- Memo Therapeutics AG, Schlieren, Switzerland
This study investigates the biodistribution of a therapeutic antibody from serum to urine across the kidney endothelial barrier, contextualizing the findings with existing literature. Our analysis shows the quantitative correlation between serum levels of the intravenously administered antibody rituximab and its urinary concentration, indicating a predictable pharmacokinetic profile. The results align with previous quantitative studies on the biodistribution of endogenous and vaccination induced IgG between serum and urine, confirming that recombinant therapeutic IgG1 passes the kidney endothelial barrier and exhibits similar biodistribution to endogenous IgG. These insights may inform the determination of optimal dosages for therapeutic antibodies targeting the urothelium or renal epithelium.
Introduction
The kidneys play a crucial role in maintaining serum levels of soluble proteins while excreting toxins, metabolic waste products, and excess ions and water. It is widely accepted that the filtration barrier, consisting of (1) podocyte pedicles, (2) the glomerular basement membrane, and (3) the fenestrated endothelium of glomerular capillaries, prevents proteins larger than 70 kDa from being excreted in the urine (Breshears and Confer, 2017). Given that the kidneys filter approximately 180 L of blood and over 2 kg of IgGs daily (Sand et al., 2015), the retention of IgGs in the bloodstream is essential.
It is commonly accepted that IgG does not pass the kidney barrier due to its size (Ryman and Meibohm, 2017). However, recent evidence suggests complex transport processes involving transcytosis allowing some serum IgG to be transported into the tubular lumen and subsequently be reabsorbed to some degree. In this context, it has been demonstrated that podocytes and proximal tubular epithelial cells, which line the lumen of the tubule, express the neonatal Fc receptor (FcRn), which actively transports IgG, along with albumin, via transcytosis (Haymann et al., 2000; Akilesh et al., 2008; Sarav et al., 2009; Sand et al., 2015; Dylewski et al., 2019). Several studies have shown that proximal tubular epithelial cells use FcRn to reabsorb IgG (Kobayashi et al., 2002; Rojas and Apodaca, 2002; Claypool et al., 2004; Sand et al., 2015; Lorentzen et al., 2023) from the filtrate and recycle it back into the bloodstream. Consequently, a portion of IgG passes through the kidney filtration barrier and enters the primary filtrate before being recycled into the blood or being partly excreted via urine (Burdon, 1971; Trinick and Laker, 1984; Reichert et al., 1997; Derré et al., 2020).
This transient presence of IgG in the tubular filtrate may play a crucial role in maintaining immunosurveillance in and around kidney tubular epithelial cells, which also serve as host cells or reservoirs for JC and BK polyomaviruses and CMV (Nickeleit et al., 2000; Li et al., 2010; Kant, 2020). In healthy individuals, these viruses are typically controlled by the immune system but may become reactivated during episodes of immunosuppression, e.g., upon transplantation.
In such cases, the biodistribution of IgG to the kidney tubular epithelium, ureter, and urothelium may be of interest for therapeutic interventions with monoclonal antibodies that target these viral infections or for other conditions affecting the kidneys, ureter or urothelium.
In the present study, we review quantitative data on the biodistribution of endogenous IgG in the serum and urine of human subjects, comparing it to data obtained upon administration with rituximab. Rituximab was chosen as a model antibody for therapeutically administered IgG due to the availability of donor samples and the presence of a sensitive, commercial anti-idiotypic ELISA for its detection.
Methods
Donors
Donors were lymphoma patients receiving rituximab. Serum and urine samples from each donor were collected during the same follow-up visit, all within 1 month after administration of rituximab.
Samples were acquired through Discovery Life Sciences (DLS), with appropriate institutional informed consent and in compliance with local ethical requirements.
Quantification of rituximab in serum and urine by ELISA
The ab237640 Rituximab ELISA kit (Abcam) featuring a sensitive anti-idiotypic antibody to Rituximab as detection reagent was used according to the manufacturer’s instructions. Briefly, serum samples were diluted in assay buffer 400x, 800x, 1,600x, 3,200x, 6,400x. Urine samples collected in boritex® tubes (Andwin Scientific, Simi valley, CA) and frozen at −18°C were used undiluted and diluted in assay buffer 4x, 10x, 20x, 40x and 100x. A serial dilution of a Rituximab standard was done in assay buffer according to the manufacturer’s recommendation, spanning from 3 to 300 ng/mL. Triplicates of sample and standard (100 µL each) were applied to the assay plate and incubated at room temperature, shaking for 1 h at 510 rpm. Subsequently, the plate was washed 3x with 300 µL wash buffer and tap-dried on a paper towel. Next, 100 µL HRP-conjugate was added to each well and incubated at room temperature for 1 h. After washing 3x with 300 µL wash buffer and tap-dried on a paper towel, 100 µL of TMB substrate was added and the plate incubated in the dark at room temperature for 15 min. Reaction was stopped with 100 µL stop solution and absorbance read at 450 nm and 650 nm (reference), using an Tecan Infinite M Nano plate reader.
The background subtracted data (Abs 450 – Abs 650 nm) was analyzed, calculating the average absorbance value for the blank control (0 ng/mL), followed by subtraction of this value from all samples. Values outside of the standard curve range were excluded from the calculation. A standard curve was generated in Graph Pad Prism 10, plotting the protein concentration (x-axis) against the corrected absorbance value (y-axis) and fitting the data with a four-parameter logistic fit. Based on this equation, the Rituximab concentration in each sample was calculated, compensating for the sample dilution.
Preparation of data from published studies as summarized
IgG concentrations indicated in the original articles were used to calculate the percentage of urine concentration. For Derré et al. these concentrations were communicated to us by the authors.
Reichert et al. provide quantitative data about IgG in serum as g/L and in urine as milligram per day. In order to be able compare this with the serum IgG concentration, we assumed a daily urine quantity of 2 L (Raman et al., 2004). Thus, concentration values of urine IgG were calculated using the daily amount divided by 2 L. As an example, the average daily urinary IgG secretion of 98 mg/day (stable renal function) resulted in 49 mg/L urine which when related to the serum concentration of 5.3 g/L in serum yielded the data point 0.92% in our graph.
Results and discussion
Concentration of rituximab in serum and urine
Serum and urine samples taken at the same follow up visit from 5 patients undergoing rituximab treatment (Supplementary Table S1) at the time of sampling were obtained and levels of rituximab in serum and urine were determined (Table 1) by ELISA.
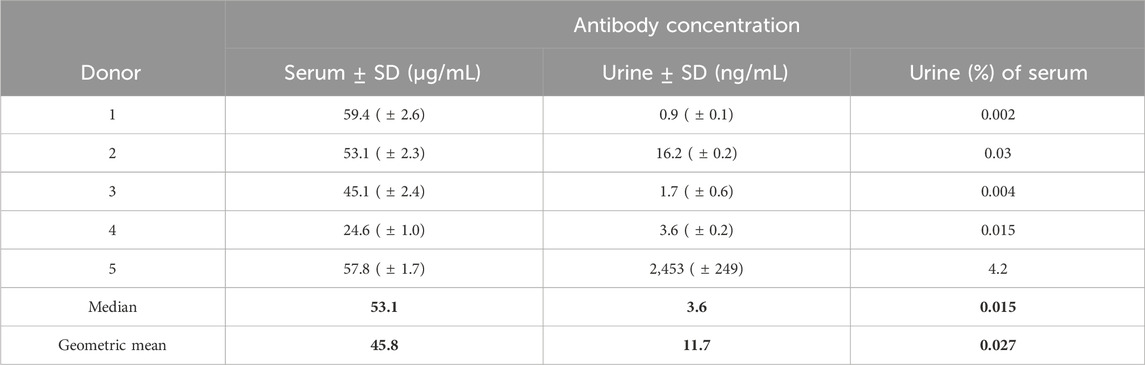
Table 1. Comparison of serum and urine rituximab levels in 5 donors receiving rituximab treatment as measured by a commercially available rituximab- specific ELISA assay.
The rituximab levels in treated donors are relatively widespread with a median percentage of 0.015% of the serum rituximab concentration present in the urine. This spread may well be due to a different stage of morbidity in the donors.
To contextualize our findings, we compared them with data from prior studies that examined serum and urine IgG levels. Some of these studies also compared urine IgG levels in healthy donors with those in patients with compromised renal or urothelial function or integrity (Burdon, 1971; Trinick and Laker, 1984; Reichert et al., 1997).
These data are summarized in Figure 1 below.
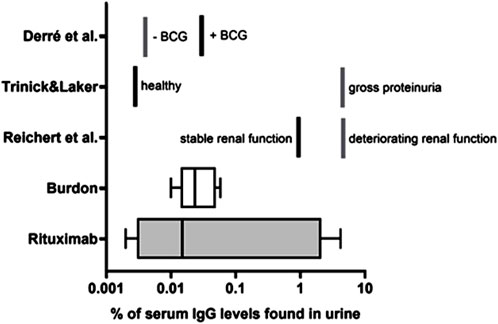
Figure 1. Urine IgG levels in relation to serum IgG Rituximab: i. v. Administered rituximab levels in serum and urine in 5 patients as measured by ELISA based on an anti-idiotypic antibody to rituximab (see also table 1 for individual levels). Burdon (Burdon, 1971): endogenous IgG levels in 4 healthy male and 4 healthy female subjects were quantified by radial diffusion in agar. Reichert et al. (Reichert et al., 1997) endogenous IgG levels in 22 patients with membranous nephropathy or nephrotic syndrome (deteriorating renal function) and normal renal function (stable renal function) were measured by immunonephelometry. Trinick & Laker (Trinick and Laker, 1984): endogenous IgG levels of 14 healthy adults (healthy) and six patients with severe proteinuria (gross proteinuria) were measured by ELISA. Derré et al. (Derré et al., 2020) IgG antibody titters in bladder cancer patients were monitored upon vaccination with a model antigen; 14 patients received Bacillus Calmette–Guérin (BCG), originally developed and used as a vaccine against tuberculosis and is now also used as a treatment for bladder cancer (+BCG) as treatment and 8 did not receive BCG (-BCG).
This comparison shows a good correlation between the different subject groups both across the previously published studies and with the rituximab treated subjects. The one outlier in the Rituximab group (right whisker of the graph), may be explained by an impaired kidney function of unknown etiology as it matches with the elevated levels found in patients with compromised kidney function (Reichert et al., 1997; Derré et al., 2020).
Overall, this data provides evidence that a small but non-negligible amount of serum IgG, whether of endogenous origin or therapeutically administered, passes the endothelial barrier and is detectable in the urine. This process is summarized in Figure 2 below.
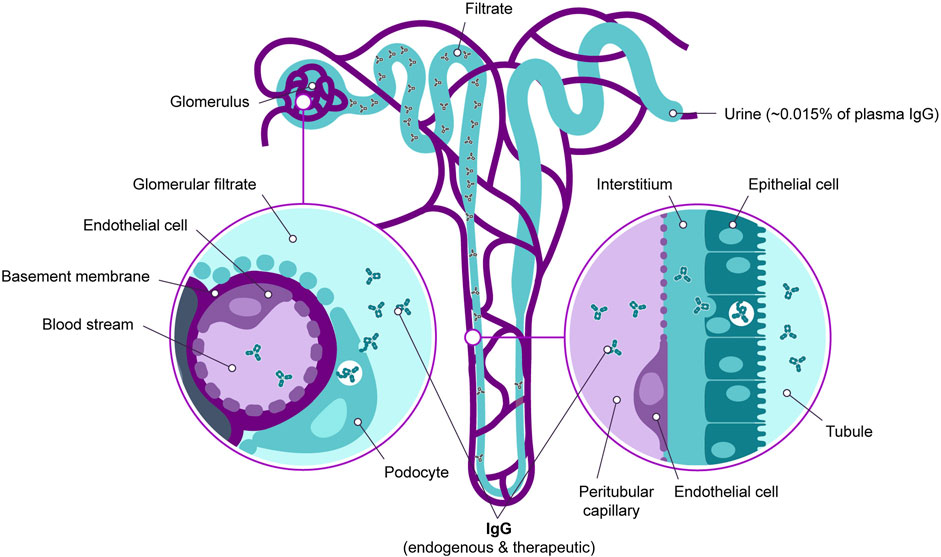
Figure 2. Passage of serum IgG through the kidney endothelial barrier. IgG in the bloodstream enters the glomerular filtrate and the tubular lumen. It is then recycled back into the bloodstream through specific transport mechanisms. Additionally, a significant fraction of IgG is secreted via the urine.
Strategies aimed at enhancing transcytosis of therapeutic antibodies across the kidney endothelial barrier, such as leveraging receptors expressed on renal endothelial cells, have, to our knowledge, not been reported. Other options such as small antibody fragments or even smaller antibody-like molecules such as darpins might offer improved tissue penetration but carry the risk of shorter half-lives and impaired interaction with IgG-specific transcytosis mechanisms due to their inability to bind to the FcRn, potentially further decreasing transcytosis efficiency (Rafidi et al., 2022). Therefore, developing ultra-potent therapeutic antibodies that remain effective at low concentrations currently represents the most promising alternative to treat diseased or infected tissue that lies behind the kidney endothelial barrier.
While previous studies have established the existence of transport mechanisms for human IgG through the endothelial cells of blood vessels to the renal epithelia, as well as the reabsorption mechanisms from the urine by proximal tubular epithelial cells via specific transport mechanisms (McCarthy et al., 2000; Pyzik et al., 2019; Bryniarski et al., 2021), they did not provide quantitative data, and such data from preclinical animal models have also not been found in the literature.
Our observation that on average 0.015% of serum IgG makes its way into the urine may serve a basis for dose finding of therapeutic antibodies intended for activity in the kidney and urogenital tract.
However, a limitation of our study is that it cannot provide quantitative data on the antibody concentration in the lumen of tubular epithelia or the urinary ducts. Nevertheless, due to the reabsorption mechanisms, the IgG concentration in the tubular lumen and ureter can be assumed to be substantially higher than the determined urine-serum ratio of 0.015%.
Author contributions
BE: Conceptualization, Data curation, Formal Analysis, Methodology, Validation, Visualization, Writing – original draft, Writing – review and editing. CE: Conceptualization, Data curation, Formal Analysis, Funding acquisition, Investigation, Methodology, Project administration, Resources, Software, Supervision, Validation, Visualization, Writing – original draft, Writing – review and editing. MH: Conceptualization, Data curation, Formal Analysis, Investigation, Methodology, Project administration, Resources, Software, Supervision, Validation, Visualization, Writing – original draft, Writing – review and editing.
Funding
The author(s) declare that financial support was received for the research and/or publication of this article. This study was funded by Memo Therapeutics AG.
Acknowledgments
We thank Laurent Derré for sharing raw data of his study “Intramuscular Immunization Induces Antigen-Specific Antibodies in Urine”.
Conflict of interest
The authors are employees of Memo Therapeutics AG and own stock or stock options of the company.
Generative AI statement
The author(s) declare that no Generative AI was used in the creation of this manuscript.
Publisher’s note
All claims expressed in this article are solely those of the authors and do not necessarily represent those of their affiliated organizations, or those of the publisher, the editors and the reviewers. Any product that may be evaluated in this article, or claim that may be made by its manufacturer, is not guaranteed or endorsed by the publisher.
Supplementary material
The Supplementary Material for this article can be found online at: https://www.frontiersin.org/articles/10.3389/fphar.2025.1572739/full#supplementary-material
References
Akilesh, S., Huber, T. B., Wu, H., Wang, G., Hartleben, B., Kopp, J. B., et al. (2008). Podocytes use FcRn to clear IgG from the glomerular basement membrane. Proc. Natl. Acad. Sci. 105 (3), 967–972. doi:10.1073/pnas.0711515105
Breshears, M. A., and Confer, A. W. (2017). “The urinary system,” in Pathologic basis of veterinary disease (Elsevier), 617–681.e1. doi:10.1016/B978-0-323-35775-3.00011-4
Bryniarski, M. A., Zhao, B., Chaves, L. D., Mikkelsen, J. H., Yee, B. M., Yacoub, R., et al. (2021). Immunoglobulin G is a novel substrate for the endocytic protein megalin. AAPS J. 23 (2), 40. doi:10.1208/s12248-021-00557-1
Burdon, D. W. (1971). Immunoglobulins of normal human urine and urethral secretions. Immunology 21 (2), 363–368.
Claypool, S. M., Dickinson, B. L., Wagner, J. S., Johansen, F. E., Venu, N., Borawski, J. A., et al. (2004). Bidirectional transepithelial IgG transport by a strongly polarized basolateral membrane Fcgamma-receptor. Mol. Biol. Cell. 15 (4), 1746–1759. doi:10.1091/mbc.E03-11-0832
Derré, L., Lucca, I., Cesson, V., Valerio, M., Cerantola, Y., Burruni, R., et al. (2020). Intramuscular immunization Induces antigen-specific antibodies in urine. Eur. Urol. Focus 6 (2), 280–283. doi:10.1016/j.euf.2018.09.003
Dylewski, J., Dobrinskikh, E., Lewis, L., Tonsawan, P., Miyazaki, M., Jat, P. S., et al. (2019). ‘Differential trafficking of albumin and IgG facilitated by the neonatal Fc receptor in podocytes in vitro and in vivo’ PLoS one, S. R. Singh (ed.) 14(2), p. e0209732. doi:10.1371/journal.pone.0209732
Haymann, J.-P., Levraud, J. P., Bouet, S., Kappes, V., Hagège, J., Nguyen, G., et al. (2000). Characterization and localization of the neonatal Fc receptor in adult human kidney. J. Am. Soc. Nephrol. 11 (4), 632–639. doi:10.1681/ASN.V114632
Kant, S. (2020). Kidney biopsy of the month: BK virus nephropathy. Renal Fellow Network. Available online at: https://www.renalfellow.org/2020/11/03/kidney-biopsy-of-the-month-bk-virus-nephropathy/ (Accessed November 25, 2024).
Kobayashi, N., Suzuki, Y., Tsuge, T., Okumura, K., Ra, C., and Tomino, Y. (2002). FcRn-mediated transcytosis of immunoglobulin G in human renal proximal tubular epithelial cells. Am. J. Physiology-Renal Physiology 282 (2), F358–F365. doi:10.1152/ajprenal.0164.2001
Li, Y. T., Emery, V. C., Surah, S., Jarmulowicz, M., Sweny, P., Kidd, I. M., et al. (2010). Extensive human cytomegalovirus (HCMV) genomic DNA in the renal tubular epithelium early after renal transplantation: relationship with HCMV DNAemia and long-term graft function. J. Med. Virology 82 (1), 85–93. doi:10.1002/jmv.21662
Lorentzen, E. M., Henriksen, S., and Rinaldo, C. H. (2023). Modelling BK polyomavirus dissemination and cytopathology using polarized human renal tubule epithelial cells. PLOS Pathog. 19 (8), e1011622. doi:10.1371/journal.ppat.1011622
McCarthy, K. M., Yoong, Y., and Simister, N. E. (2000). Bidirectional transcytosis of IgG by the rat neonatal Fc receptor expressed in a rat kidney cell line: a system to study protein transport across epithelia. J. Cell. Sci. 113 (7), 1277–1285. doi:10.1242/jcs.113.7.1277
Nickeleit, V., Hirsch, H. H., Zeiler, M., Gudat, F., Prince, O., Thiel, G., et al. (2000). BK-virus nephropathy in renal transplants-tubular necrosis, MHC-class II expression and rejection in a puzzling game. Nephrol. Dialysis, Transplant. Official Publ. Eur. Dialysis Transpl. Assoc. - Eur. Ren. Assoc. 15 (3), 324–332. doi:10.1093/ndt/15.3.324
Pyzik, M., Sand, K. M. K., Hubbard, J. J., Andersen, J. T., Sandlie, I., and Blumberg, R. S. (2019). The neonatal Fc receptor (FcRn): a misnomer? Front. Immunol. 10, 1540. doi:10.3389/fimmu.2019.01540
Rafidi, H., Rajan, S., Urban, K., Shatz-Binder, W., Hui, K., Ferl, G. Z., et al. (2022). Effect of molecular size on interstitial pharmacokinetics and tissue catabolism of antibodies. mAbs 14 (1), 2085535. doi:10.1080/19420862.2022.2085535
Raman, A., Schoeller, D. A., Subar, A. F., Troiano, R. P., Schatzkin, A., Harris, T., et al. (2004). Water turnover in 458 American adults 40-79 Yr of age. Am. J. Physiology-Renal Physiology 286 (2), F394–F401. doi:10.1152/ajprenal.00295.2003
Reichert, L. J., Koene, R. A., and Wetzels, J. F. (1997). Urinary IgG excretion as a prognostic factor in idiopathic membranous nephropathy. Clin. Nephrol. 48 (2), 79–84.
Rojas, R., and Apodaca, G. (2002). Immunoglobulin transport across polarized epithelial cells. Nat. Rev. Mol. Cell. Biol. 3 (12), 944–955. doi:10.1038/nrm972
Ryman, J. T., and Meibohm, B. (2017). Pharmacokinetics of monoclonal antibodies. CPT Pharmacometrics and Syst. Pharmacol. 6 (9), 576–588. doi:10.1002/psp4.12224
Sand, K. M. K., Bern, M., Nilsen, J., Noordzij, H. T., Sandlie, I., and Andersen, J. T. (2015). Unraveling the interaction between FcRn and albumin: opportunities for design of albumin-based therapeutics. Front. Immunol. 5, 682. doi:10.3389/fimmu.2014.00682
Sarav, M., Wang, Y., Hack, B. K., Chang, A., Jensen, M., Bao, L., et al. (2009). Renal FcRn reclaims albumin but facilitates elimination of IgG. J. Am. Soc. Nephrol. 20 (9), 1941–1952. doi:10.1681/ASN.2008090976
Keywords: monoclonal antibody, pharmacokinetic, kidney epithelial barrier, kidney tubular lumen, urine, urothelia, therapeutic antibody, serum
Citation: Eicher B, Esslinger C and Hillenbrand M (2025) Quantification of endogenous and therapeutic IgG crossing the kidney barrier from bloodstream to urine. Front. Pharmacol. 16:1572739. doi: 10.3389/fphar.2025.1572739
Received: 07 February 2025; Accepted: 04 April 2025;
Published: 25 April 2025.
Edited by:
Krishna M Boini, University of Houston, United StatesReviewed by:
Arthur Van De Vyver, Novartis, SwitzerlandSriram Inavolu, Texas A&M University Kingsville, United States
Copyright © 2025 Eicher, Esslinger and Hillenbrand. This is an open-access article distributed under the terms of the Creative Commons Attribution License (CC BY). The use, distribution or reproduction in other forums is permitted, provided the original author(s) and the copyright owner(s) are credited and that the original publication in this journal is cited, in accordance with accepted academic practice. No use, distribution or reproduction is permitted which does not comply with these terms.
*Correspondence: Matthias Hillenbrand, bWF0dGhpYXMuaGlsbGVuYnJhbmRAbWVtby10aGVyYXBldXRpY3MuY29t