- 1Department of Pharmacognosy, Faculty of Pharmacy, Istanbul University, Istanbul, Turkey
- 2Department of Pharmacognosy, Faculty of Pharmacy, Biruni University, Istanbul, Turkey
- 3Department of Pharmaceutical Botany, Faculty of Pharmacy, Istanbul University, Istanbul, Turkey
- 4Department of Pharmaceutical Microbiology, Faculty of Pharmacy, Istanbul University, Istanbul, Turkey
- 5Department of Nursing, School of Health Sciences, Batman University, Batman, Turkey
- 6Department of Pharmacognosy, Faculty of Pharmacy, Dicle University, Diyarbakır, Turkey
- 7Department of Laboratory Animals, Faculty of Veterinary, Dicle University, Diyarbakır, Turkey
- 8Dicle University Health Sciences Application and Research Center (DÜSAM), Diyarbakır, Turkey
- 9Department of Analytical Chemistry, Faculty of Pharmacy, Dicle University, Diyarbakır, Turkey
Thyme has been used for various therapeutic purposes in many different cultures, which makes it one of the most riveting medicinal plants throughout history. From its beneficial effects on the respiratory tract or the gastrointestinal system, to its unique skin-related activities, the investigation of the medicinal properties of thyme has always been an alluring topic for researchers aiming to develop conventional medications from this traditional herb. With an incentive to contribute to the extensive thyme research, three Thymus L. species namely Thymus cariensis Hub-Mor. & Jalas (endemic), Thymus praceox subsp. grossheimii (Ronniger) Jalas, and Thymus pubescens Boiss. et Kotschy ex Celak from Turkey were deeply investigated within this study. The analysis of the phytochemical constituents of the extracts was conducted by LC-MS/MS. 12 biologically important secondary metabolites (p-coumaric acid, caffeic acid, salicylic acid, quinic acid, fumaric acid, vanillin, malic acid, rutin, apigenin, naringenin, and nicotiflorin) were detected in all extracts. Their total phenolic and flavonoid contents were calculated (11.15 ± 0.17—61.12 ± 2.59 μg PEs/mg extract, 2.53 ± 0.04—40.28 ± 0.92 μg QEs/mg extract, respectively), and the antioxidant potential of the extracts was evaluated by DPPH and ABTS radical scavenging and CUPRAC activity methods, accordingly, the extracts were shown to possess significant antioxidant activity. Among them, Thymus cariensis Hub-Mor. & Jalas was the most active with IC50 values of 34.97 ± 1.00 μg/ml and 9.98 ± 0.04 μg/ml regarding the DPPH and ABTS radical scavenging assays, respectively, and an A0.5 value of 5.80 ± 0.02 μg/ml according to CUPRAC activity method. Their anticholinesterase, antityrosinase, and antiurease activities were also tested, Thymus cariensis Hub-Mor. & Jalas (35.61 ± 1.20%) and Thymus pubescens Boiss. et Kotschy ex Celak aerial part extract (33.49 ± 1.39%) exhibited moderate antibutyrylcholinesterase activity at 200 μg/ml concentration. The results of the cell viability assay indicated that the extracts demonstrated moderate-to-low cytotoxicity on A498 human renal cell lines. Furthermore, all studied extracts exerted noteworthy antimicrobial activity, especially against Candida tropicalis (MIC values: 19.53—78.12 μg/ml). The presented data substantiates the use of thyme extracts as therapeutic agents in both ethnomedicine and conventional therapies.
1 Introduction
Using medicinal plants for therapeutic purposes dates back to the Neanderthal period according to the archaeological evidence. The discovery of the 65,000-year-old pollen fossils found in Shanidar Caves (Northern Iraq), has been considered the proof of the long tradition of using particular medicinal plants for the treatment of diseases. Another remarkable example from the Neolithic period is the discovery of the famous “Ice Man” Otzi, who was later diagnosed with whipworm infection, and residue of plants with acaricidal activity was found among his possessions (Sendker and Sheridan, 2017). It is also known that the first humans were also the first cultivators of plants, not only aiming to provide themselves with the food they needed, but also the medicinal drugs for their well-being (Bonini et al., 2018).
Along with this, from a historical perspective, it is known that the most important drugs that are being commonly used today have originated from plants. Although the extraordinary developments in chemistry led to synthetic drugs superseding the natural compounds in the 20th century, in today’s world, the pharmaceutical companies and academia tend to be less enamored of single synthetic compounds as magic bullet cures due to the high costs, and logical toxicity concerns (Stahl-Biskup and Sáez, 2002). In addition to this, the increasing demand for “more natural, effective, and safer” therapeutic agents by the public has made the herbal market evolve dynamically worldwide. Resulting of this, more and more natural supplements, herbal medicines, or conventional drugs that contain plant-derived compounds can be seen on the shelves of pharmacies, and pharmacognosy, which is the multidisciplinary science of nature-derived pharmaceuticals, is becoming inevitably more popular among researchers.
Since the year 2,000, the ethnopharmacological research has been more diversified also more focused on the medicinal plants that are used as food, as well. Thymus species are unquestionably among these plants (Yeung et al., 2018). “Thyme” is the commonly used name for the members of the Thymus L. species. The great history of thyme starts with its traditional use supported by only empirical results and debatable observations but continues with scientific data provided by numerous studies including clinical trials. These developments bring about the change of thyme from being a traditional plant and a taste enhancer only, to a serious drug in evidence-based phytotherapy (Zarzuelo and Crespo, 2002). Thyme extracts and oils are the ingredients of almost every herbal product that is used for respiratory system diseases, making thyme-containing products among the most selling herbal medications in many countries. Parenthetically, the high amount of essential oil-producing of Thymus species is explained by their adaptive characteristics for dry climate conditions. Owing to their unique leave shapes with dense hairs on them, thymes are known to be quite resistant, and they can survive cold temperatures and aridness (Morales, 2002). Even when they have to face challenging environmental conditions such as drought stress, they were shown to have the ability to develop adaptation to these conditions by activating their stress response and evolving their phytochemistry accordingly (Mahdavi et al., 2020; Ashrafi et al., 2022). Over and above, the members of the genus Thymus have significant variations in their morphological characteristics that lead them to be evaluated under a great number of species mostly distributed in the Mediterranean region. In Turkey for instance, with the newly identified species, namely T. baseri Öztürk, Yaylacı, Koyuncu & Ocak, the number of Thymus species increased to 39 (Ozturk et al., 2022).
The wide medical applications of Thymus species are certainly worth mentioning. As a case in point, in Germany, the syrup made with thyme is a commonly prescribed medication by pediatrists for the respiratory problems of children. Thyme oil is known as the favorite aromatherapy oil for respiratory tract diseases (Schulz et al., 2004). As it is used the most commonly for therapeutic purposes, not only for respiratory system-related problems but also for further important diseases, the scientifically proved pharmacological activities of Thymus species are countless. Speaking of which, T. dreatensis Batt. whole plant extract was shown to demonstrate anticoagulant and antihyperlipidemic activity. T. serpyllum L. extract was shown to possess an antihypertensive effect (Michel et al., 2020). T. vulgaris L. exerted anti-tumor activity on various cell lines by reducing the tumor volume decreasing cell proliferation and inducing apoptosis (Choudhari et al., 2020). Furthermore, T. vulgaris L. is among the most studied species in terms of antibacterial activity, generally resulting in extraordinarily strong activity results (Chassagne et al., 2021). T. serrulatus Hochst. ex Benth. aqueous extract was shown to have significant antihyperglycemic activity (Haile et al., 2021).
The diverse pharmacological activities of Thymus L. species are attributed to their unique chemical characterization. Although the research has been mostly conducted on the essential oil compositions and their activities, recently the chemical profiling of different Thymus extracts prepared with different solvents is being investigated more deeply. Nonvolatile secondary metabolites of thyme include a variety of phenolic compounds, terpenoids, and phytosterols. Speaking of the phenolics, apigenin, naringenin, and their derivatives are usually detected in different Thymus extracts. Phenolic acids including rosmarinic acid and caffeic acid, and benzoic acid derivatives are also usually present in the extracts (Li et al., 2019).
It can be observed that, as the research on Thymus species continues, the status of these plants has moved from being only some traditional plants collected from some herb collectors without meeting any quality criteria to a rational drug fulfilling the safety and efficacy requirements. On that account, the use of a few Thymus species was approved by Commission E monographs, ESCOP monographs, and WHO monographs (Stahl-Biskup, 2002). That being the case, in efforts to shed more light on the properties of these species, further studies are crucial. With this impetus, this study investigates the chemical compositions and biological activities of Thymus cariensis Hub-Mor. & Jalas, Thymus praceox subsp. grossheimii (Ronniger) Jalas and Thymus pubescens Boiss. et Kotschy ex Celak were collected from different sites in Turkey. Their secondary metabolites were determined by LC-MS/MS, their total phenolic and flavonoid contents were calculated, and their antioxidant, anticholinesterase, antityrosinase, antiurease, anticancer, and antimicrobial activities were assessed. Towards a new understanding of the medicinal potential of Thymus species, findings of the current study may be contributing.
2 Materials and Methods
2.1 Plant Material
T. cariensis Hub-Mor. & Jalas was picked up from Koycegiz—Mugla, T. praceox subsp. grossheimii (Ronniger) Jalas was collected from Savsat—Artvin, and T. pubescens Boiss. et Kotschy ex Celak was picked up from Kars, Turkey, in June 2014. The scientific names of the plant taxa were checked and controlled according to the checklist of the flora of Turkey (Güner et al., 2012) and The Plant List website (The Plant List, 2013). The samples were identified by Assoc. Prof. Dr. Yeter Yesil Canturk. Voucher herbarium specimens (T. cariensis Hub-Mor. & Jalas: 116046, T. praceox subsp. grossheimii (Ronniger) Jalas: 116173, and T. pubescens: 117326) are preserved in the Istanbul University Faculty of Pharmacy Herbarium (ISTE). All details about the plant samples can be seen in Table 1.

TABLE 1. Plant names, collection details, herbarium numbers, and extract yield of the studied Thymus extracts.
2.2 Extraction
The whole plant including roots (10 g) was macerated in ethanol (100 ml) for 24 h at room temperature and then filtrated through Whatman filter paper (No. 1). The procedure was repeated twice more, and combined filtrates were concentrated by using a rotary evaporator at 40°C. The extract was stored at −20°C until the evaluation.
2.3 LC-MS/MS Analysis
A previously validated method (Yilmaz et al., 2018) was carried out to identify the LC-MS/MS analysis of the studied ethanol extracts. 37 standard phenolic compounds and a Nexera model UHPLC (Shimadzu) coupled to a tandem MS instrument were used to determine the chemical composition of the extract. Shimadzu Lab Solutions software was used to process the gathered data from LC-ESI-MS/MS.
2.4 Total Phenolic and Flavonoid Contents
The total phenolic content of the extract was determined according to the previously used method by Boga et al. (2016), which is a modified procedure described by Slinkard and Singleton (1977) for the first time. The results were calculated by using followed equation; Absorbance = 0.0409 pyrocatechol (μg) + 0.0495 (R2 = 0.9975) and given as micrograms of pyrocatechol equivalents (PEs). The total flavonoid content of the extracts was measured by using the method designed by Moreno et al. (2000), and the results here were expressed as micrograms of quercetin equivalents (QEs). The following equation was used to calculate the total flavonoid contents of the extract; Absorbance = 0.0347 quercetin (μg) + 0.1174 (R2 = 0.9992).
2.5 Antioxidant Activities
DPPH free radical scavenging, ABTS cation radical scavenging, and CUPRAC activity methods were used to investigate the antioxidant activities of the extract. A procedure described by Blois, 1958 was developed and applied to identify the DPPH free radical scavenging potential of the extract. ABTS cation radical decolorization activity of the extract was conducted by using the method designed by Re et al. (1999). The cupric reducing antioxidant capacity (CUPRAC) of the extract was determined using the method summarized by Apak et al. (2004).
2.6 Enzyme Inhibition Activity Assays
A method designed by Ellman et al. (1961) was performed to determine the acetylcholinesterase and butyrylcholinesterase inhibitory activity of the extracts. To determine the tyrosinase and urease inhibition activities of the studied extracts, the same methods were used from previously published papers (Ersoy et al., 2019; Ersoy et al., 2020).
2.7 Antimicrobial Activities
Antimicrobial activities of the studied extracts and standard compounds against the strains were carried out using the micro broth dilution method as described by the Clinical and Laboratory Standards Institute (1997, 2006, and 2010). The pathogenic strains are namely Pseudomonas aeruginosa, Escherichia coli, Klebsiella pneumoniae, Enterococcus faecalis, Proteus mirabilis, Staphylococcus epidermidis, Staphylococcus aureus, Candida albicans, Candida tropicalis, and Candida parapsilosis. Minimum inhibitory concentrations (MICs) of the extract and the standard compounds were determined by the micro broth dilution technique as described by the CLSI (1997, 2006, and 2010). Cefuroxime-sodium, cefuroxime, ceftazidime, amikacin, amphotericin B, and clotrimazole were used as standard antibacterial and antifungal agents.
2.8 Cytotoxic Activities
2.8.1 Cytotoxicity Assay on Renal and Colon Cell Lines
A two-day assay was used to determine the cytotoxic potential of the extracts. XTT bioassay is an in vitro antitumor colorimetric assay developed by the Molecular Targets Program (MTP), Assay Development and Screening Section, National Cancer Institute (NCI). The renal cancer cell lines (UO-31 and A498) and the colon cancer cell lines (COLO205 and KM12) were used in the assay. All details of the assay were performed according to Eroglu Ozkan et al., 2020.
2.8.2 Metastatic Potential Assay
The metastatic potential of the extracts was identified by using the XTT assay, developed by the Molecular Targets Program (MTP), Assay Development and Screening Section, National Cancer Institute (NCI), via comparing the effects of the extracts on high (MG63.3) and low (MG63) metastatic potential osteosarcoma cell lines. The cells were plated in tissue culture plates and allowed to attach overnight followed by a 2-day treatment with the extracts. Relative cell numbers are assessed using the XTT assay. The results were evaluated to determine which extract has activity ≤50% for MG63.3 and ≥50% for MG63, and the difference between the two values must be ≥ 50% (Eroglu Ozkan et al., 2020).
2.9 Statistical Analysis
All measurements were repeated three times. The results were evaluated using a t-test with Microsoft Excel and expressed as mean ± standard deviation. Differences were considered significant at p < 0.05.
3 Results and Discussion
3.1 LC-MS/MS Analysis of the Extracts
3.1.1 LC-MS/MS Method Validation
The LC-MS/MS method validation was carried out by a previous study (Yilmaz et al., 2018). The details were given in the following sections.
3.1.1.1 Linearity
The linearity was assayed using external standard calibration curve with six concentration levels for each analyte, and each concentration level was assayed in triplicate. The developed method showed to be linear for all compounds, between the ranges of tested concentrations during the validation of the method with R2 ≥ 0.989. The equations for the calibration curves and the determination coefficients (R2) are shown in Supplementary Table S2.
3.1.1.2 Accuracy (Recovery) and Precision (Repeatability)
For intra-day variability assessment, spiked samples were measured for six replicates within a single day, whereas spiked samples were examined in triplicate per day for three consecutive days to conduct inter-day assay. As a result of the studies conducted on the same day and on different days, recovery and % RSD values were calculated to determine the accuracy and precision (Supplementary Table S2).
The recovery was calculated with the following equation:
3.1.1.3 Limits of Detection and Quantification
To determine the LOD and LOQ values for the phytochemicals used in the LC-MS/MS method, analyte mixture was spiked at 10 identical samples prepared from a selected extract at the lowest concentration signaled by the standards and injected to the instrument.
LOD and LOQ values were calculated according to the following equations (Supplementary Table S2):
3.1.1.4 Relative Standard Uncertainty (U95)
Standard uncertainties of the analytes were determined by the accuracy (recovery) and precision (repeatability) studies according to EURACHEM Guide (2012).
3.1.2 LC-MS/MS Analysis Results of the Extracts
As mentioned, the studied T. praecox subsp. grossheimii (Ronniger) Jalas, T. pubescens Boiss. et Kotschy ex Celak, and T. cariensis Hub-Mor. & Jalas samples were collected from Turkey. Five ethanol extracts were prepared from the collected samples (aerial parts and roots extracts from T. praecox subsp. grossheimii (Ronniger) Jalas, and T. pubescens Boiss. et Kotschy ex Celak, and aerial parts extract from T. cariensis Hub-Mor. & Jalas) and the extract yield of the samples were presented in Table 1. Aiming to identify their chemical compositions, An LC-MS/MS analysis was carried out, and the obtained results are present in Table 2.
Twelve biologically important secondary metabolites were determined in all studied extracts with different concentrations: p-coumaric acid (28 ± 1.44–98.55 ± 5.09 μg/g extract), caffeic acid (5.87 ± 0.21–632.06 ± 22.37 μg/g extract), salicylic acid (18.14 ± 0.60–83.04 ± 2.73 μg/g extract), quinic acid (1077.98 ± 8.84–8,374.43 ± 68.67 μg/g extract), fumaric acid (461.82 ± 5.73–1715.45 ± 21.27 μg/g extract), vanillin (18.74 ± 0.52–163.68 ± 4.58 μg/g extract), malic acid (510.17 ± 5.76–1141.92 ± 12.90 μg/g extract), hesperetin (2.37 ± 0.13–16.48 ± 0.93 μg/g extract), naringenin (26.92 ± 1.40–2226.14 ± 115.98 μg/g extract), rutin (0.59 ± 0.01–51.76 ± 0.82 μg/g extract), apigenin (94.81 ± 6.16–593.43 ± 38.57 μg/g extract), and nicotiflorin (4.16 ± 0.11–1241.4 ± 34.26 μg/g extract).
In aerial part ethanol extract of T. cariensis Hub-Mor. & Jalas (TcA), 15 different compounds were detected. The extract was found to be the richest in terms of p-coumaric acid (98.55 ± 5.09 μg/g extract), rosmarinic acid (2501.3 ± 178.34 μg/g extract), protocatechuic acid (1463.02 ± 60.13 μg/g extract), and fumaric acid (1715.45 ± 21.27 μg/g extract). Moreover, the amounts of quinic acid (6548.64 ± 53.70 μg/g extract), malic acid (855.65 ± 9.67 μg/g extract), and caffeic acid (322.7 ± 11.42 μg/g extract) were found to be quite abundant in the extract. Salicylic acid (18.14 ± 0.60 μg/g extract), vanillin (28.05 ± 0.79 μg/g extract), hesperetin (2.37 ± 0.13 μg/g extract), naringenin (54.07 ± 2.82 μg/g extract), rutin (35.25 ± 056 μg/g extract), rhoifolin (66.22 ± 6.23 μg/g extract), and nicotiflorin (4.16 ± 0.11 μg/g extract) were the further constituents of TcA. In a previous report about the determination of phenolic compounds of the acetone and methanol extracts obtained from T. cariensis Hub-Mor. & Jalas by HPLC-DAD, luteolin (68.37 ± 3.62 mg/g), rosmarinic acid (26.51 ± 1.87–30.13 ± 2.63 mg/g), and fumaric acid (6.31 ± 0.42 mg/g) were the major compounds (Kucukaydin et al., 2021). Another study was conducted to investigate the main components of T. cariensis Hub-Mor. & Jalas hexane extract by GC-FID, and accordingly, linolenic acid (22.65%), linoleic acid (14.37%), behenic acid (12.54%), and palmitic acid (15.08%) were detected in the hexane extract, respectively (Kucukaydin et al., 2020).
T. praecox subsp. grossheimii (Ronniger) Jalas aerial parts extract (TprA) was shown to contain 16 different phytochemicals. The extract was the richest in terms of quinic acid with 8,374.43 ± 68.67 μg/g extract. The amounts of rosmarinic acid (2166.60 ± 154.48 μg/g extract), malic acid (624.59 ± 7.06 μg/g extract), fumaric acid (552.98 ± 6.86 μg/g extract), apigenin (321.49 ± 20.90 μg/g extract), caffeic acid (250.74 ± 8.88 μg/g extract), nicotiflorin (244.43 ± 6.75 μg/g extract), and naringenin (163.62 ± 8.52 μg/g extract) were also noteworthy in TprA. T. praecox subsp. grossheimii (Ronniger) Jalas roots extract (TprR) was revealed to consist of 13 compounds by this analysis. This was the most abundant extract in terms of tr-ferulic acid (85.09 ± 4.20 μg/g extract), and vanillin (163.68 ± 4.58 μg/g extract). p-coumaric acid (28 ± 1.44 μg/g extract), caffeic acid (5.87 ± 0.21 μg/g extract), salicylic acid (21.1 ± 0.69 μg/g extract), quinic acid (1077.98 ± 8.84 μg/g extract), fumaric acid (641.36 ± 7.95 μg/g extract), malic acid (616.98 ± 6.97 μg/g extract), naringenin (26.92 ± 1.40 μg/g extract), apigenin (94.81 ± 6.16 μg/g extract), and nicotiflorin (90.84 ± 2.51 μg/g extract) were also screened at meaningful amounts in the extract. Since T. praecox has several subspecies and varieties botanically, investigating their chemical compositions comparatively has been the goal of several previous studies, which are worth mentioning here in this context. For instance, a previous HPLC analysis of T. praecox subsp. grossheimii (Ronniger) Jalas suggested that gallic acid, protocatechuic aldehyde, chlorogenic acid, caffeic acid, vanillin, ferulic acid, and rosmarinic acid were screened in the methanol extract of the plant (Burnaz et al., 2017). In another study, ursolic acid, oleanolic acid rosmarinic acid and its derivatives, luteolin 5-O-β-D-glucopyranoside, and thymoquinol 2,5-O-β-diglucopyranoside were isolated from the aerial parts of T. praecox subsp. grossheimii var. grossheimii, which is another variety of the plant (Sevindik et al., 2015). Furthermore, rosmarinic acid, caffeic acid, luteolin-7-rutinoside, luteolin-7-glucoside, and apigenin-7-glucoside were found to be present in ethanol extract of another T. praecox subspecies, namely Thymus praecox ssp. arcticus (E. Durand), Jalas by HPLC–UV (Raudone et al., 2017). Another HPLC-UV analysis was carried out this time on T. praecox subsp. caucasicus var. caucasicus, indicating that quercetin, luteolin, and caffeic acid were the major constituents in the studied extracts along with different phenolic compounds such as p-coumaric acid, protocatechuic acid, and catechin (Turumtay et al., 2014). The screening of T. praecox subsp. skorpilii var. skorpilii (Velen.) Jalas methanol extract by HPLC-DAD and LC-MS/MS techniques showed the presence of chlorogenic acid, luteolin-7-O-glucoside, 3-O-feruloylquinic acid, quercetin-3-O-hexoside, and apigenin-7-O-glucuronide (Taskin et al., 2019).
The aerial parts extract of T. pubescens Boiss. et Kotschy ex Celak (TpuA) is undoubtedly the richest extract among the five studied, consisting of 21 different biologically active phytochemicals. Incidentally, quercitrin (256.98 ± 5.16 μg/g extract), chlorogenic acid (70.34 ± 0.49 μg/g extract), kaempferol (67.32 ± 1.41 μg/g extract), and chrysin (2.72 ± 0.05 μg/g extract) were the compounds that are only present in this extract. On top of that, being the richest in terms of naringenin (2226.14 ± 115.98 μg/g extract), caffeic acid (632.06 ± 22.37 μg/g extract), apigenin (593.43 ± 38.57 μg/g extract), hesperidin (233.21 ± 6.11 μg/g extract), malic acid (1141.92 ± 12.90 μg/g extract), hesperetin (16.48 ± 0.93 μg/g extract), rutin (51.76 ± 0.82 μg/g extract) isoquercitrin (205.75 ± 2.74 μg/g extract) rhoifolin (542.43 ± 51.04 μg/g extract), and nicotiflorin (1241.4 ± 34.26 μg/g extract), TpuA can be considered as a valuable source in the search for plant-derived natural compounds. In the root extract of the plant (TpuR), 16 secondary metabolites were shown to be present. The extract was to contain a significant amount of important phenolic acids, such as quinic acid (2103.87 ± 17.25 μg/g extract), rosmarinic acid 1026.79 ± 73.21 μg/g extract), malic acid (510.17 ± 5.76 μg/g extract), caffeic acid (504.09 ± 17.84 μg/g extract), and fumaric acid (461.82 ± 5.73 μg/g extract). Although the data about the chemical composition of T. pubescens Boiss. et Kotschy ex Celak extracts are rather scarce and the research is mainly focused on its volatile compounds, according to a previous study, rosmarinic acid was the main constituent of T. pubescens Boiss. et Kotschy ex Celak, and gallic acid, caffeic acid, epicatechin, luteolin, p-coumaric acid, ferulic acid, cinnamic acid, apigenin, naringenin, and salvianolic acid were also detected in the plant extract (Sarfaraz et al., 2021).
As known, the pharmacological activities of the plants are attributed to their naturally occurring phytochemicals. Polyphenols, in particular, have shown to possess significant pharmacological properties that are effective for modulating human metabolism in a manner favorable for the treatment of several ailments, as well as prevention or reduction in the risk of degenerative diseases. Within this framework, reported by the current study for the first time, the studied Thymus species showed the important presence of various phenolic compounds that could be beneficial for human health.
3.2 Total Phenolic and Flavonoid Contents of the Extracts
Total phenolic contents of the extracts were calculated from pyrocatechol calibration curve (y = 0.0408x + 0.0549, R2 = 0.9924) and expressed as µgs of pyrocatechol equivalents (PEs), and total flavonoid contents of the extracts were calculated from quercetin calibration curve (y = 0.0325x + 0.0601, R2 = 0.9984), and expressed as µgs of quercetin equivalents (QEs). The results are shown in Table 3.
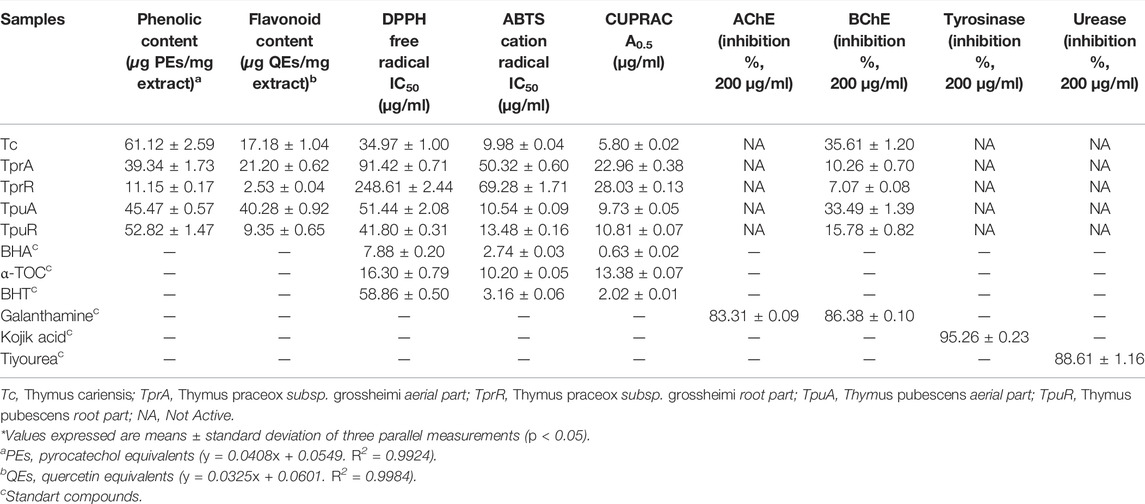
TABLE 3. Total phenolic, flavonoid contents, antioxidant activities, and enzyme inhibition activities of the studied Thymus extracts*.
Regarding the phenolic content, TcA was found as the richest extract with 61.12 ± 2.59 μg PEs/mg extract. T. pubescens Boiss. et Kotschy ex Celak extracts were reported to contain a higher amount of phenolic compounds compared to the T. praecox subsp. grossheimii (Ronniger) Jalas extracts. TpuR was the richest extract among them with 52.82 ± 1.48 μg PEs/mg extract. TpuA (45.47 ± 0.57 μg PEs/mg extract), TprA (39.34 ± 1.73 μg PEs/mg extract), and TprR (11.15 ± 0.17 μg PEs/mg extract) were also found to contain good amount of polyphenols. In terms of flavonoid content, TpuA was the richest extract with 40.28 ± 0.92 μg QEs/mg extract. Total flavonoid contents of other extracts were determined as TprA: 21.20 ± 0.62 μg QEs/mg extract, TcA: 17.18 ± 1.04 μg QEs/mg extract, TpuR: 9.35 ± 0.65 μg QEs/mg extract, and TprR: 2.53 ± 0.04 μg QEs/mg extract, respectively.
Taking a look at the previous studies conducted in this field, the total phenolic and flavonoid contents of the extracts of T. cariensis Hub-Mor. & Jalas and T. cilicicus Boiss. & Balansa were calculated. The phenolic contents of the extracts were reported to range from 42.74 ± 0.12 to 113.61 ± 0.15 μg PEs/mg, and the flavonoid contents of the extracts were reported to range from 29.57 ± 0.01 to 175.47 ± 0.21 μg QEs/mg (Kucukaydin et al., 2021). T. pubescens Boiss. et Kotschy ex Celak were found to contain 295.57 ± 1.91 μg rutin/mg extract of phenolic compounds, and 50.39 ± 0.75 μg rutin/mg extract of flavonoids (Nickavar and Esbati, 2012). In another study, total phenolic and flavonoid contents of the extracts were obtained separately from the leaves, flowers, and stems of T. praecox subsp. caucasicus var. caucasicus (Willd. ex Ronniger) Jalas were calculated. The highest phenolic content was determined in the hydrolyzed-flower extract with 27.634 ± 1.677 mg GAE g−1, and the highest flavonoid content was determined in the hydrolyzed-leaf extract with 23.639 ± 1.06 mg QE g−1. As expected, the standard mix extracts with the total plant were found to contain the highest amount of phenolics (1341.743 ± 18.710 mg GAE g−1), and flavonoids (380.519 ± 5.132 mg QE g−1) (Turumtay et al., 2014). Other Thymus L. species were also subjected to numerous studies and their total phenolic and flavonoid contents were calculated. For instance, T. convolutus Klokov, T. fallax Fisch. & C. A. Mey., T. haussknechtii Velen, T. kotschyanus var. kotschyanus Boiss. & Hohen, and T. sipyleus subsp. sipyleus var. sipyleus Boiss from Turkey were investigated in this context, phenolic contents of the extracts were found to range from 35.25 ± 0.75 to 96.58 ± 0.86 μg PEs/mg extract, and flavonoid contents of the extracts were shown to range from 1.71 ± 0.06 to 45.30 ± 0.84 μg QEs/mg extract (Boga et al., 2021a). Although the identification of phenolic compounds as the constituents of different plant extracts could only be possible by specific analytical techniques including LC-MS/MS or HPLC, using low-cost photometric assays such as the methods used in this study, it is possible to determine the sub-groups sharing basic chemical structures (Csepregi et al., 2013). To evaluate the antioxidant potential of the extracts, calculating the total phenolic and flavonoid contents is a crucial step since the antioxidant activity is usually attributed to the phenolics present in the extracts, and is considered a hallmark for important biological activities (Ersoy et al., 2020; Eroglu Ozkan et al., 2021).
3.3 Antioxidant Activity of the Extracts
Three different methods were applied to investigate the in vitro antioxidant activity of the studied Thymus extracts. Using various methods and evaluating all the gathered data altogether are crucial steps to considering every oxidation aspect (Boga et al., 2021b) The results are presented in Table 3.
TcA demonstrated the strongest antioxidant activity in all three assays. According to the DPPH radical scavenging activity assay results, the IC50 value of TcA was 34.97 ± 1.00 μg/ml, which is higher than the other four extracts and also one of the standard compounds, namely BHT (58.86 ± 0.50 μg/ml). ABTS cation radical assay was the second procedure, and TcA (9.98 ± 0.04 μg/ml) showed stronger antioxidant activity than the standard compound α-TOC (10.20 ± 0.05 μg/ml), and the other three extracts once again. Similar results were obtained from the CUPRAC assay, A0.5 values were calculated here and TcA (5.80 ± 0.02 μg/ml) was found to be more active than the standard α-TOC (13.38 ± 0.07 μg/ml) and the other extracts. On the other hand, all T. pubescens Boiss. et Kotschy ex Celak extracts appeared to possess better antioxidant capacities than T. praceox subsp. grossheimii (Ronniger) Jalas extracts according to the results of all three assays.
Understanding the antioxidant properties of thyme is of utmost importance because the antioxidant molecules play a protective role in the pathogenesis of major disease burdens such as cancer, Alzheimer’s, or diabetes mellitus. The balance between the oxidative and reducing species must be maintained in healthy humans, where oxidative stress leads to the overproduction of reactive species, therefore causing the imbalance and emerging diseases. Even supposing antioxidant supplements cannot be served as medications, it must be noted that they indirectly help to combat these diseases and consequently be beneficial for the healthspan and lifespan extension (Conti et al., 2016; Tan et al., 2018).
Total phenolic content has been reported to be a good predictor of the antioxidant activity of plant extracts, which is positively correlated with antioxidant activity (Li et al., 2018). The current study also showed parallelism with this finding since the extract of T. cariensis Hub-Mor. & Jalas contained the highest total phenolic content and demonstrated the strongest antioxidant activity among all studied extracts. The antioxidant potentials of various Thymus species have been investigated in countless studies. Although the research has mainly been focused on the essential oil of thyme, extracts, and isolated compounds such as thymol have also been investigated for their antioxidant capacities (Dauqan et al., 2017; Nagoor Meeran et al., 2017; Salehi et al., 2018; Lorenzo et al., 2019). Regarding all data acquired from these studies, thyme in general is much more important than a basic nutrient or a taste enhancer only. With its rich phenolic content and strong antioxidant properties, thyme has been revealed as a food preservative and also a functional food that provides outstanding benefits to human health (Boga et al., 2021a). Incidentally, it has been confirmed by a recent study that the infusion and decoction of thyme could be achieved by internal as well as external use for the treatment of severe diseases caused by the reactive oxygen, nitrogen, and sulfur species production and oxidative stress with its antioxidant activity and unique phenolic characterization (Martins et al., 2015).
It must be noted that there are divergent opinions about the possibility of predicting the antioxidant activity of the plant extracts by employing in vitro antioxidant screening methods, which are mainly colorimetric methods that have widespread applications (Boga et al., 2021a). Although recent studies have shown that colorimetric methods are still considered as very important tools in terms of antioxidant activity investigation, further studies are required to prove more solid evidence in this regard.
3.4 Enzyme Inhibition Activity of the Extracts
The enzyme inhibitory potentials of TcA, TprA, TprR, TpuA, and TpuR were assessed by antiacetylcholinesterase, antibutyrylcholinesterase, antityrosinase, and antiurease activity assays at 200 μg/ml concentration. The results are given in Table 3.
The anticholinesterase activity of the extracts was investigated using Ellman’s colorimetric method. Accordingly, the studied Thymus extracts did not demonstrate any inhibitory activity on the acetylcholinesterase enzyme. Taking a glance at previous studies in this regard, T. cariensis Hub-Mor. & Jalas extracts prepared with different solvents were found to show moderate to low anticholinesterase activity. For instance, the IC50 value of the methanol extract was 68.65 ± 0.08 μg/ml, whereas the acetone extract inhibited AChE with 87.10 ± 0.25 μg/ml, and the hexane extract with 190.2 ± 0.18 μg/ml, respectively (Kucukaydin et al., 2021). There have also been several studies carried out to evaluate the AChE inhibitory potential of different Thymus species, and they were shown to exert antiacetylcholinesterase activity in a dose-dependent manner (Kindl et al., 2015; Taskin et al., 2019). However, after a more detailed literature survey, it has appeared that the ethanol extracts of Thymus species were found to demonstrate very low or no inhibitory effect on AChE. In Orhan et al.’s study, dichloromethane and ethyl acetate extracts of T. praecox subsp. caucasicus var. caucasicus was shown to be moderately active, whereas the ethanol extract was comparatively much less effective (Orhan et al., 2009). In Ertas et al.’s study, methanol extract of T. nummularius M. Bieb. demonstrated weak AChE inhibitory activity with 13.31 ± 0.21% at 200 μg/ml, however, the antiacetylcholinesterase potential of the essential oil of the plant was higher with 60.05 ± 0.37%, which was concluded by the discussion that this difference was attributed to a specific component, thymol, which was present in the essential oil but not in the extract of T. nummularius M. Bieb. (Ertas et al., 2015). Ceylan et al. (2016) also indicated that the presence of thymol and carvacrol is required for anti-AChE activity. Another example of this situation could be observed in Bendjabeur et al. (2018)’s study as well, where T. algeriensis Boiss. & Reut ethanol extract was completely inactive on AChE, but the essential oil was weakly active on the same enzyme with an IC50 value of 98.84 ± 1.81 μg/ml. Moreover, ethanol extracts of the aerial parts and roots of five different thyme species were also shown to have no AChE inhibitory potential (Boga et al., 2021a). This may explain the lack of activity.
Differently from AChE inhibition activity results, all extracts could inhibit BChE weakly or moderately (7.07 ± 0.08–35.61 ± 1.20%). The abovementioned studies except for Orhan et al. (2009)’s study, investigate the antibutyrylcholinesterase potential of different Thymus species as well. It can be seen the extracts were slightly more effective on BChE in comparison to their anti-AChE effects.
The studied five Thymus extracts were found to show no inhibitory activity on both tyrosinase and urease enzymes (Table 4). Same as the anticholinesterase activity, essential oils obtained from different Thymus species were shown to possess antityrosinase potential owing to a high level of thymol present in the oils (Ceylan et al., 2016). T. argaeus (Fisch. & C.A.Mey.) Boiss. & Balansa methanolic extract was found to exert anti-tyrosinase activity with 121.52 mg KAE/g sample, whereas the decoction of the plant exerted lesser activity with 55.29 mg KAE/g sample (Zengin et al., 2019). Galuteolin, a secondary metabolite that was found in the flower extract of T. quinquecostatus Celak. demonstrated significant anti-tyrosinase activity (Kim et al., 2021). In another study, again phytochemicals isolated from T. vulgaris L. such as 6′,3′,4′-trihydroxy-5,5′-diisopropyl-2,2′-dimethylbiphenyl, thymol, oleanolic acid, ursolic acid, cirsimaritin, and xanthomicrol were capable of inhibiting tyrosinase, which also proves only specific compounds are responsible for the tyrosinase inhibitory activity and this activity is only expected when they are present in the extracts (Stefanis et al., 2019). The same conclusion is also accurate for the anti-urease activity and reported by Boga et al. (2021a)’s study again, no tyrosinase urease activity was demonstrated by the investigated ten Thymus L. ethanol extracts.
3.5 Cytotoxic Activity and Metastatic Potential of the Extracts
The cytotoxic potential of the extracts was evaluated on COLO205 and KM12 human colon, UO-31, and A498 human renal cancer cell lines, and the metastatic potential of the extracts was evaluated on MG633 high metastatic and MG63 low metastatic osteosarcoma cancer cell lines by cell viability assays. The results were summarized in Table 4. Evaluating the results, it can be seen that TprA exhibited moderate cytotoxic activity on A498 renal cell lines with 61.92% cell viability. Roots extract of the same plant, TprR also exerted moderate cytotoxic activity on COLO205 human colon cancer cell lines with 68.43% cell viability after 2 days. TpuA and TpuR extracts were also effective in terms of cytotoxicity on A498 human renal cancer cell lines with 65.31% and 68.33%, respectively. On KM12 colon cancer cell lines, only TprR extract demonstrated moderate-to-low cytotoxic activity with 75.71%. None of the extracts was shown to possess metastatic potential, also T. cariensis Hub-Mor. & Jalas extract was found to be ineffective on all the studied cell lines.
Despite the fact that enormous scientific endeavors have been made to have a better understanding of cancer, which is the second leading cause of death, in order to combat this disease, there is still an urgent need for further anti-cancer agents. Natural and plant-derived compounds with their anti-cancer properties have aroused the attention of researchers in this context. Thymus species with their well-known pharmacological effects and unique chemistry have also been investigated for their cytotoxic activity. Although the anti-cancer research has been mainly focused on different essential oils obtained from different Thymus species, recently the extracts of these plants have also been studied. T. vulgaris L., the undoubtedly most investigated species, was shown to have a cytotoxic effect on H460 large cell lung carcinoma, THP-1 human leukemia, Caco-2 human colon adenocarcinoma, and HepG2 human hepatocellular carcinoma cell lines (Ayesh et al., 2014; Oliviero et al., 2016; Taghouti et al., 2020). T. caramanicus Jalas demonstrated cytotoxic activity on KB human oral epidermoid carcinoma cell lines, also the extract was shown to potentiate the effect of the anti-cancer drug, doxorubicin (Fekrazad et al., 2017). T. kotschyanus var. kotschyanus extract was found to decrease the cell viability of A498 renal cancer cell lines by 61.77% in another study (Boga et al., 2021a). T. cariensis Hub-Mor. & Jalas was also studied for its cytotoxic activity. Methanol and acetone extracts were shown to have anti-cancerous effects on MCF-7 breast cancer cell lines, whereas the hexane extract was found to be ineffective (Kucukaydin et al., 2021a). This is important because it proves the direct effect of the extracting solvent on the cytotoxic activity. Another noteworthy finding was in terms of cytotoxicity, thyme extracts could potentially be active on human cancer cell lines but not on healthy cells. This selectiveness is rather meaningful and requires further studies to understand the pathways mediating the anti-cancer effect (Patil et al., 2021).
3.6 Antimicrobial Activity of the Extracts
The extracts, TcA, TprA, TprR, TpuA, and TpuR were investigated for their antimicrobial activity against a standard panel of pathogenic bacteria and fungi. The results are given as MIC values, and they are shown in Table 5.
All studied extracts were found to be significantly effective on C. tropicalis, which is a well-known human pathogen associated with quite high mortality rates (Boga et al., 2021a). In terms of antifungal activity against C. tropicalis, TpuA was the most effective with 19.53 μg/ml, and TcA, TprA, TpuR were the second most effective with 39.06 μg/ml. TprR also exhibited antifungal activity against C. tropicalis with 78.12 μg/ml.
In previous studies, T. mastichina (L.) L. showed antimicrobial activity against several bacteria such as E. coli, P. mirabilis, Salmonella subsp., S. aureus, MRSA, L. monocytogenes EGD, B. cereus, Candida spp., and Fusarium spp. (Rodrigues et al., 2020). The nonpolar extracts of the aerial parts T. eigii were found to exert moderate antimicrobial activity, whereas polar extracts did not show any activity against a standard panel of pathogenic bacteria and fungi (Tepe et al., 2004). In another study, antimicrobial activity of T. boissieri Halácsy, T. longicaulis C. Presl, T. leucospermus Hartvig, and T. ocheus Heldr. & Sart. ex Boiss. extracts. The extracts exerted moderate antimicrobial activity, however, after encapsulating the extracts in liposomes, the antimicrobial effect was significantly increased. This may be a useful technique when stronger antimicrobial efficacy was aimed from Thymus extracts (Gotzi et al., 2006). Reviewing the literature about the antimicrobial activity of the essential oils and extracts obtained from Thymus species, it can be observed that essential oils demonstrate superior antimicrobial activity compared to the extracts. Speaking of which, a study about the evaluation of T. vulgaris L. essential oil and plant extracts for antimicrobial properties indicated that the extracts did not show any activity, whereas essential oil was found to possess antimicrobial properties (Gedikoglu et al., 2019). Essential oil and methanol extract of T. hirtus subsp. algeriensis (Boiss. and Reut.) Murb were also investigated for their antimicrobial activities and the results showed parallelism with the other findings and only the essential oil exhibited inhibitory activity on the growth of both Gram (+) and Gram (−) bacteria (Fatma et al., 2014). T. daenensis Celak is also among Thymus L. species with proven antimicrobial properties, whose essential oil was found to be more effective than several extracts (Zarshenas and Krenn, 2015). In a nutshell, it is a very well-known fact that essential oils obtained from Thymus L. species are not only better investigated, but also stronger antimicrobial agents than other Thymus extracts.
4 Conclusion
With the help of newly developed techniques in the field of related sciences, important pharmacological properties of different Thymus L. species including have been proved and more light was shed on their unique chemical characterizations. The current study provides data about chemical profiling and biological activities of three species namely T. cariensis Hub-Mor. & Jalas, T. praceox subsp. grossheimii (Ronniger) Jalas, and T. pubescens Boiss. et Kotschy ex Celak from Turkey. All studied extracts were shown to contain pharmacologically valuable phytochemicals such as hesperetin (2.37 ± 0.13–16.48 ± 0.93 μg/g extract), naringenin (26.92 ± 1.40–2226.14 ± 115.98 μg/g extract), rutin (0.59 ± 0.01–51.76 ± 0.82 μg/g extract), apigenin (94.81 ± 6.16–593.43 ± 38.57 μg/g extract), and demonstrate important biological activities including antioxidant, antityrosinase and antimicrobial effects, meaning these species could be beneficial for human health in various aspects. Since screening chemical constituents with the assessment of the biological activities are the crucial steps to having a better understanding towards new possible therapeutic agents derived from Thymus L. species, this assessment may contribute to the related sciences including pharmacognosy. Having said that, further studies including clinical trials are undoubtedly required to shed more light on the therapeutic properties of these species.
Data Availability Statement
The original contributions presented in the study are included in the article/Supplementary Material, further inquiries can be directed to the corresponding authors.
Author Contributions
EEO: supervision, performed the experiments and statistical analysis, writing—review, and editing. EE: performed the experiments and statistical analysis, writing—original draft, review, and editing. YY: collected and identified the plant material. EM: performed the antimicrobial activity studies. SK: performed the activity studies. EC: performed the activity studies. HS: performed the activity studies. MY: performed the LC-MS/MS studies. KK: writing—review, and editing. MB: supervision, performed the experiments and statistical analysis, writing—review, and editing. All authors read and approved the final manuscript.
Conflict of Interest
The authors declare that the research was conducted in the absence of any commercial or financial relationships that could be construed as a potential conflict of interest.
The handling editor GZ declared a past co-authorship with the authors EE, EEO, EC, EMK, MB, and YYC.
Publisher’s Note
All claims expressed in this article are solely those of the authors and do not necessarily represent those of their affiliated organizations, or those of the publisher, the editors and the reviewers. Any product that may be evaluated in this article, or claim that may be made by its manufacturer, is not guaranteed or endorsed by the publisher.
Supplementary Material
The Supplementary Material for this article can be found online at: https://www.frontiersin.org/articles/10.3389/fphar.2022.923063/full#supplementary-material
References
Apak, R., Güçlü, K., Özyürek, M., and Karademir, S. E (2004). Novel Total Antioxidant Capacity Index for Dietary Polyphenols and Vitamins C and E, Using Their Cupric Ion Reducing Capability in the Presence of Neocuproine: CUPRAC Method. J. Agric. Food Chem. 52 (26), 7970–7981.
Arslan Burnaz, N., Küçük, M., and Akar, Z. (2017). An On-Line HPLC System for Detection of Antioxidant Compounds in Some Plant Extracts by Comparing Three Different Methods. J. Chromatogr. B Anal. Technol. Biomed. Life Sci. 1052, 66–72. doi:10.1016/j.jchromb.2017.03.003
Ashrafi, M., Azimi-Moqadam, M.-R., MohseniFard, E., Shekari, F., Jafary, H., Moradi, P., et al. (2022). Physiological and Molecular Aspects of Two Thymus Species Differently Sensitive to Drought Stress. BioTech 11 (2), 8. doi:10.3390/biotech11020008
Ayesh, B. M., Abed, A. A., and Faris, D. M. (2014). In Vitro inhibition of Human Leukemia THP-1 Cells by Origanum Syriacum L. And Thymus Vulgaris L. Extracts. BMC Res. Notes 7 (1), 612–616. doi:10.1186/1756-0500-7-612
Bendjabeur, S., Benchabane, O., Bensouici, C., Hazzit, M., Baaliouamer, A., and Bitam, A. (2018). Antioxidant and Anticholinesterase Activity of Essential Oils and Ethanol Extracts of Thymus Algeriensis and Teucrium Polium from Algeria. Food Meas. 12 (4), 2278–2288. doi:10.1007/s11694-018-9845-x
Blois, M. S. (1958). Antioxidant Determinations by the Use of a Stable Free Radical. Nature 181 (4617), 1199–1200.
Boga, M., Ertas, A., Eroglu-Ozkan, E., Kizil, M., Ceken, B., and Topcu, G. (2016). Phytochemical Analysis, Antioxidant, Antimicrobial, Anticholinesterase and DNA Protective Effects of Hypericum Capitatum var. Capitatum Extracts. S. Afr. J. Bot. 104, 249–257.
Boga, M., Ersoy, E., Eroglu Ozkan, E., Cinar, E., Mataraci Kara, E., Yesil Canturk, Y., et al. (2021b). Volatile and Phenolic Profiling of a Traditional Medicinal Plant, Hypericum Empetrifolium with In Vitro Biological Activities. J. Ethnopharmacol. 272, 113933. doi:10.1016/j.jep.2021.113933
Boga, M., Ozkan, E. E., Ersoy, E., Tuncay, E., Canturk, Y. Y., Cinar, E, et al. (2021a). Identification and Quantification of Phenolic and Volatile Constituents in Five Different Anatolian Thyme Species Using LC-MS/MS and GC-MS, with Biological Activities. Food Biosci. 43, 101141. doi:10.1016/j.fbio.2021.101141
Bonini, S. A., Premoli, M., Tambaro, S., Kumar, A., Maccarinelli, G., Memo, M., et al. (2018). Cannabis Sativa: A Comprehensive Ethnopharmacological Review of a Medicinal Plant with a Long History. J. Ethnopharmacol. 227, 300–315. doi:10.1016/j.jep.2018.09.004
Ceylan, R., Zengin, G., Uysal, S., Ilhan, V., Aktumsek, A., Kandemir, A., et al. (2016). GC-MS analysis and In Vitro antioxidant and enzyme inhibitory activities of essential oil from aerial parts of endemic Thymus spathulifolius Hausskn. et Velen. J. Enzyme Inhib. Med. Chem. 31 (6), 983–990. doi:10.3109/14756366.2015.1077822
Chassagne, F., Samarakoon, T., Porras, G., Lyles, J. T., Dettweiler, M., Marquez, L., et al. (2021). A Systematic Review of Plants with Antibacterial Activities: a Taxonomic and Phylogenetic Perspective. Front. Pharmacol. 2069. doi:10.3389/fphar.2020.586548
Choudhari, A. S., Mandave, P. C., Deshpande, M., Ranjekar, P., and Prakash, O. (2019). Phytochemicals in Cancer Treatment: From Preclinical Studies to Clinical Practice. Front. Pharmacol. 10, 1614. doi:10.3389/fphar.2019.01614
Conti, V., Izzo, V., Corbi, G., Russomanno, G., Manzo, V., De Lise, F., et al. (2016). Antioxidant Supplementation in the Treatment of Aging-Associated Diseases. Front. Pharmacol. 7, 24. doi:10.3389/fphar.2016.00024
Csepregi, K., Kocsis, M., and Hideg, E. (2013). On the Spectrophotometric Determination of Total Phenolic and Flavonoid Contents. Acta Biol. Hung 64 (4), 500–509. doi:10.1556/ABiol.64.2013.4.10
Dauqan, E. M., and Abdullah, A. (2017). Medicinal and Functional Values of Thyme (Thymus Vulgaris L.) Herb. J. Appl. Biol. Biotechnol. 5 (2), 0–2.
Ellman, G. L., Courtney, K. D., Andres, V., and Featherstone, R. M. (1961). A New and Rapid Colorimetric Determination of Acetylcholinesterase Activity. Biochem. Pharmacol. 7 (2), 88–95.
Eroglu Ozkan, E., Seyhan, M. F., Kurt Sirin, O., Yilmaz-Ozden, T., Ersoy, E., Hatipoglu Cakmar, S. D., et al. (2021). Antiproliferative Effects of Turkish Pomegranate (Punica Granatum L.) Extracts on MCF-7 Human Breast Cancer Cell Lines with Focus on Antioxidant Potential and Bioactive Compounds Analyzed by LC-MS/MS. J. Food Biochem. 45 (9), e13904. doi:10.1111/jfbc.13904
Eroglu Ozkan, E., Boga, M., Yilmaz, M. A., Kara, E. M., and Yesil, Y. (2020). LC-MS/MS Analyses of Ziziphora Clinopodioides Lam. From Turkey: Antioxidant, Anticholinesterase, Antimicrobial and, Anticancer Activities. Istanb. J. Pharm. 50 (1), 33–41. doi:10.26650/IstanbulJPharm.2019.0064
Ersoy, E., Ozkan, E. E., Boga, M., Yilmaz, M. A., and Mat, A. (2019). Anti-aging Potential and Anti-Tyrosinase Activity of Three Hypericum Species with Focus on Phytochemical Composition by LC‐MS/MS. Ind. Crops Prod. 141, 111735.
Ersoy, E., Eroglu Ozkan, E., Boga, M., and Mat, A. (2020). Evaluation of In Vitro Biological Activities of Three Hypericum Species (H. Calycinum, H. Confertum, and H. perforatum) from Turkey. South Afr. J. Bot. 130, 141–147. doi:10.1016/j.sajb.2019.12.017
Ertas, A., Boga, M., Yilmaz, M. A., Yesil, Y., Tel, G., Temel, H., et al. (2015). A Detailed Study on the Chemical and Biological Profiles of Essential Oil and Methanol Extract of Thymus Nummularius (Anzer Tea): Rosmarinic Acid. Industrial Crops Prod. 67, 336–345. doi:10.1016/j.indcrop.2015.01.064
EURACHEM CITAC Guide CG4 (2012). Quantifiying Uncertainty in Analytical Measurement. Availableat: https://www.eurachem.org/index.php/publications/guides/quam#translations (Accessed 02 10, 2015).
Fatma, G., Mouna, B. F., Mondher, M., and Ahmed, L. (2014). In-vitro Assessment of Antioxidant and Antimicrobial Activities of Methanol Extracts and Essential Oil of Thymus Hirtus Sp. Algeriensis. Lipids Health Dis. 13 (1), 114. doi:10.1186/1476-511X-13-114
Fekrazad, R., Afzali, M., Pasban-Aliabadi, H., Esmaeili-Mahani, S., Aminizadeh, M., and Mostafavi, A. (2017). Cytotoxic Effect of Thymus Caramanicus Jalas on Human Oral Epidermoid Carcinoma KB Cells. Braz Dent. J. 28, 72–77. doi:10.1590/0103-6440201700737
Gedikoğlu, A., Sökmen, M., and Çivit, A. (2019). Evaluation of Thymus Vulgaris and Thymbra Spicata Essential Oils and Plant Extracts for Chemical Composition, Antioxidant, and Antimicrobial Properties. Food Sci. Nutr. 7 (5), 1704–1714.
Gortzi, O., Lalas, S., Chinou, I., and Tsaknis, J. (2006). Reevaluation of Antimicrobial and Antioxidant Activity of Thymus Spp. Extracts before and after Encapsulation in Liposomes. J. Food Prot. 69 (12), 2998–3005. doi:10.4315/0362-028x-69.12.2998
Güner, A., Aslan, S., Ekim, T., Vural, M., and Babaç, M. T. (2012). A Checklist of the Flora of Turkey (Vascular Plants). Istanbul, Turkey: Nezahat Gökyiğit Botanical Garden and Flora Research Association Publication. (in Turkish).
Haile, T., Cardoso, S. M., de Oliveira Raphaelli, C., Pereira, O. R., Pereira, E. D. S., Vizzotto, M., et al. (2021). Chemical Composition, Antioxidant Potential, and Blood Glucose Lowering Effect of Aqueous Extract and Essential Oil of Thymus Serrulatus Hochst. Ex Benth. Front. Pharmacol. 12, 858. doi:10.3389/fphar.2021.621536
Kim, M. H., Kwon, B., Kim, K. S., Kim, M. S., Kim, M. J., Kim, H. J., et al. (2021). Galuteolin, Identified in the Extract of Thymus Quinquecostatus Flowers, Is Involved in Inhibiting Melanin Biosynthesis in B16/F10 Melanoma Cells. Nat. Prod. Res. 35 (23), 5389–5391. doi:10.1080/14786419.2020.1768091
Kindl, M., Blažeković, B., Bucar, F., and Vladimir-Knežević, S. (2015). Antioxidant and Anticholinesterase Potential of Six Thymus Species. Evid. Based Complement. Altern. Med. 2015 (3), 403950. doi:10.1155/2015/403950
Küçükaydın, S., Çayan, F., Tel-Çayan, G., and Duru, M. E. (2021a). HPLC-DAD Phytochemical Profiles of Thymus Cariensis and T. Cilicicus with Antioxidant, Cytotoxic, Anticholinesterase, Anti-urease, Anti-tyrosinase, and Antidiabetic Activities. South Afr. J. Bot. 143, 155–163.
Küçükaydın, S., Tel-Çayan, G., Duru, M. E., Kesdek, M., and Öztürk, M. (2021b). Chemical Composition and Insecticidal Activities of the Essential Oils and Various Extracts of Two Thymus Species: Thymus Cariensis and Thymus Cilicicus. Toxin Rev. 40 (4), 1461–1471.
Li, X., He, T., Wang, X., Shen, M., Yan, X., Fan, S., et al. (2019). Traditional Uses, Chemical Constituents and Biological Activities of Plants from the Genus Thymus. Chem BiodiversChemistry Biodivers. 16 (9), e1900254. doi:10.1002/cbdv.201900254
Li, Z., Lee, H. W., Liang, X., Liang, D., Wang, Q., Huang, D., et al. (2018). Profiling of Phenolic Compounds and Antioxidant Activity of 12 Cruciferous Vegetables. Molecules 23 (5), 1139. doi:10.3390/molecules23051139
Lorenzo, J. M., Mousavi Khaneghah, A., Gavahian, M., Marszałek, K., Eş, I., Munekata, P. E. S., et al. (2019). Understanding the Potential Benefits of Thyme and its Derived Products for Food Industry and Consumer Health: From Extraction of Value-Added Compounds to the Evaluation of Bioaccessibility, Bioavailability, Anti-inflammatory, and Antimicrobial Activities. Crit. Rev. Food Sci. Nutr. 59 (18), 2879–2895. doi:10.1080/10408398.2018.1477730
Mahdavi, A., Moradi, P., and Mastinu, A. (2020). Variation in Terpene Profiles of Thymus Vulgaris in Water Deficit Stress Response. Molecules 25 (5), 1091. doi:10.3390/molecules25051091
Martins, N., Barros, L., Santos-Buelga, C., Silva, S., Henriques, M., and Ferreira, I. C. (2015). Decoction, Infusion and Hydroalcoholic Extract of Cultivated Thyme: Antioxidant and Antibacterial Activities, and Phenolic Characterisation. Food Chem. 167, 131–137. doi:10.1016/j.foodchem.2014.06.094
Michel, J., Abd Rani, N. Z., and Husain, K. (2020). A Review on the Potential Use of Medicinal Plants from Asteraceae and Lamiaceae Plant Family in Cardiovascular Diseases. Front. Pharmacol. 11, 852. doi:10.3389/fphar.2020.00852
Morales, R. (2002). The History, Botany and Taxonomy of the Genus Thymus. Thyme genus Thymus 1, 1–43.
Moreno, M. I. N., Isla, M. I., Sampietro, A. R., and Vattuone, M. A (2000). Comparison of the Free Radical-Scavenging Activity of Propolis From Several Regions of Argentina. J. Ethnopharmacol. 71 (1–2), 109–114.
Nagoor Meeran, M. F., Javed, H., Al Taee, H., Azimullah, S., and Ojha, S. K. (2017). Pharmacological Properties and Molecular Mechanisms of Thymol: Prospects for its Therapeutic Potential and Pharmaceutical Development. Front. Pharmacol. 8, 380. doi:10.3389/fphar.2017.00380
Nickavar, B., and Esbati, N. (2012). Evaluation of the Antioxidant Capacity and Phenolic Content of Three Thymus Species. J. Acupunct. Meridian Stud. 5 (3), 119–125. doi:10.1016/j.jams.2012.03.003
Oliviero, M., Romilde, I., Beatrice, M. M., Matteo, V., Giovanna, N., Consuelo, A., et al. (2016). Evaluations of Thyme Extract Effects in Human Normal Bronchial and Tracheal Epithelial Cell Lines and in Human Lung Cancer Cell Line. Chem. Biol. Interact. 256, 125–133. doi:10.1016/j.cbi.2016.06.024
Orhan, I., Şenol, F. S., Gülpinar, A. R., Kartal, M. U. R. A. T., Şekeroglu, N., Deveci, M., et al. (2009). Acetylcholinesterase Inhibitory and Antioxidant Properties of Cyclotrichium niveum, Thymus praecox subsp. caucasicus var. caucasicus, Echinacea purpurea and E. pallida. Food Chem. Toxicol. 47 (6), 1304–1310.
Öztürk, D., Yaylaci, Ö. K., Koyuncu, O., Sezer, O., Özgişi, K., and Ocak, A. (2022). A New Species of Thymus (Lamiaceae) from Central Anatolia (Turkey). Pak. J. Bot. 54 (2), 453–459. doi:10.30848/pjb2022-2(7)
Patil, S. M., Ramu, R., Shirahatti, P. S., Shivamallu, C., and Amachawadi, R. G. (2021). A Systematic Review on Ethnopharmacology, Phytochemistry and Pharmacological Aspects of Thymus Vulgaris Linn. Heliyon 7 (5), e07054. doi:10.1016/j.heliyon.2021.e07054
Raudone, L., Zymone, K., Raudonis, R., Vainoriene, R., Motiekaityte, V., and Janulis, V. (2017). Phenological Changes in Triterpenic and Phenolic Composition of Thymus L. Species. Industrial Crops Prod. 109, 445–451. doi:10.1016/j.indcrop.2017.08.054
Re, R., Pellegrini, N., Proteggente, A., Pannala, A., Yang, M., and Rice-Evans, C. (1999). Antioxidant Activity Applying an Improved ABTS Radical Cation Decolorization Assay. Free Radic. Biol. Med. 26 (9–10), 1231–1237.
Rodrigues, M., Lopes, A. C., Vaz, F., Filipe, M., Alves, G., Ribeiro, M. P., et al. (2020). Thymus Mastichina: Composition and Biological Properties with a Focus on Antimicrobial Activity. Pharmaceuticals 13 (12), 479. doi:10.3390/ph13120479
Salehi, B., Mishra, A. P., Shukla, I., Sharifi-Rad, M., Contreras, M. D. M., Segura-Carretero, A., et al. (2018). Thymol, Thyme, and Other Plant Sources: Health and Potential Uses. Phytother. Res. 32 (9), 1688–1706. doi:10.1002/ptr.6109
Sarfaraz, D., Rahimmalek, M., and Saeidi, G. (2021). Polyphenolic and Molecular Variation in Thymus Species Using HPLC and SRAP Analyses. Sci. Rep. 11, 5019. doi:10.1038/s41598-021-84449-6
Schulz, V., Hänsel, R., Blumenthal, M., and Tyler, V. E. (2004). Rational Phytotherapy: A Reference Guide for Physicians and Pharmacists. Springer Science & Business Media.
Sendker, J., and Sheridan, H. (2017). “History and Current Status of Herbal Medicines,” in Toxicology of Herbal Products (Cham: Springer), 11–27. doi:10.1007/978-3-319-43806-1_2
Sevindik, H. G., Ozgen, U., Atila, A., Ozturk Er, H., Kazaz, C., and Duman, H. (2015). Phtytochemical Studies and Quantitative HPLC Analysis of Rosmarinic Acid and Luteolin 5-O-β-D-Glucopyranoside on Thymus Praecox Subsp. Grossheimii Var. Grossheimii. Chem. Pharm. Bull. (Tokyo) 63 (9), 720–725. doi:10.1248/cpb.c14-00877
Slinkard, K., and Singleton, V. L. (1977). Total Phenol Analyses: Automation and Comparison with Manual Methods. Am. J. Enol. Vit. 28, 49–55.
Stahl-Biskup, E. (2002). 11 Thyme as a Herbal Drug–Pharmacopoeias and Other Product Characteristics. Thyme genus Thymus 293, 1–2.
Stefanis, I., Hadjipavlou-Litina, D., Bilia, A. R., and Karioti, A. (2019). LC-MS- and NMR-Guided Isolation of Monoterpene Dimers from Cultivated Thymus Vulgaris Varico 3 Hybrid and Their Antityrosinase Activity. Planta Med. 85 (11/12), 941–946. doi:10.1055/a-0927-7041
Taghouti, M., Martins-Gomes, C., Félix, L. M., Schäfer, J., Santos, J. A., Bunzel, M., et al. (2020). Polyphenol Composition and Biological Activity of Thymus Citriodorus and Thymus Vulgaris: Comparison with Endemic Iberian Thymus Species. Food Chem. 331, 127362. doi:10.1016/j.foodchem.2020.127362
Tan, B. L., Norhaizan, M. E., Liew, W. P., and Sulaiman Rahman, H. (2018). Antioxidant and Oxidative Stress: a Mutual Interplay in Age-Related Diseases. Front. Pharmacol. 9, 1162. doi:10.3389/fphar.2018.01162
Taşkın, T., Çam, M. E., Taşkın, D., and Rayaman, E. (2019). In Vitro and In Vivo Biological Activities and Phenolic Characterization of Thymus Praecox Subsp. Skorpilii Var. Skorpilii. J. Food Meas. Charact. 13 (1), 536–544.
Tepe, B., Daferera, D., Sökmen, M., Polissiou, M., and Sökmen, A. (2004). In Vitro antimicrobial and antioxidant activities of the essential oils and various extracts of Thymus eigii M. Zohary et P.H. Davis. J. Agric. Food Chem. 52 (5), 1132–1137. doi:10.1021/jf035094l
The Plant List (2013). Theplantlist.org. Available at: http://www.theplantlist.org (Accessed may 16, 2022).
Turumtay, E. A., İslamoğlu, F., Çavuş, D., Şahin, H., Turumtay, H., and Vanholme, B. (2014). Correlation between Phenolic Compounds and Antioxidant Activity of Anzer Tea (Thymus Praecox Opiz Subsp. Caucasicus Var. Caucasicus). Industrial Crops Prod. 52, 687–694. doi:10.1016/j.indcrop.2013.11.042
Yeung, A. W. K., Heinrich, M., and Atanasov, A. G. (2018). Ethnopharmacology-A Bibliometric Analysis of a Field of Research Meandering between Medicine and Food Science? Front. Pharmacol. 9, 215. doi:10.3389/fphar.2018.00215
Yilmaz, M. A., Ertas, A., Yener, I., Akdeniz, M., Cakir, O., Altun, M., et al. (2018). A Comprehensive LC-MS/MS Method Validation for the Quantitative Investigation of 37 Fingerprint Phytochemicals in Achillea Species: A Detailed Examination of A. Coarctata and A. Monocephala. J. Pharm. Biomed. Anal. 154, 413–424. doi:10.1016/j.jpba.2018.02.059
Zarshenas, M. M., and Krenn, L. (2015). A Critical Overview on Thymus Daenensis Celak.: Phytochemical and Pharmacological Investigations. J. Integr. Med. 13 (2), 91–98. doi:10.1016/S2095-4964(15)60166-2
Zarzuelo, A., and Crespo, E. (2002). “The Medicinal and Non-medicinal Uses of Thyme,” in Thyme (Londan: CRC Press), 277–306.
Zengin, G., Zakariyyah Aumeeruddy, M., Aumeeruddy, M. Z., Babak Bahadori, M., Mollica, A., Bahadori, M. B., et al. (2019). Phenolic Profiling and In Vitro Biological Properties of Two Lamiaceae Species (Salvia Modesta and Thymus Argaeus): A Comprehensive Evaluation. Industrial Crops Prod. 128, 308–314. doi:10.1016/j.indcrop.2018.11.027
Glossary
LC-MS/MS Liquid Chromatography with Tandem Mass Spectrometry
QEs Quercetin equivalents
PEs Pyrocatechol equivalents
CUPRAC Cupric Reducing Antioxidant Capacity
IC50 Concentration of drug required for 50% inhibition
DPPH 2,2-diphenyl-1-picrylhydrazyl
ABTS 2.2'-azino-bis(3-ethylbenzothiazoline-6-sulfonic acid
A0.5 The absorbance value at 0.5 concentration
MIC Minimum Inhibitory Concentration
ESCOP European Scientific Cooperative on Phytotherapy
WHO World Health Organization
ISTE The Herbarium of the Faculty of Pharmacy of Istanbul University
UHPLC Ultra High Performance Liquid Chromatography
ESI Electrospray Ionization
CLSI Clinical and Laboratory Standards Institute
MTP Molecular Targets Program
NCI National Cancer Institute
MG63 Low Metastatic Potential Osteosarcoma Cell Lines
MG63.3 High Metastatic Potential Osteosarcoma Cell Lines
UO-31 Human Renal Cancer Cell Lines
A498 Human Renal Cancer Cell Lines
COLO205 Human Colon Cancer Cell Lines
KM12 Human Colon Cancer Cell Lines
BHT Butylhydroxytoluene
BHA Butylhydroxyanisole
ATCC American Type Culture Collection
HPLC-DAD High-Performance Liquid Chromatography with Diode-Array Detection
GAE Gallic acid equivalents
α-TOC Alpha-Tocopherol
TcA Thymus cariensis Hub-Mor. & Jalas aerial parts extract
TprA Thymus praceox subsp. grossheimii (Ronniger) Jalas aerial parts extract
TprR Thymus praceox subsp. grossheimii (Ronniger) Jalas roots extract
TpuA Thymus pubescens Boiss. Et Kotschy ex Celak aerial parts extract
TpuR Thymus pubescens Boiss. et Kotschy ex Celak roots extract
AChE Acetylcholinesterase
BChE Butyrylcholinesterase
KAE Kojic acid equivalents
MRSA Methicillin-resistant Staphylococcus aureus
Keywords: Thyme, Thymus sp., Anatolian, LC-MS/MS, antioxidant, anti-cholinesterase activity, cytotoxicity, Candida tropicalis
Citation: Eroglu Ozkan E, Ersoy E, Yesil Canturk Y, Mataraci Kara E, Cinar E, Sahin H, Karahan S, Karaca Sancaktepe K, Yilmaz MA and Boga M (2022) The Therapeutic Potential of Ethnomedicinally Important Anatolian Thyme Species: A Phytochemical and Biological Assessment. Front. Pharmacol. 13:923063. doi: 10.3389/fphar.2022.923063
Received: 18 April 2022; Accepted: 19 May 2022;
Published: 09 June 2022.
Edited by:
Gokhan Zengin, Selcuk University, TurkeyReviewed by:
Andrea Mastinu, University of Brescia, ItalyAleksandra Cvetanovic Kljakic, University of Novi Sad, Serbia
Ivo Pischel, University College London, United Kingdom
Copyright © 2022 Eroglu Ozkan, Ersoy, Yesil Canturk, Mataraci Kara, Cinar, Sahin, Karahan, Karaca Sancaktepe, Yilmaz and Boga. This is an open-access article distributed under the terms of the Creative Commons Attribution License (CC BY). The use, distribution or reproduction in other forums is permitted, provided the original author(s) and the copyright owner(s) are credited and that the original publication in this journal is cited, in accordance with accepted academic practice. No use, distribution or reproduction is permitted which does not comply with these terms.
*Correspondence: Esra Eroglu Ozkan, ZXNlcm9nbHVAaXN0YW5idWwuZWR1LnRy; Mehmet Boga, bWVobWV0Ym9nYTE5ODBAZ21haWwuY29t
†These authors have contributed equally to this work and share first authorship