- 1Department of Rehabilitation Medicine, China-Japan Union Hospital of Jilin University, Changchun, China
- 2Department of Dermatology, China-Japan Union Hospital of Jilin University, Changchun, China
We designed and synthesized recombinant phage nanofibers displaying ToAP2D peptide and investigated their antifungal effect on Sporothrix and the corresponding mechanism. Antimicrobial peptide, ToAP2D, was used as the template. The effect of synthesized recombinant phages on the immune function of CD4+ T lymphocytes in mice was tested using an enzyme-linked immunosorbent assay. The therapeutic effect and safety of recombinant phage administration on Sporothrix-infected BALB/c mice were evaluated based on survival analysis, histopathological changes, and renal and liver functions. The successfully prepared recombinant phage displaying ToAP2D peptides significantly inhibited Sporothrix growth. According to the scanning electron microscopy results, the recombinant phage caused shrinkage and rupture of Sporothrix globosa, leading to leakage of the contents. The Hoechst/propidium iodide double staining test indicated that the recombinant phage could induce cell apoptosis of Sporothrix globosa. The apoptotic pathway might be due to the accumulation of reactive oxygen species in large quantities in cells, activating caspase dependence; this reduced inflammation, prolonged the survival time, and enhanced levels of IFN-γ and IL-17 in mice. We believe that recombinant phage inhibits Sporothrix growth by adjusting the immune response of mice, inducing Sporothrix apoptosis and improving animal survival. This study offers a new approach to preparing antimicrobial peptides.
Introduction
Sporothrix can cause local skin infection and even severe systemic disseminated infection. Traditional drug therapy is still the main therapy against sporotrichosis (Barros et al., 2011). Itraconazole (ITR) is a broad spectrum triazole antifungal drug. However, antifungal drugs must be used for extended periods and are prone to the emergence of Sporothrix globosa-resistant strains (Donnelly et al., 2008). In addition, they pose potential health risks to patients, especially patients with liver and kidney injury, children, and pregnant women (Tuccori et al., 2008). Therefore, the development of novel antifungal therapies is necessary. Among these, antifungal nanomaterials are promising (Minakshi et al., 2019).
Antimicrobial peptides (AMPs) are small peptides widely distributed in natural organisms. They boast a relatively broad spectrum and selective toxicity and do not easily develop drug resistance. They can selectively inhibit or kill bacteria, fungi, viruses, and other pathogenic microbes (Gao et al., 2018). In addition, they can promote cell healing and immunoregulation, demonstrating the potential for the development of new antifungal drugs. However, AMPs, especially natural AMPs, have relatively large molecular weights, weak antifungal activity, poor stability, hemolytic toxicity, complicated extraction processes, and other disadvantages (Gao et al., 2018). Most small-molecule AMPs from insects have low antifungal activity, are easy to degrade, and cannot maintain effective concentrations for a long time (Jenssen et al., 2006). Some AMPs exhibit strong antigenicity. These limitations limit the applications of AMPs. Transformation of the structure of natural AMPs, including peptide chain length and specific amino acids, can improve pathogenic fungi inhibitory activity while reducing their toxicity so that they may have immunogenicity but no antigenicity (Gao et al., 2018), thus improving their antifungal action.
Phages are bioactive nanomaterial. Exogenous genes can be inserted into the genes of filamentous phages so that target polypeptides can be displayed on the surface of the phage in the form of a fusion protein. Phages are harmless to humans, safe, simple to prepare, and inexpensive, making them suitable for large-scale development and application (Rehman et al., 2019). Different antigen-binding polypeptides can be fully displayed on the surface of phages in the form of multicopy, maintaining the natural conformation and stability of polypeptides, thus better inducing high antifungal biofilm activity. Therefore, antigen-binding polypeptides demonstrate significant potential for the development of new antibiotics (Chen et al., 2017; Chen et al., 2019). Sporothrix has biological properties that are closest to those of Candida albicans. Therefore, we used its structure as a template and transformed it into three segments of small-molecule AMPs, ToAP2A (GSKLIPGVMKLFSKK), ToAP2C (GAKLIPGVMKLFRKK), and ToAP2D (GDKLIPGVMKLFRKK, GK), using a reasonable molecular design. The killing effects of the three scorpion venom-derived AMPs after molecular genetic improvement on Sporothrix cultured in an isolated manner were then compared using in vitro experiments. The results showed that ToAP2D showed the highest anti-Sporothrix activity, good serum stability, and no acute toxicity, indicating its potential to be developed as a new anti-Sporothrix drug (Yan et al., 2021).
We first inserted gene fragments of the improved AMP ToAP2D into phage genes, prepared many AMPs using phage display techniques, and then passively inoculated the same as Sporothrix-infected mice. We also evaluated its antifungal, organ-inflammation-reducing, and animal survival-improving effects.
Materials and methods
Animals
All experimental protocols were approved by the Ethics Committee of the Institute for Laboratory Animal Research of the China-Japan Union Hospital of Jilin University (Jilin, Changchun) and were implemented in accordance with the guidelines for laboratory animal management of Jilin University. Female BALB/c mice (8–10-week-old; body weight: 16–20 g) were purchased from Yisi Laboratory Animal Technology Co., Ltd. (Changchun, Jilin, China). To reduce pain in the experimental animals, all operations were performed under adequate anesthesia with phenobarbital.
Strains and culture conditions
Sporothrix used in the experiment was isolated from a patient with disseminated sporotrichosis. The separated strains underwent strict transformation culture and molecular characterization and were identified as Sporothrix globosa. Sporothrix globosa was first cultured on Sabouraud’s medium at 25°C. Seven days later, the cultured fungi were transferred to brain heart infusion to culture at 37°C for 7 days with a shaker speed of 150 rpm. The culture fluid was centrifuged, resuspended in PBS, and diluted to 1 × 108 cells/mL.
Antimicrobial peptide synthesis
We previously reported ToAP2D, a 15-amino acid peptide (Antifungal Activity of ToAP2D 2021), which showed higher antifungal activity against S. globosa. Antifungal peptide ToAP2D was synthesized by ChinaPeptides Co., Ltd. (Suzhou, China), and its purity was determined to be higher than 95% using reversed-phase high-performance liquid chromatography and electrospray ionization mass spectrometry.
Construction of recombinant phage
Fuse-55, the phage vector, was reproduced in the E. coli TG1 strains. The wild phages used in this experiment were maintained in our laboratory. Construction of the recombinant phage vector fuse-55 and recombinant phage are described in the corresponding literature (Malik et al., 1996; Yang et al., 2005). Briefly, the synthesized complementary oligonucleotides encoding peptide GK were connected to BglI-digested fuse-55. E. coli TG1 transfected with the recombinant phage vector inserted into the peptide was cultured in Luria-Bertani liquid medium containing ampicillin. The culture supernatant containing phage particles was collected and precipitated twice in polyethyleneglycol-6000 (PEG-6000; final concentration: 5%; 0.5M NaCl) for 12 h. The phage particles were then resuspended in 2 ml of phosphate-buffered saline (PBS). The concentration of phage particles was determined using a spectrophotometer (OD at 270 nm was 1.0, corresponding to 0.26 μg/μL).
Western blotting
Antibodies to AMPs displayed on bacteriophages were purchased from China Peptides Co., Ltd. (Suzhou, China). The protein was extracted from the recombinant phage, denatured, electrophoresed, and transferred onto the PVDG membrane, which was then placed in Tris-tricine buffer saline (TBST). Subsequently, the protein membrane was blocked with 3% skim milk powder at 37°C for 60 min, followed by membrane washing with TBST four times for 3 min each. Next, the nitrocellulose membrane was incubated with 1:2000 diluted serum in TBST and 5% skim milk at 37°C for 1 h. After adding oxidase-coupled goat anti-mouse IgG (Vector Laboratories Inc., United States), the mixture was incubated at 37°C for 1 h. Finally, the membrane was put into the chemiluminescence-based image analysis system for development for 3 min.
In Vitro bacteriostasis experiment
A total of 50 μL of bacterial solution (concentration: 1 × 108 cells/mL) was coated onto broth solid medium, and autoclaved filter paper (0.5 cm in diameter) was pasted onto the surface of the solid medium. Then, 6 μL of hybrid phage expressing peptide GDKLIPGVMKLFRKK (phage-GK (PG)) (concentration: 4, 2, and 1 mg/ml by AMP concentration) and the control sample were dropped onto the filter paper and left in an incubator at 37°C for inverted culture for 5–7 days; the zone of inhibition was then observed.
Scanning electron microscopy (SEM)
The strains were divided into experimental and control groups; the former used a laser, while the latter was not illuminated. The two groups were cultured for 4 h in a 24-well plate on a cell culture slide. The supernatant was discarded, and each well was rinsed in PBS once and then fixed with 2.5% glutaraldehyde (0.2 M PBS, pH 6.0) for 1 h at 4°C. After fixation, the disks were rinsed three times with PBS and dehydrated in a graded series of ethanol (30, 50, 70, 80, and 90% for 7 min each and 100% for 10 min). The disks were critical point dried prior to being coated with a gold sputter and observed using SEM.
Cell apoptosis study
The strains were centrifuged at 5,000 × g for 5 min. Next, the cells were collected and resuspended in 800 μL of cell staining buffer. Hoechst 33342 (5 µL) staining solution was added to the solution and mixed well by turning it upside down. Then, 5 μL of propidium iodide (PI) was added to the solution and mixed as described above. The solution was then placed in an ice bath for 30 min. It was then rinsed once with PBS, smeared, and observed under a laser scanning confocal microscope (LSCM).
Measurement of Mitochondrial Membrane Potential (MMP)
Sporothrix globosa treated with the recombinant phage (1 × 108 CFU/ml) was washed and resuspended in PBS (pH 7.4). Then, JC-1 was added to the mixture at a final concentration of 5 μM, followed by incubation at 37°C for 20 min. Fluorescence absorption was detected using a fluorescence spectrophotometer at an excitation and emission wavelength of 490 and 530 nm, respectively.
Measurement of oxidative stress
DCFH-DA was diluted in yeast extract-peptone-dextrose (YPD) liquid medium at 1:1,000 to a final concentration of 10 μM. The Sporothrix solution was obtained after overnight culture, and the cells were collected and suspended in diluted DCFH-DA. The cells, at a concentration of 1 × 108 cells/mL, were left for incubation at 37 °C for 20 min. The cells were washed with YPD liquid medium three times. Cells in the experimental and control groups were stimulated directly with reactive oxygen species (ROS)-positive controls and solvents. After 20 min, fluorescence absorption was measured using a fluorescence tester at an excitation and emission wavelength of 490 and 530 nm, respectively. ROS accumulation was calculated using the formula: (Ftest-Fblank)/(Fcontrol-Fblank), where Ftest is the sample fluorescence value after laser treatment, Fcontrol is the sample fluorescence value in the PBS treatment group, and Fblank is the fluorescence value in wells without cells.
Detection of metacaspase activation
After laser treatment, the Sporothrix solution from the experiment and control groups was centrifuged at 2000 × g for 3 min, and the cells were resuspended. Then, the cells were stained with 10 μM of CaspACE FITC-VAD-FMK for 20 min at 37°C, followed by rinsing with PBS twice. The cells were then smeared and observed under LSCM. The fluorescence absorption was tested using a fluorescence tester at an excitation and emission wavelength of 494 and 530 nm, respectively.
Treating Sporothrix-Infected Mice with Recombinant Phage
The mouse model of disseminated Sporothrix globosa was administered an intravenous injection of 0.2 ml (1 × 108 cells/mL) of Sporothrix globosa suspension. Mice were randomly divided into four groups, each containing six mice. One day after infection, the following drugs were injected into the tail vein of each mouse every 3 days (6 injections in total): (1) 25 μg of PG in 100 μL PBS (PG group), (2) 25 μg of wild-type (WT) phage particles in 100 μL PBS (mock group), (3) ITR (5 mg/kg body weight, ITR group), or (4) PBS alone (PBS group; negative control). The mice were sacrificed on days 8 and 20 of infection.
Enzyme-linked immunosorbent assay (ELISA)
On days 8 and 20, the mouse sera were isolated and frozen at −80°C before use. Mouse serum IFN-γ and IL-17 levels were measured using Mlbio (Shanghai, China) ELISA kits, according to the manufacturer’s instructions. Absorbance was measured at 450 nm using a 96-well plate reader.
Lung histopathology
Mouse lung tissues were collected from each group and fixed in 10% buffered formalin for 24 h. After dehydrating and embedding in paraffin, the tissues were sectioned at approximately 5 µm using a paraffin slicer. Subsequently, the sections were stained with H&E to analyze the degree of inflammatory cell infiltration in the lung tissues, and the ImageJ software was used to quantify the percentage area of inflammatory cells on day 20. Neutrophils have very characteristic nuclei with three to five lobes connected by thin strands; and the size of neutrophils is about 10–15 µm. Lymphocytes are small cells, 8 to 10 microns in diameter and have large round nuclei.
Survival analysis
Mice were randomly divided into four groups of 10 mice each. Sporothrix globosa suspension (0.2 ml; 5 × 108 cells/mL) was injected into the tail vein of mice. On day 1 after infection, the mice were intraperitoneally injected with the above drugs. We observed a survival time of 2 weeks after treatment.
Evaluation of liver and renal function
The mice were randomly divided into two groups of seven mice each. The experimental group was injected with 25 μg of PG in 100 μL of PBS into the tail vein, and the control group was injected with 100 μL of PBS. Blood was drawn from the tail vein on day 3, and aspartate aminotransferase (AST), alanine aminotransferase (ALT), blood urea nitrogen (BUN), and creatinine (Cr) levels were measured.
Statistical analysis
Statistical analyses were performed using SPSS v24 (SPSS, Chicago, IL, United States). Values are expressed as the mean ± standard error of the mean for normally distributed data. One-way analysis of variance (ANOVA) and the Kruskal–Wallis H or Mann–Whitney U tests were performed to evaluate between-group differences. We examined the differences in survival time between different groups using the Kaplan-Meier test. The criterion for statistical significance was set at p < 0.05.
Results
Preparation of recombinant phage
The expression of PG was tested using western blotting. According to the reaction of AMP antibodies with fusion proteins in the hybrid phage, AMP-GK was displayed on the surface of the recombinant phage (Figure 1).
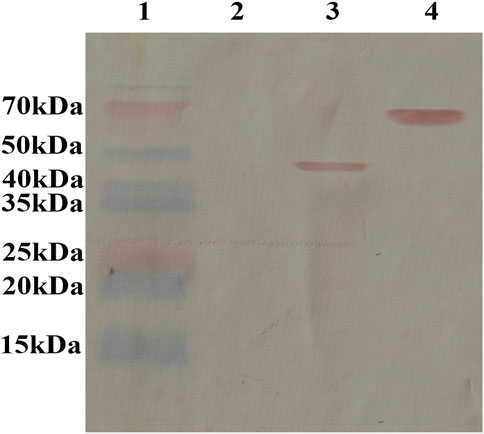
FIGURE 1. Protein expression of PG detected using western blotting. The antimicrobial peptide antibody reacted with the fusion protein in the hybrid phage, and the results showed that the antimicrobial peptide GK protein was displayed on the surface of the recombinant phage. Lane 1: marker (P12083); Lane 2: WT phage; Lane 3: recombinant phage displaying the GDKLIPGVMKLFRKK peptide; Lane 4: BSA link to GDKLIPGVMKLFRKK peptide.
In Vitro antifungal experiment
The inhibitory effect of PG on Sporothrix globosa was analyzed using the bacteriostatic circle test. The results showed that the recombinant phage had no obvious antifungal effects at a concentration of 1 mg/ml. When the concentration was increased to 2 and 4 mg/ml, the recombinant phage had an inhibitory effect on Sporothrix globosa. The inhibitory effect was obvious (11.15 ± 1.38 mm) when the concentration of recombinant phage was 4 mg/ml. This suggests that PG (antifungal peptide at a concentration of 4 mg/ml) has an effective antifungal activity against S. globosa (Table 1). Therefore, we used PG (antifungal peptide at a concentration of 4 mg/ml) for our experiments.
Morphological changes in S. globosa after recombinant phage treatment in vitro
Morphological changes on the surface of Sporothrix cells after recombinant phage treatment were observed using SEM. After the control treatment, S. globosa cells showed normal morphology, smooth and complete surface, regular shape, and clear boundaries. However, after recombinant phage treatment, Sporothrix became irregular in shape, with shrinkage and rupture of the cell membrane, cross-linked strains, and leakage of cell contents (Figure 2).
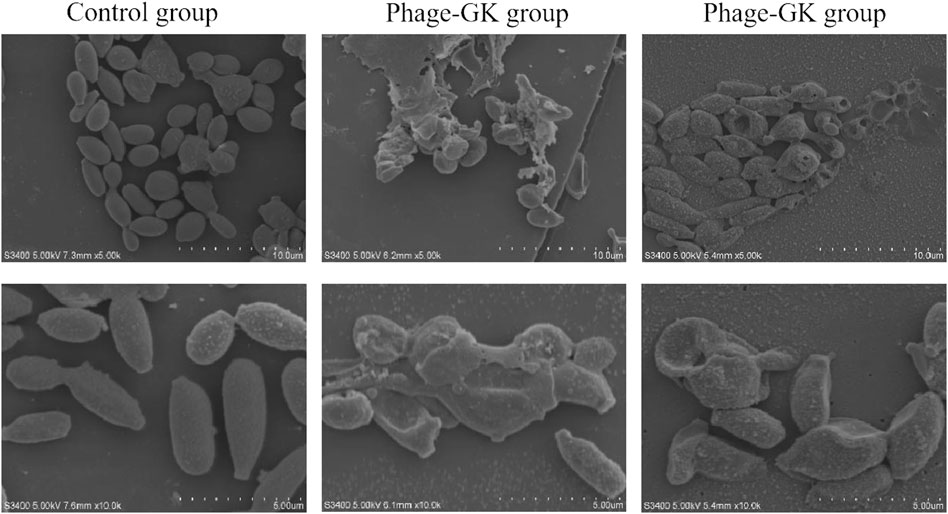
FIGURE 2. Control group under a scanning electron microscope. The normal morphology of S. globosa cells is oval-shaped and plump. Phage-GK group after PG treatment; S. globosa cells are damaged and shriveled.
Sporothrix cell apoptosis after recombinant phage treatment
Hoechst 33342 can penetrate the cell membrane and bind to the chromosomes in apoptotic cells. Apoptotic cells showed significantly enhanced fluorescence compared to normal cells after staining (Yin et al., 2014). However, PI cannot penetrate the cell membrane. Normal or apoptotic cells with complete membranes were not stained. After double staining, under a fluorescence microscope, apoptotic cells showed faint red and strong blue fluorescence; necrotic cells showed strong red and strong blue fluorescence. In the recombinant phage treatment group, strong blue and red fluorescence (Hoechst+/PI+) were observed, indicating both apoptosis and necrosis in S. globosa. In contrast, in the control group, only faint blue and red fluorescence (Hoechst+/PI-) were observed, indicating a normal state of S. globosa (Figure 3).
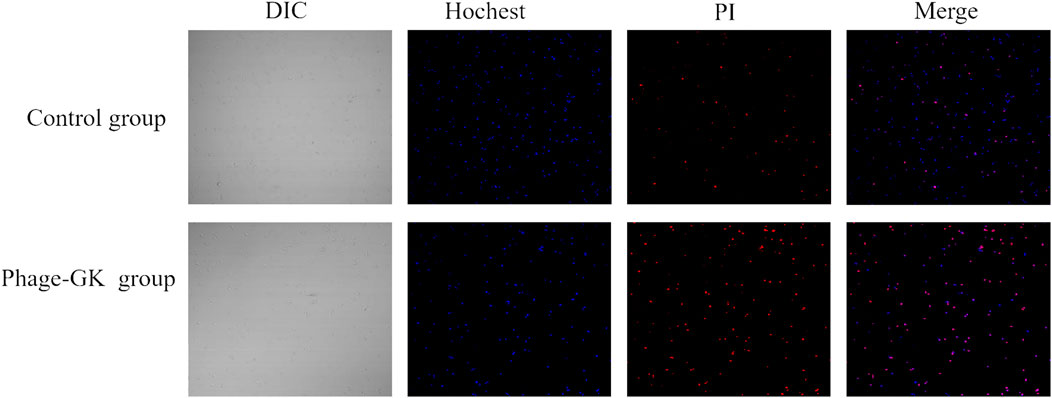
FIGURE 3. Necrosis and apoptosis of S. globosa cells after PG treatment observed using confocal laser scanning microscopy after Hoechst/PI staining.
Effect of recombinant phage treatment on MMP in sporothrix cells
Mitochondrial dysfunction initiates cell apoptosis (Eisenberg et al., 2007). At a higher mitochondrial membrane potential (MMP), JC-1 accumulates in the mitochondrial matrix and forms J-aggregates, producing red fluorescence. At lower MMPs, JC-1 cannot gather in the mitochondrial matrix but is a monomer that produces green fluorescence. The relative proportions of red and green fluorescence were used to measure mitochondrial depolarization. Through the transition of JC-1 from red to green fluorescence, it is easy to detect a decline in the cell membrane potential. Such a JC-1 transition can serve as an index for testing early cell apoptosis. According to the immunofluorescence results, compared with the control group, the recombinant phage treatment group showed a transition from red to green fluorescence. The fluorescence ratio of S. globosa cells in the control group was almost 25.84 ± 1.34, confirming no obvious depolarization. However, the fluorescence ratio of S. globosa cells in the recombinant phage group was reduced to 4.24 ± 0.26 (mean ± SEM) (p < 0.001), indicating no significant depolarization in S. globosa after the treatment (Figures 4A, B). In short, recombinant phages cause a decline in the MMP in S. globosa, indicating cell apoptosis.
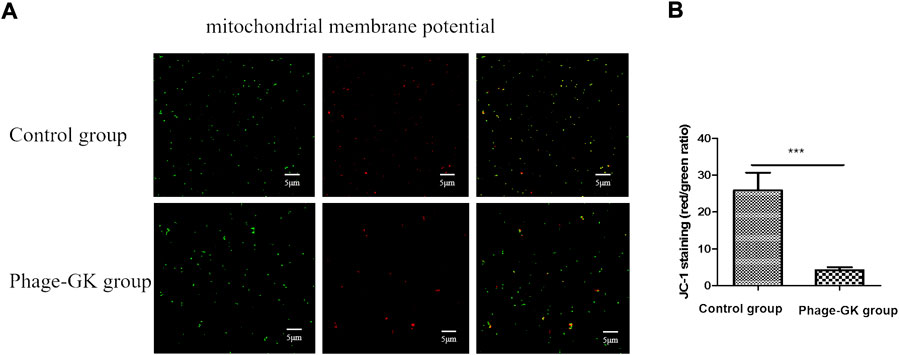
FIGURE 4. Effects of PG treatment on MMP. (A) Confocal laser scanning microscopy to evaluate the effects of PG treatment on MMP in S. globosa cells of the study and control groups. (B) Flow cytometry was used to detect the MMP. ***p < 0.001 indicates statistical significance.
Effect of recombinant phage treatment on ROS accumulation in sporothrix cells
Mitochondrial depolarization, accompanied by an endogenous increase in ROS, is a characteristic of cell apoptosis (Nury et al., 2021). After recombinant phage treatment, ROS accumulation in cells was significantly improved, without a significant difference from the positive control. No change was observed in ROS accumulation in the control group. This indicates that recombinant phage-induced cell apoptosis may be achieved through the ROS-mediated apoptosis pathway (Figure 5).
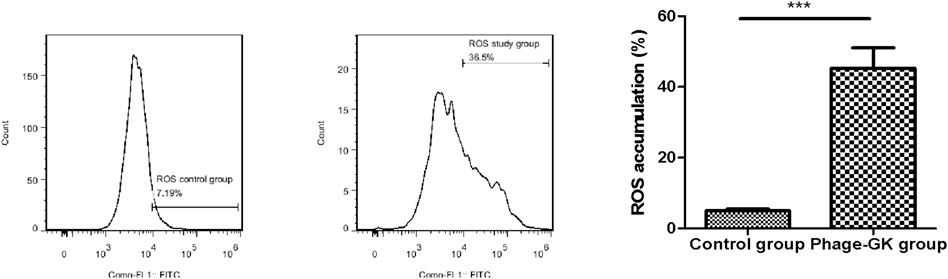
FIGURE 5. Effect of PG treatment on ROS accumulation in S. globosa cells. Flow cytometry was used to detect ROS accumulation. ***p < 0.001 indicates statistical significance.
Effect of recombinant phage treatment on caspase activity in sporothrix
The fungal apoptotic pathway consists of various regulatory factors and effectors (Sharon et al., 2009). In this study, we focused on the caspase apoptotic pathway. Based on the experimental results, a small amount of fluorescence was detected in the blank control. However, in the recombinant phage treatment group, most cells showed bright green fluorescence, indicating that the recombinant phage activated the caspase-dependent cell apoptosis pathway in Sporothrix cells (Figure 6).
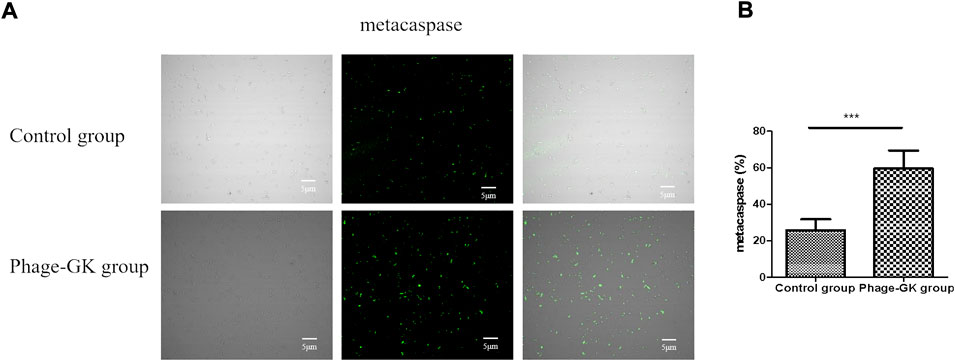
FIGURE 6. Effects of PG treatment on metacaspase activation. (A) Confocal laser scanning microscopy to evaluate the effects of PG treatment on metacaspase activation in S. globosa cells of the study and control groups as observed by staining with CaspACE FITC-VAD-FMK. DIC, differential inference contrast. (B) Flow cytometry was used to detect the metacaspase activation. ***p < 0.001 indicate statistical significance.
Changes in IFN-γ and IL-17 Levels after Phage-GK (PG) Treatment in Mice
To assess the development of cell-mediated immunity, the levels of cytokines IFN-γ and IL-17 were assessed using ELISA on days 8 and 20. As shown in Figure 7A and C, the serum IFN-γ and IL-17 levels were significantly higher in the PG group (p < 0.001) than in the PBS group on day 8. Serum IL-17 levels were also significantly higher in the PG group than those in the WT and ITR groups (p < 0.01). On day 20 (Figures 7B, D), no significant changes in IFN-γ and IL-17 levels were observed in any of the study groups. These results showed that recombinant phage displaying ToAP2D peptide induced IFN-γ and IL-17 expression. IFN-γ and IL-17 are the main cytokines secreted by Th1 and Th17 cells, respectively. We speculated that phage displaying ToAP2D peptide activate Th1 and Th17 responses, promoting antifungal immunity (Figure 7).
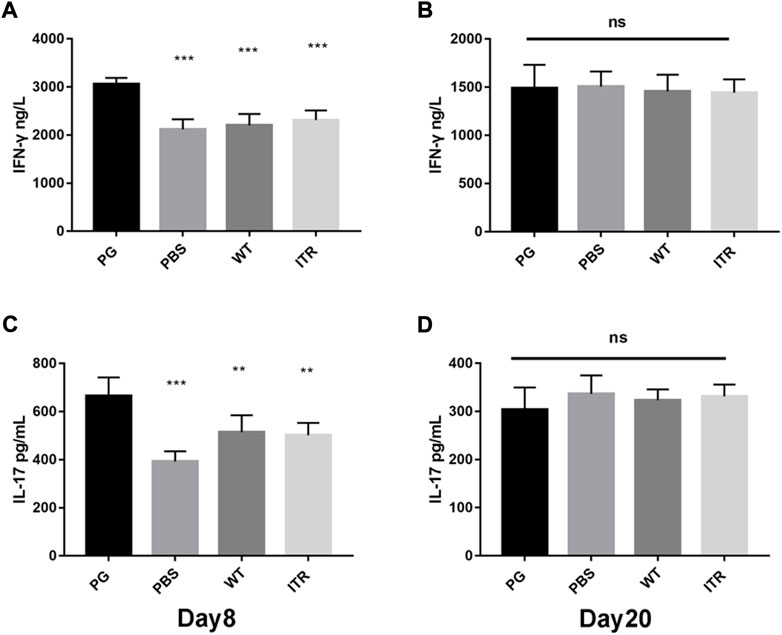
FIGURE 7. IFN-γ and IL-17 levels after PG treatment in mice. (A,B) Change in IFN-γ levels in mice on days 8 and 20 after S. globosa infection. (C,D) Change in IL-17 levels in mice on days 8 and 20 after S. globosa infection. Compared to the levels in mice from the PG group, ***p < 0.001, **p < 0.01, ns: no significance. Results shown are representative of three independent experiments. WT: wild-type phage injection; ITR: itraconazole injection; PG: phage-GK injection.
Recombinant phage effectively mitigates inflammation in mice organs
The organs showed no obvious lesions as observed under naked eye. However, after H&E staining, on day 8, mice in the PBS (55.37 ± 13.20%) (Figures 8A, E) and wild phage groups (50.34 ± 7.29%) (Figures 8B, E) showed invasion of a large number of lymphocytes and neutrophils in the lungs, indicating significant inflammation. In contrast, in the recombinant phage (24.28 ± 5.69%) (Figures 8D, E) and ITR groups (19.95 ± 4.93%) (Figures 8C, E), lymphocytes and neutrophils of only medium levels were observed, indicating mild inflammation.
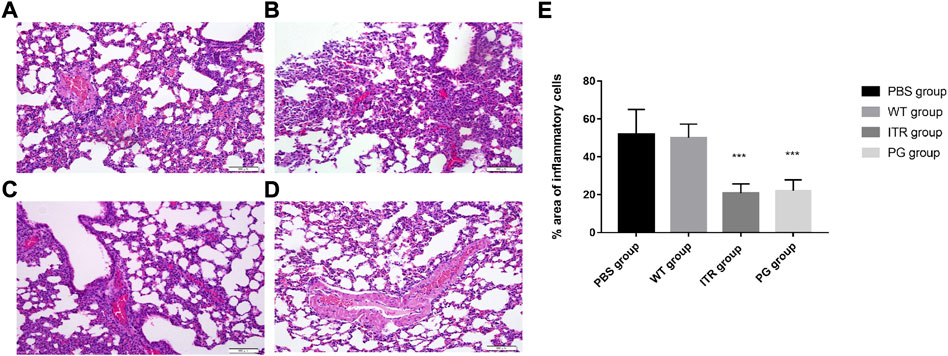
FIGURE 8. Images of H&E-stained lungs of S. globosa-infected mice treated with various formulations on day 20; magnification ×200. (A) PBS injection, (B) WT injection, (C) itraconazole injection, and (D) PG injection. (A,B) A higher number of inflammatory cells was observed. In contrast, (C,D) showed a dramatic decrease in neutrophil and lymphocyte infiltration. (E) Compared with the PBS group, the percentage area of inflammatory cells in the different treatment groups on day 20. Results are representative of three independent experiments. WT: wild-type phage injection; ITR: itraconazole injection; PG: phage-GK injection.
Recombinant phage effectively prolong the survival time of mice with sporotrichosis
The detection time for mouse survival was 14 days (Figure 9). The experimental results showed that mice in the experimental group injected with recombinant phage and ITR showed the highest 14-day survival, reaching 80 and 70%, respectively, with no significant difference. Mice injected with PBS showed significantly reduced 14-day survival (only 20%), and survival of the WT group was 30%. Therefore, the survival rate of the recombinant phage-immunized mice was significantly higher than that of the PBS and WT groups.
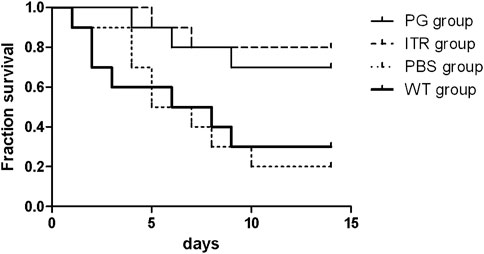
FIGURE 9. Survival rate of S. globosa-infected mice treated with PG, ITR, WT, or PBS alone. WT: wild-type phage injection; ITR: itraconazole injection; PG: phage-GK injection.
Low toxicity of recombinant phage
Mice injected with recombinant phage showed clinical biochemical indexes, including AST, ALT, urea, and creatinine, within normal limits, with no significant difference from the control group (Figure 10).
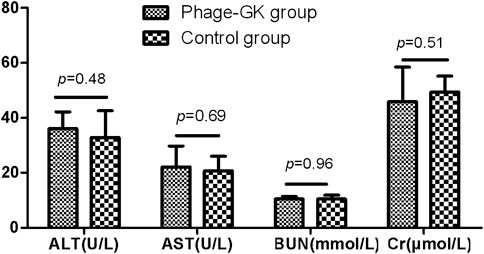
FIGURE 10. AST, ALT, BUN, and Cr levels after recombinant phage injection. AST, aspartate aminotransferase; ALT, alanine aminotransferase; BUN, blood urea nitrogen; Cr, creatinine.
Discussion
Sporotrichosis is distributed worldwide, with the number of relevant cases increasing significantly in humans and animals over the past 20 years (Chakrabarti et al., 2015; Queiroz-Telles et al., 2019). Antibiotic resistance has improved worldwide. In contrast, the development of new antibiotics has slowed significantly, increasing interest in new antimicrobial therapy (Barros et al., 2011; García Carnero et al., 2018). The treatment of fungal infections with AMP has received increasing attention. However, due to its large molecular weight, insufficient antifungal activity, poor stability, and other shortcomings, AMP treatment has been limited (Fry, 2018; Gao et al., 2018; Browne et al., 2020). In this study, we improved natural AMPs and displayed improved AMPs on the surface of the phage. This involves a simple preparation process and low cost and yields stable AMPs after restructuring. Based on the results of in vitro experiments, recombinant AMPs effectively promoted cell apoptosis and inhibited Sporothrix growth. Moreover, recombinant phages had a good therapeutic effect on Sporothrix-infected mice.
We explored the effect of recombinant phage treatment on S. globosa using LSCM, flow cytometry, SEM, and other experimental methods. After recombinant phage treatment of S. globosa, significant shrinkage was observed on the cell surface, with the leakage of cell contents in large amounts, indicating necrosis. Apoptosis and necrosis of S. globosa cells were confirmed using Hoechst/PI double staining. Apoptosis has many typical characteristics, abnormal MMP being one of the earliest events. A decline in MMP indicates a change in the mitochondrial membrane structure. According to this research, after recombinant phage treatment, MMP in S. globosa declined significantly, thus damaging the mitochondria. Mitochondria are the center for the control of cellular life activities and the regulation of cell apoptosis. Damaged mitochondria lead to abnormal leakage of electrons into the mitochondrial respiratory chain, thereby causing ROS accumulation in the mitochondria. ROS are the main mediating factor of fungal apoptosis, leading to the death of Sporothrix by oxidizing biomacromolecules in cells. According to the experimental results, after recombinant phage treatment, S. globosa cells showed a significantly enhanced ROS level. This agrees with the previous viewpoint that antifungal agents attack mitochondria by producing excessive ROS, thus inhibiting mitochondrial respiratory function and killing fungi (Kwun & Lee, 2020).
Fungal apoptosis is regulated by various regulators and effector substances (Hamann et al., 2008; Sharon et al., 2009). Currently, there are two apoptotic pathways in yeast: caspase-dependent and caspase-independent. Approximately 40% of fungal apoptosis occurs via the first pathway (Sharon et al., 2009). According to relevant research, ROS can activate metacaspases in fungi, leading to fungal apoptosis (Perrone et al., 2008; Tudzynski et al., 2012). Therefore, this experiment further explored the changes in metacaspase activity in S. globosa after recombinant phage treatment. According to the results, metacaspase in S. globosa was activated after the treatment. Under normal conditions, metacaspases are inactive; however, when external stimuli are present, metacaspases can be activated, causing fungal apoptosis. Therefore, the recombinant phage induced apoptosis in Sporothrix via the metacaspase-dependent pathway.
For the first time, we employed PG in sporotrichosis mice and reported that it could increase IFN-γ and IL-17 levels, reducing the severity of infection. T helper (Th) cells reportedly induce antigen-specific T-cell responses in the early stage of inflammation (Cosmi et al., 2014). Th1 and Th17 cell responses are involved in anti-Sporothrix infection in the body (Yan et al., 2020). Th1 cell subset is mainly involved in the activation of cellular immunity by secreting IL-2 and IFN-γ, which play important roles in inflammation. IFN-γ inhibits C. albicans growth by stimulating the generation of derived cytokines in the body, improving the phagocytic capacity of neutrophils against C. albicans, thus enhancing the body’s resistance to C. albicans infection (Tarumoto et al., 2014). Th17 cells are also a subset of T lymphocytes that mainly secrete IL-17; they have received increasing attention for their resistance to pathogen infection (Cypowyj et al., 2012; Mear et al., 2014). According to the experimental results, after injection of the recombinant phage, IFN-γ and IL-17 levels in the blood of Sporothrix-infected mice increased significantly. Therefore, phage-displaying AMPs can trigger the cellular immune response of Th1 and Th17 cells in the body, thereby achieving fungal resistance. Improvement in the levels of Th1 and Th17 in the blood of infected mice is therefore favorable for the removal of Sporothrix and alleviating the disease (Chen et al., 2016; Yan et al., 2020). At day 20, the increasing trend of IFN-γ and IL-17 discontinued. It is suggested that the experimental group can clear the pathogen in the early stage, while the control group can not induce enough immune response in the early stage. Therefore, the inflammation will not heal and aggravate the tissue damage in the control group.
Although the antifungal mechanism of AMPs remains unclear, a widely accepted theory is the cell membrane damaging effect, positively charged AMPs and negatively charged microbial cell membranes show electrostatic attraction. AMPs cause leakage of intracellular water-soluble substances by destroying the fungal plasma membrane, eventually leading to lysis and death of the fungi (Delattin et al., 2017; Polesello et al., 2017). In addition, AMPs can work on fungi in many ways, including membrane action and interaction with important organelles, such as mitochondria and nucleic acid macromolecules in fungal cells, eventually causing fungi death (Delattin et al., 2017; Polesello et al., 2017). Due to their low drug resistance and low toxicity, AMPs show corresponding research value and development potential. Microorganisms have a very low probability of developing resistance to AMPs. This is because AMPs bind to the microbial cell membrane by electrostatic attraction, meaning that microorganisms must change their membrane structure to avoid AMP attacks (Lazzaro et al., 2020). However, the negative charge of the microbial cell membrane is the result of evolution over hundreds of millions of years; it is an important conservative membrane component widely found in microbial communities. Therefore, it is particularly difficult for microorganisms to become resistant to AMPs.
Phage particles can remain stable for a long period and are highly resistant to physical and chemical factors (Sharma et al., 2017). This method is highly applicable in both experimental studies and clinical applications. Additionally, phage vectors show good immunogenicity. Small synthetic peptides usually bind to large vectors or require adjuvants to effectively trigger immune responses. However, phage vectors can produce intense immunoreactions without adjuvants. In addition, we propose a new method for the preparation of AMPs, phage displays. In our experiment, we used the self-synthesis assembly and release of phages to produce many AMP-displaying recombinant phages. Through the binding of recombinant phage with phage antibodies (Figure 1), AMP antibodies react with recombinant phage PG, indicating that the specific reaction of the antibodies with recombinant phage is with AMPs in recombinant phage rather than phage components.
ITR-resistant S. globosa strains have also been reported (Vettorato et al., 2018). The use of PG as an adjuvant treatment can lead to an overall improvement in the efficacy of antifungal chemotherapy, as well as a reduced duration of antifungal treatment and reduced inflammation reaction. Moreover, with a reduced duration of antifungal drug application, PG may avoid the adverse side effects of the drugs, especially in patients with liver dysfunction, pregnant women, and children who are not suitable for antifungal drug therapy (Kauffman et al., 2007).
Conclusion
The results of this study demonstrated that the recombinant phage effectively inhibited the growth of Sporothrix globosa by activating fungal apoptosis. During this process, the morphological structure, MMP, ROS accumulation, and metacaspase activation of Sporothrix globosa cells changed significantly. Moreover, PG induced the expression of IFN-γ and IL-17, and its therapeutic effect on mice infected with Sporothrix globosa was almost equivalent to that of ITR. In conclusion, our results suggest that the application of phage-based nanomaterials displaying ToAP2D peptides may be a new, safe, and effective method for the treatment of Sporothrix globosa infection.
Data availability statement
The original contributions presented in the study are included in the article/supplementary materials, further inquiries can be directed to the corresponding author.
Ethics statement
The animal study was reviewed and approved by Ethics Committee of the Institute for Laboratory Animal Research of China-Japan Union Hospital of Jilin University (Jilin, Changchun).
Author contributions
Conception and design of the research: FC Acquisition of data: TY and LA Analysis and interpretation of data: FC and TY Statistical analysis: FC and TY.
Funding
This study was supported partially by grants from National Natural Science Foundation of China (No.82003368) and Health research talents Special project of Jilin Province (No.2021SCZ02).
Conflict of interest
The authors declare that the research was conducted in the absence of any commercial or financial relationships that could be construed as a potential conflict of interest.
Publisher’s note
All claims expressed in this article are solely those of the authors and do not necessarily represent those of their affiliated organizations, or those of the publisher, the editors and the reviewers. Any product that may be evaluated in this article, or claim that may be made by its manufacturer, is not guaranteed or endorsed by the publisher.
Supplementary material
The Supplementary Material for this article can be found online at: https://www.frontiersin.org/articles/10.3389/fphar.2022.1022651/full#supplementary-material
References
Barros, M. B., de Almeida Paes, R., and Schubach, A. O. (2011). Sporothrix schenckii and sporotrichosis. Clin. Microbiol. Rev. 24 (4), 633–654. doi:10.1128/CMR.00007-11
Browne, K., Chakraborty, S., Chen, R., Willcox, M. D., Black, D. S., Walsh, W. R., et al. (2020). A new era of antibiotics: The clinical potential of antimicrobial peptides. Int. J. Mol. Sci. 21 (19), E7047. doi:10.3390/ijms21197047
Chakrabarti, A., Bonifaz, A., Gutierrez-Galhardo, M. C., Mochizuki, T., and Li, S. (2015). Global epidemiology of sporotrichosis. Med. Mycol. 53 (1), 3–14. doi:10.1093/mmy/myu062
Chen, F., Jiang, R., Dong, S., and Yan, B. (2019). Efficient treatment of Sporothrix globosa infection using the antibody elicited by recombinant phage nanofibers. Front. Pharmacol. 10, 160. doi:10.3389/fphar.2019.00160
Chen, F., Jiang, R., Wang, Y., Zhu, M., Zhang, X., Dong, S., et al. (2017). Recombinant phage elicits protective immune response against systemic S. Globosa infection in mouse model. Sci. Rep. 7, 42024. doi:10.1038/srep42024
Chen, F., Yan, B. L., An, L., and Jiang, R. H. (2016). Is there a relationship between the percentage of T helper cells and prognosis for sporotrichosis? Br. J. Dermatol. 174 (6), 1398–1400. doi:10.1111/bjd.14377
Cosmi, L., Maggi, L., Santarlasci, V., Liotta, F., and Annunziato, F. (2014). T helper cells plasticity in inflammation. Cytom. A 85 (1), 36–42. doi:10.1002/cyto.a.22348
Cypowyj, S., Picard, C., Maródi, L., Casanova, J. L., and Puel, A. (2012). Immunity to infection in IL-17-deficient mice and humans. Eur. J. Immunol. 42 (9), 2246–2254. doi:10.1002/eji.201242605
Delattin, N., Brucker, K., Cremer, K., Cammue, B. P., and Thevissen, K. (2017). Antimicrobial peptides as a strategy to combat fungal biofilms. Curr. Top. Med. Chem. 17 (5), 604–612. doi:10.2174/1568026616666160713142228
Donnelly, R. F., McCarron, P. A., and Tunney, M. M. (2008). Antifungal photodynamic therapy. Microbiol. Res. 163 (1), 1–12. doi:10.1016/j.micres.2007.08.001
Eisenberg, T., Büttner, S., Kroemer, G., and Madeo, F. (2007). The mitochondrial pathway in yeast apoptosis. Apoptosis 12 (5), 1011–1023. doi:10.1007/s10495-007-0758-0
Gao, Y., Fang, H., Fang, L., Liu, D., Liu, J., Su, M., et al. (2018). The modification and design of antimicrobial peptide. Curr. Pharm. Des. 24 (8), 904–910. doi:10.2174/1381612824666180213130318
García Carnero, L. C., Lozoya Pérez, N. E., González Hernández, S. E., and Martínez Álvarez, J. A. (2018). Immunity and treatment of sporotrichosis. J. Fungi 4 (3), E100. doi:10.3390/jof4030100
Hamann, A., Brust, D., and Osiewacz, H. D. (2008). Apoptosis pathways in fungal growth, development and ageing. Trends Microbiol. 16 (6), 276–283. doi:10.1016/j.tim.2008.03.003
Jenssen, H., Hamill, P., and Hancock, R. E. (2006). Peptide antimicrobial agents. Clin. Microbiol. Rev. 19 (3), 491–511. doi:10.1128/CMR.00056-05
Kauffman, C. A., Bustamante, B., Chapman, S. W., and Pappas, P. G. (2007). Clinical practice guidelines for the management of sporotrichosis: 2007 update by the infectious diseases society of America. Clin. Infect. Dis. 45 (10), 1255–1265. doi:10.1086/522765
Kwun, M. S., and Lee, D. G. (2020). Quercetin-induced yeast apoptosis through mitochondrial dysfunction under the accumulation of magnesium in Candida albicans. Fungal Biol. 124 (2), 83–90. doi:10.1016/j.funbio.2019.11.009
Lazzaro, B. P., Zasloff, M., and Rolff, J. (2020). Antimicrobial peptides: Application informed by evolution. Science 368 (6490), eaau5480. doi:10.1126/science.aau5480
Malik, P., Terry, T. D., Gowda, L. R., Langara, A., Petukhov, S. A., Symmons, M. F., et al. (1996). Role of capsid structure and membrane protein processing in determining the size and copy number of peptides displayed on the major coat protein of filamentous bacteriophage. J. Mol. Biol. 260 (1), 9–21. doi:10.1006/jmbi.1996.0378
Mear, J. B., Gosset, P., Kipnis, E., Faure, E., Dessein, R., Jawhara, S., et al. (2014). Candida albicans airway exposure primes the lung innate immune response against Pseudomonas aeruginosa infection through innate lymphoid cell recruitment and interleukin-22-associated mucosal response. Infect. Immun. 82 (1), 306–315. doi:10.1128/IAI.01085-13
Minakshi, P., Ghosh, M., Brar, B., Kumar, R., Lambe, U. P., Ranjan, K., et al. (2019). Nano-antimicrobials: A new paradigm for combating mycobacterial resistance. Curr. Pharm. Des. 25 (13), 1554–1579. doi:10.2174/1381612825666190620094041
Nury, T., Zarrouk, A., Yammine, A., Mackrill, J. J., Vejux, A., and Lizard, G. (2021). Oxiapoptophagy: A type of cell death induced by some oxysterols. Br. J. Pharmacol. 178 (16), 3115–3123. doi:10.1111/bph.15173
Perrone, G. G., Tan, S. X., and Dawes, I. W. (2008). Reactive oxygen species and yeast apoptosis. Biochim. Biophys. Acta 1783 (7), 1354–1368. doi:10.1016/j.bbamcr.2008.01.023
Polesello, V., Segat, L., Crovella, S., and Zupin, L. (2017). Candida infections and human defensins. Protein Pept. Lett. 24 (8), 747–756. doi:10.2174/0929866524666170807125245
Queiroz-Telles, F., Buccheri, R., and Benard, G. (2019). Sporotrichosis in immunocompromised hosts. J. Fungi 5 (1), E8. doi:10.3390/jof5010008
Rehman, S., Ali, Z., Khan, M., Bostan, N., and Naseem, S. (2019). The dawn of phage therapy. Rev. Med. Virol. 29 (4), e2041. doi:10.1002/rmv.2041
Sharma, S., Chatterjee, S., Datta, S., Prasad, R., Dubey, D., Prasad, R. K., et al. (2017). Bacteriophages and its applications: An overview. Folia Microbiol. 62 (1), 17–55. doi:10.1007/s12223-016-0471-x
Sharon, A., Finkelstein, A., Shlezinger, N., and Hatam, I. (2009). Fungal apoptosis: Function, genes and gene function. FEMS Microbiol. Rev. 33 (5), 833–854. doi:10.1111/j.1574-6976.2009.00180.x
Tarumoto, N., Kinjo, Y., Kitano, N., Sasai, D., Ueno, K., Okawara, A., et al. (2014). Exacerbation of invasive Candida albicans infection by commensal bacteria or a glycolipid through IFN-γ produced in part by iNKT cells. J. Infect. Dis. 209 (5), 799–810. doi:10.1093/infdis/jit534
Tuccori, M., Bresci, F., Guidi, B., Blandizzi, C., Del Tacca, M., and Di Paolo, M. (2008). Fatal hepatitis after long-term pulse itraconazole treatment for onychomycosis. Ann. Pharmacother. 42 (7), 1112–1117. doi:10.1345/aph.1L051
Tudzynski, P., Heller, J., and Siegmund, U. (2012). Reactive oxygen species generation in fungal development and pathogenesis. Curr. Opin. Microbiol. 15 (6), 653–659. doi:10.1016/j.mib.2012.10.002
Vettorato, R., Heidrich, D., Fraga, F., Ribeiro, A. C., Pagani, D. M., Timotheo, C., et al. (2018). Sporotrichosis by Sporothrix schenckii senso stricto with itraconazole resistance and terbinafine sensitivity observed in vitro and in vivo: Case report. Med. Mycol. Case Rep. 19, 18–20. doi:10.1016/j.mmcr.2017.10.001
Yan, T., Li, F., and Chen, F. (2020). Imbalance of Th1, Th17, and treg cells in peripheral blood of patients with lymphocutaneous sporotrichosis: A comparative study. Eur. J. Dermatol. 30 (4), 345–351. doi:10.1684/ejd.2020.3838
Yan, T., Li, F., Li, J., and Chen, F. (2021). Antifungal activity of ToAP2D peptide against Sporothrix globosa. Front. Bioeng. Biotechnol. 9, 761518. doi:10.3389/fbioe.2021.761518
Yang, Q., Wang, L., Lu, D. N., Gao, R. J., Song, J. N., Hua, P. Y., et al. (2005). Prophylactic vaccination with phage-displayed epitope of C. albicans elicits protective immune responses against systemic candidiasis in C57BL/6 mice. Vaccine 23 (31), 4088–4096. doi:10.1016/j.vaccine.2004.07.005
Keywords: Sporothrix, immune responses, antimicrobial peptides, ToAP2D peptide, recombinant phage
Citation: Yan T, An L and Chen F (2022) Recombinant phage displaying ToAP2D peptide with antifungal activity against Sporothrix globosa. Front. Pharmacol. 13:1022651. doi: 10.3389/fphar.2022.1022651
Received: 18 August 2022; Accepted: 20 September 2022;
Published: 07 October 2022.
Edited by:
Jianxun Ding, Changchun Institute of Applied Chemistry (CAS), ChinaReviewed by:
Xianglan Zhang, Yonsei University, South KoreaChao Huang, University of Chinese Academy of Science, China
Hassan Oliver James Morad, King’s College London, United Kingdom
Xuefu Wang, Anhui Medical University, China
Yunfeng Zhao, Ochsner LSU Health, United States
Copyright © 2022 Yan, An and Chen. This is an open-access article distributed under the terms of the Creative Commons Attribution License (CC BY). The use, distribution or reproduction in other forums is permitted, provided the original author(s) and the copyright owner(s) are credited and that the original publication in this journal is cited, in accordance with accepted academic practice. No use, distribution or reproduction is permitted which does not comply with these terms.
*Correspondence: Feng Chen, Y2hlbmZlbmdkb2N0b3JAamx1LmVkdS5jbg==