- 1Department of Pharmacy, Peking Union Medical College Hospital, Chinese Academy of Medical Science and Peking Union Medical College, Beijing, China
- 2State Key Laboratory of Complex Severe and Rare Diseases, Peking Union Medical College Hospital, Chinese Academy of Medical Science and Peking Union Medical College, Beijing, China
- 3Department of Internal Medicine, Peking Union Medical College Hospital, Chinese Academy of Medical Science and Peking Union Medical College, Beijing, China
- 4Department of General Surgery, Peking Union Medical College Hospital, Chinese Academy of Medical Science and Peking Union Medical College, Beijing, China
- 5West China School of Pharmacy, Sichuan University, Chengdu, China
- 6Department of Pharmacy, Shanghai Children’s Medical Center, School of Medicine, Shanghai Jiao Tong University, Shanghai, China
Background: Drug-induced pancreatitis is a rare disease but frequently reported, owing to the vast number of medications.
Aim: To summarize potential drugs causing pancreatitis and to speculate on underlying mechanisms.
Methods: We extracted more than 60,000 reports of pancreatitis submitted to the U.S. Food and Drug Administration Adverse Event Reporting System (January 2004 to March 2023). Data on patient age, sex, weight, time to onset, and outcome (death et al.) were collected. Disproportionality analysis was used in data mining to identify associations between drugs and pancreatitis events. Seven databases, commonly used for network pharmacology analysis, were searched to identify potential targets.
Results: Of 867 drugs with 3 or more reports, 101 drugs met all criteria using disproportionality analysis and indicated a potential risk of pancreatitis. The risk of 40 drugs had not been previously noted in “UpToDate” database. Patients taking the drugs had a similar sex distribution, were mostly 45–64 years old, and were heavier (median, 88 kg; P < 0.0001). The median time to onset was 199 days (interquartile range, 27–731.5). Ponatinib (16.48%), tigecycline (14.12%) and valproic acid (13.41%) had higher fatality rates. Potential targets related to pancreatitis were identified in 50 of the 101 drugs.
Conclusion: Clinicians providing the 101 drugs for treatment should stay vigilant to detect pancreatitis early.
Highlights
• More than 60,000 reports of pancreatitis were suspected for drugs.
• From 867 drugs with reports ≥3, 101 drugs were identified.
• The toxicological mechanisms of 50 of 101 drugs were investigated.
1 Introduction
Pancreatitis is the leading cause of gastrointestinal-related hospitalizations, associated with considerable mortality and socioeconomic burden (Peery et al., 2019). Drug-induced pancreatitis is a rare disease (Simons-Linares et al., 2019), but since the first reports of acute pancreatitis caused by chlorthalidone and cortisone in the 1950s, hundreds of different classes of commonly used drugs have been reported to cause pancreatic damage (Hung and Abreu Lanfranco, 2014). The World Health Organization database lists 2,479 episodes suspected to be caused by 525 different drugs between 1968 and 1993 (Lancashire et al., 2003). The study of drugs that may cause pancreatitis helps clinicians understand the characteristics of this rare disease and identify drug-induced pancreatitis and avoid re-administration of potentially harmful drugs.
Although many drugs have been reported, most evidence for the relationship between drugs and pancreatitis is weak, and drug-induced pancreatitis remains poorly understood (Nitsche et al., 2012). Moreover, the underlying toxicological mechanisms of drug-induced pancreatitis remain little understood (Nitsche et al., 2010; Simons-Linares et al., 2019). Possible mechanisms include direct toxic effects, accumulation of toxic metabolites or intermediates, immune response, and hypersensitivity reactions. To date, researchers have studied the correlation between the following drugs and pancreatitis using the U.S. Food and Drug Administration Adverse Event Reporting System (FAERS) database, but not systematically and comprehensively: protease inhibitors (Qin et al., 2021), tocilizumab (Kamath et al., 2021), sodium glucose co-transporter 2 inhibitors (Frent et al., 2021), glucocorticoids (Nango et al., 2019), blinatumomab (Vakharia et al., 2018), eluxadoline (Gawron and Bielefeldt, 2018; Harinstein et al., 2018; Cash et al., 2021), tocilizumab (Flaig et al., 2016), and atypical antipsychotics (Hauben, 2004). No researchers have yet conducted network pharmacology analysis combined with real-world pharmacovigilance research on drug-induced pancreatitis.
This study was a combination of real-world pharmacovigilance research based on the FAERS database and network pharmacology (Figure 1) and aimed to summarize potential drugs that can cause pancreatitis and to speculate on the underlying mechanism of drug-induced pancreatitis by analyzing reports of pancreatitis associated with drugs, and targets for drugs and pancreatitis.
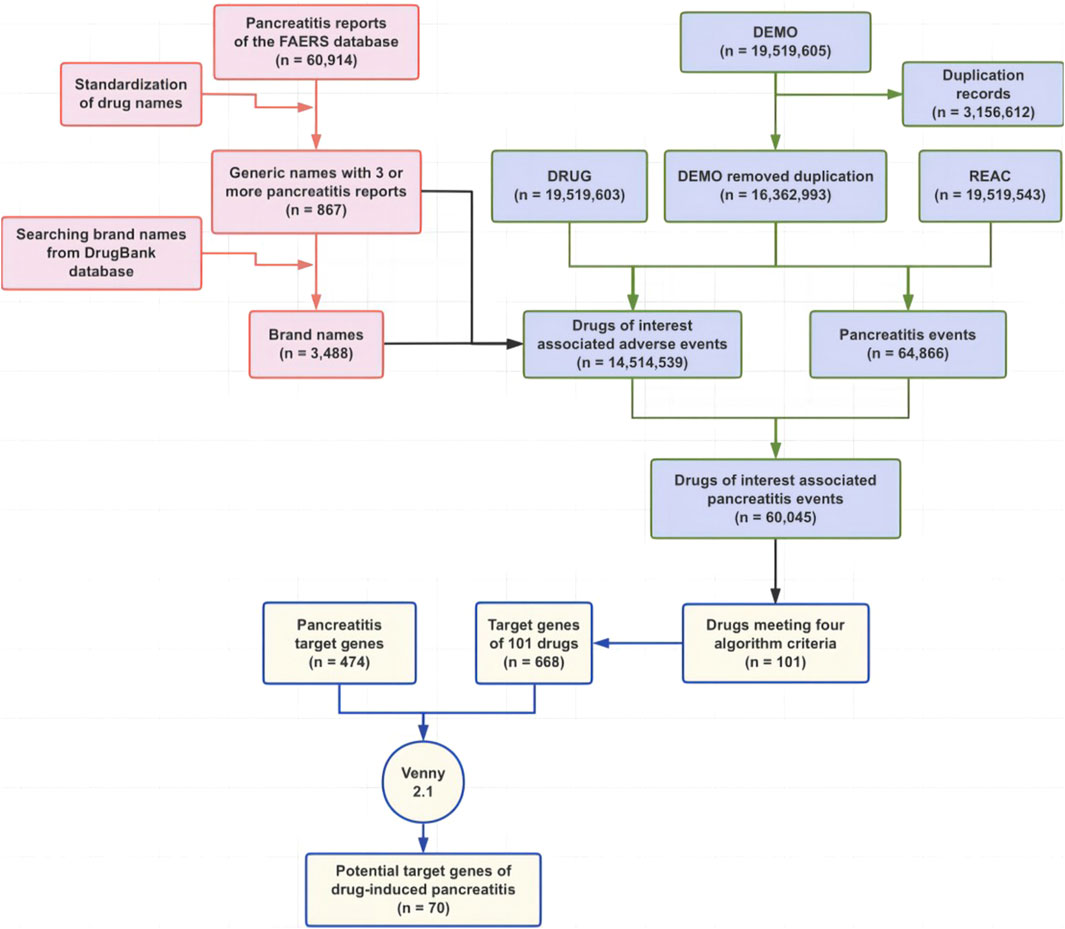
Figure 1. Flowchart of this study. Abbreviations: FAERS, Food and Drug Administration Adverse Event Reporting System.
2 Methods
2.1 Adverse event identification
Pancreatitis was standardized using the preferred term (PT) of the International Conference on Harmonization Medical Dictionary for Regular Activities (version 25.0). The following PTs were selected as pancreatitis: “Pancreatitis (10033645),” “Subacute pancreatitis (10084554),” “Pancreatitis viral (10065192),” “Pancreatitis relapsing (10033657),” “Pancreatitis necrotizing (10033654),” “Pancreatitis haemorrhagic (10033650),” “Pancreatitis fungal (10065190)”, “Pancreatitis chronic (10033649),” “Pancreatitis bacterial (10065191),” “Pancreatitis acute (10033647),” “Oedematous pancreatitis (10052400),” “Lupus pancreatitis (10067750),” “Ischaemic pancreatitis (10066127),” “Immune-mediated pancreatitis (10083072),” “Haemorrhagic necrotic pancreatitis (10076058),” “Cytomegalovirus pancreatitis (10049566),” “Autoimmune pancreatitis (10069002).”
2.2 Drug screening
The FAERS database is a public, voluntary, spontaneous reporting system, containing seven types of datasets. Data deduplication was conducted according to the FDA recommendations (Xie et al., 2025), the latest FDA_DT (date FDA received case) was selected when the CASEIDs (number for identifying a FAERS case) were the same and the higher PRIMARYID (unique number for identifying a FAERS report) was chosen when the CASEID and FDA_DT were the same. We retrieved 60,914 reports of drug-related pancreatitis events from the database (January 2004 to March 2022), using the PTs described above. Drug names in the resulting reports were manually standardized to generic names using the DrugBank database (https://go.drugbank.com), which was used as a dictionary for drug generic names and brand name data mapping.
2.3 Data mining
Reports of pancreatitis events were retrieved from the FAERS database (January 2004 to March 2023) using the generic and brand names as described above. The drugs identified as “primary suspect” in the code for the drug’s reported role in event (ROLE_COD) field of the DRUG files were studied. Based on the basic principles of disproportionality analysis, the reporting odds ratio, proportional reporting ratio, Bayesian confidence propagation neural network, and multi-item gamma Poisson shrinker algorithms were used to investigate the association between the drugs and the pancreatitis event. The equations and criteria for the four algorithms (Candore et al., 2015) are listed in Supplementary Table S1. Data deduplication was conducted according to the U.S. Food and Drug Administration (FDA) recommendations (Bian et al., 2021).
The International Drug Anatomical Therapeutic Chemical (ATC) code was used to classify drugs. The time interval between drug initiation and pancreatitis events (time to onset) and the fatality rate were further analyzed, and the former was defined as the interval between EVENT_DT (adverse event onset date) and START_DT (start date of drug administration). Records with incorrect entries or inputs (EVENT_DT earlier than START_DT) were excluded. The latter was calculated as the number of patients for whom the OUTC_COD (code for the outcome) was DE (death) divided by all the patients for whom the outcome was reported.
2.4 Network pharmacological analysis
Pancreatitis target genes were obtained from the DisGeNET database (score_gda ≥0.1, https://www.disgenet.org) and the GeneCard database (relevance score ≥10, https://www.genecards.org). Target genes of drug were obtained from the DGIdb database (https://www.dgidb.org) and the DrugBank database. The UniProt database (https://www.uniprot.org) was used for standardization of target gene names. Venny 2.1 software (https://bioinfogp.cnb.csic.es/tools/venny/) was used to obtain intersecting target genes. The STRING database (https://cn.string-db.org/cgi/input.pl) was used to analyze the protein-protein interactions (PPI) of the target genes. The DAVID database (https://david.ncifcrf.gov) was used for gene ontology (GO) and Kyoto Encyclopedia of Genes and Genomes (KEGG) pathway enrichment analyses. GO analysis included biological process (BP), cellular component (CC), and molecular function (MF).
2.5 Statistical analysis
Descriptive analyses were performed to summarize the patients’ demographic features. Continuous variables with normal distribution were described as mean ± standard deviation (x ± s). Non-normally distributed continuous variables were expressed as median [interquartile range (IQR)]. Categorical variables were described as counts (n) and percentages (%). Interval days from drug initiation to onset of pancreatitis events for drugs with the same ATC code in the first four levels and weight were compared using a nonparametric test (the Mann–Whitney U test for dichotomous variables and the Kruskal–Wallis test for more than two subgroups of respondents). Pearson’s chi-squared test or Fisher’s exact test was used to compare sex differences and fatality rate among drugs with the same ATC code in the first four levels. In addition, Pearson’s chi-squared test or Fisher’s exact test was also used to compare the fatality rate of specific drugs with the overall fatality rate of all other drugs. P < 0.05 with 95% confidence intervals (CI) was considered statistically significant. Statistical analyses were performed using SPSS (version 16.0; SPSS Inc., Chicago, IL, United States).
3 Results
3.1 Disproportionality analysis
There were 101 drugs that met all four criteria using four algorithms and 40 drugs whose “Drug Information” in the UpToDate database did not mention pancreatitis in “Adverse Reactions” (Table 1). Their ATC codes and proportion of acute pancreatitis and chronic pancreatitis events are shown in Figure 2 and Supplementary Table S2. The 101 drugs included 23 anti-infectives (ten antibacterial, ten antiviral, and three antifungal), 16 hypoglycemic drugs, and 14 antineoplastic drugs. The number of acute pancreatitis was significantly more than that of chronic pancreatitis.
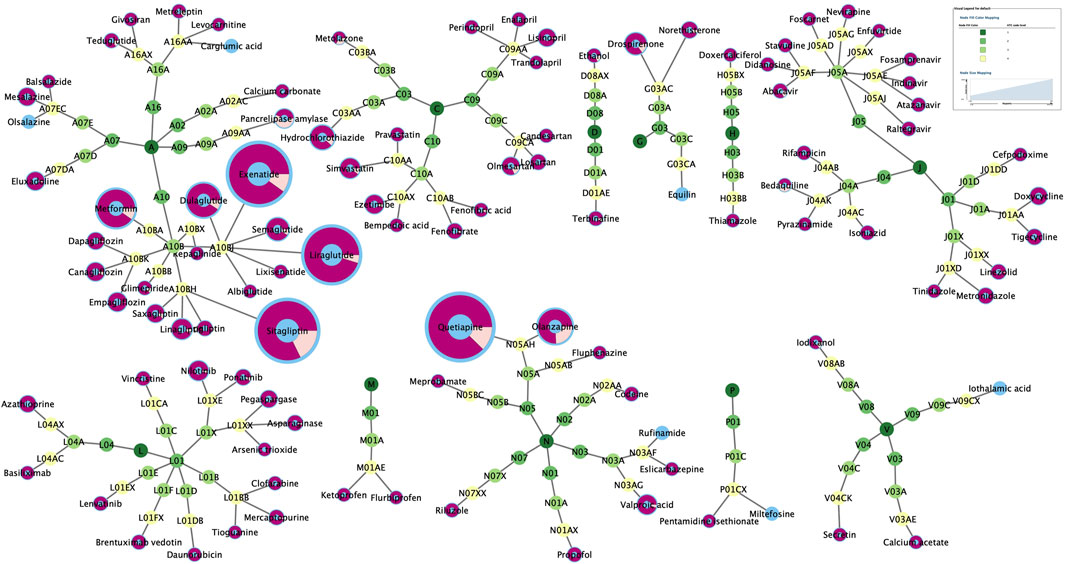
Figure 2. The classification of 101 drugs and proportion of acute pancreatitis (purple) and chronic pancreatitis (pale yellow).
Antipsychotics (quetiapine and olanzapine), glucagon-like peptide-1 (GLP-1) analogs (exenatide, liraglutide, dulaglutide), dipeptidyl peptidase 4 (DPP-4) inhibitors (sitagliptin), and metformin were the most commonly reported (59.43%). Notably, many drugs with few reports but a potential association with pancreatitis were found, for which the risk of pancreatitis had not previously been perceived.
3.2 Overall demographic characteristics
Demographic information was obtained from 67,574 reports of pancreatitis events for 867 drugs (summarized in Table 2). Complete signaling drugs were defined as those that met all four criteria using the four algorithms. Partial signaling drugs met any one to three of the four criteria (Supplementary Table S3). Complete non-signaling drugs did not meet any of the four criteria.
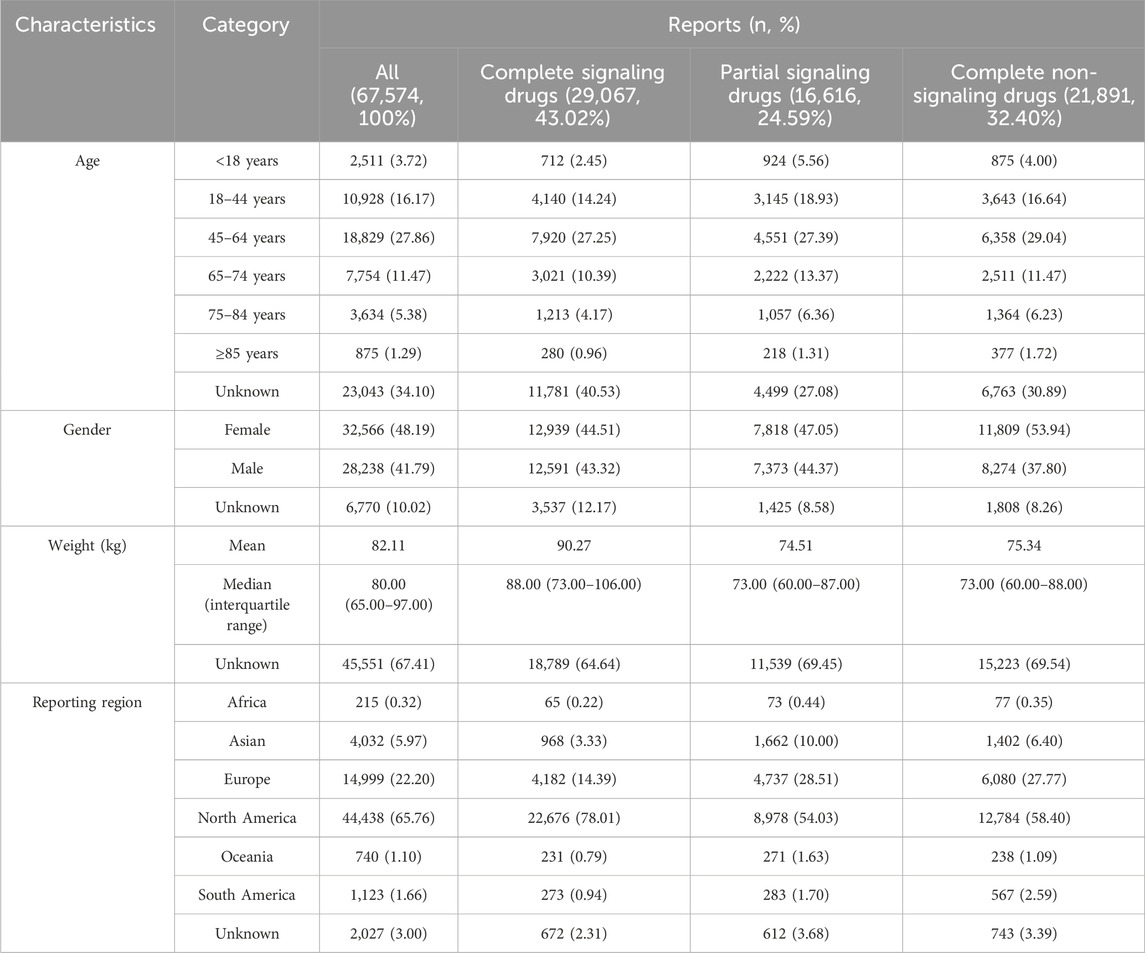
Table 2. Demographic characteristics of patients with drug-associated pancreatitis events sourced from the FAERS database (January 2004 to March 2023).
Excluding reports of unknown age, 66.82% of all pancreatitis events occurred in the 18–64 years age group and only 5.64% occurred in the <18 years age group. Excluding reports of unknown sex, pancreatitis events with signaling drugs (female: complete 50.68%, partial 51.46%) were more evenly distributed between sexes than those with non-signaling drugs (female: 58.80%). Excluding reports of unknown weight, patients with pancreatitis events with complete signaling drugs (median, 88 kg) were heavier (P < 0.0001). More than half of the events occurred in North America, followed by Europe, which may be related to the data sources.
3.3 Demographic characteristics of specific drugs
Detailed demographic information on the 101 signaling drugs is summarized in Supplementary Table S4. Pancreatitis events occurred more in the 0–18 years age group for asparaginase (85/109), carglumic acid (10/11), clofarabine (20/38), and vincristine (39/58). The following drugs were reported in significantly more female patients: doxycycline (174:87), drospirenone (582:3), eluxadoline (258:57), liraglutide (1401:1111), metreleptin (45:5), and quetiapine (1822:1490). These drugs were reported in significantly more male patients (P < 0.0001): alogliptin (34:84), atazanavir (11:40), lisinopril (83:204), sitagliptin (1216:1493), and terbinafine (23:58).
3.4 Time to onset and fatality rate
Complete information on interval days from drug initiation to the onset of pancreatitis was available for 94 of the 101 drugs, and the distribution of days between was right-skewed (Supplementary Table S5). The median was 199 days (IQR: 27–731.5). More than half of the drugs were administered within a median of 3 months (50/94), approximately two-thirds within 6 months (61/94), and most within a year (78/94). Drugs with the same ATC code in the first four levels with significant differences in interval days are shown in Figure 3. The following drugs showed significantly later onset of pancreatitis than similar drugs: sitagliptin, exenatide, drospirenone, mercaptopurine, and quetiapine. Additionally, time to onset of 20 drugs with valid report number ≥100 is summarized in Table 3, showing a clear distinction between different classes of drugs. Quetiapine (823 days), olanzapine (514 days), drospirenone (488 days), valproic acid (437 days), and simvastatin (434 days) are classified as ultra-long latency drugs (median >1 year), suggesting potential associations with the cumulative effects of long-term medication use. GLP-1 analogs: liraglutide (85 days), semaglutide (63 days), dulaglutide (42 days), along with mesalazine (27 days), pegaspargase (23 days), and nilotinib (13.5 days), are categorized as short-latency drugs (median <3 months), indicating possible acute drug reactions. Notably, pegaspargase and nilotinib, as chemotherapeutic/immunomodulatory agents, exhibit ultra-short latency, likely linked to their direct cytotoxic effects. Lisinopril (IQR: 59–2006 days) and valproic acid (IQR: 89–1712 days) demonstrate extremely wide IQR spans, reflecting significant inter-individual variability and suggesting the potential for idiosyncratic reactions in high-risk populations.
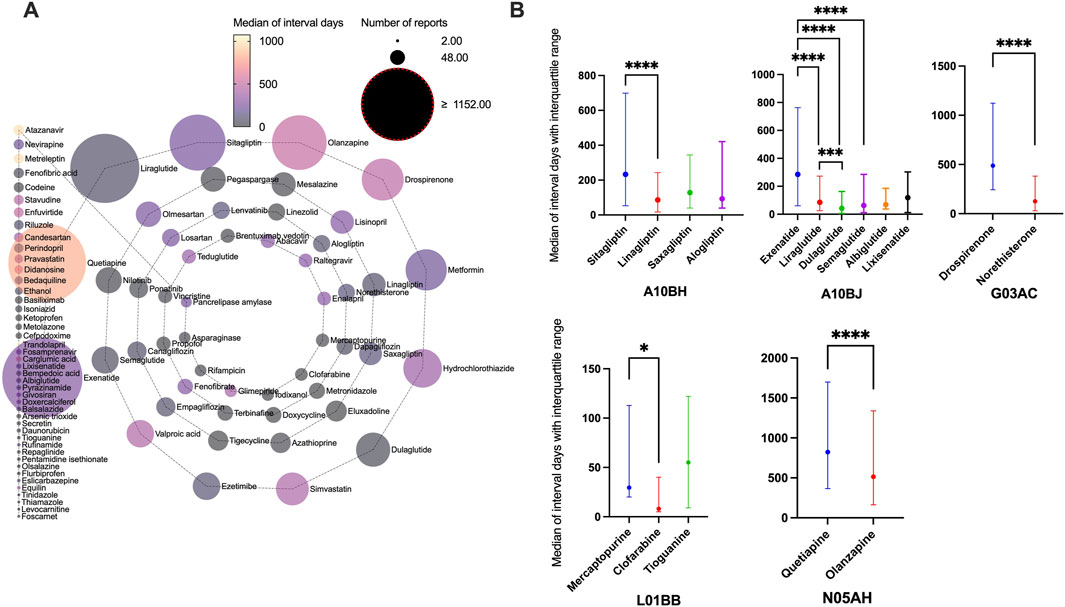
Figure 3. Time interval between drug initiation and pancreatitis event. (A) Median of interval days from drug initiation to onset of pancreatitis event for different drugs. (B) Drugs with the same Anatomical Therapeutic Chemical code in the first four levels with significant differences in interval days.
The outcomes of patients with drug-associated pancreatitis related to these 101 drugs (Supplementary Table S6) included congenital anomaly, death, disability et al. Drugs with the same ATC code in the first four levels, with significant differences between death and non-death, are shown in Figure 4. The following drugs showed significantly higher fatality rates than similar drugs: sitagliptin, exenatide, carglumic acid, trandolapril, candesartan, norethisterone, tigecycline, stavudine, clofarabine, ponatinib, and quetiapine. Outcomes in patients of drugs with valid report number ≥100 is summarized in Table 4. Ponatinib (16.48%, P < 0.0001), tigecycline (14.12%, P = 0.0001) and valproic acid (13.41%, P < 0.0001) had higher fatality rates. Losartan (0/154) and mesalazine (0/318) had zero fatality rates.
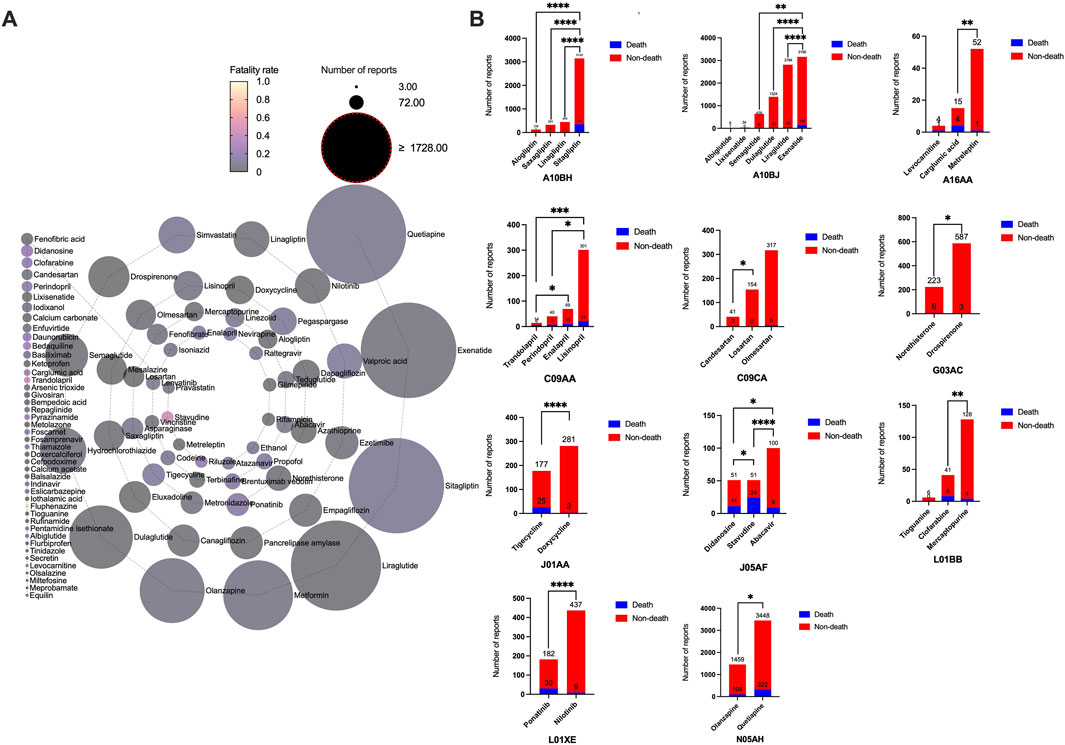
Figure 4. Outcomes of patients with drug-associated pancreatitis. (A) Fatality rate due to different categories of drugs associated pancreatitis events. (B) Drugs with the same Anatomical Therapeutic Chemical code in the first four levels with significant differences in death and non-death.
3.5 Target gene analysis
The DisGeNET and GeneCard databases were searched, yielding 474 pancreatitis target genes, excluding duplicates. Similarly, 1713 target genes were obtained from the DGIdb and DrugBank databases for the 101 complete signaling drugs. Removal of duplicates after verification yielded 668 target genes. The target genes were intersected using Venny 2.1 software to obtain 70 potential targets (Table 5). Full names of the 70 target genes were showed in Supplementary Table S7. Potential target genes for 50 of 101 drugs were obtained (Figure 5A). Sequentially, the PPI network map and the most interacting target genes of the 70 were obtained using the STRING database (Figure 5B). The following target genes had more interactions: SRC (28), EGFR (16), TP53 (16), AKT1 (15), MAPK1 (14), PIK3CA (13), HGF (12), KRAS (11), HRAS (10), IL (10).
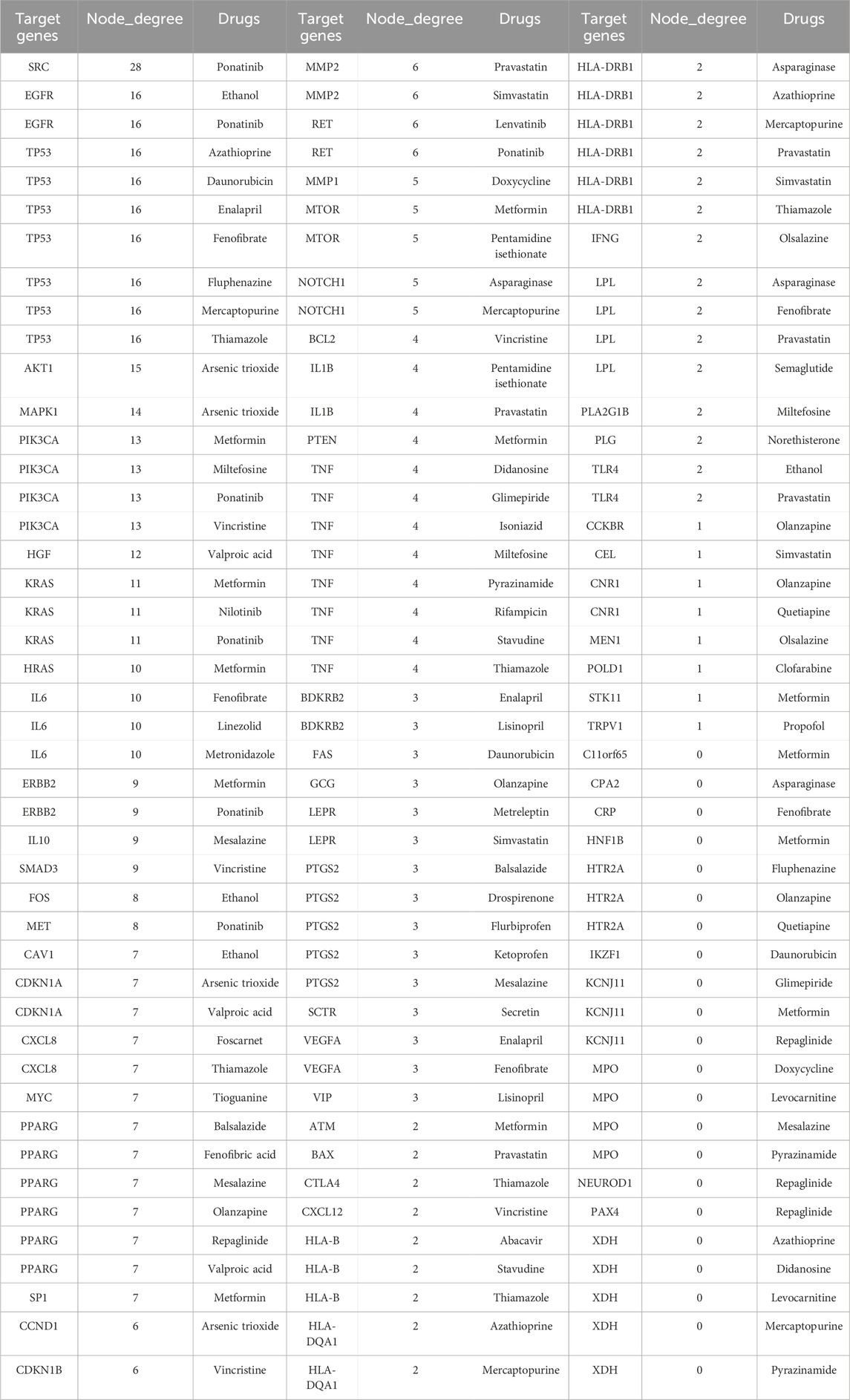
Table 5. Potential target genes of drug-induced pancreatitis, number of interactions and drugs related to the target.
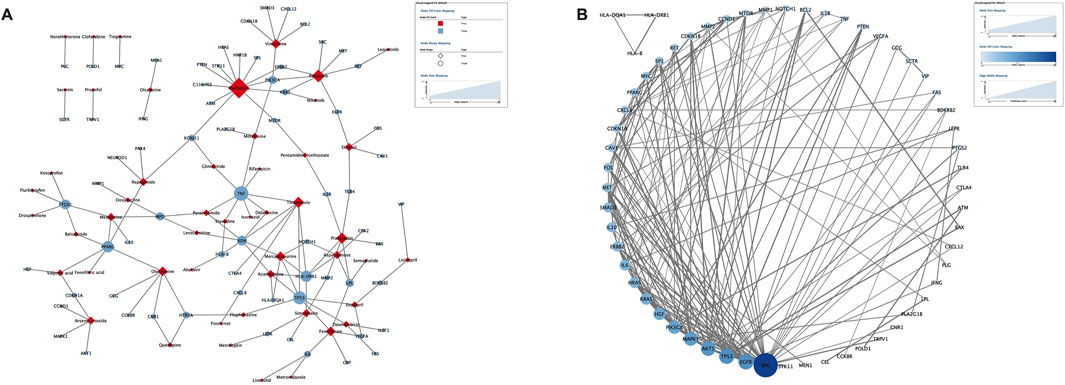
Figure 5. Potential target genes of drug-induced pancreatitis. (A) The drug-target network map of 50 drugs and 70 target genes. (B) The protein-protein interaction network from STRING database map (known interactions).
A total of 420 BPs, 41 CCs, 53 MFs, and 154 KEGG pathways were obtained from the GO/KEGG enrichment analysis (P < 0.05). The top 20 most appreciably enriched entities by gene count are shown in Supplementary Figure S1. The toxicological mechanisms of drug-induced pancreatitis may be related to these factors. The KEGG enrichment analysis suggested these drugs mainly work through multiple pathways in pathways in cancer, endocrine resistance, human cytomegalovirus infection, EGFR tyrosine kinase inhibitor resistance, proteoglycans in cancer.
4 Discussion
The overall incidence of drug-induced pancreatitis is thought to be between 0.1% and 2% (Balani and Grendell, 2008), accounting for approximately 5% of acute pancreatitis cases (Vinklerová et al., 2010; Lankisch et al., 2015). The low incidence of drug-induced pancreatitis makes prospective studies challenging. We obtained reports of drug-related pancreatitis events from the FAERS database, and comprehensively analyzed the association and demographic information, time to onset, and death reports. Toxicological mechanisms were explored using network pharmacology. Through analysis of the FAERS database, 101 drugs with the risk of pancreatitis were identified, of which 40 drugs had not been reported in the UpToDate database.
Most drug-induced pancreatitis cases (66.82%) occurred in the 18–64 years age group, possibly due to the greater number of drug users in this age group. Pancreatitis events occurred more in the 0–18 years age group for asparaginase, carglumic acid, clofarabine, and vincristine. Asparaginase, clofarabine, and vincristine are commonly used to treat acute lymphoblastic leukemia, the most common malignant tumor in childhood (Ward et al., 2014). Carglumic acid is commonly used to treat hyperammonemia in patients with a deficiency in N-acetyl glutamate synthase (a rare genetic disorder). Enzyme deficiencies of adult patients are mild, and thus carglumic acid is usually used under the age of eighteen (Nashabat et al., 2019). Women are thought to be more susceptible to drug-induced pancreatitis (Balani and Grendell, 2008). However, our demographic results were not strong enough to support this view due to influence by the different numbers of drug users by sex. Pancreatitis events associated with doxycycline, drospirenone, eluxadoline, liraglutide, metreleptin, and quetiapine were more prevalent in women. Doxycycline, drospirenone, liraglutide, and quetiapine are usually used more in women. Eluxadoline is used to treat irritable bowel syndrome (IBS) with diarrhea. Although the overall prevalence of IBS is higher in women than men (prevalence: 14%, 9%) (Lovell and Ford, 2012), the sex ratio of patients does not reach that of patients with pancreatitis. A study of eluxadoline (Cash et al., 2021) found that the proportion of female patients without a gallbladder was higher. Eluxadoline could increase the sphincter of Oddi tone, and absence of the gallbladder could lead to increased pressure in the pancreaticobiliary ductal system, which could potentiate clinical signs and symptoms of pancreatitis (Afghani et al., 2017). Metreleptin is commonly used to treat lipodystrophy, and the dosage used in women is generally greater than in men, possibly accounting for the increased incidence of pancreatitis in women. We found that patients with pancreatitis events with complete signaling drugs (median, 88 kg) were heavier (P < 0.0001). Obesity can increase the risk of pancreatitis and even aggravate the severity of pancreatitis (Khatua et al., 2017).
It is interesting that the hypoglycemic and now anti-obesity drugs (GLP-1 analogs and DDP-4 inhibitors) are significantly associated with pancreatitis (both in number of reports and strength of signal). Long-term overstimulation of GLP-1 receptors in exocrine pancreatic cells increases exocrine pancreatic secretion and could theoretically induce pancreatitis. A population-based matched case-control study found that sitagliptin and exenatide was associated with increased odds of hospitalization for acute pancreatitis (Singh et al., 2013). There are meta-analyses of clinical trials that have reported DDP-4 inhibitors to be associated with an increased risk of pancreatitis (Pinto et al., 2018; Abd El Aziz et al., 2020). No clinical trials have shown the pancreatitis risk of GLP-1 analogs. However, it should be recognized that there is a small number of observed cases of pancreatitis and wide CI of risk estimates. Despite insufficient evidence, the FDA included a label warning about the risk of acute pancreatitis of DDP-4 inhibitors and GLP-1 analogs on the basis of postmarketing data. Risk for pancreatitis in such patients is confounded by diabetes. However, a recent study found that GLP-1 analogs used for weight loss also increase pancreatitis risk (Sodhi et al., 2023). Overall, the evidence in clinical trials supporting the risk of pancreatitis in DPP-4 inhibitors and GLP-1 analogs is weak due to sample size limitations. However, their pancreatitis risk has been preliminarily demonstrated based on the results of post-marketing observational studies. For GLP-1 analogues and DPP-4 inhibitors, a baseline assessment of pancreatic risk factors (e.g., triglyceride level, gallbladder status, alcohol use history) can be performed before initiating therapy, especially in patients with preexisting metabolic syndrome (Tenner et al., 2024). Based on the median incubation period of GLP-1 analogues (42–85 days, excluding exenatide), it is recommended to focus on monitoring serum lipase/amylase during the first 3 months of treatment initiation.
Although there are studies (Singh et al., 2013; Pinto et al., 2018; Abd El Aziz et al., 2020; Dicembrini et al., 2020; Zhang et al., 2022) on whether DPP-4 inhibitors and GLP-1 analogs increase the risk of pancreatitis, few studies have focused on time to onset. We found that sitagliptin-related pancreatitis usually occurred approximately 6 months after drug initiation, while other DPP-4 inhibitors usually occurred at 3 months. The profiles of exenatide relative to those of other GLP-1 analogs were similar. In addition, we summarized the fatality rate of patients. Notably, sitagliptin (fatality rate: 10.99%) and exenatide (fatality rate: 4.79%) related pancreatitis occurred later but might be more severe. Observational studies have reported an increased risk of subclinical pancreatic inflammation and pancreatic cancer in sitagliptin and exenatide users (Cohen, 2013; Halfdanarson and Pannala, 2013; Singh et al., 2013). Chronic pancreatitis has been suggested as a potential mechanism for the development of pancreatic cancer in patients taking incretins (Halfdanarson and Pannala, 2013). Long-term subclinical pancreatitis caused by sitagliptin and exenatide is less noticeable than pancreatitis caused by other drugs, so it is more likely to develop into pancreatic cancer, leading to more serious consequences.
Quetiapine (823 days), olanzapine (514 days), drospirenone (488 days), valproic acid (437 days), and simvastatin (434 days) are classified as ultra-long latency drugs (median >1 year). Annual pancreatic function assessment (imaging plus enzymology) should be performed in patients with long-term use (>1 year), taking into account their cumulative risk profile (Gukovskaya et al., 2024). In patients with valproic acid use and unexplained abdominal pain, the differential diagnosis should be prioritized to rule out pancreatitis (because of its high fatality rate of 13.41%). Pegaspargase (23 days), and nilotinib (13.5 days), are categorized as short-latency drugs (median <3 months). Intensive monitoring can be implemented during the first month of treatment, including weekly enzyme testing during induction therapy. Prophylactic pancreatic enzyme supplementation can be considered for high-risk regimens (O’brien and Omer, 2019).
The pathway of pancreatitis has not yet been established. We assumed there were one or more pathways, and drugs acting on one or more targets in these pathways could promote or inhibit the occurrence of pancreatitis. We identified 70 potential targets of 50 drugs that induce pancreatitis. In addition, the 70 targets obtained were analyzed and SRC, EGFR and TP53 are considered core targets. Activation of SRC mediate cofilin activation (Ramos-Alvarez et al., 2023), which is important in the regulation of insulin secretin and pancreatic acinar depolymerization/reorganization (Xu et al., 2021) related with pathogenesis of pancreatitis. SRC inhibitors (such as ponatinib) may disrupt barrier function in pancreatic ductal epithelial cells by mediating a actin filaments (Yang et al., 2022). In the pancreas, SRC activation can promote repair after acinar cell injury, but excessive activation can promote necrosis, as well as chemokine/cytokine release to induce inflammation, leading to pathological inflammation (Nuche-Berenguer et al., 2016). EGFR signaling pathway plays an important role in the physiological processes of cell growth, proliferation and differentiation. Studies have shown that there is high or abnormal expression of EGFR in many solid tumors, including pancreatic cancer (Verma et al., 2019). In addition to pancreatic cancer, EGRR is also closely related to the occurrence of pancreatitis. Some studies suggest that hyperactivation of EGFR signaling is related with the induction of pancreatitis (Engle et al., 2019; Fan et al., 2020). Some studies have not found that EGFR is overexpressed in pancreatitis tissues (Chen et al., 2015). EGFR signaling activation plays an important role in inflammation-driven metaplasia and cancer initiation. Tp53 is a tumor suppressor protein encoded by tumor suppressor genes. However, Tp53 expression also promote the development of pancreatitis by acinar apoptosis and injury (Zhou et al., 2019; Tan et al., 2020). The expression of TP53 promotes the release of inflammatory factors, mediates acinar cell apoptosis and inflammatory responses, thereby exacerbating pancreatitis symptoms (Tan et al., 2020). In chronic pancreatitis, the endoplasmic reticulum stress pathway is activated. Studies have shown that endoplasmic reticulum stress markers (ATF6, XBP1, CHOP) are significantly upregulated in both chronic pancreatitis patients and mouse models, accompanied by increased TP53 expression (Zhou et al., 2019). In pancreatic tissues of chronic pancreatitis model mice, acinar cell apoptosis is markedly elevated and correlated with higher TP53 levels. Inhibition of TP53 reduces apoptosis, ameliorates pancreatic structural damage and fibrosis, and lowers the levels of inflammatory factors (IL-6, IL-1β, TNF-α). Therefore, TP53 is a double-edged sword, on the one hand has a protective effect against pancreatic cancer, on the other hand may cause pancreatitis.
Our study has several limitations. First, imperfections in information, such as incorrect inputs and incomplete reports, may lead to bias in the analysis. Second, it was not determined whether the drug had a positive or negative effect on the target; therefore, the results might have been overestimated. Third, although 101 drugs were statistically correlated with pancreatitis, this did not indicate that they were biologically related. Limited by the inherent structure of the FAERS database, the effect of multiple potential confounders could not be adjusted. For instance: a. Pre-existing conditions: Some drugs (e.g., GLP-1 analogs, DPP-4 inhibitors) are mainly used by diabetic patients, and diabetes is an independent risk factor for pancreatitis (Tenner et al., 2024). Even though these drugs show a pancreatitis risk when used for weight loss (Sodhi et al., 2023), the confounding effects of metabolic factors like hyperglycemia, obesity, or insulin resistance cannot be entirely ruled out. b. Interactions between comorbidities and polypharmacy: FAERS lacks complete drug use and clinical data (e.g., lipid levels, history of gallbladder disease), making it impossible to assess the impact of known high-risk conditions for pancreatitis, such as hypertriglyceridemia, cholelithiasis, and autoimmune diseases, which may jointly influence drug use and pancreatitis development (Tenner et al., 2024). c. Drug interactions: For example, diuretics (like hydrochlorothiazide) are often combined with angiotension converting enzyme inhibitors (like lisinopril) for hypertension treatment. Both are identified as signal drugs, but their individual effects and synergistic toxicity cannot be distinguished.
5 Conclusion
In summary, disproportionality analysis helped to identify 101 from 867 drugs with pancreatitis report number ≥3 in 64,866 reports, and network pharmacology analysis investigated the toxicological mechanisms of 50. The results of network pharmacology analysis help us to understand the mechanism of drug-induced pancreatitis. Although further investigations are warranted to establish the causality, clinicians providing these therapies should stay vigilant to detect pancreatitis early and consider drug factors to provide targeted interventions when diagnosing and treating patients with pancreatitis. In addition, baseline/follow-up pancreatic assessments are recommended for high-risk drug classes (e.g., GLP-1 analogues, antipsychotics).
Data availability statement
The original contributions presented in the study are included in the article/Supplementary Material, further inquiries can be directed to the corresponding author.
Ethics statement
Ethical approval was not required for the study involving humans in accordance with the local legislation and institutional requirements. Written informed consent to participate in this study was not required from the participants or the participants’ legal guardians/next of kin in accordance with the national legislation and the institutional requirements.
Author contributions
HX: Conceptualization, Investigation, Supervision, Writing – original draft, Writing – review and editing. LJ: Conceptualization, Investigation, Supervision, Writing – original draft, Writing – review and editing. JP: Conceptualization, Investigation, Supervision, Writing – review and editing. HH: Investigation, Writing – review and editing. MH: Writing – review and editing. BZ: Conceptualization, Investigation, Methodology, Project administration, Supervision, Writing – review and editing.
Funding
The author(s) declare that financial support was received for the research and/or publication of this article. National High Level Hospital Clinical Research Funding [2022-PUMCH-B-058] and Talent Project established by Chinese Pharmaceutical Association Hospital Pharmacy Department [CPA-Z05-ZC-2022-003].
Acknowledgments
We thank the FDA Adverse Event Reporting System database, the UpToDate database, the DisGeNET database, the GeneCard database, the DGIdb database, the, DrugBank database, the UniProt database, the STRING database, the DAVID database for data support. We would like to thank Editage (www.editage.com) for English language editing, and thank Peking Union Medical College Hospital and Chinese Pharmaceutical Association Hospital Pharmacy Department for funding.
Conflict of interest
The authors declare that the research was conducted in the absence of any commercial or financial relationships that could be construed as a potential conflict of interest.
Generative AI statement
The author(s) declare that no Generative AI was used in the creation of this manuscript.
Publisher’s note
All claims expressed in this article are solely those of the authors and do not necessarily represent those of their affiliated organizations, or those of the publisher, the editors and the reviewers. Any product that may be evaluated in this article, or claim that may be made by its manufacturer, is not guaranteed or endorsed by the publisher.
Supplementary material
The Supplementary Material for this article can be found online at: https://www.frontiersin.org/articles/10.3389/fphar.2025.1564127/full#supplementary-material
Abbreviations
FAERS, Food and Drug Administration Adverse Event Reporting System; PT, preferred term; IQR, interquartile range; FDA, the U.S. Food and Drug Administration; ATC, Anatomical Therapeutic Chemical; PPI, protein-protein interactions; GO, gene ontology; KEGG, Kyoto Encyclopedia of Genes and Genomes; BP, biological process; CC, cellular component; MF, molecular function; CI, confidence interval; GLP-1, glucagon-like peptide-1; DPP-4, dipeptidyl peptidase 4; IBS, irritable bowel syndrome.
References
Abd El Aziz, M., Cahyadi, O., Meier, J. J., Schmidt, W. E., and Nauck, M. A. (2020). Incretin-based glucose-lowering medications and the risk of acute pancreatitis and malignancies: a meta-analysis based on cardiovascular outcomes trials. Diabetes Obes. Metab. 22, 699–704. doi:10.1111/dom.13924
Afghani, E., Lo, S. K., Covington, P. S., Cash, B. D., and Pandol, S. J. (2017). Sphincter of Oddi function and risk factors for dysfunction. Front. Nutr. 4, 1. doi:10.3389/fnut.2017.00001
Balani, A. R., and Grendell, J. H. (2008). Drug-induced pancreatitis: incidence, management and prevention. Drug Saf. 31, 823–837. doi:10.2165/00002018-200831100-00002
Bian, S., Li, L., Wang, Z., Cui, L., Xu, Y., Guan, K., et al. (2021). Neuropsychiatric side reactions of leukotriene receptor antagonist, antihistamine, and inhaled corticosteroid: a real-world analysis of the Food and drug administration (fda) adverse event reporting system (faers). World Allergy Organ J. 14, 100594. doi:10.1016/j.waojou.2021.100594
Candore, G., Juhlin, K., Manlik, K., Thakrar, B., Quarcoo, N., Seabroke, S., et al. (2015). Comparison of statistical signal detection methods within and across spontaneous reporting databases. Drug Saf. 38, 577–587. doi:10.1007/s40264-015-0289-5
Cash, B. D., Lacy, B. E., Watton, C., Schoenfeld, P. S., and Weissman, D. (2021). Post-marketing reports of pancreatitis in eluxadoline-treated patients pre and post us label change. Ther. Adv. Gastroenterol. 14, 17562848211001725. doi:10.1177/17562848211001725
Chen, N. M., Singh, G., Koenig, A., Liou, G. Y., Storz, P., Zhang, J. S., et al. (2015). Nfatc1 links egfr signaling to induction of Sox9 transcription and acinar-ductal transdifferentiation in the pancreas. Gastroenterology 148, 1024–1034. doi:10.1053/j.gastro.2015.01.033
Cohen, D. (2013). Has pancreatic damage from glucagon suppressing diabetes drugs been underplayed? Bmj 346, f3680. doi:10.1136/bmj.f3680
Dicembrini, I., Montereggi, C., Nreu, B., Mannucci, E., and Monami, M. (2020). Pancreatitis and pancreatic cancer in patientes treated with dipeptidyl peptidase-4 inhibitors: an extensive and updated meta-analysis of randomized controlled trials. Diabetes Res. Clin. Pract. 159, 107981. doi:10.1016/j.diabres.2019.107981
Engle, D. D., Tiriac, H., Rivera, K. D., Pommier, A., Whalen, S., Oni, T. E., et al. (2019). The glycan Ca19-9 promotes pancreatitis and pancreatic cancer in mice. Science 364, 1156–1162. doi:10.1126/science.aaw3145
Fan, L., Hui, X., Mao, Y., and Zhou, J. (2020). Identification of acute pancreatitis-related genes and pathways by integrated bioinformatics analysis. Dig. Dis. Sci. 65, 1720–1732. doi:10.1007/s10620-019-05928-5
Flaig, T., Douros, A., Bronder, E., Klimpel, A., Kreutz, R., and Garbe, E. (2016). Tocilizumab-induced pancreatitis: case report and review of data from the fda adverse event reporting system. J. Clin. Pharm. Ther. 41, 718–721. doi:10.1111/jcpt.12456
Frent, I., Bucsa, C., Leucuta, D., Farcas, A., and Mogosan, C. (2021). An investigation on the association between sodium glucose Co-transporter 2 inhibitors use and acute pancreatitis: a vigibase study. Pharmacoepidemiol Drug Saf. 30, 1428–1440. doi:10.1002/pds.5313
Gawron, A. J., and Bielefeldt, K. (2018). Risk of pancreatitis following treatment of irritable bowel syndrome with eluxadoline. Clin. Gastroenterol. Hepatol. 16, 378–384. doi:10.1016/j.cgh.2017.08.006
Gukovskaya, A. S., Lerch, M. M., Mayerle, J., Sendler, M., Ji, B., Saluja, A. K., et al. (2024). Trypsin in pancreatitis: the culprit, a mediator, or epiphenomenon? World J. Gastroenterol. 30, 4417–4438. doi:10.3748/wjg.v30.i41.4417
Halfdanarson, T. R., and Pannala, R. (2013). Incretins and risk of neoplasia. Bmj 346, f3750. doi:10.1136/bmj.f3750
Harinstein, L., Wu, E., and Brinker, A. (2018). Postmarketing cases of eluxadoline-associated pancreatitis in patients with or without a gallbladder. Aliment. Pharmacol. Ther. 47, 809–815. doi:10.1111/apt.14504
Hauben, M. (2004). Application of an empiric bayesian data mining algorithm to reports of pancreatitis associated with atypical antipsychotics. Pharmacotherapy 24, 1122–1129. doi:10.1592/phco.24.13.1122.38098
Hung, W. Y., and Abreu Lanfranco, O. (2014). Contemporary review of drug-induced pancreatitis: a different perspective. World J. Gastrointest. Pathophysiol. 5, 405–415. doi:10.4291/wjgp.v5.i4.405
Kamath, A., Acharya, S. D., Rao, R. R., and Ullal, S. D. (2021). Assessment of pancreatitis associated with tocilizumab use using the United States Food and drug administration adverse event reporting system database. Sci. Rep. 11, 18818. doi:10.1038/s41598-021-98325-w
Khatua, B., El-Kurdi, B., and Singh, V. P. (2017). Obesity and pancreatitis. Curr. Opin. Gastroenterol. 33, 374–382. doi:10.1097/MOG.0000000000000386
Lancashire, R. J., Cheng, K., and Langman, M. J. (2003). Discrepancies between population-based data and adverse reaction reports in assessing drugs as causes of acute pancreatitis. Aliment. Pharmacol. Ther. 17, 887–893. doi:10.1046/j.1365-2036.2003.01485.x
Lankisch, P. G., Apte, M., and Banks, P. A. (2015). Acute pancreatitis. Lancet 386, 85–96. doi:10.1016/S0140-6736(14)60649-8
Lovell, R. M., and Ford, A. C. (2012). Effect of gender on prevalence of irritable bowel syndrome in the community: systematic review and meta-analysis. Am. J. Gastroenterol. 107, 991–1000. doi:10.1038/ajg.2012.131
Nango, D., Hirose, Y., Goto, M., and Echizen, H. (2019). Analysis of the association of administration of various glucocorticoids with development of acute pancreatitis using us Food and drug administration adverse event reporting system (faers). J. Pharm. Health Care Sci. 5, 5. doi:10.1186/s40780-019-0134-6
Nashabat, M., Obaid, A., Al Mutairi, F., Saleh, M., Elamin, M., Ahmed, H., et al. (2019). Evaluation of long-term effectiveness of the use of carglumic acid in patients with propionic acidemia (Pa) or methylmalonic acidemia (mma): study protocol for a randomized controlled trial. BMC Pediatr. 19, 195. doi:10.1186/s12887-019-1571-y
Nitsche, C., Maertin, S., Scheiber, J., Ritter, C. A., Lerch, M. M., and Mayerle, J. (2012). Drug-induced pancreatitis. Curr. Gastroenterol. Rep. 14, 131–138. doi:10.1007/s11894-012-0245-9
Nitsche, C. J., Jamieson, N., Lerch, M. M., and Mayerle, J. V. (2010). Drug induced pancreatitis. Best. Pract. Res. Clin. Gastroenterol. 24, 143–155. doi:10.1016/j.bpg.2010.02.002
Nuche-Berenguer, B., Ramos-Álvarez, I., and Jensen, R. T. (2016). Src kinases play a novel dual role in acute pancreatitis affecting severity but No role in stimulated enzyme secretion. Am. J. Physiol. Gastrointest. Liver Physiol. 310, G1015–G1027. doi:10.1152/ajpgi.00349.2015
O'brien, S. J., and Omer, E. (2019). Chronic pancreatitis and nutrition therapy. Nutr. Clin. Pract. 34 (Suppl. 1), S13–s26. doi:10.1002/ncp.10379
Peery, A. F., Crockett, S. D., Murphy, C. C., Lund, J. L., Dellon, E. S., Williams, J. L., et al. (2019). Burden and cost of gastrointestinal, liver, and pancreatic diseases in the United States: update 2018. Gastroenterology 156, 254–272. doi:10.1053/j.gastro.2018.08.063
Pinto, L. C., Rados, D. V., Barkan, S. S., Leitão, C. B., and Gross, J. L. (2018). Dipeptidyl peptidase-4 inhibitors, pancreatic cancer and acute pancreatitis: a meta-analysis with trial sequential analysis. Sci. Rep. 8, 782. doi:10.1038/s41598-017-19055-6
Qin, W., Zhao, B., Shang, Y., and Zhang, L. (2021). Clinical profile of acute pancreatitis following treatment with protease inhibitors: a real-world analysis of post-marketing surveillance data. Expert Opin. Drug Saf. 20, 1109–1115. doi:10.1080/14740338.2021.1933942
Ramos-Alvarez, I., Lee, L., and Jensen, R. T. (2023). Cofilin activation in pancreatic acinar cells plays a pivotal convergent role for mediating cck-stimulated enzyme secretion and growth. Front. Physiol. 14, 1147572. doi:10.3389/fphys.2023.1147572
Simons-Linares, C. R., Elkhouly, M. A., and Salazar, M. J. (2019). Drug-induced acute pancreatitis in adults: an update. Pancreas 48, 1263–1273. doi:10.1097/MPA.0000000000001428
Singh, S., Chang, H. Y., Richards, T. M., Weiner, J. P., Clark, J. M., and Segal, J. B. (2013). Glucagonlike peptide 1-based therapies and risk of hospitalization for acute pancreatitis in type 2 diabetes mellitus: a population-based matched case-control study. JAMA Intern Med. 173, 534–539. doi:10.1001/jamainternmed.2013.2720
Sodhi, M., Rezaeianzadeh, R., Kezouh, A., and Etminan, M. (2023). Risk of gastrointestinal adverse events associated with glucagon-like peptide-1 receptor agonists for weight loss. Jama 330, 1795–1797. doi:10.1001/jama.2023.19574
Tan, J. H., Cao, R. C., Zhou, L., Zhou, Z. T., Chen, H. J., Xu, J., et al. (2020). Atf6 aggravates acinar cell apoptosis and injury by regulating P53/aifm2 transcription in severe acute pancreatitis. Theranostics 10, 8298–8314. doi:10.7150/thno.46934
Tenner, S., Vege, S. S., Sheth, S. G., Sauer, B., Yang, A., Conwell, D. L., et al. (2024). American College of gastroenterology guidelines: management of acute pancreatitis. Am. J. Gastroenterol. 119, 419–437. doi:10.14309/ajg.0000000000002645
Vakharia, P., Nardone, B., Budris, W., Hoshizaki, K., Frankfurt, O., and West, D. P. (2018). Blinatumomab and pancreatitis: an analysis of faers, eudravigilance, and a large urban U.S. Patient population data. Leuk. Lymphoma 59, 1759–1761. doi:10.1080/10428194.2017.1397667
Verma, H. K., Kampalli, P. K., Lakkakula, S., Chalikonda, G., Bhaskar, L., and Pattnaik, S. (2019). A retrospective look at anti-egfr agents in pancreatic cancer therapy. Curr. Drug Metab. 20, 958–966. doi:10.2174/1389200220666191122104955
Vinklerová, I., Procházka, M., Procházka, V., and Urbánek, K. (2010). Incidence, severity, and etiology of drug-induced acute pancreatitis. Dig. Dis. Sci. 55, 2977–2981. doi:10.1007/s10620-010-1277-3
Ward, E., Desantis, C., Robbins, A., Kohler, B., and Jemal, A. (2014). Childhood and adolescent cancer statistics, 2014. CA Cancer J. Clin. 64, 83–103. doi:10.3322/caac.21219
Xie, H., Xu, Q., Zhao, B., and Wu, W. (2025). Drug-associated pancreatic cancer: insights from real-world pharmacovigilance and network pharmacology. Expert Opin. Drug Saf., 1–11. doi:10.1080/14740338.2025.2469273
Xu, J., Huang, Y., Zhao, J., Wu, L., Qi, Q., Liu, Y., et al. (2021). Cofilin: a promising protein implicated in cancer metastasis and apoptosis. Front. Cell. Dev. Biol. 9, 599065. doi:10.3389/fcell.2021.599065
Yang, H. Y., Liang, Z. H., Xie, J. L., Wu, Q., Qin, Y. Y., Zhang, S. Y., et al. (2022). Gelsolin impairs barrier function in pancreatic ductal epithelial cells by actin filament depolymerization in hypertriglyceridemia-induced pancreatitis in vitro. Exp. Ther. Med. 23, 290. doi:10.3892/etm.2022.11219
Zhang, X., Wang, M., Wang, X., Zhu, Z., Zhang, W., Zhou, Z., et al. (2022). Comparison of new glucose-lowering drugs on the risk of pancreatitis in type 2 diabetes: a network meta-analysis. Endocr. Pract. 28, 333–341. doi:10.1016/j.eprac.2021.12.007
Keywords: pancreatitis, adverse drug reaction, time to onset (TTO), toxicology, target
Citation: Xie H, Jiang L, Peng J, Hu H, Han M and Zhao B (2025) Drug-induced pancreatitis: a real-world analysis of the FDA Adverse Event Reporting System and network pharmacology. Front. Pharmacol. 16:1564127. doi: 10.3389/fphar.2025.1564127
Received: 30 January 2025; Accepted: 04 April 2025;
Published: 16 April 2025.
Edited by:
Ravi Kumar Sharma, All India Institute of Medical Sciences Bilaspur, IndiaReviewed by:
Sheng-ying Gu, Shanghai General Hospital,Mahinder Paul, Albert Einstein College of Medicine, United States
Copyright © 2025 Xie, Jiang, Peng, Hu, Han and Zhao. This is an open-access article distributed under the terms of the Creative Commons Attribution License (CC BY). The use, distribution or reproduction in other forums is permitted, provided the original author(s) and the copyright owner(s) are credited and that the original publication in this journal is cited, in accordance with accepted academic practice. No use, distribution or reproduction is permitted which does not comply with these terms.
*Correspondence: Bin Zhao, emhhb2JpbkBwdW1jaC5jbg==
†These authors have contributed equally to this work