- 1The First Affiliated Hospital of Zhejiang Chinese Medical University, Hangzhou, China
- 2School of Basic Medical Sciences, Zhejiang Chinese Medical University, Hangzhou, China
- 3The Second Affiliated Hospital of Zhejiang Chinese Medical University, Hangzhou, China
- 4Key Laboratory of Chinese Medicine Rheumatology of Zhejiang Province, Research Institute of Chinese Medical Clinical Foundation and Immunology, School of Basic Medical Sciences, Zhejiang Chinese Medical University, Hangzhou, China
- 5Research Institute of Chinese Medical Clinical Foundation and Immunology, Hangzhou, China
Ethnopharmacological relevance: Lupus nephritis (LN) is an immune complex glomerulonephritis commonly associated with systemic lupus erythematosus. Traditional Chinese medicine (TCM) has emerged as a promising adjuvant therapy for LN, due to its low toxicity and diverse therapeutic effects for long-term management.
Materials and methods: A comprehensive search of PubMed and Web of Science was conducted up to 7 June 2024, using keywords related to lupus nephritis, traditional Chinese medicine, immune cells, and kidney resident cells. Study quality were assessed based on Good Automated Manufacturing Practice guidelines, with evaluations jointly conducted by two authors.
Results: This review includes 31 research papers and summarizes seven herbal formulas and 18 phytochemicals that modulate the autoimmune pathogenesis of LN. Their mechanisms involve regulating immune cells activation, differentiation, apoptosis, as well as influencing resident kidney cells to support renal protection and immune homeostasis. Since TCM exhibit bidirectional regulation, they activate regulatory immune cells while suppress pathogenic factors. Inconsistent or inconclusive findings are discussed.
Conclusion: This review summarizes current research on herbal formulas and phytochemicals in immune cells and kidney resident cells in LN, highlighting the potential and significance of TCM treatment. It also addresses the limitations of existing studies and suggests that future research should focus on exploring the immunosuppressive and kidney-protective effects of herbal formulas and phytochemicals, as well as enhancing the clinical translation and standardization of TCM.
1 Introduction
Lupus nephritis (LN) is a type of glomerulonephritis that represents one of the most severe organ manifestations, affecting approximately 50% of the systemic lupus erythematosus (SLE) cases and posing a significant risk for morbidity and mortality (Anders et al., 2020; Parikh et al., 2020). The pathophysiology of LN is heterogeneous, including the deposition of autoantibodies and immune complexes (ICs), the activation and/or proliferation of infiltrating immune cells and kidney resident cells, and the presence of various pathogenic molecules at the site of injury (Davidson, 2016; Lech and Anders, 2013; Mohan et al., 2023).
Conventional treatments encompass steroid therapy, cyclophosphamide (CYC), and mycophenolate mofetil (MMF), while alternative therapies, including anti-BLYS agents, novel calcineurin inhibitors, CD20 blockade, and anti-interferon agents have also been explored (Askanase et al., 2023; Desai et al., 2024). These advancements have enhanced life expectancy and quality, but there has not been significant progress in improving renal failure and mortality rates (Nossent et al., 2024; Parikh et al., 2020). Besides, evidences proved that these drugs can induce cytotoxicity through immunosuppressive and pharmacologic effects, resulting in side effects such as amenorrhea/ovarian failure, cytopenia, and serious infections (Singh et al., 2016a; Singh et al., 2016b). This highlights the urgent need for multi-target therapy and the development of better long-term treatment options or strategies for LN.
The quest for novel therapies, as well as the fact that LN being more common and severe in East Asians than in African-Americans (Petri et al., 2023), have spurred the exploration of TCM. TCMs exhibit diverse and integrated pharmacological effects that help mitigate the progression of LN. Moreover, their ability of reducing adverse side effects makes them a valuable option of long-term adjuvant therapy (Dou et al., 2023). Evidence indicates that combining herbal formulas and phytochemicals such as Shenqi Dihuang decoction, Liuwei Dihuang pill, and Astragalus injection with conventional treatments like cyclophosphamide (CTX) and glucocorticoids (GC) enhances clinical efficacy and reduces SLE disease activity scores more effectively than CTX and GC alone. Moreover, this combined treatment approach has been associated with a lower risk of adverse reactions such as infection, gastrointestinal discomfort, and insomnia (Li et al., 2024), as well as a slightly higher likelihood of reducing or discontinuing glucocorticoid dosage (Li et al., 2014). Additionally, botanical drugs like Cordyceps and artemisinin may help prevent the recurrence of LN and protect kidney function (Lu, 2002).
This review uniquely integrates immune metabolic regulation with the therapeutic potential of TCM in LN, providing a comprehensive analysis of recent advances in preclinical studies. By systematically summarizing the molecular mechanisms and therapeutic implications, this review offers new insights into TCM’s role in LN treatment and identifies key directions for future research. All the herbal formulas and phytochemicals discussed in this review are within the domain of traditional Chinese medicine.
2 Methods
In this review, experimental research (in vitro and in vivo studies) published or available for early access up to 7 June 2024, were retrieved from databases such as PubMed and Web of Science. The search terms included lupus nephritis, traditional Chinese medicine (e.g., herbal prescriptions, Chinese herbal drugs, plant extracts, herbal extracts), immune cells (e.g., T lymphocytes, B lymphocytes), and kidney-resident cells (e.g., Podocytes, Mesangial cells).
The inclusion criteria were as follows: (1) studies related to the specified keywords, either individually or in combination, focusing on the therapeutic effects or mechanisms of TCM in LN; (2) studies providing experimental data from in vitro or in vivo research with detailed methodologies; and (3) full-text articles published in English.
The exclusion criteria were: (1) duplicate search results; (2) studies deemed irrelevant based on title and abstract screening; (3) records lacking mechanistic insights or providing insufficient information; and (4) purely theoretical or review articles.
Based on these criteria, a total of 31 studies investigating the mechanisms of Traditional Chinese Medicine in the treatment of LN were included in this review. The quality and methodological rigor of these studies were assessed using the criteria outlined by the Good Automated Manufacturing Practice (GAMP) best practice guidelines (https://ga-online.org/best-practice/). The evaluation was jointly conducted by authors HZY and LXL. The detailed evaluation results are provided in the supplementary materials.
3 Cellular pathogenesis of lupus nephritis
3.1 T cells
3.1.1 T cells activation
T cells constitute the majority of kidney-infiltrating immune cells in patients with LN and in lupus-prone mice, and are implicated in the development of progressive kidney failure (Linke et al., 2022; Mohan et al., 2023). T cells can either cause direct cytotoxicity or recruit other inflammatory cells, such as monocytes/macrophages, playing a crucial role in the pathogenesis of experimental and human LN. Studies have revealed that Triptolide, a diterpenoid triepoxide derived from the botanical drug Tripterygium wilfordii Hook. f., inhibits lymphocyte activation and T-cell expression of interleukin-2 at the transcriptional level, positioning it as one of the few immunosuppressants acting at the early stage of T-cell activation signaling (Qiu and Kao, 2003). This characteristic classifies triptolide as operating within a category of immunosuppressants similar to CYC and tacrolimus (Qiu et al., 1999). Furthermore, unlike CYC and tacrolimus, triptolide inhibits both Ca2+-dependent and Ca2+-independent pathways, thereby affecting T-cell activation through CD28 co-stimulation, indicating that triptolide has broader immunosuppressive effects in the treatment of conditions involving CYC-resistant T-cell activation (Gu et al., 2016; Song et al., 2023). Although there is a lack of studies on other botanical drugs, this example suffices to illustrate the broad potential of TCM in the treatment of LN, particularly as a supplement to targeted therapy.
3.1.2 T cells differentiation
In LN, dysregulated CD4+ T cell subsets include Th1, Th17, Tfh, and Treg cells. Th1 and Treg cells are decreased, while Th2, Th17, Tfh17, and Tfh cells are increased. A strong correlation between Th17 and Treg cells with renal involvement was observed (Yuan et al., 2022). These immunological mechanisms are central to TCM-related research and will be discussed further.
3.1.2.1 CD4+ Th1 T cells
Numerous clinical and experimental findings have demonstrated a pathogenic role of imbalance towards Th1 cell-mediated immune responses in LN (Chan et al., 2006; Fakhfakh et al., 2022; Linke et al., 2022), which is partly attributed to high glomerular expression of interleukin-12 (IL-12) and interleukin-18 (IL-18) (Liu et al., 2012; Tucci et al., 2010). However, TCM has demonstrated the ability to alter this cellular population imbalance. Hachimi-jio-gan (Ba-Wei-Di-Huang-Wan, HMG) modulated an imbalance toward Th1 predominance in MRL/lpr mice by inhibiting IL-12 production and ameliorating autoimmune disorders (Furuya et al., 2001). Additionally, the Th1 axis was suppressed and the Th2 axis became predominant in Sairei-to-treated MRL/lpr mice, possibly due to an increase in IL-4-producing cells and suppression of IFN-γ expression (Ito et al., 2002). Furthermore, Antroquinonol (Tsai et al., 2012) and DCB-SLE1 (Tsai et al., 2011) have been shown, in mouse models, to reduce renal production of IL-18, thereby facilitating the differential regulation of T cells in treatment. They also exhibit the ability to inhibit local renal inflammation by suppressing NF-κB activation.
3.1.2.2 CD4+ Th17 T cells
The level of Th17 cells was found to be higher in LN patients (Fakhfakh et al., 2022). Several reports indicate that interleukin-17 (IL-17) and Th17 cells play important roles in the pathogenesis of LN, with PP2A, ROCK, CREM, and CaMK4 pathways shown to facilitate IL-17 production in SLE (Koga et al., 2017). Celastrol inhibited phospho-STAT3 expression in cultured Th17 cells and upregulated phospho-STAT5 expression in induced regulatory T (iTreg) cells, thereby suppressing Th17 cell induction and promoting iTreg cell generation. It also reduced IL-17 expression in Th17 cells compared with untreated cells (Astry et al., 2015; Zhang et al., 2018). Furthermore, studies have shown that menthone could serve as a potential antirheumatic phytochemical, as its effect on regulating the number of Th1 and Th17 cells and inhibiting the release of pro-inflammatory cytokines, including TNF-α, IL-1β, and IL-6, has been confirmed (Chen X. et al., 2022). DCB-SLE1 has been shown to suppress IL-6 and IL-17 production in an accelerated severe LN model (Tsai et al., 2011), thereby regulating the number of Th17 cells. Administration of lipopolysaccharide resulted in a mixed Th1, Th2, and Th17 response in normal mice, and this effect was inhibited by in vivo Tetrandrine (Zou et al., 2019).
Interestingly, Jakiela et al. claimed that Th17 expansion was not related to LN activity, renal histology, or blood and urine inflammatory biomarkers, but was associated with a higher cumulative dose of CYC (Jakiela et al., 2018). This suggests that the use of TCM may help reduce the occurrence of side effects from chronic immunosuppressive therapy and improve treatment efficiency.
3.1.2.3 CD4+ Treg cells
Except for the germinal center-Tfr/Tfh imbalance mentioned above, it has been more commonly observed in the pathogenesis of LN that there are fewer Treg cells in LN patients’ peripheral blood than healthy individuals’, with a considerable increase in Th17 cell-to-Treg cell ratios (Li et al., 2022b).
Several have been shown to influence Treg cells in LN. Astragaloside IV (AST IV) has the ability to markedly increase the Foxp3 expression as well as IL-10 and TGF-β secretion levels in Treg in a dose-dependent manner (Li et al., 2016). Antroquinonol can enhance Treg cell suppression in accelerated severe LN (Tsai et al., 2012). Baicalin induces Foxp3 protein expression in cultured T cells, promotes Treg cell differentiation and regulatory activity. It also restores Foxp3 expression following its initial IL-6-mediated inhibition (Yang et al., 2012).
Apart from LN, the abnormal Treg/Th17 ratio is involved in the pathogenesis of many immune-mediated inflammatory diseases. Xu, etc., reviewed that there were nine active ingredients (including Oxymatrine, Baicalin, Triptolide, Paeoniflorin, Sinomenine, Celastrol, Emodin, Diosgenin and Chlorogenic acid) originating from TCM reported to have an immunological regulatory effect on the Th17/Treg axis in IMID treatment (Xu et al., 2020). The mechanism of action of these botanical drugs need to be further explored. The immune-modulating mechanisms on Th17/Treg axis by TCM might provide a broader insight into the treatment of IMID.
3.1.2.4 CD4+ Tfh cells
Follicular helper CD4 T (Tfh) cells play a significant role in germinal center formation, B-cell development, affinity maturation, and immunoglobulin class switching (Crotty, 2011; Fazilleau et al., 2009; Zhu et al., 2016). IL-21, a critical cytokine produced by Tfh cells, potently stimulates the differentiation of B cells (King et al., 2008). Tfh cells have been found to increase in LN patients and are associated with B cell activation as well as in situ inflammation (Arazi et al., 2019; Liarski et al., 2014; Yuan et al., 2022).
Follicular regulatory T (Tfr) cells, also located in the germinal center and sharing phenotypic characteristics with Tfh cells and Treg cells, inhibit Tfh cells mediated B cell responses (Xu et al., 2017; Zhu et al., 2016). Tfr cells are reported to be reduced in patients with SLE compared with healthy individuals, but this reduction is corrected after standard-of-care treatment (Liu et al., 2018; Xu et al., 2017). Additionally, a progressive reduction in Tfr cells and decreased Tfr/Tfh ratio despite increased Tregs in the renal lymph nodes of NZBWF1/j mice were repored (Kumar et al., 2022).
Artesunate (ART) has shown therapeutic effects by reducing the number of Tfh cells and maintenance of the ratio of Tfr to Tfh in the spleen of MRL/lpr mice (Dang et al., 2019). Baicalin has the ability of inhibiting Tfh cell differentiation and IL-21 production by mTOR activation inhibition, and it can promote Foxp3+ regulatory T cell differentiation, including Tfr cells (Yang et al., 2019). Although studies of the balance of Tfr and Tfh cells have been sparse, TCM has shown great therapeutic potential in this regard. (Figure 1 illustrates the differentiation and interaction of the T cell family and the effect of TCM on these processes.).
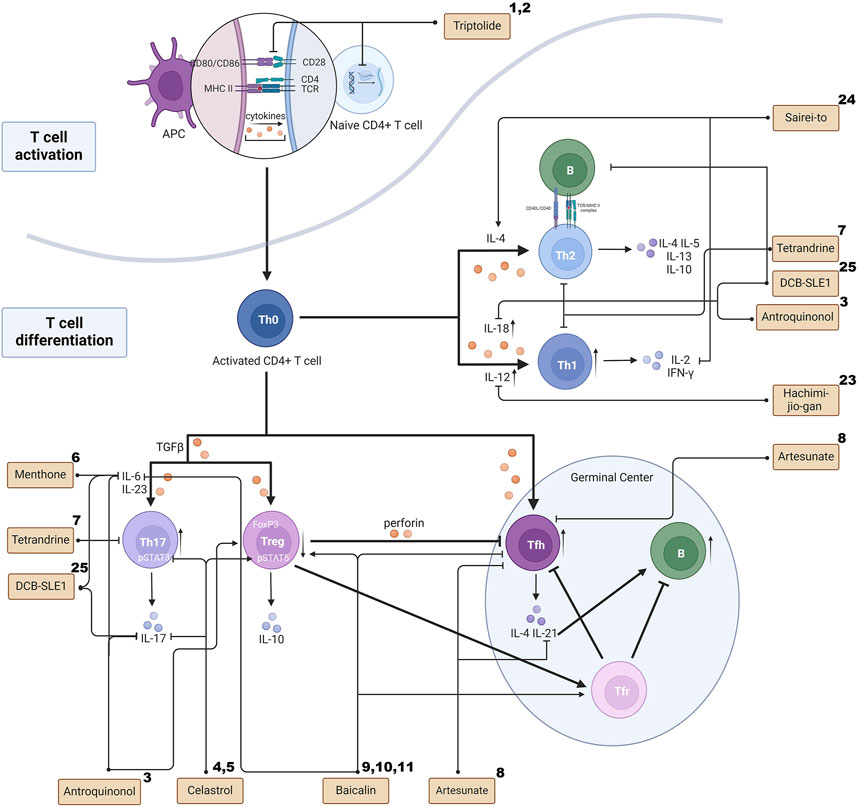
Figure 1. The effects of TCM on T cell activation, differentiation and interaction. The superscript numbers of the herbal formulas/phytochemicals correspond to the reference numbers in Tables 1, 2. Created with BioRender.com.
3.2 B cells
3.2.1 B cells and plasma cells
SLE is characterized by the loss of B-cell tolerance and presence of autoantibodies. Anti-dsDNA antibodies cross-react with several renal cell types and are thought to be central to the nephritis process (Tsokos et al., 2016). Researches has focused on the ability of TCM to reduce of autoreactive B cells. Tripterygium wilfordii Hook F (TWHF) regulates the functions of 24 differentially expressed genes (including HMOX1, ALB, and CASP1) through hydrogen bonding, mainly concentrated in the B-cell signaling pathway (Cai et al., 2023). Sairei-to downregulates the proportion of CD19 and the serum levels of IgG1 in MRL/lpr mice, thereby suppressing B cell function (Ito et al., 2002).
Additionally, the upstream regulation of B cells and the role of B cell subsets in the pathogenesis of LN have been further studied. B-cell activating factor (BAFF) and a proliferation-inducing ligand (APRIL) are the most important factors involved in the maturation and activation of B cells (Samy et al., 2017). A study shows that Langchuangping Granule (LG) could attenuate the renal injury via suppressing sBAFF level and BAFF mRNA expressions (Li et al., 2012). Jieduquyuzishen prescription (JP) can downregulate the BAFF/BAFF-R signaling pathway as effectively as GC and suppress proliferation and survival of lymphocytes activated by mBAFF(Wu et al., 2015). BAFF and APRIL are ideal targets for LN treatment, and the value of TCM in this area requires further exploration.
B cell subsets that play a role in the pathogenesis of LN include age-associated B cells (ABCs) and kidney-infiltrated plasma cells (Espeli et al., 2011; Mohan et al., 2023; Rubtsova et al., 2017). Peripheral blood ABCs are increased compared with healthy controls in patients with SLE and strongly correlate with anti-chromatin antibody levels and track with disease activity (Ramsköld et al., 2019; Wang et al., 2018). Infiltrated plasma cells are often present in the renal medulla in LN patients, especially proliferative and membranous types. These cells had the phenotypic characteristics of fully differentiated plasma cells and, similar to long-lived bone marrow plasma cells, they are not in cell cycle (Espeli et al., 2011). However, there is a lack of research on the relationship between TCM and these B cell subsets. More detailed and in-depth researches are needed.
3.2.2 Bregs
Evidences has shown that the percentages of Bregs and their secretion of IL-35 and IL-10 are significantly decreased in LN patients (Heinemann et al., 2016; Watanabe et al., 2010; Xiong et al., 2022). A decrease has been observed in CD72, a regulatory receptor on B cells, while the addition of soluble semaphorin 3A can improve its expression (Vadasz et al., 2014). Although there are no direct studies on the effects of TCM on Breg cells in LN, related research has demonstrated the role of TCM in this context. Depigmented-polymerized phleum pratense (DPG-POL-Phl p) was prominent at inducing IL-10+CD19+CD5hi and IL-10+CD19+CD5hiCD38intCD24int Breg cell subsets (Layhadi et al., 2023). Additionally, administration of Yupinfeng San, a traditional Chinese medical formula, can induce propionic acid production by intestinal bacteria to stabilize IL-10 expression in Breg cells (Zhou et al., 2021) or restore the immune suppressor function of Bregs by inhibiting the expression of Bcl2L12 (Zhou et al., 2019). S. baicalensis extract and its metabolites, baicalin and baicalein, can induce semaphorin 3A expression (Yoshioka et al., 2021), thereby potentially restoring B cells’ regulatory functions by upregulating IL-10 expression (Eiza et al., 2023).
Although IL-10 is known as a potent anti-inflammatory cytokine (Mollazadeh et al., 2019; Saraiva and O’Garra, 2010) and has been emphasized in Breg cell-related therapy, excessive IL-10 levels may lead to immune disorders and increase the risk of autoimmune diseases by disrupting the immune balance and regulating the activation of immune cells. For example, research has showen that IL-10 can increase CD8+ T cell infiltration in tissue, induce IFN-γ production, and favor effective T cell memory responses (Saraiva et al., 2020). Recent evidence indicates that IL-10 plays dual roles in SLE: it may inhibit pro-inflammatory effector functions but also seems to be a main driver of the extrafollicular antibody response, promoting direct differentiation of activated B cells into plasma cells (Biswas et al., 2022). Despite the emphasis on promoting Breg cells and IL-10, there are also studies on inhibiting IL-10 using TCM. For example, Wogonin suppresses IL-10 production in B cells via inhibition of the STAT3 and ERK signaling pathways and reduce mRNA and protein levels of the transcription factor Hif-1α(Fan et al., 2020).
The aforementioned studies have indicated that TCM may play a role in inducing Bregs and their regulatory factors in LN treatment. On the other hand, due to the pleiotropic effects of cytokines, herbal formulas particularly, offers a more comprehensive regulatory effect owing to its ambiguous targeting, which may reduce side effects and promote positive regulation. Therefore, the therapeutic role of TCM warrants more attention and further exploration. (Figure 2 illustrates the differentiation and interaction of the B cell family and the effect of TCM on these processes.).
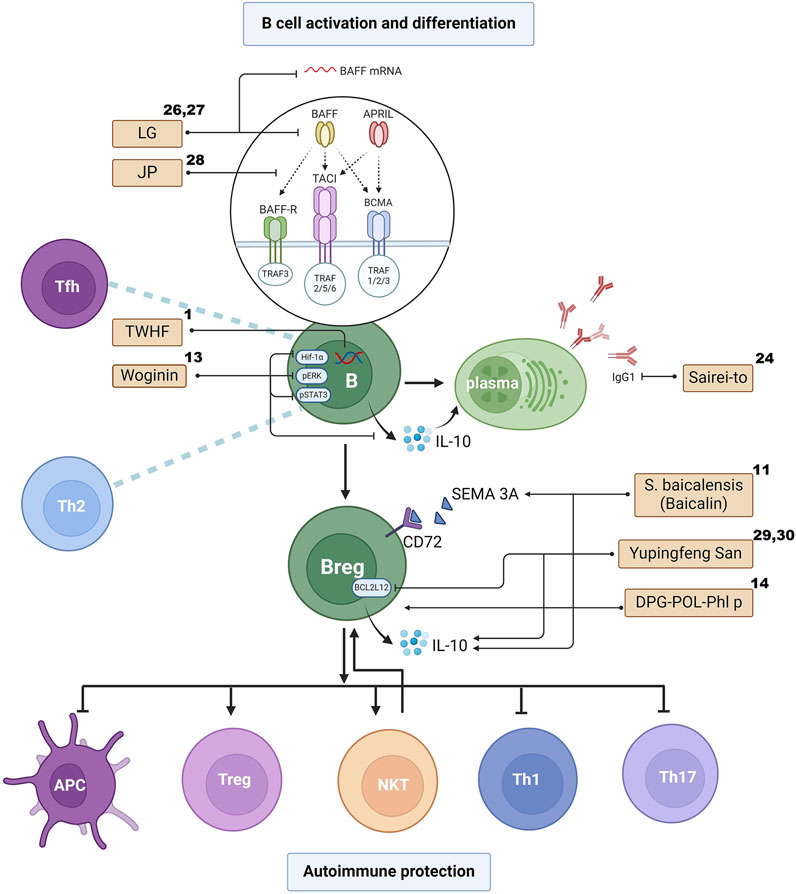
Figure 2. The effects of TCM on B cell activation, differentiation and interaction. The superscript numbers of the herbal formulas/phytochemicals correspond to the reference numbers in Tables 1, 2. Created with BioRender.com.
3.3 NK cells and NKT cells
In SLE patients, circulating levels of Natural Killer (NK) cells were diminished and their cytotoxicities were impaired (Park et al., 2009). Active nephritis in SLE is substantially associated with ILT2+ NKT cell and Ki67+ NK cell frequencies (Chen et al., 2023; Hudspeth et al., 2019). Additionally, an elevated ratio of CD56brightCD16− to CD57+CD56dimCD16+ NK cell distinguishes renal involvement in SLE (Li et al., 2023). The defective functions of NK cells may be due to changes in apoptosis-related protein expressions, such as reduced expression of TRAIL, Bcl-2, and TNFR1(Liphaus et al., 2024). The decline in NK cells may be related to increased consumption due to autoimmunity, as suggested by the fact that the high expression of MCP-1, a chemokine that induces NK cell migration and activation, thereby facilitating kidney disease-related inflammation in LN (Liu et al., 2023).
Although NK cells are one of the most important lymphocytes, their pathogenic role in LN has not received much attention, and the reason for their reduced quantity and weakened pathogenicity in LN have not been clearly studied. Herbal formulas like DCB-SLE1 have been found to suppress NK cell activity (Tsai et al., 2011). However, studies on the effects of TCM on NK cells in the context of treatment are still lacking.
3.4 Neutrophils
Neutrophils accumulate in the kidneys of patients with proliferative LN, and their products and ability to induce other immune cells may contribute to pathogenesis of the disease (Nishi and Mayadas, 2019). The herbal formulas and phytochemicals, such as the DCB-SLE1, Antroquinonol, and Citral, have been shown to reduce neutrophil infiltration in the kidney (Tsai et al., 2011; Tsai et al., 2012; Ka et al., 2015). However, some also provide protective effect on neutrophils. For example, JP can reduce the apoptosis and increase the survival of polymorphonuclear neutrophils (Wu et al., 2015).
Neutrophil extracellular traps (NETs) are fibrous networks that protrude from the membranes of activated neutrophils. Excess NETs damage normal tissues and induce inflammation and immune injury to kidneys (Lee et al., 2017). NETs are elevated in the circulation of patients with SLE, particularly in those with LN, and their abundance correlates with circulating levels of anti-double-stranded DNA (dsDNA), C3 and C4, and proteinuria (Hakkim et al., 2010; van der Linden et al., 2018). Hedyotis diffusa Willd (HDW) treatment has been proven to ameliorate the expression of STAT3, IL-17, Ly6G, and MPO in the kidney and neutrophil Extracellular Trap formation (NETosis), thereby relieving LN (Li et al., 2022a). However, the view that reduced degradation of NETs contributes to LN progression is challenged by experimental data in lupus-prone mice that genetically fail to produce NETs but still suffer from the disease (Kienhöfer et al., 2017; Nishi and Mayadas, 2019). Overall, the role of neutrophils in the pathogenesis of LN and the application of TCM in this field require further study. (Figure 3 illustrates the infiltration, apoptosis and NETosis of the neutrophil family, as well as the effect of TCM on these processes.).
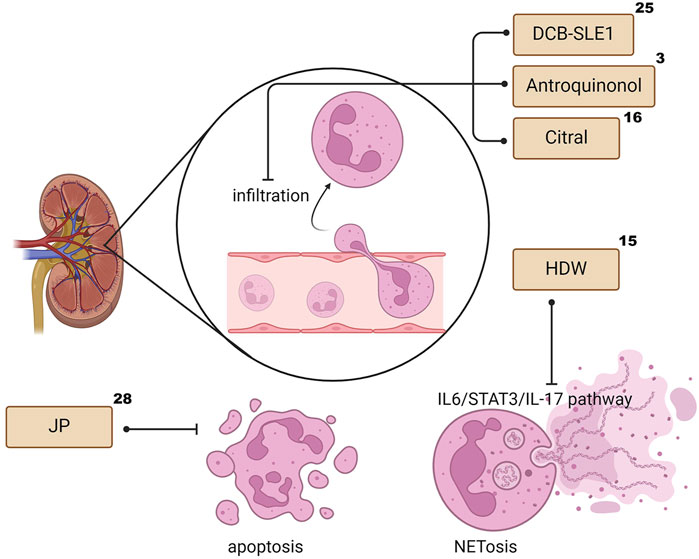
Figure 3. The effects of TCM on Neutrophil infiltration, apoptosis and NETosis. The superscript numbers of the herbal formulas/phytochemicals correspond to the reference numbers in Tables 1, 2. Created with BioRender.com.
3.5 Monocytes and macrophages
Pathogenic macrophages in LN are classified according to source and function. Along with infiltrating monocyte-derived (MoMac) macrophages, the kidneys also have a network of tissue-resident macrophages (TrMac) located around the glomeruli and tubulointerstitium (Mohan et al., 2023). TrMacs orchestrate leukocyte recruitment and are major responders to ICs, while MoMacs take up and present IC antigen (Richoz et al., 2022). Additionally, subpopulations called classically activated macrophages (M1) and alternative activated macrophages (M2) are often discussed in the context of LN mechanism (Chávez-Galán et al., 2015). While it is commonly agreed that kidney macrophages in MRL/lpr mice with spontaneous LN are skewed towards the M1 phenotype rather than the M2 phenotype (Iwata et al., 2012), research has confirmed that M2-phenotype macrophages (CD163+) are the dominant subpopulation in human LN. A significant association has been observed among CD163+ macrophages, crescents, complement activation, kidney fibrosis and progression to kidney failure (Olmes et al., 2016; Sciascia et al., 2022; Tao et al., 2021).
Macrophage depletion ameliorates nephritis induced by antibodies against the glomerular basement membrane. Demethylzeylasteral (T-96) exhibits reno-protective effects in LN by inhibiting the activation of NF-κB, reducing downstream pro-inflammatory mediators such as TNF-α, COX-2 and ICAM-1, and thus restricting macrophage infiltration (Hu et al., 2015). Similarly, LG downregulates monocyte chemoattractant protein-1 (MCP-1) in BXSB LN mice though the NF-κB signaling pathway (Li et al., 2011). Production of MCP-1 by spleen cells in (NZB x NZW) F1 mice also decreased after triptolide or tripdiolide therapy (Tao et al., 2008). Additionally, Citral alleviates the mouse ASLN model by reducing ATP-induced IL-1β secretion and caspase-1 activation in LPS-primed macrophages (Ka et al., 2015). In the studies related to the treatment of monocyte-macrophages with TCM in LN, many showed that TCM can effectively inhibit the autoimmune response of macrophages, but none have distinguished between macrophage types. As mentioned above, the anti-inflammatory or pro-inflammatory effects and the states in the autoimmune response of different types of macrophages in the development of LN are not consistent, so the therapeutic targets of drugs should be further clarified.
3.6 Dendritic cells
In LN, dendritic cells (DC) infiltrate the kidneys, function to present antigens, and organize tertiary lymphoid structures that amplify inflammation (Kurts et al., 2020; Maria and Davidson, 2017), thus playing a critical role in the evolution of LN. when treated with M1, an active metabolite of ginsenoside, the LPS-primed bone marrow-derived dendritic cells (BMDCs) significantly reduced the expression levels of NLRP3, decreased the secretion of pro-IL-1β and p-IκB, and inhibited CD4+ T cell proliferation (Lin et al., 2019). Fisetin at non-toxic concentrations suppressed the expression of costimulatory molecules CD80 and CD86, the production of cytokines IL-12, IL-6, and TNF-α, and the endocytic activity of DC during LPS-induced DC maturation (Liu et al., 2010). Bidens pilosa L. petroleum ether extract induced a semi-mature status in DCs, regardless of exposure to a maturation stimulus (Rodríguez Mesa et al., 2023). Additionally, phytochemicals such as Coumarins, Triptolide, Flavonoids from Astragalus membranaceus (Fisch.) Bunge, and Flavonoid luteolin have been confirmed to inhibit the maturation and function of DCs. However, these studies were not performed in specific LN models.
Since DCs are the key link between innate immunity and adaptive immunity and play crucial roles in both promoting immune defense and maintaining immune tolerance (Liu et al., 2021), they are attractive therapeutic targets for autoimmune disease, including LN. For example, evidence reveals that tolerance is observed when partial- or semi-maturation of DCs occurs, and the semi-mature DC phenotype seems to continuously tolerize lymph node T cells against tissue-derived self-antigens or apoptotic cells (Lutz and Schuler, 2002). Hopefully, the therapeutic potential of DCs may bring inspiration for LN treatment with traditional Chinese medicine.
3.7 Resident kidney cells
Resident kidney cells, including podocytes, mesangial cells, and tubular epithelial cells have always been considered essential factors in LN. On one hand, damage to these resident kidney cells leads to varying degrees of kidney function loss (Bhargava et al., 2023; Kwok and Tsokos, 2018). On the other hand, inflammatory stimuli to resident cells cause them to produce pro-inflammatory cytokines and present antigen, further aggravating kidney injury (Bhargava et al., 2023; Jamaly et al., 2021; Kwok and Tsokos, 2018; Sakhi et al., 2019).
3.7.1 Podocytes
Reactive oxygen species (ROS) generation can affect the integrity of the podocyte cytoskeleton, resulting in subsequent podocyte detachment from the glomerular basement membrane and onset of proteinuria (Bruno et al., 2023; Guo et al., 2024). Eucarbwenstols A-H could prevent podocyte injury through ROS modulation and regulation of mitochondrial membrane potential (Chen T. et al., 2022), thus potentially having a renoprotective effect.
Aberrant activation of the NLRP3 inflammasome, which might be partly caused by autophagy dysfunction (Biasizzo and Kopitar-Jerala, 2020), plays a significant role in the pathogenesis of LN. Inhibition of NLRP3 has been shown to ameliorate proteinuria, renal histologic lesions, and podocyte foot process effacement (Fu et al., 2017; Ummarino, 2017; Wu et al., 2021). The forementioned M1 can not only reduce ATP mediated ROS production but also inhibit the activation of NLRP3 inflammasome by enhancing autophagy induction in LPS-primed and ATP-activated podocyte (Lin et al., 2019).
Recent findings suggest that podocytes share many elements of the innate and adaptive immune systems (Bhargava and Tsokos, 2019). They produce and express complement components and receptors, as well as major histocompatibility complex and co-stimulatory molecules, which may be involved in local immune events (Moll et al., 2006; Appay et al., 1990). However, the study of TCM in these related fields has not yet been explored.
3.7.2 Mesangial cells
As the mesangium is one of the primary sites for IC deposition, mesangial cells (MCs) constantly undergo severe damage, resulting in excessive proliferation and increased extracellular matrix production (Liu M. et al., 2022). ICAM-1, a cell surface glycoprotein and an adhesion receptor that regulate leukocyte recruitment from circulation to sites of inflammation (Bui et al., 2020), was found in the mesangial area and deposited along the glomerular capillary walls in MRL/lpr mice. The distribution intensity of ICAM-1, immunoglobulins and C3 significantly decreased after treatment with stragalin in form of decoction (Chen et al., 1995). Langchuangjing Granule (LCJ) can also inhibit the increase of serum ICAM-1 content and partially improve plasma distribution, as well as suppress the atrophy of renal corpuscles and the proliferation of mesangial cells (Zhu et al., 2004).
The role of resident kidney cells in the development of LN is becoming more defined and distinct. However, there is a huge gap in the field of TCM treatment for resident kidney cells. Research on cells that play an equally important role in the pathogenesis of LN, such as tubular epithelial cells, has not been conducted. Additionally, the pathogenic capacities of resident kidney cells, such as podocytes’ abilities to present antigens and participate in the formation of crescents, have not been studied in the area of TCM. More recent studies have pointed to the restoration of kidney resident cell function using cell-targeted approaches to prevent and treat LN, and TCM should play a role in this effort. (Tables 1, 2, the plant name has been checked with http://mpns.kew.org/mpns-portal/).
4 Discussion
The pathogenesis of LN involves abnormal autoimmune responses, including autoantibody and immune complex deposition, activation of infiltrating immune cells, and damage to kidney resident cells. Traditional Chinese Medicine (TCM) treatments, primarily in the form of herbal formulas and phytochemicals, exhibit distinct therapeutic effects in LN by suppressing autoimmune activity, promoting regulatory functions, and protecting kidney cells. This review explores the role of TCM in modulating immunity and mitigating LN pathogenesis, highlighting its potential value in LN treatment.
Previous reviews on TCM treatment for LN have primarily categorized therapeutic effects based on specific drugs, detailing the mechanisms of herbal formulas and phytochemicals (Dou et al., 2023; Liu L. et al., 2022; Wang et al., 2022). In contrast, this review not only acknowledges previous research findings but also adopts an innovative approach by focusing on LN immunopathogenesis, systematically delineating TCM applications within immune mechanisms. This perspective enhances clarity, provides deeper insights into specific pathways, and offers greater research value for future studies.
However, this review has several limitations:
1.Limited coverage of certain immune cells. While this section provides a comprehensive review of T cells, B cells, neutrophils, monocytes, and dendritic cells, the discussion of others (e.g., NK cells, macrophage subtypes) remains limited due to the lack of relevant research.
2.Lack of mechanistic detail in some areas. Although various immune pathways are mentioned, some mechanisms are only briefly introduced. For example, while the relationship between resident kidney cells and immune responses is acknowledged, the specific ways in which TCM influences kidney cell-mediated immune modulation remain unexplored.
3.Absence of standardized evaluation of TCM effects. This review highlights the potential immunomodulatory effects of several herbal formulas and phytochemicals; however, there is no standardized comparison of their efficacy. Additionally, discussions on dosage, bioavailability, and pharmacokinetics of TCM compounds in LN treatment remain limited, despite their crucial role in clinical translation.
4.Insufficient discussion of clinical relevance. The review predominantly focuses on preclinical (in vitro and in vivo) studies, while clinical evidence for TCM interventions is scarcely addressed due to the diversity of herbal formulas and phytochemicals.
Therefore, the following directions for future research are proposed:
1.Expanding the study of immune cell subtypes and kidney resident cells. The autoimmune mechanisms underlying LN remain unclear, and research on TCM in LN is still incomplete and often idealized. Further investigation is needed into the roles of NK cells, specific macrophage subtypes, dendritic cells, and various kidney resident cells in LN pathogenesis, as well as their modulation by TCM.
2.Mechanistic exploration of TCM effects. Future studies should elucidate the precise molecular pathways through which TCM regulates immune responses in LN, particularly in relation to cytokine signaling, metabolic regulation, and epigenetic modifications.
3.Integration of TCM with conventional LN therapies. Research should explore the synergistic effects of TCM with standard immunosuppressive treatments (e.g., corticosteroids, MMF, anti-BAFF therapy) to assess whether TCM can enhance efficacy or mitigate side effects.
4.Clinical translation and standardization. More clinical trials are required to validate the efficacy and safety of TCM formulations. Establishing standardized dosages and assessing the bioavailability of TCM monomers will facilitate their clinical application.
5 Conclusion
This review offers insights into the cellular mechanisms of LN while primarily clarifying the immunological basis for selecting herbal formulas and phytochemicals of TCM origin in LN therapy. However, future research should address gaps in immune cell coverage, mechanistic insights, clinical applicability, and pharmacological standardization to improve the translational potential of TCM in LN treatment. With continued in-depth investigation, the development of more efficient, effective, and low-toxicity herbal formulas and phytochemicals for LN treatment is achievable.
Author contributions
ZH: Conceptualization, Data curation, Methodology, Visualization, Writing – original draft, Writing – review and editing. XL: Investigation, Visualization, Writing – review and editing. QZ: Conceptualization, Investigation, Methodology, Writing – original draft. MZ: Conceptualization, Investigation, Methodology, Writing – original draft. YF: Funding acquisition, Project administration, Supervision, Writing – review and editing. TZ: Funding acquisition, Project administration, Supervision, Writing – review and editing.
Funding
The author(s) declare that financial support was received for the research and/or publication of this article. This work was supported by the National Natural Science Foundation of China [grant number 82104798], Zhejiang Chinese Medical University school-level research fund project-natural Science Youth Exploration Project [grant number 2024JKZKTS20].
Conflict of interest
The authors declare that the research was conducted in the absence of any commercial or financial relationships that could be construed as a potential conflict of interest.
Generative AI statement
The author(s) declare that no Generative AI was used in the creation of this manuscript.
Publisher’s note
All claims expressed in this article are solely those of the authors and do not necessarily represent those of their affiliated organizations, or those of the publisher, the editors and the reviewers. Any product that may be evaluated in this article, or claim that may be made by its manufacturer, is not guaranteed or endorsed by the publisher.
Supplementary material
The Supplementary Material for this article can be found online at: https://www.frontiersin.org/articles/10.3389/fphar.2025.1523272/full#supplementary-material
References
Anders, H.-J., Saxena, R., Zhao, M.-h., Parodis, I., Salmon, J. E., and Mohan, C. (2020). Lupus nephritis. Nat. Rev. Dis. Prim. 6 (1), 7. doi:10.1038/s41572-019-0141-9
Appay, M. D., Kazatchkine, M. D., Levi-Strauss, M., Hinglais, N., and Bariety, J. (1990). Expression of CR1 (CD35) mRNA in podocytes from adult and fetal human kidneys. Kidney Int. 38 (2), 289–293. doi:10.1038/ki.1990.198
Arazi, A., Rao, D. A., Berthier, C. C., Davidson, A., Liu, Y., Hoover, P. J., et al. (2019). The immune cell landscape in kidneys of patients with lupus nephritis. Nat. Immunol. 20 (7), 902–914. doi:10.1038/s41590-019-0398-x
Askanase, A., Khalili, L., Tang, W., Mertz, P., Scherlinger, M., Sebbag, E., et al. (2023). New and future therapies: changes in the therapeutic armamentarium for SLE. Best. Pract. Res. Clin. Rheumatol. 37, 101865. doi:10.1016/j.berh.2023.101865
Astry, B., Venkatesha, S. H., Laurence, A., Christensen-Quick, A., Garzino-Demo, A., Frieman, M. B., et al. (2015). Celastrol, a Chinese herbal compound, controls autoimmune inflammation by altering the balance of pathogenic and regulatory T cells in the target organ. Clin. Immunol. 157 (2), 228–238. doi:10.1016/j.clim.2015.01.011
Bhargava, R., Li, H., and Tsokos, G. C. (2023). Pathogenesis of lupus nephritis: the contribution of immune and kidney resident cells. Curr. Opin. Rheumatol. 35 (2), 107–116. doi:10.1097/bor.0000000000000887
Bhargava, R., and Tsokos, G. C. (2019). The immune podocyte. Curr. Opin. Rheumatol. 31 (2), 167–174. doi:10.1097/bor.0000000000000578
Biasizzo, M., and Kopitar-Jerala, N. (2020). Interplay between NLRP3 inflammasome and autophagy. Front. Immunol. 11, 591803. doi:10.3389/fimmu.2020.591803
Biswas, S., Bieber, K., and Manz, R. A. (2022). IL-10 revisited in systemic lupus erythematosus. Front. Immunol. 13, 970906. doi:10.3389/fimmu.2022.970906
Bruno, V., Mühlig, A. K., Oh, J., and Licht, C. (2023). New insights into the immune functions of podocytes: the role of complement. Mol. Cell Pediatr. 10 (1), 3. doi:10.1186/s40348-023-00157-3
Bui, T. M., Wiesolek, H. L., and Sumagin, R. (2020). ICAM-1: a master regulator of cellular responses in inflammation, injury resolution, and tumorigenesis. J. Leukoc. Biol. 108 (3), 787–799. doi:10.1002/jlb.2mr0220-549r
Cai, B., Lu, H., Ye, Q., Xiao, Q., Wu, X., and Xu, H. (2023). Identification of potent target and its mechanism of action of Tripterygium wilfordii Hook F in the treatment of lupus nephritis. Int. J. Rheum. Dis. 26 (8), 1529–1539. doi:10.1111/1756-185x.14780
Chan, R. W., Lai, F. M., Li, E. K., Tam, L. S., Chow, K. M., Li, P. K., et al. (2006). Imbalance of Th1/Th2 transcription factors in patients with lupus nephritis. Rheumatol. Oxf. 45 (8), 951–957. doi:10.1093/rheumatology/kel029
Chávez-Galán, L., Olleros, M. L., Vesin, D., and Garcia, I. (2015). Much more than M1 and M2 macrophages, there are also CD169(+) and TCR(+) macrophages. Front. Immunol. 6, 263. doi:10.3389/fimmu.2015.00263
Chen, N., Dai, Y., Li, H., Long, X., Ke, J., Zhang, J., et al. (2023). Increased ILT2(+) natural killer T cells correlate with disease activity in systemic lupus erythematosus. Clin. Rheumatol. 42 (11), 3113–3121. doi:10.1007/s10067-023-06750-1
Chen, T., Ruan, D. D., Zhang, J. H., Wang, H. L., Wu, M., Wu, Q. Y., et al. (2022). Eucarbwenstols A-H, eight novel compounds from Eucalyptus robusta prevents MPC-5 injury via ROS modulation and regulation of mitochondrial membrane potential. Bioorg Chem. 129, 106159. doi:10.1016/j.bioorg.2022.106159
Chen, X., Wu, Q., Gong, Z., Ren, T., Du, Q., Yuan, Y., et al. (2022). A natural plant ingredient, menthone, regulates T cell subtypes and lowers pro-inflammatory cytokines of rheumatoid arthritis. J. Nat. Prod. 85 (4), 1109–1117. doi:10.1021/acs.jnatprod.1c01231
Chen, X., Yu, L., and Lu, Y. (1995). Image analysis for intercellular adhesion molecule-1 expression in MRI/lpr mice: effects of Chinese herb medicine. Zhonghua Yi Xue Za Zhi 75 (4), 204–206.
Crotty, S. (2011). Follicular helper CD4 T cells (TFH). Annu. Rev. Immunol. 29, 621–663. doi:10.1146/annurev-immunol-031210-101400
Dang, W. Z., Li, H., Jiang, B., Nandakumar, K. S., Liu, K. F., Liu, L. X., et al. (2019). Therapeutic effects of artesunate on lupus-prone MRL/lpr mice are dependent on T follicular helper cell differentiation and activation of JAK2-STAT3 signaling pathway. Phytomedicine 62, 152965. doi:10.1016/j.phymed.2019.152965
Davidson, A. (2016). What is damaging the kidney in lupus nephritis? Nat. Rev. Rheumatol. 12 (3), 143–153. doi:10.1038/nrrheum.2015.159
Desai, S. B., Ahdoot, R., Malik, F., Obert, M., and Hanna, R. (2024). New guidelines and therapeutic updates for the management of lupus nephritis. Curr. Opin. Nephrol. Hypertens. 33, 344–353. doi:10.1097/mnh.0000000000000969
Dou, L. L., Zhang, X. Y., Liu, D., and Liu, D. (2023). Role of traditional Chinese medicine for the treatment of lupus nephritis: mechanisms and applications. Altern. Ther. Health Med. 30, 154–165.
Eiza, N., Sabag, A. D., Kessler, O., Neufeld, G., and Vadasz, Z. (2023). CD72-semaphorin3A axis: a new regulatory pathway in systemic lupus erythematosus. J. Autoimmun. 134, 102960. doi:10.1016/j.jaut.2022.102960
Espeli, M., Bökers, S., Giannico, G., Dickinson, H. A., Bardsley, V., Fogo, A. B., et al. (2011). Local renal autoantibody production in lupus nephritis. J. Am. Soc. Nephrol. 22 (2), 296–305. doi:10.1681/asn.2010050515
Fakhfakh, R., Zian, Z., Elloumi, N., Abida, O., Bouallegui, E., Houssaini, H., et al. (2022). Th17 and Th1 cells in systemic lupus erythematosus with focus on lupus nephritis. Immunol. Res. 70 (5), 644–653. doi:10.1007/s12026-022-09296-7
Fan, L., Qiu, D., Huang, G., Chen, J., Wu, Q., Xiong, S., et al. (2020). Wogonin suppresses IL-10 production in B cells via STAT3 and ERK signaling pathway. J. Immunol. Res. 2020, 3032425. doi:10.1155/2020/3032425
Fazilleau, N., Mark, L., McHeyzer-Williams, L. J., and McHeyzer-Williams, M. G. (2009). Follicular helper T cells: lineage and location. Immunity 30 (3), 324–335. doi:10.1016/j.immuni.2009.03.003
Fu, R., Guo, C., Wang, S., Huang, Y., Jin, O., Hu, H., et al. (2017). Podocyte activation of NLRP3 inflammasomes contributes to the development of proteinuria in lupus nephritis. Arthritis Rheumatol. 69 (8), 1636–1646. doi:10.1002/art.40155
Furuya, Y., Kawakita, T., and Nomoto, K. (2001). Immunomodulating effect of a traditional Japanese medicine, hachimi-jio-gan (ba-wei-di-huang-wan), on Th1 predominance in autoimmune MRL/MP-lpr/lpr mice. Int. Immunopharmacol. 1 (3), 551–559. doi:10.1016/s1567-5769(00)00024-2
Gu, C., Liu, F., Luo, X., Zhou, X., Yang, J., and Levin, L. S. (2016). Triptolide reduces the required dose of tacrolimus by attenuating inflammation, enhancing immunosuppression, and increasing donor chimerism in a heterotopic hindlimb transplantation model. Plastic and reconstructive surgery, 138 (6), 1243–1253. doi:10.1097/PRS.0000000000002770
Guo, Z., Guo, Q., Li, X., Gao, X., Zhang, L., and Xu, K. (2024). Urinary biomarkers associated with podocyte injury in lupus nephritis. Front. Pharmacol. 15, 1324540. doi:10.3389/fphar.2024.1324540
Hakkim, A., Fürnrohr, B. G., Amann, K., Laube, B., Abed, U. A., Brinkmann, V., et al. (2010). Impairment of neutrophil extracellular trap degradation is associated with lupus nephritis. Proc. Natl. Acad. Sci. U. S. A. 107 (21), 9813–9818. doi:10.1073/pnas.0909927107
Heinemann, K., Wilde, B., Hoerning, A., Tebbe, B., Kribben, A., Witzke, O., et al. (2016). Decreased IL-10(+) regulatory B cells (Bregs) in lupus nephritis patients. Scand. J. Rheumatol. 45 (4), 312–316. doi:10.3109/03009742.2015.1126346
Hu, Q., Yang, C., Wang, Q., Zeng, H., and Qin, W. (2015). Demethylzeylasteral (T-96) treatment ameliorates mice lupus nephritis accompanied by inhibiting activation of NF-κB pathway. PLoS One 10 (7), e0133724. doi:10.1371/journal.pone.0133724
Hudspeth, K., Wang, S., Wang, J., Rahman, S., Smith, M. A., Casey, K. A., et al. (2019). Natural killer cell expression of Ki67 is associated with elevated serum IL-15, disease activity and nephritis in systemic lupus erythematosus. Clin. Exp. Immunol. 196 (2), 226–236. doi:10.1111/cei.13263
Ito, T., Seo, N., Yagi, H., Ohtani, T., Tokura, Y., Takigawa, M., et al. (2002). Unique therapeutic effects of the Japanese-Chinese herbal medicine, Sairei-to, on Th1/Th2 cytokines balance of the autoimmunity of MRL/lpr mice. J. Dermatol Sci. 28 (3), 198–210. doi:10.1016/s0923-1811(01)00161-x
Iwata, Y., Boström, E. A., Menke, J., Rabacal, W. A., Morel, L., Wada, T., et al. (2012). Aberrant macrophages mediate defective kidney repair that triggers nephritis in lupus-susceptible mice. J. Immunol. 188 (9), 4568–4580. doi:10.4049/jimmunol.1102154
Jakiela, B., Kosałka, J., Plutecka, H., Bazan-Socha, S., Sanak, M., and Musiał, J. (2018). Facilitated expansion of Th17 cells in lupus nephritis patients. Clin. Exp. Immunol. 194 (3), 283–294. doi:10.1111/cei.13196
Jamaly, S., Rakaee, M., Abdi, R., Tsokos, G. C., and Fenton, K. A. (2021). Interplay of immune and kidney resident cells in the formation of tertiary lymphoid structures in lupus nephritis. Autoimmun. Rev. 20 (12), 102980. doi:10.1016/j.autrev.2021.102980
Ka, S. M., Lin, J. C., Lin, T. J., Liu, F. C., Chao, L. K., Ho, C. L., et al. (2015). Citral alleviates an accelerated and severe lupus nephritis model by inhibiting the activation signal of NLRP3 inflammasome and enhancing Nrf2 activation. Arthritis Res. Ther. 17, 331. doi:10.1186/s13075-015-0844-6
Kienhöfer, D., Hahn, J., Stoof, J., Csepregi, J. Z., Reinwald, C., Urbonaviciute, V., et al. (2017). Experimental lupus is aggravated in mouse strains with impaired induction of neutrophil extracellular traps. JCI Insight 2 (10), e92920. doi:10.1172/jci.insight.92920
King, C., Tangye, S. G., and Mackay, C. R. (2008). T follicular helper (TFH) cells in normal and dysregulated immune responses. Annu. Rev. Immunol. 26, 741–766. doi:10.1146/annurev.immunol.26.021607.090344
Koga, T., Ichinose, K., and Tsokos, G. C. (2017). T cells and IL-17 in lupus nephritis. Clin. Immunol. 185, 95–99. doi:10.1016/j.clim.2016.04.010
Kumar, P., Balakrishnan, S., Surendra Lele, S., Setty, S., Dhingra, S., Epstein, A. L., et al. (2022). Restoration of follicular T regulatory/helper cell balance by ox40l-JAG1 cotreatment suppresses lupus nephritis in NZBWF1/j mice. J. Immunol. 208 (11), 2467–2481. doi:10.4049/jimmunol.2200057
Kurts, C., Ginhoux, F., and Panzer, U. (2020). Kidney dendritic cells: fundamental biology and functional roles in health and disease. Nat. Rev. Nephrol. 16 (7), 391–407. doi:10.1038/s41581-020-0272-y
Kwok, S. K., and Tsokos, G. C. (2018). New insights into the role of renal resident cells in the pathogenesis of lupus nephritis. Korean J. Intern Med. 33 (2), 284–289. doi:10.3904/kjim.2017.383
Layhadi, J. A., Moya, R., Tan, T. J., Lenormand, M. M., Sharif, H., Parkin, R. V., et al. (2023). Single-cell RNA sequencing identifies precise tolerogenic cellular and molecular pathways induced by depigmented-polymerized grass pollen allergen extract. J. Allergy Clin. Immunol. 151 (5), 1357–1370.e9. doi:10.1016/j.jaci.2022.11.030
Lech, M., and Anders, H.-J. (2013). The pathogenesis of lupus nephritis. J. Am. Soc. Nephrol. 24 (9), 1357–1366. doi:10.1681/asn.2013010026
Lee, K. H., Kronbichler, A., Park, D. D., Park, Y., Moon, H., Kim, H., et al. (2017). Neutrophil extracellular traps (NETs) in autoimmune diseases: a comprehensive review. Autoimmun. Rev. 16 (11), 1160–1173. doi:10.1016/j.autrev.2017.09.012
Li, D., Pan, B., Ma, N., Wang, X., Deng, X., Lai, H., et al. (2024). Efficacy and safety of Shenqi Dihuang decoction for lupus nephritis: a systematic review and meta-analysis. J. Ethnopharmacol. 323, 117602. doi:10.1016/j.jep.2023.117602
Li, G. Y., Liu, S. X., and Lu, X. Q. (2012). Effects of langchuangping granule on the expression of B lymphocyte activating factor in the peripheral blood B cells of BXSB lupus nephritis mice. Zhongguo Zhong Xi Yi Jie He Za Zhi 32 (3), 367–370.
Li, G. Y., Lu, X. Q., and Liu, S. X. (2011). Langohuangping granule down-regulated monocyte chemoattractant protein-1 via suppressing nuclear factor kappa B signaling pathway in BXSB lupus nephritis mice. Zhongguo Zhong Xi Yi Jie He Za Zhi 31 (12), 1685–1689.
Li, H., Geng, L., Cao, Z., Jin, Z., Yan, Q., Long, X., et al. (2023). CD56brightCD16-to CD57+CD56dimCD16+ NK cell ratio discriminates disease activity and renal involvement in patients with systemic lupus erythematosus. Clin. Exp. Rheumatol. 41 (9), 1768–1776. doi:10.55563/clinexprheumatol/o6bjl8
Li, J., Huang, L., Wang, S., Yao, Y., and Zhang, Z. (2016). Astragaloside IV attenuates inflammatory reaction via activating immune function of regulatory T-cells inhibited by HMGB1 in mice. Pharm. Biol. 54 (12), 3217–3225. doi:10.1080/13880209.2016.1216133
Li, M., Ma, J. J., Zhao, X. L., and Zhu, Y. (2014). Treating lupus nephritis by a drug pair of radix astragali and rehmanniae radix combined with glucocorticoid: a preliminary clinical study. Zhongguo Zhong Xi Yi Jie He Za Zhi 34 (8), 956–959.
Li, Y., Ding, T., Chen, J., Ji, J., Wang, W., Ding, B., et al. (2022a). The protective capability of Hedyotis diffusa Willd on lupus nephritis by attenuating the IL-17 expression in MRL/lpr mice. Front. Immunol. 13, 943827. doi:10.3389/fimmu.2022.943827
Li, Y., Tang, D., Yin, L., and Dai, Y. (2022b). New insights for regulatory T cell in lupus nephritis. Autoimmun. Rev. 21 (8), 103134. doi:10.1016/j.autrev.2022.103134
Liarski, V. M., Kaverina, N., Chang, A., Brandt, D., Yanez, D., Talasnik, L., et al. (2014). Cell distance mapping identifies functional T follicular helper cells in inflamed human renal tissue. Sci. Transl. Med. 6 (230), 230ra46. doi:10.1126/scitranslmed.3008146
Lin, T. J., Wu, C. Y., Tsai, P. Y., Hsu, W. H., Hua, K. F., Chu, C. L., et al. (2019). Accelerated and severe lupus nephritis benefits from M1, an active metabolite of ginsenoside, by regulating NLRP3 inflammasome and T cell functions in mice. Front. Immunol. 10, 1951. doi:10.3389/fimmu.2019.01951
Linke, A., Tiegs, G., and Neumann, K. (2022). Pathogenic T-cell responses in immune-mediated glomerulonephritis. Cells 11 (10), 1625. doi:10.3390/cells11101625
Liphaus, B. L., Silva, S. C., Palmeira, P., Silva, C. A., Goldenstein-Schainberg, C., and Carneiro-Sampaio, M. (2024). Reduced expressions of apoptosis-related proteins TRAIL, Bcl-2, and TNFR1 in NK cells of juvenile-onset systemic lupus erythematosus patients: relations with disease activity, nephritis, and neuropsychiatric involvement. Front. Immunol. 15, 1327255. doi:10.3389/fimmu.2024.1327255
Liu, C., Wang, D., Song, Y., Lu, S., Zhao, J., and Wang, H. (2018). Increased circulating CD4(+)CXCR5(+)FoxP3(+) follicular regulatory T cells correlated with severity of systemic lupus erythematosus patients. Int. Immunopharmacol. 56, 261–268. doi:10.1016/j.intimp.2018.01.038
Liu, J., Zhang, X., Cheng, Y., and Cao, X. (2021). Dendritic cell migration in inflammation and immunity. Cell Mol. Immunol. 18 (11), 2461–2471. doi:10.1038/s41423-021-00726-4
Liu, L., Zhang, L., and Li, M. (2022). Application of herbal traditional Chinese medicine in the treatment of lupus nephritis. Front. Pharmacol. 13, 981063. doi:10.3389/fphar.2022.981063
Liu, M., Zhang, L., Wang, Y., Hu, W., Wang, C., and Wen, Z. (2022). Mesangial cell: a hub in lupus nephritis. Front. Immunol. 13, 1063497. doi:10.3389/fimmu.2022.1063497
Liu, S. H., Lin, C. H., Hung, S. K., Chou, J. H., Chi, C. W., and Fu, S. L. (2010). Fisetin inhibits lipopolysaccharide-induced macrophage activation and dendritic cell maturation. J. Agric. Food Chem. 58 (20), 10831–10839. doi:10.1021/jf1017093
Liu, X., Bao, C., and Hu, D. (2012). Elevated interleukin-18 and skewed Th1:Th2 immune response in lupus nephritis. Rheumatol. Int. 32 (1), 223–229. doi:10.1007/s00296-010-1609-9
Liu, Y., Xu, K., Xiang, Y., Ma, B., Li, H., Li, Y., et al. (2023). Role of MCP-1 as an inflammatory biomarker in nephropathy. Front. Immunol. 14, 1303076. doi:10.3389/fimmu.2023.1303076
Lu, L. (2002). Study on effect of Cordyceps sinensis and artemisinin in preventing recurrence of lupus nephritis. Zhongguo Zhong Xi Yi Jie He Za Zhi 22 (3), 169–171.
Lutz, M. B., and Schuler, G. (2002). Immature, semi-mature and fully mature dendritic cells: which signals induce tolerance or immunity? Trends Immunol. 23 (9), 445–449. doi:10.1016/s1471-4906(02)02281-0
Maria, N. I., and Davidson, A. (2017). Renal macrophages and dendritic cells in SLE nephritis. Curr. Rheumatol. Rep. 19 (12), 81. doi:10.1007/s11926-017-0708-y
Mohan, C., Zhang, T., and Putterman, C. (2023). Pathogenic cellular and molecular mediators in lupus nephritis. Nat. Rev. Nephrol. 19 (8), 491–508. doi:10.1038/s41581-023-00722-z
Moll, S., Lange, S., Mihatsch, M. J., Dragic, Z., Schifferli, J. A., and Inal, J. M. (2006). CRIT is expressed on podocytes in normal human kidney and upregulated in membranous nephropathy. Kidney Int. 69 (11), 1961–1968. doi:10.1038/sj.ki.5000379
Mollazadeh, H., Cicero, A. F. G., Blesso, C. N., Pirro, M., Majeed, M., and Sahebkar, A. (2019). Immune modulation by curcumin: the role of interleukin-10. Crit. Rev. Food Sci. Nutr. 59 (1), 89–101. doi:10.1080/10408398.2017.1358139
Nishi, H., and Mayadas, T. N. (2019). Neutrophils in lupus nephritis. Curr. Opin. Rheumatol. 31 (2), 193–200. doi:10.1097/bor.0000000000000577
Nossent, J. C., Keen, H. I., Preen, D. B., and Inderjeeth, C. A. (2024). Population-wide long-term study of incidence, renal failure, and mortality rates for lupus nephritis. Int. J. Rheum. Dis. 27 (2), e15079. doi:10.1111/1756-185x.15079
Olmes, G., Büttner-Herold, M., Ferrazzi, F., Distel, L., Amann, K., and Daniel, C. (2016). CD163+ M2c-like macrophages predominate in renal biopsies from patients with lupus nephritis. Arthritis Res. Ther. 18, 90. doi:10.1186/s13075-016-0989-y
Parikh, S. V., Almaani, S., Brodsky, S., and Rovin, B. H. (2020). Update on lupus nephritis: core curriculum 2020. Am. J. Kidney Dis. 76 (2), 265–281. doi:10.1053/j.ajkd.2019.10.017
Park, Y. W., Kee, S. J., Cho, Y. N., Lee, E. H., Lee, H. Y., Kim, E. M., et al. (2009). Impaired differentiation and cytotoxicity of natural killer cells in systemic lupus erythematosus. Arthritis Rheum. 60 (6), 1753–1763. doi:10.1002/art.24556
Petri, M., Fang, C., and Goldman, D. W. (2023). East-asian lupus nephritis in the hopkins lupus cohort. Rheumatol. Immunol. Res. 4 (3), 157–161. doi:10.2478/rir-2023-0022
Qiu, D., and Kao, P. N. (2003). Immunosuppressive and anti-inflammatory mechanisms of triptolide, the principal active diterpenoid from the Chinese medicinal herb Tripterygium wilfordii Hook. f. Drugs R. D. 4 (1), 1–18. doi:10.2165/00126839-200304010-00001
Qiu, D., Zhao, G., Aoki, Y., Shi, L., Uyei, A., Nazarian, S., et al. (1999). Immunosuppressant PG490 (triptolide) inhibits T-cell interleukin-2 expression at the level of purine-box/nuclear factor of activated T-cells and NF-kappaB transcriptional activation. J. Biol. Chem. 274 (19), 13443–13450. doi:10.1074/jbc.274.19.13443
Ramsköld, D., Parodis, I., Lakshmikanth, T., Sippl, N., Khademi, M., Chen, Y., et al. (2019). B cell alterations during BAFF inhibition with belimumab in SLE. EBioMedicine 40, 517–527. doi:10.1016/j.ebiom.2018.12.035
Richoz, N., Tuong, Z. K., Loudon, K. W., Patiño-Martínez, E., Ferdinand, J. R., Portet, A., et al. (2022). Distinct pathogenic roles for resident and monocyte-derived macrophages in lupus nephritis. JCI Insight 7 (21), e159751. doi:10.1172/jci.insight.159751
Rodríguez Mesa, X. M., Contreras Bolaños, L. A., Modesti Costa, G., Mejia, A. L., and Santander González, S. P. (2023). A bidens pilosa L. Non-polar extract modulates the polarization of human macrophages and dendritic cells into an anti-inflammatory phenotype. Molecules 28 (20), 7094. doi:10.3390/molecules28207094
Rubtsova, K., Rubtsov, A. V., Thurman, J. M., Mennona, J. M., Kappler, J. W., and Marrack, P. (2017). B cells expressing the transcription factor T-bet drive lupus-like autoimmunity. J. Clin. Invest. 127 (4), 1392–1404. doi:10.1172/jci91250
Sakhi, H., Moktefi, A., Bouachi, K., Audard, V., Hénique, C., Remy, P., et al. (2019). Podocyte injury in lupus nephritis. J. Clin. Med. 8 (9), 1340. doi:10.3390/jcm8091340
Samy, E., Wax, S., Huard, B., Hess, H., and Schneider, P. (2017). Targeting BAFF and APRIL in systemic lupus erythematosus and other antibody-associated diseases. Int. Rev. Immunol. 36 (1), 3–19. doi:10.1080/08830185.2016.1276903
Saraiva, M., and O’Garra, A. (2010). The regulation of IL-10 production by immune cells. Nat. Rev. Immunol. 10 (3), 170–181. doi:10.1038/nri2711
Saraiva, M., Vieira, P., and O’Garra, A. (2020). Biology and therapeutic potential of interleukin-10. J. Exp. Med. 217 (1), e20190418. doi:10.1084/jem.20190418
Sciascia, S., Cozzi, M., Barinotti, A., Radin, M., Cecchi, I., Fenoglio, R., et al. (2022). Renal fibrosis in lupus nephritis. Int. J. Mol. Sci. 23 (22), 14317. doi:10.3390/ijms232214317
Singh, J. A., Hossain, A., Kotb, A., and Wells, G. (2016a). Risk of serious infections with immunosuppressive drugs and glucocorticoids for lupus nephritis: a systematic review and network meta-analysis. BMC Med. 14 (1), 137. doi:10.1186/s12916-016-0673-8
Singh, J. A., Hossain, A., Kotb, A., and Wells, G. A. (2016b). Comparative effectiveness of immunosuppressive drugs and corticosteroids for lupus nephritis: a systematic review and network meta-analysis. Syst. Rev. 5 (1), 155. doi:10.1186/s13643-016-0328-z
Song, J., He, G. N., and Dai, L. (2023). A comprehensive review on celastrol, triptolide and triptonide: Insights on their pharmacological activity, toxicity, combination therapy, new dosage form and novel drug delivery routes. Biomedicine and pharmacotherapy, 162, 114705. doi:10.1016/j.biopha.2023.114705
Tao, J., Zhao, J., Qi, X. M., and Wu, Y. G. (2021). Complement-mediated M2/M1 macrophage polarization may be involved in crescent formation in lupus nephritis. Int. Immunopharmacol. 101 (Pt A), 108278. doi:10.1016/j.intimp.2021.108278
Tao, X., Fan, F., Hoffmann, V., Gao, C. Y., Longo, N. S., Zerfas, P., et al. (2008). Effective therapy for nephritis in (NZB x NZW)F1 mice with triptolide and tripdiolide, the principal active components of the Chinese herbal remedy Tripterygium wilfordii Hook F. Arthritis Rheum. 58 (6), 1774–1783. doi:10.1002/art.23513
Tsai, P. Y., Ka, S. M., Chang, J. M., Chang, W. L., Huang, Y. J., Hung, L. M., et al. (2011). Therapeutic potential of DCB-SLE1, an extract of a mixture of Chinese medicinal herbs, for severe lupus nephritis. Am. J. Physiol. Ren. Physiol. 301 (4), F751–F764. doi:10.1152/ajprenal.00706.2010
Tsai, P. Y., Ka, S. M., Chang, J. M., Lai, J. H., Dai, M. S., Jheng, H. L., et al. (2012). Antroquinonol differentially modulates T cell activity and reduces interleukin-18 production, but enhances Nrf2 activation, in murine accelerated severe lupus nephritis. Arthritis Rheum. 64 (1), 232–242. doi:10.1002/art.33328
Tsokos, G. C., Lo, M. S., Costa Reis, P., and Sullivan, K. E. (2016). New insights into the immunopathogenesis of systemic lupus erythematosus. Nat. Rev. Rheumatol. 12 (12), 716–730. doi:10.1038/nrrheum.2016.186
Tucci, M., Stucci, S., Strippoli, S., and Silvestris, F. (2010). Cytokine overproduction, T-cell activation, and defective T-regulatory functions promote nephritis in systemic lupus erythematosus. J. Biomed. Biotechnol. 2010, 457146. doi:10.1155/2010/457146
Ummarino, D. (2017). Lupus nephritis: NLRP3 inflammasome ignites podocyte dysfunction. Nat. Rev. Rheumatol. 13 (8), 451. doi:10.1038/nrrheum.2017.97
Vadasz, Z., Haj, T., Balbir, A., Peri, R., Rosner, I., Slobodin, G., et al. (2014). A regulatory role for CD72 expression on B cells in systemic lupus erythematosus. Semin. Arthritis Rheum. 43 (6), 767–771. doi:10.1016/j.semarthrit.2013.11.010
van der Linden, M., van den Hoogen, L. L., Westerlaken, G. H. A., Fritsch-Stork, R. D. E., van Roon, J. A. G., Radstake, T., et al. (2018). Neutrophil extracellular trap release is associated with antinuclear antibodies in systemic lupus erythematosus and anti-phospholipid syndrome. Rheumatol. Oxf. 57 (7), 1228–1234. doi:10.1093/rheumatology/key067
Wang, S., Wang, J., Kumar, V., Karnell, J. L., Naiman, B., Gross, P. S., et al. (2018). IL-21 drives expansion and plasma cell differentiation of autoreactive CD11c(hi)T-bet(+) B cells in SLE. Nat. Commun. 9 (1), 1758. doi:10.1038/s41467-018-03750-7
Wang, Y.-J., Li, Y.-X., Li, S., He, W., Wang, Z.-R., Zhan, T.-P., et al. (2022). Progress in traditional Chinese medicine and natural extracts for the treatment of lupus nephritis. Biomed. and Pharmacother. 149, 112799. doi:10.1016/j.biopha.2022.112799
Watanabe, R., Ishiura, N., Nakashima, H., Kuwano, Y., Okochi, H., Tamaki, K., et al. (2010). Regulatory B cells (B10 cells) have a suppressive role in murine lupus: CD19 and B10 cell deficiency exacerbates systemic autoimmunity. J. Immunol. 184 (9), 4801–4809. doi:10.4049/jimmunol.0902385
Wu, D., Ai, L., Sun, Y., Yang, B., Chen, S., Wang, Q., et al. (2021). Role of NLRP3 inflammasome in lupus nephritis and therapeutic targeting by phytochemicals. Front. Pharmacol. 12, 621300. doi:10.3389/fphar.2021.621300
Wu, D. H., Xu, L., Wen, C. P., Xie, G. Q., Ji, J. J., Pan, J. L., et al. (2015). The effects of Jieduquyuzishen prescription-treated rat serum on the BAFF/BAFF-R signal pathway. PLoS One 10 (2), e0118462. doi:10.1371/journal.pone.0118462
Xiong, H., Tang, Z., Xu, Y., Shi, Z., Guo, Z., Liu, X., et al. (2022). CD19(+)CD24(high)CD27(+) B cell and interleukin 35 as potential biomarkers of disease activity in systemic lupus erythematosus patients. Adv. Rheumatol. 62 (1), 48. doi:10.1186/s42358-022-00279-8
Xu, B., Wang, S., Zhou, M., Huang, Y., Fu, R., Guo, C., et al. (2017). The ratio of circulating follicular T helper cell to follicular T regulatory cell is correlated with disease activity in systemic lupus erythematosus. Clin. Immunol. 183, 46–53. doi:10.1016/j.clim.2017.07.004
Xu, Y. Y., Wang, D. M., Liang, H. S., Liu, Z. H., Li, J. X., Wang, M. J., et al. (2020). The role of Th17/treg Axis in the traditional Chinese medicine intervention on immune-mediated inflammatory diseases: a systematic review. Am. J. Chin. Med. 48 (3), 535–558. doi:10.1142/s0192415x20500275
Yang, J., Yang, X., and Li, M. (2012). Baicalin, a natural compound, promotes regulatory T cell differentiation. BMC Complement. Altern. Med. 12, 64. doi:10.1186/1472-6882-12-64
Yang, J., Yang, X., Yang, J., and Li, M. (2019). Baicalin ameliorates lupus autoimmunity by inhibiting differentiation of Tfh cells and inducing expansion of Tfr cells. Cell Death Dis. 10 (2), 140. doi:10.1038/s41419-019-1315-9
Yoshioka, Y., Kamata, Y., Tominaga, M., Umehara, Y., Yoshida, I., Matsuoka, N., et al. (2021). Extract of Scutellaria baicalensis induces semaphorin 3A production in human epidermal keratinocytes. PLoS One 16 (4), e0250663. doi:10.1371/journal.pone.0250663
Yuan, S., Zeng, Y., Li, J., Wang, C., Li, W., He, Z., et al. (2022). Phenotypical changes and clinical significance of CD4(+)/CD8(+) T cells in SLE. Lupus Sci. Med. 9 (1), e000660. doi:10.1136/lupus-2022-000660
Zhang, J., Shan, J., Chen, X., Li, S., Long, D., and Li, Y. (2018). Celastrol mediates Th17 and Treg cell generation via metabolic signaling. Biochem. Biophys. Res. Commun. 497 (3), 883–889. doi:10.1016/j.bbrc.2018.02.163
Zhou, C. J., Ma, F., Liao, W. J., Song, L. J., Yu, D., Song, Y. N., et al. (2019). Restoration of immune suppressor function of regulatory B cells collected from patients with allergic rhinitis with Chinese medical formula Yupingfeng San. Am. J. Transl. Res. 11 (3), 1635–1643.
Zhou, C. J., Xie, B. L., Han, H. Y., Wang, Y., Wang, Y. H., Hong, J. Y., et al. (2021). Short-chain fatty acids promote immunotherapy by modulating immune regulatory property in B cells. J. Immunol. Res. 2021, 2684361. doi:10.1155/2021/2684361
Zhu, F. S., Jin, S., and Wang, Y. (2004). Experimental study on inhibitory effect of langchuangjing granule on lupoid change of kidney in lupus-prone mice. Zhongguo Zhong Xi Yi Jie He Za Zhi 24 (4), 343–347.
Zhu, Y., Zou, L., and Liu, Y. C. (2016). T follicular helper cells, T follicular regulatory cells and autoimmunity. Int. Immunol. 28 (4), 173–179. doi:10.1093/intimm/dxv079
Zou, H., He, T., and Chen, X. (2019). Tetrandrine inhibits differentiation of proinflammatory subsets of T helper cells but spares de novo differentiation of iTreg cells. Int. Immunopharmacol. 69, 307–312. doi:10.1016/j.intimp.2019.01.040
Glossary
LN lupus nephritis
SLE systemic lupus erythematosus
TCM traditional Chinese medicine
CYC cyclophosphamide
MMF mycophenolate mofetil
GC glucocorticoids
Th1 cell T helper 1
Th17 cell T helper 17
Tfh cell T follicular helper
Treg cell T regulatory
iTreg cell induced regulatory T
ABCs age-associated B cells
MoMac monocyte-derived macrophages
TrMac tissue-resident macrophages
M1 classically activated macrophages
M2 alternative activated macrophages
NK cell Natural Killer
DC dendritic cells
BMDC bone marrow-derived dendritic cell
MC mesangial cell
IL-17 interleukin-17
IL-21 interleukin-21
IL-4 interleukin-4
IL-12 interleukin-12
IL-18 interleukin-18
IL-1 interleukin-1
IL-6 interleukin-6
IL-10 interleukin-10
IL-35 interleukin-35
IL-17 interleukin-17
NETs Neutrophil extracellular traps
NETosis Neutrophil Extracellular Trap formation
AST IV Astragaloside IV
BAFF B-cell activating factor
APRIL a proliferation-inducing ligand
ROS Reactive oxygen species
MCP-1 monocyte chemoattractant protein-1
TWHF Tripterygium wilfordii Hook F
LG Langchuangping Granule
JP Jieduquyuzishen prescription
DPG-POL-Phl p Depigmented-polymerized phleum pratense
T-96 Demethylzeylasteral
LCJ Langchuangjing Granule.
Keywords: lupus nephritis, plant extracts, herbal formula, Chinese herbal, immune system, systemic lupus erythematosus
Citation: Huang Z, Li X, Zhu Q, Zhu M, Fan Y and Zhao T (2025) Traditional Chinese medicine for lupus nephritis: modulation of autoimmune pathogenesis. Front. Pharmacol. 16:1523272. doi: 10.3389/fphar.2025.1523272
Received: 05 November 2024; Accepted: 14 April 2025;
Published: 02 May 2025.
Edited by:
Weicheng Hu, Yangzhou University, ChinaReviewed by:
Gina Stella Garcia-Romo, National Autonomous University of Mexico, MexicoShokoh Parham, University of Technology Malaysia, Malaysia
Copyright © 2025 Huang, Li, Zhu, Zhu, Fan and Zhao. This is an open-access article distributed under the terms of the Creative Commons Attribution License (CC BY). The use, distribution or reproduction in other forums is permitted, provided the original author(s) and the copyright owner(s) are credited and that the original publication in this journal is cited, in accordance with accepted academic practice. No use, distribution or reproduction is permitted which does not comply with these terms.
*Correspondence: Yongsheng Fan, Znlzemp0Y21AMTYzLmNvbQ==; Ting Zhao, emhhb3RpbmdAemNtdS5lZHUuY24=
†Present address: Zhiyan Huang, The Second Affiliated Hospital of Zhejiang Chinese Medical University, Hangzhou, China
‡These authors have contributed equally to this work