- 1College of Pharmacy, Gansu University of Chinese Medicine, Lanzhou, China
- 2Department of Pharmacy, The 940th Hospital of Joint Logistic Support Force of Chinese People’s Liberation Army, Gansu Plateau Pharmaceutical Technology Center, Lanzhou, China
- 3Academy of Military Medical, Academy of Military Sciences, Beijing, China
- 4National Key Laboratory of Kidney Diseases, Beijing, China
Introduction: Phlomoides rotata (Benth. ex Hook.f.) Mathiesen (syn. Lamiophlomis rotata (Benth. ex Hook.f.) Kudô) (P. rotate) is a traditional Tibetan medicine known for its hemostatic, analgesic, and anti-inflammatory effects, as well as its high content of 8-O-Acetyl Shanzhiside methyl ester (8-OaS). Clinical and experimental studies have reported gastrointestinal side effects, such as diarrhea, loose stools, even to black stools, associated with P. rotata. Given the bitter taste characteristic, laxative and antipyretic effects of iridoid glycosides, this study aims to investigate the antipyretic and anti-inflammatory effects of 8-OaS (the primary iridoid glycosides of P. rotate) on yeast-induced pyrexia in rats. Additionally, the role 8-OaS in modulating the intestinal flora composition and metabolome profile is explored.
Methods: The pyretic rat model was established by injected subcutaneously with 20% dry yeast suspension. Serum, hypothalamic tissues and colon content were collected for the assessment of relevant indicators. The peripheral inflammatory factors and central thermoregulatory mediators were assessed using enzyme-linked immunosorbent assay (ELISA). The expressions of mRNA and protein in hypothalamic tissue were evaluated through polymerase chain reaction (PCR), immunohistochemistry, and western blotting. 16S rDNA sequencing and LC-MS/MS were performed to determine the alteration and correlation of the intestinal flora and neurotransmitters in the colonic contents and hypothalamus.
Results and discussion:: Results show that 8-OaS treatment reduced pyrogenic cytokines (such as IL-6, IL-1β), and down-regulated the level of central thermoregulatory mediators (PGE2), via multiply involved in TLR4/NF-κB and HSP70/NF-κB signaling pathways. Crucially, 8-OaS treatment significantly reduced the relative abundance of Alistipes (P < 0.01), Odoribacter (P < 0.05) and Alistipes_finegoldii (P < 0.05) in the intestinal flora. The correlation analysis demonstrated that 8-OaS treatment significantly correlated with the increasing on the abundance of Alistipes and levels of 5-hydroxytryptamine (P < 0.01), and tryptamine (P < 0.01). Our findings indicate that 8-OaS exhibits significant antipyretic and anti-inflammatory properties, potentially mediated by intestinal flora and metabolites of neurotransmitters. The results of this study may help to elucidate the antipyretic and anti-inflammatory mechanism of 8-OaS based on intestinal flora and metabolomics analysis.
Introduction
Fever represents a complex pathophysiological defense mechanism employed by the body in response to disease and foreign bacterial infections. It is a prevalent symptom associated with infectious and inflammatory conditions. The neurotransmitters such as acetylcholine (Ach), norepinephrine (NE), and serotonin (5-hydroxytryptamine/5-HT) play a significant role in thermoregulation (Voronova, 2021; Bo et al., 2019; Kesić et al., 2023). In recent years, a growing body of research has demonstrated that intestinal flora is involved in hypothalamic thermoregulatory processes by influencing the activation of neural signaling pathways and neurotransmitter expression through the gut-brain axis (Bo et al., 2019; Li S. et al., 2023; Mhanna et al., 2024; Loh et al., 2024). Lactobacillus spp. and Bifidobacterium spp. are capable of synthesizing γ-aminobutyric acid (GABA), while Streptococcus spp., Candida spp., and Enterococcus spp. have the ability to produce 5-HT (Eicher and Mohajeri, 2022). Neurotransmitters are unable to cross the blood-brain barrier (BBB) to act directly on the brain. Interestingly, certain amino acids act as precursor molecules for the synthesis of certain neurotransmitters possess the ability to traverse the BBB and influence brain function by modulating neurotransmitter-related metabolic pathways through interactions with intestinal flora. Phenylalanine can be metabolized to tyrosine, which subsequently leads to the production of neurotransmitters such as dopamine, DL-adrenaline, and NE. Bifidobacterium has been shown to elevate phenylalanine levels, while tryptophan acts as a precursor to the neurotransmitter 5-HT. Additionally, Bifidobacterium infantis is known to enhance circulating tryptophan levels (Eicher and Mohajeri, 2022). Therefore, intestinal flora influences the development of various diseases by regulating the expression of neurotransmitters within the nervous system through the gut-brain axis.
8-O-Acetyl Shanzhiside methyl ester (8-OaS), an iridoid glucoside, is isolated from the leaves of P. rotata (Benth. ex Hook.f.) Mathiesen (syn. Lamiophlomis rotata (Benth. ex Hook.f.) Kudô). Phlomoides rotata is a traditional Chinese medicinal plant utilized by the Tibet, Mongolian, and Naxi ethnic groups in China. The functions of this traditional Chinese medicine include promoting blood circulation to stop bleeding, expelling wind, and alleviating pain. And it is commonly utilized in traditional medicine to address issues such as dysmenorrhea due to blood stasis, metrorrhagia and metrostaxis, joint effusion of yellow, as well as injury of muscles and bones by falling and flashback deflate (Cui et al., 2020). Recent pharmacological researches have demonstrated that P. rotata possesses anti-inflammatory, antioxidant, antitumor, and anti-ulcer properties. It is frequently utilized in the management of postoperative bleeding, pain, arthritis, gingivitis, fractures, and various other conditions within domestic clinical practice. Its application prospects are extensive and promising. Phlomoides rotata has been formulated into various dosage forms such as granules, capsules, soft capsules, tablets, dispersible tablets, effervescent tablets, chewable tablets, and drop pills, which have been put on the market for use. Among them, Duyiwei tablets and Duyiwei capsules have been included in the Chinese Pharmacopoeia since 1995 and 2000, respectively. Iridoids, flavonoids and phenylethanoid glycosides are the main active components of P. rotata. Traditional Chinese medicine theory has established that the application of herbs with properties aimed at clearing heat and purgation, as well as bitter herbs, is an effective approach for treating heat syndrome within traditional Chinese medicine. Given the purgation properties of iridoid glycoside derived from P. rotata, our research group has further discovered that iridoid glycoside possess a beneficial effect on intestinal lubrication and promote bowel movements, which can shorten the defecation time of first black stool, increase the number of black stool, promote the intestinal propulsion rate, and alter the defecation situation in mice subjected to a constipation model induced by compound diphenol. The antipyretic and anti-inflammatory activity has been already observed for several iridoid glycosides, the most important is that of geniposide of Gardenia jasminoides Ellis as reported in the following work and the cited literature (Li et al., 2024).
8-OaS exhibits multiple pharmacological effects, including neuroprotective and analgesic properties. Its neuroprotective role following cerebral ischemia and reperfusion (I/R) injury is mediated through mechanisms that enhance angiogenesis (Jiang et al., 2011). Additionally, its protective effects in cerebral I/R injury are facilitated by the suppression of inflammatory processes (Zhang et al., 2014). It also provides protection against cognitive deficits and anxiety-like behaviors induced by sleep deprivation (Li Y. J. et al., 2023). However, there has yet to be any research that demonstrates whether 8-OaS possesses antipyretic and anti-inflammatory effects based on yeast-induced pyrexia model in rats. Therefore, given the bitter taste and laxative activity, a yeast-induced pyrexia model in rats was established to investigate whether the 8-OaS has antipyretic and anti-inflammatory effects.
This study aims to explore the antipyretic and anti-inflammatory effect of 8-OaS, focusing on the roles of intestinal flora and neurotransmitter metabolites. Our study first established a yeast-induced model pyrexia in rats, monitored the anal temperature of rats at various time points, and detected peripheral inflammatory factors as well as central thermoregulatory mediators. Furthermore, we sequenced the 16S rDNA in the intestinal flora and performed LC-MS/MS on metabolites. We then integratively analyzed their correlations to investigate the potential mechanisms underlying the antipyretic and anti-inflammatory effects of 8-OaS.
Materials and methods
Animals
A total of one hundred male sprague-dawley (SD) rats (weighing 180–220 g) were purchased from Ji’nan Pengyue Laboratory Animal Breeding Co., Ltd. (Jinan, China). The production license number is SCXK (LU) 2022-0006. They were fed adaptively under the conditions of temperature (20°C–25°C), relative humidity 40%–50%, and alternating light (12 h light/dark cycle) with unrestricted free access to sufficient food and water. The animals were allowed to habituate to the housing facilities for at least 1 week before the experiment began. All animal experiments were approved by the Animal Ethics Committee of the 940th Hospital (No. 2024KYLL145) and performed according to the Guidelines for the Care and Use of Laboratory Animals.
Reagents and chemicals
8-OaS(purity ≥ 98%; batch number: AZCC2903) was purchased from Chengdu Alfa Biotechnology Co., Ltd. (Chengdu, China); highly active dry yeast was purchased from Angel yeast Co., Ltd. (Yichang, China). Aspirin enteric-coated tablets was purchased from Hunan Xinhui Pharmacy Co., Ltd. (Changsha, China); prostaglandin E2 (PGE2) rat assay kit, tumour necrosis factor-α (TNF-α) assay kit, NF-κB test Kit, interleukin-6 (IL-6) assay kit, interleukin-1β (IL-1β) assay kit, arginine vasopressin (AVP) assay kit, 5-HT test kit, macrophage inflammatory protein-1α (MIP-1α) assay kit, interferon-γ (IFN-γ) assay kit, corticotropin-releasing hormone (CRH/CRF) and substance P (SP) assay kit were purchased from Nanjing Jiancheng Bioengineering institute (Nanjing, China). Anti-TLR4 rabbit pAb, anti-phospho-NF-κB p65 (S536) rabbit pAb, anti- TNF-α rabbit pAb, and anti-COX2/Cyclooxygenase 2 rabbit pAb were purchased from Wuhan Servicebio Technology Co., Ltd. (Wuhan, China). HSP70 antibody was purchased from Affinity Biosciences (Liyang, China).
Experimental animals and design
Three days prior to the formal experiment, one hundred SD male rats underwent adaptive measurements three times daily, as well as two additional measurements conducted before the establishment of the fever model, with a 1-h interval between each measurement. The rats were excluded if they exhibited a single temperature higher than 38°C or a temperature difference greater than 0.5°C between the two temperatures, and the average temperature of the two temperatures was taken as the basal body temperature of the rat.
The body temperatures of rats were measured using a calibrated medical thermometer. Prior to measurement, the temperature probe was prepared by establishing a physical limit marker at 3 cm from its tip to standardize insertion depth. A thin layer of medical-grade Vaseline was uniformly applied to the distal 3 cm of the probe to ensure adequate lubrication and prevent rectal tissue damage. During the procedure, each rat was humanely restrained using a dedicated rodent immobilization device. The lubricated probe was then gently inserted rectally until the pre-marked 3 cm depth was reached. The thermometer was activated, and temperature readings were automatically recorded upon completion of the device’s audible notification signal. Three consecutive measurements were obtained at 2-min intervals for each subject, with the probe thoroughly disinfected between animals using 70% ethanol.
Following the screening of body temperature, the seventy-two rats were randomly divided into six groups (n = 12): normal control group (NG, sterilized water for injection, 10 mL/kg), fever model group (MG, sterilized water for injection, 10 mL/kg); 8-OaS high dose group (8-OAS-H, 200 mg/kg); 8-OaS medium dose group (8-OAS-M, 150 mg/kg); 8-OaS low-dose group (8-OaS-L, 100 mg/kg); positive control group (PG, aspirin, 100 mg/kg). The dosage of 8-OaS is obtained based on preliminary experiments. Except for the NG, all other groups were injected subcutaneously with 20% dry yeast suspension to establish the rat fever model (Li et al., 2024; Sherif et al., 2024). After a subcutaneous injection of dry yeast for 5 h, the rats were screened out and subsequently administered corresponding drugs; the body temperature was measured continuously for 7 h following drug administration, with an interval of 1 h between each measurement. At the end of the temperature measurements, the maximum temperature rise △t at each time point was calculated (△t = body temperature at each time point - basal body temperature).
At the conclusion of temperature measurement, blood was collected from the abdominal aorta using a negative pressure tube. A volume of 5 mL was extracted from each rat and subsequently centrifuged at 2,200 g for 10 min. The supernatant was aspirated, aliquoted and stored in a refrigerator at −80°C. Additionally, the hypothalamic tissues and colonic contents were removed and stored at −80°C.
Enzyme-linked immunosorbent assay (ELISA)
ELISA was conducted to assess the levels of IL-1β, IL-6, TNF-α, IFN-γ, PGE2, MIP-1α, and other corresponding indicators in blood serum, according to the manufacturer’s protocol (Zhang et al., 2019). The sample (50 μL) or standard (50 μL) was added to the enzyme-labeled plates that have been pre-coated with antibodies, then biotin-labeled recognition antigen was added and incubated at 37°C for 30 min. After washing five times by PBST, 50 μL of HRP-conjugated avidin was added and incubated at 37°C for 30 min. After washing the plate, the plate was developed with 50 μL of TMB substrate for 15 min in the dark. The reaction was stopped with 2 M H2SO4 and optical density was measured at a wavelength of 450 nm using a spectrophotometer.
Real-time PCR
The RNA of hypothalamic tissue was extracted by the trizol method and reverse transcribed to cDNA using a reverse transcription kit. Primers were designed according to gene sequences (Ping et al., 2020), NF-κB: upstream GCTGATGGAGTACCCTGAAGC, downstream ATGTCCGCAATGGAGGAGAAG; TNF-α: upstream: CCACGCTCTTCTGTCTACTG, downstream GCTACGGGGCTTGTCACTC; IL-6: upstream GTTGCCTTCTTGGGGACTGATGT, downstream ATACTGGGTCTGTTGGGGTGGGT,; IL-1β: upstream GCTTCAAATCTCACAGCAGCAT, downstream TAGCAGGTCGTCATCATCCCAC; HSP70: Upstream CGAGGAGGTGGATTAGAGGC, downstream GCTGAGGTGTTCGCAGGAA; TRPV4: Upstream AAGTGGCGTAAGTTCGGG, downstream CGTGGTACGGTAAGGGT; GAPDH: Upstream ACAGCAACAGGGTGGTGGAC, downstream TTTGAGGGTGCAGCGAACTT. The amplification reaction of the GAPDH and the target gene was performed simultaneously for each batch of samples.
Western blotting
The total protein in the hypothalamic tissue was extracted utilizing a high-efficiency RIPA lysis buffer, which was supplemented with protease inhibitor and phosphatase inhibitor. The protein concentration was determined by the BCA protein assay kit. The protein samples were separated by 10% SDS-PAGE, run at 80 V for 40 min and then at 110 V for 80 min, before being transferred to a polyvinylidene fluoride (PVDF) membrane. After the transmembrane process, the PVDF membrane was removed and immersed in 1×TBST containing 5% skim milk powder. This mixture was sealed at room temperature for 2 h, followed by overnight incubation with primary antibodies at 4°C. The primary antibodies used in this study were against TLR4 (1:1,000), TNF-α (1:1,000), p-NF-κB p65 (1:1,000), p-IκB-α (1:1,000), HSP70 (1:1,000), COX2 (1:1,000), and GAPDH (1:1,000). After primary antibody incubation, wash with 1×TBST at room temperature, allowing for 8 min of washing each time. Subsequently, the PVDF membrane was immersed into the secondary antibody solution (1:5,000) and incubated at room temperature for 2 h. ImageJ software was utilized for the purpose of quantitative analysis (Wang et al., 2024; Hou et al., 2024).
Immunohistochemical staining
Hypothalamic tissues were paraffin sectioned and deparaffinized for hydration, antigen repair, and closure. Then it was incubated with 3% endogenous H2O2 for 10 min. Then, the tissue sections were incubated with primary antibodies against IL-1β (1:500) and IL-6 (1:500) overnight. On the following day, secondary antibody was added and reacted for another 10 min, and stained with diaminobenzidine for 1 min. Subsequently, the sections were counterstained with hematoxylin, dehydrated, and sealed for observation using a microscope. The whole positive rate was analyzed using ImageJ software.
Intestinal flora analysis
Microbial DNA was extracted utilizing the HiPure Soil DNA Extraction Kit (Magen, China) in accordance with the provided instructions. Subsequently, PCR sequencing was conducted. The amplification of the target region of the 16 S rDNA gene via PCR was performed under the following conditions: 95°C for 5 min, followed by 30 cycles of 95°C for 1 min, 60°C for 1 min, and 72°C for 1 min, and finally 72°C for 7 min using the primers (sequences upstream: CCTACGGGGNGGCWGCAG; downstream: GGACTACHVGGGTATCTAAT). Amplification system: 50 μL mixture containing 10 µL 5×Q5@ Reaction Buffer, 10 µL 5×Q5@ High GC Enhancer, 1.5 µL 2.5 mM dNTPs, 1.5 µL upstream and downstream primers (10 µM), 0.2 µL Q5@ High-Fidelity DNA Polymerase and 50 ng of template DNA. Finally, Illumina sequencing was applied. Amplification product quality was assessed using a 2% agarose gel, PCR product purification was performed using AMPure XP Beads (Beckman, United States) and quantification was conducted using Qubit 3.0. Sequencing libraries were prepared using the Illumina DNA Prep Kit (Illumina, United States). Library quality assessment was performed utilizing the ABI StepOnePlus Real-Time PCR System (Life Technologies, United States). Qualified libraries were up-sequenced using PE250 mode pooling on Novaseq 6000.
After sequencing to obtain raw reads, the initial step involves filtering out low-quality reads, followed by their subsequent assembly. The double-ended reads are spliced into tags. The tags are subsequently filtered and the resulting dataset is referred to as a clean tag. Subsequently, clustering is performed based on a clean tag to eliminate the chimeric tags detected during the cluster comparison process. The final output obtained from this procedure is referred to the as effective tag. After obtaining OTUs, OTU abundance statistics were performed based on the effective tag. The following analyses were performed: species annotation, alpha diversity analysis, beta diversity analysis, species composition analysis, and differential species screening.
Metabolomics analysis
Appropriate samples of hypothalamic tissue and colon contents were carefully placed in 2 mL centrifuge tubes. A total of 500 μL of an 80% methanol-water solution (containing 0.1% formic acid) was added to the tube. The mixture was vortexed for 1 min and subsequently ground for 5 min; after centrifugation, the supernatant was collected for LC-MS/MS analysis.
The liquid chromatography system utilized was a Waters Acquity UPLC, coupled with a mass spectrometer from AB SCIEX 5500 QQQ-MS. The chromatographic columns employed in this study were Acquity UPLC BEH C18 (1.7 µm, 2.1 mm × 100 mm) and Acquity UPLC HSS T3 (1.8 µm, 2.1 mm × 100 mm). The chromatographic separation was conducted under conditions that included a column temperature of 40°C and a flow rate of 0.20 mL/min. The composition of the mobile phase comprised two components: component A, which was water containing 0.01% formic acid, and component B, which was acetonitrile (0.01% formic acid). The total run time was 10 min, with an injection volume of 6 µL. The gradient eluting conditions for samples were as follows: 0–3 min, 95%–55% A; 3–13 min, 55%–5% A; 13–14 min, 5% A; the UHPLC elution conditions for urine samples were as follows: 0–1 min, 99% A; 1–7 min, 99%–60% A; 7–8 min, 60% A; 8–9 min, 60%–99% A; 9–10 min, 99%A. The mass spectrometric conditions were as follows: ion source, ESI; curtain gas, 35 arb; collision gas, 9 arb; ion spray voltage, 4,500 V; ion source temperature, 550°C; ion source gases, 55 arb each for Ion Source Gas1 and IonSource Gas2. The parameters for the MRM acquisition were established based on the aforementioned chromatographic and mass spectrometric conditions. Subsequently, standard solution preparations were injected into the sample vials for analysis.
Preparation of standard solutions is outlined as follows: a 100 μg/mL standard solution was prepared using 80% methanol containing 0.1% formic acid. Subsequently, a series of standard solutions with appropriate concentrations (0.24, 0.49, 0.98, 1.95, 3.91, 7.81, 15.63, 31.25, 62.5, 125, 250, and 500 ng/mL) was subsequently prepared through further dilution.
The standard curve was plotted according to the peak areas of the standards at various concentrations and their corresponding concentrations. The R2 was exceeded 0.99, which indicated that the standard curve had a superior linear relationship and could be used for the calculating the concentrations of the neurotransmitters in the samples. Finally, the integration was carried out using MultiQuant software and quantitative analyses were performed using standard curves.
Data analysis
Statistical analyses were performed using SPSS 25.0 and GraphPad Prism 8.0 software. All data was presented as mean ± standard deviation (
Results
Antipyretic and anti-inflammatory effects of 8-OaS in yeast-induced pyrexia in rats
The variations in temperature observed within 7 h post-administration are illustrated in Figure 1a. Compared with the control group, the remaining five groups exhibited a significant increase in body temperature after 5 h of modeling (P < 0.01), indicating that the yeast-induced pyrexia model in rats was successfully established. Compared with the fever model group, various dosage groups of 8-OaS demonstrated an antipyretic effect.
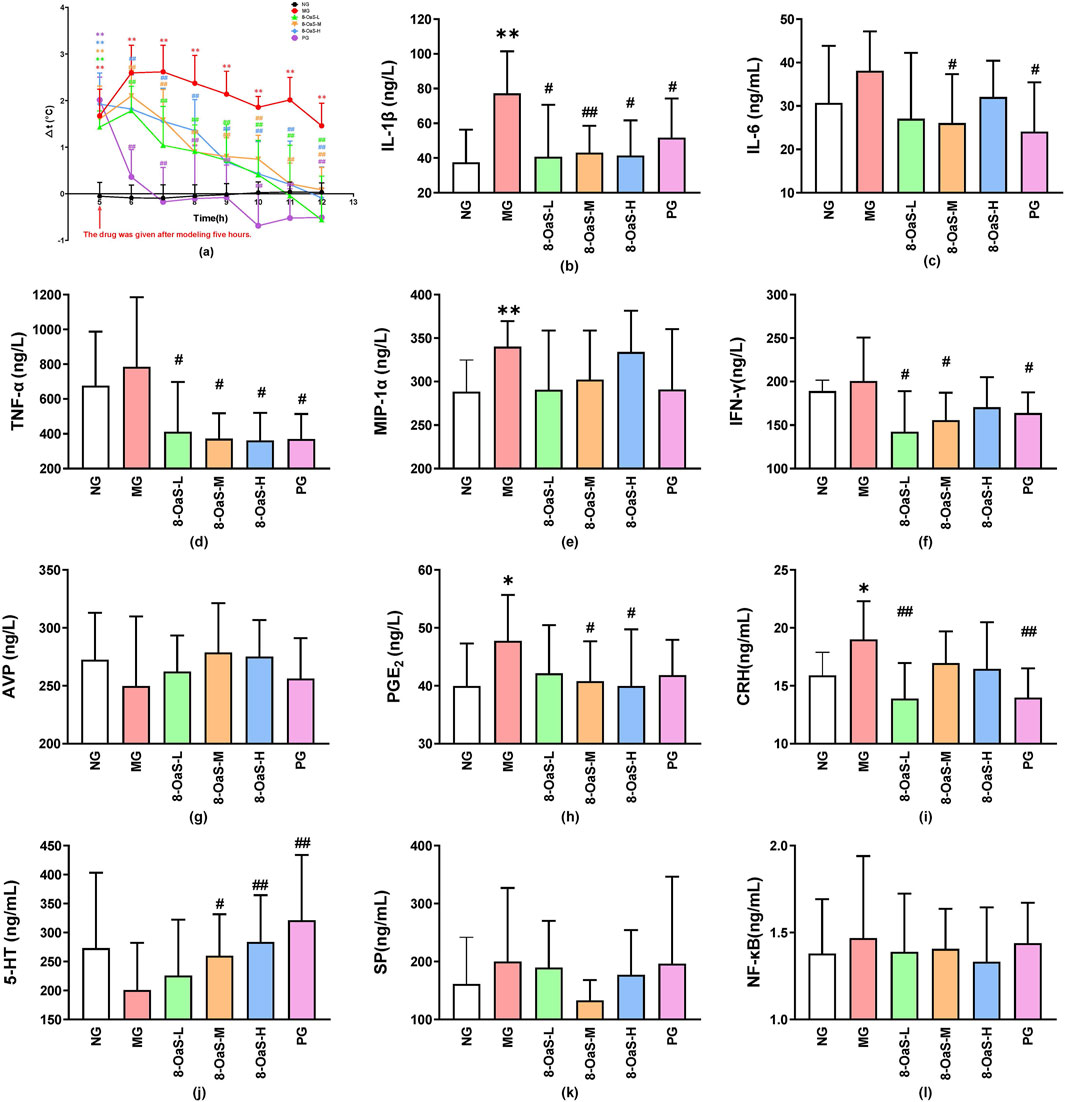
Figure 1. The antipyretic and anti-inflammatory effects of 8-OaS in yeast-induced pyrexic rats. (a) Temperature change, (b) IL-1β, (c) IL-6, (d) TNF-α, (e) MIP-1α, (f) IFN-γ, (g) AVP, (h) PGE2, (i) CRH (j) 5-HT (k) SP, and (l) NF-κB (n = 12). Note: Compared with the control group, *P < 0.05, **P < 0.01; compared with the fever model group, #P < 0.05, ##P < 0.01.
Serum cytokines, thermoregulatory mediators, neurotransmitters were closely associated to the degree of fever (Figures 1b–l). ELISA was employed to assess the alterations in cytokine levels within serum. The concentrations of IL-1β, IL-6, TNF-α, MIP-1α, IFN-γ, PGE2, CRH, SP, and NF-κB in the MG were found to be elevated. Conversely, the serum AVP, 5-HT of the MG was decreased. The above results indicated that the yeast-induced pyrexia model in rats was successfully established. After the administration of 8-OaS, the above indicators tended to recover to the normal levels.
The mRNA expression of the hypothalamus was detected by PCR. The findings are presented in Figures 2a–f. Compared with the NG, the mRNA expressions of TNF-α, IL-6, IL-1β, NF-κB, TRPV4, and HSP70 in the MG were significantly upregulated (P < 0.01), indicating that the expression of mRNA was enhanced under fever conditions. Compared with the MG, the mRNA expressions of TNF-α, IL-6, IL-1β, NF-κB, TRPV4, and HSP70 in the 8-OaS dose groups and the PG were significantly downregulated (P < 0.01).
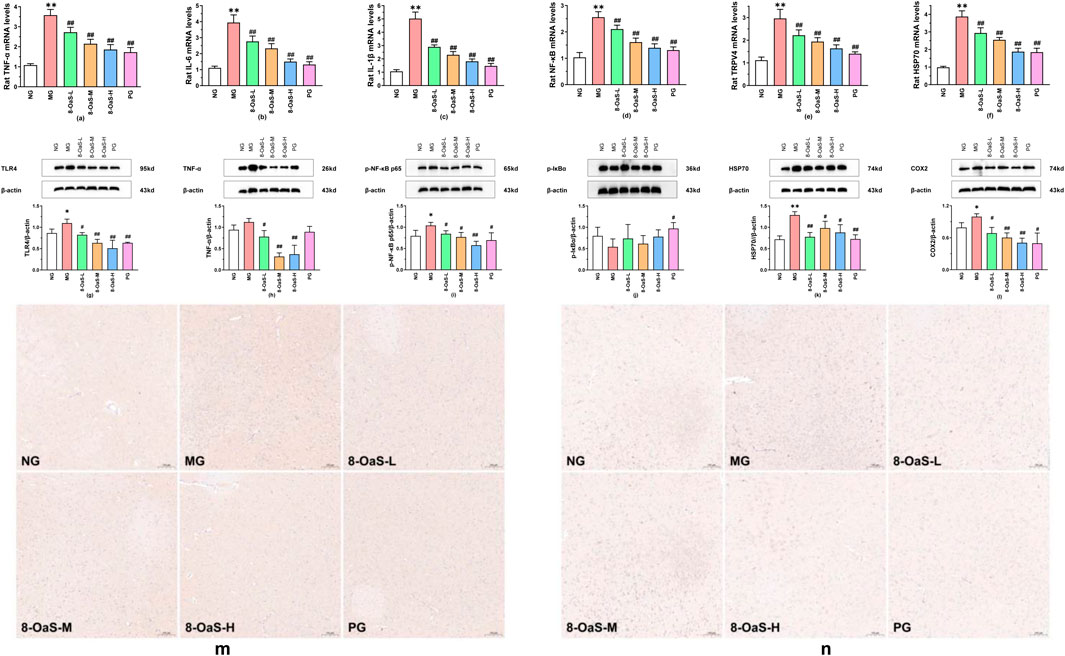
Figure 2. Effects of 8-OaS on the mRNA and proteins in yeast-induced pyrexic rats. (a-f): Effects of 8-OaS on the mRNA expression levels of TNF-α (a), IL-6 (b), IL-1β (c), NF-κB (d), TRPV4 (e), and HSP70 (f) in the hypothalamus of yeast-induced pyrexic rats. (g-l) Effects of 8-OaS on the proteins expression levels of TLR4 (g), TNF-α (h), p-NF-κB p65 (i), p-Iκ Bα (j), HSP70 (k), and COX2 (l) expression levels in the hypothalamus of yeast-induced pyrexia in rats. (m,n) Representative immunohistochemical staining results of IL-1β and IL-6 proteins in the hypothalamus (100 μm). Note: Compared with the normal control group, *P < 0.05, **P < 0.01; compared with the fever model group, #P< 0.05, ##P < 0.01.
Western blotting method was used to detect the expression of proteins in the rat hypothalamus. The results are shown in Figures 2g–l. Compared with the NG, the protein expressions of TLR4 (P < 0.05), TNF-α, p-NF-κB p65 (P < 0.05), HSP70 (P < 0.01), COX2 (P < 0.05) were upregulated and the protein expression of p-IκBα was downregulated in the MG. Compared with the MG, the protein expressions of TLR4 (P < 0.05), TNF-α (P < 0.05), p-NF-κB p65 (P < 0.05), HSP70 (P < 0.01), and COX2 (P < 0.05) were significantly decreased in the 8-OaS-L, while the protein expression of p-IκBα was increased. The protein expressions of TLR4 (P < 0.01), TNF-α (P < 0.01), p-NF-κB p65 (P < 0.05), HSP70 (P < 0.01), and COX2 (P < 0.05) in the 8-OaS-M were significantly elevated, but the protein expression of p-IκBα was reduced. The protein expressions of TLR4 (P < 0.01), TNF-α (P < 0.01), p-NF-κB p65 (P < 0.01), HSP70 (P < 0.01), and COX2 (P < 0.05) were significantly downregulated and the protein expression of p-IκBα was upregulated in the 8-OaS-H. In the PG, the protein expression of TLR4 (P < 0.01), TNF-α, p-NF-κB p65 (P < 0.05), COX2 (P < 0.05) declined and the protein expression of p-IκBα significantly augmented (P < 0.05).
Immunohistochemical staining of IL-1β and IL-6 expressions in the hypothalamus revealed a significant increase in both IL-1β and IL-6 significantly increased in the MG (Figures 2m, n). Meanwhile, a marked decreased in the expressions of IL-1β and IL-6 were observed in the 8-OaS dose groups.
Changes in the intestinal flora of yeast-induced pyrexia in rats and 8-OaS alters the composition of intestinal flora
The Veen diagram presented in Figure 3a illustrates that there were 999 OTUs in the NG, with 193 of these being unique. Additionally, there were 1,026 OTUs in the MG, with 198 of these being unique, and 910 OTUs in the 8-OaS-H, with 187 of these being unique. The taxonomic richness and homogeneity of a sample are visualized by rank abundance curves (Figure 3b). As the number of test samples increases, the curves gradually flatten, evenly distributed and concentrated. With sufficient sampling, it can be used for subsequent data analysis. The NMDS analysis revealed significant structural differences among the three groups (Figure 3c). As shown by the β diversity Adonis grouping test (Table 1) and the Anosim grouping test (Table 2), the composition of the rat intestinal flora in the MG differed significantly from that of the NG and 8-OaS-H group.
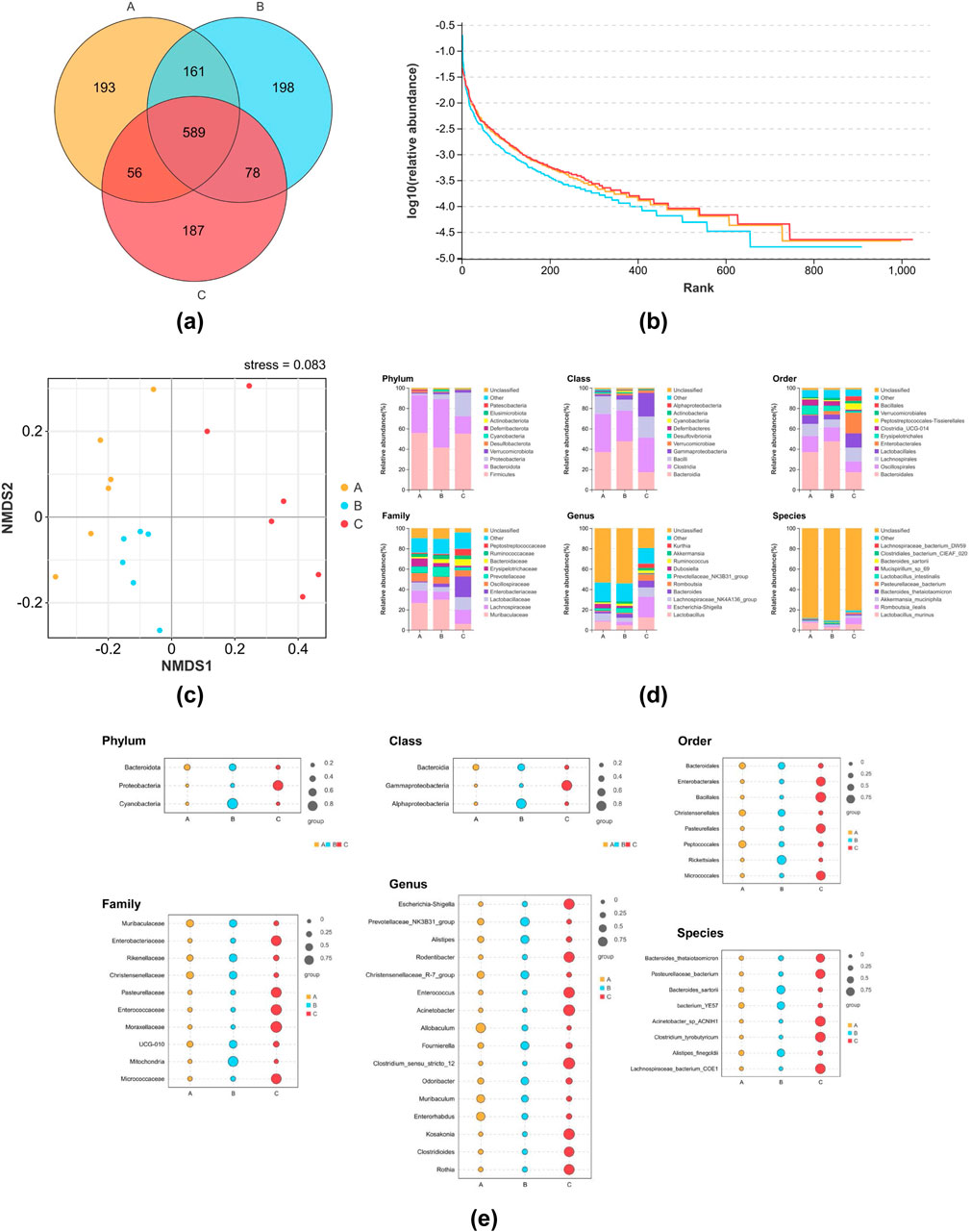
Figure 3. The effect of 8-OaS on the intestinal flora. (a) OUT Veen plots for each group; (b) Rank Abundance curves; (c) NMDS analysis based on OUT; (d) bar charts for analysis of species composition at different levels (phylum, class, order, family, genus, species); (e) bubble charts for screening and analysis of indicator species at different levels (phylum, class, order, family, genus, species). n = 6. Note: A: normal control group; B: fever model group; C: 8-OaS high dose group.
The top ten species from each group of rat intestinal flora objects, ranked according to their constitutive abundance at the taxonomic phylum, class, order, family, genus, species level, are shown in Figure 3d. After conducting fever modeling in rats and administering drug, the proportions of the top ten species exhibited distinct changes.
The analysis of indicators calculates the indicator value of every species for each subgroup, based on the abundance and frequency of occurrence of the species in the sample. A higher value indicates that the species is more likely to serve as an indicator species for that subgroup. At the phylum level (Figure 3e), Bacteroidota, Proteobacteria, and Cyanobacteria in the three comparison groups were screened as indicator species. Welch’s t-test found that compared with the NG, the relative abundance of Cyanobacteria in the MG was significantly higher (P < 0.05), while the relative abundance of Cyanobacteria in the 8-OaS group was significantly lower (P < 0.05). At the genus level (Figure 3e), Escherichia-Shigella, Prevotellaceae_NK3B31_group, Alistipes, Rodentibacter, Christensenellaceae_R-7_group, Enterococcus, Acinetobacter, Allobaculum, Fournierella, Clostridium_sensu_stricto_12, Odoribacter, Muribaculum, Enterorhabdus, Kosakonia, Clostridioides, Rothia were screened as indicator species. Welch’s t-test showed that compared with the NG, the relative abundances of Alistipes and Odoribacter in the MG were significantly increased (P < 0.01). Compared with the MG, the relative abundances of Alistipes (P < 0.01) and Odoribacter (P < 0.05) in the 8-OaS-H were significantly decreased. Bacteroides_thetaiotaomicron, Pasteurellaceae_bacterium, Bacteroides_sartorii, bacterium_YE57, Acinetobacter_sp_ ACNIH1, Clostridium_tyrobutyricum, Alistipes_finegoldii, Lachnospiraceae_bacterium_COE1 as indicator species screened at species level. Welch’s t-test showed that the relative abundance of Alistipes_finegoldii in the MG was significantly higher than that in the NG (P < 0.05). Compared with the MG, the relative abundance of Alistipes_finegoldii in the 8-OaS-H was significantly decreased (P < 0.05).
The diversity of intestinal flora was examined using the multi-level species hierarchy of LEfSe at genus level. The cladogram and LDA histogram (Figures 4a, b) indicated that 80 species exhibited differences in their relative abundances among the three groups, including 17, 41, and 22 species belonging to the NG, MG, and 8-OaS-H, respectively. The relative abundances of Clostridia_UCG_014, Eubacterium_coprostanoligenes_group, UCG_005, Acidaminococcaceae, Negativicutes, Acidaminococcales, Phascolarctobacterium, Prevotella and Allobaculum were abundant in the NG, while those of Bacteroidota, Bacteroidia, Bacteroidales, Muribaculaceae, Prevotellaceae, Prevotellaceae_NK3B31_group, Rikenellaceae, Alistipes, Erysipelatoclostridiaceae, Erysipelatoclostridium, NK4A214_group, Christensenellaceae, Christensenellales, Christensenellaceae_R_7_group, Mitochondria, Rickettsiales, RF39, Marinifilaceae, Clostridia_vadinBB60_group, and Butyricimonas were abundant in the MG. Moreover, the relative abundances of Lactobacillales, Pasteurellaceae, Pasteurellales, Rodentibacter, Enterococcus, Enterococcaceae, Clostridium_sensu_stricto_12 and Nesterenkonia were enriched in the 8-OaS-H. To explore the potential effects of 8-OaS intervention on metabolic pathways of intestinal microflora in rats induced with yeast, we conducted PICRUSt 2 analysis based on the KEGG database (Figure 4c). In contrast to rats of the MG, multiple microbial metabolic pathways were significantly changed in 8-OaS-fed rats (8-OaS-H), which were mainly characterized by the regulation of carbohydrate metabolism, metabolism of cofactors and vitamins, amino acid metabolism, metabolism of terpenoids and polyketides, metabolism of other amino acids and so on.
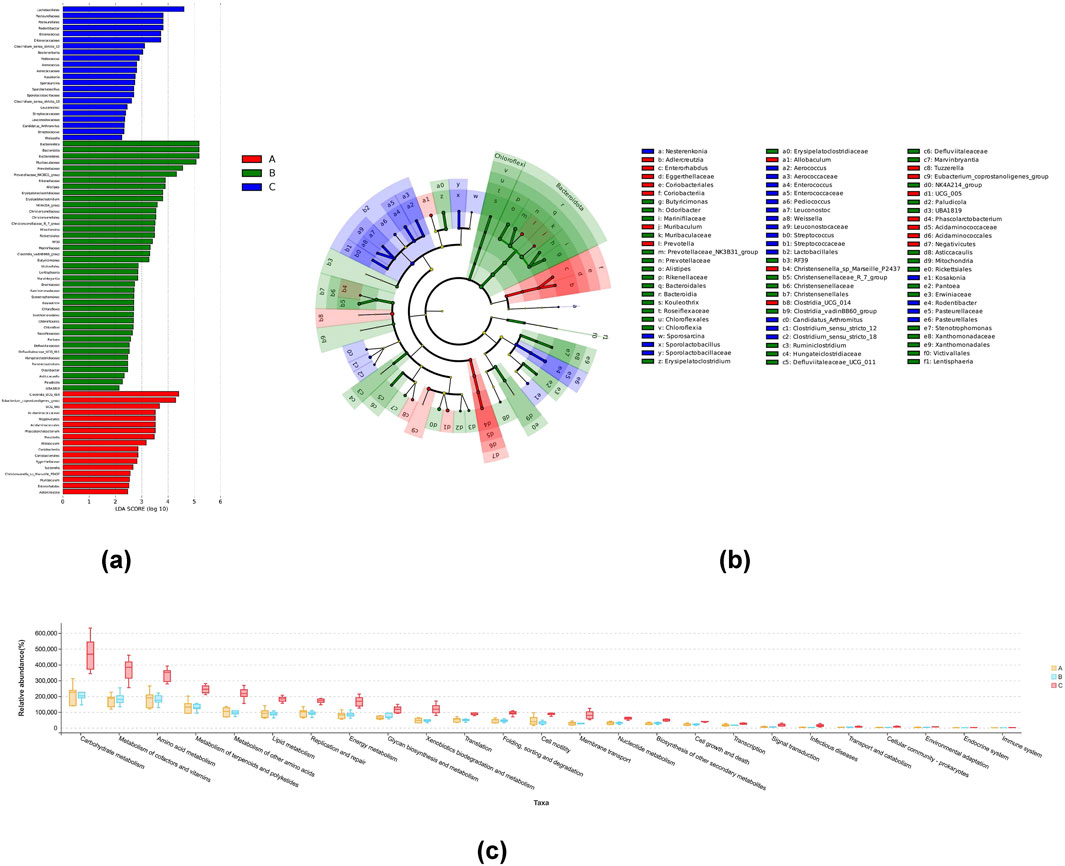
Figure 4. LEfSe analysis and the differences in metabolic pathways among different groups. LDA distribution of LEfSe results (a) based on the classification information in the genus level andthe taxonomic cladogram generated by LEfSe analysis (b). The threshold value of the LDA score was set to 2, and an LDA score >2 was considered significant. n = 6. (c) Microbial metabolic function of intestinal flora predicted by PICRUSt2. Note: A: normal control group; B: fever model group; C: 8-OaS high dose group.
Utilizing metabolomic biomarkers in hypothalamic tissues as pharmacodynamic surrogate indices to elucidate the antipyretic and anti-inflammatory effects of 8-OaS
Neurotransmitter metabolites and its variation trends in hypothalamic tissues were showed in Figure 5; Table 3. In hypothalamic tissue, neurotransmitters of the phenylalanine metabolic pathway are impacted. Compared with NG, the contents of phenylalanine and DL-adrenaline were found to be reduced in MG; however, these differences did not reach statistical significance. The contents of tyramine, dopamine and homovanillic acid were significantly decreased (P < 0.01). The levels of tyrosine and NE were significantly increased (P < 0.01), and the levels of DL-metanephrine were increased but the differences were not statistically significant. Compared with MG, the contents of phenylalanine, tyrosine, tyramine, DL-adrenaline and NE in 8-OaS-H were significantly decreased (P < 0.01), the contents of dopamine and homovanillic acid were significantly increased (P < 0.01), and the content of DL-metanephrine was increased but the difference was not statistically significant. In the neurotransmitters of L-tryptophan metabolic pathway, compared with the NG, the levels of L-tryptophan, KYN and 5-HT were significantly increased in the MG (P < 0.01). In contrast, while the level of 5-HTP was increased, the differences observed were not statistically significant. The contents of 5-HIAA (P < 0.01) and tryptamine were decreased. Compared with MG, the contents of L-tryptophan, KYN, 5-HT and tryptamine in 8-OaS-H were significantly decreased (P < 0.01), while the contents of 5-HTP were decreased but the difference was not statistically significant. The contents of 5-HIAA was significantly increased. In the neurotransmitters of glutamate metabolic pathway, the contents of glutamate, GABA and glutamine in MG were increased compared with NG, and the difference of GABA content was statistically significant (P < 0.01). Compared with the MG, the contents of glutamic acid (P < 0.01), GABA and glutamine in the 8-OaS-H were decreased. In the neurotransmitters of histidine metabolic pathway, the contents of histidine (P < 0.01) and histamine (P < 0.01) in the MG were significantly elevated compared with NG. Compared with MG, the content of histidine (P < 0.01) and histamine decreased in the 8-OaS-H. In the neurotransmitter of arginine metabolic pathway, the level of arginine in MG was significantly increased compared with NG (P < 0.01). Compared with MG, the level of arginine in 8-OaS-H was significantly decreased (P < 0.01). Among the neurotransmitters of the choline metabolic pathway, choline in the fever model group compared with NG, and the content of acetylcholine increased (P < 0.01). Compared with MG, the contents of choline and acetylcholine increased in the 8-OaS-H, but there was no statistical significance.
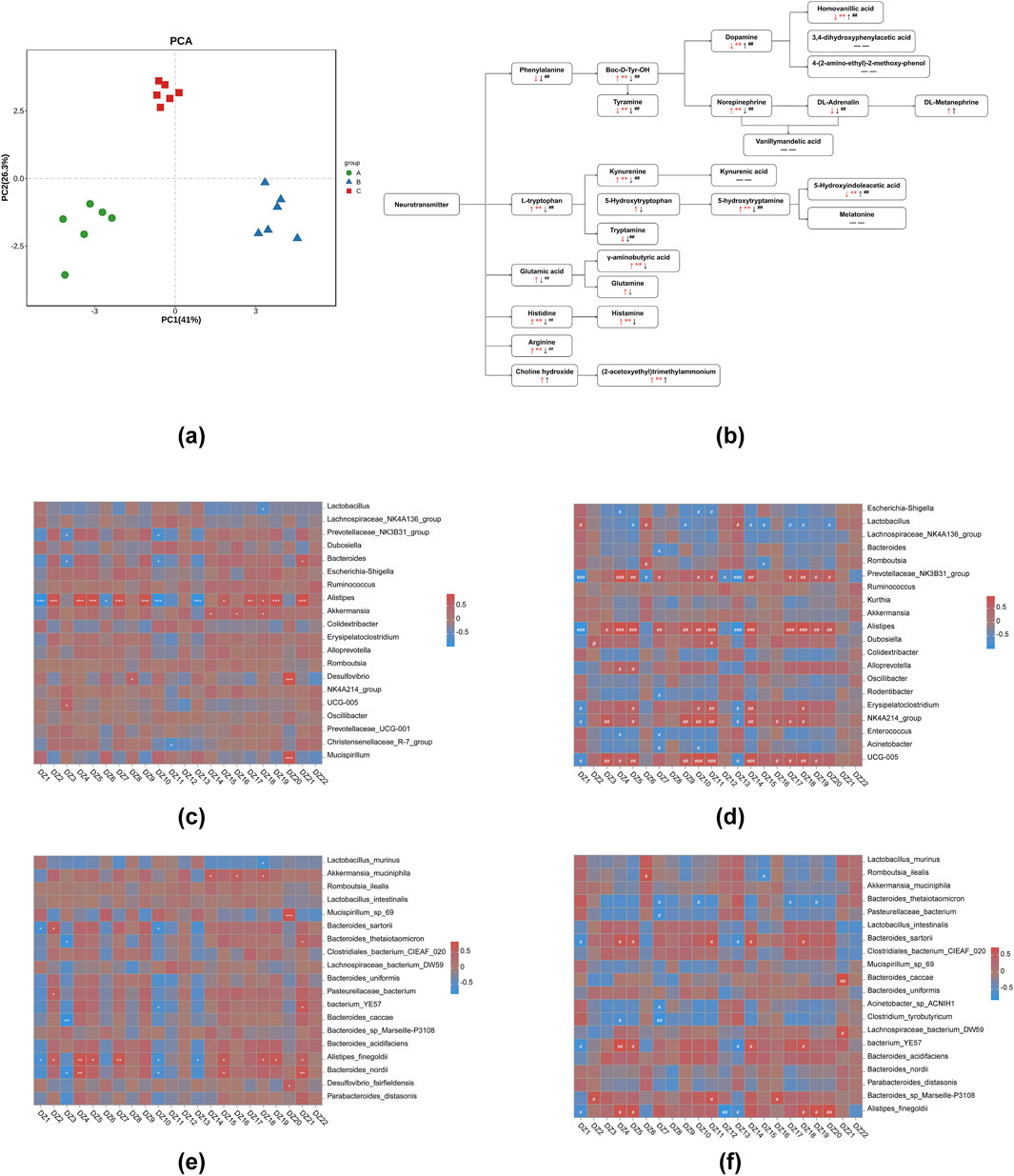
Figure 5. Metabolic analysis of potential biomarkers in hypothalamic tissues. (a) PCA analysis; (b) the significant difference of 8-OaS on neurotransmitter metabolism in hypothalamic tissue of yeast-induced pyrexia in rats (Ma et al., 2021) (Note: ↑: represents increase; ↓: represents decrease; arrows are red when the fever model group is compared with the control group; arrows are black when 8-OaS-H is compared with the fever model group. — —: indicates that the device is not detected.); (c) the heatmap of correlation analysis at genus level between NG and MG; (d) the heatmap of correlation analysis at genus level between MG and 8-OaS-H; (e) the heatmap of correlation analysis at species level between NG and MG; (f) the heatmap of correlation analysis at species level between MG and 8-OaS-H. (DZ1: 5-Hydroxyindoleacetic acid (5-HIAA); DZ2: Histamine; DZ3: DL-Adrenaline; DZ4: Norepinephrine (NE); DZ5: 5-hydroxytryptamine (Seronin; 5-HT); DZ6: Dopamine; DZ7: Histidine; DZ8: 5-Hydroxytryptophan (HTP); DZ9: Kynurenine (KYN); DZ10: Tyramine; DZ11: Tryptamine; DZ12: DL-METANEPHRINE; DZ13: Homovanillic acid; DZ14: Glutamic acid; DZ15: γ-Aminobutyric acid (GABA); DZ16: Glutamine; DZ17: Boc-D-Tyr-OH(Tyrosine); DZ18: Arginine; DZ19: L-tryptophan; DZ20: Phenylalanine; DZ21: (2-acetoxyethyl)trimethylammonium; DZ22: Choline hydroxide.). Note: Compared with control group, *P < 0.05, **P < 0.01, ***P < 0.01; Compared with the fever model group, #P < 0.05, ##P < 0.01, ###P < 0.01. A: normal control group (NG); B: fever model group (MG); C: 8-OaS high dose group (8-OaS-H).
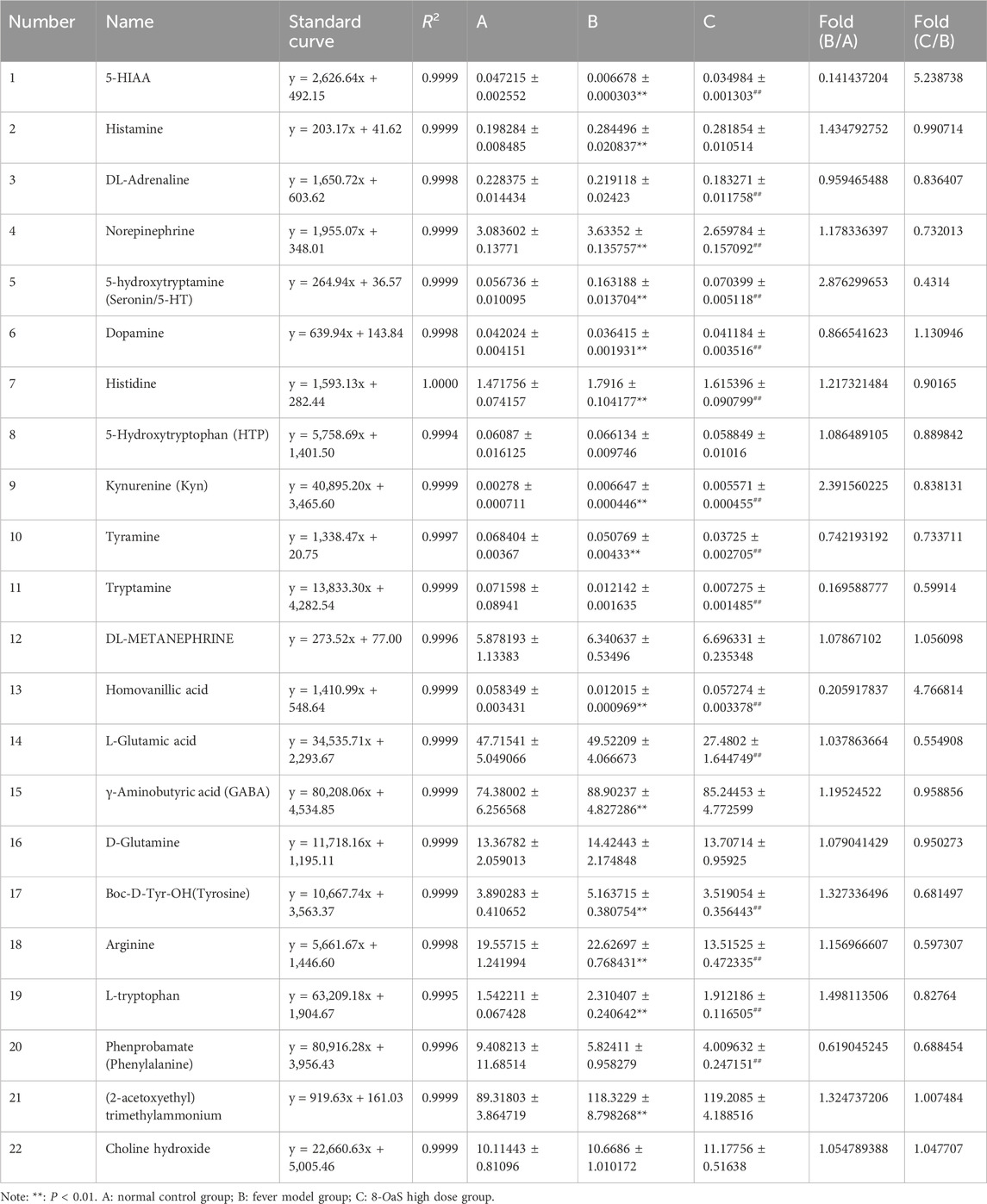
Table 3. Hypothalamic neurotransmitter metabolite standard curves and levels in yeast-induced pyrexic rats.
The pearman correlation analysis of intestinal flora and metabolomics between 8-OaS-H and the MG at the genus level (Figure 5d). It was worth noting that Alistipes have significant negative associated with 5-HIAA and homovanillic acid, and a significant positively associated with DL-adrenaline, NE, 5-HT, histidine, KYN, tyramine, tryptamine, glutamic acid, tyrosine, arginine, L-tryptophan and phenylalanine.
The pearman correlation analysis of intestinal flora and metabolomics between 8-OaS-H and the MG at the species level (Figure 5f). It is worth noting that Alistipes_finegoldii showed a significant negative correlation with 5-HIAA, DL-METANEPHRINE and homovanillic acid and a significant positive correlation with NE, 5-HT, arginine, L-tryptophan and phenylalanine.
Utilizing metabolomic biomarkers in colon contents to elucidate the antipyretic and anti-inflammatory effects of 8-OaS
In colon contents (Figure 6; Table 4), the neurotransmitters associated with the phenylalanine metabolic pathway are affected. Compared with NG, the contents of phenylalanine, tyrosine, tyramine, dopamine, NE and 3-methoxytyramine(3-MT) in MG were significantly decreased (P < 0.01), while the content of DL-metanephrine was significantly increased (P < 0.01). The content of DL-adrenaline was increased. Compared with MG, the contents of phenylalanine, tyrosine, NE, DL-adrenaline, DL-metanephrine and 3-MT in 8-OaS-H were significantly increased (P < 0.01), while the content of tyramine was increased and dopamine content decreased, but the differences were not statistically significant. In the neurotransmitters of L-tryptophan metabolic pathway, compared with NG, the contents of 5-HT and 5-HIAA acid were significantly increased in MG (P < 0.01), while the content of L-tryptophan was increased but the difference was not statistically significant. The contents of KYN, 5-HTP and tryptamine were significantly decreased (P < 0.01). Compared with MG, the levels of 5-HT and 5-HIAA acid in 8-OaS-H were significantly decreased (P < 0.01), while the contents of L-tryptophan and KYN were significantly increased (P < 0.01). The content of 5-HTP and tryptamine (P < 0.01) was decreased, but the difference of 5-HTP was not statistically significant. In the neurotransmitter of glutamate metabolic pathway, compared with NG, the content of GABA acid in MG was significantly increased (P < 0.01), and the contents of glutamate and glutamine were significantly decreased (P < 0.01). Compared with the MG, the content of GABA in 8-OaS-H was significantly decreased (P < 0.01), and the contents of glutamate and glutamine were significantly increased (P < 0.01). In the neurotransmitters of the histidine metabolic pathway, the content of histidine in MG was significantly decreased (P < 0.01) and the content of histamine was significantly increased (P < 0.01) compared with the NG. Compared with MG, the histamine and histidine contents of 8-OaS-H were significantly increased (P < 0.01). In the neurotransmitter of arginine metabolic pathway, the content of arginine in MG decreased compared with NG. Compared with MG, the content of arginine in 8-OaS-H was significantly increased (P < 0.01). In the neurotransmitters of choline metabolic pathway, the contents of choline and Ach in MG were significantly increased compared with NG (P < 0.01). Compared with MG, the choline content in 8-OaS-H was decreased without statistical significance, but the Ach content was significantly increased (P < 0.01).
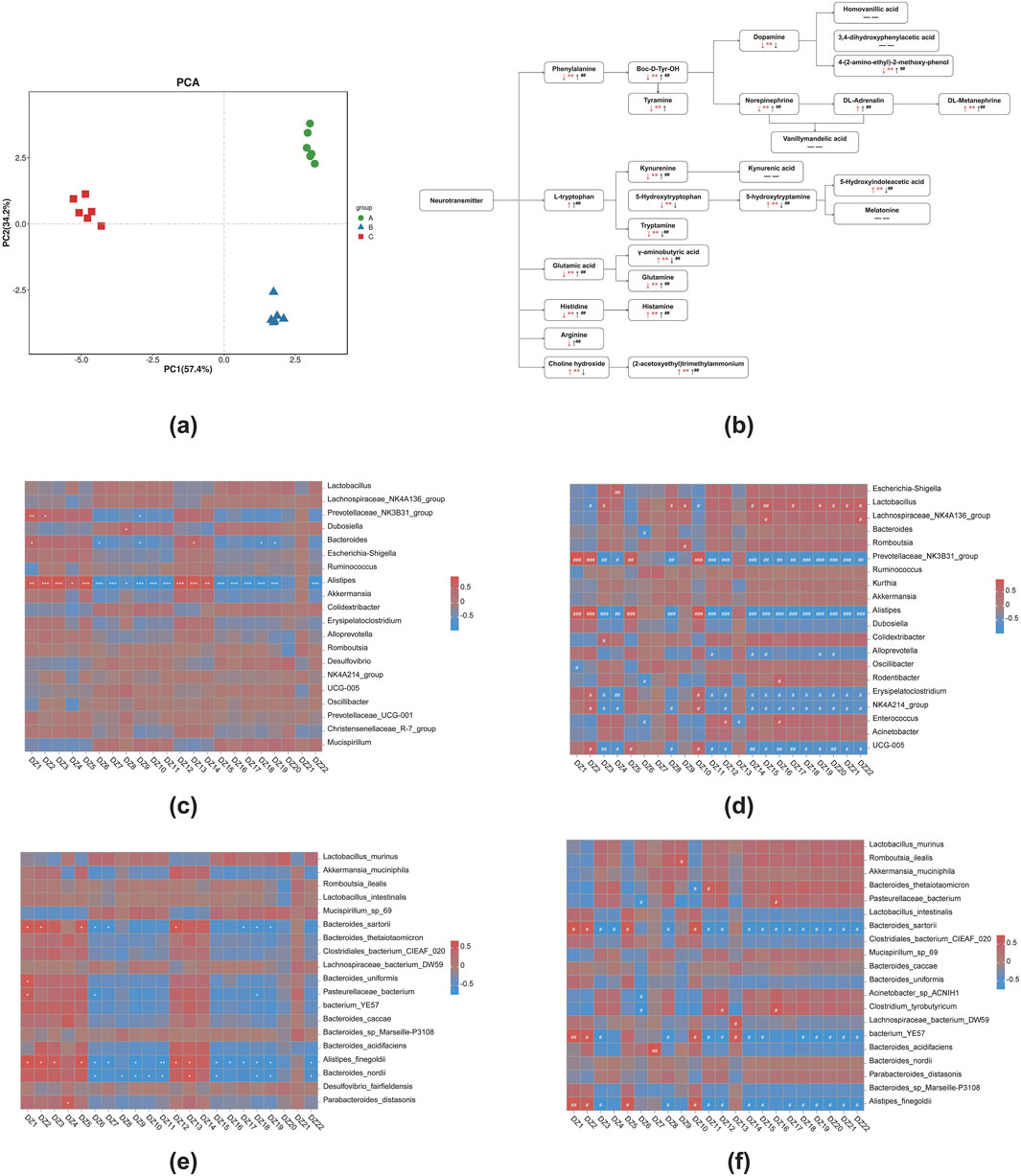
Figure 6. Metabolic analysis of potential biomarkers in colon contents. (a) PCA analysis; (b) the significant difference of 8-OaS on neurotransmitter metabolism in colon contents of yeast-induced pyrexia in rats (Ma et al., 2021) (↑: represents increase; ↓: represents decrease; arrows are red when the fever model group is compared with the control group; arrows are black when 8-OaS-H is compared with the fever model group. — —: indicates that the device is not detected.); (c) the heatmap of correlation analysis at genus level between NG and MG; (d) the heatmap of correlation analysis at genus level between MG and 8-OaS-H; (e) the heatmap of correlation analysis at species level between NG and MG; (f) the heatmap of correlation analysis at species level between MG and 8-OaS-H. (DZ1: 5-Hydroxyindoleacetic acid (5-HIAA); DZ2: γ-Aminobutyric acid (GABA); DZ3: Histamine; DZ4: DL-Adrenaline; DZ5: 5-hydroxytryptamine (Seronin; 5-HT); DZ6: Dopamine; DZ7: 5-Hydroxytryptophan (HTP); DZ8: Kynurenine (KYN); DZ9: Tyramine; DZ10: Tryptamine; DZ11: 4-(2-amino-ethyl)-2-methoxy-phenol (3-methoxytyramine); DZ12 (2-acetoxyethyl)trimethylammonium (acetylcholine; Ach); DZ13: Choline hydroxide; DZ14: DL-METANEPHRINE; DZ15: Glutamic acid; DZ16: Norepinephrine; DZ17: Glutamine; DZ18: Histidine; DZ19: Boc-D-Tyr-OH(Tyrosine); DZ20: Arginine; DZ21: L-tryptophan; DZ22: Phenylalanine.). Note: Compared with control group, *P < 0.05, **P < 0.01, ***P < 0.01; Compared with the fever model group, #P < 0.05, ##P < 0.01, ###P < 0.01. A: normal control group (NG); B: fever model group (MG); C: 8-OaS high dose group (8-OaS-H).
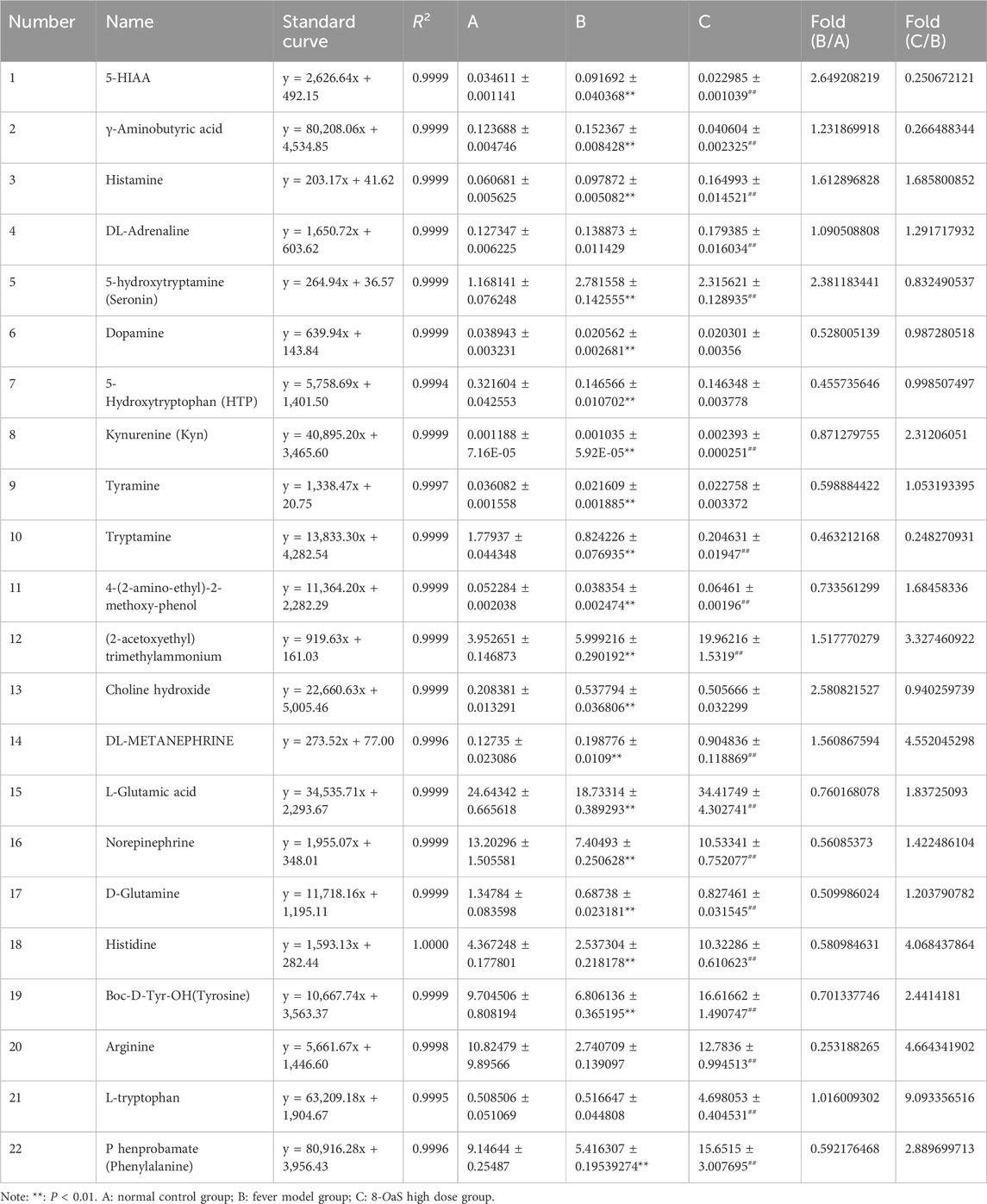
Table 4. Standard curves and levels of neurotransmitter metabolites in colon contents of yeast-induced pyrexic rats.
The pearman correlation analysis of intestinal flora and metabolomics were performed between 8-OaS-H and the MG at the genus level (Figure 6d). Alistipes showed a significant negative correlation with histamine, DL-adrenaline, KYN, 3-methoxytyramine, Ach, DL-metanephrine, glutamic acid, NE, glutamine, histidine, tyrosine, arginine, L-tryptophan and phenylalanine and a significant positive correlation with 5-HIAA, GABA, 5-HT and tryptamine. At the species level (Figure 6f), It is worth noting that Alistipes_finegoldii showed a significant negative correlation with histamine, KYN, 3-methoxytyramine, Ach, DL-metanephrine, glutamic acid, glutamine, histidine, tyrosine, arginine, L-tryptophan and phenylalanine and a significant positive correlation with 5-HIAA, GABA, 5-HT and tryptamine between 8-OaS-H and the MG.
Discussion
Phlomoides rotata contains a variety of chemical constituents such as iridoid glycosides, flavonoids and phenylethanol glycosides (Cui et al., 2020). Studies have shown that the iridoid glycosides extracts of P. rotata have a wide range of biological activities (Lei et al., 2023; Zheng et al., 2015). Among them, 8-OaS has multiple pharmacological effects, including analgesia, neuroprotection, and anti-anxiety (Li Y. J. et al., 2023; Sun et al., 2020; Zhang et al., 2018; Zhang et al., 2014). Our research group established a yeast-induced pyrexia model in rats, monitored the anal temperature of rats at various time points, selects three representative cyclohexene ether iridoid glycosides, namely, shanzhiside methylester, 8-OaS, and 8-deoxyshanzhiside, from P. rotata to investigate their antipyretic effects. In comparison to the fever model group, all treatment groups demonstrated antipyretic effects at 5 hours post-administration. Regarding the extent of body temperature reduction, the efficacy was ranked as follows: 8-OaS>8-deoxyshanzhiside > shanzhiside methylester, suggesting that 8-OaS displayed the most pronounced antipyretic effect and was comparable to that of the positive control group.
In this study, yeast-induced pyrexia model in rats was established by subcutaneous injection of 20% dry yeast on the back. After 5 h of modeling, compared with the NG, the body temperature of the other five groups showed a significant upward. Serum cytokines TNF-α, IL-6, IL-1β, MIP-1α, and IFN-γ were increased in the MG. Thermoregulatory mediator AVP and neurotransmitter 5-HT were reduced, PGE2, CRH, SP and serum NF-κB were higher in the MG. Compared with the MG, 8-OaS reversed the alterations in serum levels of cytokines in rats with yeast-induced pyrexia. Therefore, 8-OaS has a significant inhibitory effect on yeast-induced pyrexia in rats. It is suggested that the antipyretic mechanism may be related to down-regulating the expression of inflammatory factors and positive body temperature regulator, up-regulating the expression of negative body temperature regulator, and altering the content of neurotransmitters (5-HT) in serum.
The TLR4/NF-κB pathway is a bridge between exogenous and endogenous pyrogens in the fever progress. External stimuli cause TLR4 to undergo a series of phosphorylation cascade reactions, inducing phosphorylation and degradation of the NF-κB inhibitory protein IκB, which in turn leads to NF-κB activation and nuclear translocation. This triggers the expression and release of a variety of inflammatory factors (IL-1, IL-6, TNF-α, NO, and IFN-γ), leading to increased synthesis of COX-2 in the hypothalamus and elevated release of PGE2. Meanwhile, phospholipase C (PLC) was hydrolyzed to arachidonic acid (AA), which was catalyzed by COX-2 to produce PGE2. Endogenous pyrogens promote the release of positive thermoregulatory mediators, causing the thermoregulatory point to rise. It also acts on the negative thermoregulatory center to release negative regulatory mediators and inhibit hyperthermia (Evans et al., 2015; Cannon, 2013; Wrotek et al., 2020). The TLR4/NF-κB signaling pathway activated by various factors is critical for the fever course. In recent years, the antipyretic mechanisms of certain Chinese medicines, herbal extracts, and formulations (such as Tetrastigma hemsleyanum polysaccharide and baicalin) have been reported to be closely associated with this pathway. (Fu et al., 2024; Ye et al., 2015). As a result of this experiment, we found that TLR4 and NF-κB levels were elevated in rats with yeast-induced pyrexia. 8-OaS significantly inhibited the expression of TLR4 and NF-κB while elevating the p-IκB. It is suggested that 8-OaS can exert antipyretic effects through TLR4/NF-κB pathway. HSP70 family is a protective important molecule under heat stress state, rarely expressed in normal cells, but express in the heat stress (Ghadhanfar et al., 2019). Meanwhile, it has been shown that HSP70 limits body temperature increases by inhibiting NF-κB activation and endogenous thermogenesis (Lee et al., 2012). The results indicated that the expression levels of HSP70 mRNA and protein were elevated in rats with yeast-induced pyrexia, which was inhibited by 8-OaS. Transient receptor potential vanilloid 4 (TRPV4), a member of the transient receptor potential ion channel family, is widely expressed in the body, particularly in the preoptic area of the hypothalamus, and plays a role in regulating body temperature. By replicating the zebra fish fever model, it was found that TRPV1, TRPV4, and PGE2 co-regulated the development of fever, and the decreased expression of TRPV4 contributed to the generation of fever (Lee et al., 2005). However, Wang and colleagues (Wang et al., 2008) found that TRPV4 was increased in the hypothalamus of LPS-induced pyrexia in rats, which led to an increase in calcium influx and then upregulation of cAMP expression to mediate the fever process. TRPV4 was activated to participate in thermoregulation, but the specific mechanism of activation has not been clarified. The results showed that TRPV4 mRNA expression was increased in the MG. Nonetheless, the treatment of 8-OaS resulted in a downregulation of TRPV4 mRNA expression. The COX2/PGE2/EP3/cAMP pathway in the hypothalamus is an important positive regulatory pathway during fever (Zhang Y. Q. et al., 2022). The results of this study revealed that the expression of COX2 in the hypothalamus was elevated during fever, and decreased after 8-OaS administration. To summarise, 8-OaS reduces thermogenic cytokines and downregulates central thermoregulatory mediator PGE2. In addition, the mechanism of antipyretic effect of 8-OaS involves several pathways such as the TLR4/NF-κB pathway and the HSP70/NF-κB pathway.
The results of this experimental study showed that, at the genus level, the relative abundance of Alistipes and Odoribacter was significantly higher after model establishment and significantly lower following drug administration. At the species level, the relative abundance of Alistipes_finegoldii was significantly increased after modeling and significantly decreased after drug administration. Alistipes, Alistipes_finegoldii and Odoribacter have pro-inflammatory properties in rats with yeast-induced pyrexia. To summarise, 8-OaS decreases the abundance of Alistipes, Alistipes_finegoldii and Odoribacter thereby exerting antipyretic and anti-inflammatory effects.
Alistipes, Alistipes_finegoldii and Odoribacter are reported to be closely related to various diseases. Alistipes has been reported to have a close association with various conditions, including Alzheimer’s disease (AD), diabetes, colitis, depression and other diseases, and has a beneficial and harmful dual role (Jiang et al., 2015; Song et al., 2024; Ma et al., 2023; Zhang Q. et al., 2022; Wang et al., 2021; Wu et al., 2021; Vogt et al., 2017). Alistipes with pro-inflammatory properties are significantly increased in the obese population (Andoh et al., 2016; Xu et al., 2022). Under high-temperature conditions, Alistipes level in the intestinal flora of broilers was increased, whereas it was reduced in the antibiotic group (Li S. et al., 2023). Alistipes finegoldi is a gram-negative anaerobic bacterium, belongs to the genus Alistipes (Parker et al., 2020). The study found that A. finegoldi are closely related to colon cancer and inflammatory bowel disease (Radka et al., 2020; He et al., 2022; Yin et al., 2022; Zhao et al., 2020; Kang et al., 2023; Dai et al., 2018). Odoribacter is strictly anaerobic and is negatively correlated with the severity of NAFLD and inflammatory bowel disease, due to its anti-inflammatory activity by producing short-chain fatty acids (SCFAs) such as butyric acid (Xie et al., 2023). Odoribacter splanchnicus has also been associated with a positive response to fecal transplantation therapy in patients with ulcerative colitis, which correlates with reduced intestinal inflammation (Saleh et al., 2023; Zhang et al., 2020). However, it has also been suggested that Odoribacter has pro-inflammatory properties and is pathogenic: Odoribacter was inhibited in colitis mice with the human strain transplantation treatment (Dai et al., 2024). The probiotic mixture VSL#3 reduced the relative abundance of Oscillibacter in the intestinal flora of colitis-associated carcinogenesis mice model (Wang et al., 2018).
As a crucial source of peripheral neurotransmitters and hormones, intestinal flora exerts both direct and indirect effects on hypothalamic neurons via the bidirectional gut-brain axis connection. Given the pivotal role of the hypothalamus in thermoregulation, it is posited that intestinal flora may regulate heat production and body temperature via neurotransmitters within the gut-brain axis.
In the phenylalanine pathway, this study showed a significant decline in phenylalanine content in hypothalamic tissue after modeling and a significant decrease after 8-OaS administration. In contrast, the phenylalanine content was significantly reduced after modeling and significantly increased after 8-OaS administration in the colon contents. Phenylalanine is converted to tyrosine in the production of neurotransmitters, which are then converted to dopamine, NE, and DL-adrenaline. Some studies have found that serum phenylalanine decreased in the LPS induced fever model, and Huanglian-Jiedu decoction can reverse this phenomenon (Li et al., 2021).
In the tryptophan pathway, this study showed increased levels of tryptophan, KYN, 5-HTP and 5-HT and decreased levels of 5-HIAA and tryptamine in hypothalamic tissue after modeling. However, after 8-OaS administration, hypothalamic levels of tryptophan, KYN, 5-HTP, 5-HT and tryptophan were significantly decreased, and the levels of 5-HIAA were significantly higher. In contrast, the levels of tryptophan, 5-HT and 5-HIAA in colonic contents were increased and the levels of 5-HTP, KYN and tryptamine were decreased after modeling. But the levels of 5-HTP, 5-HT, 5-HIAA and tryptamine were decreased, and the levels of tryptophan, KYN were significantly increased after 8-OaS administration.
Alistipes could convert tryptophan to indole, have tryptophan metabolizing activity (Parker et al., 2020). Correlation analysis results showed that the relative abundance of Alistipes in intestinal flora significantly increased after modeling, and significantly decreased after 8-OaS administration. There was a significant positive correlation between Alistipes and body temperature, tryptophan, and 5-HT. Changes in Alistipes levels play an important role in regulating body temperature. Li et al. also found that compared with the room temperature control group, the relative abundance of Alistipes in the cecum of the high-temperature control group was increased, accompanied by increased expression of 5-HT2A and TPH2 (P < 0.05) (Li S. et al., 2023). Peripheral 5-HT cannot cross the BBB into the CNS, indicating that the central and peripheral 5-HT systems are completely independent. However, tryptophan, a precursor of 5-HT, can cross the BBB and then be metabolized to 5-HT through the catalytic conversion of central TPH2 or to KYN through the TDO pathway. In this study, although 5-HT cannot directly cross the BBB, tryptophan and its metabolic enzymes can increase the hypothalamic 5-HT content, which may link Alistipes with brain function. Furthermore, the function of central and peripheral 5-HT is different. Hypothalamic 5-HT is an important neurotransmitter involved in regulating physiological processes such as sleep, mood, and body temperature. It has been reported that the tryptophane-5-HT metabolic pathway in the hypothalamus is involved in the regulation of brain neurotransmitters, and intracerebroventricular administration of 5-HT can prevent hypotension and hypothermia (Mota et al., 2019). Systemic 5-HT-induced inhibition of BAT SNA requires GABAergic inhibition of BAT sympathetic excitatory neurons in the DMH. In addition, the endogenous release of 5-HT from rRPa (pallid nucleus of the medullary cephalic intermediate suture) inhibits BAT SNA during warming (Mota et al., 2020). In conclusion, the changes in Alistipes abundance and hypothalamic 5-HT content may be the potential causes of thermoregulation in rats with yeast-induced pyrexia.
The glutamic acid metabolic pathway also plays an important role in the fever process. As an important neurotransmitter for synaptic excitation in the central nervous system, it acts a crucial role in rapid synaptic transmission, learning and memory, as well as the development of the nervous system. Glutamatergic nerves distributed in the preoptic area (POA) of the hypothalamus and the glutamatergic ionotropic receptor NMDA play an important role in thermoregulation and fever (Machado et al., 2020; Krajewski-Hall et al., 2019; Hou et al., 2011; Simm et al., 2016; Morrison, 2016). Microinjection of L-glutamic acid (0.14 nM) into the POA of awake rats caused an increase in body temperature and brain temperature (Sengupta et al., 2014). Glutamic acid increases body heat production and decreases heat dissipation through NMDA, resulting in a fever effect. The application of NMDA-receptor antagonists can significantly reduce glutamate-induced fever (Hou et al., 2011). Glutamatergic preoptic neurons expressing EP3R mediate inflammatory fever (Machado et al., 2020). The use of paracetamol reduces glutamate concentrations (Blecharz-Klin et al., 2019). AS an excitatory neurotransmitter, it is utilized by end-vascular organs and causes an increase in body temperature leading to hyperthermia (Sterkel et al., 2017). Glutamate may be involved in orexin neurons associated with PGE2-induced fever (Takahashi et al., 2013). LPS injected intraperitoneally induces an increase in glutamate (Chang et al., 2013; Huang et al., 2008; Kao et al., 2011). Activation of glutamate receptors in the dorsomedial hypothalamus (DMH) is required for COX-independent, IL-1β-induced BAT thermogenesis (Mota and Madden, 2022). This study shows that hypothalamic glutamate levels were elevated after modeling and significantly decreased after 8-OaS administration. GABA, metabolized from glutamic acid, is the major inhibitory neurotransmitter produced and regulated mainly by astrocytes and neurons. GABA levels in the hypothalamus and colon content were significantly increased after modeling and decreased after 8-OaS administration. Therefore, the alterations in glutamic acid and GABA levels may serve as potential contributors to thermoregulation in rats with yeast-induced pyrexia by 8-OaS. The intestinal flora is involved in GABA production, as manipulation of the intestinal microbiota has been shown to affect GABA levels. Several intestinal microbes have been identified as GABA producers, including members of the genera Anabacterium, Bifidobacterium, and Lactobacillus (Eicher and Mohajeri, 2022). Xiaochaihu granules alleviate yeast-induced fever by modulating inflammatory/immune responses, neuromodulation, and metabolic modules (Chen et al., 2024). They released GABA predominantly from axons in DMH and their GABAergic terminals are increased due to prolonged heat exposure. These findings suggest that tonic gabaergic inhibitory signaling EP3R series neurons from the POA are fundamental determinants of body temperature for thermal homeostasis and fever (Nakamura et al., 2022).
The results of the histidine metabolism pathway showed that the contents of histidine and histamine in the hypothalamus were increased significantly after modeling, and the contents of histidine and histamine were decreased after 8-OaS administration. Histamine in the hypothalamic neurons regulate adaptive behavior and generate heat, in response to endogenous pyrogen (Sakata et al., 1995). The PO/AH region containing thermoregulatory neurons was identified as the main site where histamine affects body temperature. Most studies have found that histamine has a hyperthermic effect (Tabarean, 2016). However, the content of histidine was decreased significantly and histamine was increased significantly in the colon content after modeling. After 8-OaS administration, histidine and histamine content were significantly increased.
Dietary L-arginine supplementation maintains intestinal epithelial integrity during exercise under heat stress through a mechanism independent of T (core) regulation (Costa et al., 2014). Our study arginine pathway results showed a significant increase in hypothalamic tissue arginine content after modeling and a significant decrease after 8-OaS administration. Arginine content of colonic contents decreased and significantly increased after 8-OaS administration, it is suggested that arginine is involved in the regulation of fever process and 8-OaS treatment.
The choline pathway also plays an important role in the mechanism of fever. As an important parasympathetic neurotransmitter, Ach increased significantly in the hypothalamus after modeling, suggesting that Ach is involved in thermoregulation, which is consistent with the literature (Fu et al., 1996). However, there was no significant change in the hypothalamus after 8-OaS administration, suggesting that the drug had little effect on hypothalamic Ach. Levels of Ach in colon contents were significantly increased after modeling and administration. Choline, a precursor of the neurotransmitter Ach, showed no significant change in hypothalamic content but significantly increased in colon contents after modeling, but had no significant effect after administration. Ventricular injection of Ach 1 mg/only in rats increases body temperature (Simpson and Resch, 1986). 5-HT and Ach cause an increase in body temperature, and NE causes a decrease in body temperature, which is maintained by antagonistic effects on neurons in the preoptic area-anterior hypothalamus. Spleen meridian herbs regulate body temperature in rats with yeast-induced fever. Administration of Astragali Radix, a herb with warming properties, exacerbated the hyperthermia in yeast-induced pyrexic rats concomitant with elevated plasma choline concentrations. The cooling herbs Nelumbinis Semen and Coicis Semen decreased body temperature in yeast-induced pyrexic rats, accompanied by an increase in plasma histidine (Huang et al., 2020). Magnetic resonance (MRS) revealed an increase in the cerebellar acetylcholine/creatine ratio in febrile patients during fever compared to after recovery (P = 0.043) (Yang and Li, 2018). Behavioral fever leads to a significant increase in cholinergic neurotransmitter and receptor activity in target tissues (Sanhueza et al., 2021). The increase in brain Ach was accompanied by a decrease in 5-HT and 5-HIAA (Rodrigues et al., 2009).
Conclusion
The 8-OaS yielded a good antipyretic and anti-inflammatory effect, and this efficacy was related to the improvement of the intestinal flora and neurotransmitters metabolites. There was a significant positive correlation between Alistipes and body temperature, tryptophan, and 5-HT. Changes in Alistipes levels play an important role in regulating body temperature. In conclusion, the changes in Alistipes abundance and hypothalamic 5-HT content may be the potential causes of thermoregulation in pyrexic rats by 8-OaS.
Data availability statement
The original contributions presented in the study are included in the article/Supplementary Material, further inquiries can be directed to the corresponding authors.
Ethics statement
The animal study was approved by the Animal Ethics Committee of the 940th Hospital of Joint Logistic Support Force of the Chinese People’s Liberation Army. The study was conducted in accordance with the local legislation and institutional requirements.
Author contributions
XL: Conceptualization, Data curation, Formal Analysis, Investigation, Methodology, Project administration, Resources, Validation, Visualization, Writing – original draft, Writing – review and editing. TL: Conceptualization, Data curation, Formal Analysis, Software, Supervision, Writing – original draft, Writing – review and editing. KL: Formal Analysis, Resources, Validation, Visualization, Writing – review and editing. ReW: Conceptualization, Data curation, Investigation, Methodology, Validation, Writing – review and editing. JY: Software, Supervision, Validation, Visualization, Writing – review and editing. YC: Investigation, Methodology, Project administration, Writing – original draft, Writing – review and editing. RoW: Funding acquisition, Methodology, Project administration, Resources, Supervision, Visualization, Writing – review and editing. ML: Data curation, Funding acquisition, Project administration, Resources, Supervision, Writing – review and editing.
Funding
The author(s) declare that financial support was received for the research and/or publication of this article. This work was supported by Supported by the Young Scientists Fund of Gansu Province (25JRRA816), the Innovation Team Project (2023YXKY001), and the Natural Science Foundation of Gansu Province (22JR11RA014).
Conflict of interest
The authors declare that the research was conducted in the absence of any commercial or financial relationships that could be construed as a potential conflict of interest.
Publisher’s note
All claims expressed in this article are solely those of the authors and do not necessarily represent those of their affiliated organizations, or those of the publisher, the editors and the reviewers. Any product that may be evaluated in this article, or claim that may be made by its manufacturer, is not guaranteed or endorsed by the publisher.
Supplementary material
The Supplementary Material for this article can be found online at: https://www.frontiersin.org/articles/10.3389/fphar.2025.1482323/full#supplementary-material
References
Andoh, A., Nishida, A., Takahashi, K., Inatomi, O., Imaeda, H., Bamba, S., et al. (2016). Comparison of the gut microbial community between obese and lean peoples using 16S gene sequencing in a Japanese population. J. Clin. Biochem. Nutr. 59, 65–70. doi:10.3164/jcbn.15-152
Blecharz-Klin, K., Wawer, A., Pyrzanowska, J., Piechal, A., Jawna-Zboińska, K., and Widy-Tyszkiewicz, E. (2019). Hypothalamus - response to early paracetamol exposure in male rats offspring. Int. J. Dev. Neurosci. 76, 1–5. doi:10.1016/j.ijdevneu.2019.05.004
Bo, T. B., Zhang, X. Y., Wen, J., Deng, K., Qin, X. W., and Wang, D. H. (2019). The microbiota-gut-brain interaction in regulating host metabolic adaptation to cold in male Brandt's voles (Lasiopodomys brandtii). Isme J. 13, 3037–3053. doi:10.1038/s41396-019-0492-y
Cannon, J. G. (2013). Perspective on fever: the basic science and conventional medicine. Complement. Ther. Med. 21S, S54–S60. doi:10.1016/j.ctim.2011.08.002
Chang, C. H., Huang, W. T., Kao, C. H., Chen, S. H., and Lin, C. H. (2013). Tetramethylpyrazine decreases hypothalamic glutamate, hydroxyl radicals and prostaglandin-E2 and has antipyretic effects. Inflamm. Res. 62, 527–535. doi:10.1007/s00011-013-0606-3
Chen, X., Wu, H., Li, P., Peng, W., Wang, Y., Zhang, X., et al. (2024). Unraveling the mechanism of xiaochaihu granules in alleviating yeast-induced fever based on network analysis and experimental validation. Pharm. (Basel). 17, 475–505. doi:10.3390/ph17040475
Costa, K. A., Soares, A. D., Wanner, S. P., Santos, R., Fernandes, S. O., Martins Fdos, S., et al. (2014). L-arginine supplementation prevents increases in intestinal permeability and bacterial translocation in male Swiss mice subjected to physical exercise under environmental heat stress. J. Nutr. 144, 218–223. doi:10.3945/jn.113.183186
Cui, Z. H., Qin, S. S., Zang, E. H., Li, C., Gao, L., Li, Q. C., et al. (2020). Traditional uses, phytochemistry, pharmacology and toxicology of Lamiophlomis rotata (Benth.) Kudo: a review. RSC Adv. 10, 11463–11474. doi:10.1039/d0ra01050b
Dai, J., Jiang, M., Wang, X., Lang, T., Wan, L., and Wang, J. (2024). Human-derived bacterial strains mitigate colitis via modulating gut microbiota and repairing intestinal barrier function in mice. BMC Microbiol. 24, 96–111. doi:10.1186/s12866-024-03216-5
Dai, Z., Coker, O. O., Nakatsu, G., Wu, W. K. K., Zhao, L., Chen, Z., et al. (2018). Multi-cohort analysis of colorectal cancer metagenome identified altered bacteria across populations and universal bacterial markers. Microbiome 6, 70–81. doi:10.1186/s40168-018-0451-2
Eicher, T. P., and Mohajeri, M. H. (2022). Overlapping mechanisms of action of brain-active bacteria and bacterial metabolites in the pathogenesis of common brain diseases. Nutrients 14, 2661. doi:10.3390/nu14132661
Evans, S. S., Repasky, E. A., and Fisher, D. T. (2015). Fever and the thermal regulation of immunity: the immune system feels the heat. Nat. Rev. Immunol. 15, 335–349. doi:10.1038/nri3843
Fu, H. Y., Guo, S. Y., Gao, Y. J., Zhou, A. X., and Tian, J. L. (1996). Exploring the mechanism of guizhi tang's bidirectional regulation of temperature: its effect on the action of acetylcholine and norepinephrine in the hypothalamus. Chin. J. Exp. Trad. Med. Form. 2, 8–10. doi:10.13422/j.cnki.syfjx.1996.02.003
Fu, S., Bao, X., Wang, Z., Tang, Y., Wu, Q., Zhu, B., et al. (2024). Antipyretic effect of inhaled Tetrastigma hemsleyanum polysaccharide on substance and energy metabolism in yeast-induced pyrexia mice via TLR4/NF-κb signaling pathway. J. Ethnopharmacol. 323, 117732. doi:10.1016/j.jep.2024.117732
Ghadhanfar, E., Pavlik, A., and Turcani, M. (2019). Effect of thermal preconditioning on Hsp70 expression in the medulla oblongata and on hemodynamics during passive hyperthermia. Brain Res. 1723, 146404. doi:10.1016/j.brainres.2019.146404
He, Q., Zhang, Y., Ma, D., Zhang, W., and Zhang, H. (2022). Lactobacillus casei Zhang exerts anti-obesity effect to obese glut1 and gut-specific-glut1 knockout mice via gut microbiota modulation mediated different metagenomic pathways. Eur. J. Nutr. 61, 2003–2014. doi:10.1007/s00394-021-02764-0
Hou, C., Xiao, J., Wang, Y., Pan, X., Liu, K., Lu, K., et al. (2024). Astaxanthin activated the SLC7A11/GPX4 pathway to inhibit ferroptosis and enhance autophagy, ameliorating dry eye disease. Front. Pharmacol. 15, 1407659. doi:10.3389/fphar.2024.1407659
Hou, C. C., Lin, H., Chang, C. P., Huang, W. T., and Lin, M. T. (2011). Oxidative stress and pyrogenic fever pathogenesis. Eur. J. Pharmacol. 667, 6–12. doi:10.1016/j.ejphar.2011.05.075
Huang, W. T., Niu, K. C., Chang, C. K., Lin, M. T., and Chang, C. P. (2008). Curcumin inhibits the increase of glutamate, hydroxyl radicals and PGE2 in the hypothalamus and reduces fever during LPS-induced systemic inflammation in rabbits. Eur. J. Pharmacol. 593, 105–111. doi:10.1016/j.ejphar.2008.07.017
Huang, Y., Yao, P., Leung, K. W., Wang, H., Kong, X. P., Dong, T. T. X., et al. (2020). The Chinese medicinal herbs of spleen-meridian property regulate body temperature in yeast-induced fever rats. Phytomedicine 74, 152815. doi:10.1016/j.phymed.2018.12.038
Jiang, H., Ling, Z., Zhang, Y., Mao, H., Ma, Z., Yin, Y., et al. (2015). Altered fecal microbiota composition in patients with major depressive disorder. Brain Behav. Immun. 48, 186–194. doi:10.1016/j.bbi.2015.03.016
Jiang, W. L., Zhang, S. P., Zhu, H. B., and Hou, J. (2011). Effect of 8-O-acetyl shanzhiside methylester increases angiogenesis and improves functional recovery after stroke. Basic Clin. Pharmacol. Toxicol. 108, 21–27. doi:10.1111/j.1742-7843.2010.00620.x
Kang, X., Ng, S. K., Liu, C., Lin, Y., Zhou, Y., Kwong, T. N. Y., et al. (2023). Altered gut microbiota of obesity subjects promotes colorectal carcinogenesis in mice. EBioMedicine 93, 104670. doi:10.1016/j.ebiom.2023.104670
Kao, C. H., Huang, W. T., Lin, M. T., and Wu, W. S. (2011). Central interleukin-10 attenuated lipopolysaccharide-induced changes in core temperature and hypothalamic glutamate, hydroxyl radicals and prostaglandin-E(2). Eur. J. Pharmacol. 654, 187–193. doi:10.1016/j.ejphar.2010.12.038
Kesić, M., Baković, P., Farkaš, V., Bagarić, R., Kolarić, D., Štefulj, J., et al. (2023). Constitutive serotonin tone as a modulator of Brown adipose tissue thermogenesis: a rat study. Life (Basel) 13, 1436. doi:10.3390/life13071436
Krajewski-Hall, S. J., Miranda Dos Santos, F., McMullen, N. T., Blackmore, E. M., and Rance, N. E. (2019). Glutamatergic neurokinin 3 receptor neurons in the median preoptic nucleus modulate heat-defense pathways in female mice. Endocrinology 160, 803–816. doi:10.1210/en.2018-00934
Lee, C. T., Zhong, L., Mace, T. A., and Repasky, E. A. (2012). Elevation in body temperature to fever range enhances and prolongs subsequent responsiveness of macrophages to endotoxin challenge. PLoS One 7, e30077. doi:10.1371/journal.pone.0030077
Lee, K. H., Lee, C. T., Kim, Y. W., Han, S. K., Shim, Y. S., and Yoo, C. G. (2005). Heat shock protein 70 negatively regulates the heat-shock-induced suppression of the IkappaB/NF-kappaB cascade by facilitating IkappaB kinase renaturation and blocking its further denaturation. Exp. Cell Res. 307, 276–284. doi:10.1016/j.yexcr.2005.03.014
Lei, L., Wan, G., Geng, X., Sun, J., Zhang, Y., Wang, J., et al. (2023). The total iridoid glycoside extract of Lamiophlomis rotata Kudo induces M2 macrophage polarization to accelerate wound healing by RAS/p38 MAPK/NF-κB pathway. J. Ethnopharmacol. 307, 116193. doi:10.1016/j.jep.2023.116193
Li, M., Chen, S., Luo, K., Li, X., Wang, R., Yang, J., et al. (2024). Geniposide from Gardeniae Fructus exerts antipyretic effect in febrile rats through modulating the TLR4/NF-κB signaling pathway. J. Ethnopharmacol. 326, 117934. doi:10.1016/j.jep.2024.117934
Li, S., Li, X., Wang, K., Li, Y., Nagaoka, K., and Li, C. (2023). Gut microbiota intervention attenuates thermogenesis in broilers exposed to high temperature through modulation of the hypothalamic 5-HT pathway. J. Anim. Sci. Biotechnol. 14, 159. doi:10.1186/s40104-023-00950-0
Li, X., Wei, S., Ma, X., Li, H., Jing, M., Liu, H., et al. (2021). Huanglian jiedu decoction exerts antipyretic effect by inhibiting MAPK signaling pathway. Evid. Based Complement. Altern. Med. 2021, 2209574. doi:10.1155/2021/2209574
Li, Y. J., He, X. L., Zhang, J. Y., Liu, X. J., Liang, J. L., Zhou, Q., et al. (2023). 8-O-acetyl shanzhiside methylester protects against sleep deprivation-induced cognitive deficits and anxiety-like behaviors by regulating NLRP3 and Nrf2 pathways in mice. Metab. Brain Dis. 38, 641–655. doi:10.1007/s11011-022-01132-z
Loh, J. S., Mak, W. Q., Tan, L. K. S., Ng, C. X., Chan, H. H., Yeow, S. H., et al. (2024). Microbiota-gut-brain axis and its therapeutic applications in neurodegenerative diseases. Signal Transduct. Target Ther. 9, 37. doi:10.1038/s41392-024-01743-1
Ma, R. X., Hu, J. Q., Fu, W., Zhong, J., Cao, C., Wang, C. C., et al. (2023). Intermittent fasting protects against food allergy in a murine model via regulating gut microbiota. Front. Immunol. 14, 1167562. doi:10.3389/fimmu.2023.1167562
Ma, S. R., Yu, J. B., Fu, J., Pan, L. B., Yu, H., Han, P., et al. (2021). Determination and application of nineteen monoamines in the gut microbiota targeting phenylalanine, tryptophan, and glutamic acid metabolic pathways. Molecules 26, 1377. doi:10.3390/molecules26051377
Machado, N. L. S., Bandaru, S. S., Abbott, S. B. G., and Saper, C. B. (2020). EP3R-Expressing glutamatergic preoptic neurons mediate inflammatory fever. J. Neurosci. 40, 2573–2588. doi:10.1523/jneurosci.2887-19.2020
Mhanna, A., Martini, N., Hmaydoosh, G., Hamwi, G., Jarjanazi, M., Zaifah, G., et al. (2024). The correlation between gut microbiota and both neurotransmitters and mental disorders: a narrative review. Med. Baltim. 103, e37114. doi:10.1097/md.0000000000037114
Morrison, S. F. (2016). Central neural control of thermoregulation and brown adipose tissue. Auton. Neurosci. 196, 14–24. doi:10.1016/j.autneu.2016.02.010
Mota, C. M. D., Borges, G. S., Amorim, M. R., Carolino, R. O. G., Batalhão, M. E., Anselmo-Franci, J. A., et al. (2019). Central serotonin prevents hypotension and hypothermia and reduces plasma and spleen cytokine levels during systemic inflammation. Brain Behav. Immun. 80, 255–265. doi:10.1016/j.bbi.2019.03.017
Mota, C. M. D., Branco, L. G. S., Morrison, S. F., and Madden, C. J. (2020). Systemic serotonin inhibits brown adipose tissue sympathetic nerve activity via a GABA input to the dorsomedial hypothalamus, not via 5HT(1A) receptor activation in raphe pallidus. Acta Physiol. (Oxf) 228, e13401. doi:10.1111/apha.13401
Mota, C. M. D., and Madden, C. J. (2022). Neural circuits mediating circulating interleukin-1β-evoked fever in the absence of prostaglandin E2 production. Brain Behav. Immun. 103, 109–121. doi:10.1016/j.bbi.2022.04.008
Nakamura, Y., Yahiro, T., Fukushima, A., Kataoka, N., Hioki, H., and Nakamura, K. (2022). Prostaglandin EP3 receptor-expressing preoptic neurons bidirectionally control body temperature via tonic GABAergic signaling. Sci. Adv. 8, eadd5463. doi:10.1126/sciadv.add5463
Parker, B. J., Wearsch, P. A., Veloo, A. C. M., and Rodriguez-Palacios, A. (2020). The genus Alistipes: gut bacteria with emerging implications to inflammation, cancer, and mental health. Front. Immunol. 11, 906. doi:10.3389/fimmu.2020.00906
Ping, Y., Li, Y., Lü, S., Sun, Y., Zhang, W., Wu, J., et al. (2020). A study of nanometre aggregates formation mechanism and antipyretic effect in Bai-Hu-Tang, an ancient Chinese herbal decoction. Biomed. Pharmacother. 124, 109826. doi:10.1016/j.biopha.2020.109826
Radka, C. D., Frank, M. W., Rock, C. O., and Yao, J. (2020). Fatty acid activation and utilization by Alistipes finegoldii, a representative Bacteroidetes resident of the human gut microbiome. Mol. Microbiol. 113, 807–825. doi:10.1111/mmi.14445
Rodrigues, A. G., Soares, D. D., Marubayashi, U., and Coimbra, C. C. (2009). Heat loss during exercise is related to serotonin activity in the preoptic area. Neuroreport 20, 804–808. doi:10.1097/WNR.0b013e32832b8c90
Sakata, T., Kang, M., Kurokawa, M., and Yoshimatsu, H. (1995). Hypothalamic neuronal histamine modulates adaptive behavior and thermogenesis in response to endogenous pyrogen. Obes. Res. 3 (Suppl. 5), 707s–712s. doi:10.1002/j.1550-8528.1995.tb00489.x
Saleh, A., Parsa, S., Garza, M., Quigley, E. M. M., and Abraham, B. P. (2023). The role of fecal microbiota transplantation in the induction of remission in ulcerative colitis. Dig. Dis. 41, 656–665. doi:10.1159/000529591
Sanhueza, N., Fuentes, R., Aguilar, A., Carnicero, B., Vega, K., Muñoz, D., et al. (2021). Behavioural fever promotes an inflammatory reflex circuit in ectotherms. Int. J. Mol. Sci. 22, 8860. doi:10.3390/ijms22168860
Sengupta, T., Jaryal, A. K., Kumar, V. M., and Mallick, H. N. (2014). L-glutamate microinjection in the preoptic area increases brain and body temperature in freely moving rats. Neuroreport 25, 28–33. doi:10.1097/wnr.0000000000000035
Sherif, A. E., Alam, R., Asif, M., Khan, K.-u.-R., and Ur Rehman, M. S. (2024). Evaluation of the anti-inflammatory, antinociceptive, and antipyretic potential of ethanolic extract of Aristida depressa Retz through in vitro, in vivo, and in silico models. Front. Pharmacol. 15, 1326482. doi:10.3389/fphar.2024.1326482
Simm, B., Ott, D., Pollatzek, E., Murgott, J., Gerstberger, R., Rummel, C., et al. (2016). Effects of prostaglandin E2 on cells cultured from the rat organum vasculosum laminae terminalis and median preoptic nucleus. Neuroscience 313, 23–35. doi:10.1016/j.neuroscience.2015.11.034
Simpson, C. W., and Resch, G. E. (1986). Organization of central hyperthermic mechanisms in helium-cold hypothermic hamsters. Brain Res. Bull. 17, 117–121. doi:10.1016/0361-9230(86)90167-x
Song, B., He, J., Pan, X., Kong, L., Xiao, C., Keerqin, C., et al. (2024). Dietary Macleaya cordata extract supplementation improves the growth performance and gut health of broiler chickens with necrotic enteritis. J. Animal Sci. Biotechnol. 15, 374–395. doi:10.1186/s40104-023-00916-2
Sterkel, S., Akinyemi, A., Sanchez-Gonzalez, M. A., and Michel, G. (2017). Preserving brain function in a comatose patient with septic hyperpyrexia (41.6 °C): a case report. J. Med. Case Rep. 11, 40. doi:10.1186/s13256-017-1204-8
Sun, T., Luo, L., Tian, Q. Q., Wang, W. J., Liu, Q. Q., Yang, L., et al. (2020). Anxiolytic effects of 8-O-acetyl shanzhiside methylester on acute and chronic anxiety via inflammatory response inhibition and excitatory/inhibitory transmission imbalance. Neurotox. Res. 38, 979–991. doi:10.1007/s12640-020-00203-2
Tabarean, I. V. (2016). Histamine receptor signaling in energy homeostasis. Neuropharmacology 106, 13–19. doi:10.1016/j.neuropharm.2015.04.011
Takahashi, Y., Zhang, W., Sameshima, K., Kuroki, C., Matsumoto, A., Sunanaga, J., et al. (2013). Orexin neurons are indispensable for prostaglandin E2-induced fever and defence against environmental cooling in mice. J. Physiol. 591, 5623–5643. doi:10.1113/jphysiol.2013.261271
Vogt, N. M., Kerby, R. L., Dill-McFarland, K. A., Harding, S. J., Merluzzi, A. P., Johnson, S. C., et al. (2017). Gut microbiome alterations in Alzheimer's disease. Sci. Rep. 7, 13537. doi:10.1038/s41598-017-13601-y
Voronova, I. P. (2021). 5-HT receptors and temperature homeostasis. Biomolecules 11, 1914. doi:10.3390/biom11121914
Wang, C. S., Li, W. B., Wang, H. Y., Ma, Y. M., Zhao, X. H., Yang, H., et al. (2018). VSL#3 can prevent ulcerative colitis-associated carcinogenesis in mice. World J. Gastroenterol. 24, 4254–4262. doi:10.3748/wjg.v24.i37.4254
Wang, J., Liu, Y., Zong, B., Zhao, S., Li, Y., Zhang, Z., et al. (2024). Qingxuan Runmu Yin alleviates dry eye disease via inhibition of the HMOX1/HIF-1 pathway affecting ferroptosis. Front. Pharmacol. 15, 1391946. doi:10.3389/fphar.2024.1391946
Wang, L. L., Wang, L., and Cao, Y. (2008). The effects of hypothalamic TRPV4 on body temperature, cAMP content, and [Ca∼(2+)] i concentration during LPS induced fever in rats. Chin. Pharmacol. Bull. 24, 1174–1177.
Wang, Q. J., Guo, Y., Zhang, K. H., Zhang, L., Geng, S. X., Shan, C. H., et al. (2021). Night-restricted feeding improves gut health by synchronizing microbe-driven serotonin rhythm and eating activity-driven body temperature oscillations in growing rabbits. Front. Cell Infect. Microbiol. 11, 771088. doi:10.3389/fcimb.2021.771088
Wrotek, S., Sobocińska, J., Kozłowski, H. M., Pawlikowska, M., Jędrzejewski, T., and Dzialuk, A. (2020). New insights into the role of glutathione in the mechanism of fever. Int. J. Mol. Sci. 21, 1393. doi:10.3390/ijms21041393
Wu, Y., Li, Q., Liu, J., Liu, Y., Xu, Y., Zhang, R., et al. (2021). Integrating serum metabolome and gut microbiome to evaluate the benefits of lauric acid on lipopolysaccharide- challenged broilers. Front. Immunol. 12, 759323. doi:10.3389/fimmu.2021.759323
Xie, Y., Pei, F., Liu, Y., Liu, Z., Chen, X., and Xue, D. (2023). Fecal fermentation and high-fat diet-induced obesity mouse model confirmed exopolysaccharide from Weissella cibaria PFY06 can ameliorate obesity by regulating the gut microbiota. Carbohydr. Polym. 318, 121122. doi:10.1016/j.carbpol.2023.121122
Xu, Z., Jiang, W., Huang, W., Lin, Y., Chan, F. K. L., and Ng, S. C. (2022). Gut microbiota in patients with obesity and metabolic disorders - a systematic review. Genes Nutr. 17, 2. doi:10.1186/s12263-021-00703-6
Yang, Z., and Li, J. (2018). Influence of temperature on cerebellar metabolite levels. Int. J. Hyperth. 34, 273–275. doi:10.1080/02656736.2017.1333632
Ye, L., Tao, Y., Wang, Y., Feng, T., and Li, H. (2015). The effects of baicalin on the TLR2/4 signaling pathway in the peripheral blood mononuclear cells of a lipopolysaccharide-induced rat fever model. Int. Immunopharmacol. 25, 106–111. doi:10.1016/j.intimp.2014.12.028
Yin, L., Huang, G., Khan, I., Su, L., Xia, W., Law, B. Y. K., et al. (2022). Poria cocos polysaccharides exert prebiotic function to attenuate the adverse effects and improve the therapeutic outcome of 5-FU in Apc(Min/+) mice. Chin. Med. 17, 116. doi:10.1186/s13020-022-00667-8
Zhang, L., Kan, Z. C., Zhang, X. L., Fang, H., and Jiang, W. L. (2014). 8-O-acetyl shanzhiside methylester attenuates cerebral ischaemia/reperfusion injury through an anti-inflammatory mechanism in diabetic rats. Basic Clin. Pharmacol. Toxicol. 115, 481–487. doi:10.1111/bcpt.12266
Zhang, Q., Zhang, S., Wu, S., Madsen, M. H., and Shi, S. (2022). Supplementing the early diet of broilers with soy protein concentrate can improve intestinal development and enhance short-chain fatty acid-producing microbes and short-chain fatty acids, especially butyric acid. J. Anim. Sci. Biotechnol. 13, 97. doi:10.1186/s40104-022-00749-5
Zhang, W., Bai, Y., Qiao, Y., Wang, J., Li, M. Y., Wang, J. W., et al. (2018). 8-O-Acetyl shanzhiside methylester from lamiophlomis rotata reduces neuropathic pain by inhibiting the ERK/TNF-α pathway in spinal astrocytes. Front. Cell Neurosci. 12, 54. doi:10.3389/fncel.2018.00054
Zhang, W., Zou, G., Li, B., Du, X., Sun, Z., Sun, Y., et al. (2020). Fecal microbiota transplantation (FMT) alleviates experimental colitis in mice by gut microbiota regulation. J. Microbiol. Biotechnol. 30, 1132–1141. doi:10.4014/jmb.2002.02044
Zhang, X., Wang, Y., Li, S., Dai, Y., Li, X., Wang, Q., et al. (2019). The potential antipyretic mechanism of gardeniae fructus and its heat-processed products with plasma metabolomics using rats with yeast-induced fever. Front. Pharmacol. 10, 491. doi:10.3389/fphar.2019.00491
Zhang, Y. Q., Liu, Z. F., Yu, T. Y., Wang, H. R., Jiao, Y., Liu, D., et al. (2022). Effects of Tuina antipyretic six methods on the COX-2/PGE2/EP3 signaling pathway in the hypothalamus of lipopolysaccharide-induced fever infant rabbits. J. Beijing Univ. Tradit. Chin. Med. 45, 1160–1167. doi:10.3969/j.issn.1006-2157.2022.11.009
Zhao, R., Coker, O. O., Wu, J., Zhou, Y., Zhao, L., Nakatsu, G., et al. (2020). Aspirin reduces colorectal tumor development in mice and gut microbes reduce its bioavailability and chemopreventive effects. Gastroenterology 159, 969–983.e4. doi:10.1053/j.gastro.2020.05.004
Keywords: 8-O-Acetyl Shanzhiside methyl ester, yeast-induced pyrexia in rats, intestinal flora, metabolomics, neurotransmitter
Citation: Li X, Liu T, Liang K, Wang R, Yang J, Chen Y, Wang R and Li M (2025) Elucidation of the antipyretic and anti-inflammatory effect of 8-O-Acetyl Shanzhiside methyl ester based on intestinal flora and metabolomics analysis. Front. Pharmacol. 16:1482323. doi: 10.3389/fphar.2025.1482323
Received: 18 August 2024; Accepted: 14 April 2025;
Published: 28 April 2025.
Edited by:
Alessandro Venditti, Sapienza University of Rome, ItalyReviewed by:
Samir Ayoub, University of East London, United KingdomClaudio Frezza, Università Link Campus, Italy
Copyright © 2025 Li, Liu, Liang, Wang, Yang, Chen, Wang and Li. This is an open-access article distributed under the terms of the Creative Commons Attribution License (CC BY). The use, distribution or reproduction in other forums is permitted, provided the original author(s) and the copyright owner(s) are credited and that the original publication in this journal is cited, in accordance with accepted academic practice. No use, distribution or reproduction is permitted which does not comply with these terms.
*Correspondence: Rong Wang, d2FuZ3JvbmctNjlAMTYzLmNvbQ==; Maoxing Li, bGltYW94MjAyMEBhbGl5dW4uY29t
†These authors share first authorship