- 1Key Laboratory of Economic Plants and Biotechnology, Kunming Institute of Botany, Chinese Academy of Sciences, Kunming, Yunnan, China
- 2Department of Biology, Faculty of Science, Chiang Mai University, Chiang Mai, Thailand
- 3College of Forestry and Vocational Technology in Yunnan, Kunming, Yunnan, China
- 4Lijiang Yunxin Green Biological Development Co., Ltd., Lijiang, Yunnan, China
Background: Cancer is one of the top two leading causes of death worldwide. Ethnobotanical research, it is one of methods, which is able to discover effective anticancer drugs based on “prototype” of indigenous people’s historical experiences and practices. The rhizomes of Paris polyphylla var. yunnanensis (Franch.) Hand.-Mazz. have been used as botanical drugs to treat cancer by Yi, Bai, Dai, and Naxi ethnic groups in Yunnan, China, where this species is widely cultivated in a large scale in Yunnan.
Materials and methods: To identify the substances of anticancer activities based on indigenous medicine knowledge, chromatography was performed to separate saponins from the rhizomes of P. polyphylla var. yunnanensis, followed by spectroscopy to determine the structure of six isolated saponins. The cytotoxicity of five extracts and six pure compounds were evaluated by MTS method. Quantitative determination of total saponins of P. polyphylla var. yunnanensis was analyzed by HPLC. Cell cycle assay, apoptosis assay, and mitochondrial membrane potential were used to evaluate the pro-apoptotic activity in vitro.
Results: Five extracts and six pure saponins showed significant inhibitory cytotoxic activities of three human liver cancer cell lines (SMMC-7721, HepG2, and SK-HEP-1) and one non-small-cell lung cancer cell line (A549). The contents of Paris saponins I, II, and VII were 6.96% in the rhizomes of P. polyphylla var. yunnanensis, much higher than Chinese Pharmacopoeia standards (0.6%). Six saponins induced significant apoptosis and cell cycle arrest in three human cancer cell lines (A549, SMMC-7721, and HepG2), which was associated with the loss of mitochondrial membrane potential.
Conclusion: The result of this study support that cultivated P. polyphylla var. yunnanensis could be a substitute for wild resource as an anticancer medicine based on indigenous medicine knowledge.
1 Introduction
Lung cancer remains the leading cause of total cancer death, with an estimated 18% mortality, followed by colorectal (9.4%) and liver (8.3%) cancers according to global cancer statistics for 2020 (Sung et al., 2021). Hepatocellular carcinoma (HCC) is a major type of primary liver cancer and is the third leading cause of cancer-related deaths worldwide (Yang and Roberts, 2010; Liu et al., 2013), while lung cancer has the second highest morbidity rate (11.4%) next only to female breast cancer (11.7%). Lung cancer can be divided into small-cell lung cancer and non-small-cell lung cancer (NSCLC) based on cell type, the latter being responsible for over 80% of all lung cancers (Ferlay et al., 2015; Sui et al., 2016). Although cancer treatment and prognosis vary with the type of cancer, the most widely available treatments for the above two cancer types are surgery, chemotherapy, and radiotherapy. However, these treatments and their clinical effects do not always lead to a positive outcome (Yang et al., 2012). In recent years, cancer research has embraced complementary approaches such as targeted therapy inspired by indigenous medicine knowledge (Liu et al., 2021; Bi et al., 2022; Liu et al., 2022) as well as anticancer treatments by immunotherapy (Liu et al., 2018). Novel anticancer drugs with high effects, low toxicity, and low cost are urgently needed to treat these two cancers. One potential source of untapped knowledge is folk remedies, such as those described in TCM, although the effects of these traditional ethnic treatments and the identity of the underlying bioactive substance remain to be established and fully explored.
The genus Paris of the family Melanthiaceae encompasses 26 species based on current taxonomy, also known as “Chong-Lou” in Chinese (Li, 1998; Wang et al., 2015; Wang et al., 2017; Wang et al., 2018; Pei et al., 2020; Ji, 2021) and “余猫瀑 (yuq ma pvl)” in the Naxi language. The dried rhizome of Paris polyphylla var. yunnanensis (Franch.) Hand.-Mazz. and Paris polyphylla var. chinensis (Franch.) Hara are widely used in TCM and are recorded in the Chinese Pharmacopoeia (Wang et al., 2015; National Pharmacopoeia Committee, 2020; Yan et al., 2021). In fact, the dried rhizomes of these plants (Rhizoma Paridis) are an indispensable ingredient of seven preparations recorded in the Chinese Pharmacopoeia (2020). One of these seven preparations is “Qizhen capsule” (芪珍胶囊), which has traditionally served as an adjuvant therapy for lung, breast, and gastric cancer (Huang et al., 2011; Qin et al., 2018; National Pharmacopoeia Committee, 2020). P. polyphylla var. yunnanensis is widely used as a botanical drugs by eight ethnic minorities in China, constituting one ingredient from 62 recipes effective for the main treatment of multiple human diseases and afflictions, such as tumors, skin injury, poisoning, virus infections, diseases, and ailments affecting connective tissues and the respiratory, digestive, urogenital, and musculoskeletal systems. More specifically, four ethnic groups—the Yi, Bai, Dai and Naxi people—have used P. polyphylla var. yunnanensis to treat cancer (Yan et al., 2021). While TCM has been tested in human trials, the underlying pharmacological mechanism has not been clearly established, thereby limiting its potential benefits for human health.
A survey of the chemical structures of the few traditional compounds that have been isolated and identified has revealed that the main active components are often steroidal saponins (Huang and Zhou, 1962; Chen and Zhou, 1987; Kang et al., 2012; Wu et al., 2012; Qin et al., 2013; Wu et al., 2013; Wei et al., 2014; Qin et al., 2016; Ding et al., 2021), which are able to fight off cancer, furuncles, carbuncles, abscesses, bacterial infections, and inflammation, as well as stop bleeding (Yan et al., 2021). Indeed, metabolite studies in the Paris genus have led to the isolation and identification of 323 compounds, consisting of steroidal saponins (168), triterpenes (33), flavonoids (30), cholestanols (8), C-21 steroids (11), phytosterols (12), phytoecdysones (11), and other secondary metabolites (50). A total of 177 compounds have been isolated in P. polyphylla var. yunnanensis, of which 112 were steroidal saponins (Wei et al., 2014; Zhou et al., 2022). Plant extracts from the Paris genus exhibit anti-tumor, hemostasis, analgesic, anti-inflammatory, and antibacterial effects (Deng et al., 2008; Qin et al., 2012; Qin et al., 2016; Zhang et al., 2016; Ding et al., 2021; Yan et al., 2021). For instance, the anticancer activity of total steroidal saponins from the rhizomes and aboveground parts of P. polyphylla var. yunnanensis is demonstrated against five human cancer cell lines (SW-480, SMMC-7721, HL-60, MCF-7, and A-549) (Qin et al., 2018). However, the identity of the active compounds in the planting resource of this species has not been established according to promising ethnobotanical results. Moreover, the diversity in saponin chemical structures (Ding et al., 2021; Yan et al., 2021) makes their identification challenging. Here, extracted, isolated, purified, and identified individual compounds and established their anti-proliferation potential against several human cancer cell lines, suggesting new promising metabolites to fight cancer.
This study is mainly intended to validate the ethnopharmacological use of P. polyphylla var. yunnanensis, by four ethnic groups in China. An interdisciplinary approach was used to identify and quantify the active steroidal saponins from ethanol extract. The ethanol extracts were further separated to obtain pure compounds, which were identified by nuclear magnetic resonance and electrospray ionization–mass spectrometry. Anticancer properties of five crude extracts and six pure saponins were evaluated by in vitro cell viability assays against three human liver cancer cell lines and one non-small-cell lung cancer cell line. Furthermore, the effects of six pure saponins on apoptosis and cell cycle arrest on cancer cell lines were investigated, some of which were sensitive to the saponins.
2 Materials and methods
2.1 Traditional anticancer records
Four ethnic groups—the Yi, Bai, Dai, and Naxi people—have used P. polyphylla var. yunnanensis to treat cancer (Yan et al., 2021). The Yi ethnic group mix about 10 g (fresh weight) the rhizomes of P. polyphylla var. yunnanensis and Rohdea chinensis (Baker) N. Tanaka and make it into a decoction or use 3 g (dry weight) of the same mixture for the treatment of gynecological tumors. The Bai ethnic group employs 15 g each of a powder from P. polyphylla var. yunnanensis, Viola philippica Cav., Hedyotis diffusa Willd., and Scutellaria barbata D. Don and make it into a decoction for use once a day for 1–2 weeks to treat stomach and esophageal cancers. The Naxi ethnic group uses powder from P. polyphylla var. yunnanensis (20 g), H. diffusa Willd. (50 g), S. barbata D. Don (50 g), and Engleromyces goetzi P. Henn. (20 g) to prepare a decoction, which is consumed every day over 1–2 months for cancer treatment; the types of cancer were not specified. For the Dai ethnic group, there was a record treatment for cancer, but the prescription and type of cancer are unknown (Yan et al., 2021).
There are five types of cancer treatments using P. polyphylla var. yunnanensis including brain neoplasms and thyroid, laryngeal, gastric, and lung cancer, which have been recorded in the book of Yunnan Kang Ai Zhong Cao Yao (Hu and Xuan, 1982). There are 10 kinds of traditional Chinese medicine recipes for lung cancer, each using 30 g of powder as the basis for a decoction, comprising P. polyphylla var. yunnanensis, C. lacryma-jobi, S. scandens, H. cordata, Wolfiporia cocos (F. A. Wolf) Ryvarden & Gilb., Polyporus umbellatus, M. tenacissima, Asparagus densiflorus (Kunth) Jessop, Platycodon grandiflorus (Jacq.) A. DC., and Sageretia thea (Osbeck) Johnst. in various amounts. These anticancer prescriptions that include P. polyphylla var. yunnanensis, but the pharmacological and chemical properties of this species that could be effective for treating anticancer are unclear.
2.2 General experimental procedure
Deionized water was used throughout the study. Ethanol was analytical grade. 1H and 13C NMR spectra were collected on an Avance III 600 spectrometer (Bruker, Switzerland) with tetramethylsilane (TMS) as an internal standard in pyridine-d5 (Cambridge Isotope Laboratories, Inc.). An ultra-high-performance liquid chromatograph connected to a triple quadrupole tandem mass spectrometer (UPLC-Xevo TQ MS, Waters, United States) was used to obtain electrospray ionization–mass spectrometry (ESI-MS) data. Silica gel (200–300 mesh, Qingdao Haiyang Chemical Co., Ltd., Qingdao, China), a macroporous resin column (D101, Jiangsu Donghong Chemical Co., Ltd., China), and thin-layer chromatography (TLC) plates (Qingdao Haiyang Chemical Co., Ltd., China) were used to monitor the compound. The TLC spots were visualized under ultraviolet (UV) light at 254 nm and stained by spraying the TLC plates with 5% (v/v) H2SO4 in alcohol followed by heating. Semi-preparative high-performance liquid chromatography (HPLC) analysis was performed on an Agilent 1260 series system (Agilent Technologies, United States) equipped with a diode array detector, and preparative HPLC was conducted with a Hanbon series system (Jiangsu Hanbon Science & Technology Co., Ltd., China) equipped with a UV detector, using two types of C18 columns produced by Agilent (Zorbax SB-C18 column, 5 μm; ϕ 9.4 × 250 mm and ϕ 4.6 × 250 mm).
2.3 Plant materials
As an identification of Good Agriculture Practice (GAP) and geographical indication products, the rhizomes of P. polyphylla var. yunnanensis which is native and local distribution (called “Daodi” medicine in Chinese) were obtained from Lijiang Yunxin Green Biological Development Co., Ltd., in NW Yunnan Province, China, where located in Three Parallel Rivers and one of hotpot on biodiversity conservation in the world, in May 2020 and identified based on morphological features by Professor Heng Li (CAS Key Laboratory for Plant Diversity and Biogeography of East Asia, Kunming Institute of Botany, Chinese Academy of Sciences, China).
2.4 Extraction and isolation
The dried rhizomes of P. polyphylla var. yunnanensis (2.1 kg) were crushed into powder with a pulverizer and extracted in 80% (v/v) ethanol (3 × 6 L) with an ultrasonicator (40 KHZ) at 60°C for 2 h. The ethanol extract was filtered by filter paper, and the resulting filtrate was concentrated as crude extract (A, 400 g). A 300-g aliquot of the crude extract was separated on a macroporous resin column with four concentrations of ethanol to obtain an aqueous fraction (A1, 170 g), a 50% ethanol fraction (A2, 23 g), a 80% ethanol fraction (A3, 5 g), and a 95% ethanol fraction (A4, 4 g) (Supplementary Figure S7, in the Supplementary Material S2).
Fraction A3 (2.1 g) was isolated by column chromatography (silica gel, 200–300 mesh, CH2Cl2/CH3OH, 19:1→0:1, v/v) as fractions A3–1 (186.3 mg), A3–2 (498.6 mg), A3–3 (300.1 mg), and A3–4 (103.2 mg). An aliquot from fraction A3–2 (109 mg) was separated by semi-preparative HPLC (Zorbax SB-C18 column, 9.4 × 250 mm; CH3CN/0.1% [v/v] H3PO4 solution, 1 mL/min, 30°C, CH3CN, 0–15 min, 0%–20%; 15–20 min, 20%–35%; 20–25 min, 35%–55%; 25–35 min, 55%–60%; 35–40 min, 60%–75%; 40–45 min, 70%–100%; 45–55 min, 100%–100%) to obtain four saponins: Paris saponin Pb (1, 12.9 mg, pennogenin-3-O-α-L-rhamnopyranosyl- (1→2)-[α-L-rhamnopyranosyl-(1→4)]-β-D-glucopyranoside, tR = 35.05 min), Paris saponin H (2, 11.1 mg, pennogenin-3-O-α-L-arabinofuranosyl-(1→4)-[α-L-rhamnopyranosyl-(1→2)]-β-D-glycopyranoside, tR = 35.76 min), Paris saponin III (3, 23.3 mg, diosgenin-3-O-α-L-rhamnopyranosyl-(1→2)-[α-L-rhamnopyranosyl-(1→ 4)]-β-D-glucopyranoside, tR = 42.68 min), and Paris saponin I (4, 26.0 mg, diosgenin-3-O-α-L-arabinofuranosyl-(1→4)-[α-L-rhamnopyranosyl-(1→2)]-β-D-glucopyranoside, tR = 44.24 min). An aliquot of fraction A3–3 (40.1 mg) was similarly separated by preparative HPLC (Zorbax SB-C18 column, 9.4 × 250 mm; CH3CN/H2O, 3 mL/min, 30°C, CH3CN, 0–20 min, 30%–60%; 20–25 min, 60%–30%) to obtain two saponins: Paris saponin VII (5, 15.2 mg, pennogenin 3-O-α-L-rhamnopyranosyl-(1→2)-[α-L-rhamnopyranosyl-(1→4)-α-L-rhamnopyranosyl-(1→4)]-β-D-glucopyranoside, tR = 18.26 min) and Paris saponin II (6, 7.6 mg, diosgenin-3-O-α-L-rhamnopyranosyl-(1→2)-[α-L-rhamnopyranosyl-(1→4)-α-L-rhamnopyranosyl-(1→4)]-β-D-glucopyranoside, tR = 22.01 min) (Supplementary Figure S8, in the Supplementary Material S2).
2.5 Analysis and determination of total saponin content
The contents of total saponins in dry rhizomes were determined by the method described in the Chinese Pharmacopoeia (National Pharmacopoeia Committee, 2020). The dry rhizomes were crushed into powder and passed through a 50 mesh sieve (0.28 mm). The powder (498.8 mg) was placed in a conical flask with a cover, to which 25 mL ethanol was added before the weight of the system was measured. The system was refluxed for 30 min and cooled to room temperature. The weight of the entire system was determined again and brought to its original weight with ethanol. Five extracts (A, A1, A2, A3, and A4) in the analysis and determination of total saponin were prepared at concentrations of 9.45, 4.45, 11.50, 10.90, and 12.75 mg/mL in methanol, respectively. The liquid was filtered through a 0.45-μm membrane before use for HPLC analysis.
Samples were analyzed by HPLC (Agilent 1260 series system, Zorbax SB-C18 column, 5 μm, ϕ 4.6 × 250 mm), with a mobile phase consisting of CH3CN/H2O, with a flow rate of 1 mL/min, at 30°C, starting with CH3CN, 0–20 min, followed by 30%–60% CH3CN (in water, v/v); 20–25 min, 60%–30%. The injection volumes were 10 μL for the dry rhizome powder and 5 μL for each of the six extracts. Eight standard saponins (Paris saponins I, II, VII, III, V, VI, Pb, and H) were purchased from Sichuan Weikeqi Biological Technology Co., Ltd. (purity ≥98%).
2.6 Human cell lines and culture
Three human liver cancer cell lines (SMMC-7721, HepG2, and SK-HEP-1) and the non-small-cell lung cancer (NSCLC) cell line A549 were obtained from ATCC (Manassas, VA, United States). Normal human cell lines (liver cell line LO2 and lung cell line BEAS-2B) were obtained from ATCC (Manassas, VA, United States). Cells were cultured in RMPI-1640 or DMEM medium (Biological Industries, Kibbutz Beit-Haemek, Israel) supplemented with 10% (v/v) fetal bovine serum (Biological Industries) at 37°C in a humidified atmosphere with 5% (v/v) CO2.
2.7 Cell viability assays
The effects of the extracts and isolated saponins on cell growth were evaluated by the MTS assay (3-(4,5-dimethylthiazol-2-yl)-5(3-carboxymethoxyphenyl)-2-(4-sulfopheny)-2H-tetrazolium; Promega, Madison, WI, United States) on human tumor cell lines (Mosmann, 1983; Cory et al., 1991; Malich et al., 1997). In a test experiment of cell inhibition, the cell inhibition effects of six crude extracts (100 μg/mL) and six isolated saponins (40 μM) were roughly measured to ascertain whether to measure the IC50 value by MTS assay.
Each well of a 96-well plate (Multiskan Fc, Therm) was seeded with 100 μL cells at a density of 3,000–5,000 cells per well, with the cell suspension containing 10% (v/v) fetal bovine serum (DMEM or RMPI1640, Biological Industries, Kibbutz Beit-Haemek, Israel). After 12–24 h of inoculation and culture at 37°C, the extracts or saponins were added at the indicated concentrations after being dissolved in dimethyl sulfoxide (DMSO, Biological Industries, Kibbutz Beit-Haemek, Israel) in a final volume of 200 μL per well, each in triplicate. The six crude extracts were added at concentrations of 100, 20, 4, 0.8, and 0.16 μg/mL; the six isolated saponins were added at concentrations of 10, 5, 2.5, 1.25, 0.625, and 0.3125 μM. After culture for 48 h at 37°C, 20 μL of MTS solution and 100 μL of DMEM were added to each well; three blank wells were set aside with 20 μL MTS solution and 100 μL DMEM. After an incubation for another 2–4 h, the absorbance at 492 nm was measured. Cisplatin and Paclitaxel (MeilunBio) were used as a positive control in each experiment. The cell growth curve was drawn with the concentration as the abscissa and the cell survival rate as the ordinate. The IC50 value of each saponin was calculated by the two-point method (Reed and Muench, 1938).
2.8 Cell cycle analysis
Cell cycle distribution was determined by propidium iodide (PI, BD, Pharmingen) staining (Kong et al., 2016; Wei et al., 2021). Briefly, three cancer cell lines were cultured in six-well plates at a density of 3 × 105 cells per well for 24 h before treatment with each of the six isolated saponins at the indicated concentrations for 24 h. The cells were collected, centrifuged, and washed twice with ice-cold PBS. Cells were fixed in 70% (v/v) ice-cold ethanol at 4°C overnight and washed again in PBS twice. The cells were incubated with 100 μL RNase A at 37°C for 30 min in the dark, after which 400 μL PI was added to the cells with RNase A in the dark at 4°C for 15 min. The cell cycle stage was analyzed by flow cytometry (BD, FACSCelesta, United States) with FLOWJO software (FlowJo, Ashland, OR, United States).
2.9 Determination of mitochondrial membrane potential
The probe 5,6-dichloro-1,1′,3,3′- tetraethyl-imidacarbocyanine iodide (JC-10) was used as a measure of mitochondrial membrane potential (Hirose et al., 1974; Chaudhari et al., 2008). The effects of each of the six isolated saponins on mitochondrial membrane potential were determined using a JC-10 assay kit (×200, 10 μL/tube, five tubes), which was purchased from Beijing Solarbio Science & Technology Co., Ltd. (Beijing, China). Cells were cultured in a confocal dish with this method. After 12–24 h of inoculation and culture at 37°C, the purified saponins were added at the indicated concentrations. The cells were incubated at 37°C in a humidified atmosphere with 5% (v/v) CO2 for 24 h. The culture medium was removed, and the confocal dishes were washed twice with 1 mL PBS, followed by 0.5 mL incubation buffer containing 2.5 μL JC-10 (×200) at 37°C for 20 min in the dark. After incubation, the supernatant was removed, and the cells were washed twice with 1 mL JC-10 buffer and PBS. PBS (1 mL) was added to each well of the confocal dish, and the stained cells were observed with a confocal laser scanning microscope (TCS SP8X, Leica, Germany) using a ×40 objective. The wavelengths for excitation were 490 nm (green fluorescence) and 525 nm (red fluorescence). The wavelengths for emission were in the range of 495–565 nm (monomers for JC-10) and 550–620 nm (polymer for JC-10).
2.10 Apoptosis determination by annexin V-propidium iodide staining
Double staining with annexin V-fluorescein isothiocyanate (FITC) and PI was used to determine the effects of the six saponins on cell apoptosis by flow cytometry (Homburg et al., 1995; Vermes et al., 1995; Casciola-Rosen et al., 1996). The effects of the six isolated saponins on apoptosis were determined using an annexin V-FITC apoptosis kit (BD, Pharmingen); the chemotherapy drug doxorubicin (DOX, Beyotime Institute of Biotechnology, Shanghai, China) was used as the positive control. Cells were cultured in six-well plates at a density of 3 × 105 cells per well for 24 h before being treated with each of the six isolated saponins at the indicated concentrations for 24 h. The cells were collected, centrifuged, and washed twice with ice-cold phosphate-buffered saline (PBS). The cells were stained with annexin V-FITC and PI for 15 min in the dark at room temperature before analysis by flow cytometry (BD, FACSCelesta, America). Annexin V-FITC+ and PI+ (Q2) were considered late-stage apoptotic cells, while annexin V-FITC+ and PI− (Q3) cells were considered early-stage apoptotic cells. Annexin V-FITC– and PI+ (Q1) were necrotic or mechanically damaged cells, and annexin V-FITC– and PI− (Q4) cells were healthy living cells.
3 Results and discussion
3.1 Isolation and identification of substances based on indigenous medicine knowledge
The saponins 1–6 (Figure 1) were isolated from the dried rhizomes of P. polyphylla var. yunnanensis and were identified to be Paris saponin Pb (1, pennogenin-3-O-α-L-rhamnopyranosyl-(1→2)-[α-L-rhamnopyranosyl-(1→4)]-β-D-glucopyranoside) (Nakano et al., 1989; Hua et al., 2015), Paris saponin H (2, pennogenin-3-O-α-L-arabinofuranosyl-(1→4)-[α-L-rhamnopyranosyl-(1→2)]-β-D-glycopyranoside) (Chen and Zhou, 1987; Wang et al., 2007; Pang et al., 2015), Paris saponin III (3, diosgenin-3-O-α-L-rhamnopyranosyl-(1→2)-[α-L-rhamnopyranosyl- (1→4)]-β-D-glucopyranoside) (Han et al., 1999; Wang et al., 2001; Wang et al., 2007), Paris saponin I (4, diosgenin-3-O-α-L-arabinofuranosyl-(1→4)-[α-L-rhamnopyranosyl-(1→2)]-β-D-glucopyranoside) (Chen and Zhou, 1987; Huang et al., 2009; Jing et al., 2017), Paris saponin VII (5, pennogenin 3-O-α-L-rhamnopyranosyl-(1→2)-[α-L-rhamnopyranosyl-(1→4)-α-L-rhamnopyranosyl-(1→4)]-β-D-glucopyranoside) (Chen et al., 1983; Zhang et al., 2014), and Paris saponin II (6, diosgenin-3-O-α-L-rhamnopyranosyl-(1→2)-[α-L-rhamnopyranosyl-(1→4)-α-L-rhamnopyranosyl-(1→4)]-β-D-glucopyranoside) (Munday et al., 1993; Jing et al., 2017) by their nuclear magnetic resonance (NMR) and mass spectrometry (MS) data, which were provided in the Supplementary Material. These values were all consistent with the known saponins in the reported literature.
3.2 High-performance liquid chromatography analysis of total saponin contents
The Chinese Pharmacopoeia details a method for the determination of total saponin contents in P. polyphylla extracts (National Pharmacopoeia Committee, 2020), whose levels in dry TCM powder should reach at least 0.6% (National Pharmacopoeia Committee, 2020) to be effective and should include Paris saponin I (C44H70O16), Paris saponin II (C51H82O20), and Paris saponin VII (C51H82O21). Accordingly, total saponin contents in the powder of dry rhizomes and six ethanol or aqueous extracts were measured using eight saponins as standards (Table 1). Six saponins (Paris saponins I, II, VII, III, H, and Pb) were detected in dry rhizome powder; importantly, the contents for Paris saponins I, II, and VII were much higher (6.96%) than the contents set by the Chinese Pharmacopoeia. Paris saponins I, II, and VII and total saponins reached their highest contents in fraction A3, which was obtained by elution from the macroporous resin column with 80% ethanol. These two sets of saponins comprised 37.06% (Paris saponins I, II, and VII) and 59.38% (total saponins) of this fraction. Therefore, fraction A3 was subjected to further separation for the purification of the six detected saponins.
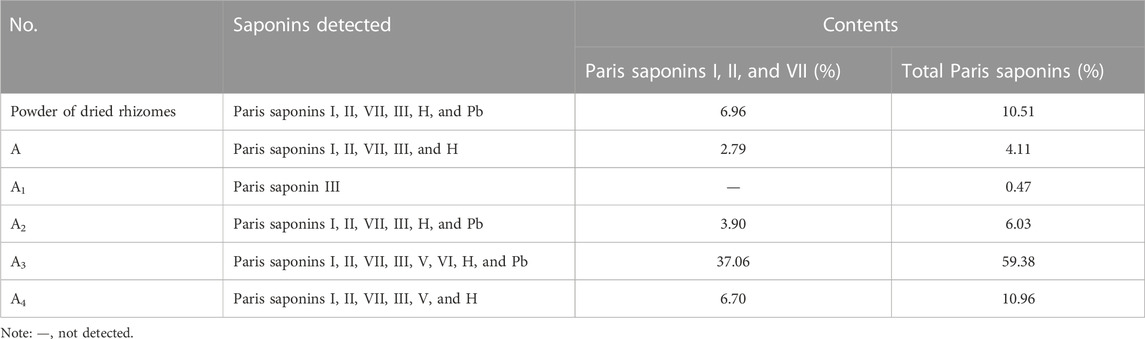
TABLE 1. Analysis and determination of total saponin contents in dried rhizome powder and six ethanol extracts.
Steroidal saponins accumulate to different levels in Paris plants each year and as a function of their natural habitat (Wang et al., 2015). In addition, distinct Paris species present different steroidal saponin profiles (Wei et al., 2014); for example, 112 steroidal saponins were detected in P. polyphylla var. yunnanensis (Zhou et al., 2022). The saponin contents of the P. polyphylla var. yunnanensis rhizomes tested here were much higher than those set by the standards of the Chinese Pharmacopoeia in 2020, which used plants grown in Lijiang, Yunnan province, China. The samples analyzed here mainly contained the eight Paris saponins I, II, VII, III, V, VI, H, and Pb (Table 1).
3.3 Evaluation of cytotoxicity activity
The growth inhibitory activity of all extracts and saponins were obtained from the dried P. polyphylla var. yunnanensis rhizomes. A preliminary experiment indicated that five crude extracts (A, A1, A2, A3, and A4) inhibit cell division when applied at 100 μg/mL in the pre-experiment. To facilitate comparisons, the IC50 values of five extracts (A, A1, A2, A3, and A4) were determined in the three human liver cancer cell lines SMMC-7721, HepG2, and SK-HEP-1 as well as in the NSCLC cell line A549 by the MTS method (Table 2). Among all crude extracts, A3 was the most active against all four human cancer cell lines tested, with IC50 values of 1.226 μg/mL (SMMC-7721), 1.878 μg/mL (HepG2), 0.878 μg/mL (SK-HEP-1), and 1.287 μg/mL (A549).
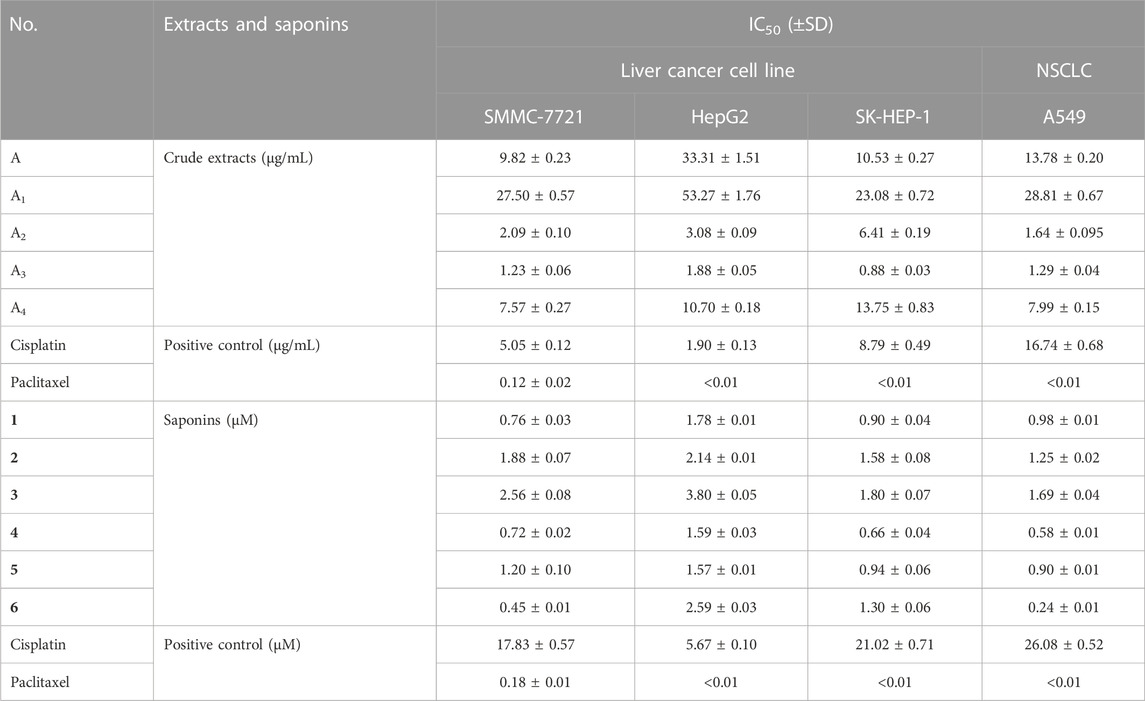
TABLE 2. IC50 values for crude extracts and saponins (1–6) from P. polyphylla var. yunnanensis against four human cancer cell lines.
Six main saponins (1–6) were obtained from the further isolated and identified in the A3 fraction (Figure 1), and their IC50 values for growth inhibition were determined using the same four human cancer cell lines. Importantly, all IC50 values were lower than those obtained with the positive control cisplatin, a commonly used chemotherapy drug (Table 2). In addition, the normal liver and lung cells (LO2 and BEAS-2B) had also been evaluated for six saponins (Table 3). However, none of the purified saponins exhibited the same level of activity as the other chemotherapy drug paclitaxel. Moreover, each saponin showed a distinct activity when tested against the four cancer cell lines, with no single saponin being most effective against all cell lines. More specifically, Paris saponin II (6) was most active against NSCLC, as evidenced by the low IC50 values. Likewise, Paris saponins I (4) and Pb (1) were most active against the three liver cancer cell lines. A previous review mentioned that toxicity evaluation suggested that Rhizome Paridis had slight liver toxicity (Ding et al., 2021). However, the present data for normal cells supported this view (Table 3).
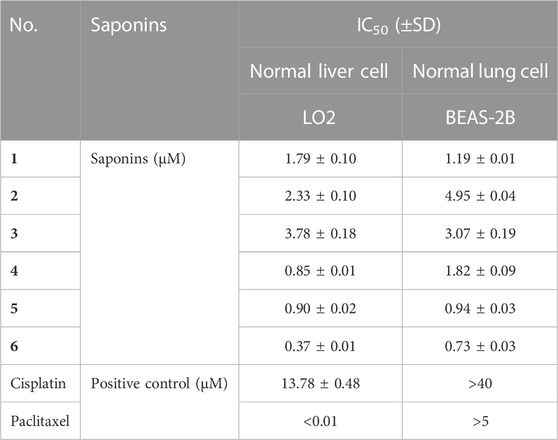
TABLE 3. IC50 values for saponins (1–6) from P. polyphylla var. yunnanensis against normal liver and lung cell lines.
Paris saponins can be divided into diosgenin-type (saponins 3, 4, and 6) and pennogenin-type (saponins 1, 2, and 5) as a function of the presence of a hydroxyl group at site 17 (chain R1 in Figure 1) (Man et al., 2013; Wang et al., 2018). However, the results showed that the activities of these two kinds of saponins provided limitation, related different results compared with pre-evidence (Table 2). Although, the species of Paris has been extensively used for cancer therapy in indigenous medicine knowledge (Yan et al., 2021), research on the anticancer properties and potential mechanisms is still a preliminary exploration mainly due to the limitation of their low in plants. With the development of artificial cultivation techniques of Paris species for the past few years, more and more studies of metabolites and pharmacology are being performed to further identify effective steroidal saponins as potential anticancer drugs (Long et al., 2015; Qin et al., 2018). The results of MTS assay further confirmed the correlation between total saponin content in crude extracts and anticarcinogenic activity. What’s more, the difference in the anticancer activity of six saponins should be highly associated with the type, binding mode and number of glycosyl (Figure 1; Tables 1, 2).
3.4 Paris saponins induce cell cycle arrest in cancer cell lines
To test whether inhibition of the growth of three human cancer cells also affected cell cycle progression (G0/G1, S, and G2/M), induction of A549, SMMC-7721, and HepG2 cell cycles was detected by propidium iodide (PI) staining and flow cytometry after six isolated saponin treatments (Figures 2Bn–Gn). The same cell lines with DMSO were only treated as a negative control (Figure 2An). Substantial changes were detected in the cell cycle progression of the three human cancer cell lines when treated with Paris saponins (Figures 2, 3).
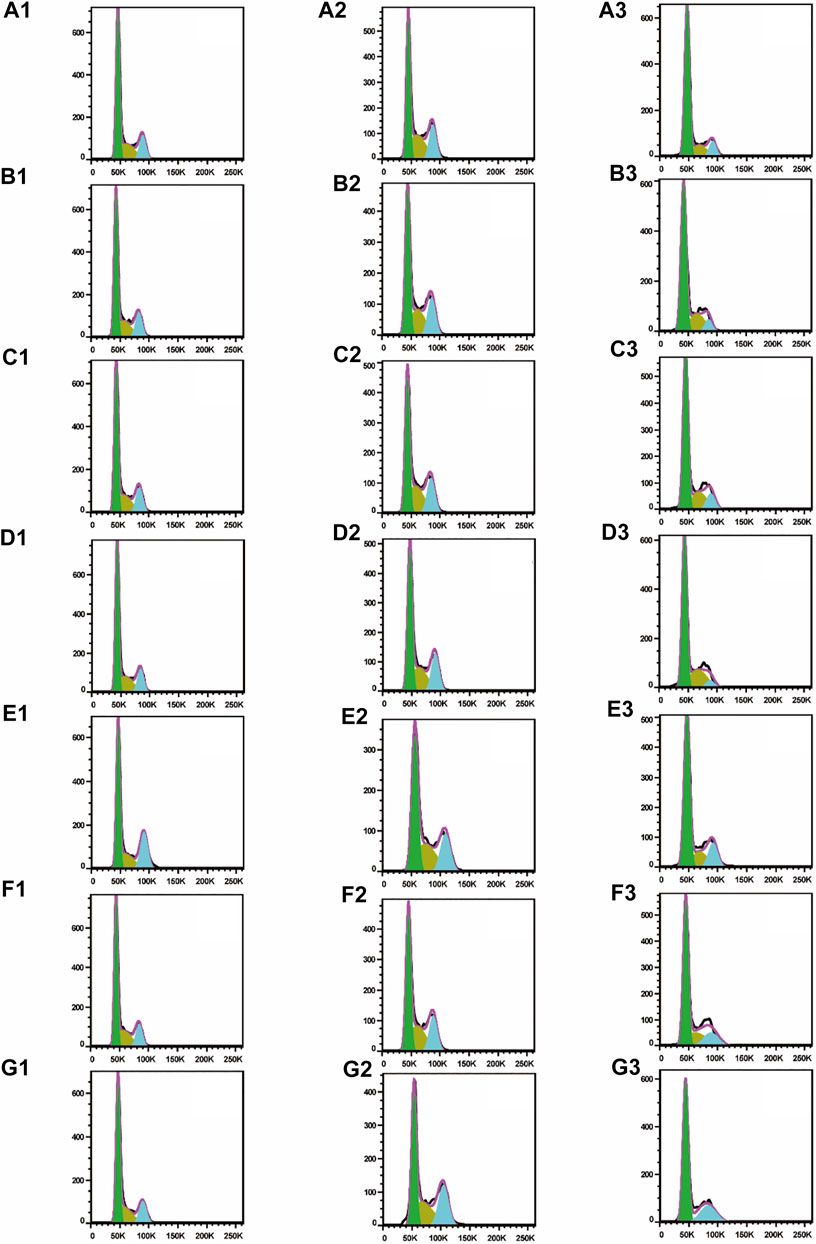
FIGURE 2. All six isolated saponins induce cell cycle arrest in three cancer cell lines. (A1–G1), A549 cells. (A2–G2), SMMC-7721 cells. (A3–G3), HepG2 cells. DMSO was used as a negative control (An). Saponin 1 (Bn), saponin 2 (Cn), saponin 3 (Dn), saponin 4 (En), saponin 5 (Fn), and saponin 6 (Gn), n = 1, 2, 3. For SMMC-7721 and HepG2 cell lines, each saponin was added at 1 μM. For A549 cells, saponins 1 and 5 were added at 0.5 μM; the other four saponins were added at 1 μM.
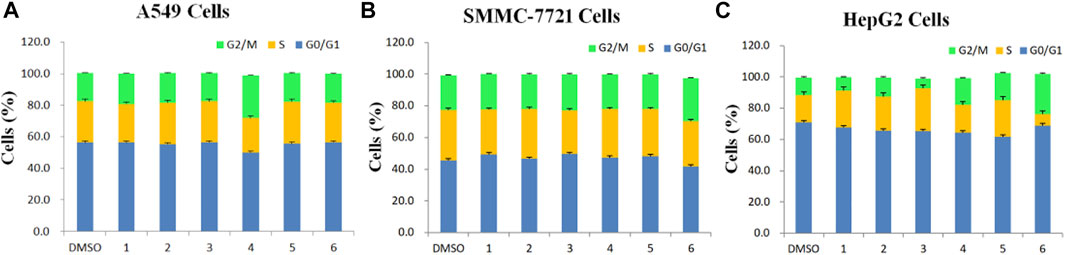
FIGURE 3. Determination of cell cycle stage in three cancer cell lines (A549, SMMC-7721 and HepG2) in the presence of purified saponins.
Compared to the DMSO control, saponin 2 increased the proportion of A549 cells in the S and G2/M phases, while saponins 1, 4, and 6 increased the proportion of cells in the G2/M phase (Figures 2A1–G1). Saponin 5 increased the proportion of cells in S phase, and saponin 3 increased the proportion of cells in the G0/G1 phase.
The six Paris saponins also induced cell cycle arrest in the SMMC-7721 cell line (Figures 2A2–G2). Compared to the DMSO control, saponin 6 increased the proportion of cells in the G2/M phase by 27.10%, while the other five saponins increased the proportion of cells in the G0/G1 phase. The HepG2 cell line was also sensitive to Paris saponins (Figures 2A3–G3). Compared to the DMSO control, saponins 4 and 6 increased the proportion of cells in the G2/M phase by 17.2% and 25.8%, respectively; saponins 1 and 3 increased the proportion of cells in S phase by 23.7% and 27.5%, respectively; and saponins 2 and 5 increased the proportion of cells in the S and G2/M phases.
Many anticancer medications inhibit the growth of tumor cells by targeting the S and G2/M phases of the cell cycle in cancer cells. Indeed, both S and G2/M phases are very important for the proliferation of malignant cancer cells (Sui et al., 2016). A closer look at the flow cytometry data revealed that the proportion of HepG2 cells at the S or G2/M phase treated with any one of the six saponins is higher relative to the DMSO control, suggesting that all six saponins block the cell cycle at the S or G2/M phases (Figure 3C). For the other liver cancer cell line, SMMC-7721, only saponin 6 caused cell cycle arrest at the G2/M phase (Figure 3B). For the A549 lung cancer cell line, only saponin 4 showed a significant effect on the relative proportions of cells in each cell cycle stage, with an increase in the proportion of cells at the G2/M phase, suggestive of cell cycle arrest (Figure 3A).
These results indicate that the six Paris saponins inhibit cancer cell growth by inducing cell cycle arrest at the S or G2/M phase.
3.5 Paris saponins cause a loss of mitochondrial membrane potential
There were two main apoptotic pathways in tumor cells, including the cell surface death receptor-mediated pathway and the mitochondrial apoptosis pathway, the latter being at the core of apoptosis induction (Sui et al., 2016). The six isolated saponins were therefore assessed for their ability to induce early apoptotic cell death in three human cancer cell lines (A549, SMMC-7721, and HepG2) using a mitochondrial membrane potential assay kit based on the potential-dependent accumulation of the JC-10 dye inside mitochondria (Figures 4, 5; Supplementary Figures S9–S11). Red fluorescence from the JC-10 probe indicates a normal mitochondrial membrane potential, while a shift to green fluorescence reflects a drop in membrane potential, which is suggestive of a cell in the early stage of apoptosis.
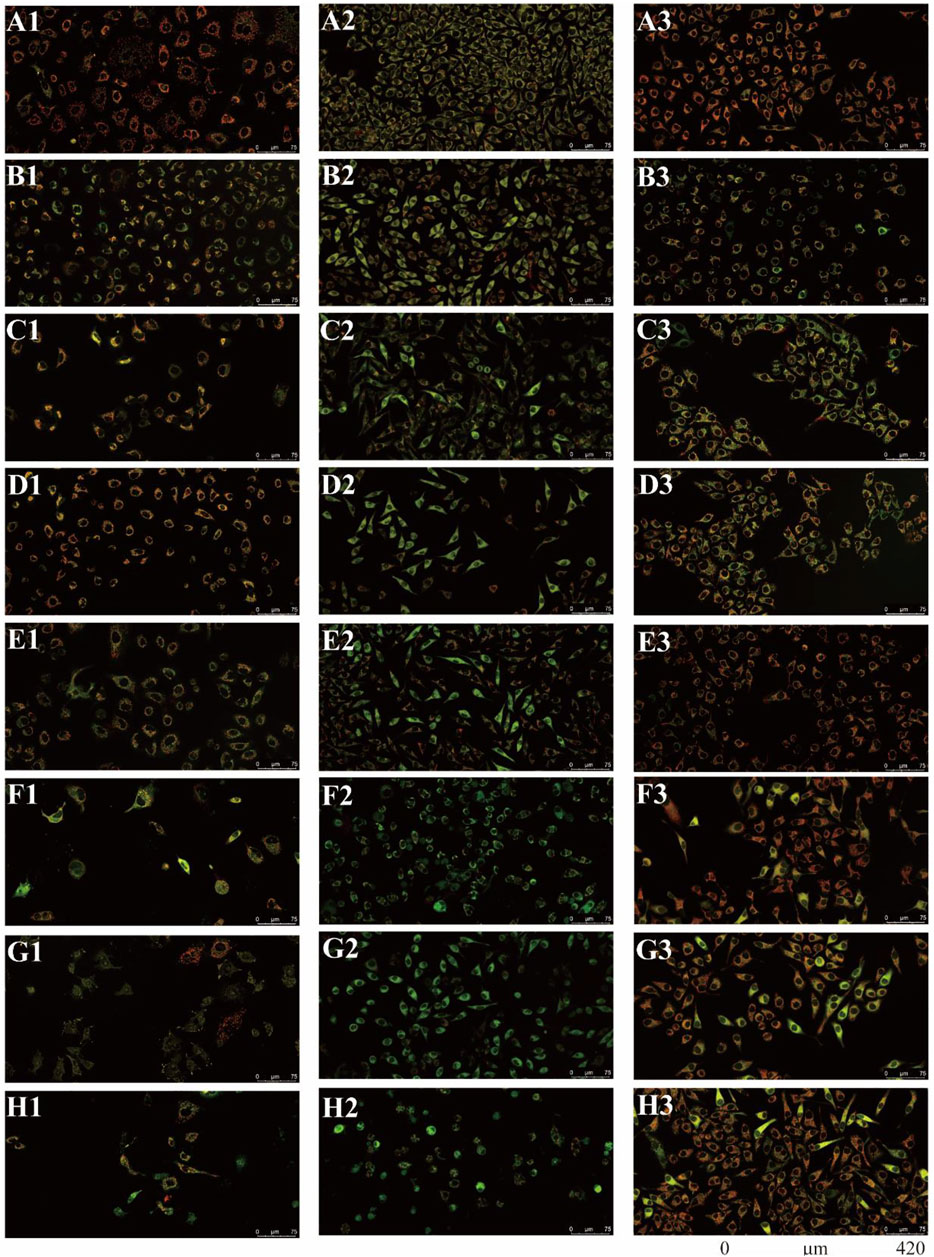
FIGURE 4. All six isolated saponins affect the mitochondrial membrane potential of three cancer cell lines. (A1–H1), A549 cells. (A2–H2), SMMC-7721 cells. (A3–H3), HepG2 cells. Untreated groups served as negative control (An). CCCP-treated groups served as a positive control at 2 μM (Bn). All saponins were added at a concentration of 2 μM: saponin 1 (Cn), saponin 2 (Dn), saponin 3 (En), saponin 4 (Fn), saponin 5 (Gn), and saponin 6 (Hn), n = 1, 2, 3.
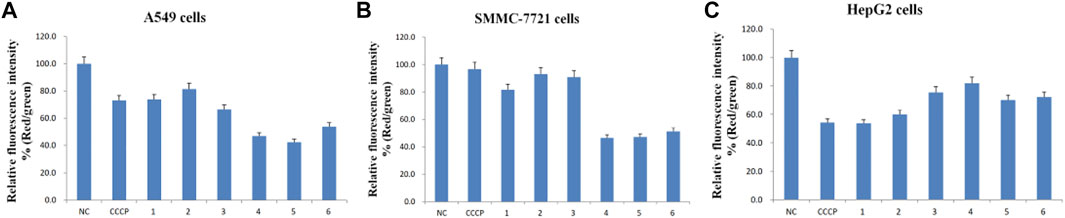
FIGURE 5. Relative fluorescence intensity (Red/green) of the six purified saponins in three cancer cell lines (A549, SMMC-7721 and HepG2).
Cancer cells showed red or yellow-green fluorescence in the absence of any treatment, indicating that their mitochondrial membrane potential is normal (Figures 4A1–A3), which used as negative control (NC). As a positive control for cells with depolarized mitochondrial membranes, cells were treated with the proton ion mitochondrial uncoupling agent carbonyl cyanide 3-chlorophenylhydrazone (CCCP), which resulted in strong green fluorescence (Figures 4B1–B3). Each cancer cell line was also treated with one of the six isolated saponins (Figures 4Cn–Hn). However, the merge by red and green fluorescence for three human cancer cells of six saponins were showed in Figure 4. Also, the merge, green channel and red channel for each human cancer cells of six saponins were showed in Supplementary Figures S9–S11 in the Supplementary Material S2, respectively. Compared to the NC and CCCP control, the green fluorescence was generally higher than red fluorescence in SMMC-7721 cells, next is A549 cells. And for HepG2 cells, the red fluorescence was higher than green fluorescence (Figure 4). Importantly, all purified saponins displayed an increase in green fluorescence over the untreated controls. This finding indicated that the six saponins purified from dried P. polyphylla var. yunnanensis rhizomes increase the permeability of the mitochondrion membrane, leading to a decrease in mitochondrial membrane potential.
JC-10 fluorescent probe can enter mitochondria in normal cells when the membrane potential of mitochondria is high, and it exists in the form of polymer, showing red fluorescence. During the period of cell apoptosis, the mitochondrial membrane potential decreases, and JC-10 is dissociated in the cytoplasm in the form of monomer, showing green fluorescence (Hirose et al., 1974; Castedo et al., 2002). In this study, the ratio of relative fluorescence intensity was used to evaluate the changes of mitochondrial membrane potential, which was indicated by the ratio of red/green fluorescence (Figure 5). The lower the ratio of relative fluorescence intensity was, the more red fluorescence and the less green fluorescence were, which was indicated more severe the damage to the cell membrane, resulting in lower mitochondrial membrane potential. As shown in Figure 5, the ratio of relative fluorescence intensity was decreased in different levels after treating with six saponins, which indicated that the six saponins caused the decrease of mitochondrial membrane potential by damaging mitochondrial membrane, and suggested that cancer cells were in the early stage of cell apoptosis.
To sum up, six Paris saponins may increase the permeability of the mitochondrion membrane in different levels, leading to a decrease in mitochondrial membrane potential.
3.6 Paris saponins induce apoptosis in cancer cell lines
Anticancer drugs inhibit the proliferation of tumor cells by increasing the apoptosis rate (Foo et al., 2015). Encouraged by the results from the mitochondrial membrane potential assay above, the effects of the six isolated saponins on apoptosis were determined by double staining with annexin V-FITC and PI in three human cancer cell lines, including A549, SMMC-7721, and HepG2 (Figure 6). Cancer cells were treated with 0.1% DMSO as a negative control (Figure 6An) and the chemotherapy drug doxorubicin (DOX) as a positive control (Figure 6Bn). These six isolated saponins significantly increased early-stage and late-stage apoptotic cells, as reflected by the proportion of annexin V-FITC+/PI− and annexin V-FITC+/PI+ cells, respectively (Figures 6Cn–Hn). A concentration of 5 μM for each saponin was sufficient to promote apoptosis in the three cancel cell lines tested here. The effects of six saponins on promoting apoptosis were equivalent to that of DOX, or even more than that of DOX.
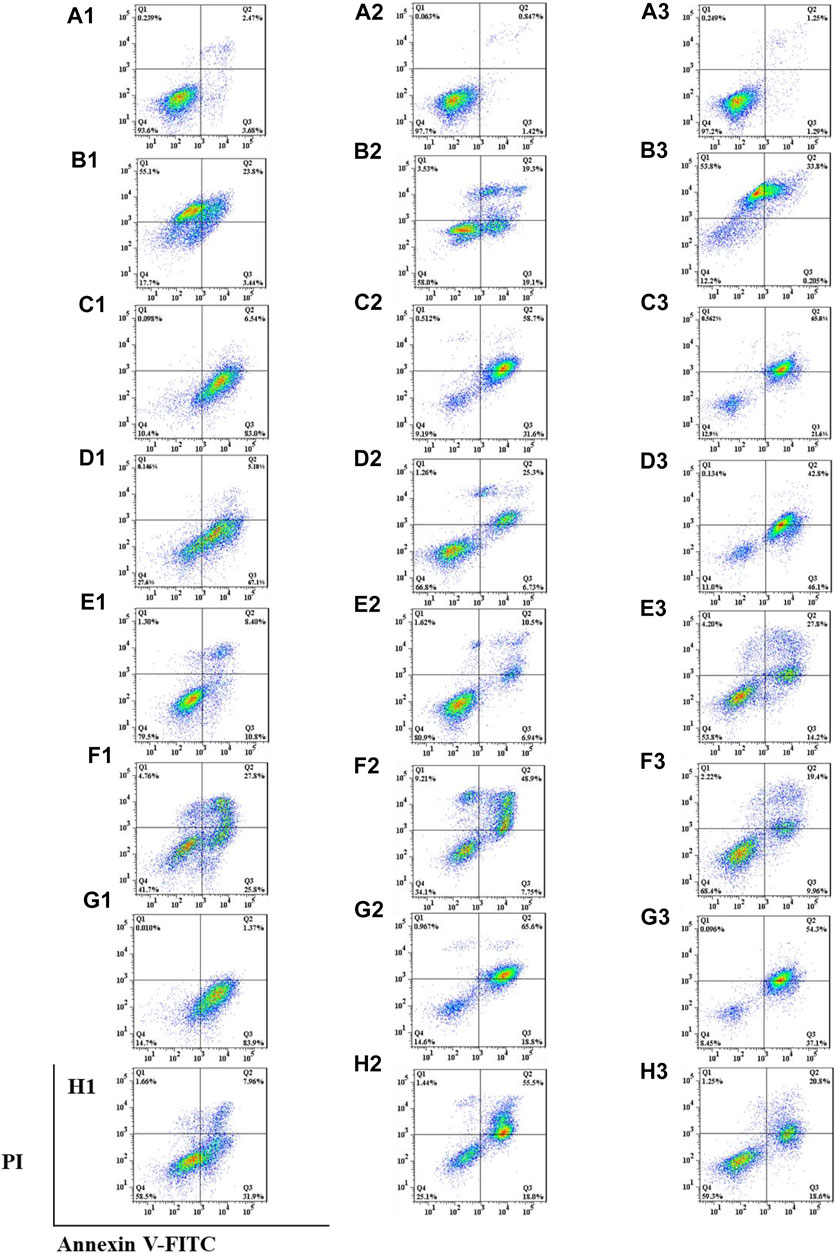
FIGURE 6. All six isolated saponins induce apoptosis in three cancer cell lines. (A1–H1), A549 cells. (A2–H2), SMMC-7721 cells. (A3–H3), HepG2 cells. As negative control, 0.1% (v/v) DMSO was used (An). DOX-treated cultures served as positive control at concentrations of 5, 1, and 5 μM (Bn). All saponins were added at a concentration of 5 μM: saponin 1 (Cn), saponin 2 (Dn), saponin 3 (En), saponin 4 (Fn), saponin 5 (Gn), and saponin 6 (Hn), n=1, 2, 3.
Apoptosis rate of each cell line was calculated in the absence or presence of each purified Paris saponin (Figure 7). Saponin 1 was the most effective in inducing apoptosis in A549 and SMMC-7721 cells and was also effective in HepG2 cells. Saponins 2 and 5 also induced strong apoptosis in A549 cells, while saponins 5 and 6 exhibited a substantial induction of apoptosis in SMMC-7721 cells. For HepG2 cells, the highest apoptosis rate was saponin 5, and next were saponins 2 and 1. Notably, saponins 5 and 1 consistently induced stronger apoptosis than the positive control DOX in all three cancer cell lines. In conclusion, six saponins induced significant apoptosis in the three human cancer cell lines, supporting their potential as inhibitory saponins against the proliferation of cancer cells.
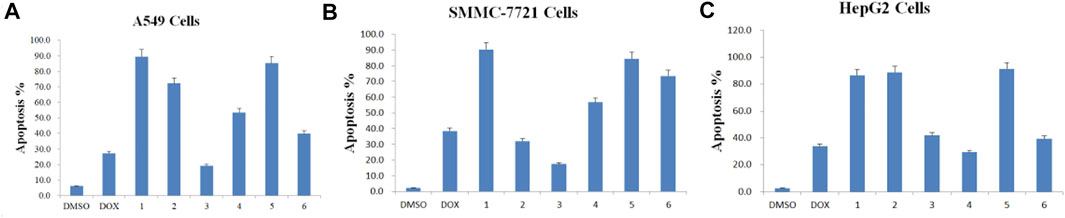
FIGURE 7. Apoptosis rate induced by each of the six purified saponins in three cancer cell lines (A549, SMMC-7721 and HepG2).
We found six saponins inhibited cancer cell growth in three human cancer cells was further demonstrated (Table 2). Moreover, the anticancer activity of six saponins were associated with induction of early- and late-stage apoptosis and cell cycle G2/M or S phase arrest in there human cancer cells (Figures 2, 6). Apoptosis, or programmed cell death, plays a crucial role in controlling cell number in many developmental and physiological settings and in chemotherapy-induced tumour-cell killing, through regulating the normal balance between cell death and survival (Hu and Kavanagh, 2003). Therefore, apoptosis inducers of cancer cells have therapeutic potential in chemoprevention and chemotherapy of cancer (Long et al., 2015). The study showed that the breakdown of mitochondrial membrane is able to enhance the permeability of outer mitochondrial membrane. The release of induced cytochrome and other apoptotic factors from mitochondria into the cytosol is critical to activate the intrinsic apoptotic pathway (Long et al., 2015). Herein, the main effects of six saponins in mitochondrial apoptosis pathway were detected by JC-10 staining and Annexin V- FITC & PI staining in human cancer cells, respectively (Figures 4, 6). Our findings suggest that anti-cancer activity of six saponins is associated with apoptotic induction through a decrease in mitochondrial membrane potential in A549, SMMC-7721, and HepG2 cells.
4 Conclusion
An ethnobotanical study of the indigenous people and local uses of P. polyphylla var. yunnanensis is established, which revealed four ethnic groups historical practice from the rhizome of this plant to treat tumors, raising the possibility that P. polyphylla var. yunnanensis may contain potential anticancer metabolites (Yan et al., 2021). Therefore, it is worth to verify the anticancer activity of indigenous and local use for cultivated P. polyphylla var. yunnanensis. Based on the results of ethnobotanical study, an interdisciplinary approach has been used to study active metabolites in this cultivated species. Five crude extracts were obtained while determined their total saponin contents and cytotoxicity activity. The fraction A3 is the highest of five parts for total saponin contents and cytotoxicity activity. Six Paris saponins from the fraction A3 were isolated, purified and their potential pro-apoptotic activity for use as anti-proliferative drugs was tested. Cultivated P. polyphylla var. yunnanensis rhizomes are a preferable source of these saponins, reaching levels 10 times higher than those set by the standards of the Chinese Pharmacopoeia (2020 version) due to this species is native distribution as well Agriculture Product of Geographical indications. Importantly, both crude extracts and the six purified saponins are effective in inhibiting cell cycle progression in four human cancer cell lines. All six saponins induce the mitochondrial apoptotic pathway, supporting their potent anticancer activities.
Consequently, the rhizomes of P. polyphylla var. yunnanensis show promising anticancer effects in vitro on three liver human cancer cell lines (SMMC-7721, HepG2, and SK-HEP-1) and one NSCLC cell line (A549). The rhizome of cultivated P. polyphylla var. yunnanensis may become an important plant source for anticancer treatment, which will be benefit to conserve this medicinal plant and heritage related indigenous and local medicine knowledge as well as sustainable development. Moreover, this study underscores how the use of indigenous and local medicine knowledge of P. polyphylla var. yunnanensis. Moreover, as a case study, this study provides a new perspective from an ethnobotanical approach to explore anticancer medicine as well as heritage rapidly disappearing indigenous medicine knowledge.
Data availability statement
The original contributions presented in the study are included in the article/Supplementary Material, further inquiries can be directed to the corresponding authors.
Author contributions
X-XY: Lab work, formal analysis, writing—original draft, writing—review and editing. Y-QZ: Writing—review and editing. YH: Investigation, writing—review and editing. TD: Writing—review and editing. HP: Writing—review and editing. AI: Writing—review and editing. L-XY: Writing—review and editing, supervision, and funding acquisition. All authors contributed to the article and approved the submitted version.
Funding
This study was supported by Paris polyphylla Project of Lijiang Yunxin Green Biological Development Co., Ltd. (No. E2524812C1) and the National Nature Science Foundation of China (31970357).
Acknowledgments
We acknowledge the Service Center for Bioactivity Screening and Department of Instrumental Analysis of Kunming Institute of Botany for measuring data. We would like to thank Plant Editors (https://planteditors.com/) for English language editing.
Conflict of interest
Author YH is employed by Lijiang Yunxin Green Biological Development Co., Ltd. This study received funding from Lijiang Yunxin Green Biological Development Co., Ltd. The funder had the following involvement : investigation, collection, writing —review and editing of the study.
The remaining authors declare that the research was conducted in the absence of any commercial or financial relationships that could be construed as a potential conflict of interest.
Publisher’s note
All claims expressed in this article are solely those of the authors and do not necessarily represent those of their affiliated organizations, or those of the publisher, the editors and the reviewers. Any product that may be evaluated in this article, or claim that may be made by its manufacturer, is not guaranteed or endorsed by the publisher.
Supplementary material
The Supplementary Material for this article can be found online at: https://www.frontiersin.org/articles/10.3389/fphar.2023.1100825/full#supplementary-material
Abbreviations
13C-NMR, 13C nuclear magnetic resonance; 1H-NMR, 1H nuclear magnetic resonance; CCCP, carbonyl cyanide 3-chlorophenylhydrazone; DMSO, dimethyl sulfoxide; DOX, doxorubicin; ESI-MS, electrospray ionization–mass spectrometry; FITC, fluorescein isothiocyanate; GAP, good agriculture practice; HCC, hepatocellular carcinoma; HPLC, high-performance liquid chromatography; IC50, half maximal inhibitory concentration; JC-10, 5,6-dichloro-1,1′,3,3′-tetraethyl-imidacarbocyanine iodide; MS, mass spectrometry; MTS, 3-(4,5-dimethylthiazol-2-yl)-5(3-carboxymethoxyphenyl)-2-(4-sulfopheny)-2H-tetrazolium; NC, negative control; NMR, nuclear magnetic resonance; NSCLC, non-small-cell lung cancer; PBS, phosphate-buffered saline; PI, propidium iodide; TCM, traditional Chinese medicine; TLC, thin-layer chromatography; TMS, tetramethylsilane; UV, ultraviolet.
References
Bi, R., Yang, X. N., Zhou, H. F., Peng, L. Y., Liu, J. X., and Zhao, Q. S. (2022). Eleven undescribed alkaloids from the rhizomes of Sinomenium acutum and their Ido1 and TDO inhibitory activities. Phytochemistry 200, 113244. doi:10.1016/j.phytochem.2022.113244
Casciola-Rosen, L., Rosen, A., Petri, M., and Schlissel, M. (1996). Surface blebs on apoptotic cells are sites of enhanced procoagulant activity: Implications for coagulation events and antigenic spread in systemic lupus erythematosus. Proc. Natl. Acad. Sci. U. S. A. 93 (4), 1624–1629. doi:10.1073/pnas.93.4.1624
Castedo, M., Ferri, K., Roumier, T., Metivier, D., Zamzami, N., and Kroemer, G. (2002). Quantitation of mitochondrial alterations associated with apoptosis. J. Immunol. Methods 265 (1), 39–47. doi:10.1016/S0022-1759(02)00069-8
Chaudhari, A. A., Seol, J. W., Kang, S. J., and Park, S. Y. (2008). Mitochondrial transmembrane potential and reactive oxygen species generation regulate the enhanced effect of CCCP on TRAIL-induced SNU-638 cell apoptosis. J. Vet. Med. Sci. 70, 537–542. doi:10.1292/jvms.70.537
Chen, C. X., and Zhou, J. (1987). The steroidal saponins of Paris axialis (2). Plant Divers. 9, 1–3. Yunnanica.
Chen, C. Y., Zhang, Y. T., and Zhou, J. (1983). Studies on the saponin components of plants in yunnan VI: Paris polyphylla saponin (2). Plant divers. 5 (1), 91–97.
Cory, A. H., Owen, T. C., Barltrop, J. A., and Cory, J. G. (1991). Use of an aqueous soluble tetrazolium formazan assay for cell-growth assays in culture. Cancer Commun. 3 (7), 207–212. doi:10.3727/095535491820873191
Deng, D., Lauren, D. R., Cooney, J. M., Jensen, D. J., Wurms, K. V., Upritchard, J. E., et al. (2008). Antifungal saponins from Paris polyphylla Smith. Planta. Med. 74, 1397–1402. doi:10.1055/s-2008-1081345
Ding, Y. G., Zhao, Y. L., Zhang, J., Zuo, Z. T., Zhang, Q. Z., and Wang, Y. Z. (2021). The traditional uses, phytochemistry, and pharmacological properties of Paris L. (liliaceae): A review. J. Ethnopharmacol. 278, 114293–114324. doi:10.1016/j.jep.2021.114293
Ferlay, J., Soerjomataram, I., Dikshit, R., Eser, S., Mathers, C., Rebelo, M., et al. (2015). Cancer incidence and mortality worldwide: Sources, methods and major patterns in GLOBOCAN 2012. Int. J. Cancer 136, E359–E386. doi:10.1002/ijc.29210
Foo, J. B., Saiful Yazan, L., Tor, Y. S., Wibowo, A., Ismail, N., How, C. W., et al. (2015). Induction of cell cycle arrest and apoptosis by betulinic acid-rich fraction from Dillenia suffruticosa root in MCF-7 cells involved p53/p21 and mitochondrial signalling pathway. J. Ethnopharmacol. 166, 270–278. doi:10.1016/j.jep.2015.03.039
Han, X. W., Yu, H., Liu, X. M., Bao, X., Yu, B., Li, C., et al. (1999). Complete1H and13C NMR assignments of diosgenyl saponins. Magn. Reson. Chem. 37 (2), 140–144. doi:10.1002/(sici)1097-458x(199902)37:2<140:aid-mrc409>3.0.co;2-s
Hirose, S., YagiNuma, N., and Inada, Y. (1974). Disruption of charge separation followed by that of the proton gradient in the mitochondrial membrane by CCCP. J. Biochem. 76, 213–216. doi:10.1093/oxfordjournals.jbchem.a130549
Homburg, C. H., Dehaas, M., Vondemborne, A. E., Verhoeven, A. J., Reutelingsperger, C. P. M., and Roos, D. (1995). Human neutrophils lose their surface Fc gamma RIII and acquire Annexin V binding sites during apoptosis in vitro. Blood 85 (2), 532–540. doi:10.1182/blood.V85.2.532.532
Hu, W., and Kavanagh, J. J. (2003). Anticancer therapy targeting the apoptotic pathway. Lancet Oncol. 4, 721–729. doi:10.1016/S1470-2045(03)01277-4
Hu, Y. Y., and Xuan, M. S. (1982). Yunnan anti-cancer Chinese herbs. Kunming: Yunnan People's Publishing House.
Hua, D., Liu, Y., Wang, X. Y., Lu, Y. Y., Li, H., and Tang, H. F. (2015). Chemical constituents of Paris polyphyllavar Smith var. latifolia. Cent. South Pharm. 13 (1), 43–46.
Huang, L. Q., Xiao, P. G., and Wang, Y. Y. (2011). Investigation on resources of rare and endangered medicinal plants in China. Shanghai: Shanghai Science and Technology Press.
Huang, W. G., and Zhou, J. (1962). Studies on steroidal saponins from Paris polyphylla. Med. Pharm. Yunnan. 64−65.
Huang, X. X., Gao, W. Y., Man, S. L., Yan, J. J., and Wang, Y. L. (2009). Chemical constituents from herbs of Paris verticillata. China J. Chin. Mat. Med. 34 (14), 1812–1815.
Ji, Y. H. (2021). A monograph of Paris (Melanthiaceae): Morphology, biology, systematics and taxonomy. Beijing: Science press. doi:10.1007/978-981-15-7903-5
Jing, S. S., Wang, Y., Li, X. J., Li, X., Huang, L. Q., Xiao, P. G., et al. (2017). Chemical constituents from Paris thibetica and their antitumor activities. Chin. Tradit. Herb. Drugs 48 (6), 1093–1098. doi:10.7501/j.issn.0253-2670.2017.06.007
Kang, L. P., Liu, Y. X., Eichhorn, T., Dapat, E., Yu, H. S., Zhao, Y., et al. (2012). Polyhydroxylated steroidal glycosides from Paris polyphylla. J. Nat. Prod. 75, 1201–1205. doi:10.1021/np300045g
Kong, Y. J., Li, F., Nian, Y., Zhou, Z., Yang, R., Qiu, M. H., et al. (2016). KHF16 is a leading structure from cimicifuga foetida that suppresses breast cancer partially by inhibiting the NF- kappa B signaling pathway. Theranostics 6 (6), 875–886. doi:10.7150/thno.14694
Liu, J. X., Ren, J., Yang, K., Chen, S., Yang, X. N., and Zhao, Q. S. (2022). Discovery and biological evaluation of tanshinone derivatives as potent dual inhibitors of indoleamine 2, 3-dioxygenase 1 and tryptophan 2, 3-dioxygenase. Eur. J. Med. Chem. 235, 114294. doi:10.1016/j.ejmech.2022.114294
Liu, J. X., Wu, X. D., Li, W. Y., Yuan, Z. F., Yang, K., and Zhao, Q. S. (2021). Discovery of pseudolaric acid A as a new Hsp90 inhibitor uncovers its potential anticancer mechanism. Bioorg. Chem. 112, 104963. doi:10.1016/j.bioorg.2021.104963
Liu, Q., Chen, F., Hou, L., Shen, L., Zhang, X., Wang, D., et al. (2018). Nanocarrier-mediated chemo-immunotherapy arrested cancer progression and induced tumor dormancy in desmoplastic melanoma. ACS Nano 12, 7812–7825. doi:10.1021/acsnano.8b01890
Liu, Y. Y., Wang, W., Fang, B., Ma, F. Y., Zheng, Q., Deng, P. Y., et al. (2013). Antitumor effect of germacrone on human hepatoma cell lines through inducing G2/M cell cycle arrest and promoting apoptosis. Eur. J. Pharmacol. 698, 95–102. doi:10.1016/j.ejphar.2012.10.013
Long, F. Y., Chen, Y. S., Zhang, L., Kuang, X., Yu, Y., Wang, L. F., et al. (2015). Pennogenyl saponins induce cell cycle arrest and apoptosis in human hepatocellular carcinoma HepG2 cells. J. Ethnopharmacol. 162, 112–120. doi:10.1016/j.jep.2014.12.065
Malich, G., Markovic, B., and Winder, C. (1997). The sensitivity and specificity of the MTS tetrazolium assay for detecting the in vitro cytotoxicity of 20 chemicals using human cell lines. Toxicology 124, 179–192. doi:10.1016/S0300-483X(97)00151-0
Man, S. L., Wang, Y. L., Li, Y. Y., Gao, W. Y., Huang, X. X., and Ma, C. Y. (2013). Phytochemistry, pharmacology, toxicology, and structure-cytotoxicity relationship of Paridis rhizome saponin. Chin. Herb. Med. 5, 33–46.
Mosmann, T. (1983). Rapid colorimetric assay for cellular growth and survival: Application to proliferation and cyto-toxicity assays. J. Immunol. Methods 65, 55–63. doi:10.1016/0022-1759(83)90303-4
Munday, S. C., Wilkins, A. L., Miles, C. O., and Holland, P. T. (1993). Isolation and structure elucidation of dichotomin, a furostanol saponin implicated in hepatogenous photosensitization of sheep grazing Panicum dichotomiflorum. J. Agric. Food Chem. 41 (2), 267–271. doi:10.1021/jf00026a025
Nakano, K., Murakami, K., Takaishi, Y., Tomimatsu, T., and Nohara, T. (1989). Studies on the constituents of heloniopsis orientalis(thunb.) C.tanaka. Tanaka. Chem. Pharm. Bull. 37, 116–118. doi:10.1248/cpb.37.116
National Pharmacopoeia Committee (2020). Pharmacopoeia of the people's Republic of China (I). Beijing: China Pharmaceutical Science and Technology Press.
Pang, X., Wen, D., Zhao, Y., Xiong, C. Q., Wang, X. Q., Yu, L. Y., et al. (2015). Steroidal saponins obtained by biotransformation of total furostanol glycosides from Dioscorea zingiberensis with Absidia coerulea. Carbohydr. Res. 402, 236–240. doi:10.1016/j.carres.2014.11.011
Pei, Y. F., Zhang, Q. Z., and Wang, Y. Z. (2020). Application of authentication evaluation techniques of ethnobotanical medicinal plant genus Paris: A review. Crit. Rev. Anal. Chem. 50, 405–423. doi:10.1080/10408347.2019.1642734
Qin, X. J., Chen, C. X., Ni, W., Yan, H., and Liu, H. Y. (2013). C (22)-steroidal lactone glycosides from stems and leaves of Paris polyphylla var. yunnanensis. Fitoterapia 84, 248–251. doi:10.1016/j.fitote.2012.12.007
Qin, X. J., Ni, W., Chen, C. X., and Liu, H. Y. (2018). Seeing the light: Shifting from wild rhizomes to extraction of active ingredients from above-ground parts of Paris polyphylla var. yunnanensis. J. Ethnopharmacol. 224, 134–139. doi:10.1016/j.jep.2018.05.028
Qin, X. J., Sun, D. J., Ni, W., Chen, C. X., Hua, Y., He, L., et al. (2012). Steroidal saponins with antimicrobial activity from stems and leaves of Paris polyphylla var. yunnanensis. Steroids 77, 1242–1248. doi:10.1016/j.steroids.2012.07.007
Qin, X. J., Yu, M. Y., Ni, W., Yan, H., Chen, C. X., Cheng, Y. C., et al. (2016). Steroidal saponins from stems and leaves of Paris polyphylla var. yunnanensis. Phytochemistry 121, 20–29. doi:10.1016/j.phytochem.2015.10.008
Reed, L. J., and Muench, H. (1938). A simple method of estimating fifty per cent endpoints. Am. J. Hyg. 27, 493–497. doi:10.1093/oxfordjournals.aje.a118408
Sui, Y. X., Li, S. G., Shi, P. Y., Wu, Y. J., Li, Y. X., Chen, W. Y., et al. (2016). Ethyl acetate extract from Selaginella doederleinii Hieron inhibits the growth of human lung cancer cells A549 via caspase-dependent apoptosis pathway. J. Ethnopharmacol. 190, 261–271. doi:10.1016/j.jep.2016.06.029
Sung, H., Ferlay, J., Siegel, R. L., Laversanne, M., Soerjomataram, I., Jemal, A., et al. (2021). Global cancer statistics 2020: GLOBOCAN estimates of incidence and mortality worldwide for 36 cancers in 185 countries. CA-A Cancer J. Clin. 71 (3), 209–249. doi:10.3322/caac.21660
Vermes, I., Haanen, C., Steffens-Nakken, H., and Reutelingsperger, C. (1995). A novel assay for apoptosis. Flow cytometric detection of phosphatidylserine expression on early apoptotic cells using fluorescein labelled Annexin V. J. Immunol. Methods 184 (1), 39–51. doi:10.1016/0022-1759(95)00072-I
Wang, Y., Gao, W. Y., Yuan, L. C., Liu, X. Q., Wang, S. J., and Chen, C. (2007). Chemical constituents from rhizome of Paris polyphylla var. yunnanensis. Chin. Trad. Herb. Drugs 38 (1), 17–20.
Wang, Y. H., Niu, H. M., Zhang, Z. Y., Hu, X. Y., and Li, H. (2015). Medicinal values and their chemical bases of Paris. China J. Chin. Mat. Med. 40, 833–839. doi:10.4268/cjcmm20150511
Wang, Y. H., Shi, M., Niu, H. M., Yang, J., Xia, M. Y., Luo, J. F., et al. (2018). Substituting one Paris for another? in vitro cytotoxic and in vivo antitumor activities of Paris forrestii, a substitute of Paris polyphylla var. yunnanensis. J. Ethnopharmacol. 218, 45–50. doi:10.1016/j.jep.2018.02.022
Wang, Z., Cai, X. Z., Zhong, Z. X., Xu, Z., Wei, N., Xie, J. F., et al. (2017). Paris nitida (melanthiaceae), a new species from Hubei and hunan, China. Phytotaxa 314, 145–149. doi:10.11646/phytotaxa.314.1.16
Wang, Z., Zhou, J., Ju, Y., Zhang, H., Liu, M., and Li, X. (2001). Effects of two saponins extracted from the Polygonatum zanlanscianense Pamp on the human leukemia (HL-60) cells. Biol. Pharm. Bull. 24 (2), 159–162. doi:10.1248/bpb.24.159
Wei, J. C., Gao, W. Y., Yan, X. D., Wang, Y., Jing, S. S., and Xiao, P. G. (2014). Chemical constituents of plants from the genus Paris. Chem. Biodivers. 11, 1277–1297. doi:10.1002/cbdv.201300083
Wei, R. R., Zhao, Y., Wang, J., Yang, X., Li, S., Wang, Y., et al. (2021). Tagitinin C induces ferroptosis through PERK-Nrf2-HO-1 signaling pathway in colorectal cancer cells. Int. J. Biol. Sci. 17 (11), 2703–2717. doi:10.7150/ijbs.59404
Wu, X., Wang, L., Wang, G. C., Wang, H., Dai, Y., Yang, X. X., et al. (2013). Triterpenoid saponins from rhizomes of Paris polyphylla var. yunnanensis. Carbohydr. Res. 368, 1–7. doi:10.1016/j.carres.2012.11.027
Wu, X., Wang, L., Wang, H., Dai, Y., Ye, W. C., and Li, Y. L. (2012). Steroidal saponins from Paris polyphylla var. yunnanensis. Phytochemistry 81, 133–143. doi:10.1016/j.phytochem.2012.05.034
Yan, X. X., Pan, Q. D., Sun, H. Y., Gao, L., Yang, R., and Yang, L. X. (2021). Traditional use of Paris polyphylla and its active components. China J. Chin. Mat. Med. 46, 6343–6352. doi:10.19540/j.cnki.cjcmm.20210901.101
Yang, G. Y., Li, X., Li, X. L., Wang, L., Li, J., Song, X., et al. (2012). Traditional Chinese medicine in cancer care: A review of case series published in the Chinese literature. Evid. Based Complement. Altern. Med. 2012, 751046. doi:10.1155/2012/751046
Yang, J. D., and Roberts, L. R. (2010). Hepatocellular carcinoma: A global view. Nat. Rev. Gastroenterol. Hepatol. 7, 448–458. doi:10.1038/nrgastro.2010.100
Zhang, C., Jia, X., Bao, J., Chen, S., Wang, K., Zhang, Y., et al. (2016). Polyphyllin VII induces apoptosis in HepG2 cells through ROS-mediated mitochondrial dysfunction and MAPK pathways. BMC Complement. Altern. Med. 9, 58. doi:10.1186/s12906-016-1036-x
Zhang, Y. B., Wu, X., Li, Y. L., and Wang, G. C. (2014). Chemical constituents of Paris polyphylla var. yunnanensis. J. Jinan Univ. Sci. Technol. 35 (1), 66–72.
Keywords: Paris polyphylla var. yunnanensis, indigenous medicine knowledge, saponins, cytotoxic effects, pro-apoptotic effects
Citation: Yan X-X, Zhao Y-Q, He Y, Disayathanoowat T, Pandith H, Inta A and Yang L-X (2023) Cytotoxic and pro-apoptotic effects of botanical drugs derived from the indigenous cultivated medicinal plant Paris polyphylla var. yunnanensis. Front. Pharmacol. 14:1100825. doi: 10.3389/fphar.2023.1100825
Received: 17 November 2022; Accepted: 10 January 2023;
Published: 26 January 2023.
Edited by:
Muhammad Riaz, Shaheed Benazir Bhutto University, PakistanReviewed by:
Liqin Ding, Tianjin University of Traditional Chinese Medicine, ChinaXie-An Yu, Shenzhen Institute for Drug Control, China
Copyright © 2023 Yan, Zhao, He, Disayathanoowat, Pandith, Inta and Yang. This is an open-access article distributed under the terms of the Creative Commons Attribution License (CC BY). The use, distribution or reproduction in other forums is permitted, provided the original author(s) and the copyright owner(s) are credited and that the original publication in this journal is cited, in accordance with accepted academic practice. No use, distribution or reproduction is permitted which does not comply with these terms.
*Correspondence: Angkhana Inta, aungkanainta@hotmail.com; Li-Xin Yang, rattan@mail.kib.ac.cn
†These authors have contributed equally to this work