- 1Department of Emergency and Intensive Care Medicine, Kagoshima University Graduate School of Medical and Dental Sciences, Kagoshima, Japan
- 2Department of Biomedical Laboratory Sciences, Faculty of Life Sciences, Kumamoto University, Kumamoto, Japan
- 3School of Life Science and Technology, Tokyo Institute of Technology, Yokohama, Japan
Oxidation of BH4, a cofactor of nitric oxide synthase (NOS), produces reactive oxygen species (ROS) through uncoupling of NOS and affects vascular endothelial dysfunction. Ascorbic acid (AsA) inhibits the oxidation of BH4 and reduces ROS. However, the kinetic changes of BH4 in sepsis and its effect on the kinetic changes in AsA administration therapy, as well as the appropriate timing of AsA administration for AsA therapy to be effective, are unclear. Mice with sepsis, induced by cecal ligation and puncture (CLP), were examined for the effect of AsA administration (200 mg/kg) on vascular endothelial cell dysfunction at two administration timings: early group (AsA administered immediately after CLP) and late group (AsA administered 12 h after CLP). Survival rates were compared between the early and late administration groups, and vascular endothelial cell damage, indicated by the dihydrobiopterin/tetrahydrobiopterin ratio, serum syndecan-1, and endothelial nitric oxide synthase, as well as liver damage, were examined. The early group showed significantly improved survival compared to the non-treatment group (p < 0.05), while the late group showed no improved survival compared to the non-treatment group. Compared to the non-treated group, the early AsA group showed less oxidation of BH4 in sepsis. Syndecan1, a marker of vascular endothelial cell damage, was less elevated and organ damage was reduced in the early AsA-treated group. In septic mice, early AsA administration immediately after CLP may protect vascular endothelial cells by inhibiting BH4 oxidation, thereby reducing organ dysfunction and improving survival.
1 Introduction
Sepsis is a life-threatening organ dysfunction caused by a dysregulated host response to infection (Beale et al., 2009). The World Health Organization reports that although sepsis mortality rates have declined in recent decades, it still causes 11 million deaths annually (Rudd et al., 2020). Currently, there is no definitive treatment for sepsis, and the recommended treatment includes early detection, early antibiotic administration, appropriate infusion therapy, and optimal timing of vasopressor administration; however, the mortality rate remains high (Evans et al., 2021).
Since vascular endothelial dysfunction is associated with the pathological progression of sepsis, maintaining vascular endothelial function is attracting attention as a new therapeutic strategy for sepsis (Becker et al., 2010; Ince et al., 2016; Uchimido et al., 2019; Lupu et al., 2020). The vascular endothelium is covered with glycocalyx, a gel-like protective layer, which plays an important role in vascular endothelial function, including vascular permeability, anticoagulation, and nitric oxide (NO) production from endothelial nitric oxide synthase (eNOS). The glycocalyx is composed of syndecan-1, heparan sulfate, hyaluronic acid, etc. In sepsis, reactive oxygen species (ROS), tumor necrosis factor-alpha (TNF-alpha), interleukin-1beta (IL-1beta), and other factors cause the glycocalyx to be shed, resulting in increased vascular permeability and coagulation and decreased NO production (Uchimido et al., 2019).
Tetrahydrobiopterin (BH4) is produced from guanosine triphosphate and acts as an essential cofactor for various enzymes. BH4 is easily oxidized to dihydrobiopterin (BH2), and the binding affinities of BH4 and BH2 to eNOS are equal. NO is produced when BH4 binds to eNOS, whereas superoxide is produced when BH2 binds to eNOS, namely uncoupling of eNOS (Vásquez-Vivar et al., 2002). The relationship between BH4 and vascular endothelial function has been reported in various diseases such as hypertension (HT), diabetes mellitus (DM), and atherosclerosis. In addition, there are indications that oxidation of BH4 affects endothelial dysfunction in all these diseases (Kolluru et al., 2012; Ismaeel et al., 2020; Kim and Han, 2020). Although it has been considered that the lack of BH4 and/or the increase of BH2 are the causes of the uncoupling of NOS, it has recently been reported that the BH2/BH4 ratio is more related to ROS generation and vascular endothelial dysfunction than the absolute value of BH4 or BH2 (Crabtree et al., 2008; Takeda et al., 2009; Pathak et al., 2014; Ismaeel et al., 2020). Inhibiting the oxidation of BH4 and preventing the increase in BH2/BH4 ratio are important for maintaining vascular endothelial function.
Ascorbic acid (AsA), also known as vitamin C, is an important antioxidant that prevents the oxidation of various substances, including BH4 (Heller et al., 2001). AsA has important effects on the maintenance of vascular endothelial functions, with multiple pathways known to exert vascular endothelial protection, including inhibition of BH4 oxidation (May and Harrison, 2013). In addition, there have been recent studies suggesting the efficacy of AsA administration in sepsis. Vitamin C levels are decreased in critically ill patients, such as those with sepsis (Carr et al., 2017). In sepsis, AsA administration has been reported to improve survival and protect microvascular functions (Tyml et al., 2008; Fowler et al., 2014; Zabet et al., 2016; Lv et al., 2021). It has been reported that AsA has multiple mechanisms of action for sepsis, one of which is by inhibiting BH4 oxidation (Moskowitz et al., 2018).
However, while AsA inhibits the oxidation of BH4, it does not reduce BH2 to BH4 (Vásquez-Vivar et al., 2001). After BH4 has been oxidized to BH2, the effect of AsA administration on this mechanism cannot be expected and may be limited by the timing of AsA administration. We hypothesized that administration of AsA at the optimal timing, before the BH2/BH4 ratio increases, would protect the glycocalyx and improve sepsis survival. In our preliminary experiments using the cecal ligation and puncture (CLP) model mice, the BH2/BH4 ratio showed an upward trend from 6 h after the onset of sepsis. Therefore, we examined the unclear kinetics of when the BH2/BH4 ratio changes in sepsis and how AsA administration before oxidation to BH2 affects the BH2/BH4 ratio and influences survival.
2 Methods
2.1 Animals
Adult C57BL/6 mice (9–11-week-old males) weighing 25 g were obtained from Kyudo (Fukuoka, Japan), housed under standard environmental conditions, and maintained at 23 ± 1°C with a 12-h light/dark cycle. All animal experiments were conducted under the rules approved by the Institutional Animal Care and Use Committee of Kagoshima University (approval number MD18126). As this was an animal study, consents for participation and publication were not applicable. We carried out the study in compliance with the ARRIVE guidelines (https://arriveguidelines.org) and the Guidelines for the Proper Conduct of Animal Experiments established by the Science Council of Japan.
2.2 CLP
As previously reported, septic shock was induced with reference to the high grade model CLP in the article by Rittirsch et al. with slight modifications (Rittirsch et al., 2009). Briefly, mice were anesthetized with isoflurane, and the mouse cecum was ligated with a 3-0 silk suture and punctured in one place with a 21-gauge needle. The cecum was retracted into the abdominal cavity, and the incision was sutured with 3-0 nylon. Only open and closed abdominal procedures were performed for sham-operated mice without CLP. During each experiment, blood, liver, and heart tissues were collected and analyzed under inhalation anesthesia. At 6, 12, and 24 h after CLP and sham operation, the blood of mice was collected by inferior vena cava puncture, after which the animals were sacrificed. At the same time point, the myocardium and liver were collected. Blood samples were centrifuged at 2,000 g for 10 min to collect plasma and stored at −80°C until analysis. After the operation, buprenorphine (0.05 mg/kg) was repeatedly administered every 12 h by subcutaneous injection.
2.3 Experimental design
2.3.1 Survival experiment: CLP vs. CLP + AsA (early)
Mice were randomized into the following groups: 1) sham (n = 10); 2) sham + AsA (early) (n = 10); 3) CLP (n = 10), and 4) CLP + AsA (early) groups (n = 9). The group that had not received AsA was given the same amount of NS needed to dissolve the AsA in the AsA group. These mice received 40 ml/kg of normal saline or AsA (200 mg/kg) by subcutaneous injection immediately after the operation and were monitored for 72 h (Figure 1A; Table 1). In the study, n refers to the number of animals. The numbers for each group were taken from similar experiments reported in the literature. AsA was administered at the optimal dose of 200 mg/kg/day for this experiment, as its effectiveness has been demonstrated previously in septic mice (Wu et al., 2003; McKinnon et al., 2007; Kim et al., 2015; Jensen et al., 2021).
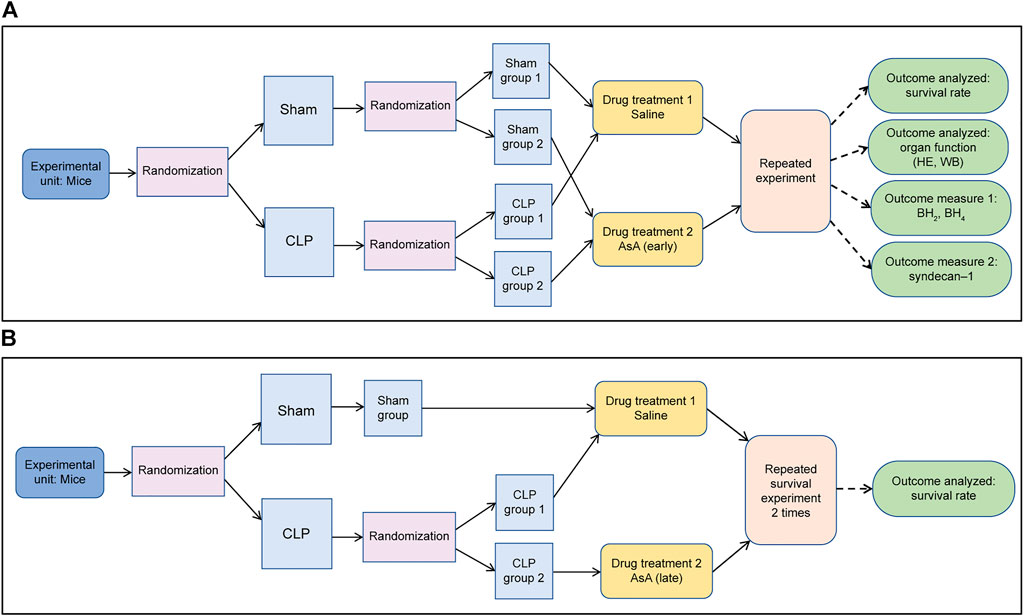
FIGURE 1. (A) The surgeon was unaware of the drug treatment that the animals received from the start of the experiment until after the injection. (B) The surgeon was unaware of the drug treatment that the animals received from the start of the experiment until after the injection.
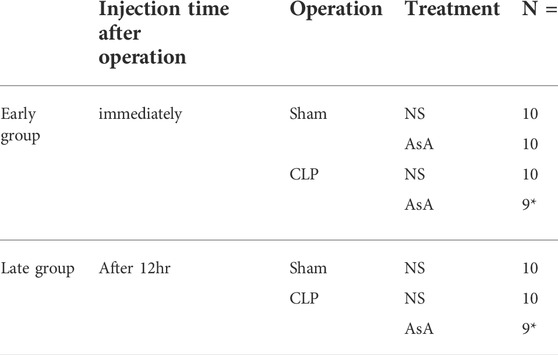
TABLE 1. Number of mice per group. ∗These groups originally numbered 10 animals, but the feces were hard and the severity of the disease could not be assessed; thus, we excluded 2 mice from the study.
2.3.2 Survival experiment: CLP vs. CLP + AsA (late)
Mice were randomized into the following groups: 1) sham (n = 10), 2) CLP (n = 10), and 3) CLP + AsA (late) (n = 9). The group that had not received AsA was given the same amount of NS needed to dissolve the AsA in the AsA group. Mice received 40 ml/kg of normal saline by subcutaneous injection immediately after the operation, and 10 ml/kg of normal saline or AsA (200 mg/kg) by subcutaneous injection at 12 h after the operation. The mice were monitored for 72 h (Figure 1B; Table 1).
2.3.3 Measurement of BH4 and BH2 and calculation of BH2/BH4
BH4 is a substance that oxidizes easily, and oxidation was prevented by adding 0.2% dithioerythritol (a final concentration). BH4 and BH2 were measured separately by the post-column oxidation method using high-performance liquid chromatography with a fluorescence detector (Tani and Ohno, 1993). The plasma samples (100 μl) were deproteinized by adding 25 µl of 1 M perchloric acid containing 0.5 mM EDTA, followed by centrifugation. The supernatants were filtered through a 0.2-μm filter. The BH2/BH4 ratio was calculated by dividing BH2 by BH4. After 6, 12, and 24 h of operation in the sham + NS group, the number of mice in each group was 7, 4, and 4, respectively. In the CLP+ NS group were 8, 6, and 7, respectively. Finally, the CLP+ AsA group was 7, 7, and 7, respectively. The number of mice in the control group was 5 (Figure 1A; Table 2).
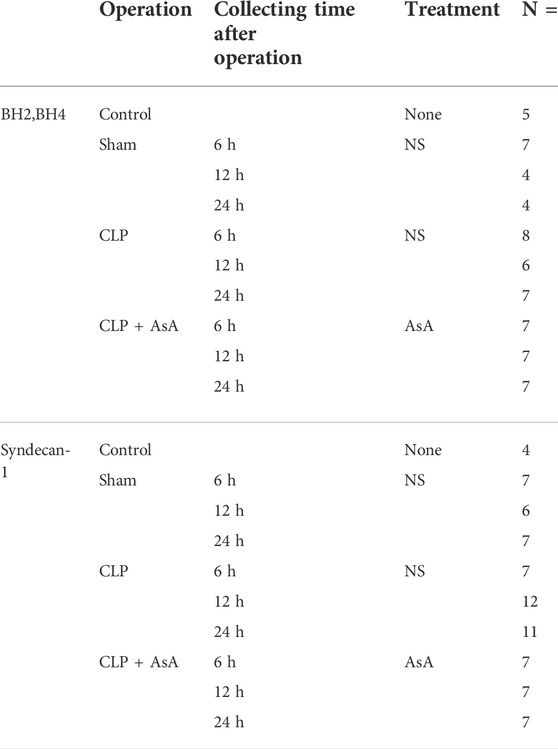
TABLE 2. Outcome measurement. ∗The numbers of each group deviated because some individuals died during the course of the study. Including the dead mice, the total is 152.
2.3.4 Measurement of Syndecan-1
Plasma syndecan-1 levels were measured using a Murine CD138 ELISA Kit (Diaclone, France). The number of mice in each group after 6, 12, and 24 h of operation in the sham + NS group was 7, 6, and 7, respectively. In the CLP+ NS group, the number was 7, 12, and 11, respectively. Finally, the CLP+ AsA group was 7, 7, and 7, respectively (Figure 1A and Table 2).
2.3.5 Western blotting analysis
The heart was homogenized in a buffer solution (T-PER Tissue Protein Extraction Reagent; Thermo Scientific, Rockford, USA). The extracted proteins were quantified (TaKaRa BCA Protein Assay Kit, Takara Holdings Inc, Japan), and the amount of protein to be applied to the gel was adjusted. The protein samples (1 μg of protein) were electrophoresed on 10% SDS-PAGE and transferred to the PVDF membrane. The membrane was blocked for 1 h (BLOCK ACE®, MEGMILK SNOW BRAND, Japan) and incubated with primary antibodies (eNOS, 1:1,000, Purified Mouse Anti-eNOS/NOS Type III, BDbioscience, USA; GAPDH, 1:20,000, Anti-GAPDH Loading Control ab8245, Abcam, UK) at 4°C overnight. After washing with Phosphate Buffered Saline with Tween (PBST) buffer, the membranes were incubated with horseradish peroxidase (HRP)-conjugated secondary antibody (1:5,000, Goat Anti-Mouse IgG H&L HRP ab205719, Abcam, UK) for 1 h at room temperature. Blots were washed with PBST, and immunoreactive bands were detected using an enhanced chemiluminescence system (ImmunoStar®, FUJIFILM Wako Chemical Corporation, Japan) (Figure 1A). Optical density for individual bands was examined using the Fluor Chem FC2 (Cell Biosciences, Santa Clara, CA, United States of America). The densitometry ratios of eNOS to GAPDH were then computed.
2.3.6 Histologic examination
Liver tissue specimens were fixed in 10% formalin and embedded in paraffin. They were stained with hematoxylin and eosin to evaluate the degree of injury (Figure 1A).
2.4 Statistical analysis
Survival rates were analyzed using the Kaplan–Meier method. Survival times were compared using the log-rank test. Data are expressed as mean ± standard error. The Kruskal–Wallis test was used to detect differences between the groups. The Bonferroni method was used for the post hoc test of this statistic. Student’s t-test was used for comparisons between the two groups of quantified western blots. Significant differences were considered if the p-value was <0.05.
3 Results
3.1 Early administration of AsA improved the survival rate of septic mice
We compared the survival rates after operation between sham, sham + AsA (early), CLP, and CLP + AsA (early) (Figure 2). None of the CLP mice used in this study survived to 45 h after the operation. All sham + NS and sham + AsA (early) mice survived for 72 h. In the CLP + AsA (early) mice group, 3 of 9 mice survived after the operation (33%).
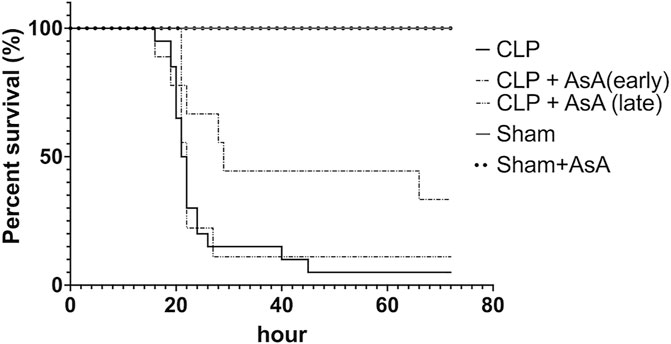
FIGURE 2. Mice subjected to CLP, as described in the Methods section, for 72 h survival study. In the CLP + AsA (early) group, AsA was injected subcutaneously immediately after the operation (n = 9). In the CLP + AsA (late) group, AsA was injected subcutaneously 12 h after the operation (n = 9). The group that had not received AsA was given the same amount of NS needed to dissolve the AsA in the AsA group. Although the timing of saline administration in the CLP group differed between the late and early groups, they were compared with the other groups as the same group because the severity of the disease did not change. The CLP + AsA (early) mice group had a significantly prolonged survival rate compared to the CLP group. *p < 0.05 versus CLP. CLP + AsA (late) mice showed no difference in the survival rate from the CLP group. *p < 0.05 versus CLP. Abbreviations: AsA, ascorbic acid; CLP, cecal ligation and puncture.
The CLP + AsA (early) mice group showed significantly higher survival rates than the CLP mice group.
Second, we compared the survival rates after operation among sham, CLP, and CLP + AsA (late) groups (Figure 2). AsA (Late) mice group received 40 ml/kg of normal saline by subcutaneous injection immediately after the operation, and AsA (200 mg/kg) by subcutaneous injection at 12 h after the operation. All sham mice survived for 72 h. In the CLP and CLP + AsA (late) mice groups, 1 of 9 mice survived after the operation (11%). The CLP + AsA (late) mice group showed no difference in survival rates compared to the CLP mice group.
3.2 BH2/BH4 ratio increased 6 h after the operation and continued to increase over time. Early administration of AsA prevented an increase in BH2/BH4 ratio
To elucidate the dynamics of BH4 and BH2 in CLP-induced sepsis and how the dynamics of the BH2/BH4 ratio change with the administration of AsA (early), we measured BH4 and BH2 and then calculated BH2/BH4. Serum BH4 and BH2 levels were determined in CLP and sham mice at 6, 12, and 24 h after the operation.
Both BH4 and BH2 showed a significant increase 24 h after the operation (Figure 3). The ratio of BH2 to BH4 was significantly elevated in the CLP group compared to the early AsA group starting at 12 h.
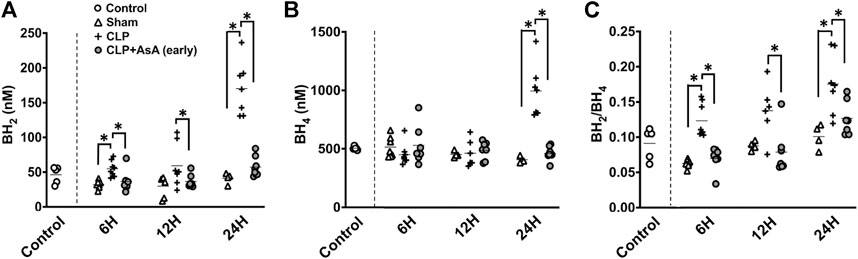
FIGURE 3. (A) BH2 concentration in the plasma was measured by HPLC. At 6 and 24 h after the operation, CLP group had significantly elevated BH2 concentration compared to CLP+ AsA (early) and Sham groups. At 12 h after the operation, CLP group had significantly elevated BH2 concentration compared to CLP+ AsA (early) group. Error bars represent SE. *p < 0.05. (B) BH4 concentration in the plasma was measured by HPLC. At 24 h after the operation, CLP group had significantly elevated BH4 concentration compared to CLP+ AsA (early) and Sham groups. At 6 and 12 h after the operation, there was no significant differences between the groups. Error bars represent SE. *p < 0.05. (C) BH2/BH4 ratio was calculated by dividing BH2 by BH4. At 6 and 24 h after the operation, CLP group had significantly elevated BH2/BH4 ratio compared to CLP+ AsA (early) and Sham groups. At 12 h after the operation, CLP group had significantly elevated BH2/BH4 ratio compared to CLP+ AsA (early) group. Error bars represent SE. *p < 0.05. Abbreviations: AsA, ascorbic acid; CLP, cecal ligation and puncture; BH4, tetrahydrobiopterin; BH2, dihydrobiopterin; SE, standard error; HPLC, high-performance liquid chromatography.
Syndecan-1 levels increased after 12 h, but early administration of AsA suppressed this increase. The expression of eNOS in myocardial tissues was also maintained by the early administration of AsA.
Syndecan-1 level and eNOS expression in myocardial tissues were measured to evaluate whether early administration of AsA protects vascular endothelial cells.
Syndecan-1 at 12 h was significantly higher in the CLP group than in the early AsA group (Figure 4). The expression of eNOS was measured to evaluate vascular endothelial cells and was assessed in myocardial tissue 12 h after operation. Four samples from the normal, CLP, and CLP + AsA (early) groups were collected and evaluated by western blotting. eNOS expression was lower in the CLP group than in the normal group. Compared to the CLP group, the early AsA group maintained eNOS expression.
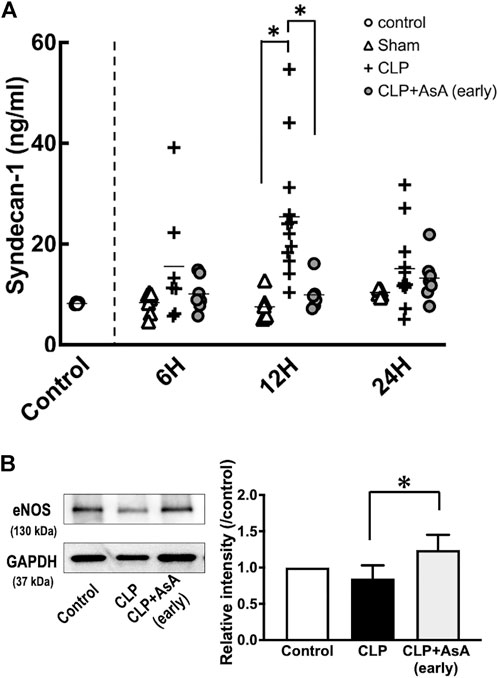
FIGURE 4. (A) Plasma syndecan-1 was measured by ELISA kit. At 12 h after the operation, CLP group had significantly elevated syndecan-1 level compared to the CLP+ AsA (early) and Sham groups. Error bars represent SE. *p < 0.05. (B) All samples were taken 12 h postoperatively. The expression of eNOS (130 kDa) in the heart was measured by western blotting; it was decreased in the CLP group but maintained in the early AsA group. eNOS was quantified and compared between the CLP and CLP + AsA (early) groups (n = 4, 4). For the quantitative experiments, measurements were repeated multiple times. eNOS expression was significantly maintained in the AsA (early) group. *p < 0.05. Abbreviations: AsA, ascorbic acid; CLP, cecal ligation and puncture; eNOS, endothelial NO synthase; SE, standard error.
3.3 Liver organ damage was reduced by early AsA administration
Finally, liver tissue was stained with hematoxylin and eosin and observed under a microscope to evaluate organ damage due to sepsis. Each sample was collected 12 h after the operation.
No histological differences were observed between the control and sham mice. In CLP mice, the arrangement of hepatocytes was markedly disorganized (Figure 5). In contrast, hepatocyte disarrangement was reduced in CLP + AsA (early) mice, although not as orderly as in the control and sham mice.
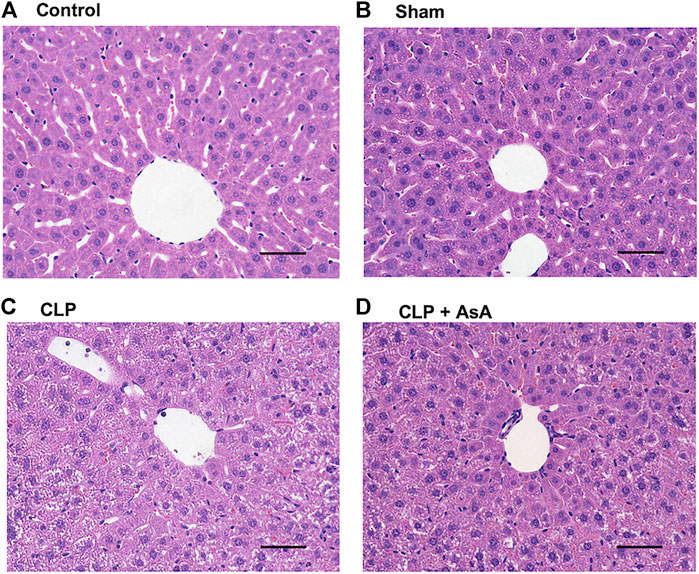
FIGURE 5. Histopathological examination of the liver of CLP-treated and untreated mice. (A,B) Normal histology of liver tissues obtained from sham and control mice. (C) Representative CLP-induced liver damage. (D) Representative liver of CLP mice treated with early AsA administration. Sham and CLP mice were killed 12 h after the operation. Original magnification, ×40. Abbreviations: AsA, ascorbic acid; CLP, cecal ligation and puncture
4 Discussion
This study showed that early administration of AsA might contribute to improved survival of septic mice (Figure 2). In particular, we found that the optimal timing of AsA administration should be early in the disease, before the BH2/BH4 ratio increases. Although the efficacy of AsA in sepsis is still under discussion, our study suggests that it may be more effective if administered at the optimal time. Here, we discuss the effect of early AsA administration on BH4 dynamics and the optimal timing of AsA administration.
BH4 plays an important role in maintaining vascular endothelial function by producing NO as a cofactor for eNOS. It also plays a role in exacerbating the pathogenesis of sepsis by overproducing NO through inducible nitric oxide synthase (iNOS) expression and producing free radicals through eNOS uncoupling (Ince et al., 2016; Dolmatova et al., 2021). The elevation of the BH2/BH4 ratio is associated with ROS development. It has been reported to correlate with vascular endothelial dysfunction in various diseases, such as HT, DM, and peripheral arterial disease. (Crabtree et al., 2008; Crabtree and Channon, 2011; Ismaeel et al., 2020). However, there are few reports on the dynamics of BH4 in the acute phase and the relationship between the BH2/BH4 ratio and vascular endothelial function in sepsis. In our septic mice experiment, the BH2/BH4 ratio was shown to increase as early as 6 h after CLP, indicating that the BH2/BH4 ratio increases in sepsis, vascular endothelial dysfunction is associated with exacerbation, as in other diseases (Figure 3C).
In case of an increase in BH2/BH4 ratio, eNOS undergoes an uncoupling reaction, which produces superoxide, instead of NO, that reacts to form peroxynitrite (ONOO-), a powerful oxidant (Stuehr et al., 2001; Vásquez-Vivar et al., 2002; Alkaitis and Crabtree, 2012). Peroxynitrite is one of the most powerful ROS, making it a major cause of vascular endothelial dysfunction. It is considered that correcting the BH2/BH4 ratio can inhibit peroxinitrite production and protect the vascular endothelium (Bendall et al., 2014). Therefore, attempts to correct the relative lack of BH4 by supplementing BH4 to prevent uncoupling of eNOS and maintain endothelial function have been reported in several diseases such as patients of coronary risk factors, HT, DM, and ischemia reperfusion (Heitzer et al., 2000; Mayahi et al., 2007; Porkert et al., 2008). In sepsis, BH4 administration reportedly improved microcirculation, circulatory indices, and survival rate in a sheep sepsis model (He et al., 2012; Dumbarton et al., 2017). However, conflicting studies have shown that inhibition of BH4 production improves sepsis mortality (Chuaiphichai et al., 2016). Thus, there are conflicting reports on the administration of BH4 for sepsis. One reason why BH4 administration is not effective in the acute phase of sepsis is that BH4 is overproduced in the acute phase of sepsis, and the overproduction of BH4 may be related to its autoxidation to BH2 (Kirsch et al., 2003). Another possible cause is that exogenous BH4 is converted to BH2 after administration (Cunnington et al., 2012). Since treatment that inhibits BH4 oxidation may be more effective than administration of BH4 to improve the BH2/BH4 ratio in the acute phase of sepsis, when BH4 production is overproduced, we focused on the antioxidant AsA.
AsA has long been used as an antioxidant in BH4 measurement methods (Tani and Ohno, 1993). In sepsis, the administration of AsA alone and the simultaneous administration of vitamin B1 and hydrocortisone have been widely studied. The simultaneous administration of vitamin B1 and hydrocortisone, in particular, has attracted attention as a type of metabolic therapy such as Hydrocortisone, ascorbic acid, and thiamine therapy (Marik et al., 2017; Marik, 2018; Fowler et al., 2019; Kim et al., 2020). In clinical practice, administration of AsA, a reducing agent, has been reported to inhibit the oxidation of BH4 (Mortensen and Lykkesfeldt, 2014). In our study, the BH2/BH4 ratio increased at 6 h after operation, and AsA administration immediately after operation significantly suppressed the increase in BH2/BH4 ratio (Figure 3C). In the AsA non-administered group, an increase in serum syndecan-1 level, an indicator of endothelial cell damage, and decreased eNOS expression, an indicator of endothelial cell protection, were observed at 12 h after operation (Figures 4A,B).
On the other hand, in the early AsA group immediately after the operation, both serum syndecan-1 level and eNOS expression level showed protective effects on vascular endothelium (Figures 4A,B). In addition, in the non-AsA-treated group, where syndecan-1 was elevated and eNOS expression was decreased, organ damage occurred after 12 h. In contrast, early AsA administration suppressed syndecan-1 elevation and reduced organ damage in the group where eNOS expression was maintained (Figure 5). Serum syndecan-1 is a known indicator of vascular endothelial damage that correlates with coagulation disorders associated with sepsis prognoses, such as persistent thrombocytopenia and disseminated intravascular coagulation (DIC) (Ostrowski et al., 2015; Hatanaka et al., 2021). The suppression of syndecan-1 elevation in the early AsA group suggests that vascular endothelial cell damage suppression resulted in less sepsis-induced organ damage. Since AsA inhibits the oxidation of BH4, the increase in BH2/BH4 ratio was suppressed when AsA was administered immediately after operation. The fact that the survival rate did not improve when AsA was administered after 12 h (late group) in the survival experiment may be due to the late timing of administration since AsA does not have the effect of reducing BH2 to BH4 (Figure 2) (Vásquez-Vivar et al., 2001).
Several recent studies have shown no positive effect of AsA administration in septic shock patients, so it remains controversial whether AsA should be administered to these patients (Fujii et al., 2020; Moskowitz et al., 2020; Scholz et al., 2021). Some studies have cited delayed administration as a limiting factor to obtaining a good effect of AsA in septic shock (Moskowitz et al., 2020). Since the reduction of BH2 to BH4 is not expected to be effective, making a difference in the survival results depending on the timing of AsA administration, as shown in our present experiment with septic mice, the studies reporting no effect of AsA therapy may be related to the fact that AsA was administered after the BH2/BH4 ratio was already elevated. The optimal timing of AsA administration for sepsis will become more important, as an experiment is currently planned to test the efficacy of early AsA administration in the emergency room for septic patients (Vandervelden et al., 2021).
This study has several limitations. First, it is unclear whether the results from this septic mouse experiment would be similar to those of human sepsis. Changes in BH4 and BH2 over time may differ between humans and mice. Second, because mice can synthesize AsA in their bodies, their bodies’ dynamics of AsA concentration may be different from those of humans. The optimal dosage needs to be discussed in both human and animal studies. Third, we have not measured intracellular BH4; some experiments have measured BH4 and BH2 in cells rather than in plasma as in our study. Although we consider that the kinetics of the two move generally in parallel, it may have been necessary to measure the BH2/BH4 ratio in vascular endothelial cells in order to correlate ROS production (eNOS function) in vascular endothelial cells with the BH2/BH4 ratio in plasma.
Moreover, in this study, we only mentioned the protective effect of AsA on vascular endothelial cells by suppressing the increase in BH2/BH4 ratio. Still, AsA has additional effects, such as catecholamine production, adrenocorticotropic hormone production, and direct scavenging of free radicals, which may improve the prognosis of sepsis through various pathways (Patak et al., 2004; Padayatty et al., 2007; Moskowitz et al., 2018; Obi et al., 2020). However, our present findings indicate that the timing of AsA administration affects prognosis and that the BH2/BH4 ratio is related to the mechanism of septic shock.
5 Conclusion
In the septic mice, an increase in the BH2/BH4 ratio, which causes vascular endothelial cell damage, occurred 6 h after the disease onset. In the present study, we suggest that administration of AsA at an earlier time before the increase in the BH2/BH4 ratio, suppressed the increase in the BH2/BH4 ratio and contributed to the improved prognosis of the septic mice. In the future, the time course of the BH2/BH4 ratio in septic patients should be evaluated to determine the optimal timing of AsA administration.
Data availability statement
The original contributions presented in the study are included in the article/supplementary material, further inquiries can be directed to the corresponding author.
Ethics statement
All animal experiments were conducted under the rules approved by the Institutional Animal Care and Use Committee of Kagoshima University (approval number MD18126).
Author contributions
YM, CK, SH, and HI contributed to the data acquisition, analysis, and interpretation. All authors contributed to data interpretation, critically revised the manuscript, and approved the final manuscript.
Funding
This work was supported by JSPS KAKENHI (Grant Number 19K09438). This work was supported by the Facility of the Laboratory Animal Science Research Support Center Institute for Research Promotion, Kagoshima University.
Acknowledgments
The authors would like to thank M. Yamada, who helped with data collection and sample collection. We would like to thank Editage (www.editage.com) for English language editing.
Conflict of interest
The authors declare that the research was conducted in the absence of any commercial or financial relationships that could be construed as a potential conflict of interest.
Publisher’s note
All claims expressed in this article are solely those of the authors and do not necessarily represent those of their affiliated organizations, or those of the publisher, the editors and the reviewers. Any product that may be evaluated in this article, or claim that may be made by its manufacturer, is not guaranteed or endorsed by the publisher.
References
Alkaitis, M. S., and Crabtree, M. J. (2012). Recoupling the cardiac nitric oxide synthases: Tetrahydrobiopterin synthesis and recycling. Curr. Heart Fail. Rep. 9, 200–210. doi:10.1007/s11897-012-0097-5
Beale, R., Reinhart, K., Brunkhorst, F. M., Dobb, G., Levy, M., Martin, G., et al. (2009). Promoting global research excellence in severe sepsis (PROGRESS): Lessons from an international sepsis registry. Infection 37, 222–232. doi:10.1007/s15010-008-8203-z
Becker, B. F., Chappell, D., Bruegger, D., Annecke, T., and Jacob, M. (2010). Therapeutic strategies targeting the endothelial glycocalyx: Acute deficits, but great potential. Cardiovasc. Res. 87, 300–310. doi:10.1093/cvr/cvq137
Bendall, J. K., Douglas, G., McNeill, E., Channon, K. M., and Crabtree, M. J. (2014). Tetrahydrobiopterin in cardiovascular health and disease. Antioxid. Redox Signal. 20 (18), 3040–3077. doi:10.1089/ars.2013.5566
Carr, A. C., Rosengrave, P. C., Bayer, S., Chambers, S., Mehrtens, J., and Shaw, G. M. (2017). Hypovitaminosis C and vitamin C deficiency in critically ill patients despite recommended enteral and parenteral intakes. Crit. Care 21, 300. doi:10.1186/s13054-017-1891-y
Chuaiphichai, S., Starr, A., Nandi, M., Channon, K. M., and McNeill, E. (2016). Endothelial cell tetrahydrobiopterin deficiency attenuates LPS-induced vascular dysfunction and hypotension. Vasc. Pharmacol. 77, 69–79. doi:10.1016/j.vph.2015.08.009
Crabtree, M. J., and Channon, K. M. (2011). Synthesis and recycling of tetrahydrobiopterin in endothelial function and vascular disease. Nitric Oxide 25, 81–88. doi:10.1016/j.niox.2011.04.004
Crabtree, M. J., Smith, C. L., Lam, G., Goligorsky, M. S., and Gross, S. S. (2008). Ratio of 5, 6, 7, 8-tetrahydrobiopterin to 7, 8-dihydrobiopterin in endothelial cells determines glucose-elicited changes in NO vs. superoxide production by eNOS. Am. J. Physiol. Heart Circ. Physiol. 294, H1530–H1540. doi:10.1152/ajpheart.00823.2007
Cunnington, C., Van Assche, T., Shirodaria, C., Kylintireas, I., Lindsay, A. C., Lee, J. M., et al. (2012). Systemic and vascular oxidation limits the efficacy of oral tetrahydrobiopterin treatment in patients with coronary artery disease. Circulation 125, 1356–1366. doi:10.1161/CIRCULATIONAHA.111.038919
Dolmatova, E. V., Wang, K., Mandavilli, R., and Griendling, K. K. (2021). The effects of sepsis on endothelium and clinical implications. Cardiovasc. Res. 117, 60–73. doi:10.1093/cvr/cvaa070
Dumbarton, T. C., Maxan, A., Farah, N., Sharawy, N., Zhou, J., Nantais, J., et al. (2017). Tetrahydrobiopterin improves microcirculation in experimental sepsis. Clin. Hemorheol. Microcirc. 67, 15–24. doi:10.3233/CH-160207
Evans, L., Rhodes, A., Alhazzani, W., Antonelli, M., Coopersmith, C. M., French, C., et al. (2021). Surviving sepsis campaign: International guidelines for management of sepsis and septic shock 2021. Intensive Care Med. 47, 1181–1247. doi:10.1007/s00134-021-06506-y
Fowler, A. A., Syed, A. A., Knowlson, S., Sculthorpe, R., Farthing, D., DeWilde, C., et al. (2014). Phase I safety trial of intravenous ascorbic acid in patients with severe sepsis. J. Transl. Med. 12, 32. doi:10.1186/1479-5876-12-32
Fowler, A. A., Truwit, J. D., Hite, R. D., Morris, P. E., DeWilde, C., Priday, A., et al. (2019). Effect of vitamin C infusion on organ failure and biomarkers of inflammation and vascular injury in patients with sepsis and severe acute respiratory failure: The CITRIS-ALI randomized clinical trial. JAMA 322, 1261–1270. doi:10.1001/jama.2019.11825
Fujii, T., Luethi, N., Young, P. J., Frei, D. R., Eastwood, G. M., French, C. J., et al. (2020). Effect of vitamin C, hydrocortisone, and thiamine vs hydrocortisone alone on time alive and free of vasopressor Support among patients with septic shock: The VITAMINS randomized clinical trial. JAMA 323, 423–431. doi:10.1001/jama.2019.22176
Hatanaka, K., Ito, T., Madokoro, Y., Kamikokuryo, C., Niiyama, S., Yamada, S., et al. (2021). Circulating syndecan-1 as a predictor of persistent thrombocytopenia and lethal outcome: A population study of patients with suspected sepsis requiring intensive care. Front. Cardiovasc. Med. 8, 730553. doi:10.3389/fcvm.2021.730553
He, X., Su, F., Velissaris, D., Salgado, D. R., de Souza Barros, D., Lorent, S., et al. (2012). Administration of tetrahydrobiopterin improves the microcirculation and outcome in an ovine model of septic shock. Crit. Care Med. 40, 2833–2840. doi:10.1097/CCM.0b013e31825b88ba
Heitzer, T., Krohn, K., Albers, S., and Meinertz, T. (2000). Tetrahydrobiopterin improves endothelium-dependent vasodilation by increasing nitric oxide activity in patients with Type II diabetes mellitus. Diabetologia 43, 1435–1438. doi:10.1007/s001250051551
Heller, R., Unbehaun, A., Schellenberg, B., Mayer, B., Werner-Felmayer, G., and Werner, E. R. (2001). L-ascorbic acid potentiates endothelial nitric oxide synthesis via a chemical stabilization of tetrahydrobiopterin. J. Biol. Chem. 276, 40–47. doi:10.1074/jbc.M004392200
Ince, C., Mayeux, P. R., Nguyen, T., Gomez, H., Kellum, J. A., Ospina-Tascón, G. A., et al. (2016). The endothelium in sepsis. Shock 45, 259–270. doi:10.1097/shk.0000000000000473
Ismaeel, A., Papoutsi, E., Miserlis, D., Lavado, R., Haynatzki, G., Casale, G. P., et al. (2020). The nitric oxide system in peripheral artery disease: Connection with oxidative stress and biopterins. Antioxidants (Basel) 9, 590. doi:10.3390/antiox9070590
Jensen, I. J., McGonagill, P. W., Berton, R. R., Wagner, B. A., Silva, E. E., Buettner, G. R., et al. (2021). Prolonged reactive oxygen species production following septic insult. ImmunoHorizons 5, 477–488. doi:10.4049/immunohorizons.2100027
Kim, H. K., and Han, J. (2020). Tetrahydrobiopterin in energy metabolism and metabolic diseases. Pharmacol. Res. 157, 104827. doi:10.1016/j.phrs.2020.104827
Kim, J., Arnaout, L., and Remick, D. (2020). Hydrocortisone, ascorbic acid, and thiamine (HAT) therapy decreases oxidative stress, improves cardiovascular function, and improves survival in Murine sepsis. Shock 53, 460–467. doi:10.1097/SHK.0000000000001385
Kim, S. R., Ha, Y. M., Kim, Y. M., Park, E. J., Kim, J. W., Park, S. W., et al. (2015). Ascorbic acid reduces HMGB1 secretion in lipopolysaccharide-activated RAW 264.7 cells and improves survival rate in septic mice by activation of Nrf2/HO-1 signals. Biochem. Pharmacol. 95, 279–289. doi:10.1016/j.bcp.2015.04.007
Kirsch, M., Korth, H-G., Stenert, V., Sustmann, R., and de Groot, H. (2003). The autoxidation of tetrahydrobiopterin revisited: Proof of superoxide formation from reaction of tetrahydrobiopterin with molecular oxygen. J. Biol. Chem. 278, 24481–24490. doi:10.1074/jbc.m211779200
Kolluru, G. K., Bir, S. C., and Kevil, C. G. (2012). Endothelial dysfunction and diabetes: Effects on angiogenesis, vascular remodeling, and wound healing. Int. J. Vasc. Med. 2012, 918267. doi:10.1155/2012/918267
Lupu, F., Kinasewitz, G., and Dormer, K. (2020). The role of endothelial shear stress on haemodynamics, inflammation, coagulation and glycocalyx during sepsis. J. Cell. Mol. Med. 24, 12258–12271. doi:10.1111/jcmm.15895
Lv, S-J., Zhang, G-H., Xia, J-M., Yu, H., and Zhao, F. (2021). Early use of high-dose vitamin C is beneficial in treatment of sepsis. Ir. J. Med. Sci. 190, 1183–1188. doi:10.1007/s11845-020-02394-1
Marik, P. E., Khangoora, V., Rivera, R., Hooper, M. H., and Catravas, J. (2017). Hydrocortisone, vitamin C, and thiamine for the treatment of severe sepsis and septic shock: A retrospective before-after study. Chest 151, 1229–1238. doi:10.1016/j.chest.2016.11.036
Marik, P. E. (2018). Vitamin C for the treatment of sepsis: The scientific rationale. Pharmacol. Ther. 189, 63–70. doi:10.1016/j.pharmthera.2018.04.007
May, J. M., and Harrison, F. E. (2013). Role of vitamin C in the function of the vascular endothelium. Antioxid. Redox Signal. 19, 2068–2083. doi:10.1089/ars.2013.5205
Mayahi, L., Heales, S., Owen, D., Casas, J. P., Harris, J., MacAllister, R. J., et al. (2007). 6R)-5, 6, 7, 8-tetrahydro-L-biopterin and its stereoisomer prevent ischemia reperfusion injury in human forearm. Arterioscler. Thromb. Vasc. Biol. 27, 1334–1339. doi:10.1161/ATVBAHA.107.142257
McKinnon, R. L., Lidington, D., and Tyml, K. (2007). Ascorbate inhibits reduced arteriolar conducted vasoconstriction in septic mouse cremaster muscle. Microcirculation 14, 697–707. doi:10.1080/10739680701410389
Mortensen, A., and Lykkesfeldt, J. (2014). Does vitamin C enhance nitric oxide bioavailability in a tetrahydrobiopterin-dependent manner? in vitro, in vivo and clinical studies. Nitric Oxide 36, 51–57. doi:10.1016/j.niox.2013.12.001
Moskowitz, A., Andersen, L. W., Huang, D. T., Berg, K. M., Grossestreuer, A. V., Marik, P. E., et al. (2018). Ascorbic acid, corticosteroids, and thiamine in sepsis: A review of the biologic rationale and the present state of clinical evaluation. Crit. Care 22, 283. doi:10.1186/s13054-018-2217-4
Moskowitz, A., Huang, D. T., Hou, P. C., Gong, J., Doshi, P. B., Grossestreuer, A. V., et al. (2020). Effect of ascorbic acid, corticosteroids, and thiamine on organ injury in septic shock: The ACTS randomized clinical trial. JAMA 324, 642–650. doi:10.1001/jama.2020.11946
Obi, J., Pastores, S. M., Ramanathan, L. V., Yang, J., and Halpern, N. A. (2020). Treating sepsis with vitamin C, thiamine, and hydrocortisone: Exploring the quest for the magic elixir. J. Crit. Care 57, 231–239. doi:10.1016/j.jcrc.2019.12.011
Ostrowski, S. R., Haase, N., Müller, R. B., Møller, M. H., Pott, F. C., Perner, A., et al. (2015). Association between biomarkers of endothelial injury and hypocoagulability in patients with severe sepsis: A prospective study. Crit. Care 19, 191. doi:10.1186/s13054-015-0918-5
Padayatty, S. J., Doppman, J. L., Chang, R., Wang, Y., Gill, J., Papanicolaou, D. A., et al. (2007). Human adrenal glands secrete vitamin C in response to adrenocorticotrophic hormone. Am. J. Clin. Nutr. 86, 145–149. doi:10.1093/ajcn/86.1.145
Patak, P., Willenberg, H. S., and Bornstein, S. R. (2004). Vitamin C is an important cofactor for both adrenal cortex and adrenal medulla. Endocr. Res. 30, 871–875. doi:10.1081/erc-200044126
Pathak, R., Pawar, S. A., Fu, Q., Gupta, P. K., Berbée, M., Garg, S., et al. (2014). Characterization of transgenic gfrp knock-in mice: Implications for tetrahydrobiopterin in modulation of normal tissue radiation responses. Antioxid. Redox Signal. 20, 1436–1446. doi:10.1089/ars.2012.5025
Porkert, M., Sher, S., Reddy, U., Cheema, F., Niessner, C., Kolm, P., et al. (2008). Tetrahydrobiopterin: A novel antihypertensive therapy. J. Hum. Hypertens. 22, 401–407. doi:10.1038/sj.jhh.1002329
Rittirsch, D., Huber-Lang, M. S., Flierl, M. A., and Ward, P. A. (2009). Immunodesign of experimental sepsis by cecal ligation and puncture. Nat. Protoc. 4, 31–36. doi:10.1038/nprot.2008.214
Rudd, K. E., Johnson, S. C., Agesa, K. M., Shackelford, K. A., Tsoi, D., Kievlan, D. R., et al. (2020). Global, regional, and national sepsis incidence and mortality, 1990-2017: Analysis for the global burden of disease study. Lancet 395, 200–211. doi:10.1016/S0140-6736(19)32989-7
Scholz, S. S., Borgstedt, R., Ebeling, N., Menzel, L. C., Jansen, G., and Rehberg, S. (2021). Mortality in septic patients treated with vitamin C: A systematic meta-analysis. Crit. Care 25, 17. doi:10.1186/s13054-020-03438-9
Stuehr, D., Pou, S., and Rosen, G. M. (2001). Oxygen reduction by nitric-oxide synthases. J. Biol. Chem. 276, 14533–14536. doi:10.1074/jbc.R100011200
Takeda, M., Yamashita, T., Shinohara, M., Sasaki, N., Takaya, T., Nakajima, K., et al. (2009). Plasma tetrahydrobiopterin/dihydrobiopterin ratio: A possible marker of endothelial dysfunction. Circ. J. 73, 955–962. doi:10.1253/circj.cj-08-0850
Tani, Y., and Ohno, T. (1993). Analysis of 6R- and 6S-tetrahydrobiopterin and other pterins by reversed-phase ion-pair liquid-chromatography with fluorimetric detection by post-column sodium nitrite oxidation. J. Chromatogr. 617, 249–255. doi:10.1016/0378-4347(93)80495-p
Tyml, K., Li, F., and Wilson, J. X. (2008). Septic impairment of capillary blood flow requires nicotinamide adenine dinucleotide phosphate oxidase but not nitric oxide synthase and is rapidly reversed by ascorbate through an endothelial nitric oxide synthase-dependent mechanism. Crit. Care Med. 36, 2355–2362. doi:10.1097/ccm.0b013e31818024f6
Uchimido, R., Schmidt, E. P., and Shapiro, N. I. (2019). The glycocalyx: A novel diagnostic and therapeutic target in sepsis. Crit. Care 23, 16. doi:10.1186/s13054-018-2292-6
Vandervelden, S., Wauters, L., Breuls, J., Fieuws, S., Vanhove, P., Hubloue, I., et al. (2021). Early administration of vitamin C in patients with sepsis or septic shock in emergency departments: A multicenter, double blinded, randomized controlled trial: The C-easie trial protocol. PLoS One 16, e0259699. doi:10.1371/journal.pone.0259699
Vásquez-Vivar, J., Martásek, P., Whitsett, J., Joseph, J., and Kalyanaraman, B. (2002). The ratio between tetrahydrobiopterin and oxidized tetrahydrobiopterin analogues controls superoxide release from endothelial nitric oxide synthase: An EPR spin trapping study. Biochem. J. 362, 733–739. doi:10.1042/0264-6021:3620733
Vásquez-Vivar, J., Whitsett, J., Martásek, P., Hogg, N., and Kalyanaraman, B. (2001). Reaction of tetrahydrobiopterin with superoxide: EPR-kinetic analysis and characterization of the pteridine radical. Free Radic. Biol. Med. 31, 975–985. doi:10.1016/s0891-5849(01)00680-3
Wu, F., Wilson, J. X., and Tyml, K. (2003). Ascorbate inhibits iNOS expression and preserves vasoconstrictor responsiveness in skeletal muscle of septic mice. Am. J. Physiol. Regul. Integr. Comp. Physiol. 285, R50–R56. doi:10.1152/ajpregu.00564.2002
Keywords: ascorbic acid, sepsis, tetrahydrobiopterin, endothelial dysfunction, syndecan
Citation: Madokoro Y, Kamikokuryo C, Niiyama S, Ito T, Hara S, Ichinose H and Kakihana Y (2022) Early ascorbic acid administration prevents vascular endothelial cell damage in septic mice. Front. Pharmacol. 13:929448. doi: 10.3389/fphar.2022.929448
Received: 02 May 2022; Accepted: 21 September 2022;
Published: 06 October 2022.
Edited by:
Lei Xi, School of Medicine, Virginia Commonwealth University, United StatesReviewed by:
Jianxiang Xue, University of South Florida, United StatesAllison M. Owen, University of Kentucky, United States
Copyright © 2022 Madokoro, Kamikokuryo, Niiyama, Ito, Hara, Ichinose and Kakihana. This is an open-access article distributed under the terms of the Creative Commons Attribution License (CC BY). The use, distribution or reproduction in other forums is permitted, provided the original author(s) and the copyright owner(s) are credited and that the original publication in this journal is cited, in accordance with accepted academic practice. No use, distribution or reproduction is permitted which does not comply with these terms.
*Correspondence: Yasuyuki Kakihana, a2FraWhhbmFAbTMua3VmbS5rYWdvc2hpbWEtdS5hYy5qcA==