- 1State Key Laboratory of Functions and Applications of Medicinal Plants & School of Pharmacy, Guizhou Medical University, Guiyang, China
- 2Microbiology and Biochemical Pharmaceutical Engineering Research Center of Guizhou Provincial Department of Education, Guizhou Medical University, Guiyang, China
- 3Experimental Animal Center of Guizhou Medical University, Guiyang, China
- 4Guizhou Provincial Engineering Research Center of Food Nutrition and Health, Guizhou Medical University, Guiyang, China
Hyperlipidemia endangers human health and has become a significant public health problem. This study aimed to investigate the mechanism of the hypolipidemic effects of Fermented Rosa roxburghii Tratt juice (FRRT) on hyperlipidemic rats and a new hypolipidemic intervention strategy was disclosed. The study revealed 12 weeks FRRT treatment significantly decreased the body weight, total cholesterol (TC), triacylglycerol (TG), low-density lipoprotein cholesterol (LDL-c), while high-density lipoprotein cholesterol (HDL-c) increased. We integrated the 16S rDNA sequencing and metabolomic profiling to evaluate the changes in the gut microbiota and metabolites. Significant changes in microbial composition accompanied marked changes in 56 feces metabolites. The results showed that FRRT could decrease the ratio of Firmicutes to Bacteroidetes, while increase the abundance of some bacterial genera (Prevotella, Paraprevotellaceae_Prevotella, Ruminococcus, Oscillospira). Metabolomics analysis displayed that the metabolisms of bile acid, amino acid and lipid were significantly affected by FRRT. Correlation analysis suggest that the reductions in serum lipids by FRRT are associated with the gut microbial community and their associated metabolites (amino acid metabolites, bile acid metabolites, and lipid metabolites). This study confirmed FRRT could be used as a new dietary and therapeutic strategy to dyslipidemia by improving the gut microbiota dysbiosis, metabolomic disorders and regulating the dyslipidemia. Our study also extended the understanding of the relationship between gut microbiota, metabolites, and lipid-lowering functions.
Introduction
Hyperlipidemia is a systemic disorder of lipid metabolism, including elevated levels of total cholesterol (TC), triglycerides (TG), low-density lipoprotein cholesterol (LDL-C) and a decreased level of high-density lipoprotein cholesterol (HDL-C). Hyperlipidemia can lead to a series of cardiovascular diseases such as coronary heart disease, cerebral thrombosis, and atherosclerosis (Abliz et al., 2014; Chen et al., 2014; Pirillo et al., 2021). With the improvement of living conditions, the excessive intake of high-fat diets, and the lack of exercise, results in more and more lipids accumulated in the body and the thus leading to Hyperlipidemia (Pirillo et al., 2021). To prevent and treat cardiovascular and cerebrovascular diseases could be by way of regulating lipid metabolism and reduce lipid levels. Therefore, it is important to explore new and effective intervention strategies for hyperlipidemia.
Studies have shown that gut microbiota can regulate the metabolism of the host of cholesterol and lipid, which is closely related to hyperlipidemia (Deng et al., 2018; Jin et al., 2018). The high-fat diet can affect the microenvironment of gut microbiota that significantly decrease the abundance of Bacteroides, while significantly increase the abundance of Firmicutes (Nguyen et al., 2017; Chen et al., 2019), leading to gut microbiota dysbiosis. The dysbiosis further disrupts lipid metabolism. which can form a vicious cycle and then causes hyperlipidemia. Some studies have shown that increasing the abundance of some beneficial bacteria could improve hyperlipidemia. Therefore, gut microbiota dysbiosis is closely related to hyperlipidemia, and gut microbiota might be a new target for the treatment of hyperlipidemia.
Bile acids (BAs) are the dominant downstream products of cholesterol catabolism, and include primary and secondary bile acids. Many studies point out that a high-fat diet may show a positive correlation with the production of secondary bile acids such as deoxycholic acid (DCA) and lithocholic acid (LCA), and evidence indicates that DCA and LCA are toxic bile acids associated with metabolic syndrome, and gastrointestinal diseases (Bernstein et al., 2006; Stenman et al., 2012; Stenman et al., 2013). Therefore, the production and excretion of BAs are critical for the maintenance of cholesterol homeostasis.
Rosa roxburghii Tratt (RRT), a medicinal plant and traditional food in southwest China, and included in the Quality Standards for Chinese and Ethnic Medicinal Herbs of Guizhou Province as ethnic medicinal herbs of Guizhou. RRT riches in organic acid, vitamin C, superoxide dismutase, flavonoids, polyphenols, polysaccharides and other active ingredients (Liu et al., 2016; Wang et al., 2021). In recent years, some studies have pointed out that Rosa roxburghii Tratt juice (RRTJ) has anti-tumour, anti-atherogenic, anti-oxidant, and anti-hyperlipidemic effects (Xu et al., 2019; Wang et al., 2021; Li et al., 2022). RRTJ also can affect the expression of proteins related to lipid metabolism to maintain metabolic disorders (Zhang et al., 2001; Lu and Bao, 2002; Song and Shen, 2020). Moreover, the extracted water-soluble polysaccharides from RRT fruit (RTFP) showed inhibitory activities against a-glucosidase and the potential to lower blood glucose, which also could reverse diabetes-induced gut microbiota dysbiosis (Huizhu Wang et al., 2018; Lei Wang et al., 2018; Hao Wang et al., 2019). The total polyphenols (PFs) from Rosa roxburghii Tratt showed modulating effects on hyperlipidemia and oxidative stress (Zhao et al., 2016; Wei et al., 2017; Lei Wang et al., 2019).
RRT contains large number of bioactive ingredients, while fresh RRT fruit is not suitable for long-term storage at room temperature. Processed RRT products by fermentation could maximize the preservation of active ingredients, and improve the taste and the flavor. At present, the mechanism of action of FRRT on hyper-lipidemia has not been explored in depth. Therefore, in this paper, we investigated the effect of FRRT on high-fat diet-induced hyperlipidemia, and unraveled the mechanism of action at the level of gut microbiota and metabolites in rats, to provide a deeper theory for FRRT as a new therapeutic strategy to prevent hyperlipidemia.
Materials and Methods
Preparation and Determination of FRRT
The FRRT (Batch Number:200715) was obtained from Guizhou Longshan Xiangfang Food Co., Ltd (Guizhou, China). The Angel active yeast BV818 was obtained from Angel Yeast Co., Ltd (Hubei, China). The fresh RRT fruit of “Guinong No.5” was selected and identified by the Key Laboratory of Natural Product Chemistry of the Chinese Academy of Sciences (Guizhou, China). FFRT was prepared as follows. After selection and cleaning, fresh RRT fruit was crushed and pressed to get RRT juice, and the RRTJ juice was filtered and reserved. Then the activated pectinase was added to RRT juice and macerated at 10°C for 16 h, and filtered. The filtered RRT juice was transferred to a temperature-controlled fermenter. The crystal sugar was added to adjust the sugar level to 200 g/L, inoculated with activated Angel active yeast BV818 200 mg/L, and fermented for 3 months. At last, FRRT was obtained by sterilization and filtration.
The contents of total polyphenols, total flavonoids, total polysaccharides were measured by UV spectrophotometry (Shimadzu, Japan), and the contents of the vitamin C were used by HPLC (Agilent, United States) (Tan et al., 2019; Guan et al., 2020; Lian et al., 2021).
Experimental Animals
Male Sprague-Dawley rats were obtained from the Laboratory Animal Center of Guizhou Medical University (Permit Number: SYXK Qian 2018-0001) and housed in an animal room (temperature: 23 ± 2°C; relative humidity = 60 ± 5%; 12 h light/12 h dark cycle). All animal experiments were performed according to protocols approved by the experimental animal ethics committee of Guizhou Medical University.
After 1 week of acclimation, twelve rats were fed a high-fat diet for 8 weeks to induce hyperlipidemia. Then, Rats were randomly divided into two groups (n = 6/group): high-fat diet (HFD) group and high-fat diet + FRRT (HFD + FRRT) group. In our previous experiments, we set up two different dose groups (5 ml/kg/day and 10 ml/kg/day), and we found that the treatment effect was better at the dose of 10 ml/kg/day. We chose the dose of 10 ml/kg/day for further experimental analysis (Supplementary Table S1). The HFD group was fed a high-fat diet for 12 weeks, and the HFD + FRRT group was fed a high-fat diet and gavaged with FRRT (10 ml/kg/day) for 12 weeks. The high-fat diet contains 79.8% basal feed, 18% lard, 0.2% sodium cholate, 2% cholesterol.
At the end of the treatment period, blood, feces, and Liver tissues were collected and processed for biochemical analysis, histological analysis, 16S rRNA gene sequence, and targeted metabolomics.
Biochemical Analysis
The blood samples were kept at room temperature for 1 hour and centrifuged at 10 min, 3000 rpm/min. The serum fraction was separated from each sample. Finally, the levels of triglyceride (TG), total cholesterol (TC), low-density lipoprotein cholesterol (LDL-c), high-density lipoprotein cholesterol (HDL-c), alanine aminotransferase (ALT), and aspartate aminotransferase (AST) in serum were measured by using commercial kits (Nanjing Jiancheng Bioengineering Institute, Nanjing, China).
Hematoxylin-Eosin Staining
The liver tissue was fixed in 10% paraformaldehyde for 24 h, embedded in paraffin, cut into 4-µm sections, and followed by hematoxylin-eosin (H&E) staining.
Gut Microbiota Analysis
The total bacterial DNA of each fecal sample was extracted from the fecal samples using OMEGA Soil DNA Kit (Omega Bio-Tek, Norcross, GA, United States). PCR amplification of the V3-V4 regions of 16S rRNA genes was performed using the bacterial primers 338F (5′-ACTCCTACGGGAGGCAGCA-3′) and 806R (5′-GGACTACHVGGGTWTCTAAT-3′), and sequencing was performed using the Illumina NovaSeq platform. Sequences were then quality filtered, denoised, merged and chimera removed using the DADA2 plugin, Sequence data analyses were mainly performed using QIIME2 (https://docs.qiime2.org/2019.4/tutorials/) and R packages (v3.2.0).
Metabolic Profiling Analysis
Targeted metabolomic analysis of stool samples was performed by Metabolo-Profile (Shanghai, China). Sample preparation and quantitative measurement were based on previously published methods (Xie et al., 2021). Briefly, 5 mg of lyophilized feces were homogenized with zirconium oxide beads, 25 µL of deionized water and 120 µL of methanol containing internal standard to extract the metabolites and then the supernatant was removed by centrifugation. The resulting supernatants were subjected to derivatization with 3-nitrophenylhydrazine (3-NPH) and N-(3-(dimethylamino)propyl)-N′-ethylcar-bodiimide (EDC)·HCl. Subsequently, the derivatized samples were analyzed by ultra-performance liquid chromatography coupled to a tandem mass spectrometry (UPLC-MS/MS) system (ACQUITY UPLC-Xevo TQ-S, Waters Corp, Milford, MA, United States). All of the standards were purchased from Sigma-Aldrich (St. Louis, MO, United States), Steraloids Inc (Newport, RI, United States) and TRC Chemicals (Toronto, ON, Canada).
Statistical Analysis
Significant differences between the two groups were analyzed by Student’s unpaired t-test using SPSS 23.0, and p < 0.05 was considered statistically significant. Raw fecal metabolome data files generated by UPLC-MS/MS were processed by Targeted Metabolome Batch Quantification (TMBQ) software (V1.0, HMI, Shenzhen, Guangdong, China). Pareto-scaled principal component analysis (PCA) and orthogonal partial least-squares discriminant analysis (OPLS-DA) were subjected to multivariate data analysis. OPLS-DA, PCA, and Z-Score heat map were analyzed by iMAP (V1.0, MetabO-Profile, Shanghai, China) platform.
Results and DISCUSSION
Chemical Composition of FRRT
The contents of total flavonoids were 13.49 ± 0.38 mg/mL, the total polysaccharides were 23.91 ± 0.26 mg/mL, the total polyphenols were 35.97 ± 0.41 mg/mL, and the vitamin C were 7.58 ± 0.10 mg/mL.
FRRT Improves Dyslipidemia in Hyperlipidemic Rats
The period of the experiment is 20 weeks, and the animal experiment procedure is shown in Figure 1A. At the end of the treatment, the bodyweight of rats with FRRT treatment significantly decreased compared with the HFD group (Figure 1B, p < 0.01). At the same time, rats with FRRT treatment exhibited lower levels of TC, TG, LDL-c, and a higher level of HDL-c compared with HFD rats (Figure 1C–F, p < 0.01), suggesting that FRRT could effectively ameliorate dyslipidemia in hyperlipidemia rats.
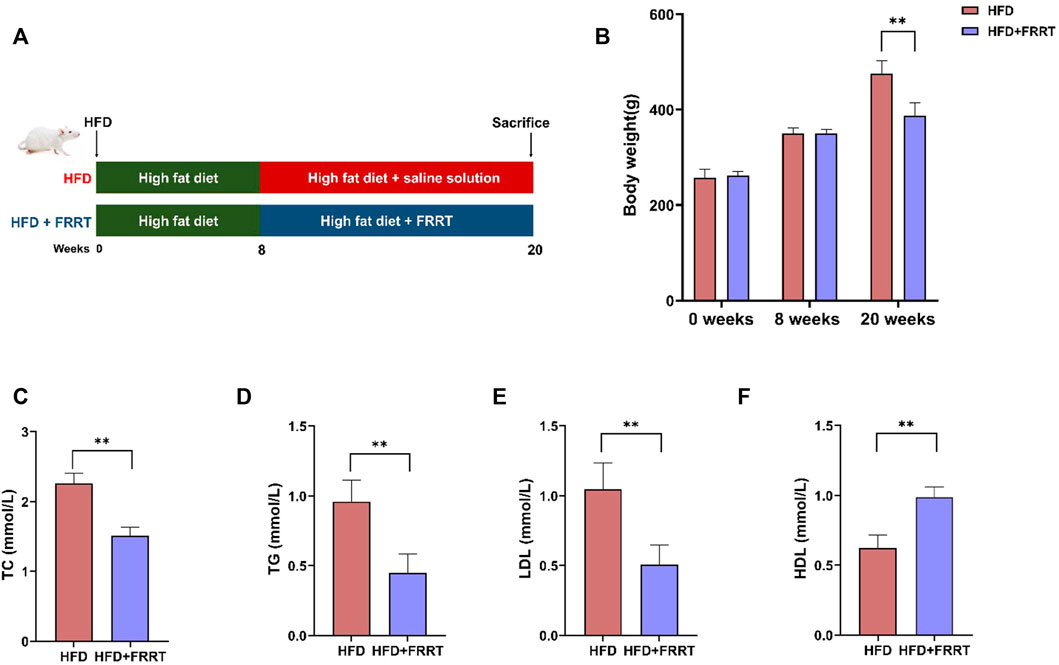
FIGURE 1. FRRT improves dyslipidemia in HFD-induced hyperlipidemic Rats. (A) Schematic diagram of FRRT treatment. (B) Rats’ body weight at weeks 0, 8, and 20. Serum TC (C), TG (D), LDL-c (E), and HDL-c (F) levels were measured using commercial kits. Data are presented as mean ± SEM (n = 6). *p < 0.05, **p < 0.01 compared with HFD group.
FRRT Improves Liver Function Damage in Hyperlipidemic Rats
We further analyzed the liver function damage in the HFD-induced hyperlipidemic Rats. Histological analysis showed that the structure of the hepatic lobule in hyperlipidemic rats is severely disturbed, the fat vacuoles occupy less than 3/4 of the entire field of view, and the fat vacuoles are fused to form a sheet. Some of the hepatocytes in the area where the fat vacuoles are concentrated are enlarged, with light cytoplasmic colorin. Some of the cells are necrotic and the nuclei are dissolved. Moreover, the volume and number of lipid accumulation and fat vacuoles in the liver were significantly reduced after FRRT treatment. The hepatocytes were regularly arranged with prominent nuclei and ribosomes and well-defined cytoplasm (Figure 2A). The liver index reflects the extent of fat deposition in the liver to some extent. After FRRT treatment, the liver index was significantly reduced in the HFD group (Figure 2B, p < 0.01). In addition, it also reduced the levels of AST and ALT (Figures 2B–D, p < 0.01), indicating that FRRT improved the liver function impairment caused by HFD.
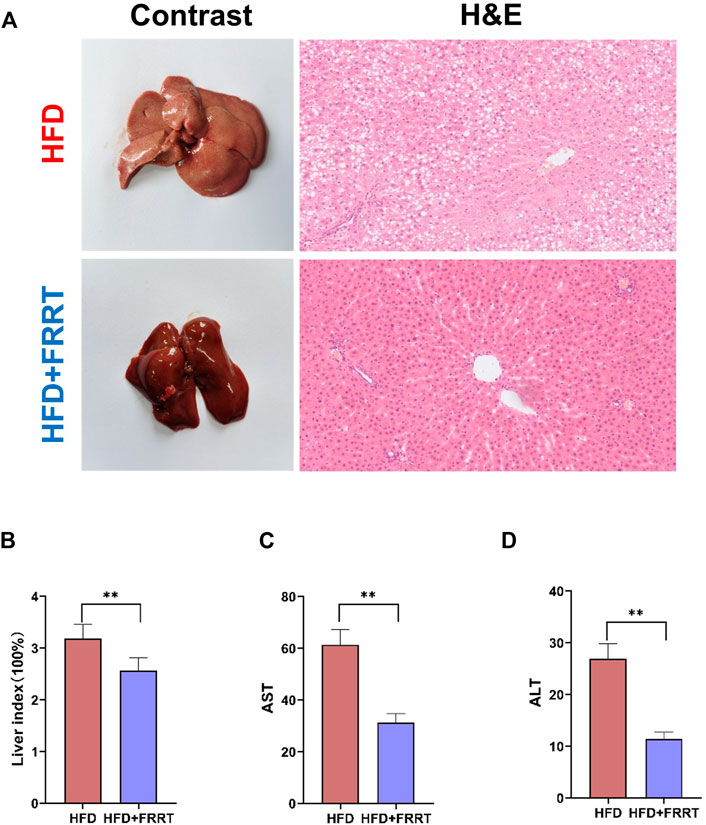
FIGURE 2. FRRT improves liver function damage in hyperlipidemic rats. (A) H&E staining images and morphology of Liver. (B) Changes in the liver index. Serum AST (C) and ALT (D) levels were measured using commercial kits. Data are presented as mean ± SEM (n = 6). *p < 0.05, **p < 0.01 compared with HFD group.
FRRT Ameliorates the Gut Microbial Dysbiosis in HFD-Induced Hyperlipidemic Rats
To investigate the effects on gut microbiota in HFD-induced hyperlipidemia rats given FFRT, we performed 16S rDNA high-throughput sequencing on rat feces. We firstly compared the microbiota diversity between the HFD and HFD + FRRT (Figure 3A). The richness and diversity of the bacterial community (Chao1, Simpson, Shannon, and observed species) were increased after FRRT treatment. Additionally, a significant difference was observed in ß-diversity based on the un-weighted (p = 0.002) and weighted (p = 0.003) UniFrac between the HFD and HFD + FRRT groups (Figure 3B). These results suggest that FRRT has a significant impact on intestinal microbiota composition.
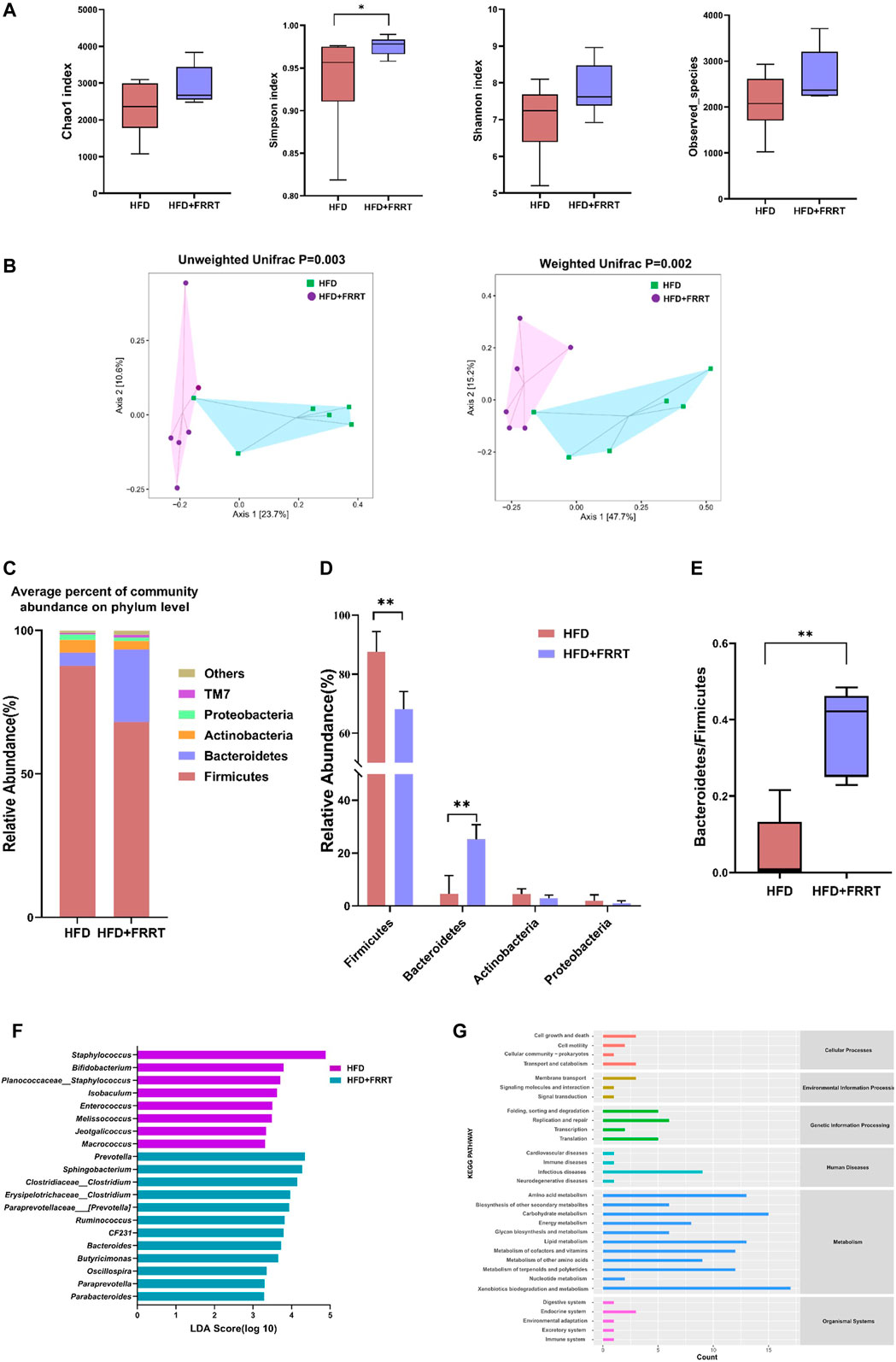
FIGURE 3. The alteration of the gut microbiome in HFD rats. (A) The a-diversity indexes of Chao1, Shannon, Simpson, and observed-species of gut microbiota between HFD and HFD + FRRT rats. (B) Unweighted based PCoA and weighted UniFrac based PCoA between gut bacterial communities of HFD and HFD + FRRT rats. p values were determined by the ADONIS test. Differential abundances of genera were determined by the Wilcoxon rank-sum test and Mann-Whitney U test. (C) The average percent of community abundance on phylum level in HFD and HFD + FRRT groups. (D) Relative abundance of Firmicutes, Bacteroidetes, Actinobacteria, Proteobacteria, and the Bacteroidetes/Firmicutes abundance ratio (E) in fecal microbiota between HFD and HFD + FRRT. (F) The LEfSe analysis of the gut microbiota differed between HFD and HFD + FRRT groups. (G) The effect of the gut microbiota modifications on predicted functional metabolic pathways obtained from PICRUSt analysis of 16S rDNA sequencing data.
Furthermore, we analyzed the effect of FRRT on intestinal microbiota at the phylum and genus levels. At the phylum levels, Firmicutes, Bacteroides, Proteobacteria, and Actinobacteria, were the dominant phyla, accounting for more than 85% of the relative abundance (Figure 3C). The FRRT treatment significantly lower the abundance of Firmicutes and increase the abundance of Bacteroides, and yielded a significantly (p < 0.05) increased Bacteroidetes to Firmicutes ratio (Figures 3D,E). At the genus levels, LEfSe analysis showed that the HFD rats had a higher abundance of Staphylococcus, Bifidobacterium, Isobaculum, Enterococcus, Melissococcus, Jeotgalicoccus, Macrococcus, whereas the HFD + FRRT rats primarily showed higher enrichment of Prevotella, Sphingobacterium, Clostridium, Erysipelotrichaceae_Clostridium, Paraprevotellaceae_Prevotella, Ruminococcus, CF231, Bacteroides, Butyricimonas, Oscillospira, Paraprevotella, Parabacteroides (Figure 3F).
PICRUSt analysis showed that the gut microbiota was predicted to be related to various metabolic functions, such as amino acid metabolism, energy metabolism, lipid metabolism, and carbohydrate metabolism (Figure 3G).
In brief, FRRT significantly affected the composition of gut microbiota in HFD-induced hyperlipidemic rats, and these altered gut microbiotas were closely related to the function of the lipid metabolism and amino acid metabolism in rats.
Alteration of the Fecal Metabolome in HFD-Induced Hyperlipidemic Rats
Metabolites produced by gut microbiota fermentation may influence host metabolism. A targeted metabolic profile analysis of 160 metabolites was conducted to study the differential metabolites in the feces. Score plots of PCA and OPLS-DA showed that the levels of fecal metabolites changed significantly after FRRT treatment (Figures 4A,B). Furthermore, by getting the intersection of the differential metabolites from univariate statistics and multi-dimensional statistics, we find 56 potential biomarkers which may play critical roles in biological processes (Figure 4C). Also, as shown in Figure 4D, these metabolites were mainly involved in amino acid metabolism (phenylalanine and tyrosine metabolism, valine, leucine and isoleucine degradation, glycine and serine metabolism. etc.), fatty acid metabolism (beta-oxidation of very-long-chain fatty acids, dodecanoic acid, myristic acid, citraconic acid. etc.), bile acids metabolism (HDCA, LCA, DCA, etc.). In brief, HFD was closely associated with altered amino acids metabolisms, bile acids metabolisms, and lipid metabolisms.
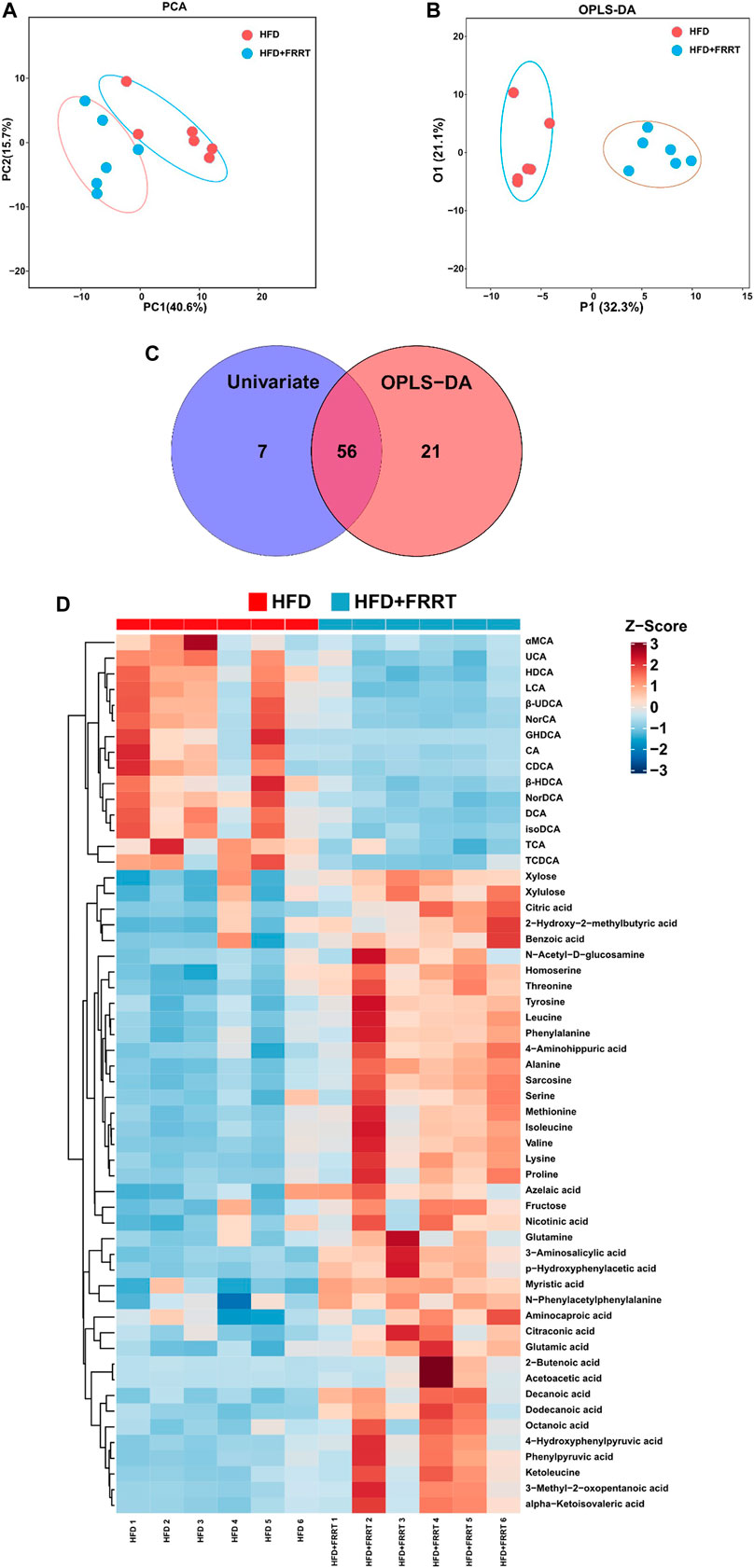
FIGURE 4. Metabolic profile as well as multivariate/Univariate analysis and metabolic pathway of metabolites between HFD and HFD + FRRT group. (A) PCA plots with the scores of the first two principal components based on 160 metabolites. (B) OPLS-DA plots with the scores of the first two principal components. (C) Venn Plot of differential metabolites, by getting union/intersection of the differential metabolites from univariate statistics and multi-dimensional statistics. (D) Heatmap analysis of potential biomarkers, the concentration value is converted to Z-score by standardized Z-score transformation.
Association Between Gut Microbial, Metabolites, and Metabolic Parameters
To further investigate the linkages between gut microbiota, metabolites, and metabolic parameters and their regulatory effects, we used Spearman’s rank correlation analysis to analyze the correlation between the 56 altered metabolites and 20 altered gut microbiota in the HFD and the HFD + FRRT group. The altered metabolites were mainly involved in amino acid metabolites (glutamine, glutamic acid, alanine, etc.), fatty acid metabolites (dodecanoic acid, myristic acid, decanoic acid, etc.), bile acids metabolites (HDCA, LCA, DCA, etc.). As shown in Figure 5A, the altered metabolites were significantly associated with Prevotella, Paraprevotellaceae_Prevotella, Ruminococcus, Oscillospira, Staphylococcus, Enterococcus, and Macrococcus.
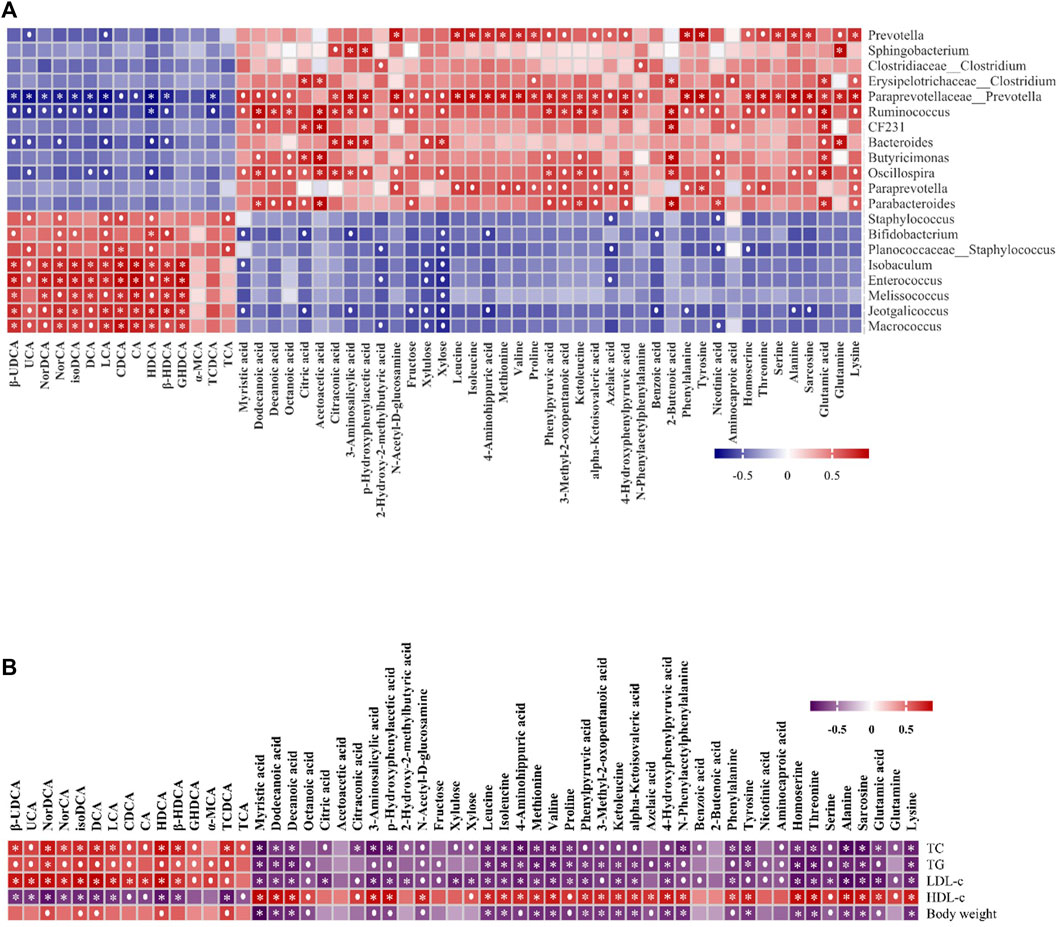
FIGURE 5. The Spearman’s correlation analysis of gut microbiota, metabolic parameters, and metabolites. (A) Correlations of 56 altered metabolites and five metabolic parameters in HFD and HFD + FRRT using Spearman correlation analysis. (B) Correlations of 56 altered metabolites and 20 altered gut microbiota in HFD and HFD + FRRT using Spearman correlation analysis.
We also performed Spearman’s rank correlation analysis of 56 altered metabolites with five metabolic parameters. The result suggested that the altered metabolites of amino acid metabolites (glutamine, glutamic acid, alanine, etc.), fatty acid metabolites (dodecanoic acid, myristic acid, decanoic acid, etc.), bile acids metabolites (HDCA, LCA, DCA, etc.) were significantly associated with blood lipid levels and bodyweight Figure 5B. The results suggested that FRRT facilitated its antihyperlipidemic effect by regulating the composition of gut microbiota and its metabolites.
Discussion
Hyperlipidemia is one of the components of metabolic syndrome, of which the pathophysiology is very complex and has been only partially elucidated. In this study, FRRT treatment can effectively improve the body weight and lipid levels in rats with high-fat diet-induced hyperlipidemia. Meanwhile, FRRT improved liver function impairment and mainly exerts a hypolipidemic effect by regulating the composition of gut microbiota and its metabolites.
The gut microbiota acts as an independent and stable ecosystem in the body. It carries out various metabolic activities in the human body and produces a series of metabolites that affect the health of the host (Fan and Pedersen, 2021). In recent decades, the gut microbiota, dominated by Firmicutes and Bacteroidetes, played an important role in the development of obesity (Turnbaugh et al., 2006; Murphy et al., 2010). Many studies demonstrated that a higher abundance ratio of Firmicutes to Bacteroidetes is positively correlated to obesity in obese mice and humans (Nguyen et al., 2017; Xiao et al., 2017; Zhao et al., 2017). Compared with the healthy rats in reported studies, the abundance of Firmicutes increased, and the abundance of Bacteroidetes decreased at the phylum in the HFD group (Chen et al., 2019). In this study, the abundance of Firmicutes was significantly decreased, and the abundance of Bacteroides was significantly increased in HFD + FRRT treatment. The ratio of Firmicutes to Bacteroidetes was also correspondingly reduced after FRRT treatment.
To further assess specific changes in the gut microbiota, we compared the relative abundance at the genus level between HFD and FRRT + HFD groups. We compared the relative abundance at the genus level between HFD and healthy rats, the relative abundances of Staphylococcus in the HFD group increased, whereas those of Oscillospira, Prevotella, Ruminococcus and Clostridium decreased compared with the healthy rats in the reported studies (Chen et al., 2019). In the current study, the FRRT significantly decreased the abundance of Staphylococcus, Bifidobacterium, Isobaculum, Enterococcus, Melissococcus, Jeotgalicoccus, Macrococcus, and increased the abundance of Prevotella, Sphingobacterium, Clostridium, Erysipelotrichaceae_Clostridium, Paraprevotellaceae_Prevotella, Ruminococcus, CF231, Bacteroides, Butyricimonas, Oscillospira, Para-prevotella, Parabacteroides. Among them, the reduction in Staphylococcus (LDA = 4.88, p = 0.037) and the increase in Prevotella (LDA = 3.94, p = 0.0033) were the most significant.
Our results showed the abundance of Staphylococcus enriched in high-fat diet-induced hyperlipidemia rats, and the relative abundance of Staphylococcus was dramatically decreased after FRRT treatment. Moreover, Staphylococcus is opportunistic pathogen genera (Foster, 2005; Wertheim et al., 2005; Jenkins et al., 2015), which can cause several types of infections, and colonization has been reported to occur easier in obese individuals and patients (van Belkum et al., 2009; Olsen et al., 2013). Besides, Enterococcus has been reported to promote intestinal inflammation by compromising the integrity of the epithelial barrier (Steck et al., 2011; Kao and Kline, 2019). We also observed the same phenomenon, but both of these microorganisms were suppressed by FRRT.
Prevotella, a dominant gut bacterium belongs to the phylum Bacteroidetes. Nutritional interventions with fiber-rich foods usually increase Prevotella abundance (Haro et al., 2017; Benítez-Páez et al., 2019; Roager et al., 2019; Ghosh et al., 2020). Increased Prevotella in gut microbiota has been reported to increase weight loss, lower cholesterol levels (Eriksen et al., 2020), and limit the action of bifidobacterial (Chung et al., 2020), which may reveal the phenomenon of the abundance of bifidobacteria decreased after FRRT treatment. However, we need further experiments to verify this phenomenon. Besides, Oscillospira abundance was also strongly associated with obesity, leanness, and human health (Tims et al., 2013; Goodrich et al., 2014; Cao et al., 2020). Numerous evidence suggest that the Oscillospira abundance plays an important role in the metabolic activities associated with hyperlipidemia and obesity (Escobar et al., 2014; Del Chierico et al., 2016; Yang et al., 2021). Meanwhile, other gut microbiota also plays important role in regulating hyperlipidemia, which demands further investigation. The results showed that the increase of several healthy microbial genera and the decrease of potentially pathogenic microorganisms were the main characteristics of FRRT in improving the disorder of gut microbiota.
Our results also showed that FRRT alleviated the high-fat diet-induced changes in amino acid metabolites (glutamine, glutamic acid, alanine, etc.), fatty acid metabolites (dodecanoic acid, myristic acid, decanoic acid, etc.), and bile acids metabolites (HDCA, LCA, DCA, etc.).
The deoxycholic and lithocholic acids of secondary bile acids were converted from primary bile acids by intestinal bacteria (Ridlon et al., 2005). Accumulating studies showed that a high-fat diet could lead to the increased production of secondary bile acids such as DCA and LCA, and evidence suggests that DCA and LCA are toxic bile acids associated with metabolic syndrome and gastrointestinal diseases (Bernstein et al., 2006; Stenman et al., 2012; Stenman et al., 2013). The farnesoid X receptor (FXR) is a bile acid (BA) receptor activated by specific BA metabolites, When FXR is activated, it inhibits the production of bile acids, inhibits the production of lipids, decreases triglycerides, and lowers blood glucose (Jiao et al., 2017; Sun et al., 2021; Xiang et al., 2021). Our studies showed that FRRT alleviated the fat-diet induced increases in fecal BAs such as DCA and LCA, which is consistent with the previous studies (Holscher et al., 2018; Wang et al., 2020; Yan et al., 2021). Our studies also showed that DCA, LCA was significantly positively correlated with TC, TG, LDL-c, and body weight and negatively correlated with the gut microbiota of Prevotella, Oscillospira, Paraprevotellaceae_Prevotella, Ruminococcus. These findings imply that FRRT may suppress FXR signaling in the liver and maintain homeostasis of BA enterohepatic circulation to improve dyslipidemia.
Meanwhile, the levels of amino acids in fecal metabolites were altered significantly. Some studies showed that amino acids play an important role in metabolism (Wu, 2009), which are mainly involved in various metabolic pathways, such as the tricarboxylic acid (TCA) cycle, gluconeogenesis, and others (Park and Han, 2018). l-alanine can affect ß cell signal transduction and apoptosis (Park and Han, 2018). High glutamine reduces glycolysis and inflammation in human fat cells and attenuates adipose tissue inflammation (Petrus et al., 2020), glutamic acid can be converted into aspartic acid through the TCA cycle, which plays an important role in glycolysis with energy metabolism (Davalli et al., 2012). Our studies showed that the contents of glutamic acid, glutamine, alanine, leucine, and isoleucine in the feces were increased in rats with FRRT treatment. The studies also showed amino acids such as glutamic acid, glutamine, alanine, leucine, and isoleucine that were significantly positively correlated with TC, TG, and gut microbiota of Oscillospira, Prevotella, Para-prevotellaceae_Prevotella, Ruminococcus. It implies that amino acids may accelerate the TCA cycle, leading to increased energy expenditure. However, the exact mechanisms are still too complex and need to be studied in more depth. In addition, some studies reported that fatty acids also play important roles in regulating energy metabolism. Such as myristic acid ameliorates hyperglycemia in genetic type 2 diabetes (Takato et al., 2017).
In this study, 16S rDNA sequencing and liquid mass spectrometry were used to study the changes in gut microbiota structure and metabolic function with FRRT treatment. In the high-fat diet-induced metabolic disorder syndrome of rats, gut microbiota composition and related metabolic pathways were significantly changed. In brief, FRRT mainly plays an important role in lowering blood lipid by affecting related bacteria (Prevotella, Oscillospira, Paraprevotellaceae_Prevotella, Ruminococcus) and their associated metabolites (amino acid metabolites, bile acid metabolites, and lipid metabolites).
FRRT significantly modified the structure and composition of gut bacteria and the levels of their related metabolites in rats. FRRT can prevent high-fat diet-induced hyperlipidemia, and mainly exerts a hypolipidemic effect by regulating the composition of gut microbiota and its metabolites. In addition, there was some limitation of the experiment that the lack of negative control, which makes it difficult to determine whether the values of the investigated parameters are close to those of healthy animals. Besides, the experiment only conducted a single-dose study and did not investigate the appropriate dose range and potential therapeutic relevance, which has limitations and incompleteness. Our study suggests that FRRT may serve as a new dietary and therapeutic strategy to improve dyslipidemia while also extending the understanding of the relationship between gut microbiota, metabolites, and lipid-lowering function. We need further studies to do so confirm the hypothesis (Deng et al., 2018; Jin et al., 2018).
Data Availability Statement
The datasets presented in this study can be found in online repositories. The names of the repository/repositories and accession number(s) can be found below: NCBI Sequence Read Archive (SRA) database under the Accession Number of SRP373909.
Ethics Statement
The animal study was reviewed and approved by Animal Experimental Ethics Committee of Guizhou Medical University.
Author Contributions
XG, PL, JJ, SZ, and MY designed the study, implemented, and performed the analysis, JJ wrote the manuscript. SZ, MY, MZ, LT, YJ, and CX prepared the tables and figures under the supervision of XG and PL. All the authors discussed the results. All authors contributed to and revised the manuscript critically.
Funding
This work was supported by the Science and Technology Fund Project of Guizhou Provincial Health and Health Commission (gzwkj 2021-454), the Guizhou provincial Science and Technology Plan Project ([2020] 4Y214), The scientific research project of the major agricultural industry of Guizhou province (KY [2019] 009), the Foundation of Guizhou Educational Committee (No. KY [2021] 008).
Conflict of Interest
The authors declare that the research was conducted in the absence of any commercial or financial relationships that could be construed as a potential conflict of interest.
Publisher’s Note
All claims expressed in this article are solely those of the authors and do not necessarily represent those of their affiliated organizations, or those of the publisher, the editors and the reviewers. Any product that may be evaluated in this article, or claim that may be made by its manufacturer, is not guaranteed or endorsed by the publisher.
Supplementary Material
The Supplementary Material for this article can be found online at: https://www.frontiersin.org/articles/10.3389/fphar.2022.883629/full#supplementary-material
References
Abliz, A., Aji, Q., Abdusalam, E., Sun, X., Abdurahman, A., Zhou, W., et al. (2014). Effect of Cydonia Oblonga Mill. Leaf Extract on Serum Lipids and Liver Function in a Rat Model of Hyperlipidaemia. J. Ethnopharmacol. 151, 970–974. doi:10.1016/j.jep.2013.12.010
Benítez-Páez, A., Kjølbæk, L., Gómez del Pulgar, E. M., Brahe, L. K., Astrup, A., Matysik, S., et al. (2019). A Multi-Omics Approach to Unraveling the Microbiome-Mediated Effects of Arabinoxylan Oligosaccharides in Overweight Humans. mSystems 4, e00209–19. doi:10.1128/mSystems.00209-19
Bernstein, H., Holubec, H., Bernstein, C., Ignatenko, N., Gerner, E., Dvorak, K., et al. (2006). Unique Dietary-Related Mouse Model of Colitis. Inflamm. Bowel Dis. 12, 278–293. doi:10.1097/01.MIB.0000209789.14114.63
Cao, C., Liu, M., Qu, S., Huang, R., Qi, M., Zhu, Z., et al. (2020). Chinese Medicine Formula Kai-Xin-San Ameliorates Depression-like Behaviours in Chronic Unpredictable Mild Stressed Mice by Regulating Gut Microbiota-Inflammation-Stress System. J. Ethnopharmacol. 261, 113055. doi:10.1016/j.jep.2020.113055
Chen, H., Miao, H., Feng, Y. L., Zhao, Y. Y., and Lin, R. C. (2014). Metabolomics in Dyslipidemia. Adv. Clin. Chem. 66, 101–119. doi:10.1016/b978-0-12-801401-1.00004-9
Chen, Q., Liu, M., Zhang, P., Fan, S., Huang, J., Yu, S., et al. (2019). Fucoidan and Galactooligosaccharides Ameliorate High-Fat Diet-Induced Dyslipidemia in Rats by Modulating the Gut Microbiota and Bile Acid Metabolism. Nutrition 65, 50–59. doi:10.1016/j.nut.2019.03.001
Chung, W. S. F., Walker, A. W., Bosscher, D., Garcia-Campayo, V., Wagner, J., Parkhill, J., et al. (2020). Relative Abundance of the Prevotella Genus within the Human Gut Microbiota of Elderly Volunteers Determines the Inter-individual Responses to Dietary Supplementation with Wheat Bran Arabinoxylan-Oligosaccharides. BMC. Microbiol. 20, 283. doi:10.1186/s12866-020-01968-4
Davalli, A. M., Perego, C., and Folli, F. B. (2012). The Potential Role of Glutamate in the Current Diabetes Epidemic. Acta Diabetol. 49, 167–183. doi:10.1007/s00592-011-0364-z
Del Chierico, F., Nobili, V., Vernocchi, P., Russo, A., De Stefanis, C., Gnani, D., et al. (2016). Gut Microbiota Profiling of Pediatric Nonalcoholic Fatty Liver Disease and Obese Patients Unveiled by an Integrated Meta-Omics-Based Approach. Hepatology 65 (2), 451–464. doi:10.1002/hep.28572
Deng, X., Ma, J., Song, M., Jin, Y., Ji, C., Ge, W., et al. (2018). Effects of Products Designed to Modulate the Gut Microbiota on Hyperlipidaemia. Eur. J. Nutr. 58, 2713–2729. doi:10.1007/s00394-018-1821-z
Eriksen, A. K., Brunius, C., Mazidi, M., Hellström, P. M., Risérus, U., Iversen, K. N., et al. (2020). Effects of Whole-Grain Wheat, rye, and Lignan Supplementation on Cardiometabolic Risk Factors in Men with Metabolic Syndrome: a Randomized Crossover Trial. Am. J. Clin. Nutr. 111, 864–876. doi:10.1093/ajcn/nqaa026
Escobar, J. S., Klotz, B., Valdes, B. E., and Agudelo, G. M. (2014). The Gut Microbiota of Colombians Differs from that of Americans, Europeans and Asians. BMC. Microbiol. 14, 311. doi:10.1186/s12866-014-0311-6
Fan, Y., and Pedersen, O. (2021). Gut Microbiota in Human Metabolic Health and Disease. Nat. Rev. Microbiol. 19, 55–71. doi:10.1038/s41579-020-0433-9
Foster, T. J. (2005). Immune Evasion by Staphylococci. Nat. Rev. Microbiol. 3, 948–958. doi:10.1038/nrmicro1289
Ghosh, T. S., Rampelli, S., Jeffery, I. B., Santoro, A., Neto, M., Capri, M., et al. (2020). Mediterranean Diet Intervention Alters the Gut Microbiome in Older People Reducing Frailty and Improving Health Status: the NU-AGE 1-year Dietary Intervention across Five European Countries. Gut 69, 1218–1228. doi:10.1136/gutjnl-2019-319654
Goodrich, J. K., Waters, J. L., Poole, A. C., Sutter, J. L., Koren, O., Blekhman, R., et al. (2014). Human Genetics Shape the Gut Microbiome. Cell 159 (4), 789–799. doi:10.1016/j.cell.2014.09.053
Guan, X. Y., Hu, G. P., and Wang, Y. (2020). Effects of Different Drying Methods on Polysaccharide Content and Antioxidant Activity of Polysaccharides from Rosa Roxburghii Tratt. J. Food Saf. Qual. 11 (08), 2598–2602. doi:10.19812/j.cnki.jfsq11-5956/ts.2020.08.045
Hao Wang, H., Ye, Y. H., Wang, H. H., Liu, J., Liu, Y. J., and Jiang, B. W. (2019). HPLC-QTOF-MS/MS Profiling, Antioxidant, and α-glucosidase Inhibitory Activities of Pyracantha Fortuneana Fruit Extracts. J. Food Biochem. 43 (5), e12821. doi:10.1111/jfbc.12821
Haro, C., García-Carpintero, S., Rangel-Zúñiga, O. A., Alcalá-Díaz, J. F., Landa, B. B., Clemente, J. C., et al. (2017). Consumption of Two Healthy Dietary Patterns Restored Microbiota Dysbiosis in Obese Patients with Metabolic Dysfunction. Mol. Nutr. Food Res. 61, 1700300. doi:10.1002/mnfr.201700300
Holscher, H. D., Guetterman, H. M., Swanson, K. S., An, R., Matthan, N. R., Lichtenstein, A. H., et al. (2018). Walnut Consumption Alters the Gastrointestinal Microbiota, Microbially Derived Secondary Bile Acids, and Health Markers in Healthy Adults: A Randomized Controlled Trial. J. Nutr. 148 (6), 861–867. doi:10.1093/jn/nxy004
Huizhu Wang, H., Li, Y., Ren, Z., Cong, Z., Chen, M., Shi, L., et al. (2018). Optimization of the Microwave-Assisted Enzymatic Extraction of Rosa Roxburghii Tratt. Polysaccharides Using Response Surface Methodology and its Antioxidant and α-d-glucosidase Inhibitory Activity. Int. J. Biol. Macromol 112, 473–482. doi:10.1016/j.ijbiomac.2018.02.003
Jenkins, A., Diep, B. A., Mai, T. T., Vo, N. H., Warrener, P., Suzich, J., et al. (2015). Differential Expression and Roles of Staphylococcus aureus Virulence Determinants during Colonization and Disease. mBio 6, e02272–14. doi:10.1128/mBio.02272-14
Jiao, N., Baker, S. S., Chapa-Rodriguez, A., Liu, W., Nugent, C. A., Tsompana, M., et al. (2017). Suppressed Hepatic Bile Acid Signalling Despite Elevated Production of Primary and Secondary Bile Acids in NAFLD. Gut 67, 1881–1891. doi:10.1136/gutjnl-2017-314307
Jin, C., Zeng, Z., Wang, C., Luo, T., Wang, S., Zhou, J., et al. (2018). Insights into a Possible Mechanism Underlying the Connection of Carbendazim-Induced Lipid Metabolism Disorder and Gut Microbiota Dysbiosis in Mice. Toxicol. Sci. 166, 382–393. doi:10.1093/toxsci/kfy205
Kao, P. H. N., and Kline, K. A. (2019). Dr. Jekyll and Mr. Hide: How Enterococcus faecalis Subverts the Host Immune Response to Cause Infection. J. Mol. Biol. 431, 2932–2945. doi:10.1016/j.jmb.2019.05.030
Lei Wang, L., Li, C., Huang, Q., and Fu, X. (2019). Polysaccharide from Rosa Roxburghii Tratt Fruit Attenuates Hyperglycemia and Hyperlipidemia and Regulates Colon Microbiota in Diabetic Db/db Mice. J. Agric. Food Chem. 68 (1), 147–159. doi:10.1021/acs.jafc.9b06247
Lei Wang, L., Zhang, B., Xiao, J., Huang, Q., Li, C., and Fu, X. (2018). Physicochemical, Functional, and Biological Properties of Water-Soluble Polysaccharides from Rosa Roxburghii Tratt Fruit. Food Chem. 249, 127–135. doi:10.1016/j.foodchem.2018.01.011
Li, H., Fang, W., Wang, Z., and Chen, Y. (2022). Physicochemical, Biological Properties, and Flavour Profile of Rosa Roxburghii Tratt, Pyracantha Fortuneana, and Rosa Laevigata Michx Fruits: A Comprehensive Review. Food Chem. 366, 130509. doi:10.1016/j.foodchem.2021.130509
Lian, Z. L., Wang, Y., Liu, Y., Zhou, X., Cheng, Z. J., Zhen, J. Z., et al. (2021). Determination of the Content of the Main Effective Components of Rosa Roxburghii Tratt Resources and Comprehensive Quality Evaluation. J. Chin. Med. Mater. 8, 1915–1922. doi:10.13863/j.issn1001-4454.2021.08.023
Liu, M. H., Zhang, Q., Zhang, Y. H., Lu, X. Y., Fu, W. M., and He, J. Y. (2016). Chemical Analysis of Dietary Constituents in Rosa Roxburghii and Rosa Sterilis Fruits. Molecules 21, 1204. doi:10.3390/molecules21091204
Lu, F. T., and Bao, S. J. (2002). Effect of Polysaccharides from Fructus Rosae Roxburghii on Stress Tolerance and Immune Function. J. GZ. Univ. Tradit. Chin. Med. 19 (2), 141–142. doi:10.3969/j.issn.1007-3213.2002.02.019
Murphy, E. F., Cotter, P. D., Healy, S., Marques, T. M., O'Sullivan, O., Fouhy, F., et al. (2010). Composition and Energy Harvesting Capacity of the Gut Microbiota: Relationship to Diet, Obesity and Time in Mouse Models. Gut 59 (12), 1635–1642. doi:10.1136/gut.2010.215665
Nguyen, T. D., Prykhodko, O., Fåk Hållenius, F., and Nyman, M. (2017). Effects of Monobutyrin and Tributyrin on Liver Lipid Profile, Caecal Microbiota Composition and SCFA in High-Fat Diet-Fed Rats. J. Nutr. Sci. 6, e51. doi:10.1017/jns.2017.54
Olsen, K., Danielsen, K., Wilsgaard, T., Sangvik, M., Sollid, J. U., Thune, I., et al. (2013). Obesity and Staphylococcus aureus Nasal Colonization Among Women and Men in a General Population. Plos. ONE. 8 (5), e63716. doi:10.1371/journal.pone.0063716
Park, J. E., and Han, J. S. (2018). A Portulaca Oleracea L. Extract Promotes Insulin Secretion via a K+ATP Channel Dependent Pathway in INS-1 Pancreatic β-cells. Nutr. Res. Pract. 12, 183–190. doi:10.4162/nrp.2018.12.3.183
Petrus, P., Lecoutre, S., Dollet, L., Wiel, C., Sulen, A., Gao, H., et al. (2020). Glutamine Links Obesity to Inflammation in Human White Adipose Tissue. Cell. Metab. 31 (2), 375–e11. doi:10.1016/j.cmet.2019.11.019
Pirillo, A., Casula, M., Olmastroni, E., Norata, G. D., and Catapano, A. L. (2021). Global Epidemiology of Dyslipidaemias. Nat. Rev. Cardiol. 18 (10), 689–700. doi:10.1038/s41569-021-00541-4
Ridlon, J. M., Kang, D. J., and Hylemon, P. B. (2005). Bile Salt Biotransformations by Human Intestinal Bacteria. J. Lipid. Res. 47, 241–259. doi:10.1194/jlr.R500013-JLR200
Roager, H. M., Vogt, J. K., Kristensen, M., Hansen, L. B. S., Ibrügger, S., Mærkedahl, R. B., et al. (2019). Whole Grain-Rich Diet Reduces Body Weight and Systemic Low-Grade Inflammation without Inducing Major Changes of the Gut Microbiome: a Randomised Cross-Over Trial. Gut 68, 83–93. doi:10.1136/gutjnl-2017-314786
Song, P., and Shen, X. (2021). Proteomic Analysis of Liver in Diet-Induced Hyperlipidemic Mice under Fructus Rosa Roxburghii Action. J. Proteomics 230, 103982. doi:10.1016/j.jprot.2020.103982
Steck, N., Hoffmann, M., Sava, I. G., Kim, S. C., Hahne, H., Tonkonogy, S. L., et al. (2011). Enterococcus faecalis Metalloprotease Compromises Epithelial Barrier and Contributes to Intestinal Inflammation. Gastroenterology 141, 959–971. doi:10.1053/j.gastro.2011.05.035
Stenman, L. K., Holma, R., Forsgård, R., Gylling, H., and Korpela, R. (2013). Higher Fecal Bile Acid Hydrophobicity Is Associated with Exacerbation of Dextran Sodium Sulfate Colitis in Mice. J. Nutr. 143 (11), 1691–1697. doi:10.3945/jn.113.180810
Stenman, L. K., Holma, R., and Korpela, R. (2012). High-fat-induced Intestinal Permeability Dysfunction Associated with Altered Fecal Bile Acids. World J. Gastroenterol. 18 (9), 923–929. doi:10.3748/wjg.v18.i9.923
Sun, L., Cai, J., and Gonzalez, F. J. (2021). The Role of Farnesoid X Receptor in Metabolic Diseases, and Gastrointestinal and Liver Cancer. Nat. Rev. Gastroenterol. Hepatol. 18 (5), 335–347. doi:10.1038/s41575-020-00404-2
Takato, T., Iwata, K., Murakami, C., Wada, Y., and Sakane, F. (2017). Chronic Administration of Myristic Acid Improves Hyperglycaemia in the Nagoya-Shibata-Yasuda Mouse Model of Congenital Type 2 Diabetes. Diabetologia 60, 2076–2083. doi:10.1007/s00125-017-4366-4
Tan, D. H., Wang, P. J., Zhang, S., Liu, Y., Zhang, M., and Gao, X. L. (2019). Study on Content Determination of Ellagic Acid in Different Medicinal Parts of Rosa Roxburghii and In Vitro Antioxidant Activity of its Ethanol Extract. China. Pharm. 30 (09), 1236–1240. doi:10.6039/j.issn.1001-0408.2019.09.17
Tims, S., Derom, C., Jonkers, D. M., Vlietinck, R., Saris, W. H., Kleerebezem, M., et al. (2013). Microbiota Conservation and BMI Signatures in Adult Monozygotic Twins. ISME. J. 7 (4), 707–717. doi:10.1038/ismej.2012.146
Turnbaugh, P. J., Ley, R. E., Mahowald, M. A., Magrini, V., Mardis, E. R., and Gordon, J. I. (2006). An Obesity-Associated Gut Microbiome with Increased Capacity for Energy Harvest. Nature 444 (7122), 1027–1031. doi:10.1038/nature05414
van Belkum, A., Melles, D. C., Nouwen, J., van Leeuwen, W. B., van Wamel, W., Vos, M. C., et al. (2009). Co-evolutionary Aspects of Human Colonisation and Infection by Staphylococcus aureus. Infect. Genet. Evol. 9, 32–47. doi:10.1016/j.meegid.2008.09.012
Wang, L., Gong, Z., Zhang, X., Zhu, F., Liu, Y., Jin, C., et al. (2020). Gut Microbial Bile Acid Metabolite Skews Macrophage Polarization and Contributes to High-Fat Diet-Induced Colonic Inflammation. Gut. Microbes 12 (1), 1–20. doi:10.1080/19490976.2020.1819155
Wang, L. T., Lv, M. J., An, J. Y., Fan, X. H., Dong, M. Z., Zhang, S. D., et al. (2021). Botanical Characteristics, Phytochemistry and Related Biological Activities of Rosa Roxburghii Tratt Fruit, and its Potential Use in Functional Foods: A Review. Food Funct. 12 (4), 1432–1451. doi:10.1039/d0fo02603d
Wei, M., Chai, W. M., Yang, Q., Wang, R., and Peng, Y. (2017). Novel Insights into the Inhibitory Effect and Mechanism of Proanthocyanidins from Pyracantha Fortuneana Fruit on α-Glucosidase. J. Food Sci. 82 (10), 2260–2268. doi:10.1111/1750-3841.13816
Wertheim, H. F., Melles, D. C., Vos, M. C., van Leeuwen, W., van Belkum, A., Verbrugh, H. A., et al. (2005). The Role of Nasal Carriage in Staphylococcus aureus Infections. Lancet Infect. Dis. 5, 751–762. doi:10.1016/S1473-3099(05)70295-4
Wu, G. (2009). Amino Acids: Metabolism, Functions, and Nutrition. Amino Acids 37, 1–17. doi:10.1007/s00726-009-0269-0
Xiang, J., Zhang, Z., Xie, H., Zhang, C., Bai, Y., Cao, H., et al. (2021). Effect of Different Bile Acids on the Intestine through Enterohepatic Circulation Based on FXR. Gut. Microbes 13 (1), 1949095. doi:10.1080/19490976.2021.1949095
Xiao, L., Sonne, S. B., Feng, Q., Chen, N., Xia, Z., Li, X., et al. (2017). High-fat Feeding rather Than Obesity Drives Taxonomical and Functional Changes in the Gut Microbiota in Mice. Microbiome 5, 43. doi:10.1186/s40168-017-0258-6
Xie, G., Wang, L., Chen, T., Zhou, K., Zhang, Z., Li, J., et al. (2021). A Metabolite Array Technology for Precision Medicine. Anal. Chem. 93 (14), 5709–5717. doi:10.1021/acs.analchem.0c04686
Xu, J., Vidyarthi, S. K., Bai, W., and Pan, Z. (2019). Nutritional Constituents, Health Benefits and Processing of Rosa Roxburghii: a Review. J. Funct. Foods 60, 103456. doi:10.1016/j.jff.2019.103456
Yan, R., Wang, K., Wang, Q., Jiang, H., Lu, Y., Chen, X., et al. (2022). Probiotic Lactobacillus Casei Shirota Prevents Acute Liver Injury by Reshaping the Gut Microbiota to Alleviate Excessive Inflammation and Metabolic Disorders. Microb. Biotechnol. 15 (1), 247–261. doi:10.1111/1751-7915.13750
Yang, J., Li, Y., Wen, Z., Liu, W., Meng, L., and Huang, H. (2021). Oscillospira - a Candidate for the Next-Generation Probiotics. Gut. Microbes 13 (1), 1987783. doi:10.1080/19490976.2021.1987783
Zhang, C., Liu, X., Qiang, H., Li, K., Wang, J., Chen, D., et al. (2001). Inhibitory Effects of Rosa Roxburghii Tratt Juice on In Vitro Oxidative Modification of Low Density Lipoprotein and on the Macrophage Growth and Cellular Cholesteryl Ester Accumulation Induced by Oxidized Low Density Lipoprotein. Clin. Chim. Acta 313 (1–2), 37–43. doi:10.1016/S0009-8981(01)00647-7
Zhao, L., Xu, L., Tao, X., Han, X., Yin, L., Qi, Y., et al. (2016). Protective Effect of the Total Flavonoids from Rosa Laevigata Michx Fruit on Renal Ischemia-Reperfusion Injury through Suppression of Oxidative Stress and Inflammation. Molecules 21 (7), 952. doi:10.3390/molecules21070952
Keywords: fermented rosa roxburghii tratt juice, gut microbiota, metabolomic, hyperlipidemia, lipid metabolism
Citation: Ji J, Zhang S, Yuan M, Zhang M, Tang L, Wang P, Liu Y, Xu C, Luo P and Gao X (2022) Fermented Rosa Roxburghii Tratt Juice Alleviates High-Fat Diet-Induced Hyperlipidemia in Rats by Modulating Gut Microbiota and Metabolites. Front. Pharmacol. 13:883629. doi: 10.3389/fphar.2022.883629
Received: 25 February 2022; Accepted: 19 April 2022;
Published: 20 May 2022.
Edited by:
Aleksandar Raskovic, University of Novi Sad, SerbiaReviewed by:
Nebojsa Pavlovic, University of Novi Sad, SerbiaAna Tomas, University of Novi Sad, Serbia
Copyright © 2022 Ji, Zhang, Yuan, Zhang, Tang, Wang, Liu, Xu, Luo and Gao. This is an open-access article distributed under the terms of the Creative Commons Attribution License (CC BY). The use, distribution or reproduction in other forums is permitted, provided the original author(s) and the copyright owner(s) are credited and that the original publication in this journal is cited, in accordance with accepted academic practice. No use, distribution or reproduction is permitted which does not comply with these terms.
*Correspondence: Peng Luo, bHVvcGVuZ0BnbWMuZWR1LmNu; Xiuli Gao, Z2FveGxAZ21jLmVkdS5jbg==