- 1College of Pharmacy, Jinan University, Guangzhou, China
- 2Institute of Molecular Rhythm and Metabolism, Guangzhou University of Chinese Medicine, Guangzhou, China
- 3School of Medicine, Jinan University, Guangzhou, China
Scutellaria baicalensis Georgi (SBG) is a traditional Chinese medicine widely used to treat disorders such as hypertension, dysentery and hemorrhaging. Here, we aimed to assess the pharmacological effects of SBG on skin aging and to investigate the underlying mechanisms. Mice with skin aging were established by treatment with D-galactose and ultraviolet-B. SBG (topical application) showed a protective effect on skin aging in mice, as evidenced by less formation of skin wrinkles, higher levels of SOD (superoxide dismutase) and HYP (hydroxyproline) as well as a lower level of MDA (malondialdehyde). In the meantime, skin MMP-1 and p53 expression were lower, epidermis was thinner and collagen amount was higher in SBG-treated mice. Anti-skin aging effects of SBG were also confirmed in NIH3T3 and HaCaT cells, as well as in mouse primary dermal fibroblasts and human primary epidermal keratinocytes. Furthermore, we found that loss of Rev-erbα (a known repressor of Bmal1) up-regulated skin BMAL1 (a clock component and a known anti-aging factor) and ameliorated skin aging in mice. Moreover, SBG dose-dependently increased the expression of BMAL1 in the skin of aged mice and in senescent NIT3H3 cells. In addition, based on a combination of Gal4 chimeric, luciferase reporter and expression assays, SBG was identified as an antagonist of REV-ERBα and thus an inducer of BMAL1 expression. In conclusion, SBG antagonizes REV-ERBα to up-regulate BMAL1 and to protect against skin aging in mice.
Introduction
Scutellaria baicalensis Georgi (SBG, also known as Huangqin in Chinese), a traditional Chinese medicine, possesses various pharmacological effects such as anti-inflammatory, antiviral, anticancer, anti-oxidant and antibacterial activities. SBG is widely used to treat diarrhea, hypertension, dysentery and hemorrhaging (Liao et al., 2021). Many types of chemicals are found in SBG, including flavones, phenylethanoids, amino acids, sterols and essential oils (Zhao et al., 2016). Of note, flavones (e.g., baicalin, baicalein, wogonin and oroxylin A) are thought to be a major class of active ingredients of SBG as they show the health-promoting effects (such as anti-inflammation, antivirus, anticancer, anti-oxidation and anti-bacteria) typically observed for SBG (Brown, 1980; Li et al., 2004). It is interesting to note that SBG has great potential to manage the skin diseases caused by sunlight irradiation (Brown, 1980). The underlying mechanisms may involve scavenging of free radicals and attenuation of lipid oxidation (Gabrielska et al., 1997). However, it remains unknown whether SBG can protect against skin aging.
Skin aging is classified into intrinsic (chronological) and extrinsic aging, and the latter is also referred to as premature skin aging or photoaging (Bocheva et al., 2019). Intrinsic aging is an unpreventable spontaneous process, whereas extrinsic aging caused by exogenous factors (e.g., ultraviolet/UV light, cigarette smoking and pollution) is preventable (Fisher et al., 2002; Bernhard et al., 2007). UV radiation is a major cause of photoaging, and can be divided into three bands [i.e., UVA (315–400 nm), UVB (280–315 nm) and UVC (100–280 nm)]. Of note, UVB is the predominant form that causes injuries to living organisms (Slominski et al., 2018a; Frommeyer et al., 2022). UV radiation not only induces skin pathology, but also exert systemic effects, including activation of hypothalamic-pituitary-adrenal axis, opioidogenic effects, and immunosuppression. Thus, UV radiation has therapeutic applications in management of various diseases such as addiction, autoimmune and mood disorders (Slominski et al., 2018a). Although skin aging is regarded as a cosmetic problem, it can result in disfigurement and skin diseases (such as skin cancers) and has profound psychological consequences (Watson et al., 2016; Narayanan et al., 2010). There are two major classes of agents for management of skin aging, namely, antioxidants and cell regulators. However, these medications (e.g., retinoid) are concerned with the lack of effectiveness and/or adverse effects (Krutmann et al., 2021; Mukherjee et al., 2006). Therefore, it is of value to search for more effective and safer therapeutic agents.
BMAL1 (Brain and muscle ARNT-like protein 1) is a transcription factor and a core component of circadian clock system, which generates and maintains circadian rhythms in most aspects of physiology and behaviors (Gekakis et al., 1998; Hogenesch et al., 1998; Bunger et al., 2000). Bmal1 and other clock genes work cooperatively to drive circadian gene expression using a negative feedback mechanism (Chen et al., 2009; Duong et al., 2011). BMAL1 forms a heterodimer with CLOCK (circadian locomotor output cycles kaput) to activate the transcription of Pers (periods) and Crys (cryptochromes) as well as many other clock-controlled genes (CCGs) (Langmesser et al., 2008). Once reaching a critical level, PER and CRY proteins in turn inhibit the activity of the BMAL1/CLOCK dimer, bringing down the levels of CCGs (Böger, 2014). As PER and CRY proteins are reduced due to degradation, a new cycle of BMAL1/CLOCK-driven transcription can begin (Duong et al., 2011). In addition to regulating circadian rhythms, BMAL1 plays a role in the development and progression of many types of diseases such as cancers (Jung et al., 2013), obesity (Hemmeryckx et al., 2011), and neurodegenerative disorders (Vieira et al., 2020). Notably, Bmal1 is also involved in aging (Khapre et al., 2011). Bmal1-deficient mice have reduced lifespan and are prone to premature aging (exemplified by sarcopenia, cataracts, reduced subcutaneous fat, decreased organ size and impaired hair growth) (Kondratov et al., 2006). Bmal1 regulates aging via modulation of the expression of major antioxidant enzymes including SOD, peroxiredoxines and glutathione peroxidase (Kondratov et al., 2009).
REV-ERBα (also known as NR1D1, nuclear receptor subfamily one group D member 1) is a nuclear receptor that participates in regulation of circadian rhythms via inhibiting BMAL1 expression (Yin and Lazar, 2005). REV-ERBα functions as a transcriptional repressor that inhibits the transcription of target genes (e.g., Bmal1) by binding to a response element (called RevRE) in the promoters and recruiting the corepressors nuclear corepressor one and histone deacetylase 3 (Liu et al., 2008; Yin et al., 2010). REV-ERBα has been also implicated in regulation of a variety of diseases including inflammatory diseases (e.g., fulminant hepatitis, pulmonary inflammation, and colitis) (Wang et al., 2020), metabolic disorders (Delezie et al., 2012) and cancers (Wang et al., 2015). SR8278 (a synthetic compound) is identified as an antagonist of REV-ERBα and widely used to probe the function of REV-ERBα (Kojetin et al., 2011; Pardee et al., 2011). Notably, we recently found that REV-ERBα restrains Propionibacterium acnes-induced skin inflammation through inhibiting the NF-κB/NLRP3 axis to protect against acne vulgaris (Li et al., 2022). However, it remains elusive whether and how REV-ERBα regulates skin aging.
In the present study, we aimed to assess the pharmacological effects of SBG on skin aging and to investigate the underlying mechanisms. Anti-skin aging effects of SBG were evaluated using mouse and cell models of aging (induced by D-galactose and/or ultraviolet-B). Skin aging was assessed by analyzing SOD, HYP, MDA, ROS and MMP-1/p53 and by measuring epidermal thickness and collagen content. The role of REV-ERBα/BMAL1 in regulating skin aging was assessed using gene knockout mice. Antagonism of REV-ERBα was determined using Gal4 chimeric assay. We for the first time demonstrated that SBG antagonizes REV-ERBα to up-regulate BMAL1 (a skin aging-inhibiting factor) and to protect against skin aging in mice.
Materials and methods
Materials
SBG was purchased from Biopurify Phytochemicals (Chengdu, China). D-galactose (D-gal) was purchased from Aladdin (Shanghai, China). Vitamin C was obtained from Yuanye Biotechnology (Shanghai, China). Biochemical kits for SOD, MDA, ROS and HYP were purchased from Jiancheng Bioengineering Institute (Nanjing, Jiangsu, China). Staining kit for senescence-associated-β-galactosidase (SA-β-gal) was obtained from Beyotime Biotechnology (Shanghai, China). Antibodies against GAPDH, MMP-1, p53, BMAL1, REV-ERBɑ, BHMT and NLRP3 were purchased from Abcam (Cambridge, United Kingdom).
Preparation of SBG extract
SBG was extracted for 90 min by refluxing in 80% ethanol (3:20, w/v), and filtered with filter paper. The filtrate was concentrated and freeze-dried. The dry residue (SBG extract) was stored at -20°C. The extraction yield was 23.3%, and the main active ingredients of SBG are shown in Supplementary Table S1. For animal experiments, SBG extract was mixed with a homemade cream containing stearic acid, triethanolamine, and propylene glycol.
Animals
C57BL/6 mice (10 weeks old) weighing 18–22 g were obtained from HFK Bioscience (Beijing, China). Rev-erbɑ−/− mice (on a C57BL/6 background) have been established and validated in our laboratory (Wang et al., 2018). All mice were maintained on a 12 h light/12 h dark cycle, with free access to food and water. Mice were individually placed in the cages to prevent offensive behaviors from other mice, that may cause injuries to the skin. Mice from the same litter (with hair growth in the anagen phase) were used for experiments. Note that we used male mice to assess the therapeutic effect of SBG on skin aging, without considering factors such as hormone-induced wrinkling of skin. Protocols for animal experiments were approved by the Institutional Animal Care and Use Committee of Guangzhou University of Chinese Medicine (Appr. Date: 2021–05-17; IACUC Issue No: ZYD-2021–112).
LC-MS/MS analysis
The main active constituents (i.e., wogonoside, baicalein, baicalin and wogonin) in SBG extract were quantified using a Shimadzu LCMS-8045 triple quadrupole liquid chromatograph mass spectrometer (LC-MS) equipped with Shimadzu-Nexera XR high-performance liquid chromatography (HPLC). The mobile phases consisted of acetonitrile (A) and water (B). Flow rate was set at 0.3 ml/min. Gradient elution program was 40% B (0–1 min), 40–10% B (1–3 min), 10% B (3–4 min) and 10–40% B (4–5 min). Mass spectrometer was operated at positive ion scan mode. The mass transition ion pairs and contents of main active constituents are provided in Supplementary Table S1
Mouse model of skin aging and drug treatment
To induce skin aging, mice were injected subcutaneously with 250 mg/kg D-gal daily in the back neck and irradiated on the back daily with 120 mJ/cm2 UVB for 6 weeks as previously described (Zhang et al., 2020). The source of radiation was a narrow band UVB bulb (Philips model PL-9 9W/01/2P) emitting photons with wavelengths between 306 and 316 nm, with a peak at 312 nm. The distance from the UVB lamp (KN-4003BL, Kernel Medical Equipment, Xuzhou, China) to the mouse back was 25 cm. Control mice were injected subcutaneously with vehicle (saline). To assess the effects of SBG on skin aging, SBG extract (25, 100 or 400 mg/kg), vitamin C (40 mg/kg) or vehicle was applied topically (once daily after UVB exposure) on the skin of mouse models for 4 weeks from the third week. Mice were sacrificed to collect skin samples, followed by qPCR, Western blotting and biochemical analyses (SOD, MDA and HYP).
Isolation of mouse primary dermal fibroblasts
Mouse primary dermal fibroblasts were isolated from newborn mice as previously described (Terao et al., 2014). The newborn mice were sacrificed by rapid cervical dislocation. Trunk skin was peeled off and incubated with 4 mg/ml dispase overnight at 4 °C. On the next day, the dermis was separated from the epidermis using forceps, and incubated with 0.25% trypsin for 10 min. After filtration, cells were centrifuged at 200 g for 10 min, resuspended in Dulbecco’s modified Eagle’s medium (DMEM) supplemented with 10% fetal bovine serum (FBS) and incubated at 37°C and 5% CO2.
Cell culture and treatment
NIH3T3, HaCaT and Primary adult human epidermal keratinocytes (HEKa) cells were obtained from the American Type Culture Collection (Rockville, MD). NIH3T3, HaCaT and HEKa were maintained in DMEM supplemented with 10% FBS, 100 U/ml penicillin and 100 mg/ml streptomycin. To induce NIH3T3 cell senescence, 8 g/L D-gal was added to the culture medium for 96 h. To induce cell senescence of HaCaT, mouse primary dermal fibroblasts and HEKa, cells were subjected to UVB irradiation (100 mJ/cm2) with a thin layer of PBS (phosphate-buffered saline) using a UVB lamp (KN-4003BL, Kernel Medical Equipment, Xuzhou, China). Cells were then treated with SBG or vehicle. On next day, cells were collected for qPCR and Western blotting.
Gal4 co-transfection assay
Gal4 co-transfectionthe assay was performed as previously described (Zhang et al., 2018). In brief, HEK293 cells were co-transfected with pGal4-Rev-erbα-LBD plasmid (200 ng), pGL-4.35-Luc reporter (100 ng, a Gal4-reponsive luciferase reporter) and pRL-TK vector (10 ng) using jetPRIME (Polyplus Transfection, Illkirch, France). On next day, cells were treated with SBG or SR8278 or vehicle. 24 h later, luciferase activities were measured using the Dual-Luciferase Reporter Assay system and GloMax 20/20 luminometer (Promega Madison, WI).
Luciferase reporter assays
Luciferase reporter assays were performed as previously described (Chen et al., 2020). In brief, NIH3T3 cells were cultured in DMEM medium (containing 10% FBS, 1% penicillin-streptomycin) and transfected with 250 ng of Bmal1 luciferase reporter plasmid and 50 ng of pRL-TK using jetPRIME (Polyplus Transfection, Illkirch, France). On next day, SBG or SR8278 or vehicle was added to the culture medium for 24 h. Cells were harvested and lysed with passive lysis buffer. Luciferase activities were detected using the Dual-Luciferase Reporter Assay system and GloMax 20/20 luminometer (Promega, Madison, WI).
H&E and masson staining
Skin samples were fixed in 10% formalin, embedded in paraffin, and cut to 4 μm-thick sections. The sections were subjected to H&E (hematoxylin and eosin) and Masson staining. The images were captured using an optical microscope (Olympus, Tokyo, Japan).
Measurement of intracellular ROS
Cells were treated with 2′7′-dichlorodihydrofluorescein diacetate (10 μM) at 37°C for 1 h. Fluorescence intensity was determined at excitation (485 nm) and emission (530 nm) wavelengths using a Synergy HT Multi-Mode Microplate Reader (BioTek, Winooski, VT).
MTT assay
MTT assays were performed to determine cell viability as previously described (Kumar et al., 2018). Briefly, cells were incubated with MTT [3-(4,5-dimethylthiazol-2-yl)-2,5 diphenyl tetrazolium bromide, 0.5 mg/ml] for 4 h, and the formazan crystals were dissolved in 100 μl DMSO. The absorbance was determined at 570 nm using a Synergy HT Multi-Mode Microplate Reader (BioTek, Winooski, VT).
SA-β-gal assay
SA-β-gal activity was measured using a SA-β-gal staining kit according to the manufacturer’s instructions (Beyotime, Shanghai, China). Briefly, cells were fixed in a fixing solution for 15 min, washed with PBS and incubated with senescence detection solution at 37°C. On next day, the number of SA-β-gal-positive cells were determined by counting blue-stained cells using an Eclipse ci-L microscope (Nikon, Tokyo, Japan).
Real-time luminescence monitoring
Real-time luminescence monitoring was performed as previously described (Hirota et al., 2008; du Pré et al., 2017). In brief, NIH3T3 cells stably overexpressed with Bmal1-dLuc were seeded into a 35 mm dish and maintained in DMEM containing 10% FBS. On next day, cells were incubated with a recording medium containing 1 μg/ml SBG or vehicle. Luminescence data (counts/s) were collected by using Lumicycle 32 (Actimetrics, Wilmette, IL).
Western blotting
Protein samples were subjected to 10% sodium dodecyl sulfate-polyacrylamide gel electrophoresis (SDS-PAGE), and transferred to PVDF membranes. The membranes were blocked with 5% skim milk, and sequentially incubated with primary and secondary antibodies. Protein bands were visualized by using enhanced chemiluminescence and Omega Lum G imaging system (Aplegen, Pleasanton, CA), and quantified with Fluorchem 5500 software (Fisher Scientific, Fair Lawn, NJ). GAPDH was used as a loading control.
qPCR (quantitative polymerase chain reaction)
RNA was extracted using RNAiso Plus reagent (Takara, Shiga, Japan) and transcribed to cDNA using PrimeScript RT Master Mix (Vazyme, Jiangsu, China). qPCR reactions were performed using the SYBR Premix Ex Taq (Vazyme, Jiangsu, China). Amplification procedures consisted of an initial denaturation at 95°C for 5 min, 40 cycles of denaturation at 95°C for 15 s, annealing at 60°C for 30 s, and extension at 72°C for 30 s. Mouse Gapdh was used as an internal control. Gene expression was determined using the 2−ΔΔCT method. Primers are provided in Table 1.
Statistical analysis
Data are presented as means ± SD (standard deviation). Comparisons between two groups were analyzed using Student’s t-test. One-way ANOVA followed by Bonferroni post hoc test was performed to compare means of more than two groups. The level of significance was set at p < 0.05 (*).
Results
SBG protects against skin aging induced by D-gal/UVB in mice
Mice with skin aging were established by treatment with D-gal/UVB as previously described (du Pré et al., 2017). As expected, D-gal/UVB-treated mice showed skin aging-like symptoms such as roughness, stiffness, and lack of elasticity (Figure 1A). These mice had lower levels of SOD activity and HYP and a higher level of MDA in the skin as compared to normal mice (Figure 1B). We also observed marked increases in the expression of Mmp-1 and p53 (two genes closely associated with skin aging) in the skin of D-gal/UVB-treated mice (Figure 1C). In addition, the epidermis (D-gal/UVB-exposed region) was thicker in aging mice than in control mice according to H&E staining (Figure 1D). These data indicated successful construction of mice with skin aging.
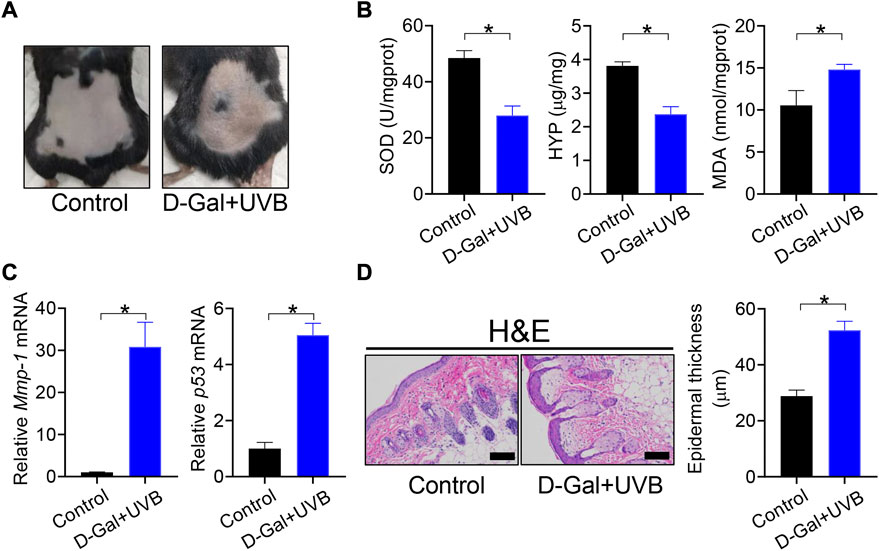
FIGURE 1. Establishment of mice with skin aging. (A) Surface examination of dorsal skin derived from D-gal/UVB-treated and control mice. (B) Skin SOD activity and HYP/MDA levels in D-gal/UVB-treated and control mice. Data are mean ± SD (n = 7). *p < 0.05 (t-test). (C) mRNA expression of Mmp-1 and p53 in the skin of D-gal/UVB-treated and control mice. Data are mean ± SD (n = 7). *p < 0.05 (t-test). (D) H&E staining (left panel) and epidermal thickness (right panel) of skin derived from D-gal/UVB-treated and control mice. Scale bar = 100 μm.
We next assessed the effects of SBG (topical application) on skin aging in mice. Like vitamin C (a known anti-aging agent and used as a positive control), SBG reduced the wrinkle formation in the skin of D-gal/UVB-treated mice (Figure 2A). Further, SBG dose-dependently increased the SOD activity and HYP level, and decreased the MDA level in the skin of aging mice (Figure 2B). Moreover, SBG reduced the mRNAs and proteins of both MMP-1 and p53 in the skin of aging mice in a dose-dependent manner (Figure 2C/D). In addition, compared to vehicle-treated aging mice, SBG-treated mice had thinner D-gal/UVB-exposed epidermis, and a higher amount of collagen (Figure 3A/B). Taken together, these findings clearly indicated a protective effect of SBG on skin aging in mice.
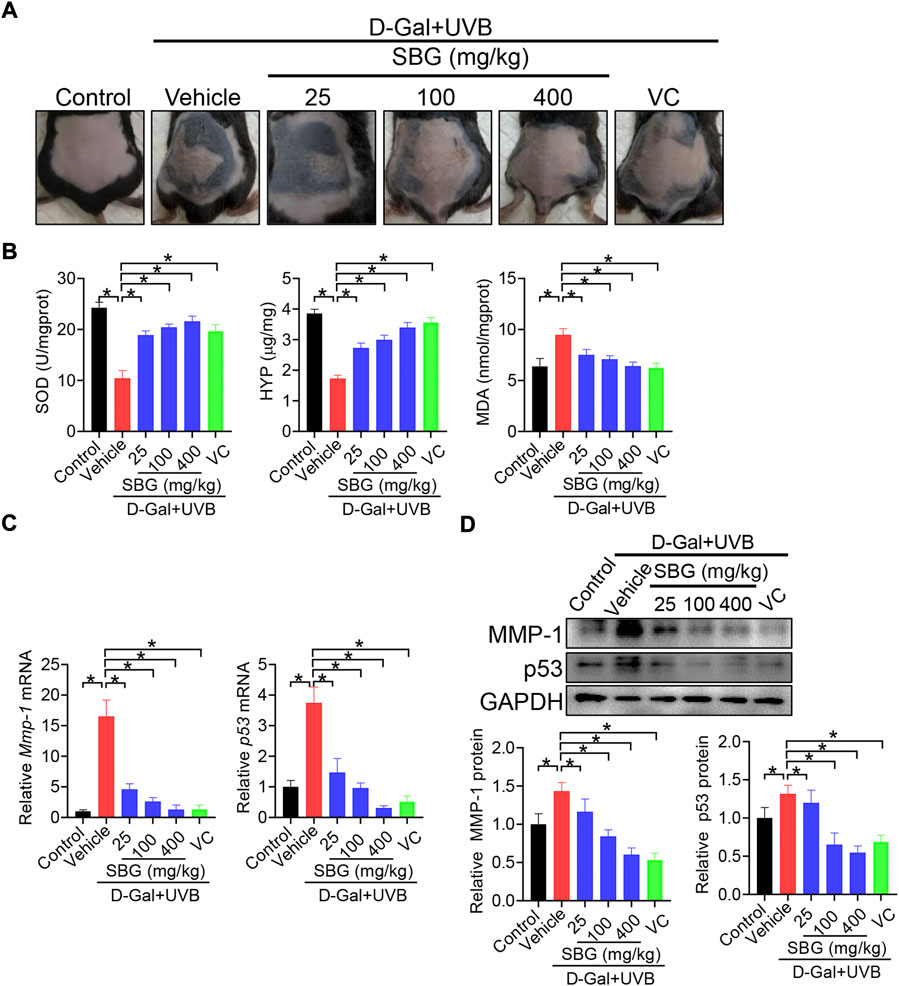
FIGURE 2. SBG protects against skin aging induced by D-gal/UVB in mice. (A) Surface examination of dorsal skin derived from D-gal/UVB-treated and control mice. (B) Skin SOD activity and HYP/MDA levels in D-gal/UVB-treated and control mice. (C) mRNA expression of Mmp-1 and p53 in the skin of D-gal/UVB-treated and control mice. (D) Protein expression (top panel) and quantification (bottom panel) of MMP-1/p53 in the skin of D-gal/UVB-treated and control mice. In panels B–D, data are mean ± SD (n = 7). *p < 0.05 (one-way ANOVA and Bonferroni post hoc test). VC, vitamin.
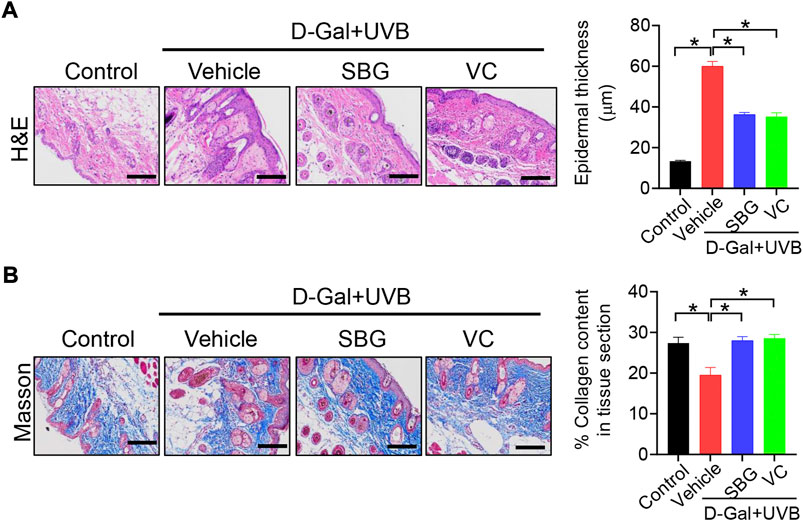
FIGURE 3. Effects of SBG on epidermal thickness and collagen content in aging mice. (A) H&E staining (left panel) and epidermal thickness (right panel) of skin derived from D-gal/UVB-treated and control mice. Scale bar = 100 μm. (B) Masson staining of the skin derived from D-gal/UVB-treated and control mice. The right panel shows collagen content in the skin. Data are mean ± SD (n = 7). *p < 0.05 (one-way ANOVA and Bonferroni post hoc test). Scale bar = 100 μm.
SBG attenuates D-gal- and UVB-induced cell senescence
We next investigated whether SBG can affect cell senescence using NIH3T3 and HaCaT cells. NIH3T3 cells were exposed to 8 g/L D-gal for 96 h to induce cell senescence as previously described (Gao et al., 2014). As expected, D-gal treatment of NIH3T3 cells resulted in senescence as evidenced by a decrease in cell viability, and elevations in ROS level and MMP-1/p53 expression, as well as an increase in senescence-associated β-galactosidase (SA-β-gal, a senescence-specific marker) activity (Figure 4). We found that SBG dose-dependently increased the cell viability and decreased the ROS level in D-gal-treated NIH3T3 cells (Figure 4A/B). Furthermore, SBG down-regulated the mRNA levels of both Mmp-1 and p53 in a dose-dependent fashion in D-gal-treated cells (Figure 4C). In line with the mRNA changes, MMP-1 and p53 proteins were reduced by SBG in the aged cells (Figure 4D). Moreover, SBG-treated senescent cells had a lower activity of SA-β-gal (Figure 4E).
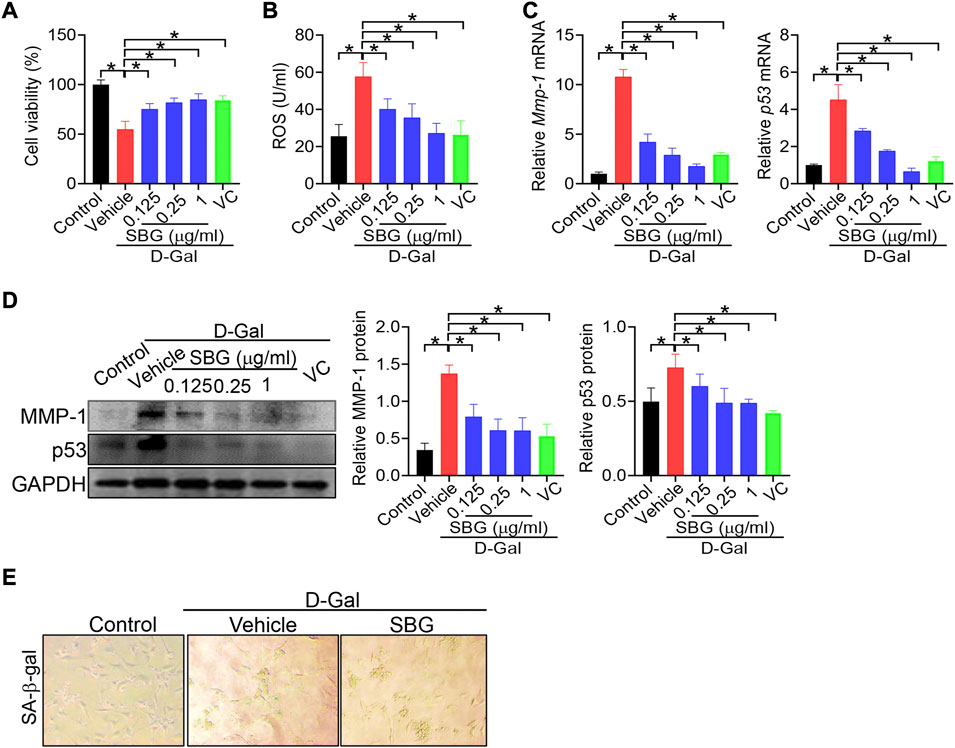
FIGURE 4. SBG attenuates D-gal-induced senescence in NIH3T3 cells. (A) Effects of SBG on the viability of D-gal-treated NIH3T3 cells. (B) Effects of SBG on ROS accumulation in D-gal-treated NIH3T3 cells. (C) Effects of SBG on Mmp-1 (left panel) and p53 (right panel) mRNA expression in D-gal-treated NIH3T3 cells. (D) Effects of SBG on protein expression of MMP-1 and p53 in D-gal-treated NIH3T3 cells. (E) Representative images showing SA-β-Gal activity in SBG-treated senescent NIH3T3 cells. In panels A–D, data are mean ± SD (n = 3). *p < 0.05 (one-way ANOVA and Bonferroni post hoc test).
We additionally examined the effects of SBG on senescene of HaCaT, mouse primary dermal fibroblasts and HEKa cells induced by UVB. SBG increased the cell viability and decreased the ROS level and MMP-1/p53 expression in the senescent cells (Figures 5, 6). These similar effects were also observed for vitamin C (Figures 5, 6). Altogether, these findings supported that SBG had a protective effect on skin aging.
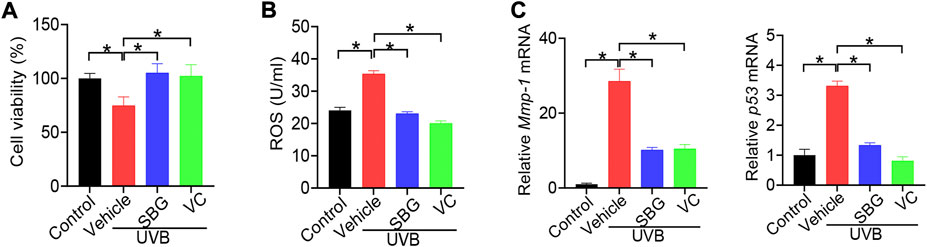
FIGURE 5. SBG attenuates UVB-induced senescence in HaCaT cells. (A) Effects of SBG on the viability of UVB-treated HaCaT cells. (B) Effects of SBG on ROS accumulation in UVB-treated HaCaT cells. (C) Effects of SBG on the mRNA expression of Mmp-1 and p53 in UVB-treated HeCaT cells. Data are mean ± SD (n = 3). *p < 0.05 (one-way ANOVA and Bonferroni post hoc test).
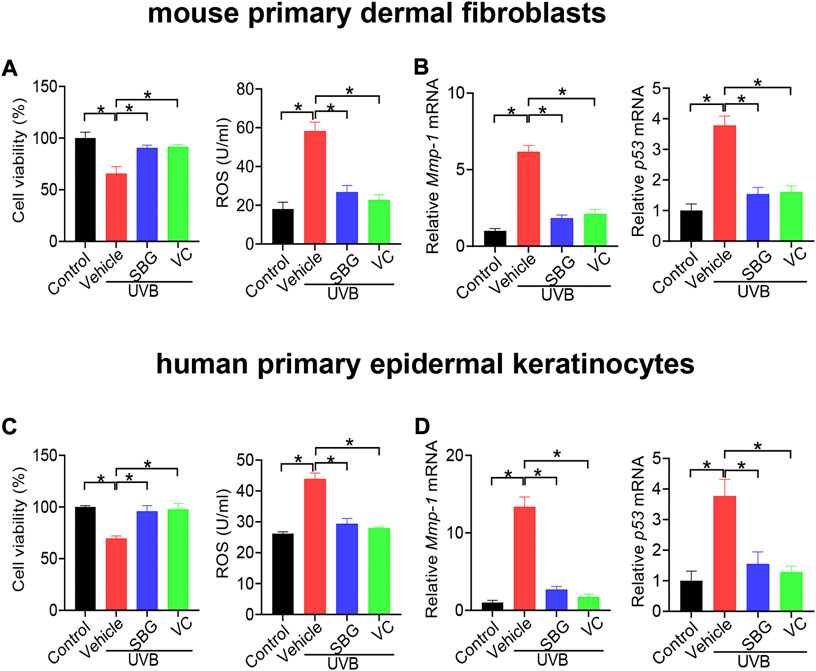
FIGURE 6. SBG attenuates UVB-induced senescence in mouse primary dermal fibroblasts and HEKa cells. (A) Effects of SBG on cell viability and ROS accumulation for UVB-treated mouse primary dermal fibroblasts. (B) Effects of SBG on mRNA expression of Mmp-1 and p53 in UVB-treated mouse primary dermal fibroblasts. (C) Effects of SBG on cell viability and ROS accumulation for UVB-treated HEKa cells. (D) Effects of SBG on mRNA expression of Mmp-1 and p53 in UVB-treated HEKa cells. Data are mean ± SD (n = 3). *p < 0.05 (one-way ANOVA and Bonferroni post hoc test).
Loss of Rev-erbα up-regulates skin BMAL1 and ameliorates skin aging in mice
Deficiency of Bmal1 has been previously shown to promote premature aging in mice, identifying Bmal1 as a key regulator of aging (Kondratov et al., 2006). Bmal1 regulates aging via modulation of the expression of antioxidant enzymes including SOD, peroxiredoxines and glutathione peroxidase (Kondratov et al., 2009). Bmal1 is a target gene of REV-ERBα, which functions as a transcriptional repressor (Crumbley and Burris, 2011). Loss of Rev-erbα leads to up-regulation of BMAL1 (Burris, 2008). Thus, we hypothesized that Rev-erbα may promote skin aging considering that Bmal1 has a protective role. To test this hypothesis, we generated and tested a mouse line with deletion of Rev-erbα gene (Rev-erbα−/− mice). Rev-erbα−/− mice were validated by qPCR, and showed the absence of wild-type Rev-erbα transcript in the skin (Figure 7A). As expected, BMAL1 mRNA and protein were up-regulated in the skin of Rev-erbα−/− mice (Figure 7B). Rev-erbα−/− and control mice were subjected to induction of skin aging by D-gal/UVB. We found that loss of Rev-erbα ameliorated skin aging as evidenced by less formation of skin wrinkles, higher levels of SOD and HYP as well as a lower level of MDA (Figure 7C/D). In the meantime, skin MMP-1 and p53 expression were lower, epidermis was thinner and collagen amount was higher in Rev-erbα−/− mice (Figures 7E–G). Taken together, Rev-erbα ablation up-regulated skin BMAL1 and ameliorated skin aging in mice. Our findings supported a critical role of REV-ERBα/BMAL1 in regulation of skin aging.
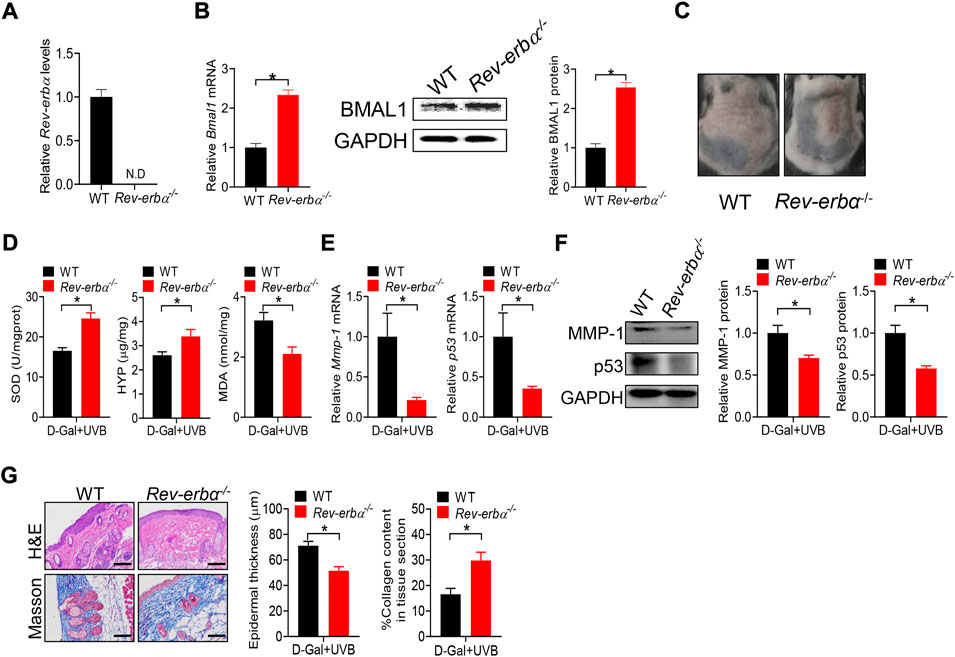
FIGURE 7. Loss of Rev-erbα up-regulates skin BMAL1 and ameliorates skin aging in mice. (A) mRNA expression of Rev-erbα in skin derived from Rev-erbα−/− and wild-type (WT) mice. (B) mRNA (left panel) and protein (right panel) expression of BMAL1 in skin derived from Rev-erbα−/− and WT mice. (C) Surface examination of dorsal skin derived from Rev-erbα−/− and WT mice treated with D-gal/UVB. (D) Skin SOD activity and HYP/MDA levels in skin derived from Rev-erbα−/− and WT mice treated with D-gal/UVB. (E) mRNA expression of Mmp-1 and p53 in the skin derived from Rev-erbα−/− and WT mice treated with D-gal/UVB. (F) Protein expression of MMP-1/p53 in skin derived from Rev-erbα−/− and WT mice treated with D-gal/UVB. (G) H&E and Masson staining showing epidermal thickness and collagen content in skin derived from Rev-erbα−/− and WT mice treated with D-gal/UVB. In panels A–B, data are mean ± SD (n = 3). In panels C–G, data are mean ± SD (n = 7). *p < 0.05 (t-test).
SBG up-regulates the clock gene Bmal1 in mouse and cell models of aging
Because Bmal1 and its upstream regulator Rev-erbα are involved in skin aging, we wondered whether Bmal1 has a role in protection of skin aging by SBG. We found that SBG dose-dependently increased the BMAL1 mRNA and protein in the skin of D-gal/UVB-treated mice (Figure 8A/B). Likewise, SBG led to increases in the mRNA and protein of BMAL1 in D-gal-treated NIT3H3 cells (Figure 8C/D). Therefore, the protective effects of SBG on skin aging can be attributed to enhanced BMAL1 expression.
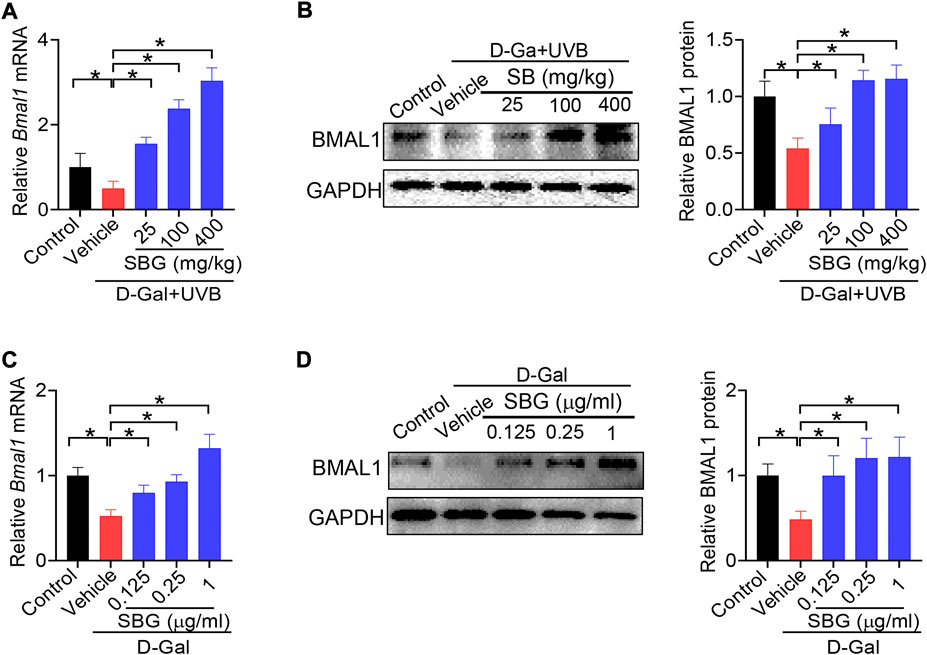
FIGURE 8. SBG up-regulates the clock gene Bmal1 in mouse and cell models of aging. (A) Effects of SBG on Bmal1 mRNA in the skin of D-gal/UVB-treated mice. (B) Effects of SBG on BMAL1 protein in the skin of D-gal/UVB-treated mice. (C) Effects of SBG on Bmal1 mRNA in D-gal-treated NIH3T3 cells. (D) Effects of SBG on BMAL1 protein in D-gal-treated NIH3T3 cells. Data are mean ± SD (n = 7). *p < 0.05 (one-way ANOVA and Bonferroni post hoc test).
SBG antagonizes REV-ERBα to induce BMAL1 expression
Bmal1 expression is directly regulated by REV-ERBα, a nuclear receptor whose activity can be modified by small molecules (Kojetin and Burris, 2014). We next tested whether SBG modulates Bmal1 expression via REV-ERBα. We first assessed the activity of SBG in HEK293 cells expressing a chimeric receptor (i.e., the DNA-binding domain of Gal4 is fused to the LBD of Rev-erbα) and a Gal4-responsive luciferase reporter (Figure 9A). SBG dose-dependently inhibited the repressor activities of REV-ERBα in the Gal4 chimeric assay (Figure 9A), suggesting SBG as a REV-ERBα antagonist. Furthermore, like SR8278, SBG dose-dependently enhanced the promoter activity of Bmal1 (−2000/+100 bp) in a luciferase reporter assay (Figure 9B). Moreover, SBG increased the mRNA and protein expression of BHMT and NLRP3 (i.e., two known targets of REV-ERBα) in addition to BMAL1 in NIH3T3 cells in a dose-dependent fashion (Figure 9C/D). In addition, SBG increased the circadian amplitude of Bmal1 gene according to cell-based circadian assay with NIH3T3 cells expressing Bmal1-dLuc reporter (Figure 9E). Taken together, these findings indicated that SBG antagonized REV-ERBα to induce BMAL1 expression.
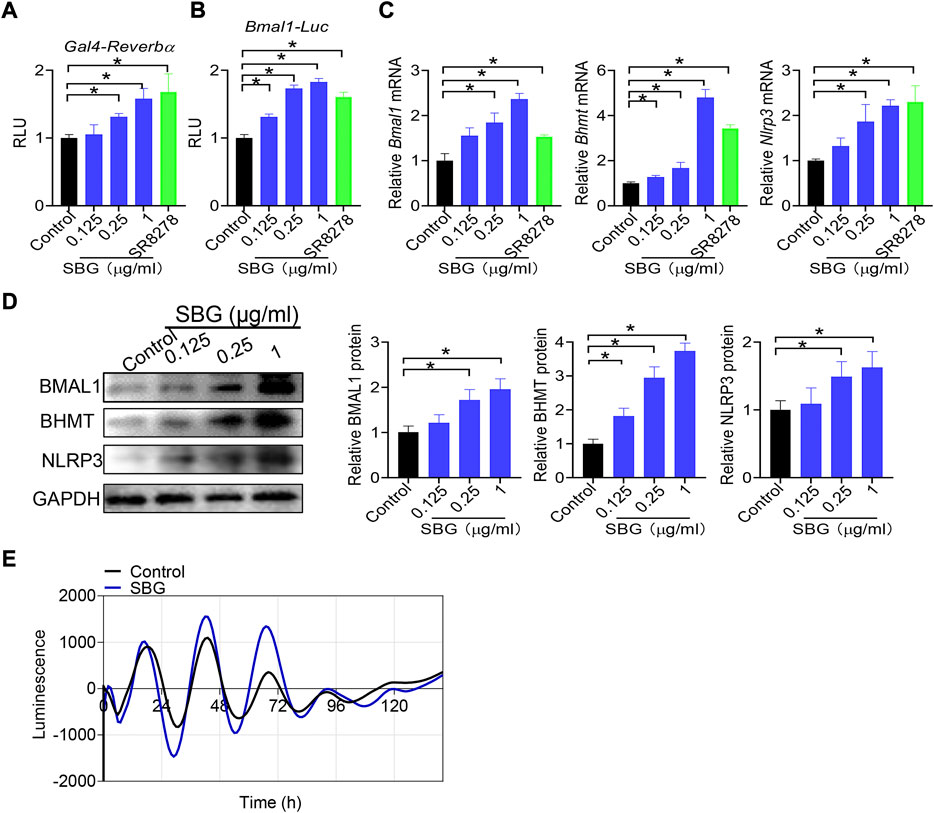
FIGURE 9. SBG antagonizes REV-ERBα to induce BMAL1 expression. (A) Dose-dependent effects of SBG on Gal4 luciferase activity. HEK293 cells were co-transfected with pGal4-Rev-erbα-LBD and pGL4.35-Luc plasmids for 24 h, followed by treatment with SBG or vehicle for 24 h. (B) Effects of SBG on Bmal1-Luc reporter activity. (C) Effects of SBG on the mRNA expression of Bmal1, Bhmt and Nlrp3 in NIH3T3 cells treated with SBG or vehicle. (D) Effects of SBG on the protein expression of BMAL1, BHMT and NLRP3 in NIH3T3 cells treated with SBG or vehicle. (E) Bioluminescent recordings of Bmal1-dLuc-overexpressed NIH3T3 cells after SBG treatment. In panels A–B, data are mean ± SD (n = 6). In panels C–D, data are mean ± SD (n = 3). *p < 0.05 (one-way ANOVA and Bonferroni post hoc test).
Discussion
In this study, we have identified SBG as a novel anti-skin aging agent. Compared with synthetic agents such as retinoid (Mukherjee et al., 2006), SBG is more advantageous in practical applications because it is a herbal medicine without safety concern. More importantly, we have uncovered that SBG protects against skin aging in mice by antagonizing REV-ERBα and increasing skin expression of BMAL1, an aging-inhibiting factor (Figure 10). The evidence for antagonism of REV-ERBα by SBG is strong. First, SBG dose-dependently inhibited the repressor activities of REV-ERBα in the Gal4 chimeric assay. Second, like SR8278 (a known REV-ERBα antagonist), SBG dose-dependently enhanced the promoter activity of Bmal1 in a luciferase reporter assay. Third, SBG increased the expression of BMAL1, BHMT and NLRP3 (three known targets of REV-ERBα and repressed by REV-ERBα) in NIH3T3 cells. Fourth, SBG increased the circadian amplitude of Bmal1 gene according to circadian assays with NIH3T3 cells expressing Bmal1-dLuc reporter (Figure 9). It is noteworthy that herbal extract instead of purified active compounds was used in current study. This will affect reproducibility because different sources of SBG and slight changes in extraction can lead to different composition of active compounds.
Our finding that REV-ERBα/BMAL1 (as core clock components) regulate skin aging supports the notion that skin pathophysiology is under the control of circadian clock. In fact, circadian clock has been implicated in regulation of several other types of skin diseases such as skin cancer, infections and sunburn (Duan et al., 2021). Because among clock components BMAL1 has a direct effect on skin aging, it likely acts to connect this skin disorder to circadian clock. Supporting this, Bmal1 was markedly down-regulated in the skin of aged mice (Figure 8). We have identified REV-ERBα as a potential target for prevention and treatment of skin aging. Compared with other targets such as BMAL1, REV-ERBα is more advantageous because it is a ligand-responsive receptor whose activity can be modified by small molecules. We found that SBG is a novel REV-ERBα antagonist. However, it remains unknown which constituents in this herbal medicine are responsible for the antagonistic action. We reasoned that baicalein (a known active constituent) may have a contribution as it can induce the expression of Bmal1, a direct target of REV-ERBα, and shows a protective effect on skin damage (Nomura et al., 2018).
This study has unraveled that SBG promotes BMAL1 expression to protect against skin aging in mice. BMAL1 functions as an anti-aging factor by up-regulating major antioxidant enzymes such as SOD, peroxiredoxines and glutathione peroxidase (Kondratov et al., 2009; Töbelmann and Dittmar, 2021). It is noteworthy that there is a possibility that other mechanisms are also involved in the protection of skin aging by SBG considering that herbal medicines usually contain hundreds of chemical constituents. The potential mechanisms include inactivation of MAPK/AP-1 and NF-κB signaling pathways, activation of TGF-β/Smad pathway, and modulation of cyclooxygenase (COX), hypoxia-Inducible factor (HIF)-1 and inducible nitric oxide synthase (iNOS) (Domaszewska-Szostek et al., 2021; Chi and Kim, 2005; Gu et al., 2020). However, whether these mechanisms have a contribution to the SBG effect on skin aging awaits further investigations.
Mouse models of skin aging were established in current study by treatment with D-gal/UVB as previously described (Jiayi et al., 2019; Zhang et al., 2020; Cao et al., 2022). Chronic injection of D-gal, a reducing sugar, results in oxidative stress including reductions of antioxidant enzymes, inflammation and apoptosis, mimicking a natural aging process (Shen et al., 2002; Shan et al., 2009; Chen et al., 2018). UVB is the most harmful constituent of UV radiation. Chronic UVB exposure can cause excessive ROS production, abnormal elastin deposition, and impairment of collagen fibers (Starcher et al., 1999; Heck et al., 2003; Lee et al., 2021). D-gal and UVB co-treatment induces a large-scale burst of free radicals, leading to the oxidative damage in the skin and skin aging-like symptoms such as wrinkling, sagging, dryness, and erythema (Zhang et al., 2020). This animal model has been widely used to elucidate cellular and molecular changes that may have a causal role in skin aging, and to screen anti-aging drugs (Jiayi et al., 2019; Zhang et al., 2020; Cao et al., 2022).
Anti-aging effects of SBG were assessed here by measuring SOD activity, MDA/HYP levels, and MMP-1/p53 expression. SOD is a major free radical scavenger in the body, and functions to remove superoxide anions (Li et al., 2020; Park et al., 2008). MDA is a final product of oxidation, and its content can directly reflect the level of lipid peroxidation (Gil et al., 2002; Zhang et al., 2014). SOD activity and MDA level can be used as indicators of the level of organism aging which is associated with oxidative stress and ROS production (Yi et al., 2019). HYP is the most abundant amino acid in collagen, and its level indirectly reflects the total collagen content (Li and Wu, 2018; Li et al., 2016). MMP-1 is a collagenase that plays an important role in degradation of dermal collagen during skin aging (Sapna et al., 2014). Thus, HYP and MMP-1 can be used as markers of skin aging process. P53 is a tumor suppressor gene that induces cell senescence by promoting the expression of growth suppressive genes (Bond et al., 1996; Luo et al., 2004), and is also regarded as a marker of skin aging. In fact, measurements of SOD activity, MDA/HYP levels, and MMP-1/p53 expression have been widely performed to assess aging and to screen anti-aging agents (Jiayi et al., 2019; Park et al., 2008; Hwang et al., 2012).
An important part of the skin response to stress is its ability for melatonin synthesis and subsequent metabolism (Slominski et al., 2017; Slominski et al., 2018b). Melatonin is indispensable for physiological skin functions and has a protective role against photoaging (Skobowiat, et al., 2018; Bocheva et al., 2022). However, whether SBG affects skin melatonin remains unknown. Like vitamin C, vitamin D exerts a variety of antiaging and photoprotective effects on the skin (Bocheva et al., 2021; Slominski et al., 2020). However, whether the anti-skin aging effect of SBG is comparable to that of vitamin D was unaddressed. It is noteworthy that UV radiation not only induces skin pathology, but also exert systemic effects, including activation of hypothalamic-pituitary-adrenal axis, opioidogenic effects, and immunosuppression. Thus, UV radiation has therapeutic applications in management of various diseases such as addiction, autoimmune and mood disorders (Slominski et al., 2018a).
In summary, SBG displays a pharmacological effect on skin aging based on mouse and cell models of aging. Mechanistically, SBG protects against skin aging in mice by antagonizing REV-ERBα and increasing skin expression of BMAL1, an aging-inhibiting factor.
Abbreviation
Bmal1, brain and muscle ARNT-like one; CCGs, clock-controlled genes; Clock, circadian locomotor output cycles kaput; Crys, cryptochromes; D-gal, D-galactose; HYP, hydroxyproline; MDA, malondialdehyde; NR1D1, nuclear receptor subfamily one group D member one; Pers, periods; qPCR, quantitative polymerase chain reaction; ROS, reactive oxygen species; SA-β-gal, senescence-associated-β-galactosidase; SBG, Scutellaria baicalensis Georgi; SOD, superoxide dismutase; UV, ultraviolet
Data availability statement
The original contributions presented in the study are included in the article/Supplementary Material, further inquiries can be directed to the corresponding authors.
Ethics statement
The animal study was reviewed and approved by Institutional Animal Care and Use Committee of Guangzhou University of Chinese Medicine
Author contributions
BW, DD, and GS designed the study; GS, YD, YL, WZ, ZW performed experiments; GS, YD, and YL and XZ collected and analyzed data; BW, DD, GS, and YL wrote the manuscript. All data were generated in-house, and no paper mill was used. All authors agree to be accountable for all aspects of work ensuring integrity and accuracy.
Funding
This work was supported by the National Natural Science Foundation of China (No. 81722049 & 82003839) and Natural Science Foundation of Guangdong Province (No. 2019A1515110892 & 2021A1515012189).
Conflict of interest
The authors declare that the research was conducted in the absence of any commercial or financial relationships that could be construed as a potential conflict of interest.
Publisher’s note
All claims expressed in this article are solely those of the authors and do not necessarily represent those of their affiliated organizations, or those of the publisher, the editors and the reviewers. Any product that may be evaluated in this article, or claim that may be made by its manufacturer, is not guaranteed or endorsed by the publisher.
Supplementary material
The Supplementary Material for this article can be found online at: https://www.frontiersin.org/articles/10.3389/fphar.2022.991917/full#supplementary-material
Abbreviations:
Bmal1, brain and muscle ARNT–like one; CCGs, clock–controlled genes; Clock, circadian locomotor output cycles kaput; Crys, cryptochromes; D–gal, D–galactose; HYP, hydroxyproline; MDA, malondialdehyde; NR1D1, nuclear receptor subfamily one group D member one; Pers, periods; qPCR, quantitative polymerase chain reaction; ROS, reactive oxygen species; SA–β–gal, senescence–associated–β–galactosidase; SBG, Scutellaria baicalensis Georgi; SOD, superoxide dismutase; UV, ultraviolet.
References
Bernhard, D., Moser, C., Backovic, A., and Wick, G. (2007). Cigarette smoke–anaging accelerator? Exp. Gerontol. 42 (3), 160–165. doi:10.1016/j.exger.2006.09.016
Bocheva, G., Slominski, R. M., Janjetovic, Z., Kim, T. K., Böhm, M., Steinbrink, K., et al. (2022). Protective role of melatonin and its metabolites in skin aging. Int. J. Mol. Sci. 23 (3), 1238. doi:10.3390/ijms23031238
Bocheva, G., Slominski, R. M., and Slominski, A. T. (2019). Neuroendocrine aspects of skin aging. Int. J. Mol. Sci. 20 (11), 2798. doi:10.3390/ijms20112798
Bocheva, G., Slominski, R. M., and Slominski, A. T. (2021). The impact of vitamin D on skin aging. Int. J. Mol. Sci. 22 (16), 9097. doi:10.3390/ijms22169097
Böger, R. (2014). [Circadian clocks and energy metabolism]. Dtsch. Med. Wochenschr. 139 (24), 1320. doi:10.1055/s-0034-1370115
Bond, J., Haughton, M., Blaydes, J., Gire, V., Wynford-Thomas, D., and Wyllie, F. (1996). Evidence that transcriptional activation by p53 plays a direct role in the induction of cellular senescence. Oncogene 13 (10), 2097–2104.
Brown, J. P. (1980). A review of the genetic effects of naturally occurring flavonoids, anthraquinones and related compounds. Mutat. Res. 75 (3), 243–277. doi:10.1016/0165-1110(80)90029-9
Bunger, M. K., Wilsbacher, L. D., Moran, S. M., Clendenin, C., Radcliffe, L. A., Hogenesch, J. B., et al. (2000). Mop3 is an essential component of the master circadian pacemaker in mammals. Cell 103 (7), 1009–1017. doi:10.1016/s0092-8674(00)00205-1
Burris, T. P. (2008). Nuclear hormone receptors for heme: REV-ERBalpha and REV-ERBbeta are ligand-regulated components of the mammalian clock. Mol. Endocrinol. 22 (7), 1509–1520. doi:10.1210/me.2007-0519
Cao, C., Xiao, Z., Tong, H., Liu, Y., Wu, Y., and Ge, C. (2022). Oral intake of chicken bone collagen peptides anti-skin aging in mice by regulating collagen degradation and synthesis, inhibiting inflammation and activating lysosomes. Nutrients 14 (8), 1622. doi:10.3390/nu14081622
Chen, M., Zhou, C., Xu, H., Zhang, T., and Wu, B. (2020). Chronopharmacological targeting of Rev-erbα by puerarin alleviates hyperhomocysteinemia in mice. Biomed. Pharmacother. = Biomedecine Pharmacother. 125, 109936. doi:10.1016/j.biopha.2020.109936
Chen, P., Chen, F., and Zhou, B. (2018). Antioxidative, anti-inflammatory and anti-apoptotic effects of ellagic acid in liver and brain of rats treated by D-galactose. Sci. Rep. 8 (1), 1465. doi:10.1038/s41598-018-19732-0
Chen, R., Schirmer, A., Lee, Y., Lee, H., Kumar, V., Yoo, S. H., et al. (2009). Rhythmic PER abundance defines a critical nodal point for negative feedback within the circadian clock mechanism. Mol. Cell 36 (3), 417–430. doi:10.1016/j.molcel.2009.10.012
Chi, Y. S., and Kim, H. P. (2005). Suppression of cyclooxygenase-2 expression of skin fibroblasts by wogonin, a plant flavone from Scutellaria radix. Prostagl. Leukot. Essent. Fat. Acids 72 (1), 59–66. doi:10.1016/j.plefa.2004.04.009
Crumbley, C., and Burris, T. P. (2011). Direct regulation of CLOCK expression by REV-ERB. PloS one 6 (3), e17290. doi:10.1371/journal.pone.0017290
Delezie, J., Dumont, S., Dardente, H., Oudart, H., Gréchez-Cassiau, A., Klosen, P., et al. (2012). The nuclear receptor REV-ERBα is required for the daily balance of carbohydrate and lipid metabolism. FASEB 26 (8), 3321–3335. doi:10.1096/fj.12-208751
Domaszewska-Szostek, A., Puzianowska-Kuźnicka, M., and Kuryłowicz, A. (2021). Flavonoids in skin senescence prevention and treatment. Int. J. Mol. Sci. 22 (13), 6814. doi:10.3390/ijms22136814
du Pré, B. C., Dierickx, P., Crnko, S., Doevendans, P. A., Vos, M. A., Geijsen, N., et al. (2017). Neonatal rat cardiomyocytes as an in vitro model for circadian rhythms in the heart. J. Mol. Cell. Cardiol. 112, 58–63. doi:10.1016/j.yjmcc.2017.08.009
Duan, J., Greenberg, E. N., Karri, S. S., and Andersen, B. (2021). The circadian clock and diseases of the skin. FEBS Lett. 595 (19), 2413–2436. doi:10.1002/1873-3468.14192
Duong, H. A., Robles, M. S., Knutti, D., and Weitz, C. J. (2011). A molecular mechanism for circadian clock negative feedback. Science 332 (6036), 1436–1439. doi:10.1126/science.1196766
Fisher, G. J., Kang, S., Varani, J., Bata-Csorgo, Z., Wan, Y., Datta, S., et al. (2002). Mechanisms of photo aging and chronological skin aging. Arch. Dermatol. 138 (11), 1462–1470. doi:10.1001/archderm.138.11.1462
Frommeyer, T. C., Gilbert, M. M., Brittain, G. V., Wu, T., Nguyen, T. Q., Rohan, C. A., et al. (2022). UVB-induced microvesicle particle release and its effects on the cutaneous microenvironment. Front. Immunol. 13, 880850. doi:10.3389/fimmu.2022.880850
Gabrielska, J., Oszmiański, J., Zyłka, R., and Komorowska, M. (1997). Antioxidant activity of flavones from Scutellaria baicalensis in lecithin liposomes. Z. fur Naturforsch. C 52 (11-12), 817–823. doi:10.1515/znc-1997-11-1215
Gao, B., Li, K., Wei, Y. Y., Zhang, J., Li, J., Zhang, L., et al. (2014). Zinc finger protein 637 protects cells against oxidative stress-induced premature senescence by mTERT-mediated telomerase activity and telomere maintenance. Cell Death Dis. 5 (7), e1334. doi:10.1038/cddis.2014.298
Gekakis, N., Staknis, D., Nguyen, H. B., Davis, F. C., Wilsbacher, L. D., King, D. P., et al. (1998). Role of the CLOCK protein in the mammalian circadian mechanism. Science 280 (5369), 1564–1569. doi:10.1126/science.280.5369.1564
Gil, P., Fariñas, F., Casado, A., and López-Fernández, E. (2002). Malondialdehyde: A possible marker of ageing. Gerontology 48 (4), 209–214. doi:10.1159/000058352
Gu, Y., Han, J., Jiang, C., and Zhang, Y. (2020). Biomarkers, oxidative stress and autophagy in skin aging. Ageing Res. Rev. 59, 101036. doi:10.1016/j.arr.2020.101036
Heck, D. E., Vetrano, A. M., Mariano, T. M., and Laskin, J. D. (2003). UVB light stimulates production of reactive oxygen species: Unexpected role for catalase. J. Biol. Chem. 278 (25), 22432–22436. doi:10.1074/jbc.C300048200
Hemmeryckx, B., Himmelreich, U., Hoylaerts, M. F., and Lijnen, H. R. (2011). Impact of clock gene Bmal1 deficiency on nutritionally induced obesity in mice. Obes. (Silver Spring, Md.) 19 (3), 659–661. doi:10.1038/oby.2010.266
Hirota, T., Lewis, W. G., Liu, A. C., Lee, J. W., Schultz, P. G., and Kay, S. A. (2008). A chemical biology approach reveals period shortening of the mammalian circadian clock by specific inhibition of GSK-3beta. Proc. Natl. Acad. Sci. U. S. A. 105 (52), 20746–20751. doi:10.1073/pnas.0811410106
Hogenesch, J. B., Gu, Y. Z., Jain, S., and Bradfield, C. A. (1998). The basic-helix-loop-helix-PAS orphan MOP3 forms transcriptionally active complexes with circadian and hypoxia factors. Proc. Natl. Acad. Sci. U. S. A. 95 (10), 5474–5479. doi:10.1073/pnas.95.10.5474
Hwang, I. S., Kim, J. E., Choi, S. I., Lee, H. R., Lee, Y. J., Jang, M. J., et al. (2012). UV radiation-induced skin aging in hairless mice is effectively prevented by oral intake of sea buckthorn (Hippophae rhamnoides L.) fruit blend for 6 weeks through MMP suppression and increase of SOD activity. Int. J. Mol. Med. 30 (2), 392–400. doi:10.3892/ijmm.2012.1011
Jiayi, M. A., Yan, G. U. O., Juan, Y. E., and Wenyu, M. A. (2019). Protective effect of lycium ruthenicum procyanidin on mice skin aging model. Chin. General Pract. 22 (15), 1851.
Jung, C. H., Kim, E. M., Park, J. K., Hwang, S. G., Moon, S. K., Kim, W. J., et al. (2013). Bmal1 suppresses cancer cell invasion by blocking the phosphoinositide 3-kinase-Akt-MMP-2 signaling pathway. Oncol. Rep. 29 (6), 2109–2113. doi:10.3892/or.2013.2381
Khapre, R. V., Kondratova, A. A., Susova, O., and Kondratov, R. V. (2011). Circadian clock protein BMAL1 regulates cellular senescence in vivo. Cell cycleGeorget. Tex.) 10 (23), 4162–4169. doi:10.4161/cc.10.23.18381
Kojetin, D. J., and Burris, T. P. (2014). REV-ERB and ROR nuclear receptors as drug targets. Nat. Rev. Drug Discov. 13 (3), 197–216. doi:10.1038/nrd4100
Kondratov, R. V., Kondratova, A. A., Gorbacheva, V. Y., Vykhovanets, O. V., and Antoch, M. P. (2006). Early aging and age-related pathologies in mice deficient in BMAL1, the core componentof the circadian clock. Genes Dev. 20 (14), 1868–1873. doi:10.1101/gad.1432206
Kondratov, R. V., Vykhovanets, O., Kondratova, A. A., and Antoch, M. P. (2009). Antioxidant N-acetyl-L-cysteine ameliorates symptoms of premature aging associated with the deficiency of the circadian protein BMAL1. Aging 1 (12), 979–987. doi:10.18632/aging.100113
Kojetin, D., Wang, Y., Kamenecka, T. M., and Burris, T. P. (2011). Identification of SR8278, asynthetic antagonist of the nuclear hemereceptor REV-ERB. ACS Chem. Biol. 6 (2), 131–136. doi:10.1021/cb1002575
Krutmann, J., Schalka, S., Watson, R., Wei, L., and Morita, A. (2021). Daily photo protection to prevent photo aging. Photodermatol. Photoimmunol. Photomed. 37 (6), 482–489. doi:10.1111/phpp.12688
Kumar, P., Nagarajan, A., and Uchil, P. D. (2018). Analysis of cell viability by the MTT assay. Cold Spring Harb. Protoc. 2018 (6). doi:10.1101/pdb.Prot095505
Langmesser, S., Tallone, T., Bordon, A., Rusconi, S., and Albrecht, U. (2008). Interaction of circadian clock proteins PER2 and CRY with BMAL1 and CLOCK. BMC Mol. Biol. 9, 41. doi:10.1186/1471-2199-9-41
Lee, J. J., Ng, S. C., Ni, Y. T., Liu, J. S., Chen, C. J., Padma, V. V., et al. (2021). Protective effects of galangin against H2O2/UVB-induced dermal fibroblast collagen degradation via hsa-microRNA-4535-mediated TGFβ/Smad signaling. Aging 13 (23), 25342–25364. doi:10.18632/aging.203750
Li, C. W., Wang, Q., Li, J., Hu, M., Shi, S. J., Li, Z. W., et al. (2016). Silver nanoparticles/chitosan oligosaccharide/poly(vinyl alcohol) nanofiber promotes wound healing by activating TGFβ1/Smad signaling pathway. Int. J. Nanomedicine 11, 373–386. doi:10.2147/IJN.S91975
Li, F., Lin, L., He, Y., Sun, G., Dong, D., and Wu, B. (2022). BMAL1 regulates Propionibacterium acnes-induced skin inflammation via REV-ERBα in mice. Int. J. Biol. Sci. 18 (6), 2597–2608. doi:10.7150/ijbs.71719
Li, H. B., Jiang, Y., and Chen, F. (2004). Separation methods used for Scutellaria baicalensis active components. J. Chromatogr. B Anal. Technol. Biomed. Life Sci. 812 (1-2), 277–290. doi:10.1016/j.jchromb.2004.06.045
Li, P., and Wu, G. (2018). Roles of dietary glycine, proline, and hydroxyproline in collagen synthesis and animal growth. Amino acids 50 (1), 29–38. doi:10.1007/s00726-017-2490-6
Li, W., Wang, S., Li, J., Wang, X., Cui, L., Chen, J., et al. (2020). Antioxidative enzyme activities in the Rhodeinae sinensis Gunther and Macrobrachium nipponense and multi-endpoint assessment under tonalide exposure. Ecotoxicol. Environ. Saf. 199, 110751. doi:10.1016/j.ecoenv.2020.110751
Liao, H., Ye, J., Gao, L., and Liu, Y. (2021). The main bioactive compounds of Scutellaria baicalensis Georgi. For alleviation of inflammatory cytokines: A comprehensive review. Biomed. Pharmacother. = Biomedecine Pharmacother. 133, 110917. doi:10.1016/j.biopha.2020.110917
Liu, A. C., Tran, H. G., Zhang, E. E., Priest, A. A., Welsh, D. K., and Kay, S. A. (2008). Redundant function of REV-ERBalpha and beta and non-essential role for Bmal1 cycling in transcriptional regulation of intracellular circadian rhythms. PLoS Genet. 4 (2), e1000023. doi:10.1371/journal.pgen.1000023
Luo, J., Li, M., Tang, Y., Laszkowska, M., Roeder, R. G., and Gu, W. (2004). Acetylation of p53 augments its site-specific DNA binding both in vitro and in vivo. Proc. Natl. Acad. Sci. U. S. A. 101 (8), 2259–2264. doi:10.1073/pnas.0308762101
Mukherjee, S., Date, A., Patravale, V., Korting, H. C., Roeder, A., and Weindl, G. (2006). Retinoids in the treatment of skin aging: An overview of clinical efficacy and safety. Clin. Interv. Aging 1 (4), 327–348. doi:10.2147/ciia.2006.1.4.327
Narayanan, D. L., Saladi, R. N., and Fox, J. L. (2010). Ultraviolet radiation and skin cancer. Int. J. Dermatol. 49 (9), 978–986. doi:10.1111/j.1365-4632.2010.04474.x
Nomura, K., Hirai, T., Nakashima, K. I., and Inoue, M. (2018). “Baicalein regulates FGF21 expression through RORα-mediated transcriptional activity,” in Proceedings for Annual Meeting of The Japanese Pharmacological Society WCP2018 (The 18th World Congress of Basic and Clinical Pharmacology) (Japanese Pharmacological Society), PO2–12.
Pardee, K., Necakov, A. S., and Krause, H. (2011). Nuclear receptors: Small molecule sensors that coordinate growth, metabolism and reproduction. Subcell. Biochem. 52, 123–153. doi:10.1007/978-90-481-9069-0_6
Park, S. H., Cho, D. M., Choi, B. D., Choi, Y. J., and Choi, J. H. (2008). Antioxidative effects of skinned mugwort (Artemisia vulgaris L.) extracts on UV-irradiated hairless mouse skin. J. Korean Soc. Food Sci. Nutr. 37 (1), 20–26. doi:10.3746/jkfn.2008.37.1.20
Sapna, G., Gokul, S., and Bagri-Manjrekar, K. (2014). Matrix metalloproteinases and periodontal diseases. Oral Dis. 20 (6), 538–550. doi:10.1111/odi.12159
Shan, Q., Lu, J., Zheng, Y., Li, J., Zhou, Z., Hu, B., et al. (2009). Purple sweet potato color ameliorates cognition deficits and attenuates oxidative damage and inflammation in aging mouse brain induced by d-galactose. J. Biomed. Biotechnol. 2009, 564737. doi:10.1155/2009/564737
Shen, Y. X., Xu, S. Y., Wei, W., Sun, X. X., Yang, J., Liu, L. H., et al. (2002). Melatonin reduces memory changes and neural oxidative damage in mice treated with D-galactose. J. Pineal Res. 32 (3), 173–178. doi:10.1034/j.1600-079x.2002.1o850.x
Skobowiat, C., Brożyna, A. A., Janjetovic, Z., Jeayeng, S., Oak, A., Kim, T. K., et al. (2018). Melatonin and its derivatives counteract the ultraviolet B radiation-induced damage in human and porcine skin ex vivo. J. Pineal Res. 65 (2), e12501. doi:10.1111/jpi.12501
Slominski, A. T., Chaiprasongsuk, A., Janjetovic, Z., Kim, T. K., Stefan, J., Slominski, R. M., et al. (2020). Photoprotective properties of vitamin D and lumisterol hydroxyderivatives. Cell biochem. Biophys. 78 (2), 165–180. doi:10.1007/s12013-020-00913-6
Slominski, A. T., Hardeland, R., Zmijewski, M. A., Slominski, R. M., Reiter, R. J., and Paus, R. (2018b). Melatonin: A cutaneous perspective on its production, metabolism, and functions. J. Invest. Dermatol. 138 (3), 490–499. doi:10.1016/j.jid.2017.10.025
Slominski, A. T., Zmijewski, M. A., Plonka, P. M., Szaflarski, J. P., and Paus, R. (2018a). How UV light touches the brain and endocrine system through skin, and why. Endocrinology 159 (5), 1992–2007. doi:10.1210/en.2017-03230
Slominski, A. T., Zmijewski, M. A., Semak, I., Kim, T. K., Janjetovic, Z., Slominski, R. M., et al. (2017). Melatonin, mitochondria, and the skin. Cell. Mol. Life Sci. 74 (21), 3913–3925. doi:10.1007/s00018-017-2617-7
Starcher, B., Pierce, R., and Hinek, A. (1999). UVB irradiation stimulates deposition of new elastic fibers by modified epithelial cells surrounding the hair follicles and sebaceous glands in mice. J. Invest. Dermatol. 112 (4), 450–455. doi:10.1046/j.1523-1747.1999.00553.x
Terao, M., Tani, M., Itoi, S., Yoshimura, T., Hamasaki, T., Murota, H., et al. (2014). 11β-hydroxysteroid dehydrogenase 1 specific inhibitor increased dermal collagen content and promotes fibroblast proliferation. PloS one 9 (3), e93051. doi:10.1371/journal.pone.0093051
Töbelmann, D., and Dittmar, M. (2021). Diurnal relationship between core clock gene BMAL1, antioxidant SOD1 and oxidative RNA/DNA damage in young and older healthy women. Exp. Gerontol. 151, 111422. doi:10.1016/j.exger.2021.111422
Vieira, E., Mirizio, G. G., Barin, G. R., de Andrade, R. V., Nimer, N., and La Sala, L. (2020). Clock genes, inflammation and the immune system-implications for diabetes, obesity and neurodegenerative diseases. Int. J. Mol. Sci. 21 (24), 9743. doi:10.3390/ijms21249743
Wang, S., Li, F., Lin, Y., and Wu, B. (2020). Targeting REV-ERBα for therapeutic purposes: Promises and challenges. Theranostics 10 (9), 4168–4182. doi:10.7150/thno.43834
Wang, S., Lin, Y., Yuan, X., Li, F., Guo, L., and Wu, B. (2018). REV-ERBα integrates colon clock with experimental colitis through regulation of NF-κB/NLRP3 axis. Nat. Commun. 9 (1), 4246–4312. doi:10.1038/s41467-018-06568-5
Wang, Y., Kojetin, D., and Burris, T. P. (2015). Anti-proliferative actions of a synthetic REV-ERBα/β agonist in breast cancer cells. Biochem. Pharmacol. 96 (4), 315–322. doi:10.1016/j.bcp.2015.06.010
Watson, M., Holman, D. M., and Maguire-Eisen, M. (2016). Ultraviolet radiation exposure and its impact on skin cancer risk. Semin. Oncol. Nurs. 32 (3), 241–254. doi:10.1016/j.soncn.2016.05.005
Yi, R., Zhang, J., Sun, P., Qian, Y., and Zhao, X. (2019). Protective effects of kuding tea (Ilex kudingcha C. J. Tseng) polyphenols on UVB-induced skin aging in SKH1 hairless mice. Mol. (Basel, Switz. 24 (6), 1016. doi:10.3390/molecules24061016
Yin, L., and Lazar, M. A. (2005). The orphan nuclear receptor Rev-erbalpha recruits the N-CoR/histone deacetylase 3 corepressor to regulate the circadian Bmal1 gene. Mol. Endocrinol. 19 (6), 1452–1459. doi:10.1210/me.2005-0057
Yin, L., Wu, N., and Lazar, M. A. (2010). Nuclear receptor rev-erbalpha: A heme receptor that coordinates circadian rhythm and metabolism. Nucl. Recept. Signal. 8, e001. doi:10.1621/nrs.08001
Zhang, S., Dong, Z., Peng, Z., and Lu, F. (2014). Anti-aging effect of adipose-derived stem cells in a mouse model of skin aging induced by D-galactose. PloS one 9 (5), e97573. doi:10.1371/journal.pone.0097573
Zhang, T., Zhao, M., Lu, D., Wang, S., Yu, F., Guo, L., et al. (2018). REV-ERBα regulates CYP7A1 through repression of liver receptor homolog-1. Drug Metab. Dispos. 46 (3), 248–258. doi:10.1124/dmd.117.078105
Zhang, Z., Zhu, H., Zheng, Y., Zhang, L., Wang, X., Luo, Z., et al. (2020). The effects and mechanism of collagen peptide and elastin peptide on skin aging induced by D-galactose combined with ultraviolet radiation. J. Photochem. Photobiol. B 210, 111964. doi:10.1016/j.jphotobiol.2020.111964
Keywords: scutellaria baicalensis georgi, skin aging, REV-erbα, BMAL1, photoaging
Citation: Sun G, Dang Y, Lin Y, Zeng W, Wu Z, Zhang X, Dong D and Wu B (2022) Scutellaria baicalensis Georgi regulates REV-ERBα/BMAL1 to protect against skin aging in mice. Front. Pharmacol. 13:991917. doi: 10.3389/fphar.2022.991917
Received: 12 July 2022; Accepted: 12 September 2022;
Published: 30 September 2022.
Edited by:
Ahmad Nazrun Shuid, MARA University of Technology, MalaysiaReviewed by:
Andrzej T Slominski, University of Alabama at Birmingham, United StatesSiti Norsyafika Kamarudin, Universiti Teknologi MARA, Malaysia
Copyright © 2022 Sun, Dang, Lin, Zeng, Wu, Zhang, Dong and Wu. This is an open-access article distributed under the terms of the Creative Commons Attribution License (CC BY). The use, distribution or reproduction in other forums is permitted, provided the original author(s) and the copyright owner(s) are credited and that the original publication in this journal is cited, in accordance with accepted academic practice. No use, distribution or reproduction is permitted which does not comply with these terms.
*Correspondence: Dong Dong, ZG9uZ2RvbmcxOTgzamRAMTYzLmNvbQ==; Baojian Wu, Ymoud3VAaG90bWFpbC5jb20=
†These authors have contributed equally to this work