- Guang’anmen Hospital, China Academy of Chinese Medicine Sciences, Beijing, China
Atherosclerosis (AS) is the pathology of atherosclerotic cardiovascular diseases (ASCVD), characterized by persistent chronic inflammation in the vessel wall, in which monocytes/macrophages play a key role. It has been reported that innate immune system cells can assume a persistent proinflammatory state after short stimulation with endogenous atherogenic stimuli. The pathogenesis of AS can be influenced by this persistent hyperactivation of the innate immune system, which is termed trained immunity. Trained immunity has also been implicated as a key pathological mechanism, leading to persistent chronic inflammation in AS. Trained immunity is mediated via epigenetic and metabolic reprogramming and occurs in mature innate immune cells and their bone marrow progenitors. Natural products are promising candidates for novel pharmacological agents that can be used to prevent or treat cardiovascular diseases (CVD). A variety of natural products and agents exhibiting antiatherosclerotic abilities have been reported to potentially interfere with the pharmacological targets of trained immunity. This review describes in as much detail as possible the mechanisms involved in trained immunity and how phytochemicals of this process inhibit AS by affecting trained monocytes/macrophages.
1 Introduction
Atherosclerotic cardiovascular diseases (ASCVD) have emerged as the most common burden of disease as a result of the aging and expanding global population (Mensah et al., 2019). As the pathology of ASCVD, atherosclerosis (AS) generates a continuous buildup of vessel-occluding plaques in the subendothelial intimal layer of coronary arteries, eventually leading to considerable blood flow restriction and essential tissue hypoxia (Libby, 2002; Gallino et al., 2014). Most cardiovascular events are caused by the rupture of atherosclerotic plaques in the arterial artery wall and the subsequent formation of an occluding thrombus.
In addition to the deposition and retention of modified lipoproteins and the buildup of immune cells in the walls of major arteries, AS is characterized by a low-grade, persistent, chronic inflammation of the arterial wall (Edgar et al., 2021). All phases of AS are mostly attributed to monocytes and monocyte-derived macrophages, which are also thought to be responsible for persistent chronic inflammation (Moore et al., 2013). The traditional view is that innate immune cells, such as macrophages, can only eliminate pathogens non-specifically through biological processes such as phagocytes (Bonilla and Oettgen, 2010). However, a growing body of research suggests that monocytes/macrophages may also develop memory capabilities similar to those of the adaptive immune system after exposure to pathogens (Arts et al., 2018). Myeloid cells of the innate immune system become more sensitive after activation with the same or different stimuli to produce a persistent inflammatory monocyte/macrophage phenotype, a phenomenon known as “trained immunity” or “innate immunological memory” (Netea et al., 2020). This persistent overactivation of the innate immune system could contribute to the incessant vascular wall inflammation that is characteristic of AS (Moore et al., 2013).
For thousands of years, herbal medicines have been widely utilized alone or as a supplementary strategy to treat various disorders in East Asia because of their reduced toxicity, fewer side effects, and cheaper cost (Wang et al., 2018). Along with the development of these natural therapies, herbal medicine is becoming more widely accepted as a supplement and alternative therapy in many countries (Liang et al., 2021). According to the most recent statistics on US-FDA (United States Food and Drug Administration) authorized drugs, herbal remedies have been a vital source of novel medications (Newman and Cragg, 2020). A growing body of scientific evidence has revealed that natural medicines and phytochemicals from natural herbal medicines exhibit promising anti-AS properties (Zhang et al., 2021a). Based on the regulation of targeting trained immunity in monocyte and macrophage, natural compounds generated from herbal remedies are surely excellent resources for selecting potential therapeutics to treat AS.
This review aims to provide more information on the role of trained immunity in the pathophysiology of AS, which might be a potential pharmacological target of natural products.
2 Trained immunity in AS
Conventional wisdom generally considers the adaptive immune system as a specific protective mechanism that can form more specialized lines of defense against re-infection with the same pathogens (Domínguez-Andrés et al., 2019). However, innate immune cells (e.g., macrophages/monocytes) have been reported to display similar immune memory, referred to as trained immunity (Conrath et al., 2015; Milutinović and Kurtz, 2016; Gourbal et al., 2018). Studies of gene-specific chromatin changes brought about by lipopolysaccharide (LPS) have first shown trained immunity characteristics of monocytes/macrophages (Foster et al., 2007). Subsequently, infectious stimuli, such as β-glucan and Bacille Calmette–Guérin (BCG), improved their reactivity to stimulation with unrelated infections or molecular patterns linked with those pathogens (Quintin et al., 2012; Saeed et al., 2014). Factors that contribute to the development of AS, such as uric acid and oxidized low-density lipoprotein (oxLDL), and other endogenous ligands can activate trained immunity (Bekkering et al., 2014; Crişan et al., 2017). Freshly isolated monocytes from patients who had symptoms of coronary artery disease (CAD) had a higher capacity to produce cytokines than those from healthy controls, and this capacity was maintained following ex vivo conversion to macrophages for 5 days (Shirai et al., 2016). The atherogenic factors are characterized by increased production of proatherogenic cytokines and chemokines like tumor necrosis factor-α (TNF-α), IL-6, monocyte chemoattractant protein-1 (MCP-1), matrix metalloproteinases 2 (MMP-2), and MMP-9 and increased foam cell formation is indeed demonstrated by large-scale phenotyping of trained macrophages in vitro (Bekkering et al., 2014).
After short activation with endogenous ligands, a persistent proinflammatory phenotype can emerge in AS monocytes/macrophages (Leentjens et al., 2018). The three key components of trained immunity are metabolic reprogramming, epigenetic reprogramming, and the promotion of myelopoiesis progenitors (Fanucchi et al., 2021) (Figure 1). First, metabolic reprogramming is responsible for the induction, maintenance, and regulation of trained immunity. Different metabolic pathways supply the required substrates for altering the structure of the respective sections of the chromatin and genome, in addition to acting as a source of energy and building components for the dynamic remodeling of the epigenetic landscape. Second, epigenetic reprogramming ultimately links metabolic changes to a cell’s gene expression and inflammatory phenotype. Finally, trained immunity in bone marrow by hematopoietic stem cells (HSCs) maintains long-term effects on circulating monocytes through differentiation into progenitor and mature cells (Mitroulis et al., 2018). In addition to atherosclerotic triggers, such as lipoproteins, glucose, diet, and microbiota-derived substances, proinflammatory cytokines secreted by monocytes/macrophages with an inflammatory phenotype may alter the tissue microenvironment by altering macrophages, the functional state of cells, leading to a vicious circle (Groh et al., 2018).
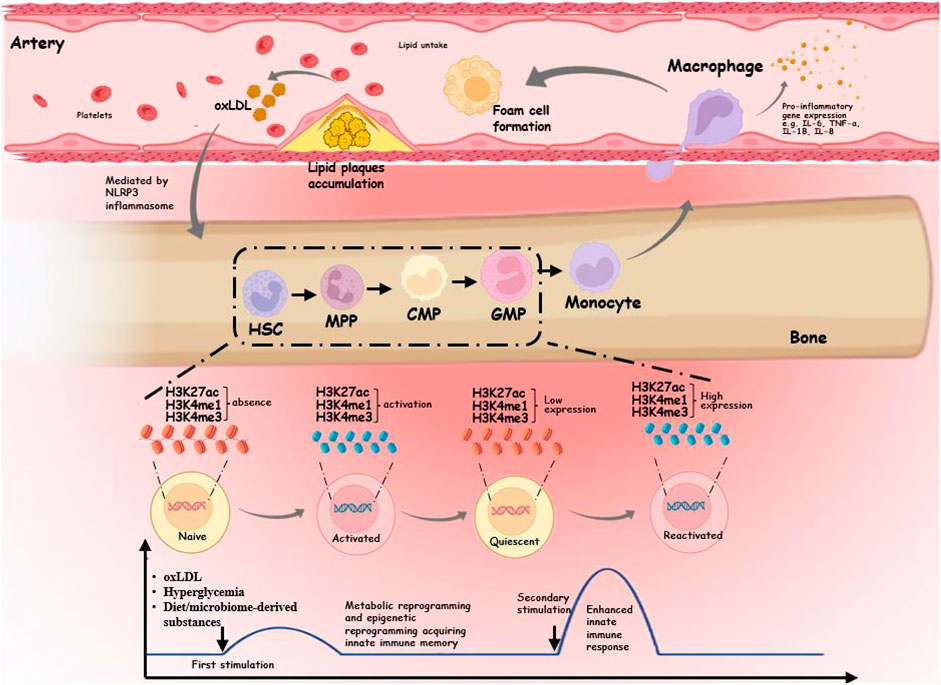
FIGURE 1. Schematic diagram of the trained immunity mechanism in atherosclerotic cardiovascular disease. In the hematopoietic system, myeloid cells exposed to endogenous triggers undergo epigenetic and metabolic reprogramming, resulting in acquiring innate immune memory. The initial gene activation is accompanied by the accumulation of H3K4me3 on the gene promoter, and the persistence of H3K4me1 or H3K27ac in secondary stimulation leads to an enhanced innate immune response. These trained myeloid cells differentiate into monocytes, which travel further into the intima to become macrophages. Trained macrophages produce high levels of proinflammatory cytokines such as TNF-α, IL-6, IL-8, and IL-18 and uptake of lipids to form foam cells. When plaques form, endogenous stimuli may be further released to form trained immunity mediated by the NLRP3 inflammasome.
2.1 Metabolic reprogramming of trained immunity in AS
The trained immune activation has to quickly access a supply of substrates to initiate the numerous metabolic processes associated with the immune response. Intracellular metabolic pathways of glucose, amino acids, lipids, and nucleic acids are altered in response to trained immune activation (Fanucchi et al., 2021). When normal cells are at rest, they obtain enough energy via metabolic processes that are extremely effective but rather slow, such as oxidative phosphorylation (OXPHOS) and fatty acid oxidation (FAO) (Augert et al., 2020). In contrast, trained immune cells continue to opt for “aerobic glycolysis,” which uses glycolysis instead of OXPHOS to generate energy under normoxic conditions, similar to the “Warburg effect” in cancer (Mills et al., 2016; Renner et al., 2017). In addition to glucose metabolism, trained immune cells exhibit altered lipid and amino acid metabolic patterns. For instance, the Krebs cycle’s anabolic redefinition to synthesize cholesterol and phospholipids from citrate and acetyl coenzyme A (CoA) is a crucial metabolic event in trained monocytes (Arts et al., 2016a). When exposed to β-glucan, cholesterol synthesis is increased, but fluvastatin, an inhibitor of the enzyme 3-hydroxy-3-methylglutaryl-coenzyme A (HMG-CoA) reductase, inhibits trained immunity by downregulating histone H3 lysine 4 trimethylation (H3K4me3) and limiting the production of proinflammatory cytokines (Bekkering et al., 2018). For the progression of AS, the control of cholesterol import and efflux is critical. Similar to glutamine, arginine, and glycine, several particular amino acids are overexpressed in atherosclerotic plaques and have AS-promoting effects (Mallat et al., 1999; Sheehan et al., 2011). The intermediate metabolites from many metabolic pathways, such as aerobic glycolysis, glutaminolysis, cholesterol metabolism, and fatty acid synthesis, not only are a source of energy for the cell but also play several significant biological roles (Groh et al., 2018). Additionally, some chemo drugs made from natural herbal products, such as resveratrol and epigallocatechin gallate, can prevent cells from reprogramming their metabolism in response to AS.
2.1.1 Glucose metabolism and AS
Although OXPHOS produces ATP more efficiently than other cellular processes (approximately 30 ATP molecules can be produced per glucose molecule during OXPHOS, whereas glycolysis can only produce two ATP molecules per glucose molecule) (Tabas and Bornfeldt, 2020). However, glycolysis produces ATP faster than OXPHOS, allowing immune cells to respond quickly to stimuli (Tabas and Bornfeldt, 2020). A clinical trial found an enhanced capacity for cytokine production in circulating monocytes obtained from ASCVD patients, which was associated with the upregulation of glycolytic enzymes (Bekkering et al., 2016; Shirai et al., 2016). This phenotype continued even after in vitro macrophage differentiation, displaying a greater glycolytic flux and a higher oxygen consumption rate (Shirai et al., 2016). Furthermore, the inhibition of specific tricarboxylic acid (TCA) cycle steps that support inflammatory processes is associated with increased glycolysis in inflammatory macrophages that primarily produce and release proinflammatory mediators, such as interleukin-1 (IL-1), TNF-α, chemokine C–C motif ligand 2 (CCL2), IL-12, and nitric oxide (NO), through inducible nitric oxide synthase (iNOS). The key proteins involved in glycolysis are introduced in the following sections.
2.1.1.1 GLUT1
Glucose transporter 1 (GLUT1; gene name SLC2A1) on the cell membrane initiates glucose uptake by monocytes/macrophages (Fukuzumi et al., 1996). LPS and oxLDL, which cause inflammation, can boost GLUT1 expression and thereby increase glucose influx. Hexokinase phosphorylates glucose inside the cell to produce glucose-6-phosphate, which is then utilized in the pentose phosphate pathway (PPP), fatty acid synthase (FAS), or glycolysis. When glucose is processed in the cytosol by glycolysis, two ATPs and pyruvates are produced. Pyruvate produced during glycolysis either enters the mitochondrial TCA cycle or is transformed to lactate by lactate dehydrogenase (Christofk et al., 2008). In plaque macrophages, GLUT1 can promote antiatherosclerotic activities. The efferocytosis procedure increases the expression of GLUT1, which promotes an increase in glucose absorption and a transition from OXPHOS to improved aerobic glycolysis, both of which are required for the effective clearance of apoptotic cells (Morioka et al., 2018). When myeloid-targeted LysM-Cre Slc2a1fl/fl animals were transplanted into Ldlr−/− mice on a Western-style diet (WTD), the amount of necrotic core in the aorta increased (Morioka et al., 2018). Another study revealed that GLUT1 deletion in hematopoietic cells inhibited myelopoiesis, monocyte recruitment to lesions, and the progression of AS in ApoE−/− mice, indicating that the main role of GLUT1 in this model was to encourage the proliferation of bone marrow HSCs and multi-potential progenitors, as well as the commitment of these cells to the bone marrow (Sarrazy et al., 2016).
2.1.1.2 HIF-1α
Hyperoxia-inducible substance 1α (HIF-1α) is activated in hypoxic circumstances, allowing cells to switch to glycolysis and create ATP when oxygen is limited. Low oxygen levels trigger the HIF-1α transcription factor to initiate glycolytic metabolism, which decreases the need for OXPHOS and increases the expression of the key glycolysis proteins GLUT1, hexokinase II (HK-II), and 6-phosphofructo-2-kinase/fructo-2, 6-bisphosphatase (PFKFB3), which increases glycolytic flux (Tawakol et al., 2015). The activated macrophages will emit a lot of cytokines and absorb a lot of glucose. Indeed, hypoxia, HIF-1α expression, and FDG (fluorodeoxyglucose) uptake in macrophages are associated with atherosclerotic plaques in animal models of AS (Folco et al., 2011; Tawakol et al., 2015; Aarup et al., 2016).
2.1.2 Lipid metabolism and AS
In homeostasis, lipoproteins taken up by macrophages are transported to lysosomes for the hydrolysis of cholesteryl esters. Free cholesterol is transported to the cytoplasm, where it is transported to the cell membrane for export or transported to the ER for re-esterification and storage in lipid droplets (LDs). Macrophages in advanced plaques provide signs of huge accumulations of free cholesterol, which suggests a breakdown in the mechanisms that keep cholesterol levels in balance. Membrane damage and metabolic dysregulation in the ER and mitochondria are required to maintain macrophage cholesterol homeostasis and reduce inflammation, which results from excessive accumulation of free cholesterol. Furthermore, high levels of modified cholesterol, oxLDL, are taken up by macrophages to form foam cells and promote plaque by secreting numerous proinflammatory cytokines and chemokines and producing MMPs that degrade plaque extracellular matrix pathogenesis (Khokha et al., 2013; Tall and Yvan-Charvet, 2015). It is reported that the induction of trained immunity in monocytes required stimulation of the cholesterol biosynthesis pathway but not cholesterol synthesis itself. oxLDL is an endogenous ligand that triggers trained immunity to activate monocytes/macrophages (Bekkering et al., 2014; Crişan et al., 2017). Another crucial characteristic of monocytes trained on β-glucan is increased cholesterol production (Netea et al., 2020). In primary human monocytes, fluvastatin, an inhibitor of HMG-CoA reductase, inhibits trained immunity (Arts et al., 2016a). Notably, β-glucan-induced training of mature myeloid cells and their progenitors requires enhanced cholesterol production. The accumulation of cholesterol esters and lipids with more saturated acyl chains is associated with the long-term myelopoiesis bias that β-glucan induced training imparts to HSCs (Mitroulis et al., 2018). The HSC population increase and myelopoiesis caused by β-glucan are reduced by HMG-CoA reductase inhibitor (Mitroulis et al., 2018).
2.1.3 Amino acid metabolism and AS
Under AS pathological conditions, amino acid metabolites play an important role in supporting the induction, maintenance, and regulation mechanisms of trained immunity (Napoli et al., 2006). Therefore, it is necessary to decipher the role of specific amino acid metabolites in the induction of trained immunity.
2.1.3.1 Glutamine
Glutamate is one of the amino acids that has been well-studied for its role in controlling inflammation (Wallace and Keast, 1992). By directly converting into glutamate, α-ketoglutarate, and succinate semialdehyde, glutamine contributes to the TCA cycle (Jha et al., 2015). Additionally, glutamate can be employed as a source of citrate for the FAS-catalyzed production of fatty acids. Recent research has demonstrated that glutaminolysis is increased in trained macrophages and is essential for the establishment of a trained macrophage phenotype in response to β-glucan (Arts et al., 2016a). In a different research study, oxidized phospholipids made of 1-palmitoyl-2-arachidonoyl-sn-glycero-3-phosphorylcholine (oxPAPC) were exposed to macrophages, which led to the development of AS, glutaminolysis, and IL-1 48. This study demonstrated that in contrast to macrophages activated with LPS alone, those exposed to oxPAPC and LPS together had a metabolic change (Di Gioia et al., 2020). This metabolic shift was characterized by increased mitochondrial respiration, glutaminolysis, and accumulation of oxaloacetate, which stabilized HIF-1α and increased IL-1β production. IL-1β immunoreactivity in CD68+ lesional cells decreased in mice with systemic suppression of this pathway, which also reduced early AS (Di Gioia et al., 2020). Furthermore, the TCA cycle’s glutamine replenishment causes fumarate to accumulate, which integrates immunological and metabolic circuits to cause monocyte epigenetic reprogramming by inhibiting KDM5 activity and boosting the methylation of histone lysine 4 residues (Arts et al., 2016a). An epigenetic program identical to trained immunity-mediated by β-glucan was induced by fumarate. To support this, glutaminolysis inhibition and cholesterol production suppression in mice decreased the induction of trained immunity by β-glucan (Arts et al., 2016a).
2.1.3.2 Arginine
In the context of AS pathology, arginine metabolism and its by-product NO are critical for the early stages of the disease (Lv et al., 2021). iNOS is ubiquitously expressed in activated and developing macrophages. NO creation by arginine is probably a factor in the metabolic transition. NO has been reported to inhibit OXPHOS in activated dendritic cells and inflammatory macrophages downstream of iNOS (Everts et al., 2012; Van den Bossche et al., 2016). Conversely, in alternatively activated macrophages and in the macrophages of atherosclerotic lesions that are regressing, Arg1 converts arginine to putrescine (Willecke et al., 2015). Under specific conditions, these two arginine metabolic routes can inhibit one another. As a result, NO inhibits ornithine decarboxylase’s ability to catalyze the conversion of ornithine to putrescine by S-nitrosylation a cysteine that is essential for the enzyme’s ability to function (Bauer et al., 2001). Conversely, ornithine decarboxylase prevents macrophages from becoming activated in an inflammatory response (Hardbower et al., 2017). Arginine metabolism has been reprogrammed, which promotes proinflammatory and healing processes.
2.1.3.3 Serine
Recent research has demonstrated that LPS-activated macrophages promote serine synthesis, PPP, and one-carbon metabolism, which synergistically drive epigenetic reprogramming of IL-1β expression. The production of S-adenosylmethionine (SAM) during LPS-induced inflammation is fueled by the synergistic integration of glucose-derived ribose and one-carbon units supplied by glucose and serine metabolism into the methionine cycle through de novo ATP synthesis. Impairment of these metabolic pathways that feed SAM generation leads to anti-inflammatory outcomes (Yu et al., 2019). According to a different research study, serine is necessary for the synthesis of glutathione and IL-1β through the action of glycine (Rodriguez et al., 2019).
2.2 Epigenetic reprogramming of trained immunity in AS
Regulating gene expression without changing the DNA sequence itself is referred to as epigenetic reprogramming. Epigenetic reprogramming enables innate immune cells to react to future stimuli with a stronger, quicker, or qualitatively different transcriptional response (Zarzour et al., 2019). Epigenetic regulatory mechanisms encompass diverse molecular processes, including histone post-translational modifications, DNA methylation, and long non-coding RNAs (lncRNAs).
2.2.1 Histone modifications
Epigenetic reprogramming occurs primarily through histone changes at the level of the chromatin structure to promote a sustained enhanced functional state of trained innate immune cells (Saeed et al., 2014). Neutralization of the positive charge of lysine residues in histones by histone acetylation increases the binding of transcription factors activating gene transcription (Bannister and Kouzarides, 2011). The specific lysine residue implicated and the sum of the additional methyl groups determine the effect of histone methylation on gene transcription. Two important epigenetic marks for trained immunity are as follows: the H3K4me3 accumulation at the gene promoter and the histone 3 lysine 27 acetylation (H3K27ac) acquisition at the gene’s distal enhancer (generated by histone 3 lysine acid 4 methylation (H3K4me1)) (Netea et al., 2020).
2.2.2 DNA methylation
DNA methylation is involved in the regulation of patterns of gene expression. DNA methyltransferases (DNMTs) use CpG-rich regions as recognition cues to methylate cytosines (m5C), which suppresses transcription. Proteins with histone-binding domains that can “read,” “write,” or “erase” histone marks may detect the tails that protrude from histone octamers (Fanucchi et al., 2021). In addition to methylation, acetylation, phosphorylation, and ubiquitination, these enzymes can catalyze the addition or removal of a broad and diverse range of other histone modifications. Different DNA methylation patterns discriminate between “responders” (those who can experience taught immunity) and “non-responders” to stimuli, such as BCG, that produce trained immunity (Verma et al., 2017). Forty-three genes had distinct methylation patterns in BCG-naive responders as opposed to non-responders in a follow-up investigation, which may be utilized to predict sensitivity to triggers of trained immunity (Das et al., 2019). Numerous studies have shown that various DNA and histone modification combinations affect whether DNA is kept in an accessible or “open” state or an inaccessible or “closed” one (Fanucchi et al., 2021). To enable quick and effective transcriptional activation, highly accessible DNA is quickly bound by the transcriptional machinery and transcription factors. This establishes a clear connection between the transcriptional state of protein-coding genes and the “openness” of DNA.
2.2.3 lncRNAs
During trained immunity, lncRNA-dependent regulation has a significant impact on the epigenetic reprogramming of immune genes (Fanucchi et al., 2021). Several lncRNAs known as immune-gene priming lncRNAs (IPLs) were found by the application of a bioinformatic pipeline that comprised 3D nuclear architecture, lncRNA and enhancer expression data, and the epigenetic status of immune genes at the genome scale (Fanucchi et al., 2019). The WD repeat-containing protein 5 (WDR5)-mixed lineage leukemia protein 1 complex is directed across the chemokine promoters by UMLILO (upstream master lncRNA of the inflammatory chemokine locus), allowing H3K4me3 epigenetic priming, according to careful analysis of a prototypical IPL known as UMLILO (Fanucchi et al., 2019). Several trained immune genes share this mechanism. Training mediated by β-glucan upregulates IPLs in a way that depends on the nuclear factor of activated T cells, which epigenetically reprograms immune genes. The Cxcl genes are not trained, and the murine chemokine topologically associating domain is devoid of an IPL. Cxcl genes are trained as a result of the insertion of UMLILO into the chemokine topologically associating domain in murine macrophages (Fanucchi et al., 2019). This offers compelling evidence that the development of trained immunity depends on lncRNA-mediated control. Further research is required to examine these pathways in various experimental contexts, as recent studies have only examined the function of IPLs in β-glucan-induced trained immune characteristics.
2.3 Modulation of myelopoiesis progenitors
The observation of trained circulating monocytes months after BCG vaccination suggests that adaptive processes induced by trained immunity involve alterations in hematopoietic progenitors at the bone marrow level (Kleinnijenhuis et al., 2012). Evidence shows that trained immunity plays a role at the bone marrow level in the context of AS. In mice, the administration of β-glucan leads to long-term transcriptional and metabolic alterations in hematopoietic stem and progenitor cells, resulting in their expansion and bias toward myelopoiesis. This enhances their ability to respond to secondary LPS stimulation and protects them from chemotherapy-induced myelosuppression (Mitroulis et al., 2018). The shared β-subunit of the IL-3/GM-CSF receptor, CD131, is linked with enhanced surface expression in this long-term reprogramming. In a mouse model of predisposition to AS, a similar process occurs when hypercholesterolemia induces enhanced myeloid proliferation and inflammation, suggesting a possible role for trained immunity in the context of traditional cardiovascular risk factors (Wang et al., 2014). Existing evidence also supports a link between enhanced glycolysis in myeloid cells and AS. In hypercholesterolemic ApoE−/− mice, leucocytes and HSPCs show enhanced GLUT1-dependent glucose absorption, which is linked to an elevated mitochondrial potential, providing evidence for a role for myeloid cell glycolysis in myelopoiesis and atherogenesis. This suggests that the mitochondria in these cells are fed by the inflow of glycolytic metabolites for OXPHOS and ATP synthesis (Sarrazy et al., 2016).
3 Endogenous triggers of trained immunity
In addition to microbial sources, endogenous molecules, such as cellular metabolites oxLDL, lipoprotein(a), and hyperglycemia, can induce trained immunity (Bekkering et al., 2014; van der Valk et al., 2016; Braza et al., 2018; Edgar et al., 2021). These endogenous triggers play a role in the development of ASCVD (Flores-Gomez et al., 2021). We will discuss the link between these endogenous triggers of trained immunity and atherosclerotic plaque formation in activated monocyte–macrophages (Table 1).
3.1 oxLDL
oxLDL is a modified lipoprotein and is one of the key atherogenic molecules within plaques that activates immune cells (Moore and Tabas, 2011). oxLDL-trained macrophages exhibit significant metabolic and epigenetic rewiring, similar to BCG and β-glucan. The mammalian target of the rapamycin (mTOR)/HIF1-α signaling pathway is necessary for the upregulation of glycolysis and OXPHOS in oxLDL-induced cells (Keating et al., 2020). The increase in glycolysis and the proinflammatory phenotype in macrophages were avoided by pharmacological suppression of the mTOR pathway and the signaling molecules involved and by inhibiting glycolysis with 2-deoxyglucose (Sohrabi et al., 2018). Epigenetic reprogramming is another characteristic of oxLDL-trained macrophages. OxLDL interacts with the myeloid cell surface receptor cluster of differentiation 36 (CD36) as a damage-associated molecular pattern (DAMP) (Moore et al., 2013). The internalization and release of oxLDL into the cytoplasm may create cholesterol crystals, which activates the NOD-, LRR-, and pyrin domain-containing protein 3 (NLRP3) inflammasome and releases IL-1β and other proinflammatory cytokines, as well as a protracted inflammatory response (Sheedy et al., 2013). Promoters of genes encoding proinflammatory and proatherogenic cytokines and chemokines, such as IL-6, TNF-α, type A scavenger receptor (SR-A), and CD36, are more likely to have the activating histone modification H3K4me3 20. OxLDL training was fully blocked by pharmacologically inhibiting histone methyltransferases, demonstrating that epigenetic alterations are what actually trained immunity by oxLDL (Bekkering et al., 2014).
3.2 Lipoprotein(a)
Lipoprotein(a) is the main circulating carrier of oxidized phospholipids, which plays an important role in atherogenesis (Boffa and Koschinsky, 2019). Monocytes from healthy donors exposed for 24 h to high lipoprotein(a) extracted from hyperlipidemic patients produced more proinflammatory cytokines during the subsequent 6 days compared to controls. Anti-oxidized phospholipid antibodies reduced the training of monocyte-derived macrophages, demonstrating that oxidized phospholipids are the mediating factor in this process (van der Valk et al., 2016). After Pam3Cys and LPS ex vivo stimulation, monocytes showed an increased ability to generate proinflammatory cytokines, including IL-6 and TNF-α (van der Valk et al., 2016). A recent study has shown that individuals with cardiovascular diseases (CVD) may have their proinflammatory monocyte activation reversed by significantly reducing their lipoprotein(a) levels, demonstrating that at least some of this proinflammatory impact is reversible (Stiekema et al., 2020).
3.3 Hyperglycemia
Hyperglycemia, a cardinal feature of diabetes, exacerbates AS progression, delays plaque regression (Parathath et al., 2011), and increases proinflammatory gene expression and resistance to induction of M2-related gene expression (American Diabetes Association, 2015). Evidence suggests that hyperglycemia induces trained immunity in HSCs and macrophages, significantly exacerbating AS (Edgar et al., 2021). High extracellular glucose stimulated the production of proinflammatory genes and the functional properties that are proatherogenic in macrophages through pathways that depend on glycolysis. These traits were sustained by diabetic mouse bone marrow-derived macrophages even when they were cultivated in physiological glucose, showing hyperglycemia-induced trained immunity. A disease-relevant and enduring kind of trained innate immunity was demonstrated by an increase in aortic root AS following bone marrow transplantation from diabetic mice into (normoglycemic) Ldlr−/− mice. HSCs and macrophages generated from the bone marrow showed a proinflammatory priming effect in diabetes, according to integrated tests for transposase-accessible chromatin, chromatin immunoprecipitation, and RNA sequencing analysis (Edgar et al., 2021). Transcription factors, notably runt-related transcription factor 1 (Runx1), are implicated as mediators of trained immunity (Himes et al., 2005). These in vitro signs of trained immunity brought on by hyperglycemia were eliminated by pharmacological suppression of Runx1.
3.4 Catecholamines
Increased sympathetic nervous system activity leads to proinflammatory leukocytosis in models of chronic psychological stress, stroke, and myocardial infarction (Dutta et al., 2012; Heidt et al., 2014; Courties et al., 2015). The pathways causing inflammatory alterations in disorders with high catecholamine levels can be explained by the fact that catecholamines cause long-lasting proinflammatory modifications in monocytes in vitro and in vivo, indicating well-trained immunity (van der Heijden et al., 2020a). After being restimulated with LPS 6 days later, monocyte-derived macrophages exposed to a relevant quantity of epinephrine/norepinephrine had higher levels of TNF-α and IL-6. Similar to oxLDL, this trained immune phenotype is connected to a higher glycolytic capability and OXPHOS. Studies using pharmacological inhibition demonstrated that the cAMP-protein kinase A pathway and the β-adrenergic receptors 1 and 2 are crucial for catecholamine-induced training (van der Heijden et al., 2020a). Patients who have pheochromocytoma and are regularly exposed to brief bursts of catecholamine production have this proinflammatory monocyte characteristic (Neumann and Young, 2019). Systemic inflammatory symptoms and an increased ex vivo cytokine response in activated monocytes were present in these individuals (Neumann and Young, 2019).
3.5 Aldosterone
Human macrophages deriving from monocytes have a long-lasting proinflammatory phenotype in vitro in response to transiently elevated aldosterone concentrations, which may be a factor in the atherosclerotic condition AS chronic inflammation of the artery wall (van der Heijden et al., 2020d). Aldosterone affects intracellular metabolism by increasing fatty acid synthesis, but it does not influence glycolysis and OXPHOS, as found in oxLDL training (van der Heijden et al., 2020c). Additionally, training by aldosterone is linked to the enrichment of H3K4me3 at the promoters of proinflammatory cytokines, including TNF-α and IL-6, demonstrating that aldosterone trains monocyte-derived macrophages in vitro. However, circulating monocytes are not more capable of producing cytokines in individuals with primary hyperaldosteronism. The macrophages of individuals with primary hyperaldosteronism only express more TNF-α following ex vivo differentiation into macrophages in autologous serum (van der Heijden et al., 2020b). These findings imply that aldosterone differs from the trained immune systems that have been well-established and elaborated by other stimuli.
3.6 Hyperlipidemia
Recent research examined the possibility that a WTD, which is high in fats, sweets, and salt and lacks fiber, might lead to trained immunity (Christ et al., 2018). Circulating monocytes and their myeloid progenitors in AS-prone Ldlr−/− mice were significantly affected by proinflammatory transcriptional and epigenetic reprogramming from this WTD over 4 weeks. Increased inflammatory responses to subsequent innate immunological stimulation were brought on by the food intervention. Even when the mice were shifted to a typical chow diet for an additional 4 weeks, this trained immune phenotype was maintained despite circulating cholesterol levels and systemic inflammatory indicators reverting to normal (Christ et al., 2018).
4 Inhibitors of targeting trained immunity
Pharmacological inhibitors of histone methyltransferases and inhibitors of glycolysis, glutaminolysis, and the mevalonate pathway could restrain trained immunity. Following intraperitoneal injection of β-glucan, the effects of pharmacologically suppressing glutaminolysis and the pathway that produces mevalonate on the formation of trained immunity have been established in mouse models in vivo (Arts et al., 2016a). As a result, it would allow the development of innovative pharmaceutical methods to lower the risk of ASCVD and maybe lessen its negative consequences. In this section, we systematically summarize all reported drugs that suppress trained immunity based on multiple publications.
4.1 Agents that modulate metabolic reprogramming
4.1.1 Wortmannin
The fungus metabolite wortmannin was demonstrated to function as a selective inhibitor of AKT/phosphoinositide 3-kinase (PI3K) (Ui et al., 1995). The intermediate stimulation of the Akt/PI3K pathway is what causes mTOR to become active (Kelley et al., 1999). As stimulation with β-glucan caused a high phosphorylation of Akt, β-glucan was responsible for inducing this signal pathway in monocytes. Additionally, mTOR activation was inhibited as a result of Akt phosphorylation inhibition. Monocyte-trained immunity by β-glucan was suppressed by the Akt inhibitor wortmannin in a dose-dependent manner (Cheng et al., 2014).
4.1.2 Rapamycin
Accumulated mevalonate enhances the AKT-mTOR pathway during the establishment of trained immunity, which then triggers HIF1-α activation and a switch from OXPHOS to glycolysis. This response results in circulating monocytes with a trained immunity phenotype (Bekkering et al., 2018). The inhibition of mTOR with rapamycin prevents mevalonate-induced trained immunity. Additionally, BCG-induced trained immunity and β-glucan-induced trained immunity depend on the development of the histone marks H3K4me3 and H3K9me3, which are inhibited by the pharmacological regulation of rate-limiting glycolysis enzymes with rapamycin 30 88. Although rapamycins potently suppress trained immunity in vitro and T-cell proliferation in vivo, they exert little effect on innate immune cells (Braza et al., 2018).
4.1.3 AICAr
One of the most widely utilized pharmacological AMP-activated protein kinase (AMPK) activity modulators is the nucleoside 5-aminoimidazole-4-carboxamide (AICAr). Early research on AMPK’s function in the physiological control of metabolism and the etiology of cancer was mostly centered on the use of AICAr as an AMPK activator (Višnjić et al., 2021). AICAr produces dose-dependent inhibition of β-glucan-induced trained immunity by indirectly inhibiting mTOR (Cheng et al., 2014).
4.1.4 Metformin
Metformin is extensively used as a first-line therapy for type 2 diabetes and has a high safety profile (McCreight et al., 2016). Metformin acts through AMPK activation and subsequent mTOR inhibition. Metformin completely inhibits the protective effects of mice receiving metformin during and after primary infection with low-inoculum C. albicans, which increases survival during disseminated candidiasis brought on by a primary C. albicans injection. In vitro, metformin suppresses trained immunity induced by β-glucan (Cheng et al., 2014). Metformin also inhibits trained immunity by inhibiting the formation of histone marks, H3K4me3 and H3K9me3, by regulating the rate-limiting enzymes of glycolysis 30 88.
4.1.5 Ascorbate
Ascorbate (vitamin C) is an essential micronutrient in primates and serves as an antioxidant and a cofactor for various enzymatic activities represented by prolyl hydroxylases (Fujii et al., 2022). Because the induction of glycolysis by mTOR is mediated by the activation of HIF1-α and stimulation of glycolytic enzymes and ascorbate inhibits HIF-1α expression, it inhibits training immune in a dose-dependent manner (Cheng et al., 2014).
4.1.6 ZVAD-fmk
Western diet feeding of Ldlr−/− mice induces systemic inflammation, which induces long-lasting trained immunity in myeloid cells (Christ et al., 2018). NLRP3 is a key pathway mediating Western diet-induced trained immunity, and the use of small-molecule inhibitors that block NLRP3 signaling can mitigate its potentially deleterious effects in inflammatory diseases (Christ et al., 2018). ZVAD-fmk (benzyloxycarbonyl-Val-Ala-Asp-fluoromethylketone), a pan-caspase inhibitor, inhibits NLRP3 inflammasome activation in atherosclerotic mice, reducing the accumulation of serum IL-1β and plaque cholesterol crystals (Sheedy et al., 2013).
4.1.7 2-DG
A d-glucose mimic, 2-deoxy-d-glucose (2-DG), inhibits glycolysis by producing and accumulating intracellularly 2-deoxy-d-glucose-6-phosphate (2-DG6P), which then inhibits the activity of hexokinase and glucose-6-phosphate isomerase and results in cell death (Pajak et al., 2019). BCG immunization causes immunometabolic activation and epigenetic reprogramming, whereas 2-DG’s restriction of glycolysis during BCG-induced training cancels out the enhanced cytokine production (Arts et al., 2016b). In addition, the inhibitory effect of 2-DG on glycolysis also inhibits histone methylation and prevents mevalonate-induced trained immunity (Arts et al., 2016b).
4.1.8 3PO
3-(3-Pyridinyl)-1-(4-pyridinyl)-2-propen-1-one (3PO), a small-molecule inhibitor of PFKFB3, inhibits glycolytic flow and is cytostatic to malignant cells (Clem et al., 2008). In cells trained with oxLDL, PFKFB3, a critical rate-limiting enzyme in glycolysis, is increased. The in vitro training protocol’s dose-dependent attenuation of the oxLDL-augmented production of TNF-α and IL-6 upon subsequent stimulation with LPS was achieved by co-incubating 3PO with oxLDL for the first 24 h (Clem et al., 2008).
4.1.9 Fluvastatin
Fluvastatin, the first fully synthetic HMG-CoA reductase (HMGCR) inhibitor, is reported to prevent the growth and spread of certain malignancies (Cai and Zhao, 2021). Fluvastatin prevents trained immunity by downregulating H3K4me3 and blocking the production of proinflammatory cytokines (Arts et al., 2016a). Fluvastatin also prevents the enhanced foam cell production brought on by training with oxLDL and stops the epigenetic reprogramming of BCG, β-glucan, and oxLDL-induced trained immunity (Arts et al., 2016a). Additionally, following OxLDL-induced trained immunity, scavenger receptor CD36 and SR-A mRNA expression increase, whereas cholesterol efflux transporter ATP binding cassette transporter A1 (ABCA1) and ATP binding cassette transporter G1 (ABCG1) decrease. These effects may be reversed by adding fluvastatin (Bekkering et al., 2018).
4.1.10 Cerulenin
Cerulenin is a potent and specific inhibitor of type II FAS found in various bacteria and mammalian tissues (Tomoda et al., 1984). It is an antifungal antibiotic discovered in a culture filtrate of Cephalosporium caerulens (Porrini et al., 2014). Aldosterone levels above normal are linked to a higher risk of CVD in people and the induction of trained immunity in primary human monocytes (van der Heijden et al., 2019). Aldosterone’s trained immunity was reduced when cells were pre-incubated with the fatty acid synthesis inhibitor cerulenin for 1 h before re-stimulating with P3C (van der Heijden et al., 2019).
4.2 Agents that modulate epigenetic reprogramming
4.2.1 Ro5-3335
Extracellular glucose promotes macrophage-trained immunity and induces a pro-atherogenic phenotype through a glycolysis-dependent pathway. Runx1, which mediates trained immunity produced by hyperglycemia, is implicated by the pattern of open chromatin (Edgar et al., 2021). A benzodiazepine identified from the screen, Ro5-3335, has a direct interaction with Runx1 (Cunningham et al., 2012). In vitro hyperglycemia-induced trained immunity was reversed by pharmacological suppression of Runx1 with Ro5-3335 4.
4.2.2 MTA
The histone methyltransferase inhibitor 5′-deoxy-5′-methylthioadenosine (MTA) is a non-selective methyltransferase inhibitor. OxLDL causes monocytes to develop a proinflammatory phenotype that persists over time and speeds up AS. MTA completely reverses the methylation of histones, which is required for the change in chromatin architecture that results in increased gene transcription, and thus completely reverses the trained immunity phenotype induced by oxLDL (Bekkering et al., 2014).
4.2.3 Resveratrol
Sirtuin 1 is a nicotinamide adenine dinucleotide (NAD+)-dependent protein deacetylase and master metabolic regulator (Deng et al., 2019). Phytochemical resveratrol, which is abundant in the skin of red grapes and wine, has been studied extensively for its ability to stimulate Sirtuin 1 activity (Lee et al., 2019). Given that histone acetylation is necessary for β-glucan-induced trained immunity, trained immunity in the presence of the histone deacetylase activator resveratrol prevented trained SHIP-1-deficient macrophages from producing more TNF-α (Saz-Leal et al., 2018).
4.2.4 EGCG
The compound epigallocatechin-3-gallate (EGCG) has been discovered to be a new histone acetyltransferase inhibitor (HATi) with broad specificity for the majority of HAT enzymes (Choi et al., 2009). EGCG can also inhibit trained immunity that relies on β-glucan-induced epigenetic reprogramming (Ifrim et al., 2014).
5 Antiatherosclerotic herbal medicine potentially targeting trained immunity
The notion that trained monocytes/macrophages exhibit a broad range of pro-atherogenic phenotypes, including increased production of cytokines/chemokines and foam cells, has recently been extensively supported experimentally (Leentjens et al., 2018). Trained immunity occurs not only in circulating monocytes but also in myeloid progenitors, ensuring a long-term state of hyperactivation of innate immune cells. Trained immunity is mediated by metabolic and epigenetic reprogramming at the level of histone methylation. Theoretically, these processes are amenable to pharmacological intervention. In the past few decades, more studies have shown that various naturally occurring anti-atherogenic natural products, such as flavonoids, phenols, terpenoids, carotenoids, phenylpropanoids, and alkaloids, may be involved in the regulation of pharmacological targets of trained immunity (Supplementary Table S2). We systematically summarize all relevant literature to investigate all potential natural products against trained immunity in ASCVD.
5.1 Flavonoids
Flavonoids are a group of secondary plant metabolites often employed by vegetables for growth and microbial defense (Izzo et al., 2020). Flavonoids can be further classified as flavones, flavonols, flavanones, isoflavonoids, anthocyanins, flavanols, or catechins based on structural distinctions (Kumar and Pandey, 2013). Due to their antioxidant, anti-inflammatory, anti-mutagenic, anti-aging, cardioprotective, antiviral/bacterial, and anticarcinogenic qualities and their ability to influence enzyme performance, they are linked to a variety of positive health impacts. Flavonoids are thought to mediate epigenetic changes, including DNA methylation, histone modifications, and non-coding RNAs (Fatima et al., 2021). We examine some significant natural products that may target monocyte/macrophage and trained immunity in AS in this section.
5.1.1 Alpinetin
Alpinetin (7-hydroxy-5-methoxyflavanone), a flavonoid, is the main active component of Alpinia katsumadai Hayata, a traditional medicinal plant. It engages in various biological processes that affect the NF-κB, MAPK, and PI3K signaling pathways, such as antibacterial, anti-ROS, anticancer, and anti-inflammatory actions (Huo et al., 2012; Wu et al., 2020a; Zhang et al., 2020). The inhibition of the NLRP3 inflammasome may be one way of suppressing trained immunity (Christ et al., 2018). Mechanistically, alpinetin inhibits NLRP3-mediated anti-inflammatory activity and reduces mitochondrial ROS production and HIF-1α transcription, thereby inhibiting HIF-1α signaling (Zhang et al., 2020; Zhu et al., 2021). The expression of the toll-like receptor 4 (TLR4) stimulated by LPS may be dramatically downregulated by alpinetin; alpinetin was reported to have had an anti-inflammatory impact by preventing the production of TNF-α, IL-6, and IL-1β in LPS-stimulated human macrophages (Hu et al., 2013).
5.1.2 Anthocyanins
Anthocyanins are water-soluble glycosides of polyhydroxyl and polymethoxyl derivatives of 2-phenylbenzopyrylium or flavylium salts and are partially responsible for the pigmentation of berries (Azzini et al., 2017). The major anthocyanins in plant foods are glycoside forms of anthocyanidins, including pelargonidin, cyanidin, delphinidin, peonidin, petunidin, and malvidin (Khoo et al., 2017). The bioavailability of anthocyanins is higher than previously thought because the parent compounds are immediately absorbed and converted to bioactive metabolites that remain in circulation (Scalbert et al., 2005; Czank et al., 2013). Anthocyanins increase total antioxidant capacity, antioxidant defense enzymes, and high-density lipoprotein (HDL) antioxidant properties in preclinical and clinical populations through multiple measures, thereby reducing CVD risk factors and mortality in patients with coronary heart disease (Garcia and Blesso, 2021). An essential mediator of trained immunity, the NLRP3-caspase-1 inflammasome, is directly activated upstream by ROS, which is an important mediator of trained immunity (Sun et al., 2020). Preclinical research suggests that anthocyanidins regulate cellular cholesterol efflux from macrophages, hepatic paraoxonase 1 expression, and activity to affect reverse cholesterol transport (RCT) and HDL function beyond simple HDL cholesterol content (Millar et al., 2017). In human populations (such as those who are hyperlipidemic, hypertensive, or diabetic), dietary anthocyanin intake is linked to positive changes in serum biomarkers related to HDL function. These changes include an increase in HDL cholesterol concentration and HDL antioxidant and cholesterol efflux capacities (Millar et al., 2017).
The powdered wild blueberry (Vaccinium angustifolium) component high in anthocyanins also reduced lipid buildup in macrophages generated from THP-1 (Del Bo et al., 2016). In accordance with additional studies, the black rice anthocyanin-rich extract blocked the generation of oxLDL and decreased total cholesterol (TC) and LDL-cholesterol (LDL-C) while boosting the amount of HDL-cholesterol (HDL-C) in serum from rats and ApoE−/− mice. In order to lower the risk of an embolism, it also decreased the area of atherosclerotic plaque and improved the stability of the plaque (Xia et al., 2006). In hypercholesterolemic rabbits, fatty streak development and lipid metabolism were slightly influenced by pomegranate peel extract containing anthocyanins (Sharifiyan et al., 2016). This evidence suggests that the potential of anthocyanins to regulate inflammation, lipid buildup, and macrophage may play a role in how an anthocyanin-rich diet lowers the risk of developing CVD.
5.1.2.1 Cyanidin-3-O-β-glucoside
Cyanidin-3-O-glucoside (C3G) is the anthocyanin with the greatest abundance. C3G is abundantly found in fresh fruits, including grapes, berries, blood oranges, peaches, and apples, and in beverages and colored cereals, such as purple rice and maize (Fang, 2015). One investigation found that methylated proteins, particularly H3K4, lose mono- or dimethyl groups when exposed to C3G or its metabolites, which block the enzyme lysine-specific demethylase 1, which controls histone methylation (Abdulla et al., 2013), thereby directly affecting histone-modifying enzymes (Persico et al., 2021). The results reported here show how dietary C3G intake may effectively control H3K4me3 in the mouse liver, especially in promoter areas (Persico et al., 2021). Recent research in a rat model of high-fat diet (HFD)-induced AS examined the antiatherosclerotic potential of C3G. The findings demonstrated that adding 150 mg/kg of C3G to the diet significantly reduced body weight, visceral adiposity, TG, TC, free fatty acids, and AS index (Um et al., 2013). C3G protected ApoE−/− mice against endothelial dysfunction and AS brought on by hypercholesterolemia by preventing the buildup of cholesterol and 7-oxysterol in the aorta (Wang et al., 2012).
5.1.3 Baicalin
Baicalin is a flavonoid active ingredient extracted from the roots of Scutellaria baicalensis Georgi, a plant used for many years in Chinese traditional medicine to treat various inflammatory illnesses (Liu and Liu, 2017; Riham et al., 2019). One study showed that baicalin could control metabolic diseases in vivo. The therapy with baicalin in HFD rats markedly improved fasting blood glucose levels (Guo et al., 2009). Furthermore, baicalin is reported to inactivate succinate dehydrogenase (SDH) to inhibit ROS production and protect glutamine synthetase (GS) protein stability from oxidative stress to improve glutamate handling and reduce excitotoxicity (Song et al., 2020a).
5.1.4 Chrysin
Chrysin (5,7-dihydroxyflavone) is a flavonoid that naturally occurs in food and is frequently found in honey and propolis, among other plant extracts (Song et al., 2020b). Chrysin possesses various biological qualities, including anti-inflammatory, anti-bacterial, antidiabetic, anticancer, antioxidant, and anti-allergenic actions (Kasala et al., 2015; Mani and Natesan, 2018). One study showed that chrysin has a good expansion effect on human HSCs due to its antioxidant properties by delaying HSC differentiation, inhibiting ROS-activated apoptosis, and regulating cyclin-dependent kinase inhibitors, which can maintain the self-renewal and multilineage differentiation potential of human HSCs (Litviňuková et al., 2020). Chrysin is an emerging histone deacetylase inhibitor for epigenetic regulation in cancer studies (Ganai et al., 2021).
Chrysin may reduce inflammation by modulating M1/M2 status. It promotes the anti-inflammatory M2 phenotype and suppresses the M1 phenotype in peritoneal and cultured macrophages in vitro by activating PPAR-γ (Feng et al., 2014). One study showed that chrysin inhibited NLRP3 inflammasome activation and increased IL-1β levels to reduce synovitis (Liao et al., 2020). Another study showed that chrysin inhibits ROS-mediated Akt/mTOR signaling in cells and induces autophagy (He et al., 2021). The study showed that the overexpression of PPARγ, liver X receptor (LXR)α, ABCA1, and ABCG1 expression led to a considerable increase in HDL-mediated RAW264.7 macrophage cholesterol efflux following chrysin treatment (Lin et al., 2015b).
5.1.5 Daidzein
Daidzein, a substance mostly present in soy foods and plants such as red clover, is one of the most studied and potent phytoestrogens (Yang et al., 2012). Studies in non-human primates have shown that dietary intake of soy protein can interfere with related epigenetic changes that may influence the etiology of complex diseases (Howard et al., 2011). By stimulating the PPARγ-LXRα-ABCA1 pathway, daidzein protected low-density lipoprotein (LDL) from oxidation and increased paraoxonase-1 (PON-1) activity in Huh7 cells, which may control cholesterol efflux (Schrader et al., 2012; Ikhlef et al., 2016). Furthermore, daidzein therapy decreased blood cholesterol and increased TG levels in middle-aged male rats given HFD designed to induce AS (Sosić-Jurjević et al., 2007). These suggest that daidzein has antiatherosclerotic potential.
5.1.6 Ellagic acid
Ellagic acid (EA) is a dilactone of hexahydroxydiphenic acid that may be found in various nuts, fruits, and vegetables, such as pomegranates, walnuts, black raspberries, raspberries, almonds, and strawberries (Galano et al., 2014). According to in vitro, in vivo, and clinical investigations, it has a wide range of physiological actions, including anti-inflammatory, antioxidant, antibacterial, anticarcinogenic, antiplasmodial, antiviral, hepatoprotective, antifibrotic, immunomodulatory, and neuroprotective activities (Gupta et al., 2021). A study showed that EA promotes hematopoietic progenitor cell proliferation and megakaryocyte differentiation (Gao et al., 2014). Other studies have shown that EA interrupts the sequential histone remodeling steps of adipocyte differentiation by reducing the coactivator-associated arginine methyltransferase 1 (CARM1) activity, including histone acetylation and dissociation of HDAC9 from chromatin (Kang et al., 2014). Pomegranate peel polyphenols, in particular pomegranate ellagic acid (PEA), also boosted ApoA1-mediated macrophage cholesterol efflux by upregulating ABCA1 and LXRα and inhibited macrophage lipid buildup by lowering the expression of CD36 (Zhao et al., 2016).
5.1.7 EGCG
EGCG, a typical polyphenol flavonoid molecule with eight free hydroxyl groups, is the most common (Chakrawarti et al., 2016). Research has revealed that EGCG has antibacterial, antiviral, antioxidant, anti-arteriosclerosis, anti-thrombosis, anti-vascular proliferation, anti-inflammatory, and anti-tumor activities (Liu and Yan, 2019). As a histone acetyltransferase inhibitor, EGCG can significantly inhibit the training of monocytes (Ifrim et al., 2014). In different research, EGCG-loaded nanoparticles targeted macrophages via their CD36 receptor, reduced the release of inflammatory factors by mouse peritoneal macrophages, and reduced the lesion surface area of arterial plaques in LDLr−/− mice (Zhang et al., 2019). EGCG also inhibited the oxLDL-induced overexpression of SR-A in the same cell line, reducing oxLDL absorption and the formation of foam cells (Chen et al., 2017). EGCG regulated macrophage polarization toward the M2 state. EGCG decreased the expression of proinflammatory M1 mediators, iNOS, TNF-α, IL-1β, and IL-6, in the LPS-administered lung microenvironment and increased the expression of KLF4, Arg1, and ym1, which enhanced the M2 phenotype of macrophages (Almatroodi et al., 2020).
5.1.8 Hesperidin
Hesperidin (3′,5,7,-trihydroxy-4′-methoxyflavanone), a flavanone family of flavonoids, is a derivative of hesperetin, which is present in citrus fruits, such as oranges and grapefruit (Muhammad et al., 2019). Hesperidin has several pharmacological effects, with the main ones being the stimulation of antioxidation, the inhibition of the generation of proinflammatory cytokines, and the inhibition of the proliferation of cancer cells (Li and Schluesener, 2017). A study using metabolic tracing studies showed that TLR signaling in mouse and human macrophages redirects metabolic flux to increase acetyl-CoA for glucose production, thereby enhancing histone acetylation (Christ and Latz, 2019). According to a preclinical study, hesperetin provided neuroprotection by controlling the TLR4/NF-κB signaling pathway in response to the harmful effects of LPS (Muhammad et al., 2019). Hesperetin decreased the generation of foam cells produced from THP-1 by promoting ABCA1 expression by boosting the activities of the ABCA1 promoter and LXR enhancer, upregulating the ApoA1-mediated cholesterol efflux (Iio et al., 2012).
5.1.9 Icariin
Icariin, one of the primary ingredients in epimedium, is an 8-isopentane flavonoside that has several pharmacological benefits, including enhancement of cardiovascular function, promotion of hematological function, prevention of neuronal damage, and anti-osteoporosis properties (El-Shitany and Eid, 2019). Icariin decreased RAW264.7 macrophage infiltration at atherosclerotic lesions by reducing the CX3CR1–CX3CL1 interaction, which is directly related to monocyte adhesion and migration (Wang et al., 2016a).
5.1.10 Pratensein
Pratensein is a compound extracted from Radix Polygala roots. It has anti-inflammatory, anti-apoptotic, and antioxidant effects (Liu et al., 2016b). Pratensein increases the expression of the ABCA1 protein and HDL levels in HepG2 cells (Gao et al., 2008). AS is brought on by passive LDL transport across damaged endothelial cells. Recent research has revealed a novel therapeutic target in the fight against AS: scavenger receptor class B type I (SR-BI)-mediated endothelial LDL transcytosis. This process increases LDL entry into the arterial wall and the development of AS (Huang et al., 2019). Further investigation found that pratensein increased the expression of CLA-1, a human homolog of SR-BI, indicating that it may have some bearing on the in vitro process of cholesterol efflux (Yang et al., 2009).
5.1.11 Puerarin
Puerarin, an isoflavone component extracted from the herb Radix Puerariae, is often employed in China to treat inflammatory and immunological disorders (Yang et al., 2021). Its powerful pharmacological effects are a result of the compounds’ many bioactivities. Puerarin’s anti-inflammatory processes, which include the control of important signals, including TLR, Nrf2, HDAC, and PPARα, and the enhancement of organelle function (Ni et al., 2020; Niu et al., 2020; Chen et al., 2021), have been thoroughly investigated in recent years (Chang et al., 2021). An earlier study investigated the epigenetic mechanism through which puerarin suppresses MCP-1 production using high-glucose circumstances. It was shown that puerarin dramatically reduced high glucose’s ability to upregulate H3K4 di- and tri-methylation (H3K4me2/3) on the MCP-1 gene promoter, suggesting that it may be useful in treating diabetes-related vascular damage (Han et al., 2015). Puerarin promoted ABCA1-mediated cholesterol efflux via pathways involving miRNA-7, serine/threonine kinase 11 (STK11), and the AMPK-PPARγ-LXRα-ABCA1 cascade, therefore reducing cellular lipid buildup in THP-1 macrophages (Li et al., 2017a).
5.1.12 Quercetin
Quercetin (3,3′,4′,5,7-pentahydroxyflavone) is one of the most prevalent plant flavonoids and a key dietary antioxidant in the human diet (Boots et al., 2008). It can be found in various traditional Chinese herbal medicines, tea, fruit, and other vegetables and has also been proven effective in clinical studies (Ferry et al., 1996). The antioxidant, anti-inflammatory, antiviral, anticancer, and antifibrotic effects of quercetin should be preserved (Russo et al., 2012). Moreover, quercetin stimulates autophagy in the hematopoietic stem/progenitor cell compartment of myelodysplastic bone marrow (Daw and Law, 2021). Qu also promotes apoptosis through DNA demethylation activity, HDAC inhibition, and enrichment of H3ac and H4ac in the promoter regions of genes that enhance apoptotic pathways (Alvarez et al., 2018).
In vitro studies have shown that quercetin can inhibit two stages of macrophage differentiation and polarization: macrophage infiltration (from monocytes to macrophages) and macrophage subtype conversion (from M2 to M1 subtypes). Quercetin downregulated the expression of M1 macrophage markers and proinflammatory cytokines and upregulated the expression of M2 macrophage markers and anti-inflammatory cytokines in BMDM under both basal and LPS-stimulated conditions (Dong et al., 2014b). Jia et al. (2019) demonstrated that in apoE−/− mice fed with HFD, quercetin protects against AS by regulating the expression of proprotein convertase subtilisin/kexin type 9 (PCSK9), CD36, PPARγ, LXRα, and ABCA1. In THP-1-derived foam cells, quercetin increased ApoA1-mediated cholesterol efflux and promoted ABCA1 and PPARγ expression by activating PPARγ signaling (Sun et al., 2015). Quercetin has also been linked to reduced AS in ApoE−/− mice by enhancing RCT, which depends on ABCA1 and ABCG1 (Cui et al., 2017).
5.1.13 Silymarin
Silymarin is extracted from the seeds of Silybum marianum L. Gaertn. (also known as milk thistle). Silymarin is a blend of flavonoids, primarily silybin, silydianin, silychristin, and other active components (Rašković et al., 2011). In addition to protecting the liver and lowering enzymes and lipids, this combination has antioxidant, anti-inflammatory, and anticancer properties (Zhao et al., 2021). Studies have shown that silibinin may interfere with epigenetic cellular mechanisms, including increasing the total DNMT activity, while reducing histone deacetylase (HDAC) expression levels (Anestopoulos et al., 2016). The silymarin compounds, isosilybin A, isosilybin B, silychristin, and isosilychristin, increased ABCA1 protein expression in THP-1 cells. Due to its PPARγ activating qualities, isosilybin A, in particular, enhanced cholesterol efflux from THP-1 macrophages (Wang et al., 2015b).
5.2 Phenols
Phenolic chemicals are found all over the plant world and have more than 8,000 distinct known structures. Phenols can be classified as monophenols, binary phenols, or polyphenols, depending on the number of phenolic hydroxyl groups in the chemical structure (Xiao et al., 2012). Through various mechanisms, phenolic compounds have various pharmacological and biological actions. These activities include the control of various cell signaling pathways, gene expression, and antioxidation.
5.2.1 Curcumin
Curcumin ((1E, 6E)-1,7-bis(4-hydroxy-3-methoxyphenyl)-1,6-heptadiene-3,5-dione) is a polyphenolic derivative produced from turmeric (Curcuma longa) (Wu et al., 2020b). Curcumin can control inflammation in in vitro and in vivo studies. This property makes curcumin an effective treatment for various inflammatory disorders, including obesity, diabetes, CVD, bronchial asthma, and rheumatoid arthritis (Chen et al., 2019a). Studies have demonstrated a direct inhibitory effect of curcumin on NLRP3 inflammasome activation in macrophages, which can prevent HFD-induced insulin resistance and inhibit LPS-priming and NLRP3 inflammasome activation pathways in macrophages (Yin et al., 2018). Curcumin may also act as an epigenetic regulator, including the inhibition of DNMTs, regulation of histone modifications via the regulation of histone acetyltransferases (HATs) and HDACs, regulation of miRNA, action as a DNA-binding agent, and interaction with transcription factors (Hassan et al., 2019). Additionally, c-Jun N-terminal kinases (JNK), histone methyltransferase p300, and transcriptional factor activating protein-1 (AP-1) were all inhibited by curcumin (Huwait et al., 2011).
In terms of regulating M1/M2 macrophages, curcumin can enhance the secretion of M2 macrophage markers, such as macrophage mannose receptor (MMR), Arg-1, PPAR-γ, IL-4, and/or IL-13. These effects have been observed in experimental autoimmune myocarditis (EAM) models and hyaline membrane disease, where curcumin polarizes M0 and M1 macrophages to an M2 phenotype (Saqib et al., 2018). A previous study indicated that without having a sizable impact on other cholesterol transporters, curcumin dramatically reduced the oxLDL-induced lipid buildup in J774.A1 macrophages by reducing the SR-A-dependent oxLDL uptake and enhancing the ABCA1-dependent cholesterol efflux (Zhao et al., 2012). The evidence suggests that curcumin inhibits the production of SR-A through a ubiquitin/proteasome mechanism. Additionally, through LXRα-dependent transcriptional regulation, curcumin increased ABCA1 expression (Zhao et al., 2012).
5.2.2 Paeonol
Paeonol is an active component of the Chinese herbal remedy Moutan Cortex (Pae, 2-hydroxy-4-methoxyacetophenone), which is obtained from the root bark (Chen et al., 2019b). Pae has several physiologic benefits, including anti-inflammatory and antioxidant properties (Mei et al., 2019). LncRNAs are RNA molecules longer than 200 nucleotides interacting with target genes at the transcriptional level. They function as competing endogenous RNA (ceRNA) sponges to control mRNA expression and promote cisplatin-induced nephrotoxicity by regulating AKT/TSC/mTOR-mediated autophagy (Jing et al., 2021). Pae can inhibit the expression of lnc-MEG3 to alleviate renal injury in mice (Jing et al., 2021).
In ApoE−/− mice, Pae therapy decreased the development of atherosclerotic lesions, slowed systemic inflammation, and enhanced ABCA1 expression (Zhao et al., 2013b).
5.2.3 Polydatin
The main active ingredient of Polygonum cuspidatum Sieb. et Zucc. (Polygonaceae), a plant widely used in traditional medicine worldwide, particularly in China and Japan, is polydatin (Du et al., 2013). Polydatin clearly possesses hypoglycemic, antiatherosclerotic, hypolipidemic, hypouricemic, and anti-inflammatory effects, according to the findings of the literature review (Luo et al., 2022). In oxLDL-stimulated ApoE−/− mouse macrophages, a 48 h polydatin therapy decreased TC, FC, and CE levels and TNF-α and IL-1β production. The mechanism generating these effects is linked to the stimulation of PPARγ-dependent ABCA1 overexpression and the decrease in CD36 expression (Wu et al., 2015a).
5.2.4 Protocatechuic acid
In vegetables, fruits, and rice, protocatechuic acid (PCA, 3,4-dihydroxybenzoic acid) has been demonstrated to enhance vasodilation in apolipoprotein E-deficient rats with AS via the eNOS-mediated endothelium-dependent pathway (Bai et al., 2021). PCA may also control lipid metabolism by inhibiting the expression of HMG-CoA reductase (Liu et al., 2010). PCA also prevent ED intercellular adhesion molecule 1 (ICAM-1) and vascular cell adhesion molecule 1 (VCAM-1)-dependent monocyte adherence to activated HUVECs as well as CCL2-mediated monocyte transmigration, inhibiting the effects of cholesterol metabolism in addition to decreasing the progression of AS in ApoE−/− mice (Wang et al., 2010b; Stumpf et al., 2013).
5.2.5 Resveratrol
Resveratrol (RV), the most studied stilbene structure, is present in typical food sources such as grapes, berries, peanuts, and red wine, and, in some herbs, it is regarded as a strong antioxidant, among other properties (Sarubbo et al., 2017). In fact, research has identified several RV advantageous properties, making it possible for it to play crucial roles in the treatment of diseases including cancer, CVD, and AD, as well as other degenerative brain illnesses (Choi et al., 2012). Recent evidence suggests that the beneficial effects of RV may be related to altered epigenetic mechanisms. After being taken orally, RV can cause several chemical modifications, including oxidation, dehydroxylation, and demethylation, which can either directly inhibit the activity of epigenetic enzymes such as DNMTs, HDACs, or HATs or change the amount of substrate that is available for those enzymatic reactions (Griñán-Ferré et al., 2021). Since β-glucan-induced trained immunity depends on histone acetylation, under the action of the histone deacetylase activator RV (a sirtuin 1 activator), trained immunity is significantly inhibited and partially suppressed enhancement of IL-6 production (Cheng et al., 2014).
Regarding the effect on macrophages, RV can reverse the oxysterol-induced M2/M1 phenotypic switch (Buttari et al., 2014). RV regulates microglial M1/M2 polarization through PGC-1α under neuroinflammatory injury. Similar studies have shown that malibatol A (MA), an oligomer of RV, inhibits the expression of proinflammatory cytokines and M1 markers (CD16, CD32, and CD86) while increasing M2 in LPS-stimulated microglia markers (CD206 and YM-1) (Pan et al., 2015). RV has the atherosclerotic protective mechanism by regulating monocyte/macrophage differentiation, among other mechanisms, including inhibition of LDL oxidation, enhanced endothelial protection, reduction of trimethylamine N-oxide (TMAO) by gut flora, and inhibition of vascular smooth muscle cell (VSMC) proliferation and migration (Vasamsetti et al., 2016). RV suppressed LPS-induced RAW264.7 foam cell development by lowering ROS production and MCP1 expression through the Akt/Foxo3a and AMPK/Sirt1 pathways, which rely on NADPH oxidase 1 (Nox1) (Park et al., 2009; Dong et al., 2014a). According to clinical research, RV decreases the amount of TC and TG in individuals with dyslipidemia (Simental-Mendía and Guerrero-Romero, 2019).
5.2.6 Salicylic acid
Radix Salvia miltiorrhiza (Danshen), which produces salvianolic acid B (SalB), has several medicinal actions, including antioxidant, anticancer, anti-inflammatory, and antiatherosclerotic qualities (Tang et al., 2022). Research showed that SalB induced the production of ABCA1 in differentiated THP-1 macrophages, which helped the HDL and ApoA-1-mediated cholesterol export. Additional mechanism experiments revealed that PPARγ and LXRα inhibitors might decrease the overexpression of ABCA1 triggered by SalB, which suggested that SalB promoted cholesterol efflux through a PPARγ/LXRα/ABCA1-dependent mechanism in THP-1 macrophages to minimize lipid buildup (Yue et al., 2015). Salvianolic acid B was discovered using a high-throughput screening experiment to be a powerful CD36 antagonist that prevents oxLDL absorption in RAW264.7 macrophages (Wang et al., 2010c).
5.3 Terpenoids
Terpenoids, also known as isoprenoids, are isoprene-based natural compounds having critical functions in every organism’s metabolism (Bergman et al., 2019). The terpenoid family of natural compounds, which includes several plant terpenoids, has been a valuable source of medicinal discoveries.
5.3.1 Betulinic acid
Betulinic acid (BA), a natural pentacyclic triterpenoid, is an active compound in the bark of the birch tree Betula spp. (Betulaceae). BA has many biological effects, including anti-inflammatory, antiviral, antioxidant, and anticancer properties (Appiah et al., 2018). Research found that betulinic acid reduced atherosclerotic lesions, TG, TC, and LDL-C levels in ApoE−/− mice by blocking the NF-κB signaling pathway and miR-33 expression (Zhao et al., 2013c). In RAW264.7 and THP-1 cells, betulin (a derivative of betulinic acid) consistently improved ABCA1/ABCG1-mediated cholesterol efflux by preventing the synthesis of SREBPs, which bound to E-box motifs in the ABCA1 promoter (Gui et al., 2016).
5.3.2 Ginsenosides
The main active compounds in ginseng are called ginsenosides, which are triterpene glycosides of the dammarane type (Nah et al., 2007). Ginsenosides are expressed by the formula Rx, where x represents the separation from the thin-layer chromatography origin (Kim et al., 2017). The segments are labeled A for the most polar and H for the least polar (Kim et al., 2017). Protopanaxadiols, protopanaxatriols, and oleanane are the three main families of ginsenosides (ginsenoside Ro). Ginsenosides Rb1, Rb2, Rb3, Rc, Rd, Rg3, and Rh3 are examples of protopanaxadiols, which include sugar moieties on the C-3 position of dammarane-type triterpenes. Ginsenosides Re, Rf, Rg1, Rg2, and Rh1 are examples of the sugar moieties on the C-6 position dammarane-type triterpenes that make up protopanaxatriol (Lü et al., 2009; Kim et al., 2018). Ginseng is a well-liked supplement due to its wide range of pharmacological and therapeutic effects on aging, cancer, the cardiovascular system, diabetes, immune-regulatory function, and inflammation (Im, 2020). Ginsenoside Rg1 exerts positive effects on mesenchymal stem cells (MSCs). Ginsenoside Rg1 can influence HSC proliferation and migration, control HSCs/hematopoietic progenitor cell (HPC) differentiation, and slow down HSC aging. These findings may offer new approaches for increasing the homing rate of HSCs during HSC transplantation and for the treatment of graft-versus-host disease (GVHD) and other diseases caused by HSCs/HPC dysplasia (He and Yao, 2021).
Regarding the effect on macrophage polarization, ginsenoside Rg3 showed a positive effect on M2 polarization. After treatment with LPS, isolated mouse peritoneal macrophages significantly expressed several M1 marker genes, such as COX-2 (cyclooxygenase), iNOS, IL-1β, and TNFα. Pretreatment with Rg3 successfully restored a representative M2 marker (arginase-1), which was reduced after treatment with LPS (Koh et al., 2018). Ginsenoside Rg3 significantly reduced ox-LDL-induced atherosclerotic pathological changes in ApoE−/− mice fed with HFD, upregulated PPARγ, and inhibited the activation of focal adhesion kinase (FAK) in the aorta, thus inhibiting the expression of VCAM-1 and ICAM-1 in the intima (Geng et al., 2020). Another crucial component of ginseng is ginsenoside Rd. Data from a RAW264.7 cell model showed that Rd inhibited the expression of the SR-A protein, followed by a decrease in the uptake of oxLDL and in the amount of cholesterol inside the cell (Li et al., 2011). An in vivo investigation revealed that Rd administration decreased the atherosclerotic plaque areas and oxLDL absorption in ApoE−/− mice.
5.3.3 Tanshinone IIA
Extracted from Salvia miltiorrhiza Bunge, tanshinone IIA (Tan IIA) is a significant lipophilic diterpene (Ono, 2018). Tan IIA can prevent or decrease the advancement of several illnesses, including cardiovascular diseases, cancers, cerebrovascular diseases, and Alzheimer’s disease, according to several experimental and clinical studies (Ding et al., 2020). One study demonstrated that TIIA treatment attenuated high glucose-induced kidney damage by modulating the DNA methylation of related genes Nmu, Fgl2, Glo, and Kcnip2 (Li et al., 2019). Tanshinone IIA consistently boosted ERK/Nrf2/HO-1 loop-mediated ABCA1- and ABCG1-mediated cholesterol efflux and decreased SR-A-mediated oxLDL absorption by inhibiting AP-1, which reduced cholesterol buildup in cells (Liu et al., 2014a). Another investigation using peritoneal macrophages from rats and macrophages generated from THP-1 revealed that tanshinone IIA treatment greatly raised ABCA1 mRNA and protein expression while considerably reducing CD36, suggesting simultaneous effects on cholesterol intake and efflux (Jia et al., 2016). In addition, a clinical trial has demonstrated that tanshinone IIA lowers hs-CRP in CAD patients (Li et al., 2017c).
5.3.4 Ursolic acid
Ursolic acid (3B-hydroxy-12-urc-12-en-28-oic acid) is a pentacyclic triterpenoid produced from plants that have antioxidant, anti-inflammatory, and neuroprotective properties (Rong et al., 2022). A study on skin cancer reported that ursolic acid therapy reduces hypermethylated CpG islands of the Nrf2 gene promoter region in mouse epidermal cells, restoring Nrf2 expression, accomplished by lowering the production of epigenetic-modifying enzymes such as DNA methyltransferases (Kim et al., 2016). A recent study found that ursolic acid improved the transport of cholesterol from LDL-loaded macrophages to ApoA-1 through autophagy without changing the levels of ABCA1 and ABCG1 mRNA or protein in MPMs (Leng et al., 2016). In vivo, UA therapy dramatically decreased the size of the atherosclerotic lesion and increased macrophage autophagy in LDLR−/− mice (Leng et al., 2016).
5.3.5 Zerumbone
A naturally occurring substance called zerumbone is derived from pinecone or shampoo ginger, Zingiber zerumbet L. Smith, and contains several pharmacological properties, including antiulcer, antioxidant, anticancer, and antibacterial (Rosa et al., 2019). Earlier research found that zerumbone significantly reduced the inflammatory response caused by LPS in in vitro and ex vivo trials using the macrophages employed in this investigation by inhibiting the activation of the ERK-MAPK and NF-κB signaling pathways and the NLRP3 inflammasome (Su et al., 2021). Studies in a rabbit model fed cholesterol show that zerumbone can stop the development of atherosclerotic lesions (Hemn et al., 2015). Zerumbone reduced the expression of SR-A and CD36 mRNA in vitro studies by controlling AP-1 and NF-κB suppression, which blocked the absorption of acLDL by THP-1 macrophages (Eguchi et al., 2007). Additionally, zerumbone treatment of THP-1 macrophages resulted in a considerable decrease in cholesterol levels through increasing the mRNA and protein levels of ABCA1, but not ABCG1, and ERK1/2 phosphorylation (Zhu and Liu, 2015).
5.4 Carotenoids
Carotenoids represent a class of pigmented terpenoids. The human diet contains around 50 of more than 700 carotenoids identified in nature, with about half present in human blood and tissues (Krinsky and Johnson, 2005). Lycopene, lutein, zeaxanthin, β-cryptoxanthin, α-carotene, and β-carotene are the main carotenoids in human serum (Krinsky and Johnson, 2005). According to epidemiologic research, it may be linked to better cognitive and visual abilities and a lower chance of developing chronic conditions, including cancer, CVD, and age-related macular degeneration (AMD) (Moran et al., 2018).
5.4.1 Astaxanthin
A natural xanthophyll carotenoid called astaxanthin (3,3′-dihydroxy-β,β′-carotene-4,4′-dione) is present in various marine species, such as Haematococcus pluvialis, Chlorella zofingiensis, Chlorococcum, and Phaffia rhodozyma (Hussein et al., 2006). It has been suggested that it has anti-inflammatory, antioxidant, and neuroprotective properties, and research from different experimental models has demonstrated that these properties are linked to a decreased expression of proinflammatory cytokines and a decreased production of ROS and free radicals (Kim et al., 2020). Xue et al. (2017) reported that astaxanthin prevents oxidative stress and apoptosis, which reduces the hematopoietic damage caused by whole-body radiation in mice. Yang et al. (2017) stated that astaxanthin could demethylate certain promoters of particular genes, which may help increase the stability of the total chromatin structure. Only at high dosages does astaxanthin enhance the expression of ABCA1/G1 (up to 2.0- and 3.2-fold at the protein level), which promotes ApoA-1/HDL-mediated cholesterol efflux (Iizuka et al., 2012).
5.4.2 β-Carotene
β-Carotene (BC), a precursor to vitamin A, is present in a greater variety of fruits and vegetables. It is frequently used in foods as an antioxidant and natural colorant (Zhao et al., 2020). Lower overall, CVD, heart disease, stroke, cancer, and other causes of death are linked to a higher β-carotene biochemical state (Huang et al., 2018). Kim et al. (2019) suggested that BC can regulate epigenetic modifications for its anticancer effects in colon cancer stem cells. Furthermore, endogenous β-carotene 15,15′-monooxygenase 1 may convert 9-cis-c into 9-cis retinoic acid or other retinoids, activate the retinoid X receptor (RXR), and stop foam cell formation and the development of AS (Zolberg Relevy et al., 2015).
5.4.3 Lycopene
Lycopene, a member of the carotenoid family, is mostly found in foods such as tomatoes (particularly the red kind), watermelons, and red pomelo (Zhan et al., 2021). Lycopene is widely recognized for its anti-inflammatory and antioxidant properties and ability to affect important bodily metabolic processes (Han et al., 2016). Napolitano et al. (2007) demonstrated that lycopene reduced cholesterol buildup by upregulating IL-10 secretion in human peripheral blood monocyte-derived macrophages (HMDMs) and THP-1 macrophages and downregulating SR-A mRNA expression and lipid synthesis. Furthermore, HMG-CoA reductase inhibition, RhoA inactivation, an increase in PPARγ and LXRα activation, and ultimately an improvement in ABCA1 and caveolin 1 expression may all contribute to the potential cascade impact of lycopene in lowering foam cell formation (Palozza et al., 2011).
5.4.4 Retinoids
Retinol, generally known as vitamin A1, and its natural derivatives, 9-cis retinoic acid (9-cis-RA) and all-trans retinoic acid (ATRA), are thought to be prospective therapeutic agents for the prevention of AS development because they can promote macrophage cholesterol efflux. Retinoids reportedly cause epigenetic alterations that cause stem cell differentiation (Gudas, 2013). Through binding to the RARs, ATRA modifies how the retinoic acid receptors (RARs) interact with various protein elements of the transcription complex at multiple genes in stem cells. The epigenetic marks on histones or DNA are added to or removed by some of these protein components of the transcription complex, altering the chromatin structure and leading to the departure from the self-renewing, pluripotent stem cell state (Gudas, 2013). According to research, 9-cis-RA and ATRA can significantly increase the expression of ABCA1, ABCG1, and ApoE in THP-1 macrophages and the efflux of cholesterol to ApoA-1 in RAW264.7 macrophages (Langmann et al., 2005). Additionally, 9-cis-RA has been connected to the ABCA1-mediated cholesterol efflux from J774 macrophages, THP-1-derived macrophages, and RAW264.7 macrophages (Schwartz et al., 2000; Kiss et al., 2005).
5.5 Phenylpropanoids
5.5.1 Ferulic acid
Ferulic acid (FA) ((E)-3-(4-hydroxy-3-methoxy-phenyl) prop-2-enoic acid), a caffeic acid derivative, can be isolated from several Chinese herbal medicines, including Cimicifuga racemosa, Angelica sinensis, and Rhizoma Ligustici Chuanxiong, as well as from plants that are commonly found in our diet, including Oryza sativa, Glycine max, and Zea mays (Li et al., 2022). FA has free radical scavenging and antioxidant properties that have a wide range of potential applications in the prevention and treatment of CVD and the management of cancer, as well as hepatoprotective, antimicrobial, and anti-inflammatory therapies (Li et al., 2022). FA, a metabolite of chlorogenic acid, has an improving effect on HDL-mediated cholesterol efflux from macrophages by increasing the expression of ABCG1 and SR-BI (Uto-Kondo et al., 2010).
5.5.2 Chlorogenic acid
Chlorogenic acid (CA) is a phenolic molecule from the hydroxycinnamic family found in drinks made from herbs, fruits, and vegetables. It is recognized for its antioxidant capabilities against free radicals (Santana-Gálvez et al., 2017). In ApoE−/− mice fed a diet high in cholesterol, CA decreased the percentage and total atherosclerotic lesion area, as well as the aortic dilatation and serum levels of TC, LDL-C, and TG (Wu et al., 2014). Through the upregulation of the transcription of PPARγ, LXRα, ABCA1, and ABCG1 in in vitro mechanistic studies, CA repressed foam cell growth and decreased the oxLDL-induced neutral lipid and cholesterol accumulation in RAW264.7 macrophages (Wu et al., 2014). In HepG2 cells, chlorogenic acid enhanced mRNA expression of ABCA1, CYP7A1, and AMPKα2 and facilitated the efflux of TC and triacylglycerol (Hao et al., 2016).
5.5.3 Lignans
The largest concentration of lignans, which are bioactive, non-nutritive, non-caloric phenolic plant chemicals, is found in flax and sesame seeds (Peterson et al., 2010). Dietary lignans demonstrate strong antiviral, antioxidant, anticancer, and antiatherosclerotic properties via functioning as phytoestrogens (Peterson et al., 2010).
5.5.3.1 Arctigenin
Across hundreds of years, people all over the world have used the roots of Arctium lappa, also known as larger burdock, as food and traditional herbal medicine. The seeds of the Arctium lappa plant contain arctigenin, a phenylpropanoid dibenzylbutyrolactone lignin (Nam and Nam, 2020). The antibacterial, antiviral, antioxidant, anti-inflammatory, and anticancer properties of arctigenin have been demonstrated (Maxwell et al., 2018). Arctigenin boosted the expression of ApoE, ABCA1, and ABCG1 in oxLDL-loaded THP-1 macrophages, increasing cholesterol efflux (Xu et al., 2013).
5.5.3.2 Honokiol
Honokiol (HKL) [2-(4-hydroxy-3-prop-2-enyl-phenyl)-4-prop-2-enyl-phenol] is a naturally occurring biphenolic chemical with a low molecular weight, which is obtained from the bark of magnolia trees and is utilized in traditional Chinese medicine (Fried and Arbiser, 2009). It possesses analgesic, anti-inflammatory, antioxidant, anti-tumor, and neuroprotective activities as a pharmaceutical (Pillai et al., 2015). Honokiol could activate the RXR/LXR heterodimer, inducing the ABCA1 expression and improving cholesterol efflux from MPMs (Kotani et al., 2010). According to another study, honokiol boosted ABCA1 expression by interacting with RXRβ. Additionally, it boosted the expression of ABCG1 and ApoE (Jung et al., 2010).
5.5.3.3 Sesamin
Sesamin, a naturally occurring lignin compound, is isolated from sesame seeds and has many positive health effects, including anti-inflammatory, anticancer, anti-hypertension, anti-thrombotic, antidiabetic, anti-atherogenic, anti-obesity, and lipolytic effects (Dalibalta et al., 2020). It also can reduce damage to the intestine, kidneys, heart, brain, and liver (Wang et al., 2021b). Studies have shown that sesamin inhibits LPS-induced macrophage-derived chemokine expression through ER, PPAR-α, MAPK-p38 pathway, NFκB-p65 pathway, and epigenetic regulation (Hsieh et al., 2014). Sesamin boosted cholesterol efflux from RAW264.7 macrophages and decreased the oxLDL-induced accumulation of cholesterol, most likely by activating PPARγ, LXRα, and ABCG1 (Liu et al., 2014b). Sesamin reduced AS in ApoE−/− mice by stifling vascular inflammation, according to in vivo research (Wu et al., 2010).
5.6 Alkaloids
5.6.1 Berberine
Berberine (BBR) is a naturally occurring substance extracted from herbs, including Coptis chinensis and Berberis vulgaris. BBR has been identified as a safe and effective treatment for type 2 diabetes and hyperlipidemia with new mechanisms since 2004 (Kong et al., 2004). Over the past 10 years, several studies have demonstrated the clinical effectiveness of BBR in decreasing lipids and glucose (Wang et al., 2021c). The investigation results demonstrated that BBR administration mostly impacted enzymes involved in histone acetylation and methylation (Zhang et al., 2016). The expression of the proteins H3K4me3, H3K27me3, and H3K36me3 reduced after BBR administration, according to Western blotting tests conducted concurrently (Zhang et al., 2016). By encouraging LXRα/ABCA1-dependent cholesterol efflux, BBR reduced the development of foam cells in THP-1 macrophages (Lee et al., 2010). Nevertheless, ABCG1, SR-BI, CD36, and SR-A were unaffected by berberine. The impact of BBR on macrophages is also mediated by other pathways, including AMPK/Sirt1 activation, autophagy induction, and adipocyte enhancer-binding protein 1 suppression (Huang et al., 2012; Chi et al., 2014; Kou et al., 2017). Moreover, in a rat model of adjuvant arthritis, BBR treatment restrained the phagocytic function of macrophages and restored the balance of M1/M2 by reducing the levels of M1 cytokines (TNF-α, IL-1β, and IL-6), increasing the levels of M2 cytokines (IL-10 and TGF-β1), increasing the expression of arginase 1(Arg1) (M2 marker), and decreasing the expression of iNOS (M1 marker) (Zhou et al., 2019).
5.6.2 Piperine
Long and black peppers contain piperine (Srinivasan, 2007). Previous research has demonstrated that piperine has a variety of pharmacological properties. In terms of pharmaceuticals, piperine decreases depressive disorders, prevents hepatotoxicity, and reduces obesity and diabetes (Nogara et al., 2016). According to studies, piperine suppresses the development of adipocytes by dynamically controlling histone modifications and regulating the expression of genes involved in adipogenesis and lipolysis (Park et al., 2019). In THP-1-differentiated human macrophages, piperine was likewise observed to increase ABCA1 protein expression. However, it did not affect ABCG1 or SR-BI expression (Wang et al., 2017a).
5.6.3 Rutaecarpine
Rutaecarpine (8,13-dihydro-7H-indolo-[2′,3′:3.4]-pyrido [2,1-b]-quinazolin-5-one) is an alkaloid first isolated from E. rutaecarpa. Earlier studies demonstrated that rutaecarpine possesses many biological and pharmacological features, including the ability to cause diuresis, sweating, uterotonic action, brain function improvement, antinociception, and anti-obesity (Jayakumar et al., 2021). Studies showed that rutaecarpine increased cholesterol efflux by upregulating the expression of ABCA1 and SR-BI in vitro (RAW264.7 macrophages and HepG2 cells) and in vivo (ApoE−/− mice) (without changing ABCG1 and CD36) (Xu et al., 2014). This reduced the lipid buildup and foam cell formation. Through this method, rutaecarpine decreased the growth of atherosclerotic plaque in ApoE−/− mice (Xu et al., 2014).
5.7 Others
5.7.1 Astragalus polysaccharides
The primary active ingredient of Astragalus membranaceus, Astragalus polysaccharides (APS), is widely used in clinical applications as an immunomodulator (Li et al., 2012). It has several bio-activities, including anti-inflammatory, proliferative, and immune-regulating effects, and a molecular weight of 3.6 × 104 Da (Sun et al., 2021a). Studies have demonstrated that APS significantly abrogates LPS-induced IL-6 levels in THP-1 macrophages (Long et al., 2022). ABCA1 expression in foam cells exposed to TNF-α increases in response to APS (Wang et al., 2010a). As a result, APS increases the outflow of cholesterol and reduces fat accumulation. According to further research, TNF-α-induced NF-κB activation in foam cells generated from THP-1 was reversed by APS (Wang et al., 2010a).
5.7.2 Diosgenin
Diosgenin has gained more attention recently due to its efficacy in treating several metabolic diseases, including diabetes, CVD, neurological conditions, osteoporosis, and hyperlipidemia, as well as its anticancer effects, which are mediated via multiple targets and regulate a variety of signals (Sun et al., 2021b). By preventing the nuclear translocation of the Notch intracellular domain in THP-1 cells, diosgenin prevented AS (Binesh et al., 2018). By preventing the induction of ICAM1, VCAM1, and endothelial lipase, it also prevented the adherence of TNF-α-induced leukocytes to activated endothelium cells (Wu et al., 2015b). Diosgenin is a naturally occurring compound capable of modulating M1 polarization (Saqib et al., 2018). Additionally, dioscin prevented systemic inflammation and the LOX-1/NF-κB pathway in MPMs from rats with atherosclerotic arteries, inhibiting the absorption of oxLDL (Wang et al., 2017b).
5.7.3 Panax notoginseng saponins
The primary bioactive components of Panax notoginseng (P. notoginseng) are known as Panax notoginseng saponins (PNS), which include several saponins of the dammarane type (Xu et al., 2019). PNS have several cardiovascular preventive properties, including avoiding endothelial dysfunction, boosting blood flow, inhibiting the production of foam cells, antioxidation, anti-inflammation, and antithrombosis (Yuan et al., 2011). According to Duan et al. (2022), PNS regulate the miR-194 promoter, miR-194, and MAPK methylation using cellular assays and blinded, controlled trials. PNS increased ABCA1 expression in macrophages, which reduced the buildup of cholesterol esters (Jia et al., 2010). PNS, at a dosage of 100 mg/kg per day, reduced foam cell development in rats with AS caused by zymosan A, according to in vivo research (Yuan et al., 2011).
5.7.4 Emodin
Emodin is an anthraquinone derivative isolated from Polygonum multiflorum (Ma et al., 2015). It has various therapeutic actions, including anti-tumor, anti-inflammatory, antioxidant, and anti-virus properties (Zheng et al., 2019). Studies have shown that emodin can bidirectionally regulate macrophage polarization and epigenetic regulation of macrophage memory (Iwanowycz et al., 2016). Emodin prevented H3K27 trimethylation (H3K27m3) marks from being removed from, and H3K27ac marks from being added to, genes needed for M1 or M2 polarization of macrophages (Iwanowycz et al., 2016). By activating the PPARγ/LXRα/ABCA1 signaling pathway, emodin boosted ApoA-1-mediated cholesterol efflux from THP-1 macrophages. Emodin also reduced diet-induced AS in rabbits (Hei et al., 2006; Fu et al., 2014).
6 Summary and perspectives
Evidence suggests that low-grade inflammation, predominantly driven by the immune system, plays a critical role in the development of AS (Leentjens et al., 2018). Although anti-inflammatory medications, such as canakinumab and colchicine, have been recently proven to lower the risk of CVD, there are still significant side effects and a high residual risk (Ridker et al., 2017; Tardif et al., 2019). Therefore, innovative therapies are urgently needed, and trained immunity provides interesting new pharmacological targets for new drug therapies. With enhanced production of pro-atherosclerotic cytokines/chemokines and higher foam cell generation, trained monocytes and macrophages showed a strong pro-atherosclerotic character. This is accomplished by epigenetic reprogramming of histone methylation levels and metabolic rewiring. These processes occur not only in circulating monocytes but also in myeloid progenitor cells, which ensure a long-term state of hyperactivation of innate immune cells. This review describes the aforementioned mechanisms in detail.
Although trained immunity is an immunological memory that is not disease-specific, different trained immune programs have different levels of disease specificity (Mulder et al., 2019). Natural products serve as a desirable resource in the search for novel therapies due to their high structural variety and biodiversity. Many natural products have been potential candidates for regulating immune training through different mechanisms, such as RV and EGCG. This paper provides an overview of anti-ASCVD natural products, such as flavonoids, phenols, terpenoids, carotenoids, phenylpropanoids, and alkaloids, that potentially modulate trained immunity. Although in vivo studies of AS models already exist for these natural compounds, making these compounds more promising, there is currently less evidence that these natural compounds can directly modulate training immunity. Further studies are needed to reveal possible pathways by which natural products act on trained immunity.
Data availability statement
The original contributions presented in the study are included in the article/Supplementary Material, further inquiries can be directed to the corresponding author.
Author contributions
The research project was designed by JW and CC, organized by JW and JH, and reviewed and critiqued by Y-ML. The first draft of the manuscript was written by JW, CC, and JH and reviewed and critiqued by CC. All authors contributed to the graphical analysis, drafting, and critical revision of the paper and agreed to take responsibility for all aspects of the work.
Funding
This work was supported by grants from the Programs Foundation for Leading Talents in National Administration of Traditional Chinese Medicine of China “Qihuang scholars” Project (no. 0201000401), the National Natural Science Foundation of China (no. 81974556), and the National Key Research and Development Program of China (no. 2020YFC2002701).
Conflict of interest
The authors declare that the research was conducted in the absence of any commercial or financial relationships that could be construed as a potential conflict of interest.
Publisher’s note
All claims expressed in this article are solely those of the authors and do not necessarily represent those of their affiliated organizations or those of the publisher, the editors, and the reviewers. Any product that may be evaluated in this article, or claim that may be made by its manufacturer, is not guaranteed or endorsed by the publisher.
Supplementary material
The Supplementary Material for this article can be found online at: https://www.frontiersin.org/articles/10.3389/fphar.2023.1109576/full#supplementary-material
References
Aarup, A., Pedersen, T. X., Junker, N., Christoffersen, C., Bartels, E. D., Madsen, M., et al. (2016). Hypoxia-inducible factor-1α expression in macrophages promotes development of atherosclerosis. Arterioscler. Thromb. Vasc. Biol. 36 (9), 1782–1790. doi:10.1161/atvbaha.116.307830
Abdulla, A., Zhao, X., and Yang, F. (2013). Natural polyphenols inhibit lysine-specific demethylase-1 in vitro. J. Biochem. Pharmacol. Res. 1 (1), 56–63.
Almatroodi, S. A., Almatroudi, A., Alsahli, M. A., Aljasir, M. A., Syed, M. A., and Rahmani, A. H. (2020). Epigallocatechin-3-Gallate (EGCG), an active compound of green tea attenuates acute lung injury regulating macrophage polarization and krüpple-like-factor 4 (KLF4) expression. Molecules 25 (12), 2853. doi:10.3390/molecules25122853
Alvarez, M. C., Maso, V., Torello, C. O., Ferro, K. P., and Saad, S. T. O. (2018). The polyphenol quercetin induces cell death in leukemia by targeting epigenetic regulators of pro-apoptotic genes. Clin. Epigenetics 10 (1), 139. doi:10.1186/s13148-018-0563-3
American Diabetes Association (2015). Cardiovascular disease and risk management. Diabetes Care 38 (1), S49–S57. doi:10.2337/dc15-S011
Anestopoulos, I., Sfakianos, A. P., Franco, R., Chlichlia, K., Panayiotidis, M. I., Kroll, D. J., et al. (2016). A novel role of silibinin as a putative epigenetic modulator in human prostate carcinoma. Molecules 22 (1), 62. doi:10.3390/molecules22010062
Anukunwithaya, T., Poo, P., Hunsakunachai, N., Rodsiri, R., Malaivijitnond, S., and Khemawoot, P. (2018). Absolute oral bioavailability and disposition kinetics of puerarin in female rats. BMC Pharmacol. Toxicol. 19 (1), 25. doi:10.1186/s40360-018-0216-3
Appiah, B., Amponsah, I. K., Poudyal, A., and Mensah, M. L. K. (2018). Identifying strengths and weaknesses of the integration of biomedical and herbal medicine units in Ghana using the WHO health systems framework: A qualitative study. BMC Complement. Altern. Med. 18 (1), 286. doi:10.1186/s12906-018-2334-2
Arts, R. J., Novakovic, B., Ter Horst, R., Carvalho, A., Bekkering, S., Lachmandas, E., et al. (2016). Glutaminolysis and fumarate accumulation integrate immunometabolic and epigenetic programs in trained immunity. Cell. Metab. 24 (6), 807–819. doi:10.1016/j.cmet.2016.10.008
Arts, R. J. W., Carvalho, A., La Rocca, C., Palma, C., Rodrigues, F., Silvestre, R., et al. (2016). Immunometabolic pathways in BCG-induced trained immunity. Cell. Rep. 17 (10), 2562–2571. doi:10.1016/j.celrep.2016.11.011
Arts, R. J. W., Moorlag, S., Novakovic, B., Li, Y., Wang, S. Y., Oosting, M., et al. (2018). BCG vaccination protects against experimental viral infection in humans through the induction of cytokines associated with trained immunity. Cell. Host Microbe 23 (1), 89–100. doi:10.1016/j.chom.2017.12.010
Augert, A., Mathsyaraja, H., Ibrahim, A. H., Freie, B., Geuenich, M. J., Cheng, P. F., et al. (2020). MAX functions as a tumor suppressor and rewires metabolism in small cell lung cancer. Cancer Cell. 38 (1), 97–114. doi:10.1016/j.ccell.2020.04.016
Azzini, E., Giacometti, J., and Russo, G. L. (2017). Antiobesity effects of anthocyanins in preclinical and clinical studies. Oxid. Med. Cell. Longev. 2017, 2740364. doi:10.1155/2017/2740364
Bai, L., Kee, H. J., Han, X., Zhao, T., Kee, S. J., and Jeong, M. H. (2021). Protocatechuic acid attenuates isoproterenol-induced cardiac hypertrophy via downregulation of ROCK1-Sp1-PKCγ axis. Sci. Rep. 11 (1), 17343. doi:10.1038/s41598-021-96761-2
Bannister, A. J., and Kouzarides, T. (2011). Regulation of chromatin by histone modifications. Cell. Res. 21 (3), 381–395. doi:10.1038/cr.2011.22
Bao, L., Zhang, Y., Wei, G., Wang, Y., Ma, R., Cheng, R., et al. (2015). The anti-atherosclerotic effects of puerarin on induced-atherosclerosis in rabbits. Biomed. Pap. Med. Fac. Univ. Palacky. Olomouc Czech Repub. 159 (1), 53–59. doi:10.5507/bp.2013.096
Bauer, P. M., Buga, G. M., Fukuto, J. M., Pegg, A. E., and Ignarro, L. J. (2001). Nitric oxide inhibits ornithine decarboxylase via S-nitrosylation of cysteine 360 in the active site of the enzyme. J. Biol. Chem. 276 (37), 34458–34464. doi:10.1074/jbc.M105219200
Bechor, S., Zolberg Relevy, N., Harari, A., Almog, T., Kamari, Y., Ben-Amotz, A., et al. (2016). 9-cis β-carotene increased cholesterol efflux to HDL in macrophages. Nutrients 8 (7), 435. doi:10.3390/nu8070435
Bekkering, S., Arts, R. J. W., Novakovic, B., Kourtzelis, I., van der Heijden, C. D. C. C., Li, Y., et al. (2018). Metabolic induction of trained immunity through the mevalonate pathway. Cell. 172 (1-2), 135–146. doi:10.1016/j.cell.2017.11.025
Bekkering, S., Quintin, J., Joosten, L. A., van der Meer, J. W. M., Netea, M. G., and Riksen, N. P. (2014). Oxidized low-density lipoprotein induces long-term proinflammatory cytokine production and foam cell formation via epigenetic reprogramming of monocytes. Arterioscler. Thromb. Vasc. Biol. 34 (8), 1731–1738. doi:10.1161/atvbaha.114.303887
Bekkering, S., van den Munckhof, I., Nielen, T., Lamfers, E., Dinarello, C., Rutten, J., et al. (2016). Innate immune cell activation and epigenetic remodeling in symptomatic and asymptomatic atherosclerosis in humans in vivo. Atherosclerosis 254, 228–236. doi:10.1016/j.atherosclerosis.2016.10.019
Bergman, M. E., Davis, B., and Phillips, M. A. (2019). Medically useful plant terpenoids: Biosynthesis, occurrence, and mechanism of action. Molecules 24 (21), 3961. doi:10.3390/molecules24213961
Binesh, A., Devaraj, S. N., and Devaraj, H. (2018). Inhibition of nuclear translocation of notch intracellular domain (NICD) by diosgenin prevented atherosclerotic disease progression. Biochimie 148, 63–71. doi:10.1016/j.biochi.2018.02.011
Biswas, S., Kar, A., Sharma, N., Haldar, P. K., and Mukherjee, P. K. (2021). Synergistic effect of ursolic acid and piperine in CCl(4) induced hepatotoxicity. Ann. Med. 53 (1), 2009–2017. doi:10.1080/07853890.2021.1995625
Boffa, M. B., and Koschinsky, M. L. (2019). Oxidized phospholipids as a unifying theory for lipoprotein(a) and cardiovascular disease. Nat. Rev. Cardiol. 16 (5), 305–318. doi:10.1038/s41569-018-0153-2
Bonilla, F. A., and Oettgen, H. C. (2010). Adaptive immunity. J. Allergy Clin. Immunol. 125 (2), S33–S40. doi:10.1016/j.jaci.2009.09.017
Boots, A. W., Haenen, G. R., and Bast, A. (2008). Health effects of quercetin: From antioxidant to nutraceutical. Eur. J. Pharmacol. 585 (2-3), 325–337. doi:10.1016/j.ejphar.2008.03.008
Braza, M. S., van Leent, M. M. T., Lameijer, M., Sanchez-Gaytan, B. L., Arts, R. J. W., Perez-Medina, C., et al. (2018). Inhibiting inflammation with myeloid cell-specific nanobiologics promotes organ transplant acceptance. Immunity 49 (5), 819–828. doi:10.1016/j.immuni.2018.09.008
Buttari, B., Profumo, E., Segoni, L., D'Arcangelo, D., Rossi, S., Facchiano, F., et al. (2014). Resveratrol counteracts inflammation in human M1 and M2 macrophages upon challenge with 7-oxo-cholesterol: Potential therapeutic implications in atherosclerosis. Oxid. Med. Cell. Longev. 2014, 257543. doi:10.1155/2014/257543
Cai, Y., and Zhao, F. (2021). Fluvastatin suppresses the proliferation, invasion, and migration and promotes the apoptosis of endometrial cancer cells by upregulating Sirtuin 6 (SIRT6). Bioengineered 12 (2), 12509–12520. doi:10.1080/21655979.2021.2009415
Cao, R., Zhao, Y., Zhou, Z., and Zhao, X. (2018). Enhancement of the water solubility and antioxidant activity of hesperidin by chitooligosaccharide. J. Sci. Food Agric. 98 (6), 2422–2427. doi:10.1002/jsfa.8734
Chakrawarti, L., Agrawal, R., Dang, S., Gupta, S., and Gabrani, R. (2016). Therapeutic effects of EGCG: A patent review. Expert Opin. Ther. Pat. 26 (8), 907–916. doi:10.1080/13543776.2016.1203419
Chang, X., Zhang, T., Liu, D., Meng, Q., Yan, P., Luo, D., et al. (2021). Puerarin attenuates LPS-induced inflammatory responses and oxidative stress injury in human umbilical vein endothelial cells through mitochondrial quality control. Oxid. Med. Cell. Longev. 2021, 6659240. doi:10.1155/2021/6659240
Chen, B., Li, H., Ou, G., Ren, L., Yang, X., and Zeng, M. (2019). Curcumin attenuates MSU crystal-induced inflammation by inhibiting the degradation of IκBα and blocking mitochondrial damage. Arthritis Res. Ther. 21 (1), 193. doi:10.1186/s13075-019-1974-z
Chen, G., Jia, P., Yin, Z. Y., Kong, S. Z., Xiang, Z. B., and Zheng, X. X. (2019). Paeonol ameliorates monosodium urate-induced arthritis in rats through inhibiting nuclear factor-κB-mediated proinflammatory cytokine production. Phytother. Res. 33 (11), 2971–2978. doi:10.1002/ptr.6472
Chen, S. J., Kao, Y. H., Jing, L., Chuang, Y. P., Wu, W. L., Liu, S. T., et al. (2017). Epigallocatechin-3-gallate reduces scavenger receptor A expression and foam cell formation in human macrophages. J. Agric. Food Chem. 65 (15), 3141–3150. doi:10.1021/acs.jafc.6b05832
Chen, X., Huang, C., Sun, H., Hong, H., Jin, J., Bei, C., et al. (2021). Puerarin suppresses inflammation and ECM degradation through Nrf2/HO-1 axis in chondrocytes and alleviates pain symptom in osteoarthritic mice. Food Funct. 12 (5), 2075–2089. doi:10.1039/d0fo03076g
Cheng, S. C., Quintin, J., Cramer, R. A., Shepardson, K. M., Saeed, S., Kumar, V., et al. (2014). mTOR- and HIF-1α-mediated aerobic glycolysis as metabolic basis for trained immunity. Science 345 (6204), 1250684. doi:10.1126/science.1250684
Chi, L., Peng, L., Pan, N., Hu, X., and Zhang, Y. (2014). The anti-atherogenic effects of berberine on foam cell formation are mediated through the upregulation of sirtuin 1. Int. J. Mol. Med. 34 (4), 1087–1093. doi:10.3892/ijmm.2014.1868
Choi, D. Y., Lee, Y. J., Hong, J. T., and Lee, H. J. (2012). Antioxidant properties of natural polyphenols and their therapeutic potentials for Alzheimer's disease. Brain Res. Bull. 87 (2-3), 144–153. doi:10.1016/j.brainresbull.2011.11.014
Choi, K. C., Jung, M. G., Lee, Y. H., Yoon, J. C., Kwon, S. H., Kang, H. B., et al. (2009). Epigallocatechin-3-gallate, a histone acetyltransferase inhibitor, inhibits EBV-induced B lymphocyte transformation via suppression of RelA acetylation. Cancer Res. 69 (2), 583–592. doi:10.1158/0008-5472.Can-08-2442
Christ, A., Günther, P., Lauterbach, M. A. R., Duewell, P., Biswas, D., Pelka, K., et al. (2018). Western diet triggers NLRP3-dependent innate immune reprogramming. Cell. 172 (1-2), 162–175. doi:10.1016/j.cell.2017.12.013
Christ, A., and Latz, E. (2019). The Western lifestyle has lasting effects on metaflammation. Nat. Rev. Immunol. 19 (5), 267–268. doi:10.1038/s41577-019-0156-1
Christofk, H. R., Vander Heiden, M. G., Harris, M. H., Ramanathan, A., Gerszten, R. E., Wei, R., et al. (2008). The M2 splice isoform of pyruvate kinase is important for cancer metabolism and tumour growth. Nature 452 (7184), 230–233. doi:10.1038/nature06734
Clem, B., Telang, S., Clem, A., Yalcin, A., Meier, J., Simmons, A., et al. (2008). Small-molecule inhibition of 6-phosphofructo-2-kinase activity suppresses glycolytic flux and tumor growth. Mol. Cancer Ther. 7 (1), 110–120. doi:10.1158/1535-7163.Mct-07-0482
Conrath, U., Beckers, G. J., Langenbach, C. J., and Jaskiewicz, M. R. (2015). Priming for enhanced defense. Annu. Rev. Phytopathol. 53, 97–119. doi:10.1146/annurev-phyto-080614-120132
Cotler, S., Buggé, C. J., and Colburn, W. A. (1983). Role of gut contents, intestinal wall, and liver on the first pass metabolism and absolute bioavailability of isotretinoin in the dog. Drug Metab. Dispos. 11 (5), 458–462.
Courties, G., Herisson, F., Sager, H. B., Heidt, T., Ye, Y., Wei, Y., et al. (2015). Ischemic stroke activates hematopoietic bone marrow stem cells. Circ. Res. 116 (3), 407–417. doi:10.1161/circresaha.116.305207
Crişan, T. O., Cleophas, M. C. P., Novakovic, B., Erler, K., van de Veerdonk, F. L., Stunnenberg, H. G., et al. (2017). Uric acid priming in human monocytes is driven by the AKT-PRAS40 autophagy pathway. Proc. Natl. Acad. Sci. U. S. A. 114 (21), 5485–5490. doi:10.1073/pnas.1620910114
Cui, Y., Hou, P., Li, F., Liu, Q., Qin, S., Zhou, G., et al. (2017). Quercetin improves macrophage reverse cholesterol transport in apolipoprotein E-deficient mice fed a high-fat diet. Lipids Health Dis. 16 (1), 9. doi:10.1186/s12944-016-0393-2
Cunningham, L., Finckbeiner, S., Hyde, R. K., Southall, N., Marugan, J., Yedavalli, V. R. K., et al. (2012). Identification of benzodiazepine Ro5-3335 as an inhibitor of CBF leukemia through quantitative high throughput screen against RUNX1-CBFβ interaction. Proc. Natl. Acad. Sci. U. S. A. 109 (36), 14592–14597. doi:10.1073/pnas.1200037109
Czank, C., Cassidy, A., Zhang, Q., Morrison, D. J., Preston, T., Kroon, P. A., et al. (2013). Human metabolism and elimination of the anthocyanin, cyanidin-3-glucoside: A (13)C-tracer study. Am. J. Clin. Nutr. 97 (5), 995–1003. doi:10.3945/ajcn.112.049247
Dalibalta, S., Majdalawieh, A. F., and Manjikian, H. (2020). Health benefits of sesamin on cardiovascular disease and its associated risk factors. Saudi Pharm. J. 28 (10), 1276–1289. doi:10.1016/j.jsps.2020.08.018
Das, J., Verma, D., Gustafsson, M., and Lerm, M. (2019). Identification of DNA methylation patterns predisposing for an efficient response to BCG vaccination in healthy BCG-naïve subjects. Epigenetics 14 (6), 589–601. doi:10.1080/15592294.2019.1603963
Daw, S., and Law, S. (2021). Quercetin induces autophagy in myelodysplastic bone marrow including hematopoietic stem/progenitor compartment. Environ. Toxicol. 36 (2), 149–167. doi:10.1002/tox.23020
Del Bo, C., Cao, Y., Roursgaard, M., Riso, P., Porrini, M., Loft, S., et al. (2016). Anthocyanins and phenolic acids from a wild blueberry (Vaccinium angustifolium) powder counteract lipid accumulation in THP-1-derived macrophages. Eur. J. Nutr. 55 (1), 171–182. doi:10.1007/s00394-015-0835-z
Del Rio, D., Stalmach, A., Calani, L., and Crozier, A. (2010). Bioavailability of coffee chlorogenic acids and green tea flavan-3-ols. Nutrients 2 (8), 820–833. doi:10.3390/nu2080820
Deng, Z., Li, Y., Liu, H., Xiao, S., Li, L., Tian, J., et al. (2019). The role of sirtuin 1 and its activator, resveratrol in osteoarthritis. Biosci. Rep. 39 (5). doi:10.1042/bsr20190189
Di Gioia, M., Spreafico, R., Springstead, J. R., Mendelson, M. M., Joehanes, R., Levy, D., et al. (2020). Endogenous oxidized phospholipids reprogram cellular metabolism and boost hyperinflammation. Nat. Immunol. 21 (1), 42–53. doi:10.1038/s41590-019-0539-2
Ding, B., Lin, C., Liu, Q., He, Y., Ruganzu, J. B., Jin, H., et al. (2020). Tanshinone IIA attenuates neuroinflammation via inhibiting RAGE/NF-κB signaling pathway in vivo and in vitro. J. Neuroinflammation 17 (1), 302. doi:10.1186/s12974-020-01981-4
Domínguez-Andrés, J., Ferreira, A. V., Jansen, T., Smithers, N., Prinjha, R. K., Furze, R. C., et al. (2019). Bromodomain inhibitor I-BET151 suppresses immune responses during fungal-immune interaction. Eur. J. Immunol. 49 (11), 2044–2050. doi:10.1002/eji.201848081
Dong, J., Zhang, X., Zhang, L., Bian, H. X., Xu, N., Bao, B., et al. (2014). Quercetin reduces obesity-associated ATM infiltration and inflammation in mice: A mechanism including ampkα1/SIRT1. J. Lipid Res. 55 (3), 363–374. doi:10.1194/jlr.M038786
Dong, W., Wang, X., Bi, S., Pan, Z., Liu, S., Yu, H., et al. (2014). Inhibitory effects of resveratrol on foam cell formation are mediated through monocyte chemotactic protein-1 and lipid metabolism-related proteins. Int. J. Mol. Med. 33 (5), 1161–1168. doi:10.3892/ijmm.2014.1680
Du, Q. H., Peng, C., and Zhang, H. (2013). Polydatin: A review of pharmacology and pharmacokinetics. Pharm. Biol. 51 (11), 1347–1354. doi:10.3109/13880209.2013.792849
Duan, L., Liu, Y., Li, J., Zhang, Y., Dong, Y., Liu, C., et al. (2022). Panax notoginseng saponins alleviate coronary artery disease through hypermethylation of the miR-194-MAPK pathway. Front. Pharmacol. 13, 829416. doi:10.3389/fphar.2022.829416
Dutta, P., Courties, G., Wei, Y., Leuschner, F., Gorbatov, R., Robbins, C. S., et al. (2012). Myocardial infarction accelerates atherosclerosis. Nature 487 (7407), 325–329. doi:10.1038/nature11260
Edgar, L., Akbar, N., Braithwaite, A. T., Krausgruber, T., Gallart-Ayala, H., Bailey, J., et al. (2021). Hyperglycemia induces trained immunity in macrophages and their precursors and promotes atherosclerosis. Circulation 144 (12), 961–982. doi:10.1161/circulationaha.120.046464
Eguchi, A., Kaneko, Y., Murakami, A., and Ohigashi, H. (2007). Zerumbone suppresses phorbol ester-induced expression of multiple scavenger receptor genes in THP-1 human monocytic cells. Biosci. Biotechnol. Biochem. 71 (4), 935–945. doi:10.1271/bbb.60596
El-Shitany, N. A., and Eid, B. G. (2019). Icariin modulates carrageenan-induced acute inflammation through HO-1/Nrf2 and NF-kB signaling pathways. Biomed. Pharmacother. 120, 109567. doi:10.1016/j.biopha.2019.109567
Everts, B., Amiel, E., van der Windt, G. J., Freitas, T. C., Chott, R., Yarasheski, K. E., et al. (2012). Commitment to glycolysis sustains survival of NO-producing inflammatory dendritic cells. Blood 120 (7), 1422–1431. doi:10.1182/blood-2012-03-419747
Evtyugin, D. D., Magina, S., and Evtuguin, D. V. (2020). Recent advances in the production and applications of ellagic acid and its derivatives. A review. Molecules 25 (12), 2745. doi:10.3390/molecules25122745
Faisal, W., O'Driscoll, C. M., and Griffin, B. T. (2010). Bioavailability of lycopene in the rat: The role of intestinal lymphatic transport. J. Pharm. Pharmacol. 62 (3), 323–331. doi:10.1211/jpp.62.03.0006
Fang, J. (2015). Classification of fruits based on anthocyanin types and relevance to their health effects. Nutrition 31 (11-12), 1301–1306. doi:10.1016/j.nut.2015.04.015
Fanucchi, S., Domínguez-Andrés, J., Joosten, L. A. B., Netea, M. G., and Mhlanga, M. M. (2021). The intersection of epigenetics and metabolism in trained immunity. Immunity 54 (1), 32–43. doi:10.1016/j.immuni.2020.10.011
Fanucchi, S., Fok, E. T., Dalla, E., Shibayama, Y., Borner, K., Chang, E. Y., et al. (2019). Immune genes are primed for robust transcription by proximal long noncoding RNAs located in nuclear compartments. Nat. Genet. 51 (1), 138–150. doi:10.1038/s41588-018-0298-2
Fatima, N., Baqri, S. S. R., Bhattacharya, A., Koney, N. K. K., Husain, K., Abbas, A., et al. (2021). Role of flavonoids as epigenetic modulators in cancer prevention and therapy. Front. Genet. 12, 758733. doi:10.3389/fgene.2021.758733
Felgines, C., Texier, O., Besson, C., Lyan, B., Lamaison, J. L., and Scalbert, A. (2007). Strawberry pelargonidin glycosides are excreted in urine as intact glycosides and glucuronidated pelargonidin derivatives in rats. Br. J. Nutr. 98 (6), 1126–1131. doi:10.1017/s0007114507764772
Feng, X., Qin, H., Shi, Q., Zhang, Y., Zhou, F., Wu, H., et al. (2014). Chrysin attenuates inflammation by regulating M1/M2 status via activating PPARγ. Biochem. Pharmacol. 89 (4), 503–514. doi:10.1016/j.bcp.2014.03.016
Feng, X., Wang, K., Cao, S., Ding, L., and Qiu, F. (2020). Pharmacokinetics and excretion of berberine and its nine metabolites in rats. Front. Pharmacol. 11, 594852. doi:10.3389/fphar.2020.594852
Ferry, D. R., Smith, A., Malkhandi, J., Fyfe, D. W., deTakats, P. G., AnDerson, D., et al. (1996). Phase I clinical trial of the flavonoid quercetin: Pharmacokinetics and evidence for in vivo tyrosine kinase inhibition. Clin. Cancer Res. 2 (4), 659–668.
Flores-Gomez, D., Bekkering, S., Netea, M. G., and Riksen, N. P. (2021). Trained immunity in atherosclerotic cardiovascular disease. Arterioscler. Thromb. Vasc. Biol. 41 (1), 62–69. doi:10.1161/atvbaha.120.314216
Folco, E. J., Sheikine, Y., Rocha, V. Z., Christen, T., Shvartz, E., Sukhova, G. K., et al. (2011). Hypoxia but not inflammation augments glucose uptake in human macrophages: Implications for imaging atherosclerosis with 18fluorine-labeled 2-deoxy-D-glucose positron emission tomography. J. Am. Coll. Cardiol. 58 (6), 603–614. doi:10.1016/j.jacc.2011.03.044
Foster, S. L., Hargreaves, D. C., and Medzhitov, R. (2007). Gene-specific control of inflammation by TLR-induced chromatin modifications. Nature 447 (7147), 972–978. doi:10.1038/nature05836
Fried, L. E., and Arbiser, J. L. (2009). Honokiol, a multifunctional antiangiogenic and antitumor agent. Antioxid. Redox Signal 11 (5), 1139–1148. doi:10.1089/ars.2009.2440
Fu, X., Xu, A. G., Yao, M. Y., Guo, L., and Zhao, L. S. (2014). Emodin enhances cholesterol efflux by activating peroxisome proliferator-activated receptor-γ in oxidized low density lipoprotein-loaded THP1 macrophages. Clin. Exp. Pharmacol. Physiol. 41 (9), 679–684. doi:10.1111/1440-1681.12262
Fujii, J., Osaki, T., and Bo, T. (2022). Ascorbate is a primary antioxidant in mammals. Molecules 27 (19), 6187. doi:10.3390/molecules27196187
Fukuzumi, M., Shinomiya, H., Shimizu, Y., Ohishi, K., and UtSumi, S. (1996). Endotoxin-induced enhancement of glucose influx into murine peritoneal macrophages via GLUT1. Infect. Immun. 64 (1), 108–112. doi:10.1128/iai.64.1.108-112.1996
Fullerton, M. D., Ford, R. J., McGregor, C. P., LeBlond, N. D., Snider, S. A., Stypa, S. A., et al. (2015). Salicylate improves macrophage cholesterol homeostasis via activation of Ampk. J. Lipid Res. 56 (5), 1025–1033. doi:10.1194/jlr.M058875
Galano, A., Francisco Marquez, M., and Pérez-González, A. (2014). Ellagic acid: An unusually versatile protector against oxidative stress. Chem. Res. Toxicol. 27 (5), 904–918. doi:10.1021/tx500065y
Gallino, A., Aboyans, V., Diehm, C., Cosentino, F., Stricker, H., Falk, E., et al. (2014). Non-coronary atherosclerosis. Eur. Heart J. 35 (17), 1112–1119. doi:10.1093/eurheartj/ehu071
Ganai, S. A., Sheikh, F. A., and Baba, Z. A. (2021). Plant flavone Chrysin as an emerging histone deacetylase inhibitor for prosperous epigenetic-based anticancer therapy. Phytother. Res. 35 (2), 823–834. doi:10.1002/ptr.6869
Gani, S. A., Muhammad, S. A., Kura, A. U., Barahuie, F., Hussein, M. Z., and Fakurazi, S. (2019). Effect of protocatechuic acid-layered double hydroxide nanoparticles on diethylnitrosamine/phenobarbital-induced hepatocellular carcinoma in mice. PLoS One 14 (5), e0217009. doi:10.1371/journal.pone.0217009
Gao, J., Xu, Y., Yang, Y., Yang, Y., Zheng, Z., Jiang, W., et al. (2008). Identification of upregulators of human ATP-binding cassette transporter A1 via high-throughput screening of a synthetic and natural compound library. J. Biomol. Screen 13 (7), 648–656. doi:10.1177/1087057108320545
Gao, X., Wu, J., Zou, W., and Dai, Y. (2014). Two ellagic acids isolated from roots of Sanguisorba officinalis L. promote hematopoietic progenitor cell proliferation and megakaryocyte differentiation. Molecules 19 (4), 5448–5458. doi:10.3390/molecules19045448
Garcia, C., and Blesso, C. N. (2021). Antioxidant properties of anthocyanins and their mechanism of action in atherosclerosis. Free Radic. Biol. Med. 172, 152–166. doi:10.1016/j.freeradbiomed.2021.05.040
Geng, J., Fu, W., Yu, X., Lu, Z., Liu, Y., Sun, M., et al. (2020). Ginsenoside Rg3 alleviates ox-LDL induced endothelial dysfunction and prevents atherosclerosis in ApoE(-/-) mice by regulating PPARγ/FAK signaling pathway. Front. Pharmacol. 11, 500. doi:10.3389/fphar.2020.00500
Gobalakrishnan, S., Asirvatham, S. S., and Janarthanam, V. (2016). Effect of silybin on lipid profile in hypercholesterolaemic rats. J. Clin. Diagn Res. 10 (4), Ff01–5. doi:10.7860/jcdr/2016/16393.7566
Godugu, C., Patel, A. R., Doddapaneni, R., Somagoni, J., and Singh, M. (2014). Approaches to improve the oral bioavailability and effects of novel anticancer drugs berberine and betulinic acid. PLoS One 9 (3), e89919. doi:10.1371/journal.pone.0089919
Gourbal, B., Pinaud, S., Beckers, G. J. M., Van Der Meer, J. W. M., Conrath, U., and Netea, M. G. (2018). Innate immune memory: An evolutionary perspective. Immunol. Rev. 283 (1), 21–40. doi:10.1111/imr.12647
Griñán-Ferré, C., Bellver-Sanchis, A., Izquierdo, V., Corpas, R., Roig-Soriano, J., Chillon, M., et al. (2021). The pleiotropic neuroprotective effects of resveratrol in cognitive decline and Alzheimer’s disease pathology: From antioxidant to epigenetic therapy. Ageing Res. Rev. 67, 101271. doi:10.1016/j.arr.2021.101271
Groh, L., Keating, S. T., Joosten, L. A. B., Netea, M. G., and Riksen, N. P. (2018). Monocyte and macrophage immunometabolism in atherosclerosis. Semin. Immunopathol. 40 (2), 203–214. doi:10.1007/s00281-017-0656-7
Gudas, L. J. (2013). Retinoids induce stem cell differentiation via epigenetic changes. Semin. Cell. Dev. Biol. 24 (10-12), 701–705. doi:10.1016/j.semcdb.2013.08.002
Gui, Y. Z., Yan, H., Gao, F., Xi, C., Li, H. H., and Wang, Y. P. (2016). Betulin attenuates atherosclerosis in apoE(-/-) mice by up-regulating ABCA1 and ABCG1. Acta Pharmacol. Sin. 37 (10), 1337–1348. doi:10.1038/aps.2016.46
Guo, H. X., Liu, D. H., Ma, Y., Liu, J. f., Wang, Y., Du, Z. y., et al. (2009). Long-term baicalin administration ameliorates metabolic disorders and hepatic steatosis in rats given a high-fat diet. Acta Pharmacol. Sin. 30 (11), 1505–1512. doi:10.1038/aps.2009.150
Gupta, A., Singh, A. K., Kumar, R., Jamieson, S., Pandey, A. K., and Bishayee, A. (2021). Neuroprotective potential of ellagic acid: A critical review. Adv. Nutr. 12 (4), 1211–1238. doi:10.1093/advances/nmab007
Han, G. M., Soliman, G. A., Meza, J. L., Islam, K. M. M., and Watanabe-Galloway, S. (2016). The influence of BMI on the association between serum lycopene and the metabolic syndrome. Br. J. Nutr. 115 (7), 1292–1300. doi:10.1017/s0007114516000179
Han, P., Gao, D., Zhang, W., Liu, S., Yang, S., and Li, X. (2015). Puerarin suppresses high glucose-induced MCP-1 expression via modulating histone methylation in cultured endothelial cells. Life Sci. 130, 103–107. doi:10.1016/j.lfs.2015.02.022
Hao, H., Wang, G., Cui, N., Li, J., Xie, L., and Ding, Z. (2006). Pharmacokinetics, absorption and tissue distribution of tanshinone IIA solid dispersion. Planta Med. 72 (14), 1311–1317. doi:10.1055/s-2006-951698
Hao, S., Xiao, Y., Lin, Y., Mo, Z., Chen, Y., Peng, X., et al. (2016). Chlorogenic acid-enriched extract from Eucommia ulmoides leaves inhibits hepatic lipid accumulation through regulation of cholesterol metabolism in HepG2 cells. Pharm. Biol. 54 (2), 251–259. doi:10.3109/13880209.2015.1029054
Hardbower, D. M., Asim, M., Luis, P. B., Singh, K., Barry, D. P., Yang, C., et al. (2017). Ornithine decarboxylase regulates M1 macrophage activation and mucosal inflammation via histone modifications. Proc. Natl. Acad. Sci. U. S. A. 114 (5), E751-E760–e60. doi:10.1073/pnas.1614958114
Hassan, F. U., Rehman, M. S., Khan, M. S., Ali, M. A., Javed, A., Nawaz, A., et al. (2019). Curcumin as an alternative epigenetic modulator: Mechanism of action and potential effects. Front. Genet. 10, 514. doi:10.3389/fgene.2019.00514
He, F., and Yao, G. (2021). Ginsenoside Rg1 as a potential regulator of hematopoietic stem/progenitor cells. Stem Cells Int. 2021, 4633270. doi:10.1155/2021/4633270
He, X. W., Yu, D., Li, W. L., Zheng, Z., Lv, C. L., Li, C., et al. (2016). Anti-atherosclerotic potential of baicalin mediated by promoting cholesterol efflux from macrophages via the PPARγ-LXRα-ABCA1/ABCG1 pathway. Biomed. Pharmacother. 83, 257–264. doi:10.1016/j.biopha.2016.06.046
He, Y., Shi, Y., Yang, Y., Huang, H., Feng, Y., Wang, Y., et al. (2021). Chrysin induces autophagy through the inactivation of the ROS-mediated Akt/mTOR signaling pathway in endometrial cancer. Int. J. Mol. Med. 48 (3), 172. doi:10.3892/ijmm.2021.5005
Hei, Z. Q., Huang, H. Q., Tan, H. M., Liu, P. q., Zhao, L. z., Chen, S. r., et al. (2006). Emodin inhibits dietary induced atherosclerosis by antioxidation and regulation of the sphingomyelin pathway in rabbits. Chin. Med. J. Engl. 119 (10), 868–870. doi:10.1097/00029330-200605020-00012
Heidt, T., Sager, H. B., Courties, G., Dutta, P., Iwamoto, Y., Zaltsman, A., et al. (2014). Chronic variable stress activates hematopoietic stem cells. Nat. Med. 20 (7), 754–758. doi:10.1038/nm.3589
Hemn, H. O., Noordin, M. M., Rahman, H. S., Hazilawati, H., Zuki, A., and Chartrand, M. S. (2015). Antihypercholesterolemic and antioxidant efficacies of zerumbone on the formation, development, and establishment of atherosclerosis in cholesterol-fed rabbits. Drug Des. Devel Ther. 9, 4173–4208. doi:10.2147/dddt.S76225
Himes, S. R., Cronau, S., Mulford, C., and Hume, D. A. (2005). The Runx1 transcription factor controls CSF-1-dependent and -independent growth and survival of macrophages. Oncogene 24 (34), 5278–5286. doi:10.1038/sj.onc.1208657
Howard, T. D., Ho, S. M., Zhang, L., Chen, J., Cui, W., Slager, R., et al. (2011). Epigenetic changes with dietary soy in cynomolgus monkeys. PLoS One 6 (10), e26791. doi:10.1371/journal.pone.0026791
Hsieh, C. C., Kuo, C. H., Kuo, H. F., Chen, Y. S., Wang, S. L., Chao, D., et al. (2014). Sesamin suppresses macrophage-derived chemokine expression in human monocytes via epigenetic regulation. Food Funct. 5 (10), 2494–2500. doi:10.1039/c4fo00322e
Hu, K., Yang, Y., Tu, Q., and Luo, Y. (2013). Alpinetin inhibits LPS-induced inflammatory mediator response by activating PPAR-γ in THP-1-derived macrophages. Eur. J. Pharmacol. 721 (1-3), 96–102. doi:10.1016/j.ejphar.2013.09.049
Hu, Y., Sun, B., Liu, K., Yan, M., Zhang, Y., Miao, C., et al. (2016). Icariin attenuates high-cholesterol diet induced atherosclerosis in rats by inhibition of inflammatory response and p38 MAPK signaling pathway. Inflammation 39 (1), 228–236. doi:10.1007/s10753-015-0242-x
Huang, J., Weinstein, S. J., Yu, K., Mannisto, S., and Albanes, D. (2018). Serum beta carotene and overall and cause-specific mortality. Circ. Res. 123 (12), 1339–1349. doi:10.1161/circresaha.118.313409
Huang, L., Chambliss, K. L., Gao, X., Yuhanna, I. S., Behling-Kelly, E., Bergaya, S., et al. (2019). SR-B1 drives endothelial cell LDL transcytosis via DOCK4 to promote atherosclerosis. Nature 569 (7757), 565–569. doi:10.1038/s41586-019-1140-4
Huang, Z., Dong, F., Li, S., Chu, M., Zhou, H., Lu, Z., et al. (2012). Berberine-induced inhibition of adipocyte enhancer-binding protein 1 attenuates oxidized low-density lipoprotein accumulation and foam cell formation in phorbol 12-myristate 13-acetate-induced macrophages. Eur. J. Pharmacol. 690 (1-3), 164–169. doi:10.1016/j.ejphar.2012.07.009
Huo, M., Chen, N., Chi, G., Yuan, X., Guan, S., Li, H., et al. (2012). Traditional medicine alpinetin inhibits the inflammatory response in Raw 264.7 cells and mouse models. Int. Immunopharmacol. 12 (1), 241–248. doi:10.1016/j.intimp.2011.11.017
Hussein, G., Sankawa, U., Goto, H., Matsumoto, K., and Watanabe, H. (2006). Astaxanthin, a carotenoid with potential in human health and nutrition. J. Nat. Prod. 69 (3), 443–449. doi:10.1021/np050354+
Huwait, E. A., Greenow, K. R., Singh, N. N., and Ramji, D. P. (2011). A novel role for c-Jun N-terminal kinase and phosphoinositide 3-kinase in the liver X receptor-mediated induction of macrophage gene expression. Cell. Signal 23 (3), 542–549. doi:10.1016/j.cellsig.2010.11.002
Ifrim, D. C., Quintin, J., Joosten, L. A., Jacobs, C., Jansen, T., Jacobs, L., et al. (2014). Trained immunity or tolerance: Opposing functional programs induced in human monocytes after engagement of various pattern recognition receptors. Clin. Vaccine Immunol. 21 (4), 534–545. doi:10.1128/cvi.00688-13
Iio, A., Ohguchi, K., Iinuma, M., Nozawa, Y., and Ito, M. (2012). Hesperetin upregulates ABCA1 expression and promotes cholesterol efflux from THP-1 macrophages. J. Nat. Prod. 75 (4), 563–566. doi:10.1021/np200696r
Iizuka, M., Ayaori, M., Uto-Kondo, H., Yakushiji, E., Takiguchi, S., Nakaya, K., et al. (2012). Astaxanthin enhances ATP-binding cassette transporter A1/G1 expressions and cholesterol efflux from macrophages. J. Nutr. Sci. Vitaminol. (Tokyo) 58 (2), 96–104. doi:10.3177/jnsv.58.96
Ikhlef, S., Berrougui, H., Kamtchueng Simo, O., and Khalil, A. (2016). Paraoxonase 1-treated oxLDL promotes cholesterol efflux from macrophages by stimulating the PPARγ-LXRα-ABCA1 pathway. FEBS Lett. 590 (11), 1614–1629. doi:10.1002/1873-3468.12198
Im, D. S. (2020). Pro-resolving effect of ginsenosides as an anti-inflammatory mechanism of panax ginseng. Biomolecules 10 (3), 444. doi:10.3390/biom10030444
Iwanowycz, S., Wang, J., Altomare, D., Hui, Y., and Fan, D. (2016). Emodin bidirectionally modulates macrophage polarization and epigenetically regulates macrophage memory. J. Biol. Chem. 291 (22), 11491–11503. doi:10.1074/jbc.M115.702092
Izzo, S., Naponelli, V., and Bettuzzi, S. (2020). Flavonoids as epigenetic modulators for prostate cancer prevention. Nutrients 12 (4), 1010. doi:10.3390/nu12041010
Jayakumar, T., Lin, K. C., Chang, C. C., Hsia, C. W., Manubolu, M., Huang, W. C., et al. (2021). Targeting MAPK/NF-κB pathways in anti-inflammatory potential of rutaecarpine: Impact on src/FAK-mediated macrophage migration. Int. J. Mol. Sci. 23 (1), 92. doi:10.3390/ijms23010092
Jha, A. K., Huang, S. C., Sergushichev, A., Lampropoulou, V., Ivanova, Y., Loginicheva, E., et al. (2015). Network integration of parallel metabolic and transcriptional data reveals metabolic modules that regulate macrophage polarization. Immunity 42 (3), 419–430. doi:10.1016/j.immuni.2015.02.005
Jia, L. Q., Zhang, N., Xu, Y., Chen, W. n., Zhu, M. l., Song, N., et al. (2016). Tanshinone IIA affects the HDL subfractions distribution not serum lipid levels: Involving in intake and efflux of cholesterol. Arch. Biochem. Biophys. 592, 50–59. doi:10.1016/j.abb.2016.01.001
Jia, Q., Cao, H., Shen, D., Li, S., Yan, L., Chen, C., et al. (2019). Quercetin protects against atherosclerosis by regulating the expression of PCSK9, CD36, PPARγ, LXRα and ABCA1. Int. J. Mol. Med. 44 (3), 893–902. doi:10.3892/ijmm.2019.4263
Jia, Y., Li, Z. Y., Zhang, H. G., Li, H. B., Liu, Y., and Li, X. H. (2010). Panax notoginseng saponins decrease cholesterol ester via up-regulating ATP-binding cassette transporter A1 in foam cells. J. Ethnopharmacol. 132 (1), 297–302. doi:10.1016/j.jep.2010.08.033
Jiang, J., Mo, Z. C., Yin, K., Zhao, G. J., Lv, Y. C., Ouyang, X. P., et al. (2012). Epigallocatechin-3-gallate prevents TNF-α-induced NF-κB activation thereby upregulating ABCA1 via the Nrf2/Keap1 pathway in macrophage foam cells. Int. J. Mol. Med. 29 (5), 946–956. doi:10.3892/ijmm.2012.924
Jiang, Z., Sang, H., Fu, X., Liang, Y., and Li, L. (2015). Alpinetin enhances cholesterol efflux and inhibits lipid accumulation in oxidized low-density lipoprotein-loaded human macrophages. Biotechnol. Appl. Biochem. 62 (6), 840–847. doi:10.1002/bab.1328
Jing, X., Han, J., Zhang, J., Chen, Y., Yuan, J., Wang, J., et al. (2021). Long non-coding RNA MEG3 promotes cisplatin-induced nephrotoxicity through regulating AKT/TSC/mTOR-mediated autophagy. Int. J. Biol. Sci. 17 (14), 3968–3980. doi:10.7150/ijbs.58910
Jung, C. G., Horike, H., Cha, B. Y., Uhm, K. O., Yamauchi, R., Yamaguchi, T., et al. (2010). Honokiol increases ABCA1 expression level by activating retinoid X receptor beta. Biol. Pharm. Bull. 33 (7), 1105–1111. doi:10.1248/bpb.33.1105
Kang, I., Okla, M., and Chung, S. (2014). Ellagic acid inhibits adipocyte differentiation through coactivator-associated arginine methyltransferase 1-mediated chromatin modification. J. Nutr. Biochem. 25 (9), 946–953. doi:10.1016/j.jnutbio.2014.04.008
Kasala, E. R., Bodduluru, L. N., Madana, R. M., V, A. K., Gogoi, R., and Barua, C. C. (2015). Chemopreventive and therapeutic potential of chrysin in cancer: Mechanistic perspectives. Toxicol. Lett. 233 (2), 214–225. doi:10.1016/j.toxlet.2015.01.008
Keating, S. T., Groh, L., van der Heijden, C. D. C. C., Rodriguez, H., Dos Santos, D. C., Fanucchi, S., et al. (2020). Rewiring of glucose metabolism defines trained immunity induced by oxidized low-density lipoprotein. J. Mol. Med. Berl. 98 (6), 819–831. doi:10.1007/s00109-020-01915-w
Kelley, T. W., Graham, M. M., Doseff, A. I., Pomerantz, R. W., Lau, S. M., Ostrowski, M. C., et al. (1999). Macrophage colony-stimulating factor promotes cell survival through Akt/protein kinase B. J. Biol. Chem. 274 (37), 26393–26398. doi:10.1074/jbc.274.37.26393
Kesharwani, S. S., and Bhat, G. J. (2020). Formulation and nanotechnology-based approaches for solubility and bioavailability enhancement of zerumbone. Med. Kaunas. 56 (11), 557. doi:10.3390/medicina56110557
Khokha, R., Murthy, A., and Weiss, A. (2013). Metalloproteinases and their natural inhibitors in inflammation and immunity. Nat. Rev. Immunol. 13 (9), 649–665. doi:10.1038/nri3499
Khoo, H. E., Azlan, A., Tang, S. T., and Lim, S. M. (2017). Anthocyanidins and anthocyanins: Colored pigments as food, pharmaceutical ingredients, and the potential health benefits. Food Nutr. Res. 61 (1), 1361779. doi:10.1080/16546628.2017.1361779
Kim, D., Kim, Y., and Kim, Y. (2019). Effects of β-carotene on expression of selected MicroRNAs, histone acetylation, and DNA methylation in colon cancer stem cells. J. Cancer Prev. 24 (4), 224–232. doi:10.15430/jcp.2019.24.4.224
Kim, H., Ramirez, C. N., Su, Z. Y., and Kong, A. N. T. (2016). Epigenetic modifications of triterpenoid ursolic acid in activating Nrf2 and blocking cellular transformation of mouse epidermal cells. J. Nutr. Biochem. 33, 54–62. doi:10.1016/j.jnutbio.2015.09.014
Kim, J., Byeon, H., Im, K., and Min, H. (2018). Effects of ginsenosides on regulatory T cell differentiation. Food Sci. Biotechnol. 27 (1), 227–232. doi:10.1007/s10068-017-0255-3
Kim, J. H., Yi, Y. S., Kim, M. Y., and Cho, J. Y. (2017). Role of ginsenosides, the main active components of Panax ginseng, in inflammatory responses and diseases. J. Ginseng Res. 41 (4), 435–443. doi:10.1016/j.jgr.2016.08.004
Kim, R. E., Shin, C. Y., Han, S. H., and Kwon, K. J. (2020). Astaxanthin suppresses pm2.5-induced neuroinflammation by regulating Akt phosphorylation in BV-2 microglial cells. Int. J. Mol. Sci. 21 (19), 7227. doi:10.3390/ijms21197227
Kishimoto, Y., Tani, M., Uto-Kondo, H., Iizuka, M., Saita, E., Sone, H., et al. (2010). Astaxanthin suppresses scavenger receptor expression and matrix metalloproteinase activity in macrophages. Eur. J. Nutr. 49 (2), 119–126. doi:10.1007/s00394-009-0056-4
Kiss, R. S., Maric, J., and Marcel, Y. L. (2005). Lipid efflux in human and mouse macrophagic cells: Evidence for differential regulation of phospholipid and cholesterol efflux. J. Lipid Res. 46 (9), 1877–1887. doi:10.1194/jlr.M400482-JLR200
Kleinnijenhuis, J., Quintin, J., Preijers, F., Joosten, L. A. B., Ifrim, D. C., Saeed, S., et al. (2012). Bacille Calmette-Guerin induces NOD2-dependent nonspecific protection from reinfection via epigenetic reprogramming of monocytes. Proc. Natl. Acad. Sci. U. S. A. 109 (43), 17537–17542. doi:10.1073/pnas.1202870109
Koh, Y. C., Yang, G., Lai, C. S., Weerawatanakorn, M., and Pan, M. H. (2018). Chemopreventive effects of phytochemicals and medicines on M1/M2 polarized macrophage role in inflammation-related diseases. Int. J. Mol. Sci. 19 (8), 2208. doi:10.3390/ijms19082208
Kong, W., Wei, J., Abidi, P., Lin, M., Inaba, S., Li, C., et al. (2004). Berberine is a novel cholesterol-lowering drug working through a unique mechanism distinct from statins. Nat. Med. 10 (12), 1344–1351. doi:10.1038/nm1135
Kotani, H., Tanabe, H., Mizukami, H., Makishima, M., and Inoue, M. (2010). Identification of a naturally occurring rexinoid, honokiol, that activates the retinoid X receptor. J. Nat. Prod. 73 (8), 1332–1336. doi:10.1021/np100120c
Kou, J. Y., Li, Y., Zhong, Z. Y., Jiang, Y. Q., Li, X. S., Han, X. B., et al. (2017). Berberine-sonodynamic therapy induces autophagy and lipid unloading in macrophage. Cell. Death Dis. 8 (1), e2558. doi:10.1038/cddis.2016.354
Kou, M. C., Chiou, S. Y., Weng, C. Y., Wang, L., Ho, C. T., and Wu, M. J. (2013). Curcuminoids distinctly exhibit antioxidant activities and regulate expression of scavenger receptors and heme oxygenase-1. Mol. Nutr. Food Res. 57 (9), 1598–1610. doi:10.1002/mnfr.201200227
Krinsky, N. I., and Johnson, E. J. (2005). Carotenoid actions and their relation to health and disease. Mol. Asp. Med. 26 (6), 459–516. doi:10.1016/j.mam.2005.10.001
Kumar, S., and Pandey, A. K. (2013). Chemistry and biological activities of flavonoids: An overview. ScientificWorldJournal 2013, 162750. doi:10.1155/2013/162750
Langmann, T., Liebisch, G., Moehle, C., Schifferer, R., Dayoub, R., Heiduczek, S., et al. (2005). Gene expression profiling identifies retinoids as potent inducers of macrophage lipid efflux. Biochim. Biophys. Acta 1740 (2), 155–161. doi:10.1016/j.bbadis.2004.11.016
Lee, S. H., Lee, J. H., Lee, H. Y., and Min, K. J. (2019). Sirtuin signaling in cellular senescence and aging. BMB Rep. 52 (1), 24–34. doi:10.5483/BMBRep.2019.52.1.290
Lee, T. S., Pan, C. C., Peng, C. C., Kou, Y. R., Chen, C. Y., Ching, L. C., et al. (2010). Anti-atherogenic effect of berberine on LXRalpha-ABCA1-dependent cholesterol efflux in macrophages. J. Cell. Biochem. 111 (1), 104–110. doi:10.1002/jcb.22667
Leentjens, J., Bekkering, S., Joosten, L. A. B., Netea, M. G., Burgner, D. P., and Riksen, N. P. (2018). Trained innate immunity as a novel mechanism linking infection and the development of atherosclerosis. Circ. Res. 122 (5), 664–669. doi:10.1161/circresaha.117.312465
Leng, S., Iwanowycz, S., Saaoud, F., Wang, J., Wang, Y., Sergin, I., et al. (2016). Ursolic acid enhances macrophage autophagy and attenuates atherogenesis. J. Lipid Res. 57 (6), 1006–1016. doi:10.1194/jlr.M065888
Li, C., and Schluesener, H. (2017). Health-promoting effects of the citrus flavanone hesperidin. Crit. Rev. Food Sci. Nutr. 57 (3), 613–631. doi:10.1080/10408398.2014.906382
Li, C., Wang, Q., Ren, T., Zhang, Y., Lam, C. W. K., Chow, M. S. S., et al. (2016). Non-linear pharmacokinetics of piperine and its herb-drug interactions with docetaxel in Sprague-Dawley rats. J. Pharm. Biomed. Anal. 128, 286–293. doi:10.1016/j.jpba.2016.05.041
Li, C. H., Gong, D., Chen, L. Y., Zhang, M., Xia, X. D., Cheng, H. P., et al. (2017). Puerarin promotes ABCA1-mediated cholesterol efflux and decreases cellular lipid accumulation in THP-1 macrophages. Eur. J. Pharmacol. 811, 74–86. doi:10.1016/j.ejphar.2017.05.055
Li, J., Xie, Z. Z., Tang, Y. B., Zhou, J. G., and Guan, Y. Y. (2011). Ginsenoside-Rd, a purified component from panax notoginseng saponins, prevents atherosclerosis in apoE knockout mice. Eur. J. Pharmacol. 652 (1-3), 104–110. doi:10.1016/j.ejphar.2010.11.017
Li, L., Ni, J., Li, M., Chen, J., Han, L., Zhu, Y., et al. (2017). Ginsenoside Rg3 micelles mitigate doxorubicin-induced cardiotoxicity and enhance its anticancer efficacy. Drug Deliv. 24 (1), 1617–1630. doi:10.1080/10717544.2017.1391893
Li, Q., Bao, J. M., Li, X. L., Zhang, T., and Shen, X. h. (2012). Inhibiting effect of Astragalus polysaccharides on the functions of CD4+CD25 highTreg cells in the tumor microenvironment of human hepatocellular carcinoma. Chin. Med. J. Engl. 125 (5), 786–793.
Li, S., Jiao, Y., Wang, H., Shang, Q., Lu, F., Huang, L., et al. (2017). Sodium tanshinone IIA sulfate adjunct therapy reduces high-sensitivity C-reactive protein level in coronary artery disease patients: A randomized controlled trial. Sci. Rep. 7 (1), 17451. doi:10.1038/s41598-017-16980-4
Li, W., Sargsyan, D., Wu, R., Li, S., Wang, L., Cheng, D., et al. (2019). DNA methylome and transcriptome alterations in high glucose-induced diabetic nephropathy cellular model and identification of novel targets for treatment by tanshinone IIA. Chem. Res. Toxicol. 32 (10), 1977–1988. doi:10.1021/acs.chemrestox.9b00117
Li, X., Wu, J., Xu, F., Chu, C., Li, X., Shi, X., et al. (2022). Use of ferulic acid in the management of diabetes mellitus and its complications. Molecules 27 (18), 6010. doi:10.3390/molecules27186010
Li, X., Zhou, Y., Yu, C., Yang, H., Zhang, C., Ye, Y., et al. (2015). Paeonol suppresses lipid accumulation in macrophages via upregulation of the ATP-binding cassette transporter A1 and downregulation of the cluster of differentiation 36. Int. J. Oncol. 46 (2), 764–774. doi:10.3892/ijo.2014.2757
Liang, Z., Currais, A., Soriano-Castell, D., Schubert, D., and Maher, P. (2021). Natural products targeting mitochondria: Emerging therapeutics for age-associated neurological disorders. Pharmacol. Ther. 221, 107749. doi:10.1016/j.pharmthera.2020.107749
Liao, T., Ding, L., Wu, P., Zhang, L., Li, X., Xu, B., et al. (2020). Chrysin attenuates the NLRP3 inflammasome cascade to reduce synovitis and pain in KOA rats. Drug Des. Devel Ther. 14, 3015–3027. doi:10.2147/dddt.S261216
Libby, P. (2002). Inflammation in atherosclerosis. Nature 420 (6917), 868–874. doi:10.1038/nature01323
Lin, H. L., Cheng, W. T., Chen, L. C., Ho, H. O., Lin, S. Y., and Hsieh, C. M. (2021). Honokiol/magnolol-loaded self-assembling lecithin-based mixed polymeric micelles (lbMPMs) for improving solubility to enhance oral bioavailability. Int. J. Nanomedicine 16, 651–665. doi:10.2147/ijn.S290444
Lin, M. C., Ou, T. T., Chang, C. H., Chan, K. C., and Wang, C. J. (2015). Protocatechuic acid inhibits oleic acid-induced vascular smooth muscle cell proliferation through activation of AMP-activated protein kinase and cell cycle arrest in G0/G1 phase. J. Agric. Food Chem. 63 (1), 235–241. doi:10.1021/jf505303s
Lin, X. L., Liu, M. H., Hu, H. J., Feng, H. r., Fan, X. J., Zou, W. w., et al. (2015). Curcumin enhanced cholesterol efflux by upregulating ABCA1 expression through AMPK-SIRT1-LXRα signaling in THP-1 macrophage-derived foam cells. DNA Cell. Biol. 34 (9), 561–572. doi:10.1089/dna.2015.2866
Litviňuková, M., Talavera-López, C., Maatz, H., Reichart, D., Worth, C. L., Lindberg, E. L., et al. (2020). Cells of the adult human heart. Nature 588 (7838), 466–472. doi:10.1038/s41586-020-2797-4
Liu, B., and Yan, W. (2019). Lipophilization of EGCG and effects on antioxidant activities. Food Chem. 272, 663–669. doi:10.1016/j.foodchem.2018.08.086
Liu, C., Wang, W., Lin, W., Ling, W., and Wang, D. (2016). Established atherosclerosis might be a prerequisite for chicory and its constituent protocatechuic acid to promote endothelium-dependent vasodilation in mice. Mol. Nutr. Food Res. 60 (10), 2141–2150. doi:10.1002/mnfr.201600002
Liu, C. H., Zhang, W. D., Wang, J. J., and Feng, S. D. (2016). Senegenin ameliorate acute lung injury through reduction of oxidative stress and inhibition of inflammation in cecal ligation and puncture-induced sepsis rats. Inflammation 39 (2), 900–906. doi:10.1007/s10753-016-0322-6
Liu, N., Wu, C., Sun, L., Zheng, J., and Guo, P. (2014). Sesamin enhances cholesterol efflux in RAW264.7 macrophages. Molecules 19 (6), 7516–7527. doi:10.3390/molecules19067516
Liu, R., Li, J., Cheng, Y., Huo, T., Xue, J., Liu, Y., et al. (2015). Effects of ellagic acid-rich extract of pomegranates peel on regulation of cholesterol metabolism and its molecular mechanism in hamsters. Food Funct. 6 (3), 780–787. doi:10.1039/c4fo00759j
Liu, W. H., Lin, C. C., Wang, Z. H., Mong, M. C., and Yin, M. C. (2010). Effects of protocatechuic acid on trans fat induced hepatic steatosis in mice. J. Agric. Food Chem. 58 (18), 10247–10252. doi:10.1021/jf102379n
Liu, X., and Liu, C. (2017). Baicalin ameliorates chronic unpredictable mild stress-induced depressive behavior: Involving the inhibition of NLRP3 inflammasome activation in rat prefrontal cortex. Int. Immunopharmacol. 48, 30–34. doi:10.1016/j.intimp.2017.04.019
Liu, Z., Wang, J., Huang, E., Gao, S., Li, H., Lu, J., et al. (2014). Tanshinone IIA suppresses cholesterol accumulation in human macrophages: Role of heme oxygenase-1. J. Lipid Res. 55 (2), 201–213. doi:10.1194/jlr.M040394
Long, H., Lin, H., Zheng, P., Hou, L., Zhang, M., Lin, S., et al. (2022). WTAP mediates the anti-inflammatory effect of Astragalus mongholicus polysaccharide on THP-1 macrophages. Front. Pharmacol. 13, 1023878. doi:10.3389/fphar.2022.1023878
Lü, J. M., Yao, Q., and Chen, C. (2009). Ginseng compounds: An update on their molecular mechanisms and medical applications. Curr. Vasc. Pharmacol. 7 (3), 293–302. doi:10.2174/157016109788340767
Lu, Z., He, B., Chen, J., Wu, L. J., Chen, X. B., Ye, S. Q., et al. (2021). Optimisation of the conversion and extraction of arctigenin from fructus arctii into arctiin using fungi. Front. Microbiol. 12, 663116. doi:10.3389/fmicb.2021.663116
Luo, J., Chen, S., Wang, L., Zhao, X., and Piao, C. (2022). Pharmacological effects of polydatin in the treatment of metabolic diseases: A review. Phytomedicine 102, 154161. doi:10.1016/j.phymed.2022.154161
Lv, B., Chen, S., Tang, C., Jin, H., Du, J., and Huang, Y. (2021). Hydrogen sulfide and vascular regulation - an update. J. Adv. Res. 27, 85–97. doi:10.1016/j.jare.2020.05.007
Ma, J., Zheng, L., He, Y. S., and Li, H. J. (2015). Hepatotoxic assessment of Polygoni Multiflori Radix extract and toxicokinetic study of stilbene glucoside and anthraquinones in rats. J. Ethnopharmacol. 162, 61–68. doi:10.1016/j.jep.2014.12.045
Mallat, Z., Heymes, C., Ohan, J., Faggin, E., Leseche, G., and Tedgui, A. (1999). Expression of interleukin-10 in advanced human atherosclerotic plaques: Relation to inducible nitric oxide synthase expression and cell death. Arterioscler. Thromb. Vasc. Biol. 19 (3), 611–616. doi:10.1161/01.atv.19.3.611
Mani, R., and Natesan, V. (2018). Chrysin: Sources, beneficial pharmacological activities, and molecular mechanism of action. Phytochemistry 145, 187–196. doi:10.1016/j.phytochem.2017.09.016
Maxwell, T., Lee, K. S., Kim, S., and Nam, K. S. (2018). Arctigenin inhibits the activation of the mTOR pathway, resulting in autophagic cell death and decreased ER expression in ER-positive human breast cancer cells. Int. J. Oncol. 52 (4), 1339–1349. doi:10.3892/ijo.2018.4271
McCreight, L. J., Bailey, C. J., and Pearson, E. R. (2016). Metformin and the gastrointestinal tract. Diabetologia 59 (3), 426–435. doi:10.1007/s00125-015-3844-9
Mei, L., He, M., Zhang, C., Miao, J., Wen, Q., Liu, X., et al. (2019). Paeonol attenuates inflammation by targeting HMGB1 through upregulating miR-339-5p. Sci. Rep. 9 (1), 19370. doi:10.1038/s41598-019-55980-4
Mensah, G. A., Roth, G. A., and Fuster, V. (2019). The global burden of cardiovascular diseases and risk factors: 2020 and beyond. J. Am. Coll. Cardiol. 74 (20), 2529–2532. doi:10.1016/j.jacc.2019.10.009
Millar, C. L., Duclos, Q., and Blesso, C. N. (2017). Effects of dietary flavonoids on reverse cholesterol transport, HDL metabolism, and HDL function. Adv. Nutr. 8 (2), 226–239. doi:10.3945/an.116.014050
Mills, E. L., Kelly, B., Logan, A., Costa, A. S. H., Varma, M., Bryant, C. E., et al. (2016). Succinate dehydrogenase supports metabolic repurposing of mitochondria to drive inflammatory macrophages. Cell. 167 (2), 457–470. doi:10.1016/j.cell.2016.08.064
Milutinović, B., and Kurtz, J. (2016). Immune memory in invertebrates. Semin. Immunol. 28 (4), 328–342. doi:10.1016/j.smim.2016.05.004
Min, K. J., Um, H. J., Cho, K. H., and Kwon, T. K. (2013). Curcumin inhibits oxLDL-induced CD36 expression and foam cell formation through the inhibition of p38 MAPK phosphorylation. Food Chem. Toxicol. 58, 77–85. doi:10.1016/j.fct.2013.04.008
Mitroulis, I., Ruppova, K., Wang, B., Chen, L. S., Grzybek, M., Grinenko, T., et al. (2018). Modulation of myelopoiesis progenitors is an integral component of trained immunity. Cell. 172 (1-2), 147–161. doi:10.1016/j.cell.2017.11.034
Moore, K. J., Sheedy, F. J., and Fisher, E. A. (2013). Macrophages in atherosclerosis: A dynamic balance. Nat. Rev. Immunol. 13 (10), 709–721. doi:10.1038/nri3520
Moore, K. J., and Tabas, I. (2011). Macrophages in the pathogenesis of atherosclerosis. Cell. 145 (3), 341–355. doi:10.1016/j.cell.2011.04.005
Moran, N. E., Mohn, E. S., Hason, N., Erdman, J. W., and Johnson, E. J. (2018). Intrinsic and extrinsic factors impacting absorption, metabolism, and health effects of dietary carotenoids. Adv. Nutr. 9 (4), 465–492. doi:10.1093/advances/nmy025
Morioka, S., Perry, J. S. A., Raymond, M. H., Medina, C. B., Zhu, Y., Zhao, L., et al. (2018). Efferocytosis induces a novel SLC program to promote glucose uptake and lactate release. Nature 563 (7733), 714–718. doi:10.1038/s41586-018-0735-5
Muhammad, T., Ikram, M., Ullah, R., Rehman, S. U., and Kim, M. O. (2019). Hesperetin, a citrus flavonoid, attenuates LPS-induced neuroinflammation, apoptosis and memory impairments by modulating TLR4/NF-κB signaling. Nutrients 11 (3), 648. doi:10.3390/nu11030648
Mulder, W. J. M., Ochando, J., Joosten, L. A. B., Fayad, Z. A., and Netea, M. G. (2019). Therapeutic targeting of trained immunity. Nat. Rev. Drug Discov. 18 (7), 553–566. doi:10.1038/s41573-019-0025-4
Nah, S. Y., Kim, D. H., and Rhim, H. (2007). Ginsenosides: Are any of them candidates for drugs acting on the central nervous system? CNS Drug Rev. 13 (4), 381–404. doi:10.1111/j.1527-3458.2007.00023.x
Nam, G. S., and Nam, K-S. (2020). Arctigenin attenuates platelet activation and clot retraction by regulation of thromboxane A2 synthesis and cAMP pathway. Biomed. Pharmacother. 130, 110535. doi:10.1016/j.biopha.2020.110535
Napoli, C., de Nigris, F., Williams-Ignarro, S., Pignalosa, O., Sica, V., and Ignarro, L. J. (2006). Nitric oxide and atherosclerosis: An update. Nitric Oxide 15 (4), 265–279. doi:10.1016/j.niox.2006.03.011
Napolitano, M., De Pascale, C., Wheeler-Jones, C., Botham, K. M., and Bravo, E. (2007). Effects of lycopene on the induction of foam cell formation by modified LDL. Am. J. Physiol. Endocrinol. Metab. 293 (6), E1820–E1827. doi:10.1152/ajpendo.00315.2007
Nelson, K. M., Dahlin, J. L., Bisson, J., Graham, J., Pauli, G. F., and Walters, M. A. (2017). The essential medicinal chemistry of curcumin. J. Med. Chem. 60 (5), 1620–1637. doi:10.1021/acs.jmedchem.6b00975
Netea, M. G., Domínguez-Andrés, J., Barreiro, L. B., Chavakis, T., Divangahi, M., Fuchs, E., et al. (2020). Defining trained immunity and its role in health and disease. Nat. Rev. Immunol. 20 (6), 375–388. doi:10.1038/s41577-020-0285-6
Neumann, H. P. H., and Young, W. F. (2019). Pheochromocytoma and paraganglioma. N. Engl. J. Med. 381 (6), 552–565. doi:10.1056/NEJMra1806651
Newman, D. J., and Cragg, G. M. (2020). Natural products as sources of new drugs over the nearly four decades from 01/1981 to 09/2019. J. Nat. Prod. 83 (3), 770–803. doi:10.1021/acs.jnatprod.9b01285
Ni, S. Y., Zhong, X. L., Li, Z. H., Huang, D. J., Xu, W. T., Zhou, Y., et al. (2020). Puerarin alleviates lipopolysaccharide-induced myocardial fibrosis by inhibiting PARP-1 to prevent HMGB1-mediated TLR4-NF-κb signaling pathway. Cardiovasc Toxicol. 20 (5), 482–491. doi:10.1007/s12012-020-09571-9
Niu, L., Luo, R., Zou, M., Sun, Y., Fu, Y., Wang, Y., et al. (2020). Puerarin inhibits Mycoplasma gallisepticum (MG-HS)-induced inflammation and apoptosis via suppressing the TLR6/MyD88/NF-κB signal pathway in chicken. Int. Immunopharmacol. 88, 106993. doi:10.1016/j.intimp.2020.106993
Nogara, L., Naber, N., Pate, E., Canton, M., Reggiani, C., and Cooke, R. (2016). Piperine's mitigation of obesity and diabetes can be explained by its up-regulation of the metabolic rate of resting muscle. Proc. Natl. Acad. Sci. U. S. A. 113 (46), 13009–13014. doi:10.1073/pnas.1607536113
Noh, K., Oh do, G., Nepal, M. R., Jeong, K. S., Choi, Y., Kang, M. J., et al. (2016). Pharmacokinetic interaction of chrysin with caffeine in rats. Biomol. Ther. Seoul. 24 (4), 446–452. doi:10.4062/biomolther.2015.197
Ono, K. (2018). Alzheimer's disease as oligomeropathy. Neurochem. Int. 119, 57–70. doi:10.1016/j.neuint.2017.08.010
Pajak, B., Siwiak, E., Sołtyka, M., Priebe, A., Zielinski, R., Fokt, I., et al. (2019). 2-Deoxy-d-Glucose and its analogs: From diagnostic to therapeutic agents. Int. J. Mol. Sci. 21 (1), 234. doi:10.3390/ijms21010234
Palozza, P., Simone, R., Catalano, A., Parrone, N., Monego, G., and Ranelletti, F. O. (2011). Lycopene regulation of cholesterol synthesis and efflux in human macrophages. J. Nutr. Biochem. 22 (10), 971–978. doi:10.1016/j.jnutbio.2010.08.010
Pan, J., Jin, J. L., Ge, H. M., Yin, K. l., Chen, X., Han, L. j., et al. (2015). Malibatol A regulates microglia M1/M2 polarization in experimental stroke in a PPARγ-dependent manner. J. Neuroinflammation 12, 51. doi:10.1186/s12974-015-0270-3
Parathath, S., Grauer, L., Huang, L. S., Sanson, M., Distel, E., Goldberg, I. J., et al. (2011). Diabetes adversely affects macrophages during atherosclerotic plaque regression in mice. Diabetes 60 (6), 1759–1769. doi:10.2337/db10-0778
Park, D. W., Baek, K., Kim, J. R., Lee, J. J., Ryu, S. H., Chin, B. R., et al. (2009). Resveratrol inhibits foam cell formation via NADPH oxidase 1- mediated reactive oxygen species and monocyte chemotactic protein-1. Exp. Mol. Med. 41 (3), 171–179. doi:10.3858/emm.2009.41.3.020
Park, S. H., Kim, J. L., Lee, E. S., Han, S. Y., Gong, J. H., Kang, M. K., et al. (2011). Dietary ellagic acid attenuates oxidized LDL uptake and stimulates cholesterol efflux in murine macrophages. J. Nutr. 141 (11), 1931–1937. doi:10.3945/jn.111.144816
Park, U. H., Hwang, J. T., Youn, H., Kim, E. J., and Um, S. J. (2019). Piperine inhibits adipocyte differentiation via dynamic regulation of histone modifications. Phytother. Res. 33 (9), 2429–2439. doi:10.1002/ptr.6434
Persico, G., Casciaro, F., Marinelli, A., Tonelli, C., Petroni, K., and Giorgio, M. (2021). Comparative analysis of histone H3K4me3 distribution in mouse liver in different diets reveals the epigenetic efficacy of cyanidin-3-O-glucoside dietary intake. Int. J. Mol. Sci. 22 (12), 6503. doi:10.3390/ijms22126503
Peterson, J., Dwyer, J., Adlercreutz, H., Scalbert, A., Jacques, P., and McCullough, M. L. (2010). Dietary lignans: Physiology and potential for cardiovascular disease risk reduction. Nutr. Rev. 68 (10), 571–603. doi:10.1111/j.1753-4887.2010.00319.x
Pillai, V. B., Samant, S., Sundaresan, N. R., Raghuraman, H., Kim, G., Bonner, M. Y., et al. (2015). Honokiol blocks and reverses cardiac hypertrophy in mice by activating mitochondrial Sirt3. Nat. Commun. 6, 6656. doi:10.1038/ncomms7656
Porrini, L., Cybulski, L. E., Altabe, S. G., Mansilla, M. C., and de Mendoza, D. (2014). Cerulenin inhibits unsaturated fatty acids synthesis in Bacillus subtilis by modifying the input signal of DesK thermosensor. Microbiologyopen 3 (2), 213–224. doi:10.1002/mbo3.154
Qiao, L., Zhang, X., Liu, M., Liu, X., Dong, M., Cheng, J., et al. (2017). Ginsenoside Rb1 enhances atherosclerotic plaque stability by improving autophagy and lipid metabolism in macrophage foam cells. Front. Pharmacol. 8, 727. doi:10.3389/fphar.2017.00727
Quintin, J., Saeed, S., Martens, J. H. A., Giamarellos-Bourboulis, E. J., Ifrim, D. C., Logie, C., et al. (2012). Candida albicans infection affords protection against reinfection via functional reprogramming of monocytes. Cell. Host Microbe 12 (2), 223–232. doi:10.1016/j.chom.2012.06.006
Rašković, A., Stilinović, N., Kolarović, J., Vasovic, V., Vukmirovic, S., and Mikov, M. (2011). The protective effects of silymarin against doxorubicin-induced cardiotoxicity and hepatotoxicity in rats. Molecules 16 (10), 8601–8613. doi:10.3390/molecules16108601
Renner, K., Singer, K., Koehl, G. E., Geissler, E. K., Peter, K., Siska, P. J., et al. (2017). Metabolic hallmarks of tumor and immune cells in the tumor microenvironment. Front. Immunol. 8, 248. doi:10.3389/fimmu.2017.00248
Ridker, P. M., Everett, B. M., Thuren, T., MacFadyen, J. G., Chang, W. H., Ballantyne, C., et al. (2017). Antiinflammatory therapy with canakinumab for atherosclerotic disease. N. Engl. J. Med. 377 (12), 1119–1131. doi:10.1056/NEJMoa1707914
Riham, I. E-G., Gaber, S. A. A., and Nasr, M. (2019). Polymeric nanocapsular baicalin: Chemometric optimization, physicochemical characterization and mechanistic anticancer approaches on breast cancer cell lines. Sci. Rep. 9 (1), 11064. doi:10.1038/s41598-019-47586-7
Rodriguez, A. E., Ducker, G. S., Billingham, L. K., Martinez, C. A., Mainolfi, N., Suri, V., et al. (2019). Serine metabolism supports macrophage IL-1β production. Cell. Metab. 29 (4), 1003–1011. doi:10.1016/j.cmet.2019.01.014
Rong, Z. J., Cai, H. H., Wang, H., Liu, G. H., Zhang, Z. W., Chen, M., et al. (2022). Ursolic acid ameliorates spinal cord injury in mice by regulating gut microbiota and metabolic changes. Front. Cell. Neurosci. 16, 872935. doi:10.3389/fncel.2022.872935
Rosa, A., Caprioglio, D., Isola, R., Appendino, G., and Falchi, A. M. (2019). Dietary zerumbone from shampoo ginger: New insights into its antioxidant and anticancer activity. Food Funct. 10 (3), 1629–1642. doi:10.1039/c8fo02395f
Russo, M., Spagnuolo, C., Tedesco, I., Bilotto, S., and Russo, G. L. (2012). The flavonoid quercetin in disease prevention and therapy: Facts and fancies. Biochem. Pharmacol. 83 (1), 6–15. doi:10.1016/j.bcp.2011.08.010
Saeed, S., Quintin, J., Kerstens, H. H., Rao, N. A., Aghajanirefah, A., Matarese, F., et al. (2014). Epigenetic programming of monocyte-to-macrophage differentiation and trained innate immunity. Science 345 (6204), 1251086. doi:10.1126/science.1251086
Salunkhe, R., Gadgoli, C., Naik, A., and Patil, N. (2021). Pharmacokinetic profile and oral bioavailability of diosgenin, charantin, and hydroxychalcone from a polyherbal formulation. Front. Pharmacol. 12, 629272. doi:10.3389/fphar.2021.629272
Santana-Gálvez, J., Cisneros-Zevallos, L., and Jacobo-Velázquez, D. A. (2017). Chlorogenic acid: Recent advances on its dual role as a food additive and a nutraceutical against metabolic syndrome. Molecules 22 (3), 358. doi:10.3390/molecules22030358
Saqib, U., Sarkar, S., Suk, K., Mohammad, O., Baig, M. S., and Savai, R. (2018). Phytochemicals as modulators of M1-M2 macrophages in inflammation. Oncotarget 9 (25), 17937–17950. doi:10.18632/oncotarget.24788
Sarrazy, V., Viaud, M., Westerterp, M., Ivanov, S., Giorgetti-Peraldi, S., Guinamard, R., et al. (2016). Disruption of Glut1 in hematopoietic stem cells prevents myelopoiesis and enhanced glucose flux in atheromatous plaques of ApoE(-/-) mice. Circ. Res. 118 (7), 1062–1077. doi:10.1161/circresaha.115.307599
`Sarubbo, F., Moranta, D., Asensio, V. J., Miralles, A., and Esteban, S. (2017). Effects of resveratrol and other polyphenols on the most common brain age-related diseases. Curr. Med. Chem. 24 (38), 4245–4266. doi:10.2174/0929867324666170724102743
Saz-Leal, P., Del Fresno, C., Brandi, P., Martinez-Cano, S., Dungan, O. M., Chisholm, J. D., et al. (2018). Targeting SHIP-1 in myeloid cells enhances trained immunity and boosts response to infection. Cell. Rep. 25 (5), 1118–1126. doi:10.1016/j.celrep.2018.09.092
Scalbert, A., Manach, C., Morand, C., Remesy, C., and Jimenez, L. (2005). Dietary polyphenols and the prevention of diseases. Crit. Rev. Food Sci. Nutr. 45 (4), 287–306. doi:10.1080/1040869059096
Schrader, C., Ernst, I. M., Sinnecker, H., Soukup, S. T., Kulling, S. E., and Rimbach, G. (2012). Genistein as a potential inducer of the anti-atherogenic enzyme paraoxonase-1: Studies in cultured hepatocytes in vitro and in rat liver in vivo. J. Cell. Mol. Med. 16 (10), 2331–2341. doi:10.1111/j.1582-4934.2012.01542.x
Schwartz, K., Lawn, R. M., and Wade, D. P. (2000). ABC1 gene expression and ApoA-I-mediated cholesterol efflux are regulated by LXR. Biochem. Biophys. Res. Commun. 274 (3), 794–802. doi:10.1006/bbrc.2000.3243
Secretan, P. H., Thirion, O., Sadou Yayé, H., Damy, T., Astier, A., Paul, M., et al. (2021). Simple approach to enhance green tea epigallocatechin gallate stability in aqueous solutions and bioavailability: Experimental and theoretical characterizations. Pharm. (Basel) 14 (12), 1242. doi:10.3390/ph14121242
Sharifiyan, F., Movahedian-Attar, A., Nili, N., and Asgary, S. (2016). Study of pomegranate (Punica granatum L.) peel extract containing anthocyanins on fatty streak formation in the renal arteries in hypercholesterolemic rabbits. Adv. Biomed. Res. 5, 8. doi:10.4103/2277-9175.175241
Sheedy, F. J., Grebe, A., Rayner, K. J., Kalantari, P., Ramkhelawon, B., Carpenter, S. B., et al. (2013). CD36 coordinates NLRP3 inflammasome activation by facilitating intracellular nucleation of soluble ligands into particulate ligands in sterile inflammation. Nat. Immunol. 14 (8), 812–820. doi:10.1038/ni.2639
Sheehan, A. L., Carrell, S., Johnson, B., Stanic, B., Banfi, B., and Miller, F. J. (2011). Role for Nox1 NADPH oxidase in atherosclerosis. Atherosclerosis 216 (2), 321–326. doi:10.1016/j.atherosclerosis.2011.02.028
Shirai, T., Nazarewicz, R. R., Wallis, B. B., Yanes, R. E., Watanabe, R., Hilhorst, M., et al. (2016). The glycolytic enzyme PKM2 bridges metabolic and inflammatory dysfunction in coronary artery disease. J. Exp. Med. 213 (3), 337–354. doi:10.1084/jem.20150900
Simental-Mendía, L. E., and Guerrero-Romero, F. (2019). Effect of resveratrol supplementation on lipid profile in subjects with dyslipidemia: A randomized double-blind, placebo-controlled trial. Nutrition 58, 7–10. doi:10.1016/j.nut.2018.06.015
Sohrabi, Y., Lagache, S. M. M., Schnack, L., Godfrey, R., Kahles, F., Bruemmer, D., et al. (2018). mTOR-dependent oxidative stress regulates oxLDL-induced trained innate immunity in human monocytes. Front. Immunol. 9, 3155. doi:10.3389/fimmu.2018.03155
Song, I. S., Nam, S. J., Jeon, J. H., Park, S. J., and Choi, M. K. (2021). Enhanced bioavailability and efficacy of silymarin solid dispersion in rats with acetaminophen-induced hepatotoxicity. Pharmaceutics 13 (5), 628. doi:10.3390/pharmaceutics13050628
Song, S., Gao, K., Niu, R., Wang, J., Zhang, J., Gao, C., et al. (2020). Inclusion complexes between chrysin and amino-appended β-cyclodextrins (ACDs): Binding behavior, water solubility, in vitro antioxidant activity and cytotoxicity. Mater Sci. Eng. C Mater Biol. Appl. 106, 110161. doi:10.1016/j.msec.2019.110161
Song, X., Gong, Z., Liu, K., Kou, J., and Liu, B. (2020). Baicalin combats glutamate excitotoxicity via protecting glutamine synthetase from ROS-induced 20S proteasomal degradation. Redox Biol. 34, 101559. doi:10.1016/j.redox.2020.101559
Sosić-Jurjević, B., Filipović, B., Ajdzanović, V., Brkic, D., Ristic, N., Stojanoski, M. M., et al. (2007). Subcutaneously administrated genistein and daidzein decrease serum cholesterol and increase triglyceride levels in male middle-aged rats. Exp. Biol. Med. (Maywood) 232 (9), 1222–1227. doi:10.3181/0703-bc-82
Srinivasan, K. (2007). Black pepper and its pungent principle-piperine: A review of diverse physiological effects. Crit. Rev. Food Sci. Nutr. 47 (8), 735–748. doi:10.1080/10408390601062054
Stiekema, L. C. A., Prange, K. H. M., Hoogeveen, R. M., Verweij, S. L., Kroon, J., Schnitzler, J. G., et al. (2020). Potent lipoprotein(a) lowering following apolipoprotein(a) antisense treatment reduces the pro-inflammatory activation of circulating monocytes in patients with elevated lipoprotein(a). Eur. Heart J. 41 (24), 2262–2271. doi:10.1093/eurheartj/ehaa171
Stompor-Gorący, M., and Machaczka, M. (2021). Recent advances in biological activity, new formulations and prodrugs of ferulic acid. Int. J. Mol. Sci. 22 (23), 12889. doi:10.3390/ijms222312889
Stumpf, C., Fan, Q., Hintermann, C., Raaz, D., Kurfurst, I., Losert, S., et al. (2013). Anti-inflammatory effects of danshen on human vascular endothelial cells in culture. Am. J. Chin. Med. 41 (5), 1065–1077. doi:10.1142/s0192415x13500729
Su, C. C., Wang, S. C., Chen, I. C., Chiu, F. Y., Liu, P. L., Huang, C. H., et al. (2021). Zerumbone suppresses the LPS-induced inflammatory response and represses activation of the NLRP3 inflammasome in macrophages. Front. Pharmacol. 12, 652860. doi:10.3389/fphar.2021.652860
Sun, F., Yang, X., Ma, C., Zhang, S., Yu, L., Lu, H., et al. (2021). The effects of diosgenin on hypolipidemia and its underlying mechanism: A review. Diabetes Metab. Syndr. Obes. 14, 4015–4030. doi:10.2147/dmso.S326054
Sun, J., Wei, S., Zhang, Y., and Li, J. (2021). Protective effects of Astragalus polysaccharide on sepsis-induced acute kidney injury. Anal. Cell. Pathol. (Amst) 2021, 7178253. doi:10.1155/2021/7178253
Sun, L., Li, E., Wang, F., Wang, T., Qin, Z., Niu, S., et al. (2015). Quercetin increases macrophage cholesterol efflux to inhibit foam cell formation through activating PPARγ-ABCA1 pathway. Int. J. Clin. Exp. Pathol. 8 (9), 10854–10860.
Sun, Y., Lu, Y., Saredy, J., Wang, X., Drummer Iv, C., Shao, Y., et al. (2020). ROS systems are a new integrated network for sensing homeostasis and alarming stresses in organelle metabolic processes. Redox Biol. 37, 101696. doi:10.1016/j.redox.2020.101696
Szabó, R., Rácz, C. P., and Dulf, F. V. (2022). Bioavailability improvement strategies for icariin and its derivates: A review. Int. J. Mol. Sci. 23 (14), 7519. doi:10.3390/ijms23147519
Tabas, I., and Bornfeldt, K. E. (2020). Intracellular and intercellular aspects of macrophage immunometabolism in atherosclerosis. Circ. Res. 126 (9), 1209–1227. doi:10.1161/circresaha.119.315939
Tall, A. R., and Yvan-Charvet, L. (2015). Cholesterol, inflammation and innate immunity. Nat. Rev. Immunol. 15 (2), 104–116. doi:10.1038/nri3793
Tang, F. T., Cao, Y., Wang, T. Q., Wang, L. J., Guo, J., Zhou, X. S., et al. (2011). Tanshinone IIA attenuates atherosclerosis in ApoE(-/-) mice through down-regulation of scavenger receptor expression. Eur. J. Pharmacol. 650 (1), 275–284. doi:10.1016/j.ejphar.2010.07.038
Tang, Y., Wa, Q., Peng, L., Zheng, Y., Chen, J., Chen, X., et al. (2022). Salvianolic acid B suppresses ER stress-induced NLRP3 inflammasome and pyroptosis via the AMPK/FoxO4 and syndecan-4/rac1 signaling pathways in human endothelial progenitor cells. Oxid. Med. Cell. Longev. 2022, 8332825. doi:10.1155/2022/8332825
Tardif, J. C., Kouz, S., Waters, D. D., Bertrand, O. F., Diaz, R., Maggioni, A. P., et al. (2019). Efficacy and safety of low-dose colchicine after myocardial infarction. N. Engl. J. Med. 381 (26), 2497–2505. doi:10.1056/NEJMoa1912388
Tawakol, A., Singh, P., Mojena, M., Pimentel-Santillana, M., Emami, H., MacNabb, M., et al. (2015). HIF-1α and PFKFB3 mediate a tight relationship between proinflammatory activation and anerobic metabolism in atherosclerotic macrophages. Arterioscler. Thromb. Vasc. Biol. 35 (6), 1463–1471. doi:10.1161/atvbaha.115.305551
Tomoda, H., Kawaguchi, A., Omura, S., and Okuda, S. (1984). Cerulenin resistance in a cerulenin-producing fungus. II. Characterization of fatty acid synthetase from Cephalosporium caerulens. J. Biochem. 95 (6), 1705–1712. doi:10.1093/oxfordjournals.jbchem.a134784
Trichard, L., Fattal, E., Besnard, M., and Bochot, A. (2007). Alpha-cyclodextrin/oil beads as a new carrier for improving the oral bioavailability of lipophilic drugs. J. Control Release 122 (1), 47–53. doi:10.1016/j.jconrel.2007.06.004
Ui, M., Okada, T., Hazeki, K., and Hazeki, O. (1995). Wortmannin as a unique probe for an intracellular signalling protein, phosphoinositide 3-kinase. Trends Biochem. Sci. 20 (8), 303–307. doi:10.1016/s0968-0004(00)89056-8
Um, M. Y., Ahn, J., and Ha, T. Y. (2013). Hypolipidaemic effects of cyanidin 3-glucoside rich extract from black rice through regulating hepatic lipogenic enzyme activities. J. Sci. Food Agric. 93 (12), 3126–3128. doi:10.1002/jsfa.6070
Uto-Kondo, H., Ayaori, M., Ogura, M., Nakaya, K., Ito, M., Suzuki, A., et al. (2010). Coffee consumption enhances high-density lipoprotein-mediated cholesterol efflux in macrophages. Circ. Res. 106 (4), 779–787. doi:10.1161/circresaha.109.206615
Van den Bossche, J., Baardman, J., Otto, N. A., van der Velden, S., Neele, A. E., van den Berg, S. M., et al. (2016). Mitochondrial dysfunction prevents repolarization of inflammatory macrophages. Cell. Rep. 17 (3), 684–696. doi:10.1016/j.celrep.2016.09.008
van der Heijden, C., Groh, L., Keating, S. T., Kaffa, C., Noz, M. P., Kersten, S., et al. (2020). Catecholamines induce trained immunity in monocytes in vitro and in vivo. Circ. Res. 127 (2), 269–283. doi:10.1161/circresaha.119.315800
van der Heijden, C., Keating, S. T., Groh, L., Joosten, L. A. B., Netea, M. G., and Riksen, N. P. (2020). Aldosterone induces trained immunity: The role of fatty acid synthesis. Cardiovasc Res. 116 (2), 317–328. doi:10.1093/cvr/cvz137
van der Heijden, C., Smeets, E. M. M., Aarntzen, E., Noz, M. P., Monajemi, H., Kersten, S., et al. (2020). Arterial wall inflammation and increased hematopoietic activity in patients with primary aldosteronism. J. Clin. Endocrinol. Metab. 105 (5), e1967–e1980. doi:10.1210/clinem/dgz306
van der Heijden, C. D. C. C., Keating, S. T., Groh, L., Joosten, L. A. B., Netea, M. G., and Riksen, N. P. (2019). Aldosterone induces trained immunity: The role of fatty acid synthesis. Cardiovasc. Res. 116 (2), 317–328. doi:10.1093/cvr/cvz137
van der Heijden, C. D. C. C., Keating, S. T., Groh, L., Joosten, L. A. B., Netea, M. G., and Riksen, N. P. (2020). Aldosterone induces trained immunity: The role of fatty acid synthesis. Cardiovasc. Res. 116 (2), 317–328. doi:10.1093/cvr/cvz137
van der Valk, F. M., Bekkering, S., Kroon, J., Yeang, C., Van den Bossche, J., van Buul, J. D., et al. (2016). Oxidized phospholipids on lipoprotein(a) elicit arterial wall inflammation and an inflammatory monocyte response in humans. Circulation 134 (8), 611–624. doi:10.1161/circulationaha.116.020838
Vasamsetti, S. B., Karnewar, S., Gopoju, R., Gollavilli, P. N., Narra, S. R., Kumar, J. M., et al. (2016). Resveratrol attenuates monocyte-to-macrophage differentiation and associated inflammation via modulation of intracellular GSH homeostasis: Relevance in atherosclerosis. Free Radic. Biol. Med. 96, 392–405. doi:10.1016/j.freeradbiomed.2016.05.003
Verma, D., Parasa, V. R., Raffetseder, J., Martis, M., Mehta, R. B., Netea, M., et al. (2017). Anti-mycobacterial activity correlates with altered DNA methylation pattern in immune cells from BCG-vaccinated subjects. Sci. Rep. 7 (1), 12305. doi:10.1038/s41598-017-12110-2
Višnjić, D., Lalić, H., Dembitz, V., Tomić, B., and Smoljo, T. (2021). AICAr, a widely used AMPK activator with important AMPK-independent effects: A systematic review. Cells 10 (5), 1095. doi:10.3390/cells10051095
Wallace, C., and Keast, D. (1992). Glutamine and macrophage function. Metabolism 41 (9), 1016–1020. doi:10.1016/0026-0495(92)90130-3
Wang, C. Y., Yen, C. C., Hsu, M. C., and Wu, Y. T. (2020). Self-nanoemulsifying drug delivery systems for enhancing solubility, permeability, and bioavailability of sesamin. Molecules 25 (14), 3119. doi:10.3390/molecules25143119
Wang, D., Wei, X., Yan, X., Jin, T., and Ling, W. (2010). Protocatechuic acid, a metabolite of anthocyanins, inhibits monocyte adhesion and reduces atherosclerosis in apolipoprotein E-deficient mice. J. Agric. Food Chem. 58 (24), 12722–12728. doi:10.1021/jf103427j
Wang, F., Shan, Q., Chang, X., Li, Z., and Gui, S. (2021). Paeonol-loaded PLGA nanoparticles as an oral drug delivery system: Design, optimization and evaluation. Int. J. Pharm. 602, 120617. doi:10.1016/j.ijpharm.2021.120617
Wang, L., Bao, Y., Yang, Y., Wu, Y., Chen, X., Si, S., et al. (2010). Discovery of antagonists for human scavenger receptor CD36 via an ELISA-like high-throughput screening assay. J. Biomol. Screen 15 (3), 239–250. doi:10.1177/1087057109359686
Wang, L., Palme, V., Rotter, S., Schilcher, N., Cukaj, M., Wang, D., et al. (2017). Piperine inhibits ABCA1 degradation and promotes cholesterol efflux from THP-1-derived macrophages. Mol. Nutr. Food Res. 61 (4), 1500960. doi:10.1002/mnfr.201500960
Wang, L., Rotter, S., Ladurner, A., Heiss, E. H., Oberlies, N. H., Dirsch, V. M., et al. (2015). Silymarin constituents enhance ABCA1 expression in THP-1 macrophages. Molecules 21 (1), E55. doi:10.3390/molecules21010055
Wang, M., Subramanian, M., Abramowicz, S., Murphy, A. J., Gonen, A., Witztum, J., et al. (2014). Interleukin-3/granulocyte macrophage colony-stimulating factor receptor promotes stem cell expansion, monocytosis, and atheroma macrophage burden in mice with hematopoietic ApoE deficiency. Arterioscler. Thromb. Vasc. Biol. 34 (5), 976–984. doi:10.1161/atvbaha.113.303097
Wang, P., He, L. Y., Shen, G. D., and Yang, J. L. (2017). Inhibitory effects of Dioscin on atherosclerosis and foam cell formation in hyperlipidemia rats. Inflammopharmacology 25 (6), 633–642. doi:10.1007/s10787-017-0341-4
Wang, S., Zhang, X., Liu, M., Luan, H., Ji, Y., Guo, P., et al. (2015). Chrysin inhibits foam cell formation through promoting cholesterol efflux from RAW264.7 macrophages. Pharm. Biol. 53 (10), 1481–1487. doi:10.3109/13880209.2014.986688
Wang, Y., Tong, Q., Ma, S. R., Zhao, Z. X., Pan, L. B., Cong, L., et al. (2021). Oral berberine improves brain dopa/dopamine levels to ameliorate Parkinson's disease by regulating gut microbiota. Signal Transduct. Target Ther. 6 (1), 77. doi:10.1038/s41392-020-00456-5
Wang, Y., Wang, Y. S., Song, S. L., Liang, H., and Ji, A. G. (2016). Icariin inhibits atherosclerosis progress in Apoe null mice by downregulating CX3CR1 in macrophage. Biochem. Biophys. Res. Commun. 470 (4), 845–850. doi:10.1016/j.bbrc.2016.01.118
Wang, Y., Wen, J., Almoiliqy, M., Wang, Y., Liu, Z., Yang, X., et al. (2021). Sesamin protects against and ameliorates rat intestinal ischemia/reperfusion injury with involvement of activating Nrf2/HO-1/NQO1 signaling pathway. Oxid. Med. Cell. Longev. 2021, 5147069. doi:10.1155/2021/5147069
Wang, Y., Zhang, Y., Wang, X., Liu, Y., and Xia, M. (2012). Cyanidin-3-O-β-glucoside induces oxysterol efflux from endothelial cells: Role of liver X receptor alpha. Atherosclerosis 223 (2), 299–305. doi:10.1016/j.atherosclerosis.2012.06.004
Wang, Y. F., Yang, X. F., Cheng, B., Mei, C. L., Li, Q. X., Xiao, H., et al. (2010). Protective effect of Astragalus polysaccharides on ATP binding cassette transporter A1 in THP-1 derived foam cells exposed to tumor necrosis factor-alpha. Phytother. Res. 24 (3), 393–398. doi:10.1002/ptr.2958
Wang, Z., Liu, Y., Xue, Y., Hu, H., Ye, J., Li, X., et al. (2016). Berberine acts as a putative epigenetic modulator by affecting the histone code. Toxicol Vitro 36, 10–17. doi:10.1016/j.tiv.2016.06.004
Wang, Z., Qi, F., Cui, Y., Zhao, L., Sun, X., Tang, W., et al. (2018). An update on Chinese herbal medicines as adjuvant treatment of anticancer therapeutics. Biosci. Trends 12 (3), 220–239. doi:10.5582/bst.2018.01144
Wenzel, E., and Somoza, V. (2005). Metabolism and bioavailability of trans-resveratrol. Mol. Nutr. Food Res. 49 (5), 472–481. doi:10.1002/mnfr.200500010
Willecke, F., Yuan, C., Oka, K., Chan, L., Hu, Y., Barnhart, S., et al. (2015). Effects of high fat feeding and diabetes on regression of atherosclerosis induced by low-density lipoprotein receptor gene therapy in LDL receptor-deficient mice. PLoS One 10 (6), e0128996. doi:10.1371/journal.pone.0128996
Wu, C., Luan, H., Zhang, X., Wang, S., Zhang, X., Sun, X., et al. (2014). Chlorogenic acid protects against atherosclerosis in ApoE-/- mice and promotes cholesterol efflux from RAW264.7 macrophages. PLoS One 9 (9), e95452. doi:10.1371/journal.pone.0095452
Wu, D., Li, S., Liu, X., Xu, J., Jiang, A., Zhang, Y., et al. (2020). Alpinetin prevents inflammatory responses in OVA-induced allergic asthma through modulating PI3K/AKT/NF-κB and HO-1 signaling pathways in mice. Int. Immunopharmacol. 89 (1), 107073. doi:10.1016/j.intimp.2020.107073
Wu, M., Liu, M., Guo, G., Zhang, W., and Liu, L. (2015). Polydatin inhibits formation of macrophage-derived foam cells. Evid. Based Complement. Altern. Med. 2015, 729017. doi:10.1155/2015/729017
Wu, R., Wang, L., Yin, R., Hudlikar, R., Li, S., Kuo, H. D., et al. (2020). Epigenetics/epigenomics and prevention by curcumin of early stages of inflammatory-driven colon cancer. Mol. Carcinog. 59 (2), 227–236. doi:10.1002/mc.23146
Wu, S., Xu, H., Peng, J., Wang, C., Jin, Y., Liu, K., et al. (2015). Potent anti-inflammatory effect of dioscin mediated by suppression of TNF-α-induced VCAM-1, ICAM-1and EL expression via the NF-κB pathway. Biochimie 110, 62–72. doi:10.1016/j.biochi.2014.12.022
Wu, W. H., Wang, S. H., Kuan, , Kao, Y. S., Wu, P. J., Liang, C. J., et al. (2010). Sesamin attenuates intercellular cell adhesion molecule-1 expression in vitro in TNF-alpha-treated human aortic endothelial cells and in vivo in apolipoprotein-E-deficient mice. Mol. Nutr. Food Res. 54 (9), 1340–1350. doi:10.1002/mnfr.200900271
Xia, X., Ling, W., Ma, J., Xia, M., Hou, M., Wang, Q., et al. (2006). An anthocyanin-rich extract from black rice enhances atherosclerotic plaque stabilization in apolipoprotein E-deficient mice. J. Nutr. 136 (8), 2220–2225. doi:10.1093/jn/136.8.2220
Xiao, M., Yang, H., Xu, W., Ma, S., Lin, H., Zhu, H., et al. (2012). Inhibition of 03B1-KG-dependent histone and DNA demethylases by fumarate and succinate that are accumulated in mutations of FH and SDH tumor suppressors. Genes Dev. 26 (12), 1326–1338. doi:10.1101/gad.191056.112
Xu, C., Wang, W., Wang, B., Zhang, T., Cui, X., Pu, Y., et al. (2019). Analytical methods and biological activities of Panax notoginseng saponins: Recent trends. J. Ethnopharmacol. 236, 443–465. doi:10.1016/j.jep.2019.02.035
Xu, X., Li, Q., Pang, L., Huang, G., Huang, J., Shi, M., et al. (2013). Arctigenin promotes cholesterol efflux from THP-1 macrophages through PPAR-γ/LXR-α signaling pathway. Biochem. Biophys. Res. Commun. 441 (2), 321–326. doi:10.1016/j.bbrc.2013.10.050
Xu, Y., Liu, Q., Xu, Y., Liu, C., Wang, X., He, X., et al. (2014). Rutaecarpine suppresses atherosclerosis in ApoE-/- mice through upregulating ABCA1 and SR-BI within RCT. J. Lipid Res. 55 (8), 1634–1647. doi:10.1194/jlr.M044198
Xue, X. L., Han, X. D., Li, Y., Chu, X. F., Miao, W. M., Zhang, J. L., et al. (2017). Astaxanthin attenuates total body irradiation-induced hematopoietic system injury in mice via inhibition of oxidative stress and apoptosis. Stem Cell. Res. Ther. 8 (1), 7. doi:10.1186/s13287-016-0464-3
Yang, J., Wu, M., Fang, H., Su, Y., Zhang, L., and Zhou, H. (2021). Puerarin prevents acute liver injury via inhibiting inflammatory responses and ZEB2 expression. Front. Pharmacol. 12, 727916. doi:10.3389/fphar.2021.727916
Yang, S. H., Liao, C. C., Chen, Y., Syu, J. P., Jeng, C. J., and Wang, S. M. (2012). Daidzein induces neuritogenesis in DRG neuronal cultures. J. Biomed. Sci. 19 (1), 80. doi:10.1186/1423-0127-19-80
Yang, Y., Fuentes, F., Shu, L., Wang, C., Pung, D., Li, W., et al. (2017). Epigenetic CpG methylation of the promoter and reactivation of the expression of GSTP1 by astaxanthin in human prostate LNCaP cells. Aaps J. 19 (2), 421–430. doi:10.1208/s12248-016-0016-x
Yang, Y., Jiang, W., Wang, L., Zhang, Z. B., Si, S. Y., and Hong, B. (2009). Characterization of the isoflavone pratensein as a novel transcriptional up-regulator of scavenger receptor class B type I in HepG2 cells. Biol. Pharm. Bull. 32 (7), 1289–1294. doi:10.1248/bpb.32.1289
Ye, W., Lin, X., Zhang, Y., Xu, Y., Sun, R., Wen, C., et al. (2018). Quantification and pharmacokinetics of alpinetin in rat plasma by UHPLC-MS/MS using protein precipitation coupled with dilution approach to eliminate matrix effects. J. Pharm. Biomed. Anal. 152, 242–247. doi:10.1016/j.jpba.2017.12.046
Yin, H., Guo, Q., Li, X., Tang, T., Li, C., Wang, H., et al. (2018). Curcumin suppresses IL-1β secretion and prevents inflammation through inhibition of the NLRP3 inflammasome. J. Immunol. 200 (8), 2835–2846. doi:10.4049/jimmunol.1701495
Yu, W., Wang, Z., Zhang, K., Chi, Z., Xu, T., Jiang, D., et al. (2019). One-carbon metabolism supports S-adenosylmethionine and histone methylation to drive inflammatory macrophages. Mol. Cell. 75 (6), 1147–1160. doi:10.1016/j.molcel.2019.06.039
Yuan, X., Chen, J., and Dai, M. (2016). Paeonol promotes microRNA-126 expression to inhibit monocyte adhesion to ox-LDL-injured vascular endothelial cells and block the activation of the PI3K/Akt/NF-κB pathway. Int. J. Mol. Med. 38 (6), 1871–1878. doi:10.3892/ijmm.2016.2778
Yuan, Z., Liao, Y., Tian, G., Li, H., Jia, Y., Zhang, H., et al. (2011). Panax notoginseng saponins inhibit Zymosan A induced atherosclerosis by suppressing integrin expression, FAK activation and NF-κB translocation. J. Ethnopharmacol. 138 (1), 150–155. doi:10.1016/j.jep.2011.08.066
Yue, J., Li, B., Jing, Q., and Guan, Q. (2015). Salvianolic acid B accelerated ABCA1-dependent cholesterol efflux by targeting PPAR-γ and LXRα. Biochem. Biophys. Res. Commun. 462 (3), 233–238. doi:10.1016/j.bbrc.2015.04.122
Zaheer, K., and Humayoun Akhtar, M. (2017). An updated review of dietary isoflavones: Nutrition, processing, bioavailability and impacts on human health. Crit. Rev. Food Sci. Nutr. 57 (6), 1280–1293. doi:10.1080/10408398.2014.989958
Zarzour, A., Kim, H. W., and Weintraub, N. L. (2019). Epigenetic regulation of vascular diseases. Arterioscler. Thromb. Vasc. Biol. 39 (6), 984–990. doi:10.1161/atvbaha.119.312193
Zhan, J., Yan, Z., Kong, X., Liu, J., Lin, Z., Qi, W., et al. (2021). Lycopene inhibits IL-1β-induced inflammation in mouse chondrocytes and mediates murine osteoarthritis. J. Cell. Mol. Med. 25 (7), 3573–3584. doi:10.1111/jcmm.16443
Zhang, F., Xia, Y., Yan, W., Zhang, H., Zhou, F., Zhao, S., et al. (2016). Sphingosine 1-phosphate signaling contributes to cardiac inflammation, dysfunction, and remodeling following myocardial infarction. Am. J. Physiology-Heart Circulatory Physiology 310 (2), H250–H261. doi:10.1152/ajpheart.00372.2015
Zhang, J., Nie, S., Zu, Y., Abbasi, M., Cao, J., Li, C., et al. (2019). Anti-atherogenic effects of CD36-targeted epigallocatechin gallate-loaded nanoparticles. J. Control Release 303, 263–273. doi:10.1016/j.jconrel.2019.04.018
Zhang, Q., Liu, J., Duan, H., Li, R., Peng, W., and Wu, C. (2021). Activation of Nrf2/HO-1 signaling: An important molecular mechanism of herbal medicine in the treatment of atherosclerosis via the protection of vascular endothelial cells from oxidative stress. J. Adv. Res. 34, 43–63. doi:10.1016/j.jare.2021.06.023
Zhang, T., Guo, S., Zhu, X., Qiu, J., Deng, G., and Qiu, C. (2020). Alpinetin inhibits breast cancer growth by ROS/NF-κB/HIF-1α axis. J. Cell. Mol. Med. 24 (15), 8430–8440. doi:10.1111/jcmm.15371
Zhang, X., Chen, S., Duan, F., Liu, A., Li, S., Zhong, W., et al. (2021). Prebiotics enhance the biotransformation and bioavailability of ginsenosides in rats by modulating gut microbiota. J. Ginseng Res. 45 (2), 334–343. doi:10.1016/j.jgr.2020.08.001
Zhao, G., Zhang, X., Wang, H., and Chen, Z. (2020). Beta carotene protects H9c2 cardiomyocytes from advanced glycation end product-induced endoplasmic reticulum stress, apoptosis, and autophagy via the PI3K/Akt/mTOR signaling pathway. Ann. Transl. Med. 8 (10), 647. doi:10.21037/atm-20-3768
Zhao, G. J., Tang, S. L., Lv, Y. C., Ouyang, X. P., He, P. P., Yao, F., et al. (2013). Antagonism of betulinic acid on LPS-mediated inhibition of ABCA1 and cholesterol efflux through inhibiting nuclear factor-kappaB signaling pathway and miR-33 expression. PLoS One 8 (9), e74782. doi:10.1371/journal.pone.0074782
Zhao, J. F., Ching, L. C., Huang, Y. C., Chen, C. Y., Chiang, A. N., Kou, Y. R., et al. (2012). Molecular mechanism of curcumin on the suppression of cholesterol accumulation in macrophage foam cells and atherosclerosis. Mol. Nutr. Food Res. 56 (5), 691–701. doi:10.1002/mnfr.201100735
Zhao, J. F., Jim Leu, S. J., Shyue, S. K., Su, K. H., Wei, J., and Lee, T. S. (2013). Novel effect of paeonol on the formation of foam cells: Promotion of lxrα-ABCA1-dependent cholesterol efflux in macrophages. Am. J. Chin. Med. 41 (5), 1079–1096. doi:10.1142/s0192415x13500730
Zhao, L., Wei, Y., Huang, Y., He, B., Zhou, Y., and Fu, J. (2013). Nanoemulsion improves the oral bioavailability of baicalin in rats: In vitro and in vivo evaluation. Int. J. Nanomedicine 8, 3769–3779. doi:10.2147/ijn.S51578
Zhao, S., Li, J., Wang, L., and Wu, X. (2016). Pomegranate peel polyphenols inhibit lipid accumulation and enhance cholesterol efflux in raw264.7 macrophages. Food Funct. 7 (7), 3201–3210. doi:10.1039/c6fo00347h
Zhao, X., Wang, H., Yang, Y., Gou, Y., Wang, Z., Yang, D., et al. (2021). Protective effects of silymarin against D-gal/LPS-induced organ damage and inflammation in mice. Drug Des. Devel Ther. 15, 1903–1914. doi:10.2147/dddt.S305033
Zhao, Y., Lin, S., Fang, R., Shi, Y., Wu, W., Zhang, W., et al. (2022). Mechanism of enhanced oral absorption of a nano-drug delivery system loaded with trimethyl chitosan derivatives. Int. J. Nanomedicine 17, 3313–3324. doi:10.2147/ijn.S358832
Zheng, X. Y., Yang, S. M., Zhang, R., Wang, S. M., Li, G. B., and Zhou, S. W. (2019). Emodin-induced autophagy against cell apoptosis through the PI3K/AKT/mTOR pathway in human hepatocytes. Drug Des. Devel Ther. 13, 3171–3180. doi:10.2147/dddt.S204958
Zhou, J., Yu, Y., Yang, X., Wang, Y., Song, Y., Wang, Q., et al. (2019). Berberine attenuates arthritis in adjuvant-induced arthritic rats associated with regulating polarization of macrophages through AMPK/NF-кB pathway. Eur. J. Pharmacol. 852, 179–188. doi:10.1016/j.ejphar.2019.02.036
Zhou, L., Hu, X., Han, C., Niu, X., Han, L., Yu, H., et al. (2022). Comprehensive investigation on the metabolism of emodin both in vivo and in vitro. J. Pharm. Biomed. Anal. 223, 115122. doi:10.1016/j.jpba.2022.115122
Zhou, M., Xu, H., Pan, L., Wen, J., Guo, Y., and Chen, K. (2008). Emodin promotes atherosclerotic plaque stability in fat-fed apolipoprotein E-deficient mice. Tohoku J. Exp. Med. 215 (1), 61–69. doi:10.1620/tjem.215.61
Zhu, S., and Liu, J. H. (2015). Zerumbone, A natural cyclic sesquiterpene, promotes ABCA1-dependent cholesterol efflux from human THP-1 macrophages. Pharmacology 95 (5-6), 258–263. doi:10.1159/000381722
Zhu, Z., Hu, R., Li, J., Xing, X., Chen, J., Zhou, Q., et al. (2021). Alpinetin exerts anti-inflammatory, anti-oxidative and anti-angiogenic effects through activating the Nrf2 pathway and inhibiting NLRP3 pathway in carbon tetrachloride-induced liver fibrosis. Int. Immunopharmacol. 96, 107660. doi:10.1016/j.intimp.2021.107660
Zolberg Relevy, N., Bechor, S., Harari, A., Ben-Amotz, A., Kamari, Y., Harats, D., et al. (2015). The inhibition of macrophage foam cell formation by 9-cis β-carotene is driven by BCMO1 activity. PLoS One 10 (1), e0115272. doi:10.1371/journal.pone.0115272
Glossary
ASCVD atherosclerotic cardiovascular diseases
AS atherosclerosis
ABCA1 ATP-binding cassette transporter A1
APS Astragalus polysaccharides
ATRA all-trans retinoic acid
AMD age-related macular degeneration
ABCG1 ATP-binding cassette transporter G1
ATP adenosine triphosphate
AMPK AMP-activated protein kinase
AP-1 activating protein-1
BCG bacille Calmette–Guérin
BA betulinic acid
BC β-carotene
BBR berberine
CA chlorogenic acid
CD36 cluster of differentiation 36
CAD coronary artery disease
CoA acetyl coenzyme A
CCL2 chemokine C–C motif ligand 2
CVD cardiovascular diseases
C3G Cyanidin-3-O-glucoside
chrysin 5,7-dihydroxyflavone
CARM1 coactivator-associated arginine methyltransferase 1
ceRNA competing endogenous RNA
DAMP damage-associated molecular pattern
DNMTs DNA methyltransferases
EGCG epigallocatechin-3-gallate
EA ellagic acid
FAO fatty acid oxidation
FAS fatty acid synthase
FAK focal adhesion kinase
GM-CSF granulocyte–macrophage colony-stimulating factor
GS glutamine synthetase
GVHD graft-versus-host disease
HATi histone acetyltransferase inhibitor
HATs histone acetyltransferases
HDACs histone deacetylases
HDL-C HDL-cholesterol
HFD high-fat diet
HDL High-density lipoprotein
HSCs hematopoietic stem cells
HMG-CoA 3-hydroxy-3-methylglutaryl-coenzyme A
H3K4me3 histone H3 lysine 4 trimethylation
HIF-1α hyperoxia-inducible substance 1α
HK-II hexokinase II
H3K27ac histone 3 lysine 27 acetylation
H3K4me1 histone 3 lysine 4 methylation
hesperidin 3′,5,7,-trihydroxy-4′-methoxyflavanone
H3K4me2/3 H3K4 di- and tri-methylation
HDAC1–2 histone deacetylase 1–2
HPC hematopoietic progenitor cell
HMDMs human peripheral blood monocyte-derived macrophages
H3K27m3 H3K27 trimethylation
iNOS
inducible nitric oxide synthase
IL interleukin
ICAM-1 intercellular adhesion molecule 1
JNK c-Jun N-terminal kinases
LDL low-density lipoprotein
LDs lipid droplets
LXR liver X receptor
LPS lipopolysaccharide
lncRNAs long non-coding RNAs
LDL-C LDL-cholesterol
MCP-1 monocyte chemoattractant protein-1
MMP-2 matrix metalloproteinases 2
m5C methylate cytosine
mTOR mammalian target of rapamycin
MSCs mesenchymal stem cells
NLRP3
NOD-, LRR-, and pyrin domain-containing protein 3
NO nitric oxide
Nox1 NADPH oxidase 1
oxLDL oxidized LDL
OXPHOS oxidative phosphorylation
oxPAPC oxidized phospholipids made of 1-palmitoyl-2-arachidonoyl-sn-glycero-3-phosphorylcholine
PPARγ peroxisome proliferator-activated receptor γ
PPP pentose phosphate pathway
PFKFB3 6-phosphofructo-2-kinase/fructo-2, 6-bisphosphatase
PDK1 pyruvate dehydrogenase kinase isozyme 1
PI3K phosphoinositide 3-kinase
PON-1 paraoxonase-1
PEA pomegranate ellagic acid
PCSK9 proprotein convertase subtilisin/kexin type 9
PCA protocatechuic acid
PNS Panax notoginseng saponins
quercetin
3,3',4',5,7-pentahydroxyflavone
RXR retinoid X receptor
ROS reactive oxygen species
Runx1 Runt-related transcription factor 1
RCT reverse cholesterol transport
RV resveratrol
RARs retinoic acid receptors
SalB salvianolic acid B
SAM S-adenosylmethionine
SR-A type A scavenger receptor
SR-BI scavenger receptor class B type I
SDH succinate dehydrogenase
STK11 serine/threonine kinase 11
TNF-α tumor necrosis factor-α
TCA cycle tricarboxylic acid cycle
TLR4 Toll-like receptor 4
TC total cholesterol
TMAO trimethylamine N-oxide
Tan IIA tanshinone IIA
ursolic acid
3B-hydroxy-12-urc-12-en-28-oic acid
9-cis-RA
9-cis retinoic acid
VCAM-1 vascular cell adhesion molecule 1
VSMCs vascular smooth muscle cells
WTD Western-style diet
WDR5 WD repeat-containing protein 5
2-DG
2-deoxy-d-glucose
2-DG6P
2-deoxy-d-glucose-6-phosphate.
Keywords: trained immunity, monocyte/macrophage, atherosclerosis, natural products, epigenetic reprogramming, metabolic reprogramming
Citation: Wang J, Liu Y-M, Hu J and Chen C (2023) Trained immunity in monocyte/macrophage: Novel mechanism of phytochemicals in the treatment of atherosclerotic cardiovascular disease. Front. Pharmacol. 14:1109576. doi: 10.3389/fphar.2023.1109576
Received: 29 November 2022; Accepted: 27 January 2023;
Published: 21 February 2023.
Edited by:
Pallavi R. Devchand, University of Calgary, CanadaReviewed by:
Wei Zhou, China Pharmaceutical University, ChinaShen Xiang-chun, Guizhou Medical University, China
Copyright © 2023 Wang, Liu, Hu and Chen. This is an open-access article distributed under the terms of the Creative Commons Attribution License (CC BY). The use, distribution or reproduction in other forums is permitted, provided the original author(s) and the copyright owner(s) are credited and that the original publication in this journal is cited, in accordance with accepted academic practice. No use, distribution or reproduction is permitted which does not comply with these terms.
*Correspondence: Cong Chen, NzA2NDI3MDgxQHFxLmNvbQ==