- 1Département de Chimie, Université Côte d’Azur, Nice, France
- 2Group Bioactivities of Natural compounds and derivatives (BIONA), Formerly Group Immunity and Inflammation (GIMIN), Institut Pasteur of New Caledonia, Member of the Pasteur Network, Noumea, New Caledonia
- 3Neuroinflammation Group, Macquarie Medical School, Macquarie University, Sydney, NSW, Australia
- 4Institut de Science et d’Ingénierie Supramoléculaires, Université de Strasbourg, Strasbourg, France
- 5PHARMADEV, UMR152, Institut de Recherche pour le Développement (IRD), Noumea Center, Noumea, New Caledonia
- 6Institut des Sciences Exactes et Appliqués (ISEA), EA7484, Université de Nouvelle-Calédonie, Noumea, New Caledonia
Introduction: Formerly named Plectranthus forsteri, Coleus forsteri (Benth.) A.J.Paton, 2019 is a Lamiaceae traditionally used to treat flu-like symptoms and shock-related ecchymosis, especially in the Pacific region. Few studies investigated chemical composition and anti-inflammatory potential of this plant.
Method: Herein, we investigated anti-inflammatory potential of C. forsteri ethanolic (ePE) and cyclohexane (cPE) plant extract on LPS-induced human macrophages models and quantified cytokines and quinolinic acid (QUIN) as inflammatory markers.
Results: Our results show that extract of ePE and cPE significantly inhibit inflammatory cytokine IL-6 and TNF-α induced by LPS on PMA-derived THP-1 macrophages. QUIN production is also diminished under ePE and cPE treatment in activated human monocyte-derived macrophages (MDMs). Seven abietane diterpenes were characterized from C. forsteri cPE including coleon U (1), coleon U-quinone (2), 8α,9α-epoxycoleon U-quinone (3), horminone or 7α-hydroxyroyleanone (4), 6β,7α-dihydroxyroyleanone (5), 7α-acetoxy-6β-hydroxyroyleanone (6) and 7α-formyloxy-6β-hydroxyroyleanone (7).
Discussion: We discussed potential contributions of these molecules from C. forsteri extracts for their anti-inflammatory activities.
1 Introduction
Immune-mediated inflammatory diseases (IMIDs) represent a group of diseases commonly sharing immune dysregulation (Kuek et al., 2007; El-Gabalawy et al., 2010). IMIDs include rheumatoid arthritis (RA), the spondyloarthritis (SpA) disease spectrum, systemic lupus erythematosus (SLE), connective tissue disorders, cutaneous inflammatory conditions like psoriasis and atopic dermatitis, inflammatory bowel disease (IBD), Crohn’s disease (CD) and ulcerative colitis (UC), asthma and autoimmune neurological diseases such as multiple sclerosis. The prevalence of IMIDs varies depending on the populations, and the overall prevalence of IMIDs in the Western society was estimated between 5% and 7% in 2010 (El-Gabalawy et al., 2010). More recently, 362,150 deaths were attributed to the top 15 IMIDs from 2013 to 2017 in the U.S. (Singh et al., 2020). The prevalence of IBD globally increased from 1990 to 2017 to reach 6.8 million cases worldwide in 2017 (Alatab et al., 2020). IMIDS has been considered for a long time to affect populations mainly from developed countries. Recent epidemiological studies showed that IMIDs is becoming increasingly common among immigrants from developing countries with accelerating incidence of IBD in recently industrialized regions (Ng et al., 2017; Agrawal et al., 2019; Quaresma et al., 2022). IMIDs are characterized by acute or chronic inflammation that can affect any organ and biological systems, leading to morbidity, reduced quality of life and premature death (El-Gabalawy et al., 2010).
Cytokines are critical mediators of the inflammatory process. They are produced to trigger the host immune response during infectious diseases and are also major drivers of the pathogenic inflammatory response in IMIDs. Exacerbated cytokine production translate as an amplification of the inflammatory responses leading to multiple deleterious biological effects. Treatments with corticoids and non-steroidal anti-inflammatory drugs (NSAIDs) are commonly prescribed to regulate the inflammatory response in IMIDs but long term therapy are associated with severe side effects, such as gastrointestinal ulceration and bleeding, osteoporosis, hypertension and glaucoma (Gautam and Jachak, 2009; Kondo and Amano, 2018; Wongrakpanich et al., 2018). The development of new therapies is targeting signaling pathways linked with inflammatory cytokines such as tumor necrosis factor (TNF), interleukins (ILs) or interferons (IFNs) mainly with the use of monoclonal antibodies (Kuek et al., 2007; Kondo and Amano, 2018; McInnes and Gravallese, 2021). Although these therapies show some clinical efficacy, patients might be or might become refractory to monoclonal antibody treatment as observed in 20%–40% of IBD-suffering patients. These type of therapies can also be associated with the development of opportunistic diseases related to immunosuppressive mechanisms (Hoffmann et al., 2017; Baker and Isaacs, 2018). These targeted treatments are also used to limit acute inflammation; “cytokine storm”, in patients with sepsis or COVID-19 (Athale et al., 2022) with variable success (Huang et al., 2019).
The kynurenine pathway (KP) is a major catabolic pathway of the essential amino acid tryptophan (TRP). The enzyme 2,3-dioxygenase (IDO1) is activated in the KP by inflammation molecules leading to the production of several bioactive metabolites such as kynurenine (KYN) and quinolinic acid (QUIN). QUIN induces the production of free radicals and exerts neuronal excitotoxicity resulting in neuronal damage and death. Activated human macrophages and peripheral monocytes showed a significant elevation of KP enzyme expression and metabolite production (Guillemin et al., 2003a; Guillemin et al., 2003b; Guillemin et al., 2003c; Jones et al., 2015). Dysregulation of the KP and abnormal levels of the KP metabolites were reported in autoimmune neurological diseases such as multiple sclerosis and contribute to their pathogenesis (Lovelace et al., 2016; Pires et al., 2022; Sundaram et al., 2022). Several clinical trials are currently assessing inhibitors of the KP targeting the IDO1 enzyme (Modoux et al., 2021; Pires et al., 2022). Natural products (NPs) are also explored as potential IDO1 inhibitors (Chen et al., 2012; Bhat et al., 2021).
Multiple NPs including plant extracts from traditional medicines are known to have significant anti-inflammatory effects by regulating of cytokine production and can be used for several inflammatory diseases such as RA, atopic dermatitis and IBD (Ghasemian et al., 2016; Luo et al., 2020; Wu et al., 2021; Zeng et al., 2022). Although the traditional knowledge about medicinal NPs is progressively getting lost, traditional plants are still commonly used in the Pacific region (Rageau and Schmid, 1973; Bourdy et al., 1992; Whistler, 1992). Among those plants, the large and medicinally important tropical plant genus Plectranthus sensu lato (Lamiaceae, subfamily Nepetoideae) was recently split into three separate genera based on molecular and morphological evidence: Plectranthus sensu stricto (72 species), Coleus (294 species) and Equilabium (42 species) (Paton et al., 2018; Paton et al., 2019). Previously classified as a Plectranthus species, Coleus forsteri (Benth.) A.J.Paton, 2019 (Figure 1) is an herbaceous plant found in the Southwest Pacific especially in New Caledonia, Vanuatu, Fiji Island, Tonga (Rageau and Schmid, 1973; Lormée et al., 2011; Paton et al., 2019). Named “Hmitre” or “Hnizi” or “Arnica kanak” by New Caledonian traditional peoples, the whole plant or leave extracts of C. forsteri are traditionally used to treat flu symptoms or as analgesic against shock (Rageau and Schmid, 1973; Suprin, 2008; Lormée et al., 2011). To our knowledge, only few chemical studies characterized polyphenols and diterpenoids from C. forsteri methanolic extract and essential oil, but no anti-inflammatory bioactivity has been published (Grayer et al., 2003; Wellsow et al., 2006; Kubínová et al., 2013). Herein, we assessed the anti-inflammatory potential and phytochemical profile of C. forsteri whole plant extracts. For this purpose, we studied the effect of the plant extracts on cytokine and QUIN production using lipopolysaccharide (LPS)-induced human macrophage in vitro models. Molecules from the plant cyclohexane extract were also characterized.
2 Materials and methods
2.1 Chemicals and reagents
All chemicals and reagents were obtained from Sigma (St Louis, United States), unless otherwise stated.
2.2 Plant collection and identification
C. fosteri whole plants were manually collected in Noumea, South Province, New Caledonia (S22°16', E166°27'). Authorization for sampling was accorded by the South Province (N°20798-2017/ARR/DENV du 25/09/2017). Specimen sample was referenced (NL189F2/QMC3) and conserved by the ISEA unit at the University of New Caledonia (UNC). Species identification was confirmed by botanists from the French National Research for Sustainable Development, New Caledonia IRD Center.
2.3 Preparation of plant extract
Whole plants were dried at 37 °C for 2 weeks and grounded. Dried powder (60 g) was extracted twice in 600 ml of cyclohexane for 4 h with ultrasonic treatment followed by two extractions with 600 ml of EtOH/H2O (70:30) for 4 h with ultrasonic treatment. Cyclohexane and hydroalcoholic extracts were filtered on Büchner and solvents evaporated to obtain 1.8 g and 7.9 g of dried extracts respectively, corresponding to 3.0% and 13.1% extraction yields. These cyclohexane (cPE) and ethanol (ePE) extracts of C. fosteri whole plant were referenced (QMC3 cyclo and QMC3 EtOH respectively) and conserved at −20°C until proceeding to biological assays.
2.4 Cell cultures and differentiation
The human THP-1 cell line (ATCC® TIB-202™) and the monocyte-derived macrophage (MDM) primary culture were used as in vitro models to study the anti-inflammatory effect of C. forsteri extracts. Cultures were maintained at 37°C in humidified atmosphere at 5% CO2 in specific culture media described below. THP-1 cells were cultured in RPMI 1640 medium (Corning; 10-043-CV) supplemented with HEPES 1M (Pan Biotech; P05-01100), 10% Fetal Bovine Serum (Dominique Dutscher; S 181B-500), 1% L-glutamine (Gibco; 35050-061) and 1% antibiotic (Antibiotic-Antimycotic 100X, Gibco; 15240-062). Monocytes were counted and 5 × 106 cells were resuspended at each passage once a week. Cells were seeded in 24-wells cell culture plates (1 × 106 cells/well, NEST, 0999052) and differentiated into macrophages after treatment with Phorbol 12-myristate 13-acetate (PMA, Sigma-Aldrich; P8139) at 10 ng/ml for 48 h (Schwende et al., 1996; Leu et al., 2019). Monocyte-derived macrophages (MDMs) were obtained from human blood monocytes purified from peripheral blood mononuclear cells (PBMCs). Briefly, PBMCs were isolated from healthy buffy coats obtained from the Australian Red Cross blood service under material supply agreement 17-07NSW-06. Buffy coats were diluted at equal volume of phosphate-buffered saline (PBS) and layered over a density gradient Lymphoprep™ (STEMCELL Technologies; 07861) and centrifuged at 400 × g for 40 min at 20°C with no brake. The isolated PBMC layer was collected and washed with PBS twice at 100 × g for 10 min at 20°C with brake. Cell pellets were resuspended in RPMI-1640 (ThermoFisher Scientific; 11875093) with 10% human serum (Australian Red Cross), 1% antibiotic-antimycotic (ThermoFisher Scientific; 15240062) and seeded in 24-well plate (∼0.25 × 106 cells/well) and left to recover overnight at 37°C with 5% CO2 in a humidified atmosphere. Next day, the cells were washed twice with PBS. The adherent monocytes were cultured in complete RPMI with recombinant human (rh) GM-CSF (50 ng/ml) (BioLegend; 572903) for 6 days to allow macrophage differentiation (Manéglier et al., 2009; Gray et al., 2013).
2.5 Induction and treatments
PMA-differentiated THP-1 macrophages were stimulated with LPS from E. coli 0111:B4 (Sigma-Aldrich; L2630) at 1 μg/ml with or without exposure to plant extracts at the final concentration of 25 μg/ml in DMSO 0.025%. Controls include dexamethasone (Sigma–Aldrich; D4902-100 MG) at 127.5 nM and cells without treatment. Following 24 h of induction, supernatants were centrifuged at 1,200 rpm for 7 min at 4°C and one part was used for cytotoxicity assay while the other was conserved at −20°C until cytokines quantification. Experiments were performed three times with 3 replicates for each condition. MDMs were washed twice with PBS to remove rhGM-CSF and then induced with LPS from E. coli O55:B5 (Sigma-Aldrich; L6529-1 MG) at 20 ng/ml during 48 h in presence or not of plant extracts at the final concentrations of 10 μg/ml, 25 μg/ml or 50 μg/ml in DMSO 0.05%. Experiments were reproduced three times with at least 2 replicates for each condition. After treatments, supernatants were collected and kept at −20°C for QUIN quantification.
2.6 Determination of cell mortality
Cytotoxicity was evaluated by quantifying the release of lactate dehydrogenase (LDH) in the culture supernatant that correlates with the amount of cell death and membrane damage, providing an accurate measurement of cellular toxicity (Haslam et al., 2000). For THP-1, LDH was quantified using the commercial CytoTox 96® Non-Radioactive Cytotoxicity Assay (Promega; G1780) following the manufacturer’s specifications. Absorbance at 450 nm (A450) was read using a microplate spectrophotometer (Multiskan™ FC, Thermo Fisher Scientific). LDH in the supernatants was normalized against absorbance obtained for total lysed cells, and results were expressed as percent of cytotoxicity (Sinyeue et al., 2022). For MDMs, LDH was quantified using the commercial LDH-Glo™ Cytotoxicity Assay (Promega; J2381) following the manufacturer’s specifications. The luminescent signal was read, using a luminescence mode of BMG CLARIOstar Plus plate reader. LDH in the supernatants of treatments was normalized against that obtained from negative control and results were expressed as percent of cytotoxicity.
2.7 Quantification of cytokines by ELISA
Cytokines production in supernatants was quantified according to the manufacturer’s instructions for human TNF-α, human IL-6, human IL-10 and human IL-1β (DuoSet, R&D Systems). Absorbance was measured at 570 nm and 450 nm on the microplate spectrophotometer (Multiskan ™ FC, Thermo Fisher Scientific). Afterward, the protein concentrations were calculating using the absorbance based on the regression equation with each cytokine standard and expressed as picograms per milliliter (pg/ml).
2.8 Quantification of QUIN by gas chromatography/mass spectrometry (GC/MS)
GC/MS was used to quantify QUIN concentrations in cell culture supernatants as previously described (Smythe et al., 2002; Guillemin et al., 2003b; Guillemin et al., 2007). 100 µL of the deproteinized sample were derivatized for quantification of QUIN using an Agilent 7890A GC system coupled with Agilent 5975C mass spectrometry detector and Agilent 7693 A autosampler (Agilent Technologies Inc., Santa Clara, CA). The separation of QUIN was carried out with a DB-5MS column, 0.25 µm film thickness, 0.25 mm × 30 m capillary column (Agilent Technologies Inc., Santa Clara, CA) for 12 min. Concentrations of QUIN were analyzed using Agilent GC/MSD ChemStation software (Edition 02.02.1431). A series of deuterated and non-deuterated standards for QUIN were used for a six-point calibration curve to interpolate the quantity of the sample readout.
2.9 Chemical fractionation
The cPE extract was first analyzed by HPLC/MS and the major compounds present in this fraction isolated by semi preparative HPLC to yield compounds (1) to (7). Dried extracts were solubilized in methanol at 10 mg/ml for analytical and semi preparative experiments, the injected volume being different, and filtered on a 0.45 μM PTFE filter before HPLC analysis. The cPE samples were analyzed by HPLC-UV-DAD (Waters 2695) at different wavelengths, using a RP Nucleodur C18ec column (250 by 4.6 mm, 5 μm particle size, Macherey Nagel) column and using water plus formic acid 0.05% as solvent A, and acetonitrile as solvent B plus formic acid 0.05%, and coupled with mass spectrometry (MS) using an ion trap Bruker Esquire HCT Ultra mass spectrometry instrument equipped with an electrospray ion source in positive and negative mode (data were viewed by using Hystar Bruker software). The analytical conditions were optimized to enhance the separation of the compounds present in this fraction. An isocratic method (solvent A: 20%, solvent 80B: %, flow rate: 1.5 ml min−1) was chosen. HPLC quality solvents were purchased from Fischer Chemicals (Leicestershire, United Kingdom).
2.10 Structural elucidation
Chemical structures were determined by 1D NMR 1H (600 MHz), 13C (150 MHz) and 2D homo using the NMR equipment from Brucker with the following parameters: 600 MHz Avance III, equipped with a 5 mm BBFO + probe, and hetero-coupling methods (COSY, HSQCed, HMBC and NOESY) in CDCl3 at 298 K and data were compared to those found in the literature. The numeration for the carbon atoms is shown in Figure 4 for coleon U (1) and is the classical one used for abietane diterpenes. The results obtained for 1H and 13C NMR are gathered in tables 2 and 3. δ in ppm (s: singlet, d: doublet, t: triplet, q: quadruplet, quint: quintuplet, sept: septuplet, m: multiplet, l: large, J in Hz, number of protons). Mass spectra were in accordance with the structures found (Table 1).
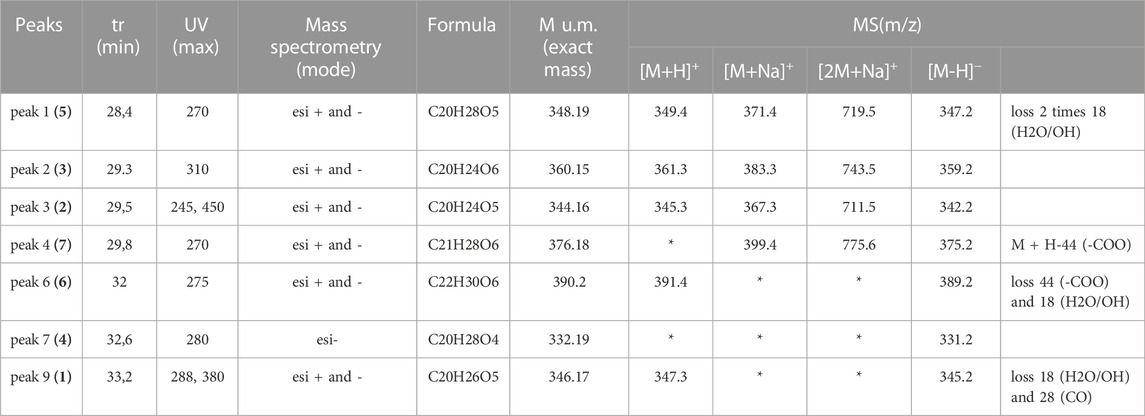
TABLE 1. Mass Spectrometry data for compounds (1) to (7) isolated from C. forsteri cyclohexane extract.
2.11 Statistical analyses
Results are provided as mean ± standard deviation (SD). Statistical analyses were performed using the software statistical package Prism 9.0 (GraphPad Software LLC, United States). Cytotoxicity and inhibitory effect on QUIN production were evaluated using a Kruskal-Wallis test followed by a Dunn’s multiple comparison test. Inhibitory response on cytokine production was evaluated using a Mann–Whitney non-parametric test to compare distribution between treatments and LPS induction. p values <0.05 were considered significant.
3 Results
3.1 Anti-inflammatory potential of C. forsteri extracts on LPS-induced cytokines in human THP-1 macrophages
To evaluate potential plant extract cytotoxicity on THP-1 cells, LDH was quantified after incubation with plant extracts at 25 μg/ml. No significant increase of cytotoxicity was observed between the LPS-induced cells with or without plant extract treatment, with cytotoxicity ranging from 18.53 ± 4.029% to 21.35 ± 5.938% (Figure 2A). Thus, concentration at 25 μg/ml for plant extract was confirmed for further studies. Incubation with cPE and ePE at 25 μg/ml decreased the production of LPS-induced TNF-α from 925.5 ± 341.1 pg/ml in LPS condition to 493.8 ± 113.9 and 417.6 ± 176.4 pg/ml when treated with cPE and ePE, respectively (Figure 2B). Similarly, plant extracts also decrease LPS-dependent IL-6 production (Figure 2C) compared to the LPS-treated cells. Results showed that plant extracts have different effects on IL-1β cytokine production depending on ethanolic or cyclohexane extracts. Only the ePE significantly reduced the production of IL-1β to 139.3 ± 16.99 pg/ml compared IL-1β level at 203.9 ± 57.45 pg/ml for LPS-treated cells (Figure 2D). In contrast, there was no significant effect on the production of LPS-dependent IL-10 production regardless the exposure of plant extract, with a concentration of cytokine ranging from 82.12 ± 17.09 to 130.6 ± 72.58 pg/ml (Figure 2E).
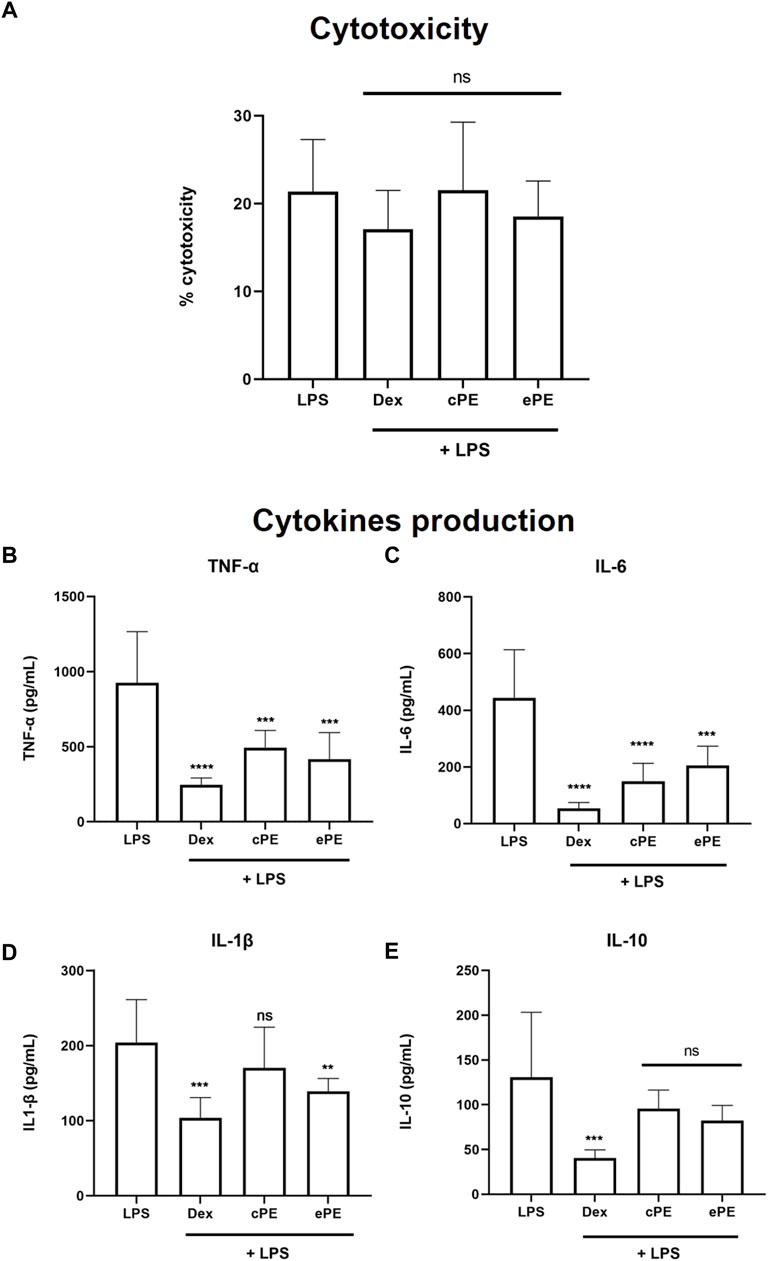
FIGURE 2. Inhibitory effect of C. forsteri extracts on cytokine production. Anti-inflammatory potential of C. forsteri cyclohexane (cPE) and ethanolic (ePE) plant extract at 25 μg/ml was evaluated on cytokines produced by PMA-THP-1 macrophages induced with LPS (1 μg/ml) for 24 h. Dexamethasone (DEX) at 127 nM was used as positive inhibitory control. Cytotoxicity of cPE and ePE was evaluated on LPS-induced PMA-THP-1 macrophages (A) as described in Materials and Methods. Cytokines IL-1β (B), IL-6 (C), IL-10 (D) and TNF-α (E) were quantified using ELISA technique. Mann-Whitney analyses were used for comparison between treatments compared to control or LPS condition. *, p < 0.05; **, p < 0.01; ***, p < 0.005; ****, p < 0.001; ns, non-significant.
3.2 Inhibitory effect of C. forsteri extracts on LPS-dependent quinolinic acid production in human primary MDMs
Cytotoxicity of C. forsteri ePE and cPE was analyzed on MDMs (Figure 3A) and results revealed no significant increase of cell mortality for ePE (from 14.33 ± 48.58 to 59.83 ± 40.41%) and cPE (from 41.50 ± 110.9 to 89.83 ± 89.20%) treatments compared to LPS-treated cells (103.5 ± 84.77%) and regardless of plant extract concentration. QUIN production was measured, and induction confirmed with LPS with a QUIN concentration at 1,574 ± 482.4 nM. Inhibitory effect on QUIN production (Figures 3B, C) was observed for both ePE (p-value = 0.02250) and cPE (p-value = 0.01761) extracts compared to LPS condition with significant activity for ePE at 10 μg/ml (QUIN concentration at 671.5 ± 325.5 nM) and cPE at 50 μg/ml (QUIN concentration at 441.2 ± 338.8 nM).
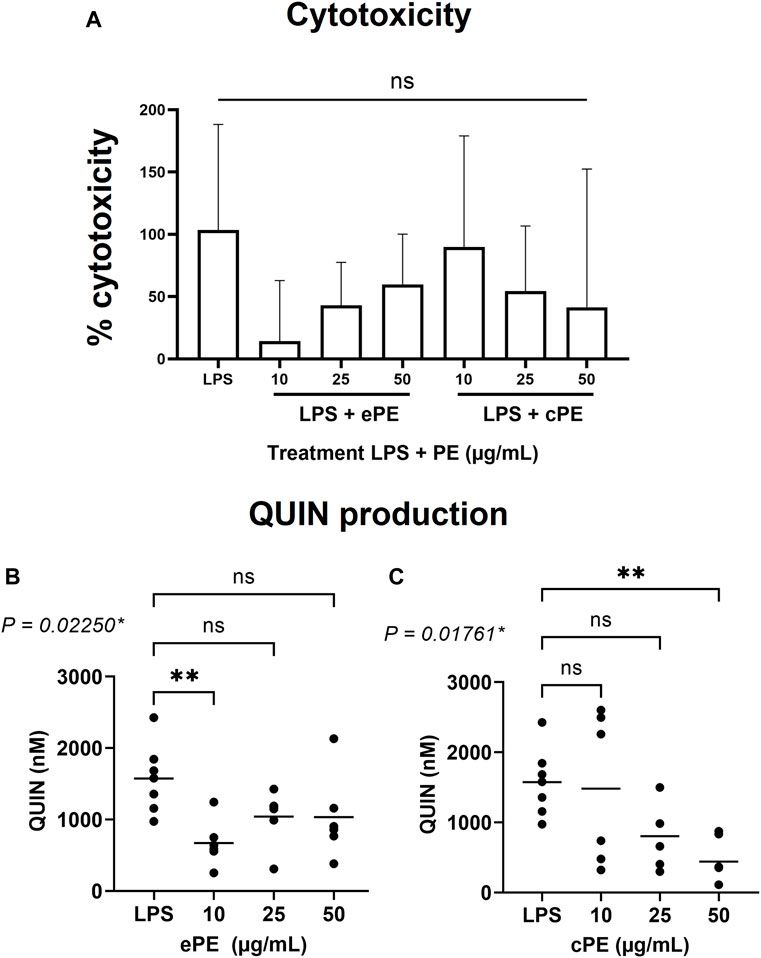
FIGURE 3. Inhibitory effect of C. forsteri extracts on QUIN production. Anti-inflammatory potential of C. forsteri cyclohexane (cPE) and ethanolic (ePE) plant extract at 10, 25 or 50 μg/ml were evaluated on QUIN produced by MDMs induced with LPS (20 ng/ml) for 48H. Cytotoxicity of cPE and ePE was evaluated on MDMs (A) as described in Materials and Methods. QUIN was quantified by GC/MS after treatment with ePE (B) and cPE (C) at various concentrations. Kruskal-Wallis test followed by a Dunn’s multiple comparison test were used for comparison between treatments compared to LPS condition. *, p < 0.05; **, p < 0.01; ns, non-significant.
4.3 Chemical profile of cyclohexane extract of C. forsteri leaf extract
Seven abietane diterpenes (Figure 4) were characterized from C. forsteri cPE: coleon U (1) (Toshio et al., 1977; Wellsow et al., 2006), coleon U-quinone (2) (Alder et al., 1984), 8α,9α-epoxycoleon U-quinone (3) (Alder et al., 1984), horminone or 7α-hydroxyroyleanone (4) (Hensch et al., 1975), 6β,7α-dihydroxyroyleanone (5) (Hensch et al., 1975), 7α-acetoxy-6β-hydroxyroyleanone (6) (Hensch et al., 1975) and 7α-formyloxy-6β-hydroxyroyleanone (7) (Toshio et al., 1977). Purity of isolated compounds was verified by HPLC (see Supplementary Material S1). The compounds (1) to (7) differ from the presence of several polar groups (alcohol, ketone, etc.,) brought in evidence by the loss of 18, 28 or 44 m. a.u in mass spectrometry (Table 1). The abietane skeleton is a 20 carbons diterpene consisting in 3 fused 6-membered rings that contains a methyl group at the C10, 2 methyl groups at the C4 and an isopropyl group at the C13 (Supplementary Table S1). These alkyl groups are common to the seven isolated compounds and evidenced by 1H NMR (Supplementary Table S2) showing characteristic signals (singlet for methyl, septuplet and two doublets for isopropyl).
4 Discussion
Plectranthus sensu lato is a large and widespread genus with an important range of ethnobotanical uses including medicinal purposes (Lukhoba et al., 2006; Rice et al., 2011). Phytochemical studies have shown that this genus, including Coleus species, is a rich source of diterpenes exerting promising biological activities with abietane diterpenes as the most abundant group (Abdel-Mogib et al., 2002; Gáborová et al., 2022). Interestingly, commercial extract of C. amboinicus was shown to inhibit maturation and release of inflammatory cytokine IL-1β through inhibition of nuclear factor NF-kB translocation (Leu et al., 2019). Formerly classified among Plectranthus genus, C. forsteri is a traditional plant used in the Pacific region especially in New Caledonia, to treat flu-like symptoms and shock-related ecchymosis (Rageau and Schmid, 1973; Suprin, 2008; Lormée et al., 2011). However, only few pharmacological studies have been undertaken on C. forsteri. Polyphenols and diterpenoids as well as bioactive caffeic acid esters nepetoidins A and B were found in leaf extract of C. forsteri (Grayer et al., 2003; Wellsow et al., 2006; Kubínová et al., 2013). Herein, our results showed that cyclohexane (cPE) and ethanolic (ePE) extracts of C. forsteri exert inhibitory activity on the levels of the inflammatory cytokines IL-6 and TNF-α and of the deleterious KP metabolite QUIN produced by human macrophages under inflammatory conditions. Composition of the cyclohexane extract was analyzed and 7 known abietane diterpenes were characterized: coleon U (1), coleon U-quinone (2), 8α,9α-epoxycoleon U-quinone (3), horminone or 7α-hydroxyroyleanone (4), 6β,7α-dihydroxyroyleanone (5), 7α-acetoxy-6β-hydroxyroyleanone (6) and 7α-formyloxy-6β-hydroxyroyleanone (7). Previously, nepetoidins A and B were isolated from a methanolic extract of C. forsteri while they were not found in cyclohexane extract in our study (Kubínová et al., 2013).
Coleon U (1) and coleon U-quinone (2) were previously isolated from C. forsteri validating our results (Wellsow et al., 2006). Both molecules showed antibacterial activity against phytopathogenic Pseudomonas syringae (Wellsow et al., 2006). Coleon U-quinone (2) purified from C. hadiensis (formerly P. madagascariensis) exerted antibacterial against pathogenic Staphylococcus aureus and Enterococcus faecalis while coleon U (1) isolated from C. grandidentatus native of Africa, showed interesting antimicrobial activity against methicillin-resistant S. aureus (MRSA) and vancomycin-resistant Enterococcus (VRE) (Gaspar-Marques et al., 2006; Kubínová et al., 2014). Coleon U (1) also showed antifungal activity on Bacillus subtilis (Wellsow et al., 2006). Coleon U (1) and coleon U-quinone (2) also exhibited inhibitory activity on human cancer cell line proliferation (Marques et al., 2002; Matias et al., 2019; Ntungwe et al., 2022). Interestingly, coleon U (1) isolated from C. grandidentatus also showed antiproliferative activity on human T- and B-cell (Cerqueira et al., 2004).
In this study, compound 8α,9α-epoxycoleon U-quinone (3) was newly isolated from C. forsteri. It was previously isolated from C. xanthanthus and showed cytotoxic activity toward human leukemia cells (Mei et al., 2002). Together with coleon U (1) and coleon U-quinone (2), 8α,9α-epoxycoleon U-quinone (3) was also found in C. mutabilis leaves and compounds (1–3) were shown to inhibit P-glycoprotein (P-gp) activity in lung cancer cell line (Ntungwe et al., 2022). Related to Multidrug Resistance (MDR), P-gp is a membrane transporter involved in immune response and induced during chronic inflammatory diseases as RA, IBD and SLE (Liu et al., 2015; Wu et al., 2018; Ahmed Juvale et al., 2022). It was shown to induce the release of cytokines from PBMCs treated with anti-inflammatory methotrexate and dexamethasone (Pawlik et al., 2005). Thus, inhibitory action of compounds (1–3) on P-gp might contribute to the anti-inflammatory activity of C. forsteri through the regulation of inflammatory cytokine production.
Horminone or 7α-hydroxyroyleanone (4) is a well-studied royleanone compound isolated from several Plectranthus s.l. Species exerting various bioactivities ranging from antitumoral and cytotoxic activities on various cancer cell lines to antimicrobial properties on MRSA and VRE as well as against Vibrio cholerae and Mycobacterium tuberculosis (Gáborová et al., 2022). Horminone (4) inhibitory effect was also studied on KP metabolites and was shown to slightly regulate IDO1 activity and KYN and TRP production (Becker et al., 2018). Thus, horminone (4) might contribute to the C. forsteri inhibitory activity on QUIN production through the regulation of IDO1 enzymatic activity.
Herein, 6β,7α-dihydroxyroyleanone (5) is newly isolated from C. forsteri while 7α-acetoxy-6β-hydroxyroyleanone (6) was previously isolated from C. forsteri methanolic extract (Kubínová et al., 2013). Both compounds exert antimicrobial activity as well as cytotoxicity and inhibitory effect on the growth of human cancer cell lines (Marques et al., 2002; Gaspar-Marques et al., 2006; Matias et al., 2019; Gáborová et al., 2022). It is noticeable that 7α-acetoxy-6β-hydroxyroyleanone (6) was shown to yield a potent antiproliferative activity against human lymphocytes with higher efficacy than the immunosuppressor cyclosporin (Cerqueira et al., 2004). Interestingly, compound (5) and synthetic royleanone derivatives of (5) and (6) were shown to inhibit P-gp (Garcia et al., 2020).
The last compound 7α-formyloxy-6β-hydroxyroyleanone (7) is newly isolated from C. forsteri. It was previously found in other Coleus species (Gáborová et al., 2022). However, bioactivities of compound (7) were barely studied. No antimicrobial activity was reported and low cytotoxicity on cancer cell line was reported (Matias et al., 2019; Gáborová et al., 2022).
Antibacterial activities of abietane diterpenes (1), (2), (4), (5) and (6) could participate in biological properties of C. forsteri during infection to clear microbial pathogens while anti-inflammatory potential could be related to compounds (1), (3), (4), (5) and (6) through the regulation KP and/or of P-gp production. Altogether, our results enhance biological properties of C. forsteri highlighting the beneficial use of its extracts in the Pacific traditional remedies.
Data availability statement
The original contributions presented in the study are included in the article/Supplementary Material, further inquiries can be directed to the corresponding author.
Author contributions
Conceptualization: MM, MoN, EH, and GG; experiments on MDM: SC; experiments on THP-1: ML and KH; chemical extraction and analyses: MNi, CA; figures and legends: MM, CA, and MNi; validation and data analysis: SC, MNi, ML, and MM; statistical analysis: MM; supervision and project administration: MM; funding acquisition and resources: MM; initial draft preparation: MM, writing Mat/Met: MM, ML, MNi, and CA; review and Editing: all authors; English editing: GG and SC. All authors have read and agreed to the published version of the manuscript.
Funding
This research was funded by the “Fonds de cooperation économique, sociale et Culturelle pour le Pacifique” of “Fonds Pacifique” (Project 1707, N° SPP 28-1-2017) supported by the French Ministry of Foreign Affairs, the French Ministry of Higher Education, Research and Innovation (MESRI), the Institut Pasteur of New Caledonia (IPNC) and the Pasteur Network. Prof Guillemin is supported by the National Health and Medical Research Council (NHMRC), Fellowship #APP1176660, and by Macquarie University. MM position is financed by the Government of New Caledonia.
Acknowledgments
The authors are thankful to Pierre CABAILLON for sharing initial information on traditional plants used in New Caledonia and for the selecting process of plants of interest. We are thankful to Gaëtan HERBETTE from the analytical platform Spectropole, FR1739, at Aix-Marseille University, for NMR experiments. We also thank Marie-Claude QAENG and Julie CAGLIERO.
Conflict of interest
The authors declare that the research was conducted in the absence of any commercial or financial relationships that could be construed as a potential conflict of interest.
Publisher’s note
All claims expressed in this article are solely those of the authors and do not necessarily represent those of their affiliated organizations, or those of the publisher, the editors and the reviewers. Any product that may be evaluated in this article, or claim that may be made by its manufacturer, is not guaranteed or endorsed by the publisher.
Supplementary material
The Supplementary Material for this article can be found online at: https://www.frontiersin.org/articles/10.3389/fphar.2022.1081310/full#supplementary-material
References
Abdel-Mogib, M., Albar, H. A., and Batterjee, S. M. (2002). Chemistry of the genus Plectranthus. Mol. A J. Synthetic Chem. Nat. Prod. Chem. 7 (2), 271–301. doi:10.3390/70200271
Agrawal, M., Shah, S., Patel, A., Pinotti, R., Colombel, J. F., and Burisch, J. (2019). Changing epidemiology of immune-mediated inflammatory diseases in immigrants: A systematic review of population-based studies. J. Autoimmun. 105, 102303. doi:10.1016/j.jaut.2019.07.002
Ahmed Juvale, I. I., Abdul Hamid, A. A., Abd Halim, K. B., and Che Has, A. T. (2022). P-Glycoprotein: New insights into structure, physiological function, regulation and alterations in disease. Heliyon 8 (6), e09777. doi:10.1016/j.heliyon.2022.e09777
Alatab, S., Sepanlou, S. G., Ikuta, K., Vahedi, H., Bisignano, C., Safiri, S., et al. (2020). The global, regional, and national burden of inflammatory bowel disease in 195 countries and territories, 1990–2017: A systematic analysis for the global burden of disease study 2017. Lancet Gastroenterology Hepatology 5 (1), 17–30. doi:10.1016/S2468-1253(19)30333-4
Alder, A. C., Rüdi, P., and Eugster, H. (1984). Drüsenfarbstoffe aus Labiaten: Die polaren Diterpenoide aus Plectranthus argentatus S. T. BLAKE. Helvetica Chim. Acta 67 (6), 1523–1530. doi:10.1002/hlca.19840670616
Athale, J., Busch, L. M., and O'Grady, N. P. (2022). Cytokine release syndrome and sepsis: Analogous clinical syndromes with distinct causes and challenges in management. Infect. Dis. Clin. North Am. 36 (4), 735–748. doi:10.1016/j.idc.2022.07.001
Baker, K. F., and Isaacs, J. D. (2018). Novel therapies for immune-mediated inflammatory diseases: What can we learn from their use in rheumatoid arthritis, spondyloarthritis, systemic lupus erythematosus, psoriasis, Crohn's disease and ulcerative colitis? Ann. Rheum. Dis. 77 (2), 175–187. doi:10.1136/annrheumdis-2017-211555
Becker, K., Schwaiger, S., Waltenberger, B., Fuchs, D., Pezzei, C. K., Schennach, H., et al. (2018). Immunomodulatory effects of diterpene quinone derivatives from the roots of horminum pyrenaicum in human PBMC. Oxid. Med. Cell Longev. 2018, 2980295. doi:10.1155/2018/2980295
Bhat, A., Tan, V., Heng, B., Chow, S., Basappa, S., Essa, M. M., et al. (2021). Papaverine, a phosphodiesterase 10A inhibitor, ameliorates quinolinic acid-induced synaptotoxicity in human cortical neurons. Neurotox. Res. 39 (4), 1238–1250. doi:10.1007/s12640-021-00368-4
Bourdy, G., Cabalion, P., Amade, P., and Laurent, D. (1992). Traditional remedies used in the Western Pacific for the treatment of ciguatera poisoning. J. Ethnopharmacol. 36 (2), 163–174. doi:10.1016/0378-8741(92)90017-l
Cerqueira, F., Cordeiro-Da-Silva, A., Gaspar-Marques, C., Simões, F., Pinto, M. M. M., and Nascimento, M. S. J. (2004). Effect of abietane diterpenes from Plectranthus grandidentatus on T- and B-lymphocyte proliferation. Bioorg. Med. Chem. 12 (1), 217–223. doi:10.1016/j.bmc.2003.10.006
Chen, S., Corteling, R., Stevanato, L., and Sinden, J. (2012). Natural inhibitors of indoleamine 3, 5-dioxygenase induced by interferon-gamma in human neural stem cells. Biochem. Biophys. Res. Commun. 429 (1-2), 117–123. doi:10.1016/j.bbrc.2012.10.009
El-Gabalawy, H., Guenther, L. C., and Bernstein, C. N. (2010). Epidemiology of immune-mediated inflammatory diseases: Incidence, prevalence, natural history, and comorbidities. J. Rheumatol. Suppl. 85, 2–10. doi:10.3899/jrheum.091461
Gáborová, M., Šmejkal, K., and Kubínová, R. (2022). Abietane diterpenes of the genus Plectranthus sensu lato. Molecules 27 (1), 166. doi:10.3390/molecules27010166
Garcia, C., Isca, V. M. S., Pereira, F., Monteiro, C. M., Ntungwe, E., Sousa, F., et al. (2020). Royleanone derivatives from Plectranthus spp. as a novel class of P-glycoprotein inhibitors. Front. Pharmacol. 11, 557789. doi:10.3389/fphar.2020.557789
Gaspar-Marques, C., Rijo, P., Simões, M. F., Duarte, M. A., and Rodriguez, B. (2006). Abietanes from Plectranthus grandidentatus and P. hereroensis against methicillin- and vancomycin-resistant bacteria. Phytomedicine 13 (4), 267–271. doi:10.1016/j.phymed.2005.06.002
Gautam, R., and Jachak, S. M. (2009). Recent developments in anti-inflammatory natural products. Med. Res. Rev. 29 (5), 767–820. doi:10.1002/med.20156
Ghasemian, M., Owlia, S., and Owlia, M. B. (2016). Review of anti-inflammatory herbal medicines. Adv. Pharmacol. Sci. 2016, 9130979. doi:10.1155/2016/9130979
Gray, L. R., Tachedjian, G., Ellett, A. M., Roche, M. J., Cheng, W. J., Guillemin, G. J., et al. (2013). The NRTIs lamivudine, stavudine and zidovudine have reduced HIV-1 inhibitory activity in astrocytes. PLoS One 8 (4), e62196. doi:10.1371/journal.pone.0062196
Grayer, R. J., Eckert, M. R., Veitch, N. C., Kite, G. C., Marin, P. D., Kokubun, T., et al. (2003). The chemotaxonomic significance of two bioactive caffeic acid esters, nepetoidins A and B, in the Lamiaceae. Phytochemistry 64 (2), 519–528. doi:10.1016/s0031-9422(03)00192-4
Guillemin, G. J., Brew, B. J., Noonan, C. E., Knight, T. G., Smythe, G. A., and Cullen, K. M. (2007). Mass spectrometric detection of quinolinic acid in microdissected Alzheimer's disease plaques. Int. Congr. Ser. 1304, 404–408. doi:10.1016/j.ics.2007.07.012
Guillemin, G. J., Croitoru-Lamoury, J., Dormont, D., Armati, P. J., and Brew, B. J. (2003a). Quinolinic acid upregulates chemokine production and chemokine receptor expression in astrocytes. Glia 41 (4), 371–381. doi:10.1002/glia.10175
Guillemin, G. J., Smith, D. G., Smythe, G. A., Armati, P. J., and Brew, B. J. (2003b). Expression of the kynurenine pathway enzymes in human microglia and macrophages. Adv. Exp. Med. Biol. 527, 105–112. doi:10.1007/978-1-4615-0135-0_12
Guillemin, G. J., Smythe, G. A., Veas, L. A., Takikawa, O., and Brew, B. J. (2003c). A beta 1-42 induces production of quinolinic acid by human macrophages and microglia. Neuroreport 14 (18), 2311–2315. doi:10.1097/00001756-200312190-00005
Haslam, G., Wyatt, D., and Kitos, P. A. (2000). Estimating the number of viable animal cells in multi-well cultures based on their lactate dehydrogenase activities. Cytotechnology 32 (1), 63–75. doi:10.1023/a:1008121125755
Hensch, M., Rüedi, P., and Eugster, C. H. (1975). Horminon, Taxochinon und weitere Royleanone aus 2 abessinischen Plectranthus-Spezies (Labiatae). Helvetica Chim. Acta 58 (7), 1921–1934. doi:10.1002/hlca.19750580707
Hoffmann, M., Schwertassek, U., Seydel, A., Weber, K., Hauschildt, S., and Lehmann, J. (2017). Therapeutic efficacy of a combined sage and bitter apple phytopharmaceutical in chronic DSS-induced colitis. Sci. Rep. 7 (1), 14214. doi:10.1038/s41598-017-13985-x
Huang, M., Cai, S., and Su, J. (2019). The pathogenesis of sepsis and potential therapeutic targets. Int. J. Mol. Sci. 20 (21), 5376. doi:10.3390/ijms20215376
Jones, S. P., Franco, N. F., Varney, B., Sundaram, G., Brown, D. A., de Bie, J., et al. (2015). Expression of the kynurenine pathway in human peripheral blood mononuclear cells: Implications for inflammatory and neurodegenerative disease. PLoS One 10 (6), e0131389. doi:10.1371/journal.pone.0131389
Kondo, T., and Amano, K. (2018). Era of steroid sparing in the management of immune-mediated inflammatory diseases. Immunol. Med. 41 (1), 6–11. doi:10.1080/09114300.2018.1451593
Kubínová, R., Pořízková, R., Navrátilová, A., Farsa, O., Hanáková, Z., Bačinská, A., et al. (2014). Antimicrobial and enzyme inhibitory activities of the constituents of Plectranthus madagascariensis (Pers.) Benth. J. Enzyme Inhib. Med. Chem. 29 (5), 749–752. doi:10.3109/14756366.2013.848204
Kubínová, R., Švajdlenka, E., Schneiderová, K., Hanáková, Z., Dall'Acqua, S., and Farsa, O. (2013). Polyphenols and diterpenoids from Plectranthus forsteri ‘marginatus. Biochem. Syst. Ecol. 49, 39–42. doi:10.1016/j.bse.2013.03.029
Kuek, A., Hazleman, B. L., and Ostor, A. J. (2007). Immune-mediated inflammatory diseases (IMIDs) and biologic therapy: A medical revolution. Postgrad. Med. J. 83 (978), 251–260. doi:10.1136/pgmj.2006.052688
Leu, W. J., Chen, J. C., and Guh, J. H. (2019). Extract from Plectranthus amboinicus inhibit maturation and release of interleukin 1β through inhibition of NF-κB nuclear translocation and NLRP3 inflammasome activation. Front. Pharmacol. 10, 573. doi:10.3389/fphar.2019.00573
Liu, J., Zhou, F., Chen, Q., Kang, A., Lu, M., Liu, W., et al. (2015). Chronic inflammation up-regulates P-gp in peripheral mononuclear blood cells via the STAT3/Nf-κb pathway in 2, 4, 6-trinitrobenzene sulfonic acid-induced colitis mice. Sci. Rep. 5 (1), 13558. doi:10.1038/srep13558
Lormée, N., Cabalion, P., and Hnawia, E. (2011). Hommes et plantes de Maré: Îles Loyauté, Nouvelle-Calédonie. Marseille: IRD Éditions.
Lovelace, M. D., Varney, B., Sundaram, G., Franco, N. F., Ng, M. L., Pai, S., et al. (2016). Current evidence for a role of the kynurenine pathway of tryptophan metabolism in multiple sclerosis. Front. Immunol. 7, 246. doi:10.3389/fimmu.2016.00246
Lukhoba, C. W., Simmonds, M. S., and Paton, A. J. (2006). Plectranthus: A review of ethnobotanical uses. J. Ethnopharmacol. 103 (1), 1–24. doi:10.1016/j.jep.2005.09.011
Luo, C., Zou, L., Sun, H., Peng, J., Gao, C., Bao, L., et al. (2020). A review of the anti-inflammatory effects of rosmarinic acid on inflammatory diseases. Front. Pharmacol. 11, 153. doi:10.3389/fphar.2020.00153
Manéglier, B., Malleret, B., Guillemin, G. J., Spreux-Varoquaux, O., Devillier, P., Rogez-Kreuz, C., et al. (2009). Modulation of indoleamine-2, 3-dioxygenase expression and activity by HIV-1 in human macrophages. Fundam. Clin. Pharmacol. 23 (5), 573–581. doi:10.1111/j.1472-8206.2009.00703.x
Marques, C. G., Pedro, M., Simões, M. F., Nascimento, M. S., Pinto, M. M., and Rodríguez, B. (2002). Effect of abietane diterpenes from Plectranthus grandidentatus on the growth of human cancer cell lines. Planta Med. 68 (9), 839–840. doi:10.1055/s-2002-34407
Matias, D., Nicolai, M., Saraiva, L., Pinheiro, R., Faustino, C., Diaz Lanza, A., et al. (2019). Cytotoxic activity of royleanone diterpenes from Plectranthus madagascariensis benth. ACS Omega 4 (5), 8094–8103. doi:10.1021/acsomega.9b00512
McInnes, I. B., and Gravallese, E. M. (2021). Immune-mediated inflammatory disease therapeutics: Past, present and future. Nat. Rev. Immunol. 21 (10), 680–686. doi:10.1038/s41577-021-00603-1
Mei, S. X., Jiang, B., Niu, X. M., Li, M. L., Yang, H., Na, Z., et al. (2002). Abietane diterpenoids from Coleus xanthanthus. J. Nat. Prod. 65 (5), 633–637. doi:10.1021/np0102049
Modoux, M., Rolhion, N., Mani, S., and Sokol, H. (2021). Tryptophan metabolism as a pharmacological target. Trends Pharmacol. Sci. 42 (1), 60–73. doi:10.1016/j.tips.2020.11.006
Ng, S. C., Shi, H. Y., Hamidi, N., Underwood, F. E., Tang, W., Benchimol, E. I., et al. (2017). Worldwide incidence and prevalence of inflammatory bowel disease in the 21st century: A systematic review of population-based studies. Lancet 390 (10114), 2769–2778. doi:10.1016/s0140-6736(17)32448-0
Ntungwe, E. N., Stojanov, S. J., Duarte, N. M., Candeias, N. R., Díaz-Lanza, A. M., Vágvölgyi, M., et al. (2022). C(20)-nor-Abietane and three abietane diterpenoids from Plectranthus mutabilis leaves as P-glycoprotein modulators. ACS Med. Chem. Lett. 13 (4), 674–680. doi:10.1021/acsmedchemlett.1c00711
Paton, A. J., Mwanyambo, M., Govaerts, R. H. A., Smitha, K., Suddee, S., Phillipson, P. B., et al. (2019). Nomenclatural changes in Coleus and Plectranthus (Lamiaceae): A tale of more than two genera. PhytoKeys 129, 1–158. doi:10.3897/phytokeys.129.34988
Paton, A., Mwanyambo, M., and Culham, A. (2018). Phylogenetic study of Plectranthus, Coleus and allies (Lamiaceae): Taxonomy, distribution and medicinal use. Botanical J. Linn. Soc. 188 (4), 355–376. doi:10.1093/botlinnean/boy064
Pawlik, A., Baśkiewicz-Masiuk, M., Machaliński, B., Safranow, K., and Gawrońska-Szklarz, B. (2005). Involvement of P-glycoprotein in the release of cytokines from peripheral blood mononuclear cells treated with methotrexate and dexamethasone. J. Pharm. Pharmacol. 57 (11), 1421–1425. doi:10.1211/jpp.57.11.0007
Pires, A. S., Sundaram, G., Heng, B., Krishnamurthy, S., Brew, B. J., and Guillemin, G. J. (2022). Recent advances in clinical trials targeting the kynurenine pathway. Pharmacol. Ther. 236, 108055. doi:10.1016/j.pharmthera.2021.108055
Quaresma, A. B., Damiao, A. O. M. C., Coy, C. S. R., Magro, D. O., Hino, A. A. F., Valverde, D. A., et al. (2022). Temporal trends in the epidemiology of inflammatory bowel diseases in the public healthcare system in Brazil: A large population-based study. Lancet Regional Health – Am. 13, 100298. doi:10.1016/j.lana.2022.100298
Rice, L. J., Brits, G., Potgieter, C., and staden, V. (2011). Plectranthus: A plant for the future? South Afr. J. Bot. 77, 947–959. doi:10.1016/j.sajb.2011.07.001
Schwende, H., Fitzke, E., Ambs, P., and Dieter, P. (1996). Differences in the state of differentiation of THP-1 cells induced by phorbol ester and 1, 25-dihydroxyvitamin D3. J. Leukoc. Biol. 59 (4), 555–561. doi:10.1002/jlb.59.4.555
Singh, R., Yen, E., and Wu, M. (2020). Burden of immune-mediated inflammatory diseases (IMID): Race/ethnicity, sex, and geographic variation in the United States [abstract]. Arthritis Rheumatol. 72 (10).
Sinyeue, C., Matsui, M., Oelgemöller, M., Bregier, F., Chaleix, V., Sol, V., et al. (2022). Synthesis and investigation of flavanone derivatives as potential new anti-inflammatory agents. Molecules 27 (6), 1781. doi:10.3390/molecules27061781
Smythe, G. A., Braga, O., Brew, B. J., Grant, R. S., Guillemin, G. J., Kerr, S. J., et al. (2002). Concurrent quantification of quinolinic, picolinic, and nicotinic acids using electron-capture negative-ion gas chromatography-mass spectrometry. Anal. Biochem. 301 (1), 21–26. doi:10.1006/abio.2001.5490
Sundaram, G., Bessede, A., Gilot, D., Staats Pires, A., Sherman, L. S., Brew, B. J., et al. (2022). Prophylactic and therapeutic effect of kynurenine for experimental autoimmune encephalomyelitis (EAE) disease. Int. J. Tryptophan Res. 15, 11786469221118657. doi:10.1177/11786469221118657
Suprin, B. (2008). Plantes du littoral en nouvelle-calédonie: Arbres et autres ligneux, herbes et autres non ligneaux, lianes et sarmenteux. Noumea: Editions Photosynthèse.
Toshio, M., Peter, R., and Eugster, C. H. (1977). Diterpenoide Drüsenfarbstoffe aus Labiaten: Coleone U, V, W und 14-O-Formyl-coleon-V sowie 2 Royleanone ausPlectranthus myrianthusBRIQ.;cis-undtrans-A/B-6, 7-Dioxoroyleanon. Helvetica Chim. Acta 60 (8), 2770–2779. doi:10.1002/hlca.19770600830
Wellsow, J., Grayer, R. J., Veitch, N. C., Kokubun, T., Lelli, R., Kite, G. C., et al. (2006). Insect-antifeedant and antibacterial activity of diterpenoids from species of Plectranthus. Phytochemistry 67 (16), 1818–1825. doi:10.1016/j.phytochem.2006.02.018
Wongrakpanich, S., Wongrakpanich, A., Melhado, K., and Rangaswami, J. (2018). A comprehensive review of non-steroidal anti-inflammatory drug use in the elderly. Aging Dis. 9 (1), 143–150. doi:10.14336/ad.2017.0306
Wu, S., Pang, Y., He, Y., Zhang, X., Peng, L., Guo, J., et al. (2021). A comprehensive review of natural products against atopic dermatitis: Flavonoids, alkaloids, terpenes, glycosides and other compounds. Biomed. Pharmacother. 140, 111741. doi:10.1016/j.biopha.2021.111741
Wu, Y. J., Wang, C., and Wei, W. (2018). The effects of DMARDs on the expression and function of P-gp, MRPs, BCRP in the treatment of autoimmune diseases. Biomed. Pharmacother. 105, 870–878. doi:10.1016/j.biopha.2018.06.015
Zeng, L., Yang, T., Yang, K., Yu, G., Li, J., Xiang, W., et al. (2022). Efficacy and safety of curcumin and curcuma longa extract in the treatment of arthritis: A systematic review and meta-analysis of randomized controlled trial. Front. Immunol. 13, 891822. doi:10.3389/fimmu.2022.891822
Glossary
CD Crohn’s disease
cPE cyclohexane plant extract
DMSO dimethyl sulfoxide
ePE ethanolic plant extract
EtOH ethanol
FBS fetal bovine serum
GC/MS gas chromatography/mass spectrometry
GM-CSF granulocyte-macrophage colony-stimulating factor
HEPES 4-(2-hydroxyethyl)-1-piperazineethanesulfonic acid
IBD inflammatory bowel disease
IDO1 2,3-dioxygenase
IFNs interferons
ILs interleukins
IMIDs immune-mediated inflammatory diseases
IPNC Institut Pasteur of New Caledonia
KP kynurenine pathway
KYN kynurenine
LDH lactate dehydrogenase
LPS lipopolysaccharide
MDMs monocyte-derived macrophages
MRSA methicillin-resistant Staphylococcus aureus
MS mass spectrometry
NSAIDs non-steroidal anti-inflammatory drugs
PBMCs peripheral blood mononuclear cells
P-gp P-glycoprotein
PMA phorbol 12-myristate 13-acetate
QUIN quinolinic acid
RA rheumatoid arthritis
RPMI Roswell Park Memorial Institute
SD standard deviation
SLE systemic lupus erythematosus
SpA spondyloarthritis
TNF tumor necrosis factor
TRP tryptophan
UC ulcerative colitis
UNC University of New Caledonia
VRE vancomycin-resistant Enterococcus
Keywords: Coleus forsteri, traditional plant, anti-inflammatory, macrophage, cytokine, quinolinic acid (QUIN), abietane, diterpene
Citation: Nicolas M, Lasalo M, Chow S, Antheaume C, Huet K, Hnawia E, Guillemin GJ, Nour M and Matsui M (2023) Anti-inflammatory activities of Coleus forsteri (formerly Plectranthus forsteri) extracts on human macrophages and chemical characterization. Front. Pharmacol. 13:1081310. doi: 10.3389/fphar.2022.1081310
Received: 27 October 2022; Accepted: 22 December 2022;
Published: 09 January 2023.
Edited by:
Ali El Halawany, Cairo University, EgyptReviewed by:
Nunziatina De Tommasi, University of Salerno, ItalyPatrícia Mendonça Rijo, Universidade Lusófona, Portugal
Copyright © 2023 Nicolas, Lasalo, Chow, Antheaume, Huet, Hnawia, Guillemin, Nour and Matsui. This is an open-access article distributed under the terms of the Creative Commons Attribution License (CC BY). The use, distribution or reproduction in other forums is permitted, provided the original author(s) and the copyright owner(s) are credited and that the original publication in this journal is cited, in accordance with accepted academic practice. No use, distribution or reproduction is permitted which does not comply with these terms.
*Correspondence: Mariko Matsui, bW1hdHN1aUBwYXN0ZXVyLm5j
†These authors have contributed equally to this work and share first authorship.
‡These authors have contributed equally to this work and share last authorship.
§These authors have contributed equally to this work