and migraine: It’s complicated. Curr. Pain Headache Rep. 25 (3), 16. doi:10.1007/s11916-020-00931-2
Morano, A., Fanella, M., Albini, M., Cifelli, P., Palma, E., Giallonardo, A., et al. (2020). Cannabinoids in the treatment of epilepsy: Current status and future prospects. Neuropsychiatr. Dis. Treat. 16, 381–396. doi:10.2147/NDT.S203782
Narwani, V., Bourdillon, A., Nalamada, K., Manes, R., and Hildrew, D. (2020). Does cannabis alleviate tinnitus? A review of the current literature. Laryngoscope Investig. Otolaryngol. 5 (6), 1147–1155. doi:10.1002/lio2.479
Nestoros, J., Vakonaki, E., Tzatzarakis, M., Alegakis, A., Skondras, M., and Tsatsakis, A. (2017). Long lasting effects of chronic heavy cannabis abuse. Am. J. Addict. 26 (4), 335–342. doi:10.1111/ajad.12529
Newsham-West, D., Darlington, L. C., and Smith, P. (1998). Potent effects of a selective cannabinoid receptor agonist on some Guinea pig medial vestibular nucleus neurons. Eur. J. Pharmacol. 348 (1), R1–R2. doi:10.1016/s0014-2999(98)00237-4
Parker, L., Limebeer, C., Rock, E., Litt, D., Kwiatkowska, M., and Piomelli, D. (2009). The FAAH inhibitor URB-597 interferes with cisplatin- and nicotine-induced vomiting in the Suncus murinus (house musk shrew). Physiol. Behav. 97, 121–124. doi:10.1016/j.physbeh.2009.02.014
Peelle, J., and Wingfield, A. (2016). The neural consequences of age-related hearing loss. Trends Neurosci. 39 (7), 486–497. doi:10.1016/j.tins.2016.05.001
Perin, P., Mabou Tagne, A., Enrico, P., Marino, F., Cosentino, M., Pizzala, R., et al. (2020). Cannabinoids, inner ear, hearing, and tinnitus: A neuroimmunological perspective. Front. Neurol. 11, 505995. doi:10.3389/fneur.2020.505995
Post, J., Loch, S., Lerner, R., Remmers, F., Lomazzo, E., Lutz, B., et al. (2018). Antiepileptogenic effect of SubchronicPalmitoylethanolamide treatment in a mouse model of acute epilepsy. Front. Mol. Neurosci. 11, 67. doi:10.3389/fnmol.2018.00067
Poudel, S., Quinonez, J., Choudhari, J., Au, Z., Paesani, S., Thiess, A., et al. (2021). Medical cannabis, headaches, and migraines: A review of the current literature. Cureus 13, e17407. doi:10.7759/cureus.17407
Pradeep, A., Thomas, S., Roberts, E., Proudlock, F., and Gottlob, I. (2008). Reduction of congenital nystagmus in a patient after smoking cannabis. Strabismus 16 (1), 29–32. doi:10.1080/09273970701821063
Ramkumar, V., Sheth, S., Dhukhwa, A., Al Aameri, R., Rybak, L., and Mukherjea, D. (2022). Transient receptor potential channels and auditory functions. Antioxid. Redox Signal. 36 (16-18), 1158–1170. doi:10.1089/ars.2021.0191
Russo, E. (2016). Clinical endocannabinoid deficiency reconsidered: Current research supports the theory in migraine, fibromyalgia, irritable bowel, and other treatment-resistant syndromes. Syndromes. Cannabis Cannabinoid Res. 1 (1), 154–165. doi:10.1089/can.2016.0009
Scheffer, I., Halford, J., Miller, I., Nabbout, R., Sanchez-Carpintero, R., Shiloh-Malawsky, Y., et al. (2021). Add-on cannabidiol in patients with Dravet syndrome: Results of a long-term open-label extension trial. Epilepsia 62 (10), 2505–2517. doi:10.1111/epi.17036
Schon, F., Hart, P., Hodgson, T., Pambakian, A., Ruprah, M., Williamson, E., et al. (1999). Suppression of pendular nystagmus by smoking cannabis in a patient with multiple sclerosis. Neurology 53 (9), 2209–2210. doi:10.1212/wnl.53.9.2209-a
Sha, S., and Schacht, J. (2016). Emerging therapeutic interventions against noise-induced hearing loss. Expert Opin. Investig. Drugs 26 (1), 85–96. doi:10.1080/13543784.2017.1269171
Smith, D., Stanley, C., Foss, T., Boles, R., and McKernan, K. (2017). Rare genetic variants in the endocannabinoid system genes CNR1 and DAGLA are associated with neurological phenotypes in humans. PLOS ONE 12 (11), e0187926. doi:10.1371/journal.pone.0187926
Sorensen, C., DeSanto, K., Borgelt, L., Phillips, K., and Monte, A. (2016). Cannabinoid hyperemesis syndrome: Diagnosis, pathophysiology, and treatment—A systematic review. J. Med. Toxicol. 13 (1), 71–87. doi:10.1007/s13181-016-0595-z
Strupp, M., Thurtell, M., Shaikh, A., Brandt, T., Zee, D., and Leigh, R. (2011). Pharmacotherapy of vestibular and ocular motor disorders, including nystagmus. J. Neurol. 258 (7), 1207–1222. doi:10.1007/s00415-011-5999-8
Suárez, J., Bermúdez-Silva, F., Mackie, K., Ledent, C., Zimmer, A., Cravatt, B., et al. (2008). Immunohistochemical description of the endogenous cannabinoid system in the rat cerebellum and functionally related nuclei. J. Comp. Neurol. 509 (4), 400–421. doi:10.1002/cne.21774
Toal, K., Radziwon, K., Holfoth, D., Xu-Friedman, M., and Dent, M. (2016). Audiograms, gap detection thresholds, and frequency difference limens in cannabinoid receptor 1 knockout mice. Hear. Res. 332, 217–222. doi:10.1016/j.heares.2015.09.013
Trattner, B., Berner, S., Grothe, B., and Kunz, L. (2013). Depolarization-induced suppression of a glycinergic synapse in the superior olivary complex by endocannabinoids. J. Neurochem. 127 (1), 78–90. doi:10.1111/jnc.12369
Van de Donk, T., Niesters, M., Kowal, M., Olofsen, E., Dahan, A., and van Velzen, M. (2018). An experimental randomized study on the analgesic effects of pharmaceutical-grade cannabis in chronic pain patients with fibromyalgia. Pain 160 (4), 860–869. doi:10.1097/j.pain.0000000000001464
Vázquez, M., Guevara, N., Maldonado, C., Guido, P., and Schaiquevich, P. (2020). Potential pharmacokinetic drug-drug interactions between cannabinoids and drugs used for chronic pain. Biomed. Res. Int. 2020, 3902740–3902749. doi:10.1155/2020/3902740
Weber, M., Goldman, B., and Truniger, S. (2010). Tetrahydrocannabinol (THC) for cramps in amyotrophic lateral sclerosis: A randomised, double-blind crossover trial. J. Neurol. Neurosurg. Psychiatry 81 (10), 1135–1140. doi:10.1136/jnnp.2009.200642
Weiss, B., Freytag, S., Kloos, B., Haubner, F., Sharaf, K., Spiegel, J., et al. (2021). Cannabinoid receptor 2 agonism is capable of preventing lipopolysaccharide induced decreases of cochlear microcirculation – a potential approach for inner ear pathologies. Otol. Neurotol. 42 (9), e1396–e1401. doi:10.1097/MAO.0000000000003280
Weizman, L., Dayan, L., Brill, S., Nahman-Averbuch, H., Hendler, T., Jacob, G., et al. (2018). Cannabis analgesia in chronic neuropathic pain is associated with altered brain connectivity. Neurology 91 (14), e1285–e1294. doi:10.1212/WNL.0000000000006293
Whiting, P., Wolff, R., Deshpande, S., Di Nisio, M., Duffy, S., Hernandez, A., et al. (2015). Cannabinoids for medical use: A systematic review and meta-analysis. JAMA 313 (24), 2456–2473. doi:10.1001/jama.2015.6358
Wishart, D., Feunang, Y., Guo, A., Lo, E., Marcu, A., Grant, J., et al. (2017). DrugBank 5.0: A major update to the DrugBank database for 2018. Nucleic Acids Res. 46 (D1), D1074–D1082. doi:10.1093/nar/gkx1037
Zajicek, J., Hobart, J., Slade, A., Barnes, D., and Mattison, P. (2012). MUltiple sclerosis and extract of cannabis: Results of the MUSEC trial. J. Neurol. Neurosurg. Psychiatry 83 (11), 1125–1132. doi:10.1136/jnnp-2012-302468
Zheng, Y., Reid, P., and Smith, P. (2015). Cannabinoid CB1 receptor agonists do not decrease, but may increase acoustic trauma-induced tinnitus in rats. Front. Neurol. 6, 60. doi:10.3389/fneur.2015.00060
Zheng, Y., Stiles, L., Hamilton, E., Smith, P., and Darlington, C. (2010). The effects of the synthetic cannabinoid receptor agonists, WIN55, 212-2 and CP55, 940, on salicylate-induced tinnitus in rats. Hear. Res. 268 (1-2), 145–150. doi:10.1016/j.heares.2010.05.015
Zogopoulos, P., Vasileiou, I., Patsouris, E., and Theocharis, S. (2013). The role of endocannabinoids in pain modulation. Fundam. Clin. Pharmacol. 27 (1), 64–80. doi:10.1111/fcp.12008
Zou, S., and Kumar, U. (2018). Cannabinoid receptors and the endocannabinoid system: Signaling and function in the central nervous system. Int. J. Mol. Sci. 19 (3), 833. doi:10.3390/ijms19030833
Keywords: hearing, vertigo, cannabinoids, cannabis, THC-tetrahydrocannabinol, CBD-cannabidiol
Citation: Guerra J, Naidoo V and Cacabelos R (2022) Potential effects of cannabinoids on audiovestibular function: A narrative review. Front. Pharmacol. 13:1010296. doi: 10.3389/fphar.2022.1010296
Received: 02 August 2022; Accepted: 23 November 2022;
Published: 20 December 2022.
Edited by:
Hidayat Hussain, Leibniz Institute of Plant Biochemistry, GermanyReviewed by:
Abir El-Alfy, Medical College of Wisconsin, United StatesLuana Lionetto, Sapienza University of Rome, Italy
Copyright © 2022 Guerra, Naidoo and Cacabelos. This is an open-access article distributed under the terms of the Creative Commons Attribution License (CC BY). The use, distribution or reproduction in other forums is permitted, provided the original author(s) and the copyright owner(s) are credited and that the original publication in this journal is cited, in accordance with accepted academic practice. No use, distribution or reproduction is permitted which does not comply with these terms.
*Correspondence: Joaquin Guerra, neuroorl@euroespes.com