- 1Department of Pharmacy, The Third Affiliated Hospital of Southern Medical University, Guangzhou, Guangdong, China
- 2School of Pharmaceutical Sciences, Southern Medical University, Guangzhou, Guangdong, China
- 3Department of Rheumatology and Immunology, The Third Affiliated Hospital of Southern Medical University, Guangzhou, Guangdong, China
Introduction: Hydroxychloroquine (HCQ) has been demonstrated to be potential to enhance the therapeutic efficacy of methotrexate (MTX) for rheumatoid arthritis (RA) patients. However, the pharmacokinetics (PK) alterations and underlying mechanisms differentiating MTX-HCQ combination therapy from MTX monotherapy remain uncharted.
Methods: Thirty-three Sprague-Dawley rats were divided into single-dose and multiple-dose groups, with each group further randomized into an MTX monotherapy group an Hydroxychloroquine monotherapy group (HTG), and an MTX-HCQ combination therapy group Blood samples were collected at various time points before and after dosing to determine drug concentrations in plasma and red blood cells (RBC). The area under the concentration-time curve (AUC) for each compound was calculated, and pharmacokinetics models were established to analyze parameter variations across groups.
Results: In the single-dose group, the CTG exhibited a significant increase in the RBC MTX Cmax compared to the MTG (P = 0.023), whereas the AUC of RBC MTX showed an increasing trend (P = 0.056). In the multiple-dose group, the CTG demonstrated significant increases in plasma MTX Cmax and AUC (P = 0.023, P = 0.028, respectively) as well as RBC MTX Cmax and AUC (P = 0.010, P = 0.003, respectively). The RBC MTX polyglutamates (MTXPG2 and MTXPG3) also showed an increasing trend in Cmax and AUC for the CTG. Additionally, the CTG displayed a significant reduction in clearance rate (CLe) (P = 0.001). No significant differences were observed in the Cmax or AUC of HCQ or desethylhydroxychloroquine (DHCQ) in plasma or RBC across dosing groups.
Conclusion: These findings provide insights into the enhanced efficacy, faster onset, and prolonged effect of MTX-HCQ combination therapy compared to MTX monotherapy. The observed increases in MTX Cmax and AUC suggest the need for careful monitoring of MTX-related adverse effects, particularly in patients with renal insufficiency, during combination treatment with HCQ.
1 Introduction
Rheumatoid arthritis (RA) is a prevalent systemic autoimmune disease characterized by inflammatory responses, chronic synovitis, and bone destruction. Its primary clinical manifestations include progressive joint damage, dysfunction, deformity, and, ultimately, the potential for disability (Combe et al., 2017; Kong and Wen, 2019; Smolen et al., 2016). Methotrexate (MTX) serves as the cornerstone medication for managing RA, achieving treatment objectives related to disease control and symptom alleviation by inhibiting key enzymes within the folate pathway (Friedman and Cronstein, 2019; Visser & Van Der Heijde, 2009). However, in clinical practice, MTX exhibits a slow onset of action, requiring 1–3 months for therapeutic effects to materialize, and there are notable individual variances in its efficacy (Salliot & Van Der Heijde, 2009). Specifically, 30%–40% of patients demonstrate an inadequate response, with some also experiencing adverse reactions (Ebina et al., 2019; Huang et al., 2020; Huerta-García et al., 2021). Although the underlying reasons for these disparities remain to be elucidated, variability in the pharmacokinetics (PK) of MTX and its metabolite, methotrexate polyglutamates (MTXPGs), at the target site concentrations may contribute to the differences in both efficacy and adverse effects.
Hydroxychloroquine (HCQ), a 4-aminoquinoline antimalarial agent, exhibits anti-inflammatory and immunomodulatory properties. Research has shown that HCQ can increase the plasma concentration of MTX, thereby enhancing its bioavailability. The concurrent administration of HCQ and MTX can accelerate the time to onset, improve early-stage efficacy, and maximize the therapeutic benefits of treatment (Carmichael et al., 2002; Schapink et al., 2019). MTX is predominantly eliminated by the kidneys within 24 h, rendering plasma trough concentrations of MTX generally less informative.
Red blood cells (RBC) provide easily accessible specimens, and elevated concentrations of RBC MTXPGs correlate with reduced disease activity (Van De Meeberg et al., 2023). Consequently, RBC MTXPGs serve as reliable biomarkers for assessing treatment efficacy (Takahashi et al., 2017). In the early phases of the study, high-performance liquid chromatography (HPLC) methods were established to quantify the concentrations of various test substances in plasma and RBC, including MTX in plasma, MTXPGs in RBC, and HCQ along with its metabolite desethylhydroxychloroquine (DHCQ) in both plasma and RBC (Gui-jie et al., 2024). Our primary focus is to examine the PK differences among three groups: the MTX monotherapy group (MTG), the HCQ monotherapy group (HTG), and the MTX-HCQ combination therapy group (CTG). This investigation aims to elucidate the interaction between MTX and HCQ in Sprague-Dawley (SD) rats. Additionally, by constructing PK models, we seek to deepen our understanding and analysis of the differences in the processes of drug absorption, distribution, metabolism, and excretion (ADME) in these animal subjects. The ultimate goal is to provide a PK perspective that contributes to the understanding of the accelerated onset and improved efficacy observed with the combination of MTX and HCQ in the clinical management of patients with RA.
2 Materials and methods
2.1 Drugs and compounds
Methotrexate tablets were sourced from SPH Sine Pharmaceutical Laboratories Co., Ltd., whereas HCQ sulfate tablets were obtained from SPH Zhongxi Pharmaceutical Co., Ltd. Both MTX (purity 99.8%) and HCQ (purity 99.8%) were acquired from the National Institutes for Food and Drug Control of China. The following compounds were purchased from Schircks Laboratories in Switzerland: 4-amino-10-methylpteroyldiglutamic acid (MTXPG2, purity 97%), 4-amino-10-methylpteroyltriglutamic acid (MTXPG3, purity 97%), 4-amino-10-methylpteroyltetraglutamic acid (MTXPG4, purity 97%), and 4-amino-10-methylpteroylpentaglutamic acid (MTXPG5, purity 97%). DHCQ was obtained from Panphy Chemical Corporation, and H2O2 (3%) was procured from Hebei Jianning Pharmaceutical Co., Ltd.
2.2 Instruments
The study utilized an Ultimate 3,000 dual ternary HPLC system, equipped with dual ternary gradient pumps, an auxiliary pump, an automatic sampler, a column oven, a diode array detector, and a fluorescence detector. The photochemical reactions were carried out using the PHRE-15 reactor, which features a 254 nm low-pressure mercury ultraviolet lamp and contains 2 m of 1/16-inch (o.d.) Teflon FEP tubing (0.25 mm i.d.), arranged as a knitted coil.
2.3 Animals
Male SD rats, weighing between 160 and 200 g, were obtained from the Guangdong Medical Laboratory Animal Center. The animals were housed in an environment maintained at a temperature of 25°C ± 1°C and a relative humidity of 60% for a period of 3–7 days. During this acclimatization period, the rats had ad libitum access to water and standard rat food. The project was approved by the Experimental Animal Ethics Committee of Southern Medical University.
2.4 Experimental design
The SD rats were divided into three groups: the blank group, the single-dose group, and the multiple-dose group (over 1 month). Methotrexate tablets were dissolved in a solution of 0.9% NaCl: 5% NaHCO3 (4:1), whereas HCQ tablets were dissolved in 0.9% NaCl. The dosage and interval of administration of the drug in rats are based on the usage and dosage of the drug in adults, and the conversion is based on the equivalent dose between animals and humans (Reagan-Shaw et al., 2008).
2.4.1 Blank group
Two SD rats were maintained under standard feeding conditions to provide blank blood samples.
2.4.2 Single-dose group
A total of fifteen SD rats were randomly assigned to three groups. Each group received intragastric administrations of: MTX at 3.5 mg/kg (MTG, n = 5), HCQ at 40.1 mg/kg (HTG, n = 5), or a combination of MTX at 3.5 mg/kg and HCQ at 40.1 mg/kg (CTG, n = 5).
2.4.3 Multiple-dose group
Eighteen SD rats were again randomly divided into three groups. The groups underwent weekly intragastric administrations of MTX at 3.5 mg/kg (MTG, n = 6), daily administrations of HCQ at 40.1 mg/kg (HTG, n = 6), or a combination of MTX at 3.5 mg/kg weekly with HCQ at 40.1 mg/kg daily (CTG, n = 6). Additionally, the MTG group received an equivalent volume of saline on days when the drug was not administered.
The rats were administered drugs intragastrically as previously described. An approximate volume of 0.5 mL of blood was collected from the orbital venous plexus of each rat prior to and following drug administration. Blood sampling time points were established at 0, 15, 30 min, 1, 3, 6, 10, 24, 48, 72, and 96 h. The collected blood was transferred into heparinized EP tubes. The samples were subsequently centrifuged at 3.0 × 103 rpm min−1 for 5 min to separate plasma and RBC. The RBC were washed twice with three times their volume of cold physiological saline and then stored in a −25°C refrigerator for future analysis.
2.5 Bioanalytical methodology
2.5.1 Detection of plasma MTX, RBC MTX and RBC MTXPG2-5 concentrations
The concentrations were measured utilizing a methodologically validated high-performance liquid chromatography with fluorescence detection (HPLC-FLD) technique.
The sample pretreatment method is as follows: 1) For plasma samples, 100 μL of plasma was mixed with 10 μL of a water/mixed standard solution of each concentration, thoroughly mixed for 10 s, and subsequently 100 μL of a 10% perchloric acid solution was added to precipitate proteins. The mixture was vortexed for 3 min and then centrifuged at 1.2 × 104 rpm min−1 for 10 min at 10°C. A total of 150 μL of the supernatant was transferred to a sample vial for analysis. 2) For RBC samples, 100 μL of freeze-thawed RBC was initially combined with 100 μL of water to reduce viscosity, followed by the addition of 10 μL of a water/mixed standard solution of each concentration. After mixing for 10 s, 200 μL of a 10% perchloric acid solution was added for protein precipitation. This mixture was vortexed for 3 min and centrifuged at 1.2 × 104 rpm min−1 for 10 min at 10°C, after which 300 μL of the supernatant was transferred to a sample vial for analysis.
The chromatographic conditions are as follows: The photochemical reactor was connected online following the analytical column and prior to the fluorometric detector. The analytical column utilized was an Athena C18-WP (250 mm × 4.6 mm, 3 μm), and the SPE column was a C18-WP (20 mm × 4 mm, 5 μm). Mobile phase A1 consisted of 10 mM ammonium acetate (pH adjusted to 9.00 with 25% aqueous ammonia), A2 contained 10 mM ammonium acetate (pH = 7.00), with both A1 and B1 being acetonitrile. A 3% hydrogen peroxide (H2O2) solution was introduced post-column to derivatize the target compounds using an auxiliary pump. A volume of 100 μL was injected for samples processed from plasma, whereas 200 μL was injected for samples processed from RBC. The separation was carried out by a programmed gradient elution scheme. For specific conditions, see Supplementary Table S1-1 in Annex 1.
The lower limits of quantification were determined to be 1.0 nM for MTX in plasma and RBC, and 2.0 nM for MTXPG2 and MTXPG3 in RBC.
2.5.2 Detection of plasma HCQ, plasma DHCQ, RBC HCQ and RBC DHCQ concentrations
The concentrations were measured using a methodologically validated HPLC approach.
The sample pretreatment method is as follows: 1) For the plasma samples, 100 μL of plasma is taken, followed by the addition of 10 μL of a water/mixed standard solution corresponding to each concentration. The mixture is then thoroughly mixed for 10 s, after which 150 μL of a methanol-copper sulfate solution is added to precipitate proteins. This combined solution is vortexed for 3 min and subsequently centrifuged at 12,000 rpm min−1 for 10 min at 10°C. Finally, 150 μL of the supernatant is transferred to a sample vial for analysis. 2) For the RBC samples, 50 μL of freeze-thawed RBC is combined with 10 μL of a water/mixed standard solution of each concentration. The mixture is evenly mixed for 10 s, and then 250 μL of a methanol-copper sulfate solution is added to precipitate proteins. Similar to the plasma samples, the mixed solution is vortexed for 3 min and centrifuged at 12,000 rpm min−1 for 10 min at 10°C. A total of 150 μL of the resulting supernatant is transferred to a sample vial for analysis using an ultraviolet detector.
The chromatographic conditions are as follows: The analytical column employed is the Athena C18-WP (150 mm × 4.6 mm, 5 μm), whereas the SPE column is the C18-WP (20 mm × 4 mm, 5 μm). The mobile phases are defined as follows: A1 consists of a 0.006% aqueous phosphoric acid solution (pH = 3.00), A2 is a 10 mM ammonium acetate solution (pH = 7.00), and both B1 and A1 are composed of acetonitrile. For both the plasma and RBC samples processed, 100 μL is injected for analysis. The separation was carried out by a programmed gradient elution scheme. For specific conditions, see Supplementary Table S1-2 in Annex 1.
The lower limits of quantification were determined to be 15.6 ng/mL for both HCQ and DHCQ in both plasma and RBC. The specificity, standard curve and lower limit of quantification, accuracy, precision, and stability of the method were evaluated in accordance with the Guiding Principles for Verification of Quantitative Analysis Methods of 9,012 Biological Samples of China Pharmacopoeia (2020 edition), with all results meeting the established standards.
2.6 Statistical analysis
The area under the concentration-time curve (AUC) was calculated using the linear trapezoidal method. The calculation equations are as follows: Equations 1, 2. Among them,
The selection of statistical tests (independent samples t-test, Welch’s t-test, or Mann-Whitney U test) was based on the normality distribution of the data and the results of homogeneity of variance testing. Appropriate analytical methods was applied to assess potential differences in the PK parameters of MTX between the MTG and CTG groups. Similarly, this methods was employed to investigate differences in the PK parameters of HCQ between the HTG and CTG groups, with statistical significance determined at p ≤ 0.05.
2.7 PK model establishment
By comparing the goodness of fit of the model, methotrexate selected a two-compartment model (2CM) that incorporates an oral absorption compartment. Additionally, a three-compartment model that includes RBC volume (V3) was employed to describe the conversion of MTX to MTXPG2 and MTXPG3 within RBCs, as illustrated in Figure 1. MTX present in the plasma can penetrate RBCs, forming MTXPGs, specifically MTXPG2 and MTXPG3. These compounds are then metabolized back into MTX, which is ultimately released back into the plasma.
The model equations and initial conditions are such as Equations 3-9.
Aa represents the amount of MTX in the absorption compartment, which is the administered dose. ka is the absorption rate constant. C1, C2, CRBC,MTX are the drug concentrations of MTX in the central compartment (V1), peripheral compartment (V2), and RBC compartment (VRBC), respectively. A1, A2, and ARBC,MTX are the amounts of the drug. CRBC, MTXPG2 and CRBC, MTXPG3 are the concentrations of MTXPG2 and MTXPG3 in RBC, respectively. CLe is the systemic clearance rate. CL1 is the distribution clearance rate between V1 and V2. CLRBC is the distribution clearance rate between V1 and VRBC. k12 is the rate constant for the conversion of MTX to MTXPG2. k21 is the rate constant for the conversion of MTXPG2 to MTX. k13 is the rate constant for the conversion of MTX to MTXPG3. k31 is the rate constant for the conversion of MTXPG3 to MTX.
3 Results
3.1 Concentration-time curve and PK parameters of MTX and MTXPG2-3
The detection method developed in this study effectively identifies MTX and MTXPG2-5 in RBC; however, only MTX and MTXPG2-3 were detectable in rat erythrocytes, while MTXPG4-5 were below the detection limit and could not be detected. Figure 2 illustrates the concentration-time curves of MTX in both plasma and RBC for the single-dose groups (MTG and CTG), whereas the concentration-time curves of MTX, MTXPG2, and MTXPG3 in plasma and RBC from the multiple-dose groups are also displayed in Figure 2. The Cmax of MTX in the single-dose group was higher (MTG: 306.3 nM, CTG: 258.9 nM) compared to that in the multiple-dose group (MTG: 91.5 nM, CTG: 161.1 nM). The metabolism of plasma MTX was rapid in both groups, with concentrations becoming undetectable after 6 and 10 h, respectively (the lower limit of quantification was 1 nM). In the RBC of the single-dose group, only MTX was detectable, with a Cmax reached after 48 h (MTG: 47.8 nM, CTG: 71.1 nM). In contrast, both MTX, MTXPG2, and MTXPG3 concentrations were detectable in the RBC of the multiple-dose group. Drug concentrations in the blood remained relatively stable in the first 24 h, reaching Cmax levels (MTG: MTX: 59.1 nM, MTXPG2: 4.5 nM, MTXPG3: 5.3 nM; CTG: MTX: 98.6 nM, MTXPG2: 5.3 nM, MTXPG3: 7.3 nM). Up to the conclusion of the study at 96 h, the concentrations of MTX, MTXPG2, and MTXPG3 in the RBC of both groups remained detectable, indicating a relatively slow metabolic process.
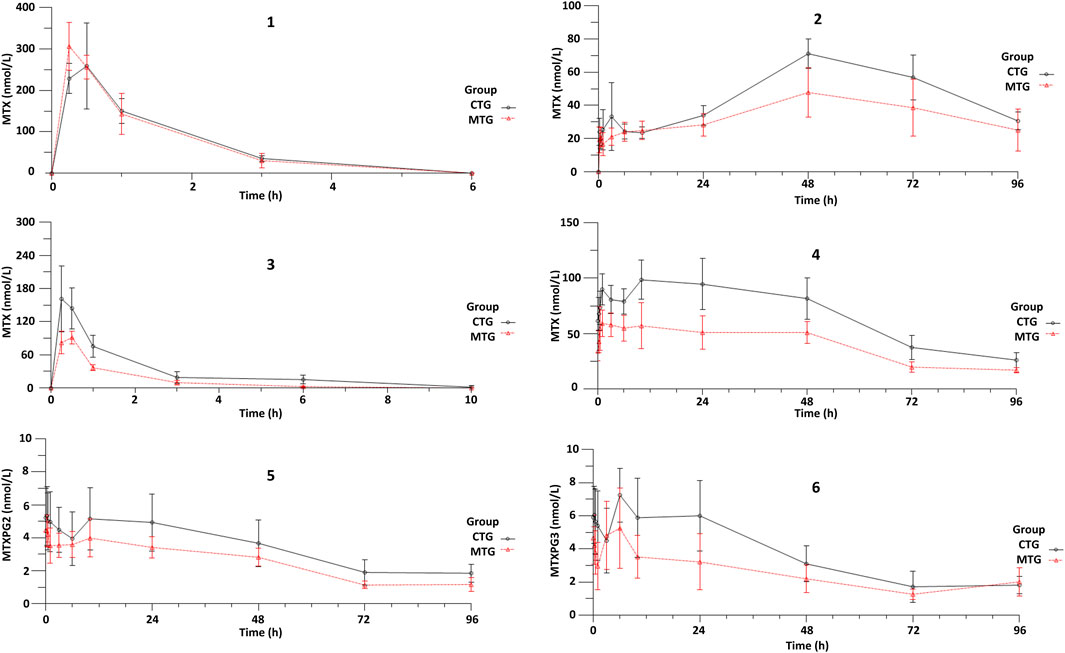
Figure 2. The concentration-time curve of MTX, MTXPG2, and MTXPG3 in Plasma and RBC of the CTG and MTG (1: plasma MTX of single-dose group, 2: RBC MTX of single-dose group, 3: plasma MTX of multiple-dose group, 4: RBC MTX of multiple-dose group, 5: RBC MTXPG2 of multiple-dose group, 6: RBC MTXPG3 of multiple-dose group).
The comparisons of the AUC and PK parameters for each group are presented in Table 1. As illustrated in Figure 2, within the single-dose group, the Cmax of MTX in RBC from the CTG exhibited a significant increase compared to the MTG (P = 0.023), whereas the AUC0-last of MTX in RBC displayed a noticeable increasing trend (P = 0.056). In the multiple-dose group, a significant increase was observed in the Cmax, AUC0-last, and AUC0-
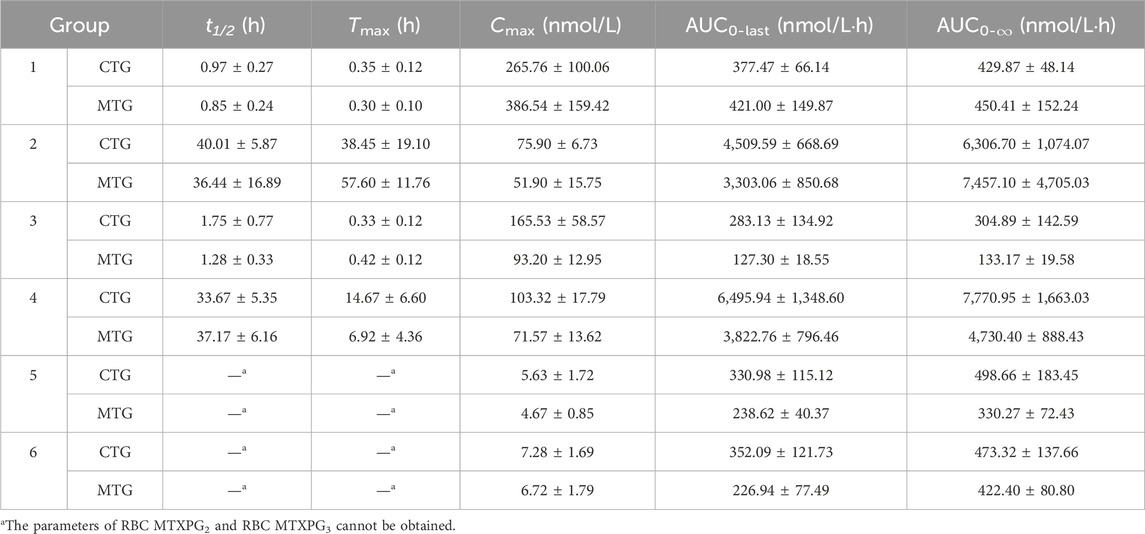
Table 1. The PK parameters of MTX, MTXPG2, and MTXPG3 in Plasma and RBC of the CTG and MTG (1: plasma MTX of single-dose group, 2: RBC MTX of single-dose group, 3: plasma MTX of multiple-dose group, 4: RBC MTX of multiple-dose group, 5: RBC MTXPG2 of multiple-dose group, 6: RBC MTXPG3 of multiple-dose group).
3.2 Concentration-time curve and PK parameters of HCQ and DHCQ
No significant differences were noted in the Cmax, AUC0-last, and AUC0-
3.3 PK model of MTX
Modeling was conducted utilizing the concentration data of MTX, MTXPG2, and MTXPG3 from blood samples of SD rats within each group. A two-compartment model with an absorption compartment was found to provide a better fit for the concentration data of plasma MTX. The physiological RBC volume for healthy SD rats was used as the volume of the third compartment, established at 0.030 L/kg. The PK model parameters for the CTG and MTG in the single-dose group are presented in Table 2. When compared to the MTG, the central compartment V1 for the CTG was significantly increased (P = 0.047). The PK model parameters for the CTG and MTG in the multiple-dose group are provided in Table 3. In comparison to the MTG, the V1 and CLe for the CTG were significantly decreased (P = 0.010, P = 0.001), whereas the V2 was significantly increased (P = 0.016), and the ka showed a tendency to increase (P = 0.074).
4 Discussion
The Cmax and AUC of CTG in plasma and RBC were significantly greater than those of MTG, indicating that HCQ influences the PK behavior of MTX in rats. However, there were no significant differences in the Cmax and AUC of HCQ and its metabolite, DHCQ, in the plasma and RBC of rats under any administration conditions. This suggests that MTX does not impact the metabolism and absorption of HCQ, a finding consistent with the clinical research conducted by Carmichael et al. (2002).
MTX is primarily transported into cells through active uptake mechanisms involving transporters such as the reduced folate carrier 1 (RFC1/SLC19A1) and the organic anion transporting polypeptides (OATPs) (Tang et al., 2024). Once inside the cell, additional glutamate residues are added by folate polyglutamate synthetase (FPGS) to form MTXPGs that accumulate intracellularly. This process can be reversed by γ-glutamyl hydrolase (GGH), which converts MTXPGs back to their monoglutamate form. Subsequently, these monoglutamate MTXPGs are extruded from the cell via efflux transporters, including multidrug resistance protein 1 (MDR1/ABCB1), multidrug resistance-associated protein 1 (MRP1), and breast cancer resistance protein (BCRP) (Stamp et al., 2013). Research indicates that HCQ does not exhibit inhibitory effects on MRP1 and OATPs such as OATP1B1, OATP1B3, and OATP2B1, nor does it stimulate the regulation of the ABCB1 gene. However, HCQ can inhibit ABCB1 and reduce the efflux of MTX when the concentration exceeds 10 μM (Weiss et al., 2020). This is consistent with the notable increase in the Cmax of MTX in RBC of the CTG during single-dose administration, as well as the upward trend in AUC0-last. The significant increases in the Cmax, AUC0-last, and AUC0-
Most of MTX is excreted in its original form, with approximately 75% eliminated through renal tubular secretion. During the process of renal tubular secretion, OATPs and RFC-1 transport MTX from the bloodstream to the basolateral membrane. Subsequently, ABCB1, MRP1, and BCRP transport MTX across the apical membrane into the urine and out of the body (Iwaki et al., 2017). Therefore, the concurrent use of ABCB1 inhibitors may elevate the plasma levels of MTX in both plasma and tissues, potentially enhancing its efficacy as well as toxicity (Liu, 2019). In the context of multiple-dose administration, Cmax, AUC0-last, and AUC0-
Modeling the plasma concentration data of MTX revealed that a two-compartment model with an absorption compartment better captures its variation pattern. RBCs can act as a drug reservoir, influencing the corresponding drug concentration at the target site. By including them as an independent third compartment in the model, the simulation of drug distribution in the body becomes more accurate and aligns more closely with physiological processes. In the case of multiple-dose administration within the CTG, significant decreases in V1 and CLe were noted, along with a substantial increase in V2 and a tendency for ka to rise. The decrease in V1 indicates an increase in the distribution of the drug within the central compartment, whereas the concurrent increase in V2 suggests a decrease in drug distribution in the peripheral compartment. This indicates a significant alteration in drug distribution within the body, characterized by a greater concentration in the central compartment and a reduction in tissue distribution. The notable decrease in CLe implies a reduction in the drug clearance rate, indicating that HCQ may inhibit the body’s ability to eliminate MTX, thereby prolonging its presence in the body. The observed tendency for ka to increase suggests that HCQ may enhance the intestinal absorption of MTX.
In conclusion, the use of combination therapy has enhanced the bioavailability of MTX and increased its exposure in SD rats. These results may explain why the combination of MTX-HCQ in clinical practice results in improved efficacy, a faster onset of action, and a longer duration of continuous effect compared to MTX monotherapy. The alterations in PK parameters of MTX observed in this study are significant for its clinical application. Both single-dose and multiple-dose administrations of MTX in the CTG showed significant increases in Cmax and AUC in the blood. Given that HCQ may inhibit kidney transporters involved in MTX elimination, patients with renal insufficiency may be at greater risk of toxicity due to reduced drug clearance (Wang et al., 2018). Our data suggest that co-administration of HCQ-MTX may exacerbate systemic accumulation in these populations, requiring dose adjustment and rigorous TDM. It is recommended to be alert for adverse reactions during combined therapy with methotrexate and HCQ, and to monitor blood concentrations if necessary, especially in patients with renal insufficiency. Future clinical trials should validate these PK interactions and establish evidence-based dose-adjustment protocols for at-risk populations.
This study elucidates MTX-HCQ pharmacokinetic interactions but has limitations: 1) Healthy rat models differ pathophysiologically from rheumatoid arthritis patients; 2) Tissue distribution (e.g., synovium) and transporter activity were not directly measured; 3) Short-term dosing may underestimate cumulative toxicity; 4) Rodent-human extrapolation requires PBPK/clinical validation. Translational studies integrating human cell models and therapeutic monitoring are needed.
5 Conclusion
In summary, this research provides valuable insights into the clinical benefits associated with combination therapies. The findings may clarify the reasons behind the enhanced efficacy, faster onset of action, and prolonged duration of effect observed with the combination of MTX and HCQ, as compared to monotherapy with MTX alone. Given the significant increases in Cmax and AUC, it is crucial to remain particularly vigilant regarding the adverse reactions of MTX during treatment with HCQ, especially in patients with renal insufficiency. Future research should focus on elucidating the mechanisms underlying the drug-drug interactions identified in this study. Furthermore, exploring the potential impact of combination therapies on patient outcomes, including efficacy, toxicity, and overall survival, would be beneficial.
Data availability statement
The original contributions presented in the study are included in the article/Supplementary Material, further inquiries can be directed to the corresponding author.
Ethics statement
The animal study was approved by Experimental Animal Ethics Committee of Southern Medical University. The study was conducted in accordance with the local legislation and institutional requirements.
Author contributions
GZ: Conceptualization, Data curation, Investigation, Methodology, Software, Visualization, Writing – original draft, Writing – review and editing. RW: Software, Visualization, Writing – original draft. GC: Investigation, Software, Writing – original draft. SL: Data curation, Writing – original draft. HJ: Resources, Writing – review and editing. WC: Investigation, Writing – review and editing. QL: Methodology, Supervision, Writing – review and editing.
Funding
The author(s) declare that no financial support was received for the research and/or publication of this article.
Conflict of interest
The authors declare that the research was conducted in the absence of any commercial or financial relationships that could be construed as a potential conflict of interest.
Generative AI statement
The author(s) declare that no Generative AI was used in the creation of this manuscript.
Publisher’s note
All claims expressed in this article are solely those of the authors and do not necessarily represent those of their affiliated organizations, or those of the publisher, the editors and the reviewers. Any product that may be evaluated in this article, or claim that may be made by its manufacturer, is not guaranteed or endorsed by the publisher.
Supplementary material
The Supplementary Material for this article can be found online at: https://www.frontiersin.org/articles/10.3389/fphar.2025.1561001/full#supplementary-material
References
Carmichael, S. J., Beal, J., Day, R. O., and Tett, S. E. (2002). Combination therapy with methotrexate and hydroxychloroquine for rheumatoid arthritis increases exposure to methotrexate. J. Rheumatol. 29 (10), 2077–2083.
Combe, B., Landewe, R., Daien, C. I., Hua, C., Aletaha, D., Álvaro-Gracia, J. M., et al. (2017). 2016 update of the EULAR recommendations for the management of early arthritis. Ann. Rheum. Dis. 76 (6), 948–959. doi:10.1136/annrheumdis-2016-210602
Ebina, K., Hashimoto, M., Yamamoto, W., Hirano, T., Hara, R., Katayama, M., et al. (2019). Drug tolerability and reasons for discontinuation of seven biologics in elderly patients with rheumatoid arthritis -The ANSWER cohort study. PLoS One 14 (5), e0216624. doi:10.1371/journal.pone.0216624
Friedman, B., and Cronstein, B. (2019). Methotrexate mechanism in treatment of rheumatoid arthritis. Jt. Bone Spine 86 (3), 301–307. doi:10.1016/j.jbspin.2018.07.004
Gui-jie, Z., Ting, L., Hong-yu, J., Wen-ying, C., and Qiang, L. (2024). Determination concentration of methotrexate and its polyglutamates in human erythrocyte by high-performance liquid chromatographic fluorescence method. Chin. J. Clin. Pharmacol. 40 (01), 117–120. doi:10.13699/j.cnki.1001-6821.2024.01.024
Huang, J., Fan, H., Qiu, Q., Liu, K., Lv, S., Li, J., et al. (2020). Are gene polymorphisms related to adverse events of methotrexate in patients with rheumatoid arthritis? A retrospective cohort study based on an updated meta-analysis. Ther. Adv. Chronic Dis. 11, 2040622320916026. doi:10.1177/2040622320916026
Huerta-García, A. P., Rodríguez-Báez, A. S., Medellín-Garibay, S. E., Portales-Pérez, D. P., Martínez-Martínez, M. U., Abud-Mendoza, C., et al. (2021). Methotrexate triglutamate as a determinant of clinical response in Mexican patients with rheumatoid arthritis: pharmacokinetics and dose recommendation. J. Clin. Pharmacol. 61 (8), 1118–1130. doi:10.1002/jcph.1837
Iwaki, M., Shimada, H., Irino, Y., Take, M., and Egashira, S. (2017). Inhibition of methotrexate uptake via organic anion transporters OAT1 and OAT3 by glucuronides of nonsteroidal anti-inflammatory drugs. Biol. Pharm. Bull. 40 (6), 926–931. doi:10.1248/bpb.b16-00970
Kong, X. Y., and Wen, C. P. (2019). On research progress of western and Chinese medicine treatment on pre-rheumatoid arthritis. Chin. J. Integr. Med. 25 (9), 643–647. doi:10.1007/s11655-019-3223-3
Liu, X. (2019). ABC family transporters. Adv. Exp. Med. Biol. 1141, 13–100. doi:10.1007/978-981-13-7647-4_2
Reagan-Shaw, S., Nihal, M., and Ahmad, N. (2008). Dose translation from animal to human studies revisited. Faseb J. 22 (3), 659–661. doi:10.1096/fj.07-9574LSF
Salliot, C., and Van Der Heijde, D. (2009). Long-term safety of methotrexate monotherapy in patients with rheumatoid arthritis: a systematic literature research. Ann. Rheum. Dis. 68 (7), 1100–1104. doi:10.1136/ard.2008.093690
Schapink, L., van den Ende, C. H. M., Gevers, L., van Ede, A. E., and den Broeder, A. A. (2019). The effects of methotrexate and hydroxychloroquine combination therapy vs methotrexate monotherapy in early rheumatoid arthritis patients. Rheumatol. Oxf. 58 (1), 131–134. doi:10.1093/rheumatology/key275
Smolen, J. S., Aletaha, D., and McInnes, I. B. (2016). Rheumatoid arthritis. Lancet 388 (10055), 2023–2038. doi:10.1016/s0140-6736(16)30173-8
Stamp, L. K., Hazlett, J., Highton, J., and Hessian, P. A. (2013). Expression of methotrexate transporters and metabolizing enzymes in rheumatoid synovial tissue. J. Rheumatol. 40 (9), 1519–1522. doi:10.3899/jrheum.130066
Takahashi, C., Kaneko, Y., Okano, Y., Taguchi, H., Oshima, H., Izumi, K., et al. (2017). Association of erythrocyte methotrexate-polyglutamate levels with the efficacy and hepatotoxicity of methotrexate in patients with rheumatoid arthritis: a 76-week prospective study. RMD Open 3 (1), e000363. doi:10.1136/rmdopen-2016-000363
Tang, F., Zou, L., Chen, J., and Meng, F. (2024). Cellular pharmacokinetic of methotrexate and its modulation by folylpolyglutamate synthetase and γ-glutamyl hydrolase in tumor cells. PLoS One 19 (6), e0302663. doi:10.1371/journal.pone.0302663
Van De Meeberg, M. M., Hebing, R. C. F., Nurmohamed, M. T., Fidder, H. H., Heymans, M. W., Bouma, G., et al. (2023). A meta-analysis of methotrexate polyglutamates in relation to efficacy and toxicity of methotrexate in inflammatory arthritis, colitis and dermatitis. Br. J. Clin. Pharmacol. 89 (1), 61–79. doi:10.1111/bcp.15579
Visser, K., and Van Der Heijde, D. (2009). Optimal dosage and route of administration of methotrexate in rheumatoid arthritis: a systematic review of the literature. Ann. Rheum. Dis. 68 (7), 1094–1099. doi:10.1136/ard.2008.092668
Wang, W., Zhou, H., and Liu, L. (2018). Side effects of methotrexate therapy for rheumatoid arthritis: a systematic review. Eur. J. Med. Chem. 158, 502–516. doi:10.1016/j.ejmech.2018.09.027
Keywords: methotrexate, methotrexate polyglutamates, hydroxychloroquine, rheumatoid arthritis, pharmacokinetics
Citation: Zhang G, Wang R, Chen G, Liu S, Jie H, Chen W and Li Q (2025) Hydroxychloroquine increases the exposure of methotrexate in plasma and red blood cells: a pharmacokinetic interaction study in rats in vivo. Front. Pharmacol. 16:1561001. doi: 10.3389/fphar.2025.1561001
Received: 15 January 2025; Accepted: 14 April 2025;
Published: 24 April 2025.
Edited by:
Jiangxin Wang, Shenzhen University, ChinaReviewed by:
Zixi Chen, Shenzhen University, ChinaZheng Yuan, China Academy of Chinese Medical Sciences, China
Chao Li, Shenzhen University, China
Copyright © 2025 Zhang, Wang, Chen, Liu, Jie, Chen and Li. This is an open-access article distributed under the terms of the Creative Commons Attribution License (CC BY). The use, distribution or reproduction in other forums is permitted, provided the original author(s) and the copyright owner(s) are credited and that the original publication in this journal is cited, in accordance with accepted academic practice. No use, distribution or reproduction is permitted which does not comply with these terms.
*Correspondence: Qiang Li, bGlxaWFuZ3NtdUAxNjMuY29t