- 1School of Clinical Medicine, Chengdu University of Traditional Chinese Medicine, Chengdu, China
- 2Department of Gynecology, Hospital of Chengdu University of Traditional Chinese Medicine, Chengdu, China
The application of botanical drugs and their metabolites in the treatment of pelvic inflammatory disease (PID) has garnered significant attention. Owing to their broad-spectrum activity, global accessibility, and structural diversity, botanical drugs have emerged as promising candidates for adjunctive or alternative therapies. This review systematically summarizes botanical drugs and their metabolites, focusing on their antimicrobial potential against endogenous and exogenous pathogens associated with PID. Specifically, it addresses various underlying antibacterial mechanisms, including interference with bacterial cell membranes and cell walls, inhibition of pathogen-specific efflux pumps, modulation of pathogen-related gene expression, and synergistic effects when combined with conventional antibiotics. This review highlights the therapeutic promise of botanical drugs and their metabolites, emphasizing critical findings regarding their inhibitory effects on PID-associated pathogens. Such insights provide valuable guidance for future therapeutic strategies and may support ongoing antibiotic discovery and development.
1 Introduction
Pelvic inflammatory disease (PID) is a severe infectious-inflammatory condition affecting the female upper genital tract, characterized by endometritis, salpingitis, oophoritis, and/or pelvic peritonitis (Brunham et al., 2015). It is associated with significant reproductive sequelae, including tubal-factor infertility, ectopic pregnancy, and chronic pelvic pain (Haggerty et al., 2010). The etiology of PID primarily involves polymicrobial infections, notably Neisseria gonorrhoeae (Quillin and Seifert, 2018), Chlamydia trachomatis (Darville, 2021) and anaerobic or atypical bacteria (Mitchell et al., 2021). Current clinical guidelines advocate broad-spectrum antimicrobial regimens (Smith et al., 2007; Petrina et al., 2019)—typically combining ceftriaxone, doxycycline, metronidazole, and levofloxacin—with a transition to oral therapy after 24–48 h of clinical improvement, typically spanning 14 days (Workowski et al., 2021). While randomized controlled trials and clinical studies demonstrate resolution of symptoms and pathogens with these regimens (Wiesenfeld et al., 2021; Petrina et al., 2019), prolonged antibiotic use raises concerns such as drug hypersensitivity, gut dysbiosis, and emerging multidrug resistance (Petrina et al., 2019; Horton et al., 2015; Scott et al., 2016). Cephalosporins, a cornerstone of PID therapy, are associated with underreported central nervous system-related adverse drug reactions (ADRs) (Cock, 2015; Bhattacharyya et al., 2016; Grill and Maganti, 2011). Surveillance data from the FDA Adverse Event Reporting System (FAERS) reveal a progressive rise in doxycycline-associated ADRs, with 9,641 cases documented between 2003 and 2024 (Xiong et al., 2024). These challenges underscore the urgent need for alternative therapies.
Botanical drugs, particularly those derived from traditional Asian medicine, represent a promising Frontier (Dushime et al., 2020). Natural metabolites from plants such as Solanum nigrum [Solanaceae; S. nigrum L.] and Althaea officinalis [Malvaceae; A. officinalis L.] exhibit antibacterial and anti-inflammatory properties historically leveraged in PID management (Khare, 2008). Preclinical studies highlight the efficacy of Distemonanthus benthamianus [Fabaceae; D. benthamianus Baill] and Solanum torvum [Solanaceae; S. torvum Sw.] extracts against N. gonorrhoeae, with metabolites like esculetin disrupting bacterial cell wall integrity (Obiang et al., 2019). Despite these advances, mechanistic insights into botanical therapies remain fragmented.
This review synthesizes current evidence on phytopharmaceuticals and their metabolites targeting PID-associated pathogens, aiming to identify novel therapeutic strategies while addressing critical research gaps in molecular mechanisms and clinical translatability.
2 Scope and terminology
This review involved an extensive search of relevant databases and resources, including PubMed, Embase, China National Knowledge Infrastructure (CNKI), and the Pharmacopoeia of the People’s Republic of China. A combination of Medical Subject Headings (MeSH) and keyword searches was employed, with terms such as “C. trachomatis,” “N. gonorrhoeae,” “botanical drug,” “pharmacological actions,” “herb,” “herbal medicine,” “pelvic inflammatory disease,” “endometritis,” and “salpingitis.” The search strategy is provided in Supplementary Table 1, with the final search conducted up to 12 November 2024.
Predefined inclusion criteria: 1) Studies focusing on botanical drugs for pelvic inflammatory disease (PID); 2) Studies published in English or Chinese; 3) Phytochemical composition of the herbal intervention was identified. Exclusion criteria: 1) Studies with unavailable full text; 2) Duplicate publications; 3) Non-original research (e.g., reviews, commentaries).
The accuracy of all plant names was confirmed using Plants of the World Online (http://www.plantsoftheworldonline.org). The botanical drug extracts discussed in this study are produced through well-defined extraction and preparation methods. The primary bioactive compounds of these botanical drugs are their metabolites. Furthermore, compound formulations of botanical drugs have been subjected to mass spectrometric analysis, enabling clear identification of their metabolites. All the composition of botanical drugs associating with PID are in Table 1.
3 Pathogens of PID
The common pathogens of PID can be briefly categorized into two groups based on the route of causation: 1) exogenous pathogens, primarily sexually transmitted microorganisms such as N. Gonorrhoeae, C. trachomatis and Mycoplasma genitalium; 2) endogenous pathogens, which include bacteria associated with bacterial vaginosis (BV) as well as other anaerobic, parthenogenetic, and aerobic microorganisms, such as Haemophilus influenzae, Escherichia coli and Mycobacterium anisopliae. The co-occurrence of microorganisms from both categories can lead to synergistic interactions that exacerbate the clinical progression of the disease. For instance, the presence of aerobic bacteria can cause tissue necrosis and induce anaerobic conditions, which, in turn, promote the growth of anaerobic bacteria and the formation of tubo-ovarian abscesses (Sweet, 1975).
3.1 Exogenous pathogens
C. trachomatis is the most prevalent cause of bacterial sexually transmitted infections worldwide, accounts for an estimated 131 million new cases each year (Newman et al., 2015). As an obligate intracellular bacterium, C. trachomatis has a specialized life cycle that predisposes it to persistent or recurrent infections (Brunham and Rey-ladino, 2005; Paavonen, 2012). A significant portion of these infections, particularly among women, are asymptomatic or subclinical, with silent carriers constituting up to 70% of the female cases (Hoenderboom et al., 2017). When untreated, C. trachomatis can ascend from the lower genital tract to affect the uterus, fallopian tubes, and ovaries, frequently leading to PID (Haggerty et al., 2010). Such developments considerably increase the risk of severe reproductive health issues, including ectopic pregnancies and infertility. The link between C. trachomatis infections and these detrimental reproductive health outcomes has been further validated by recent research (Oakeshott et al., 2010; Davies et al., 2016; Reekie et al., 2018).
N. Gonorrhoeae, a Gram-negative diplococcus bacterium, predominantly targets the genitourinary system, as well as the rectum and pharynx. Many of these infections are asymptomatic, complicating detection and treatment efforts (Keshvani et al., 2019). Without appropriate therapeutic intervention, the bacterium can lead to severe health issues including tubal infections, PID, ectopic pregnancies, infertility, and even cause blindness in neonates. Notably, N. Gonorrhoeae stands as the second most widespread bacterial sexually transmitted infection worldwide, trailing only C. trachomatis (Unemo et al., 2019), with an alarming rise in cases from 78 million in 2012 to 87 million by 2016 (Rowley et al., 2019; Newman et al., 2015). The emergence of strains resistant to critical antibiotics such as ceftriaxone and azithromycin has been documented, signaling a pressing challenge in the treatment landscape (Jennison et al., 2019; Eyre et al., 2019). This situation has heightened the urgency for innovative public health strategies and the development of alternative therapeutic measures, particularly in light of limited effective antibiotic options (Tedijanto et al., 2020). Accordingly, the World Health Organization (WHO) has classified N. Gonorrhoeae as a “priority pathogen,” underscoring the critical need for new therapeutic avenues to manage this persistent public health threat (Tacconelli et al., 2018).
3.2 Endogenous pathogens
Bacteria associated with bacterial vaginosis is characterized by a transformation of the vaginal microbiota, shifting from a predominance of Lactobacillus species to a varied composition featuring parthenogenetic and anaerobic microorganisms (Hillier et al., 1996). This alteration is associated with the pathogenesis of PID. Specifically, anaerobic Gram-negative bacilli, frequently identified among the BV-associated microbiota, have been isolated from the upper genital tract in patients suffering from endometritis and salpingitis, highlighting their potential contribution to the development of PID. In a study encompassing 545 women with symptoms indicative of PID, significant associations were found between the detection of BV-related genera and species like Sneathia, Atopobium vaginae and BVAB1 in cervical or endometrial samples, and the histological confirmation of endometritis, using PCR techniques (Haggerty et al., 2016). In a similar vein, research conducted in Kenya noted that individuals diagnosed with salpingitis were more likely to exhibit PCR-detected BV-associated bacteria in their tubal samples than control subjects without salpingitis (Hebb et al., 2004). Moreover, a comprehensive longitudinal study involving 2,958 participants demonstrated that a diagnosis of BV, based on either Amsel criteria or Nugent score, was linked with an elevated risk of developing PID (Turpin et al., 2021). Another longitudinal cohort study underscored that the presence of BV-associated microorganisms, as determined through culturing techniques, doubled the incidence of PID among the studied population (Ness et al., 2005).
In many instances, PID has been identified as polymicrobial in nature. In Kenyan patients with laparoscopically confirmed acute salpingitis, analysis of tubal specimens revealed the presence of 3–10 distinct phylotypes of 16S rRNA DNA, including organisms typically associated with the oropharyngeal and gastrointestinal tracts, in addition to those linked to BV (Hebb et al., 2004). Investigations into participants with laparoscopically confirmed tubal infections have detected a variety of anaerobic organisms (including Mycobacterium spp., Clostridium spp.) and parthenogenetic or aerobic organisms (such as E. coli, Streptococcus spp., Staphylococcus spp., H. influenzae) isolated via culture methods from the fallopian tubes or peritoneal samples.
4 Antibacterial effect of botanical drugs and its metabolites on exogenous pathogens
4.1 Chlamydia trachomatis
C. trachomatis primarily targets urogenital epithelial cells, specifically the columnar and transitional types, causing extensive functional disruptions and structural damage to the fallopian tubes. Such damage often leads to the formation of pelvic adhesions. The persistence of these infections is greatly influenced by the pathogen’s unique biphasic life cycle. This cycle involves alternating between two distinct morphological states: the Elementary Body (EB) and the Reticulate Body (RB) (Abdelrahman and Belland, 2005). The EB is the extracellular, metabolically inactive form that plays a critical role in the dissemination of the infection and the initial invasion of host cells. It adheres to susceptible host cells and penetrates them effectively. Following entry, the EB is encapsulated by a phagosome from the host cell, which initiates its transformation into the RB. This transformation marks a pivotal shift in the pathogen’s lifecycle as it moves from an inert state into an active intracellular replication phase, characterized by increased bacterial metabolism and the transition from the EB to the RB form (Belland et al., 2003) (Figure 1). The complete developmental cycle, encompassing the conversion from RB back to EB, is completed within approximately 40–48 h, at which point newly formed EBs are released to infect adjacent cells (Lee et al., 2018). While antibiotic therapy is generally effective against C. trachomatis infections, the pathogen can re-initiate its developmental cycle following the discontinuation of treatment, resulting in persistent reinfection and an elevated risk of antibiotic resistance (Kintner et al., 2014). Consequently, the development of innovative strategies to prevent recurrent C. trachomatis infections represents an urgent and critical area of research.
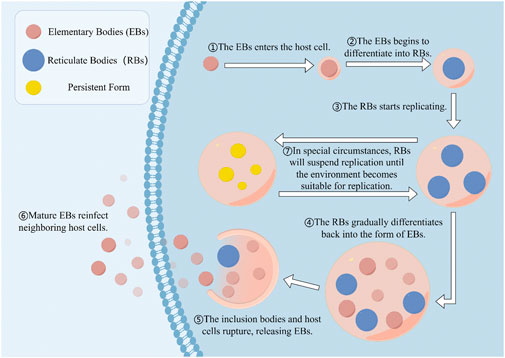
Figure 1. The developmental cycle of Chlamydia trachomatis. Infectious EBs (elementary bodies) enter host cells, whereupon the EBS begin to differentiate into replicable RBs (reticulate bodies). During the RBs replication stage, metabolites of the host cells provide the material basis for the pathogen. After several rounds of replication, RBs redifferentiate into EBs and are released upon rupture of the cell membrane or lysis of the host cell, subsequently infecting other cells. In stressful environments, RBs will cease replication and transform into a stable form until the stress disappears.
Research has highlighted the potential of certain metabolites to disrupt the life cycle of C. trachomatis. Among these, Andrographolide (Andro), a diterpenoid lactone isolated from the plant Andrographis paniculata [Acanthaceae; A. paniculata (Burm.f.) Wall. ex Nees], has been shown to effectively inhibit the infection process of C. trachomatis within cultured human cervical epithelial cells. Andrographolide’s mechanism is multifunctional in its interaction with the pathogen and the host cells. It notably inhibits the transformation of C. trachomatis from its metabolically active RB stage to its infectious EB form. Furthermore, Andrographolide significantly diminishes the release of pro-inflammatory cytokines such as IL-6, C-X-C motif chemokine ligand 8 (CXCL-8), and interferon-gamma- IP-10 by epithelial cells triggered by the infection (Hua et al., 2015). This study have investigated the cytotoxicity of Andro in vitro, using various concentrations to identify a range with minimal cytotoxic effects. However, the role of Andro-induced downregulation of IL-6, CXCL-8, and IP-10 in host immunity—particularly within the context of host-pathogen interactions—remains unclear. Therefore, additional in vivo studies are warranted to elucidate these mechanisms.
Baicalin, a flavonoid derived from Scutellaria baicalensis [Lamiaceae; S. baicalensis Georgi], has demonstrated notable inhibitory effects against C. trachomatis serovar D infection in Hep-2 cells. Huang Hao et al. reported that baicalin significantly reduces C. trachomatis infection in a dose-dependent manner, achieving over 75% inhibition at concentrations ranging from 0.12 to 0.48 mg/mL (Hao et al., 2009). Furthermore, baicalin treatment was shown to upregulate RFX5 expression while concurrently downregulating the chlamydial protease-like activity factor (CPAF), a protease implicated in the degradation of RFX5. These findings highlight CPAF as a primary molecular target of baicalin, underscoring its critical role in modulating RFX5 expression. Subsequent investigations further revealed that baicalin effectively suppressed the overexpression of Toll-like receptor 2 (TLR2) and Toll-like receptor 4 (TLR4) in C. trachomatis-infected mice cervical tissues (Hao et al., 2011). In addition, baicalin significantly reduced the number and size of chlamydial inclusion bodies, inhibited the activation of CPAF, and prevented the degradation of RFX5 and upstream stimulatory factor 1 (USF-1). Notably, baicalin also mitigated the downregulation of major histocompatibility complex class I (MHC I) and CD1d expression on the surface of infected cells. This suggests that baicalin plays a crucial role in preserving antigen presentation and enhancing the host immune response during C. trachomatis infection (Hao et al., 2013). Nevertheless, toxicity assessments of baicalin were not performed in the referenced studies, and further investigation is required to determine its safety profile.
4.2 Neisseria gonorrhoeae
N. gonorrhoeae is a Gram-negative bacterium and one of the primary pathogens responsible for PID. N. gonorrhoeae expresses three major outer membrane proteins: the porin ion channel protein (PorB), opacity-associated (Opa) protein, and reduction-modifiable protein (Rmp). In addition to these, it possesses two key pathogen-associated molecular patterns (PAMPs): lipooligosaccharide (LOS) and type IV pili (Edwards and Apicella, 2004). Allelic variation is common among PorB isoforms across gonococcal strains, predominantly occurring within the surface-exposed loops of the porin molecules (Garvin et al., 2008). In terms of antigenic variation, a single N. gonorrhoeae strain can encode up to 11 distinct opa genes, enabling the constitutive expression of multiple Opa protein variants (Bhat et al., 1991). These characteristics allow N. gonorrhoeae to establish persistent infections, as the human immune system struggles to develop effective immunological memory against the pathogen. Furthermore, macrophages infected with N. gonorrhoeae exhibit diminished capacity to stimulate T-cell responses (Escobar et al., 2018). Notably, during macrophage infection, the expression levels of the antibody receptor CD64 fail to elevate, which is hypothesized to attenuate antibody-dependent cellular cytotoxicity (ADCC) responses against the bacterium (Ortiz et al., 2015). Consequently, exploring botanical agents with antimicrobial activity as sources of antimicrobial metabolites—through mechanisms such as disrupting gonococcal adhesion or activating anti-inflammatory pathways to inhibit infection—holds promise for developing novel therapeutic strategies against N. gonorrhoeae.
Coscinium fenestratum [Menispermaceae; C. fenestratum (Gaertn.) Colebr.], traditionally sourced from regions spanning India to Malesia, has been identified for its medicinal properties, particularly in combating bacterial infections. Prominent in vitro experiments have revealed that the stem extract of C. fenestratum possesses robust antigonococcal properties, demonstrating a minimum inhibitory concentration (MIC) of 47.39 μg/mL against the N. gonorrhoeae ATCC 49226 strain. Following these findings, the extract was processed through column chromatography, resulting in the isolation of berberine, a significant metabolite. Subsequent evaluations of berberine confirmed its substantial antigonococcal efficacy, with it exhibiting average MIC values of 13.51 μg/mL against the N. gonorrhoeae ATCC 49226 and 17.66 μg/mL against various clinical isolates. Additionally, an acute toxicity assessment conducted on the crude methanolic extract from the C. fenestratum stem in laboratory mice showed no signs of mortality or adverse symptoms throughout the duration of a 1-week observational period (Chomnawang et al., 2009). The acute toxicity assessment of the methanolic crude extract derived from C. fenestratum stems revealed no mortality or abnormal clinical signs in either control or treatment groups throughout the 7-day observation period, indicating a favorable safety profile for this botanical preparation. In this study, although screening of plants with anti-gonococcal activity was performed, and comparative analyses with reference metabolites preliminarily identified the primary active components in the herbal extract, along with acute toxicity tests in animal models, the potential presence of additional antimicrobial metabolites in the extract remains to be elucidated. Furthermore, the antimicrobial mechanisms, as well as the safe dosage and risks associated with long-term use, require further exploration.
Helichrysum caespititium [Asteraceae; H. caespititium (DC.) Sond.], a botanical drug native to the regions extending from Zimbabwe to South Africa, has garnered attention for its significant pharmacological properties. Earlier research confirmed that its non-polar extract possesses exceptionally strong antimicrobial and antioxidant properties (Mamabolo et al., 2017). Subsequent studies have built upon these findings, underscoring the efficacy of H. caespititium in treating gonorrheal infections and exhibiting antimicrobial, anti-quorum sensing, and antibiofilm capabilities. Notably, one metabolite in particular, 10-methyl-8-(propan-17-ylidene)naphthalen-9-yl)-11-vinyl-14-hydroxyfuran-16-one (CF6), isolated from the chloroform extract of the plant, showed potent activity against N. gonorrhoeae. The MIC of CF6 was determined to be 60 μg/mL against the reference strain N. gonorrhoeae ATCC 49226. The metabolite’s anti-quorum sensing potential is particularly notable, believed to be due to its ability to competitively bind to the receptor proteins of Chromobacterium violaceum, potentially blocking the active binding sites essential for bacterial communication. Furthermore, CF6 effectively inhibited the cell attachment of N. gonorrhoeae. The underlying mechanism of this inhibitory effect may involve CF6 disrupting the cohesiveness of bacterial extracellular polysaccharides, thereby reducing bacterial extracellular polysaccharides structural integrity and impairing cell attachment (Bassey et al., 2021). Thus, this botanical drug and its metabolites hold potential for development as therapeutic agents against N. gonorrhoeae.
Ocimum tenuiflorum [Lamiaceae; O. tenuiflorum L.], the native range of this species is Tropical and Subtropical Asia to W. Pacific. Ocimum tenuiflorum has been traditionally used since ancient times to treat various diseases, including those caused by microorganisms. Bioassay-guided purification of the hexane extract from the leaves of O. tenuiflorum led to the isolation of H12c as the active metabolite. Characterization identified H12c as eugenol, which exhibited a MIC range of 85–256 mg/L. Notably, this study revealed a strong inhibitory effect of eugenol against both common and multidrug-resistant strains of N. gonorrhoeae. Furthermore, the 50% lethal dose (LD50) of H12c was determined to be 2 g/kg body weight in rats, indicating a low toxicity profile (Shokeen et al., 2008). Given its efficacy and low toxicity, eugenol represents a promising candidate for clinical development to address the rising prevalence of resistant N. gonorrhoeae isolates.
Magnolia officinalis [Magnoliaceae; M. officinalis Rehder and E.H.Wilson], a traditional Chinese botanical drug, is derived from the dried bark, root bark, and branch bark of M. officinalis, a plant belonging to the Magnoliaceae family. As documented in the Compendium of Materia Medica (Bencao Gangmu), it is named “Houpo” due to its thick bark and robust wood structure. Honokiol, a key phenolic metabolite extracted from the bark of M. officinalis, exhibits diverse biological activities. Previous studies have demonstrated that honokiol exerts immunomodulatory effects by enhancing interferon production and simultaneously reducing reactive oxygen species (ROS) and pro-inflammatory cytokine levels in macrophages infected with Staphylococcus aureus(Choi et al., 2015). Recent research has provided further insights into its mechanisms of action. Detailed investigations reveal that honokiol reduces the production of hydrogen peroxide (H2O2) and inhibits the phosphorylation of ERK1/2 in macrophages infected with N. gonorrhoeae. Furthermore, honokiol suppresses the expression of the pro-inflammatory mediator interleukin-6 (IL-6) and inducible nitric oxide synthase (iNOS) induced by N. gonorrhoeae through a pathway independent of NLRP3 inflammasome activation (Hua et al., 2024). On the other hand, this study has certain limitations, primarily the absence of an animal study to assess the efficacy of honokiol against N. gonorrhoeae in a living organism. Further research, including in vivo studies, is needed to provide a more comprehensive understanding of honokiol’s potential as a therapeutic agent against N. gonorrhoeae infections (Figure 2) (Table 2).
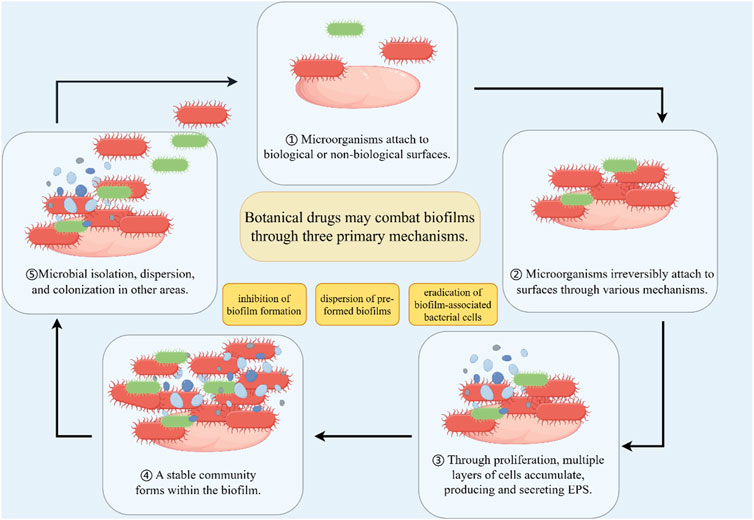
Figure 2. Biofilm and biofilm inhibition. The process of microbial biofilm formation. Microorganisms first reversibly attach to the surface of cells or substances. Subsequently, microbial cells irreversibly colonize through flagella and other mechanisms. A sufficient number of microorganisms form multiple layers of cells and accumulate outside the cells, while producing extracellular polymeric substances (EPS). Form a stable three-dimensional community during the development process. Microorganisms can diffuse from aggregated biofilms and return to a planktonic state.
5 Antibacterial effect of botanical drugs and its metabolites on endogenous pathogens
PID is predominantly caused by ascending infections originating in the vagina or cervix, involving both aerobic bacteria (e.g., S. aureus, E. coli) and anaerobic microorganisms (Eschenbach et al., 1975). The polymicrobial nature of PID, characterized by co-infections involving N. gonorrhoeae, C. trachomatis, and diverse vaginal microbiota, significantly complicates therapeutic interventions due to synergistic pathogen interactions and enhanced antimicrobial tolerance. Furthermore, the escalating prevalence of antimicrobial resistance (AMR), driven by widespread antibiotic misuse, has intensified the urgency to develop complementary therapeutic strategies targeting these recalcitrant infections. Over the past decade, there has been a growing interest in exploring complementary and alternative therapeutic strategies to combat infections caused by these pathogens. Notably, botanical drugs and its metabolites have demonstrated potential in effectively controlling infections caused by endogenous pathogens, including drug-resistant bacteria. This is achieved through mechanisms such as the inhibition of biofilm formation, inhibition of efflux pumps, suppression of quorum sensing (QS), and prevention of plasmid transfer (Table 3).
5.1 Inhibits efflux pumps
Efflux pumps in the cytoplasmic membrane are vital transport proteins that expel various substrates from the cytoplasm, as described by Hersch and colleagues (Hersch et al., 2020). These pumps are crucial for ejecting antimicrobial agents from bacterial cells, lowering their intracellular levels and promoting antibiotic resistance development. In S. aureus, the multidrug efflux pump proteins QacA, NorA and Smr are well-known (Foster, 2016; Jang, 2016). Research indicates the operation of multiple active efflux systems in MRSA, including QacB, QacJ and Smr, which significantly contribute to its multidrug resistance (Xiao Neng et al., 2007) (Figure 3).
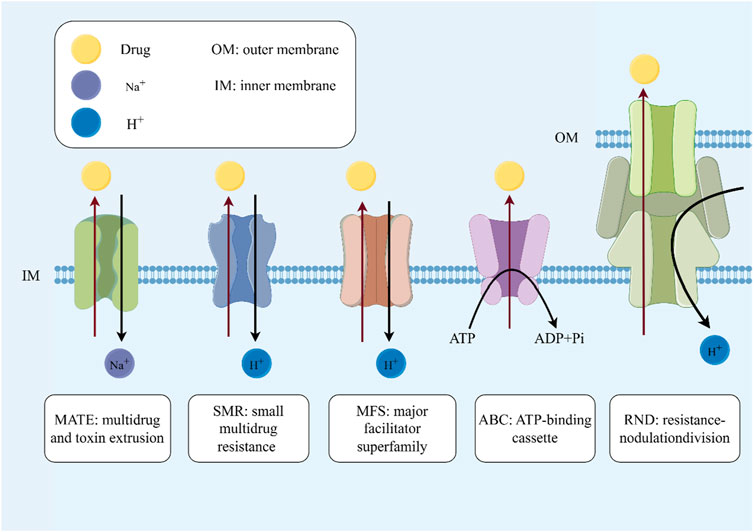
Figure 3. Inhibits outflow pumps. The mechanism of efflux pumps: the five efflux pumps shown in the figure are important mechanisms for bacteria to develop drug resistance. They can transport substrates while expelling drugs or other substances that are not conducive to bacterial growth, thereby increasing drug resistance. It is worth noting that the ABC pump is an efflux pump system that relies on ATP hydrolysis. The energy provided by ATP hydrolysis is used to eliminate various substances from the cell, including antibiotics, metabolic waste, etc.
Zanthoxylum nitidum [Rutaceae; Z. nitidum (Roxb.) DC.], belonging to the family Rutaceae and known for its traditional medicinal uses in China for detoxification, pain relief, and blood stasis, contains 6-acetonyl-dihydrofagaridine. This novel coumarin, extracted from the plant’s roots, has shown effectiveness in reducing the MIC by impeding drug efflux in combination with ampicillin (Zeng et al., 2022). However, this study mainly focused on evaluating their anti-MRSA activity, while lacking an in-depth, systematic exploration of specific antibacterial mechanisms, such as effects on bacterial cell membranes, cell walls, or the regulation of related gene expression.
Another notable, 20(S)-Rh2 from Panax ginseng [Araliaceae; P. ginseng C.A.Mey. ], has exhibited potential in augmenting antibiotic effects by targeting the NorA efflux pump in S. aureus, enhancing the intracellular presence of ciprofloxacin (CIP) and thus its antibacterial properties (Zhang et al., 2014). Although studies have hypothesized 20(S)-Rh2 as a potential inhibitor of NorA pumps, there may be a lack of direct molecular-level evidence, such as specific structural binding data, protein expression levels, or in-depth mechanistic studies such as gene regulation.
From the Chilean Huilliche medicinal repertoire, Persea lingue [Lauraceae; P. lingue (Ruiz and Pav.) Nees], also from the Lauraceae family, has been studied for its content of kaempferol-3-O-α-L-(2,4-bis-E-p-coumaroyl)rhamnoside. In enriched everted membrane vesicles of E. coli, this metabolite inhibits the NorA efflux pump, significantly boosting the effectiveness of ciprofloxacin against strains overexpressing NorA (Holler et al., 2012). Although this study has identified inhibitory activity against NorA pumps, the specific molecular mechanism of action has not been explored in depth.
From the Brazilian Cerrado, Fridericia platyphylla [Bignoniaceae; F. platyphylla (Cham.) L.G.Lohmann] yields chalcones that impact bacterial resistance by binding to NorA and MepA efflux pumps at sites also targeted by norfloxacin. This binding inhibits the efflux activity, suggesting a promising role for these metabolites in combination therapies aimed at NorA and MepA-mediated resistance in S. aureus (de Sousa Andrade et al., 2020). However, this study did not provide data on the pharmacokinetics (e.g., absorption, distribution, metabolism, excretion) and safety (toxicology) of extracts and isolated botanical metabolites.
5.2 Inhibits quorum sensing
Quorum sensing (QS) is a crucial bacterial communication mechanism that regulates collective behaviors such as virulence factor secretion and biofilm formation. This process includes the synthesis, release, and detection of specific signaling molecules on a population-wide scale (Kim et al., 2017). Quorum sensing inhibitors (QSIs), which can reduce bacterial pathogenicity and enhance antibiotic effectiveness while minimizing resistance risks, have been studied extensively (Saeki et al., 2020; Naga et al., 2023) (Figure 4). Natural metabolites from botanical drugs are increasingly recognized as potent sources for QSI development.
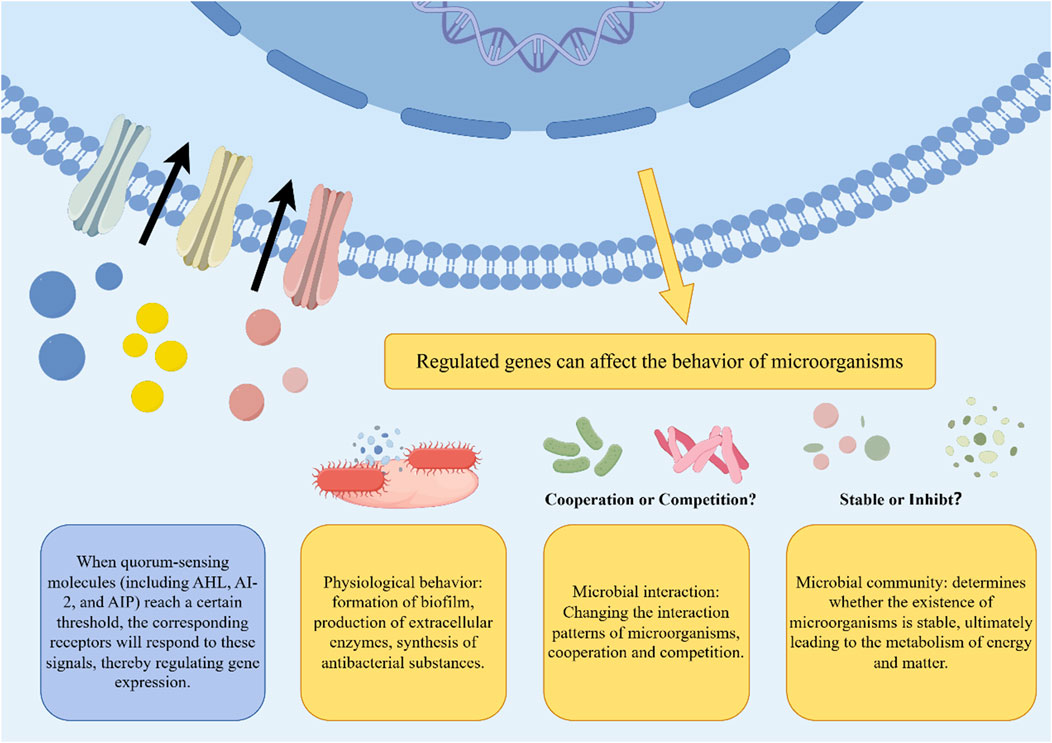
Figure 4. The impact of quorum sensing on microorganisms. Some quorum sensing molecules exist in the extracellular environment. After reaching a certain threshold, the relevant receptors will react to it by regulating genes, ultimately affecting physiological behaviors such as biofilm formation, extracellular enzyme production, and synthesis of antibacterial substances. This will cause the microbial community to change the current balance, and competition or cooperation is uncertain.
In Northern Nigeria, the annual botanical drug Polydora serratuloides [P. serratuloides (DC.) H. Rob.] is traditionally utilized. Isolation of keto-hirsutinolides from its leaves has demonstrated their activity as QSIs within a concentration range of 0.33–5.25 mg/mL-1, indicating their potential in novel antibiotic development (Aliyu et al., 2021). Ongoing studies are needed to explore how these metabolites affect bacterial biofilm formation and the specific pathways through which they modulate the QS system.
The traditional Chinese botanical drug Campsis grandiflora [Bignoniaceae; C. grandiflora (Thunb.) K. Schum.], also known as “Lingxiaohua”, is noted for its properties in clearing heat and cooling blood as recorded in the Tang Materia Medica (Tang Bencao). Recent research has shown that the ethanol extract of C. grandiflora flowers (CFEE) inhibits violacein production in C. violaceum 026 and reduces swarming in E. coli K-12 and Pseudomonas aeruginosa PAO1 in a dose-dependent fashion. Malic acid and succinic acid were identified as effective AI-1 QSIs in these studies, playing key roles in the anti-quorum-sensing activities of CFEE (Zhang et al., 2020). Future investigations should focus on the molecular interactions and specific targets of malic and succinic acids in quorum sensing inhibition.
5.3 Inhibits of plasmid transfer
Plasmids play a pivotal role in the dissemination of antibiotic resistance and serve as a key mechanism by which pathogenic strains acquire resistance to antibiotics (de Toro et al., 2014). The activity and horizontal transmission of resistance plasmids markedly increase the prevalence of resistance determinants within bacterial populations, thereby accelerating the emergence and spread of multidrug-resistant bacteria (Mc Carlie et al., 2020). Plasmids play a pivotal role in the dissemination of antibiotic resistance and serve as a key mechanism by which pathogenic strains acquire resistance to antibiotics (de Toro et al., 2014). The activity and horizontal transmission of resistance plasmids markedly increase the prevalence of resistance determinants within bacterial populations, thereby accelerating the emergence and spread of multidrug-resistant bacteria (Mc Carlie et al., 2020) (Figure 5).
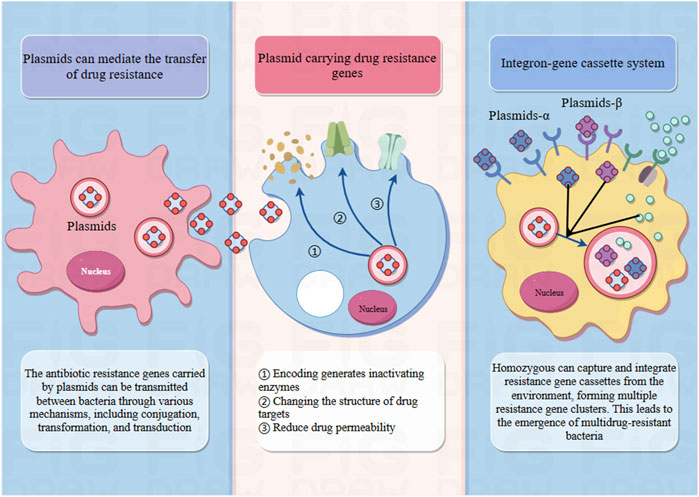
Figure 5. The role of plasmids in drug resistance. Plasmids are scattered DNA fragments in pathogens that do not belong to the nucleus and can perform coding work. Some plasmids carry resistance genes that can encode the production of inactivating enzymes, alter the structure of drug targets, and reduce drug permeability. And the antibiotic resistance genes carried by these plasmids can spread between bacteria through various mechanisms, including conjugation, transformation, and transduction, leading to the transmission of drug resistance. In addition, the integrin gene box system can also be used. Homozygous individuals can capture and integrate resistance gene cassettes from the environment, forming multiple clusters of resistance genes. This has led to the emergence of multidrug-resistant bacteria.
Mallotus philippensis [Euphorbiaceae; M. philippensis (Lam.) Müll.Arg.], a medicinal plant originating from Asia and Australia, is renowned for its diverse therapeutic applications. Research has revealed that the chloroform extract from the leaves of M. philippensis contains potent bioactive metabolites, specifically rottlerin [5,7-dihydroxy-2,2-dimethyl-6-(2,4,6-trihydroxy-3-methyl-5-acetylbenzyl)-8-cinnamoyl-1,2-chromene] and a notable red metabolite [8-cinnamoyl-5,7-dihydroxy-2,2,6-trimethylchromene]. These metabolites have been validated for their significant antibacterial activities, particularly effective against a spectrum of clinically important Gram-positive bacteria, including MRSA. Research further demonstrates that at a concentration of 100 mg/L, both rottlerin and the red metabolite ssuccessfully inhibited the conjugal transfer of resistance plasmids such as pKM101, TP114, pUB307, and R6K among strains of E. coli (Oyedemi et al., 2016). This indicates the potential of these extracts not only as antibacterial agents but also as inhibitors of horizontal gene transfer, which is crucial for preventing the spread of antibiotic resistance. The study underscored the value of these extracts in significantly reducing the dissemination of resistance genes, thereby offering a novel approach to enhance the efficacy of existing antibiotics and mitigate the challenges posed by resistant bacterial strains.
6 Synergy with antibiotics
The synergistic effects of combining botanicals with antibiotics have been demonstrated in numerous studies (An et al., 2011; Zuo et al., 2014), particularly in the context of traditional Chinese Medicine (TCM) (Zuo et al., 2012). TCM has been practiced for thousands of years and has proven effective in treating a wide range of infectious diseases. When used in combination with antibiotics, botanicals have been shown to enhance therapeutic efficacy and reduce the development of resistance against pathogens associated with PID (Table 4).
Indigofera suffruticosa [Fabaceae; I. suffruticosa Mill.], indigenous to the Antilles and Central America and widely known as “anileira” or “anil,” is traditionally utilized both for indigo dye extraction and various medicinal purposes (Barros and Teixeira, 2008). Its diverse pharmacological benefits, including anti-inflammatory (Chen et al., 2013), anti-convulsant (Almeida et al., 2013) and wound-healing properties (Luiz-Ferreira et al., 2011), have been substantiated through scientific research. Further investigations have demonstrated the antimicrobial prowess of I. suffruticosa, particularly via its organic extracts. A study utilizing ether, chloroform, and acetone as solvents revealed significant antimicrobial activities against various strains of S. aureus, including MRSA (Bezerra dos Santos et al., 2015). Notably, all organic extracts displayed inhibition zones exceeding 30.0 mm against MRSA strains, with diethyl ether extract achieving the highest inhibition at 30.08 ± 2.69 mm. Despite this, solvent-specific differences in effectiveness were not statistically significant (p > 0.05). MIC and most potent bactericidal concentration (MBC) for these extracts varied, with ranges from 0.78 to 6.25 mg/mL and 3.12–25.0 mg/mL, respectively. Among these, the acetone extract demonstrated the lowest MIC and MBC values, outperforming ether and chloroform extracts. Moreover, the acetone extract exhibited both bacteriostatic and bactericidal effects, while the others were primarily bactericidal, possibly due to a higher flavonoid content in the acetone extract. When tested in combination with erythromycin, the acetone extract showed varying interactions, ranging from synergistic to non-interactive based on the ratio of drug to extract. Similarly, the chloroform extract displayed synergistic and additive effects in most combinations, with one showing non-interaction. Ether extract combinations mainly showed additive effects. A significant correlation was observed in the interactions of erythromycin with acetone and chloroform extracts. The organic extracts of I. suffruticosa represent promising natural candidates for novel anti S. aureus formulations, owing to their antimicrobial activity against MRSA strains and the synergistic potential observed when combined with erythromycin. However, the underlying mechanisms of action require further investigation. Additionally, this synergistic effect necessitates validation through in vivo studies, while the safe dosage and therapeutic efficacy of these extracts for PID treatment must be confirmed via well-designed clinical trials.
The genus Chimonanthus includes rare and endangered plants native to Anhui Province, often found growing in roadside or sparse plateau forests at elevations of 150–400 m. Classified as a dual-use resource for medicine and food (Cheng et al., 2007), Chimonanthus salicifolius [Calycanthaceae; C. salicifolius S.Y.Hu] are noted in the Xinhua Compendium of Materia Medica for their uses in aromatization, clearing heat, detoxification, and preventing colds and influenza (Medicine, 1975). Pharmacological studies have highlighted the plant’s anti-inflammatory, anti-hyperglycemic, anti-cancer and anti-microbial properties (Chen et al., 2017; Sun et al., 2017). While research on this genus remains limited, reports confirm its notable antimicrobial activity (Wu et al., 2017). A preliminary investigation into the interaction between the ethanolic extract of C. salicifolius and antibiotics demonstrated promising antimicrobial effects. Specifically, the leaf extract exhibited resistance against foodborne pathogens (Wang et al., 2018a). Further studies explored the synergistic effects of the C. salicifolius ethanolic extract combined with streptomycin (Wang et al., 2020). The mixture of ethanol extract and streptomycin effectively inhibited S. aureus and E. coli. Mechanistically, the SE extract enhances antimicrobial action by disrupting cell membranes, leading to efflux of nucleic acids, phosphorus, and cytoplasmic contents, while also interfering with total protein synthesis. These findings suggest the potential of C. salicifolius as a complementary therapeutic agent in antimicrobial treatments. It seemed that the plant could be developed as antibiotic regulators and the product preventing drug-resistant bacteria. The antimicrobial signaling pathways, toxicological data and require to be further explored.
Monoon longifolium [Annonaceae; M. longifolium (Sonn.) B. Xue and R.M.K.Saunders] is utilized in traditional medicinal practices across regions from Zimbabwe to South Africa, specifically for treating fever, skin conditions, diabetes, hypertension, and helminthiasis (Wang et al., 2018b). Recent systematic reviews have confirmed the potent antimicrobial effects of both aqueous and methanolic extracts from the leaves of M. longifolium (Chanda and Nair, 2010; Faizi et al., 2008). The extracts have pronounced antibacterial efficacy, particularly against S. aureus, where MICs have reached as low as 0.039 mg/mL. They also exhibit moderate effectiveness against E. coli, with MIC values observed at 5 and 10 mg/mL (Savu et al., 2022). Additionally, the MBCs for the extracts against S. aureus were recorded at 0.078 mg/mL and 0.156 mg/mL for PlLm and PlLe extracts, respectively. These extracts have also been tested against a clinical MRSA isolate, demonstrating strong antibacterial activity with MIC values of 0.312 and 0.624 mg/mL, and an MBC of 10 mg/mL. Regarding antifungal capabilities, the lowest minimum inhibitory or fungicidal concentration (MIC/MFC) noted was 5 mg/mL for various extracts of M. longifolium. Moreover, when these extracts were combined with penicillin, there was a notable enhancement in antibacterial activity, reducing MIC values by 33–66 times. Monoon longifolium leaf extracts may serve as a promising source of metabolites for developing novel antimicrobial agents to combat antibiotic resistance. However, comprehensive evaluations—including toxicological profiling, preclinical safety assessments, and clinical trials—are imperative to validate their therapeutic potential and ensure translational applicability.
7 Limitations
While this review underscores the antimicrobial potential of botanical drugs and their bioactive metabolites against pathogens such as N. gonorrhoeae and C. trachomatis—positioning them as promising candidates for PID therapy—the majority of cited evidence derives from in vitro assays or preclinical animal models. Notably, some studies fail to elucidate critical pharmacological parameters, including safety profiles, effective dosage ranges, or potential adverse effects associated with long-term administration. Furthermore, current clinical applications of these botanicals often rely on anecdotal empirical practices rather than robust clinical trial data, necessitating rigorous validation through standardized interventional studies to establish optimized therapeutic regimens. Additionally, although antibacterial mechanisms such as efflux pump inhibition and membrane disruption are discussed, the molecular pathways underpinning these actions remain poorly characterized. A significant limitation arises from the predominant focus on single bacterial strains (e.g., S. aureus isolates or limited drug-resistant variants), with minimal exploration of clinically relevant, multidrug-resistant pathogens. Consequently, the generalizability and translational relevance of these findings to complex human infections remain constrained.
8 Future prospects
Botanical drugs, as a vast biochemical reservoir, present tremendous potential for further exploration and development. Future research should prioritize clinical trials to rigorously evaluate the efficacy and safety of botanical drugs and their metabolites in the treatment of PID. Specifically focusing on improving inflammatory markers, relieving patients’ pain, increasing the negative conversion rate of exogenous pathogens associated with PID, alleviating chronic pelvic pain, and evaluating safety indicators. In addition, investigations into the synergistic mechanisms between botanical drugs and antibiotics are necessary to optimize combination ratios. Toxicity assessments and pharmacokinetic studies for such combination therapies should also be undertaken. Furthermore, developing innovative drug formulations is a critical avenue for future research. The integration of nanotechnology or other advanced drug delivery systems could significantly improve the bioavailability and target specificity of botanical drugs, thereby facilitating their translation into clinical practice.
9 In conclusion
Botanical drugs and their metabolites have been used to treat infectious diseases since ancient times, serving as a rich reservoir of chemical diversity and antimicrobial activity that continues to influence modern medicine. Over the past decades, numerous studies have highlighted the antimicrobial properties of botanical drugs and their metabolites, partially elucidating their underlying mechanisms, including biofilm inhibition, quorum sensing disruption, efflux pump suppression, and plasmid transfer blockade. These findings underscore the considerable promise of botanical drugs and their metabolites as therapeutic options for PID, offering novel possibilities for antimicrobial treatments.
Despite extensive documentation of the antimicrobial properties of botanical drugs through in vitro and in vivo studies, clinical research in this area remains limited, constraining their translational potential for practical application. One significant barrier is the insufficient efficacy concentration of plant-derived metabolites. Many metabolites used in TCM contain bioactive substances at relatively low concentrations, which may not be effective in clinical settings. This limitation is particularly pronounced when crude plant extracts are used, where the concentration of metabolites often falls below the therapeutic threshold required for optimal antimicrobial activity.
Additionally, the development of innovative drug delivery technologies, such as nanoparticles, liposomes, or plant-based exosomes, could offer potential solutions to these challenges. Plant exosomes, for example, have emerged as a promising delivery system for plant-derived metabolites. These exosomes can encapsulate bioactive molecules and facilitate their transport across biological barriers, thereby improving the stability and bioavailability of the metabolites. The use of plant exosomes could enable more targeted and sustained release of metabolites, thereby enhancing their therapeutic efficacy and reducing side effects.
In conclusion, botanical drugs and their metabolites represent innovative therapeutic strategies for treating PID while also contributing to efforts addressing the global challenge of antibiotic resistance. Although challenges remain, their remarkable potential underscores the need for continued exploration and development.
Author contributions
H-ZZ: Conceptualization, Data curation, Investigation, Methodology, Supervision, Validation, Writing–original draft. P-JY: Conceptualization, Data curation, Investigation, Methodology, Writing–original draft. Q-FG: Data curation, Investigation, Software, Writing–original draft. JW: Data curation, Writing–original draft. X-LJ: Conceptualization, Methodology, Supervision, Writing–review and editing. S-BW: Conceptualization, Methodology, Resources, Supervision, Writing–review and editing.
Funding
The author(s) declare that financial support was received for the research and/or publication of this article. The work were supported by the National Natural Science Foundation of China (No. 82174431), the Guidelines for the clinical application of integrated traditional Chinese and Western medicine in the treatment of pelvic inflammatory diseases (Project No: SATCM-2015-BZ402-56) and Sichuan Provincial Natural Science Foundation (2023NSFSC0619).
Conflict of interest
The authors declare that the research was conducted in the absence of any commercial or financial relationships that could be construed as a potential conflict of interest.
Generative AI statement
The author(s) declare that no Generative AI was used in the creation of this manuscript.
Publisher’s note
All claims expressed in this article are solely those of the authors and do not necessarily represent those of their affiliated organizations, or those of the publisher, the editors and the reviewers. Any product that may be evaluated in this article, or claim that may be made by its manufacturer, is not guaranteed or endorsed by the publisher.
Supplementary material
The Supplementary Material for this article can be found online at: https://www.frontiersin.org/articles/10.3389/fphar.2025.1545917/full#supplementary-material
References
Abdelrahman, Y. M., and Belland, R. J. (2005). The chlamydial developmental cycle. FEMS Microbiol. Rev. 29, 949–959. doi:10.1016/j.femsre.2005.03.002
Aliyu, A. B., Koorbanally, N. A., Moodley, B., and Chenia, H. Y. (2021). Sesquiterpene lactones from Polydora serratuloides and their quorum sensing inhibitory activity. Nat. Prod. Res. 35, 4517–4523. doi:10.1080/14786419.2020.1739037
Almeida, E. D., Chaves, T. M., Luna, R. D. A., Silva, A. D., AragãO-Neto, A. C., Silva, L. D., et al. (2013). Anticonvulsant effect of Indigofera suffruticosa Mill: indication of involvement of the GABAergic system. Afr. J. Pharm. Pharmacol. 7, 622–628. doi:10.5897/ajpp12.1262
An, J., Zuo, G. Y., Hao, X. Y., Wang, G. C., and Li, Z. S. (2011). Antibacterial and synergy of a flavanonol rhamnoside with antibiotics against clinical isolates of methicillin-resistant Staphylococcus aureus (MRSA). Phytomedicine 18, 990–993. doi:10.1016/j.phymed.2011.02.013
Barros, G. M. C. C., and Teixeira, S. D. P. (2008). Estudo farmacobotânico de duas espécies de Anileira (Indigofera suffruticosa e Indigofera truxillensis, Leguminosae) com propriedades farmacológicas. Rev. Bras. Farmacogn. 18, 287–294. doi:10.1590/s0102-695x2008000200024
Bassey, K., Mamabolo, P., and Cosa, S. (2021). An Andrographolide from Helichrysum caespitium (DC.) Sond. Ex harv., (asteraceae) and its antimicrobial, antiquorum sensing, and antibiofilm potentials. Biol. (Basel) 10, 1224. doi:10.3390/biology10121224
Belland, R. J., Zhong, G., Crane, D. D., Hogan, D., Sturdevant, D., Sharma, J., et al. (2003). Genomic transcriptional profiling of the developmental cycle of Chlamydia trachomatis. Proc. Natl. Acad. Sci. U. S. A. 100, 8478–8483. doi:10.1073/pnas.1331135100
Bezerra Dos Santos, A. T., AraúJO, T. F. D. S., Nascimento Da Silva, L. C., Silva, C. B. D., Oliveira, A. F. M. D., AraúJO, J. M., et al. (2015). Organic extracts from Indigofera suffruticosa leaves have antimicrobial and synergic actions with erythromycin against Staphylococcus aureus. Front. Microbiol. 6, 13. doi:10.3389/fmicb.2015.00013
Bhat, K. S., Gibbs, C. P., Barrera, O., Morrison, S. G., JäHNIG, F., Stern, A., et al. (1991). The opacity proteins of Neisseria gonorrhoeae strain MS11 are encoded by a family of 11 complete genes. Mol. Microbiol. 5, 1889–1901. doi:10.1111/j.1365-2958.1991.tb00813.x
Bhattacharyya, S., Darby, R. R., Raibagkar, P., Gonzalez Castro, L. N., and Berkowitz, A. L. (2016). Antibiotic-associated encephalopathy. Neurology 86, 963–971. doi:10.1212/WNL.0000000000002455
Brunham, R. C., Gottlieb, S. L., and Paavonen, J. (2015). Pelvic inflammatory disease. N. Engl. J. Med. 372, 2039–2048. doi:10.1056/NEJMra1411426
Brunham, R. C., and Rey-Ladino, J. (2005). Immunology of Chlamydia infection: implications for a Chlamydia trachomatis vaccine. Nat. Rev. Immunol. 5, 149–161. doi:10.1038/nri1551
Chanda, S., and Nair, R. (2010). Antimicrobial activity of Polyalthia longifolia (Sonn.) Thw. var. pendula leaf extracts against 91 clinically important pathogenic microbial strains. Chin. Med. 1, 31–38. doi:10.4236/cm.2010.12006
Chen, T. Y., Sun, H. L., Yao, H. T., Lii, C. K., Chen, H. W., Chen, P. Y., et al. (2013). Suppressive effects of Indigofera suffruticosa Mill extracts on lipopolysaccharide-induced inflammatory responses in murine RAW 264.7 macrophages. Food Chem. Toxicol. 55, 257–264. doi:10.1016/j.fct.2012.12.056
Cheng, W. L., Liu, N. X., Hua, J. W., and Zhuge, H. (2007). “A preliminary study on the standard operating procedure (SOP) for the standardized production of Willow Leaf Wax Melon,” in Research and practice on Chinese medicines, 3–6.
Chen, H., Ouyang, K., Jiang, Y., Yang, Z., Hu, W., Xiong, L., et al. (2017). Constituent analysis of the ethanol extracts of Chimonanthus nitens Oliv. leaves and their inhibitory effect on α-glucosidase activity. Int. J. Biol. Macromol. 98, 829–836. doi:10.1016/j.ijbiomac.2017.02.044
Choi, E. J., Kim, H. I., Kim, J. A., Jun, S. Y., Kang, S. H., Park, D. J., et al. (2015). The herbal-derived honokiol and magnolol enhances immune response to infection with methicillin-sensitive Staphylococcus aureus (MSSA) and methicillin-resistant S. aureus (MRSA). Appl. Microbiol. Biotechnol. 99, 4387–4396. doi:10.1007/s00253-015-6382-y
Chomnawang, M. T., Trinapakul, C., and Gritsanapan, W. (2009). In vitro antigonococcal activity of Coscinium fenestratum stem extract. J. Ethnopharmacol. 122, 445–449. doi:10.1016/j.jep.2009.01.036
Cock, H. R. (2015). Drug-induced status epilepticus. Epilepsy Behav. 49, 76–82. doi:10.1016/j.yebeh.2015.04.034
Darville, T. (2021). Pelvic inflammatory disease due to Neisseria gonorrhoeae and Chlamydia trachomatis: immune evasion mechanisms and pathogenic disease pathways. J. Infect. Dis. 224, S39–s46. doi:10.1093/infdis/jiab031
Davies, B., Turner, K. M. E., FrøLUND, M., Ward, H., May, M. T., Rasmussen, S., et al. (2016). Risk of reproductive complications following chlamydia testing: a population-based retrospective cohort study in Denmark. Lancet Infect. Dis. 16, 1057–1064. doi:10.1016/S1473-3099(16)30092-5
DE Sousa Andrade, L. M., DE Oliveira, A. B. M., Leal, A., DE AlcâNTARA Oliveira, F. A., Portela, A. L., DE Sousa Lima Neto, J., et al. (2020). Antimicrobial activity and inhibition of the NorA efflux pump of Staphylococcus aureus by extract and isolated compounds from Arrabidaea brachypoda. Microb. Pathog. 140, 103935. doi:10.1016/j.micpath.2019.103935
DE Toro, M., GarcilláON-Barcia, M. P., and DE LA Cruz, F. (2014). Plasmid diversity and adaptation analyzed by massive sequencing of Escherichia coli plasmids. Microbiol. Spectr. 2. doi:10.1128/microbiolspec.PLAS-0031-2014
Dushime, R., Zhu, Y., Wu, H., Saez, D., Shukla, K., Brown-Harding, H., et al. (2020). Discovery of spilanthol endoperoxide as a redox natural compound active against mammalian Prx3 and Chlamydia trachomatis infection. Antioxidants 9, 1220. doi:10.3390/antiox9121220
Edwards, J. L., and Apicella, M. A. (2004). The molecular mechanisms used by Neisseria gonorrhoeae to initiate infection differ between men and women. Clin. Microbiol. Rev. 17, 965–981. doi:10.1128/CMR.17.4.965-981.2004
Eschenbach, D. A., Buchanan, T. M., Pollock, H. M., Forsyth, P. S., Alexander, E. R., Lin, J. S., et al. (1975). Polymicrobial etiology of acute pelvic inflammatory disease. N. Engl. J. Med. 293, 166–171. doi:10.1056/NEJM197507242930403
Escobar, A., Rodas, P. I., and AcuñA-Castillo, C. (2018). Macrophage–Neisseria gonorrhoeae interactions: a better understanding of pathogen mechanisms of immunomodulation. Front. Immunol. 9, 3044. doi:10.3389/fimmu.2018.03044
Eyre, D. W., Town, K., Street, T., Barker, L., Sanderson, N., Cole, M. J., et al. (2019). Detection in the United Kingdom of the Neisseria gonorrhoeae FC428 clone, with ceftriaxone resistance and intermediate resistance to azithromycin, October to December 2018. Euro Surveill. 24, 1900147. doi:10.2807/1560-7917.ES.2019.24.10.1900147
Faizi, S., Khan, R. A., Mughal, N. R., Malik, M. S., Sajjadi, K. E., and Ahmad, A. (2008). Antimicrobial activity of various parts of Polyalthia longifolia var. pendula: isolation of active principles from the leaves and the berries. Phytother. Res. 22, 907–912. doi:10.1002/ptr.2414
Foster, T. J. (2016). The remarkably multifunctional fibronectin binding proteins of Staphylococcus aureus. Eur. J. Clin. Microbiol. Infect. Dis. 35, 1923–1931. doi:10.1007/s10096-016-2763-0
Garvin, L. E., Bash, M. C., Keys, C., Warner, D. M., Ram, S., Shafer, W. M., et al. (2008). Phenotypic and genotypic analyses of Neisseria gonorrhoeae isolates that express frequently recovered PorB PIA variable region types suggest that certain P1a porin sequences confer a selective advantage for urogenital tract infection. Infect. Immun. 76, 3700–3709. doi:10.1128/IAI.00265-08
Grill, M. F., and Maganti, R. K. (2011). Neurotoxic effects associated with antibiotic use: management considerations. Br. J. Clin. Pharmacol. 72, 381–393. doi:10.1111/j.1365-2125.2011.03991.x
Haggerty, C. L., Gottlieb, S. L., Taylor, B. D., Low, N., Xu, F., and Ness, R. B. (2010). Risk of sequelae after Chlamydia trachomatis genital infection in women. J. Infect. Dis. 201 (Suppl. 2), S134–S155. doi:10.1086/652395
Haggerty, C. L., Totten, P. A., Tang, G., Astete, S. G., Ferris, M. J., Norori, J., et al. (2016). Identification of novel microbes associated with pelvic inflammatory disease and infertility. Sex. Transm. Infect. 92, 441–446. doi:10.1136/sextrans-2015-052285
Hao, H., Aixia, Y., Dan, L., Lei, F., Nancai, Y., and Wen, S. (2009). Baicalin suppresses expression of Chlamydia protease-like activity factor in Hep-2 cells infected by Chlamydia trachomatis. Fitoterapia 80, 448–452. doi:10.1016/j.fitote.2009.06.004
Hao, H., Lei, F., and Yan Dong, Y. (2011). Baicalin downregulates expression of TLR2 and TLR4 gene in chlamydia trachomatis-infected mice. Chin. J. Clin. Pharmacol. Ther. 16, 606–610.
Hao, H., Li Li, T., Han Ju, H., and Chun, W. E. I. S. (2013). Baicalin inhibits downregulation of MHCⅠand CD1d expression in Chlamydia trachomatisinfected cells. Chin. J. Hosp. Pharm. 33, 1750–1754. doi:10.13286/j.cnki.chinhosppharmacyj.2013.21.006
Hebb, J. K., Cohen, C. R., Astete, S. G., Bukusi, E. A., and Totten, P. A. (2004). Detection of novel organisms associated with salpingitis, by use of 16S rDNA polymerase chain reaction. J. Infect. Dis. 190, 2109–2120. doi:10.1086/425929
Hersch, S. J., Watanabe, N., Stietz, M. S., Manera, K., Kamal, F., Burkinshaw, B., et al. (2020). Envelope stress responses defend against type six secretion system attacks independently of immunity proteins. Nat. Microbiol. 5, 706–714. doi:10.1038/s41564-020-0672-6
Hillier, S. L., Kiviat, N. B., Hawes, S. E., Hasselquist, M. B., Hanssen, P. W., Eschenbach, D. A., et al. (1996). Role of bacterial vaginosis-associated microorganisms in endometritis. Am. J. Obstet. Gynecol. 175, 435–441. doi:10.1016/s0002-9378(96)70158-8
Hoenderboom, B. M., VAN Oeffelen, A. A., VAN Benthem, B. H., VAN Bergen, J. E., Dukers-Muijrers, N. H., GöTZ, H. M., et al. (2017). The Netherlands Chlamydia cohort study (NECCST) protocol to assess the risk of late complications following Chlamydia trachomatis infection in women. BMC Infect. Dis. 17, 264. doi:10.1186/s12879-017-2376-y
Holler, J. G., Christensen, S. B., Slotved, H. C., Rasmussen, H. B., GúZMAN, A., Olsen, C. E., et al. (2012). Novel inhibitory activity of the Staphylococcus aureus NorA efflux pump by a kaempferol rhamnoside isolated from Persea lingue Nees. J. Antimicrob. Chemother. 67, 1138–1144. doi:10.1093/jac/dks005
Horton, D. B., Scott, F. I., Haynes, K., Putt, M. E., Rose, C. D., Lewis, J. D., et al. (2015). Antibiotic exposure and juvenile idiopathic arthritis: a case-control study. Pediatrics 136, e333–e343. doi:10.1542/peds.2015-0036
Hua, K. F., Hsu, H. T., Huang, M. S., Chiu, H. W., Wong, W. T., Peng, C. H., et al. (2024). Honokiol exhibits anti-NLRP3 inflammasome and antimicrobial properties in Neisseria gonorrhoeae-infected macrophages. J. Inflamm. Res. 17, 3499–3513. doi:10.2147/JIR.S454221
Hua, Z., Frohlich, K. M., Zhang, Y., Feng, X., Zhang, J., and Shen, L. (2015). Andrographolide inhibits intracellular Chlamydia trachomatis multiplication and reduces secretion of proinflammatory mediators produced by human epithelial cells. Pathog. Dis. 73, 1–11. doi:10.1093/femspd/ftu022
Jang, S. (2016). Multidrug efflux pumps in Staphylococcus aureus and their clinical implications. J. Microbiol. 54, 1–8. doi:10.1007/s12275-016-5159-z
Jennison, A. V., Whiley, D., Lahra, M. M., Graham, R. M., Cole, M. J., Hughes, G., et al. (2019). Genetic relatedness of ceftriaxone-resistant and high-level azithromycin resistant Neisseria gonorrhoeae cases, United Kingdom and Australia, February to April 2018. Euro Surveill. 24, 1900118. doi:10.2807/1560-7917.ES.2019.24.8.1900118
Keshvani, N., Gupta, A., and Incze, M. A. (2019). I Am worried about gonorrhea: what do I need to know? JAMA Intern Med. 179, 132. doi:10.1001/jamainternmed.2018.4345
Khare, C. P. (2008). Indian medicinal plants: an illustrated dictionary. Springer Science and Business Media.
Kim, M. K., Zhao, A., Wang, A., Brown, Z. Z., Muir, T. W., Stone, H. A., et al. (2017). Surface-attached molecules control Staphylococcus aureus quorum sensing and biofilm development. Nat. Microbiol. 2, 17080. doi:10.1038/nmicrobiol.2017.80
Kintner, J., Lajoie, D., Hall, J., Whittimore, J., and Schoborg, R. V. (2014). Commonly prescribed β-lactam antibiotics induce C. trachomatis persistence/stress in culture at physiologically relevant concentrations. Front. Cell Infect. Microbiol. 4, 44. doi:10.3389/fcimb.2014.00044
Lee, J. K., Enciso, G. A., Boassa, D., Chander, C. N., Lou, T. H., Pairawan, S. S., et al. (2018). Replication-dependent size reduction precedes differentiation in Chlamydia trachomatis. Nat. Commun. 9, 45. doi:10.1038/s41467-017-02432-0
Luiz-Ferreira, A., Cola, M., Barbastefano, V., Farias-Silva, E., Calvo, T. R., DE Almeida, A. B., et al. (2011). Indigofera suffruticosa Mill as new source of healing agent: involvement of prostaglandin and mucus and heat shock proteins. J. Ethnopharmacol. 137, 192–198. doi:10.1016/j.jep.2011.05.006
Mamabolo, M. P., Muganza, F. M., and Olivier, M. T. (2017). Free radical scavenging and antibacterial activities of Helichrysum caespititium (DC) harv. Extracts. Biol. Med. 9, 1–7. doi:10.4172/0974-8369.1000417
Mc Carlie, S., Boucher, C. E., and Bragg, R. R. (2020). Molecular basis of bacterial disinfectant resistance. Drug Resist Updat 48, 100672. doi:10.1016/j.drup.2019.100672
Medicine, P. G. F. T. N. C. O. C. H. (1975). National Compendium of Chinese herbal medicine beijing. Beijing, China: People's Health Press.
Mitchell, C. M., Anyalechi, G. E., Cohen, C. R., Haggerty, C. L., Manhart, L. E., and Hillier, S. L. (2021). Etiology and diagnosis of pelvic inflammatory disease: looking beyond gonorrhea and Chlamydia. J. Infect. Dis. 224, S29–S35. doi:10.1093/infdis/jiab067
Naga, N. G., EL-Badan, D. E., Ghanem, K. M., and Shaaban, M. I. (2023). It is the time for quorum sensing inhibition as alternative strategy of antimicrobial therapy. Cell Commun. Signal 21, 133. doi:10.1186/s12964-023-01154-9
Ness, R. B., Kip, K. E., Hillier, S. L., Soper, D. E., Stamm, C. A., Sweet, R. L., et al. (2005). A cluster analysis of bacterial vaginosis-associated microflora and pelvic inflammatory disease. Am. J. Epidemiol. 162, 585–590. doi:10.1093/aje/kwi243
Newman, L., Rowley, J., Vander Hoorn, S., Wijesooriya, N. S., Unemo, M., Low, N., et al. (2015). Global estimates of the prevalence and incidence of four curable sexually transmitted infections in 2012 based on systematic review and global reporting. PLoS One 10, e0143304. doi:10.1371/journal.pone.0143304
Oakeshott, P., Kerry, S., Aghaizu, A., Atherton, H., Hay, S., Taylor-Robinson, D., et al. (2010). Randomised controlled trial of screening for Chlamydia trachomatis to prevent pelvic inflammatory disease: the POPI (prevention of pelvic infection) trial. Bmj 340, c1642. doi:10.1136/bmj.c1642
Obiang, C. S., Ngoua Meye Misso, R. L., Ndong Atome, G. R., Ondo, J. P., Obame Engonga, L. C., and Emvo, E. N. (2019). Phytochemical analyses, antimicrobial and antioxidant activities of stem bark extracts of Distemonanthus benthamianus H. Baill. and fruit extracts of Solanum torvum Sw. from Gabon. Asian Pac. J. Trop. Biomed. 9, 209–216. doi:10.4103/2221-1691.259001
Ortiz, M. C., Lefimil, C., Rodas, P. I., Vernal, R., Lopez, M., AcuñA-Castillo, C., et al. (2015). Neisseria gonorrhoeae modulates immunity by polarizing human macrophages to a M2 profile. PLoS One 10, e0130713. doi:10.1371/journal.pone.0130713
Oyedemi, B. O., Shinde, V., Shinde, K., Kakalou, D., Stapleton, P. D., and Gibbons, S. (2016). Novel R-plasmid conjugal transfer inhibitory and antibacterial activities of phenolic compounds from Mallotus philippensis (Lam.) Mull. Arg. J. Glob. Antimicrob. Resist 5, 15–21. doi:10.1016/j.jgar.2016.01.011
Paavonen, J. (2012). Chlamydia trachomatis infections of the female genital tract: state of the art. Ann. Med. 44, 18–28. doi:10.3109/07853890.2010.546365
Petrina, M. A. B., Cosentino, L. A., Wiesenfeld, H. C., Darville, T., and Hillier, S. L. (2019). Susceptibility of endometrial isolates recovered from women with clinical pelvic inflammatory disease or histological endometritis to antimicrobial agents. Anaerobe 56, 61–65. doi:10.1016/j.anaerobe.2019.02.005
Quillin, S. J., and Seifert, H. S. (2018). Neisseria gonorrhoeae host adaptation and pathogenesis. Nat. Rev. Microbiol. 16, 226–240. doi:10.1038/nrmicro.2017.169
Reekie, J., Donovan, B., Guy, R., Hocking, J. S., Kaldor, J. M., Mak, D. B., et al. (2018). Risk of pelvic inflammatory disease in relation to Chlamydia and gonorrhea testing, repeat testing, and positivity: a population-based cohort study. Clin. Infect. Dis. 66, 437–443. doi:10.1093/cid/cix769
Rowley, J., Vander Hoorn, S., Korenromp, E., Low, N., Unemo, M., Abu-Raddad, L. J., et al. (2019). Chlamydia, gonorrhoea, trichomoniasis and syphilis: global prevalence and incidence estimates, 2016. Bull. World Health Organ 97, 548–562p. doi:10.2471/BLT.18.228486
Saeki, E. K., Kobayashi, R. K. T., and Nakazato, G. (2020). Quorum sensing system: target to control the spread of bacterial infections. Microb. Pathog. 142, 104068. doi:10.1016/j.micpath.2020.104068
Savu, M., Simo, M. K., Fopokam, G. X., Olaru, S. M., Cioanca, O., Boyom, F. F., et al. (2022). New insights into the antimicrobial potential of polyalthia longifolia-antibiofilm activity and synergistic effect in combination with penicillin against Staphylococcus aureus. Microorganisms 10, 1943. doi:10.3390/microorganisms10101943
Scott, F. I., Horton, D. B., Mamtani, R., Haynes, K., Goldberg, D. S., Lee, D. Y., et al. (2016). Administration of antibiotics to children before age 2 Years increases risk for childhood obesity. Gastroenterology 151, 120–129. doi:10.1053/j.gastro.2016.03.006
Shokeen, P., Bala, M., Singh, M., and Tandon, V. (2008). In vitro activity of eugenol, an active component from Ocimum sanctum, against multiresistant and susceptible strains of Neisseria gonorrhoeae. Int. J. Antimicrob. Agents 32, 174–179. doi:10.1016/j.ijantimicag.2008.03.018
Smith, K. J., Ness, R. B., Wiesenfeld, H. C., and Roberts, M. S. (2007). Cost-effectiveness of alternative outpatient pelvic inflammatory disease treatment strategies. Sex. Transm. Dis. 34, 960–966. doi:10.1097/olq.0b013e3181161d47
Sun, Q., Zhu, J., Cao, F., and Chen, F. (2017). Anti-inflammatory properties of extracts from Chimonanthus nitens Oliv. leaf. PLoS One 12, e0181094. doi:10.1371/journal.pone.0181094
Sweet, R. L. (1975). Anaerobic infections of the female genital tract. Am. J. Obstet. Gynecol. 122, 891–901. doi:10.1016/0002-9378(75)90736-x
Tacconelli, E., Carrara, E., Savoldi, A., Harbarth, S., Mendelson, M., Monnet, D. L., et al. (2018). Discovery, research, and development of new antibiotics: the WHO priority list of antibiotic-resistant bacteria and tuberculosis. Lancet Infect. Dis. 18, 318–327. doi:10.1016/S1473-3099(17)30753-3
Tedijanto, C., Grad, Y. H., and Lipsitch, M. (2020). Potential impact of outpatient stewardship interventions on antibiotic exposures of common bacterial pathogens. Elife 9, e52307. doi:10.7554/eLife.52307
Turpin, R., Tuddenham, S., He, X., Klebanoff, M. A., Ghanem, K. G., and Brotman, R. M. (2021). Bacterial vaginosis and behavioral factors associated with incident pelvic inflammatory disease in the longitudinal study of vaginal flora. J. Infect. Dis. 224, S137–s144. doi:10.1093/infdis/jiab103
Unemo, M., Seifert, H. S., Hook, E. W., Hawkes, S., Ndowa, F., and Dillon, J. R. (2019). Gonorrhoea. Nat. Rev. Dis. Prim. 5, 79. doi:10.1038/s41572-019-0128-6
Wang, N., Chen, H., Xiong, L., Liu, X., Li, X., An, Q., et al. (2018a). Phytochemical profile of ethanolic extracts of Chimonanthus salicifolius SY Hu. leaves and its antimicrobial and antibiotic-mediating activity. Industrial crops Prod. 125, 328–334. doi:10.1016/j.indcrop.2018.09.021
Wang, N., Liu, X., Li, J., Zhang, Q., Li, X., An, Q., et al. (2020). Antibacterial mechanism of the synergistic combination between streptomycin and alcohol extracts from the Chimonanthus salicifolius S. Y. Hu. leaves. J. Ethnopharmacol. 250, 112467. doi:10.1016/j.jep.2019.112467
Wang, T., Wu, X., Liu, Y., Luo, Y., Li, X., Zhou, Y., et al. (2018b). Chemical constituents from ethanol extract of Polyalthia rumphii branches and their cytotoxicity evaluation. Rev. Bras. Farmacogn. 28, 235–238. doi:10.1016/j.bjp.2018.03.004
Wiesenfeld, H. C., Meyn, L. A., Darville, T., Macio, I. S., and Hillier, S. L. (2021). A randomized controlled trial of ceftriaxone and doxycycline, with or without metronidazole, for the treatment of acute pelvic inflammatory disease. Clin. Infect. Dis. 72, 1181–1189. doi:10.1093/cid/ciaa101
Workowski, K. A., Bachmann, L. H., Chan, P. A., Johnston, C. M., Muzny, C. A., Park, I., et al. (2021). Sexually transmitted infections treatment guidelines, 2021. MMWR Recomm. Rep. 70, 1–187. doi:10.15585/mmwr.rr7004a1
Wu, Y. X., Wang, X., Jiang, H. T., Ding, K. J., Zhao, C. L., and Hu, C. Y. (2017). Study on total polyphenol content and antioxidant, antimicrobial activities of different polarity fractions of ChimonanthussalicifolicusHu leaves. Food and Mach. 33, 150–154. doi:10.13652/j.issn.1003-5788.2017.08.033
Xiao Neng, M., Li, J. G., Tang, Y. C., Zhang, Y., Zhu, J. X., Tan, S. Q., et al. (2007). The action of active effiux system on multi-drug resistance in methicillin resistant Staphylococcus aureus. Chin. J. Tuberc. Respir. Dis. 30, 40–43. doi:10.3760/j:issn:1001-0939.2007.01.010
Xiong, C. Y., Yang, Y. M., Zhou, Y., He, T. S., Luo, X. W., Wang, J., et al. (2024). Systematic analysis of the adverse effects of commonly used clinical tetracycline drugs based on the FAERS database. Expert Opin. Drug Saf., 1–9. doi:10.1080/14740338.2024.2392865
Zeng, Q., Wang, Z. J., Chen, S., Wang, H., Xie, T. Z., Xu, X. J., et al. (2022). Phytochemical and anti-MRSA constituents of Zanthoxylum nitidum. Biomed. Pharmacother. 148, 112758. doi:10.1016/j.biopha.2022.112758
Zhang, J., Sun, Y., Wang, Y., Lu, M., He, J., Liu, J., et al. (2014). Non-antibiotic agent ginsenoside 20(S)-Rh2 enhanced the antibacterial effects of ciprofloxacin in vitro and in vivo as a potential NorA inhibitor. Eur. J. Pharmacol. 740, 277–284. doi:10.1016/j.ejphar.2014.07.020
Zhang, J., Xu, F., Yao, L., Wang, L., Wang, M., and Wang, G. (2020). Ethanol extract of Campsis grandiflora flower and its organic acid components have inhibitory effects on autoinducer type 1 quorum sensing. Molecules 25, 4727. doi:10.3390/molecules25204727
Zuo, G. Y., Han, Z. Q., Hao, X. Y., Han, J., Li, Z. S., and Wang, G. C. (2014). Synergy of aminoglycoside antibiotics by 3-Benzylchroman derivatives from the Chinese drug Caesalpinia sappan against clinical methicillin-resistant Staphylococcus aureus (MRSA). Phytomedicine 21, 936–941. doi:10.1016/j.phymed.2014.03.004
Keywords: antimicrobial, Chlamydia trachomatis, mechanism, Neisseria gonorrhoeae, pelvic inflammatory disease
Citation: Zhong H-Z, Yan P-J, Gao Q-F, Wu J, Ji X-L and Wei S-B (2025) Therapeutic potential of botanical drugs and their metabolites in the treatment of pelvic inflammatory disease. Front. Pharmacol. 16:1545917. doi: 10.3389/fphar.2025.1545917
Received: 16 December 2024; Accepted: 21 March 2025;
Published: 10 April 2025.
Edited by:
Shanshan Guo, China Academy of Chinese Medical Sciences, ChinaReviewed by:
Yuxiang Fei, China Pharmaceutical University, ChinaKiranmai Mandava, St.Pauls College of Pharmacy, India
Copyright © 2025 Zhong, Yan, Gao, Wu, Ji and Wei. This is an open-access article distributed under the terms of the Creative Commons Attribution License (CC BY). The use, distribution or reproduction in other forums is permitted, provided the original author(s) and the copyright owner(s) are credited and that the original publication in this journal is cited, in accordance with accepted academic practice. No use, distribution or reproduction is permitted which does not comply with these terms.
*Correspondence: Shao-Bin Wei, d2Vpc2hhb2JpbjU2MjBAMTYzLmNvbQ==; Xiao-Li Ji, aml4aWFvbGkxMDMwQHlhaG9vLmNvbQ==
†These authors have contributed equally to this work and share first authorship