- 1Clinical Psychology and Psychotherapy, University of Zurich, Zurich, Switzerland
- 2Department of Psychiatry – Child and Adolescent Inpatient Service, Icahn School of Medicine at Mount Sinai, New York, NY, USA
Epidemiological studies show low rates of diagnosed depression in men compared to women. At the same time, high rates of alcohol use disorders (AUDs) and completed suicide are found among men. These data suggest that a male-specific pattern for depression may exist that is linked to AUDs and suicidal behavior. To date, no underlying neuroendocrine model for this specific pattern of male depression has been suggested. In this paper, we integrate findings related to this specific pattern of depression with underlying steroid secretion patterns, polymorphisms, and methylation profiles of key genes in order to detail an original neuroendocrine model of male-specific depression. Low circulating levels of sex steroids seem to increase the vulnerability for male depression, while concomitant high levels of glucocorticoids further intensify this vulnerability. Interactions of hypothalamus–pituitary–gonadal (HPG) and hypothalamus–pituitary–adrenocortical (HPA) axis-related hormones seem to be highly relevant for a male-specific pattern of depression linked to AUDs and suicidal behavior. Moreover, genetic variants and the epigenetic profiles of the androgen receptor gene, well-known depression related genes, and HPA axis-related genes were shown to further interact with men’s steroid secretion and thus may further contribute to the proposed male-specific pattern for depression. This mini-review points out the multilevel interactions between the HPG and HPA axis for a male-specific pattern of depression linked to AUDs and suicidal behavior. An integration of multilevel interactions within the three-hit concept of vulnerability and resilience concludes the review.
Introduction
Epidemiological studies suggest that women experience depressive disorders at two to three times the rate of men (1, 2). However, for men in Western countries, concomitant low levels of diagnosed depression alongside high rates of alcohol use disorders (AUDs) and suicide are reported (3, 4). Suicide and AUDs are more strongly intertwined within males than females (5). This finding is supported by a study showing that men are more likely to have elevated blood alcohol levels at the time of completed suicide (6). Furthermore, over 2% of traffic accidents are classified as road traffic suicides, of which are committed to around 90% by men, and AUDs are regarded as important risk factor (7, 8). Some consider AUDs and suicidal behavior to be dysfunctional coping mechanism products of depression (9), while others with supporting longitudinal data show instead a more complex bidirectional relationship between depression and AUDs (10). Together, these data raise the question of whether there exists a unique and potentially underdiagnosed male-specific pattern of depression that is linked with AUDs and suicidal behavior (3, 11).
KEY CONCEPT 1 Male-specific pattern of depression
The male population generally has lower rates of depression compared to the female population; however, depression in men is associated with higher rates of alcohol dependence and suicide than the rates seen in women. These behavior patterns are thought to represent dysfunctional coping mechanisms in depression, thus creating a unique subcategory of patients.
The assumption of a male depression has generated much investigation in the field of psychiatry. These investigations are high yield in determining how best to reduce male AUDs and suicide rates, particularly in special populations of sociopolitical importance such as war veterans and others (12–14). Despite many efforts, to date, no simple neuropsychiatric model has been proposed to account for men’s increased AUDs and suicide rate relative to their lower rates of depression.
Emerging interest in the interplay between neuropsychiatry and endocrinology may yield an improved cross-disciplinary model to account for a male-specific pattern for depression, its interrelation with AUDs, and suicide. In this mini-review, we intend to examine evidence supporting an underlying neuroendocrine model for a male-specific pattern for depression linked to AUDs and suicidal behavior. Understanding the pathophysiology of a male-specific susceptibility to depression, AUDs, and suicidal behavior might enable the allied mental health fields to develop tailored and highly effective combined treatments consisting of psychotherapeutic and pharmacological interventions.
KEY CONCEPT 2 Cross-disciplinary model
A model integrating scientific methods and evidence from both neuropsychiatry and endocrinology, to promote a better understanding of gender-specific clinical manifestations such as male-specific depression.
Changes in Steroid Hormone Concentrations
Testosterone, the end product of the hypothalamus–pituitary–gonadal (HPG) axis, has been investigated extensively as putative biomarker of depression. Studies indicate that hypogonadal men are more likely to develop depression (15). Testosterone treatment has been shown to exhibit beneficial effects on mood in men (16, 17). These effects may be age-specific as low levels of testosterone seem to be associated with suicidal behavior in older men, while high testosterone levels might be associated with suicidal behavior in youth (18–20).
KEY CONCEPT 3 Hypothalamus–pituitary–gonadal axis
Gonadotropin-releasing hormone is secreted from the hypothalamus and stimulates production of luteinizing hormone (LH) and follicle-stimulating hormone (FSH) in the pituitary gland. LH and FSH then stimulate production of estrogen and testosterone in the gonads. Testosterone tends to be lowered in specific subcategories of men with depression.
KEY CONCEPT 4 Hypothalamus–pituitary–adrenocortical axis
Corticotropin-releasing hormone is secreted from the hypothalamus and stimulates the secretion of adrenocorticotropic hormone (ACTH) in the pituitary gland. ACTH then stimulates the production of cortisol in the adrenal glands. Cortisol tends to be elevated in patients with depression, which is considered a stress-related disease.
Despite these findings and a large body of literature demonstrating a beneficial influence of testosterone on well-being and depression in men (21–24), a recent review concludes that the study of men as a large heterogeneous population yields no consistent relationship between testosterone and mood (25). These studies may lead some to conclude that there is no association between testosterone, depression, and suicide attempts (26). However, statistically significant findings may emerge when examining specific subgroups of men where an association between low testosterone levels and depression is more pronounced. For example, treatment-resistant depressive men, men with major depression and comorbid human immunodeficiency virus infection, hypogonadal men, dysthymic men, and elderly men (>60 years) often have lower testosterone levels (25). These may be specific populations of special importance in exploring the relationship between testosterone to men’s mental health.
Additionally, depressed individuals appear to have a dysregulated and hyperactive hypothalamus–pituitary–adrenocortical (HPA) axis. HPA axis disturbances have been linked to the development and maintenance of depression (27). Depression is considered a stress-related disease with altered glucocorticoid receptor (GR) signaling and reduced glucocorticoid responsiveness (28, 29). Cortisol, the end product of the HPA axis, generally tends to be significantly elevated in depressed subjects (30). Higher circadian cortisol secretion patterns were also found in subgroups of depressed patients compared to healthy controls (31). However, several moderators need to be taken into account, when interpreting the literature on cortisol and depression including methodological (time of day, challenge tests, or body substrate), sample-related (age or symptom severity), and depression-subtype (melancholic, psychotic, or atypical depression) moderators (27). Highest cortisol concentrations in depressed compared to healthy individuals were found when assessing cortisol in the afternoon, using challenge tests, the use of blood or cerebrospinal fluid samples as compared to salivary or urine samples, older age and greater symptom severity/hospitalization status were given, and psychotic or melancholic depression was exhibited.
Other neuroactive steroids such as dehydroepiandrosterone (DHEA) and estradiol are also implicated in the onset and maintenance of depression. These agents may all have potential antidepressant effects. DHEA works concomitantly as a precursor hormone of testosterone or estradiol while also exerting independent effects on different body systems such as the HPA axis (32). Low DHEA levels have consistently been related to depressive symptoms (33). Moreover, the use of DHEA as an antidepressant therapy has shown some success (34). Similarly, low levels of estradiol have been associated with more depressive symptoms in men (35). Carrier and colleagues report concomitant testosterone and estradiol administration to exhibit antidepressant-like effects in male gonadectomized rats, suggesting that testosterone’s protective effect may be mediated, in part, by its aromatization to estradiol (36).
Similarly, in both, chronic alcohol-dependent patients and moderate chronic alcohol consumers reduced testosterone and DHEA levels and increased basal levels of cortisol are reported (37, 38). As alcohol intake contributes to HPA axis activation, chronic heavy alcohol use leads to chronic HPA axis activation accompanied by the loss of normal diurnal cortisol secretion pattern; this persists during withdrawal and is mostly reestablished after 1–4 weeks after abstinence (39). In contrast, alcohol intake inhibits the HPG axis and suppresses testosterone production (40). Testosterone suppression might further contribute to depressed mood leading to disproportionately high suicide rates seen in men with comorbid depression and AUDs (41). Reduced basal androgens and elevated glucocorticoids seem therefore to be a shared endocrine phenotype in male depression and AUDs.
Genetic Risk Constellation
Genetic factors determine steroid secretion and action, while steroids regulate gene expression via intracellular receptor binding (42). For example, testosterone action is modulated by the CAG repeat length polymorphism in the X-chromosome-bound androgen receptor (AR) gene. It is proposed that longer CAG repeat length causes lower transcriptional activity of genes activated by testosterone binding (43). A longer (>23) and a shorter (≤20) than average amount of CAG triplets have been suggested as risk alleles (44). Longer CAG repeat length is associated with more depressive symptoms (45). Simultaneous assessment of the CAG repeat length, testosterone levels, and depressive symptoms showed that low testosterone levels were associated with depression in men with the shorter allele only (46). However, this finding was not replicated (43), and for boys, testosterone was negatively associated with depressive symptoms only when expressing the longer allele (44). In addition, reduced CAG repeat length was associated with increased craving symptoms but was not significantly different between AUD patients and healthy controls (47). The CAG polymorphism may thus be a mediator that warrants consideration in future studies.
KEY CONCEPT 5 Steroid secretion
Different patterns of steroid secretion are associated with different clinical manifestations. For example, low circulating levels of sex steroids increase vulnerability for male depression, and this vulnerability is further increased by high levels of glucocorticoids.
KEY CONCEPT 6 Polymorphism
Differences in the DNA sequence that account for the variation between different individuals. For example, longer CAG repeat has been associated with more depressive symptoms.
The Val66Met polymorphism in the brain-derived neurotrophic factor (BDNF) gene causing deficient BDNF protein secretion affects the neuroplasticity processes crucial for depression (48). Research reports an association between the Val66Met polymorphism and depression (49–52). Sex steroids were shown to increase BDNF protein levels in human neurons suggesting sex steroids to have additional protective effects against depression due to the promotion of neuroplasticity (53). Similar effects were reported for other recently developed antidepressant medications further underlining the importance of increasing neuroplasticity in depressed individuals (54). However, a meta-analysis including 28 studies questions the assumed beneficial effects of BDNF by postulating no association between genetic variants in BDNF and major depression (55). It is possible that BDNF affects depression only via the interaction with other polymorphisms or steroid secretion (56). In addition, in female suicide attempters HPA axis hyperactivity was associated with decreased BDNF (57). A trend toward decreased BDNF serum levels was also reported in AUD patients (58), though these results could not be replicated in another study (59). In sum, throughout the literature one encounters an inconsistent picture for the relationship between BDNF and depressive disorders, AUDs, and suicidal behavior.
More consistent findings have been reported for two common variants in the 5-hydroxytryptamine transporter-linked polymorphic region (5HTTLPR). Homozygous and heterozygous carriers of the short allele variant were found to be at increased risk of major depressive disorder. Notably, homozygous carriers of the short allele are also at increased risk for alcohol dependence (60), lending an interesting biological footprint to the association between AUDs, depression, and suicide in men. Examining the concomitant effects of another polymorphism (C1019G) from the serotonin receptor gene, 5HT1A and the Val66Met polymorphism of the BDNF gene revealed increased risk for depression when expressing both risk variants (61). In addition, carriers of the long allele with concomitant higher levels of testosterone showed lower cortisol secretion after threat indicating neuropsychiatric resilience for this combination in humans (62). A recent review on genetic association studies of suicidal behavior identified 5HTTLPR and BDNF among few others as most promising candidates (63). Male-specific endocrine factors may play a key role in this association.
Recently, a genetic variant within the gene encoding for FK506 binding protein 5 (FKBP5) was shown to be associated with major depression (64). FKBP5 is considered to regulate intracellular GR signaling (65). Furthermore, FKBP5 ablation in mice was shown to increase antidepressant behavior (66). As an example of the delicate system interplays, FKBP5 is a glucocorticoid-induced negative regulator of the GR, yet at the same time, it is a positive regulator of the AR. This concomitant action of FKBP5 suggests that it functions as a reciprocal modulator of glucocorticoid- and androgen-mediated physiology (65).
The gene NR3C1, which encodes the GR, a crucial element for modulation of HPA axis function, has been extensively examined with regard to depression. An in vitro mouse model showed testosterone treatment to downregulate NR3C1 expression linking androgens with NR3C1 inhibition (67). It has been shown that three polymorphisms (rs6198, rs6191, and rs33388) within the NR3C1 resulting in GR resistance are associated with major depression and the predominance of depression in the course of bipolar disorder (68). Three other single nucleotide polymorphisms [SNPs (BclI, N363S, and ER22/23EK)] of NR3C1 were also associated with the increased recurrence of depressive disorders (69). However, there is conflicting literature reporting a lack of association: a 4-year prospective study investigated 683 subjects with major depression in remission with regard to time until recurrence of a major depressive episode. GR polymorphisms (9β, ER22/23EK, BclI, TthIIIl, NR3C1-1, and N363S) were not associated with recurrence of depression (70). Another study investigating the association between polymorphisms in NR3C1 and suicide attempts in 597 affective disorder patients reports no difference between groups with and without a history of suicide attempts (71). Recent studies show SNPs in FKBP5 and NR3C1 to be associated with alcohol drinking and crucial for alcohol abuse interventions (72, 73).
In conclusion, these results indicate subgroup-specific effects of polymorphisms and interaction with other SNPs, and steroid hormone levels to be relevant for a specific pattern for depression linked to AUDs and suicidal behavior in men. Studies show an interchange between candidate genes for depression and those within the endocrine system relevant for circulating levels of androgens and glucocorticoids, which warrant further investigation.
Epigenetic Risk Constellation
Examining potential gene–environment interactions, methylation studies for the aforementioned risk alleles were investigated with regard to a male-specific pattern for depression. To date, methylation of the AR promoter region was primarily investigated in relation to prostate cancer, where DNA hypermethylation of the AR promoter region occurs leading to AR downregulation (74, 75). As low levels of testosterone are associated with depression in men and hypermethylation of the AR promoter region reduces testosterone signaling and action at target cells, hypermethylation of the AR might be associated to neuropsychiatric manifestations in men, including depression, AUDs, and suicidal behavior.
KEY CONCEPT 7 Methylation
The addition of methyl groups to DNA, usually resulting in inhibition of gene transcription.
Brain-derived neurotrophic factor has recently become the focus of methylation studies associated with depression. Several studies indicate promoter methylation of BDNF to be associated with major depression (76). BDNF promoter methylation was shown to be associated with cortical thickness in patients with recurrent major depression (77). Another study revealed that higher BDNF promoter methylation was associated with more depressive symptoms and increased risk of drug addiction (78). Therefore, BDNF promoter methylation might independently contribute to the etiology and maintenance of depression, AUDs, and suicidal behavior in men, and it may mediate the effects of methylation on endocrine system receptors and signaling.
Similarly, homozygous and heterozygous carriers of the short variant of the 5HTTLPR exhibit higher mean 5HTT methylation and have therefore a lower 5HTT expression in peripheral blood mononuclear cells (79). Higher 5HTT methylation, but not HTTLPR polymorphism, was associated with more stress reactivity in infant macaques (80). Patients with depressive disorder were also shown to have a higher mean methylation level of the 5HTT gene than healthy controls (81). However, in patients with alcohol dependence, no different 5HTT methylation pattern was found (82).
FK506 binding protein 5 and NR3C1 were shown to be associated with depression and suicide attempts (83, 84). Roy and colleagues report for a sample consisting predominantly of men (90%) with a history of substance abuse a significant interaction between childhood trauma and variants of FKBP5 to raise the risk of attempting suicide (84). Therefore, their methylation status was further examined with regard to depression, indicating independent effects on risk for depression establishment and severity (31, 83). A 10% higher methylation rate of FKBP5 intron 7 for individuals with a lifetime history of major depression compared to healthy controls was found (85). Maternal depression during pregnancy was associated with NR3C1 hypermethylation in the newborn (86). Early-life stress-induced methylation of the NR3C1 was associated with subsequent demethylation of FKBP5, which thereby links these two stress responsive genes via epigenetic alterations (87). Replication of these findings in exclusively male samples is needed to further elucidate the epigenetic profile of the investigated male-specific pattern for depression. However, combat veterans with diagnosed PTSD often suffer from comorbid depression and AUDs. An intervention study reported that in combat veterans, who were receiving psychotherapy, the methylation of the NR3C1 promoter pretreatment significantly predicted subsequent treatment outcome, while FKBP5 methylation increased with regard to treatment (88).
Taken together, the influence of the gene–environment interaction causes endocrine alterations in depression and AUDs, which are determined by both genetic variants and epigenetic profiles. Examining further genetic variants and epigenetic profiles would be highly interesting to further capture relevant alterations underlying the relationship between depression, AUDs, and suicide in men (89, 90).
Changes in Endocrine Stress Reactivity
Patterns of stress reactivity, or the physiological response of the individual to psychosocial stressors, may also reveal insights into male-specific behavioral health that intertwine with endocrine functioning.
Numerous studies confirmed an increase in cortisol by exposure to acute psychosocial stress (28). A meta-analysis indicates HPA axis response to psychosocial stress of depressed subjects is similar to healthy controls. However, depressed subjects with high basal cortisol were found to have increased cortisol production and higher cortisol levels during psychosocial stress (91). Indeed, in numerous studies reduced responsiveness to glucocorticoids has been reported for depressed patients assessed via the combined dexamethasone-suppression/corticotropin-releasing hormone (DEX-CRH) test indicating impaired GR signaling (29). This is further supported by meta-analytic findings of higher cortisol levels during the recovery period in depressed patients compared to healthy controls, while cortisol secretion patterns during stress were similar (92). This is of particular interest for a male-specific pattern for depression, as men show generally higher HPA responses to psychosocial stress, and estradiol seems to exert buffering effects (93). In contrast, for individuals with AUDs, there are blunted HPA axis responses to psychosocial stress and exogenous CRH provocation (38, 94). Interestingly, in depressed patients with suicidal behavior, blunted responses to the DEX-CRH test were also found in comparison to depressed patients without suicidal behavior (95). The existing data point to a chronic hyperactive HPA axis with a blunted acute stress response for a male-specific pattern for depression linked to AUDs and suicidal behavior.
Recently, sex steroids were also shown to depict a stress-dependent rise (96). However, there are conflicting findings (97). In addition to cortisol, sex steroids might represent additional physiological markers for the stress reactivity after a psychosocial stressor (28, 96). Attenuated DHEA-S response during acute psychosocial stress has been demonstrated in healthy men perceiving stress at work and patients with burnout (98, 99). Studies on testosterone or estradiol with regard to depression and their stress reactivity to psychosocial stress are scarce. Decreased baseline plasma testosterone was seen in adult male rats after an immobilization stress (100), while another study reported an increase in plasma testosterone after immobilization stress (101). In the forced swimming test estradiol significantly increased, while no change was observed for testosterone in rats (102). Taken together, conflicting literature is reported with regard to depressive symptoms and the reactive secretion of sex steroids in response to psychosocial stress.
Integration into the Three-Hit Concept of Vulnerability and Resilience
In conclusion, the three-hit concept of vulnerability and resilience, fully described elsewhere (88), offers a theoretical framework integrating the interactions of the HPA and HPG axis-related polymorphisms, methylation profiles, steroid secretion, and endocrine stress reactivity with regard to depression, AUDs, and suicidal behavior in men.
KEY CONCEPT 8 Three-hit concept of vulnerability and resilience
A proposed model stating that stress in early life can affect behavioral adaptation to stress later in life. The first hit is genetic predisposition; the second hit is early-life environment, which programs phenotypes by epigenetic regulation; and the third hit is later-life challenges.
Genetic risk variants determine an initial vulnerability for depressive disorders, AUDs, and suicidal behavior in men. Hit one represents polymorphisms in the AR, BDNF, 5HTTLPR, FKBP5, and NR3C1, which independently increase the risk of developing depression, AUDs, and suicidal behavior in men. Following the common variant hypothesis, combinations of risk alleles such as a long variant (>23) of the CAG repeat length in the AR, a short variant of the 5HTTLPR, and one of the three polymorphisms of the NR3C1 (rs6198, rs6191, and rs33388) increase the risk for these conditions in men multiplicatively.
Early-life environment and its experience, representing hit two, such as adequate or inadequate nutrition, childhood traumas, and adverse childhood experiences, and optimal or lacking parent–child affective attunement and dyadic regulation create unique phenotypes via the interaction with multigenic input by epigenetic regulation. Epigenetic regulation of HPA or HPG axis-related gene sites help the developing organism adapt to altered environmental conditions. Hypermethylation or hypomethylation of key genes for the development of depression in men, such as the AR, BDNF, 5HTT, FKBP5, and NR3C1 constitute independent risk and resilience factors for depression, AUDs, and suicidal behavior and further interact with the prior described genetic predisposition. This interaction forms a differential susceptibility to later-life challenges.
Later-life environment, such as exposure to trauma or major critical life events such as military combat, prostate cancer, or divorce, is considered hit three. As shown in Figure 1, depending on the interaction of programed phenotypes with later-life challenges, a man would either suffer from depression, AUDs, or suicidal behavior or he would develop mental resilience. Finally, the established pattern of depression, AUDs, and suicidal behavior influences steroid secretion, endocrine stress reactivity, and the epigenetic profile. Therefore, experimental human studies examining HPA and HPG axis activity and function in parallel also in response to stress are needed to untangle the complex interactions between the genetic predisposition, early-life environment, and later-life challenges underlying this male-specific pattern of depression, AUDs, and suicidal behavior.
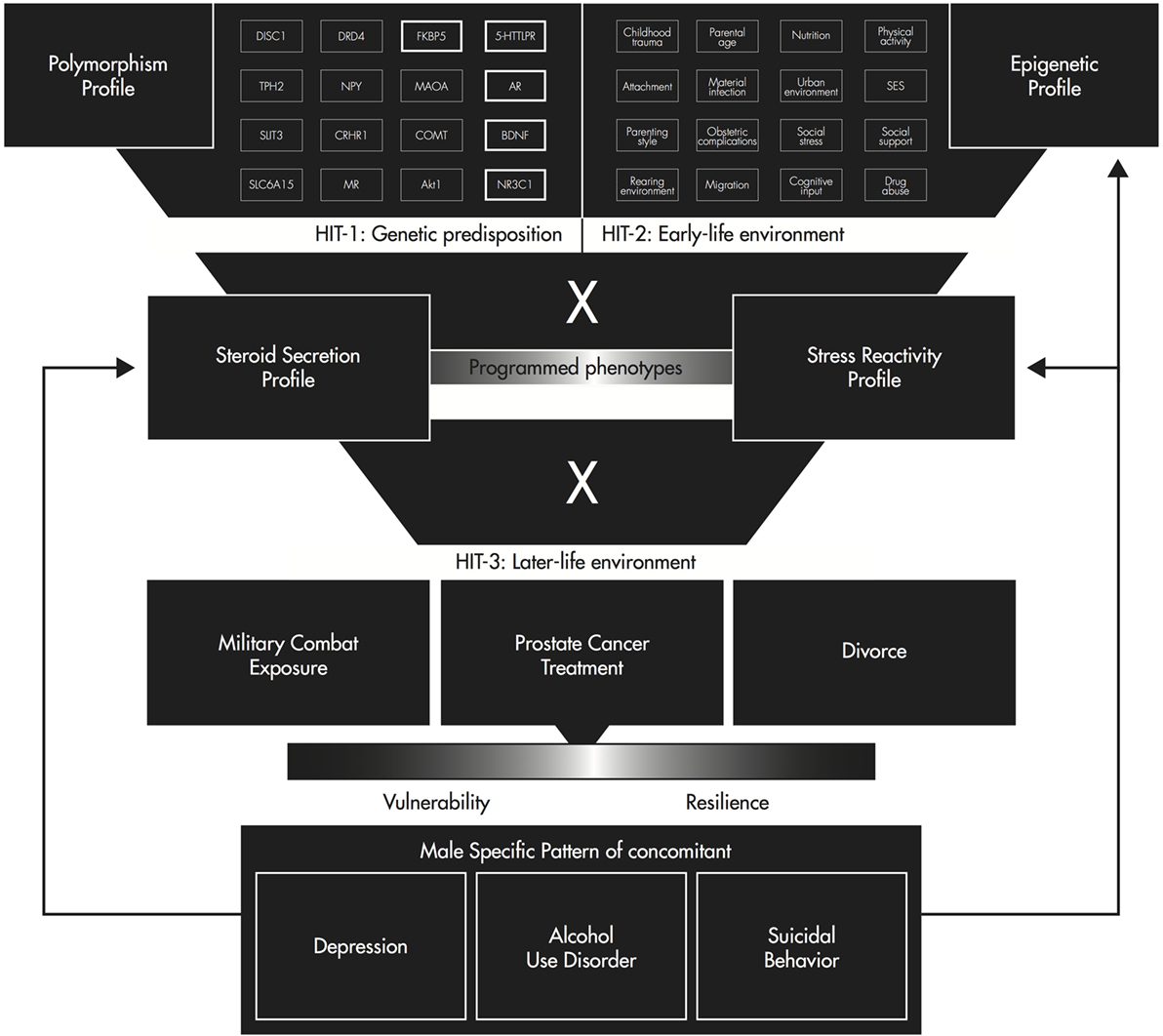
Figure 1. Three-hit concept of vulnerability and resilience for a male-specific pattern of depression, alcohol use disorder, and suicidal behavior [adapted from Ref. (103)].
Limitations
Some limitations should be taken into consideration when interpreting the literature.
The reported studies examined biological differences between patients with depression, AUDs, suicidal behavior, and healthy controls and were not testing our proposed model specifically. Therefore, large-scale studies with male samples are needed to determine the actual overlap in depression, AUDs, and suicidal behavior using male-specific psychometric instruments as suggested by Rice and colleagues (104). We also cannot rule out that the results described here are partially confounded by a publication bias caused by non-publication of null results in this research area. A third limitation is that no restrictions were made with regard to inclusion of studies reporting hormonal data. Circadian rhythmicity, prior activities, intraindividual changes in subsequent days, huge interindividual differences in circulating concentrations, used body substrates, sampling techniques, and hormone-assays are potential confounding issues hindering the establishment of reference ranges. Finally, we examined only genetic, epigenetic, and endocrine data, while additional relevant areas such as immunological and functional neuroimaging data were beyond the purview of this mini-review.
Conclusion
The high rate of AUDs and suicides juxtaposed to the low rate of diagnosed depression in men led to the assumption of an underdiagnosed male depression related to AUDs and suicidal behavior. We here provide evidence for overlapping neuroendocrine conditions underlying these disorders in men adding a biological perspective to theories on socialization and masculinity trying to explain the discrepancy of depression diagnosis in men and women (105). In men, depression, AUDs, and suicidal behavior seem to interact dynamically and to be associated with multiple biological risk factors such as decreased basal androgen and increased glucocorticoid levels, a blunted cortisol stress response and SNPs and hypermethylation/hypomethylation in candidate genes (AR, BDNF, 5HTTLPR, FKBP5, and NR3C1). Once established, these disorders further cause additional dysregulations in the HPA and HPG axes. Further research at the intersection of neuropsychiatry and endocrinology will advance our neurobiologically informed understanding of men’s mental health.
Author Contributions
AW conducted a systematic review of the topics literature, which he integrated in a first draft of this mini-review. He subsequently reviewed further editing from coauthors. TR substantially contributed to the conception of the work, included further literature, and edited the first draft. YK critically reviewed the literature used, updated it, and edited a subsequent draft. UE critically reviewed the manuscript and edited it to its final form.
Conflict of Interest Statement
The authors declare that the research was conducted in the absence of any commercial or financial relationships that could be construed as a potential conflict of interest.
The reviewer NK and handling Editor declared their shared affiliation, and the handling Editor states that the process nevertheless met the standards of a fair and objective review.
Funding
There was no funding supporting the development of this manuscript.
References
1. Baer N, Schuler D, Füglister-Dousse S, Moreau-Gruet F. Depressionen in der Schweiz. Daten zur Epidemiologie, Behandlung und sozial-beruflichen Integration (Obsan Bericht 56). Neuchâtel: Schweizerisches Gesundheitsobservatorium (2013). 56 p.
2. World Federation For Mental Health (WFMH). Depression: A Global Crisis. World Mental Health Day. (2012). 32 p. Available from: http://www.who.int/mental_health/management/depression/wfmh_paper_depression_wmhd_2012.pdf
3. Oliffe JL, Phillips M. Depression, men and masculinities: a review and recommendations. J Men’s Health (2008) 5:194–202. doi:10.1016/j.jomh.2008.03.016
4. Merikangas KR, McClair VL. Epidemiology of substance use disorders. Hum Genet (2012) 131:779–89. doi:10.1007/s00439-012-1168-0
5. Oliffe JL, Hannan-Leith MN, Ogrodniczuk JS, Black N, Mackenzie CS, Lohan M, et al. Men’s depression and suicide literacy: a nationally representative Canadian survey. J Ment Health (2016) 25(6):520–6. doi:10.1080/09638237.2016.1177770
6. Kaplan MS, McFarland BH, Huguet N, Conner K, Caetano R, Giesbrecht N, et al. Acute alcohol intoxication and suicide: a gender-stratified analysis of the National Violent Death Reporting System. Inj Prev (2012) 19(1):38–43. doi:10.1136/injuryprev-2012-040317
7. Pompili M, Serafini G, Innamorati M, Montebovi F, Palermo M, Campi S, et al. Car accidents as a method of suicide: a comprehensive overview. Forensic Sci Int (2012) 223:1–9. doi:10.1016/j.forsciint.2012.04.012
8. Gauthier S, Reisch T, Ajdacic-Gross V, Bartsch C. Road traffic suicide in Switzerland. Traffic Inj Prev (2015) 16(8):768–72. doi:10.1080/15389588.2015.1021419
9. Kopp MS, Réthelyi J. Where psychology meets physiology: chronic stress and premature mortality – the Central-Eastern European health paradox. Brain Res Bull (2004) 62:351–67. doi:10.1016/j.brainresbull.2003.12.001
10. Brière FN, Rohde P, Seeley JR, Klein D, Lewinsohn PM. Comorbidity between major depression and alcohol use disorder from adolescence to adulthood. Compr Psychiatry (2014) 55:526–33. doi:10.1016/j.comppsych.2013.10.007
11. Kilmartin C. Depression in men: communication, diagnosis and therapy. J Men’s Health Gend (2005) 2:95–9. doi:10.1016/j.jmhg.2004.10.010
12. Rice TR, Sher L. Suicidal behavior in war veterans. Expert Rev Neurother (2012) 12:611–24. doi:10.1586/ern.12.31
13. Kimbrel NA, Meyer EC, DeBeer BB, Gulliver SB, Morissette SB. A 12-month prospective study of the effects of PTSD-depression comorbidity on suicidal behavior in Iraq/Afghanistan-era veterans. Psychiatry Res (2016) 243:97–9. doi:10.1016/j.psychres.2016.06.011
14. Ralevski E, Southwick S, Jackson E, Jane JS, Russo M, Petrakis I. Trauma- and stress-induced response in veterans with alcohol dependence and comorbid post-traumatic stress disorder. Alcohol Clin Exp Res (2016) 40(8):1–9. doi:10.1111/acer.13120
15. Westley CJ, Amdur RL, Irwig MS. High rates of depression and depressive symptoms among men referred for borderline testosterone levels. J Sex Med (2015) 12:1753–60. doi:10.1111/jsm.12937
16. Snyder PJ, Bhasin S, Cunningham GR, Matsumoto AM, Stephens-Shields AJ, Cauley JA, et al. Effects of testosterone treatment in older men. N Engl J Med (2016) 374:611–24. doi:10.1056/NEJMoa1506119
17. Wainwright SR, Workman JL, Tehrani A, Hamson DK, Chow C, Lieblich SE, et al. Testosterone has antidepressant-like efficacy and facilitates imipramine-induced neuroplasticity in male rats exposed to chronic unpredictable stress. Horm Behav (2016) 79:58–69. doi:10.1016/j.yhbeh.2016.01.001
18. Sher L. Low testosterone levels may be associated with suicidal behavior in older men while high testosterone levels may be related to suicidal behavior in adolescents and young adults: a hypothesis. Int J Adolesc Med Health (2013) 25:263–8. doi:10.1515/ijamh-2013-0060
19. Rice TR, Sher L. Adolescent suicide and testosterone. Int J Adolesc Med Health (2015) 27(4):1–7. doi:10.1515/ijamh-2015-0058
20. Rice TR, Sher L. Low testosterone levels in aging men may mediate the observed increase in suicide in this age group. Int J Disabil Hum Dev. doi:10.1515/ijdhd-2016-0007
21. Walther A, Ehlert U. Steroid secretion and psychological well-being in men 40+. In: Rice T, Sher L, editors. Neurobiology of Men’s Mental Health. New York: Nova (2015). p. 287–322.
22. Yeap BB. Hormonal changes and their impact on cognition and mental health of ageing men. Maturitas (2014) 79:227–35. doi:10.1016/j.maturitas.2014.05.015
23. Khera M. Male hormones and men’s quality of life. Curr Opin Urol (2016) 26(2):152–7. doi:10.1097/MOU.0000000000000256
24. Walther A, Phillip M, Lozza N, Ehlert U. The rate of change in declining steroid hormones: a new parameter of healthy aging in men? Oncotarget (2016) 7:1–28. doi:10.18632/oncotarget.11752
25. Johnson JM, Nachtigall LB, Stern TA. The effect of testosterone levels on mood in men: a review. Psychosomatics (2013) 54:509–14. doi:10.1016/j.psym.2013.06.018
26. Perez-Rodriguez MM, Lopez-Castroman J, Martinez-Vigo M, Diaz-Sastre C, Ceverino A, Núñez-Beltrán A, et al. Lack of association between testosterone and suicide attempts. Neuropsychobiology (2011) 63:125–30. doi:10.1159/000318085
27. Stetler C, Miller GE. Depression and hypothalamic-pituitary-adrenal activation: a quantitative summary of four decades of research. Psychosom Med (2011) 73:114–26. doi:10.1097/PSY.0b013e31820ad12b
28. Kudielka BM, Wüst S. Human models in acute and chronic stress: assessing determinants of individual hypothalamus-pituitary-adrenal axis activity and reactivity. Stress (2010) 13:1–14. doi:10.3109/10253890902874913
29. Raison CL, Miller AH. When not enough is too much: the role of insufficient glucocorticoid signaling in the pathophysiology of stress-related disorders. Am J Psychiatry (2003) 160:1554–65. doi:10.1176/appi.ajp.160.9.1554
30. Ehlert U, Gaab J, Heinrichs M. Psychoneuroendocrinological contributions to the etiology of depression, posttraumatic stress disorder, and stress-related bodily disorders: the role of the hypothalamus-pituitary-adrenal axis. Biol Psychol (2001) 57:141–52. doi:10.1016/S0301-0511(01)00092-8
31. Menke A, Klengel T, Rubel J, Brückl T, Pfister H, Lucae S, et al. Genetic variation in FKBP5 associated with the extent of stress hormone dysregulation in major depression. Genes Brain Behav (2013) 12:289–96. doi:10.1111/gbb.12026
33. Samaras N, Papadopoulou MA, Samaras D, Ongaro F. Off-label use of hormones as an antiaging strategy: a review. Clin Interv Aging (2014) 9:1175–86. doi:10.2147/CIA.S48918
34. Herbert J. Cortisol and depression: three questions for psychiatry. Psychol Med (2013) 43:449–69. doi:10.1017/S0033291712000955
35. Castanho TC, Moreira PS, Portugal-Nunes C, Novais A, Costa PS, Palha JA, et al. The role of sex and sex-related hormones in cognition, mood and well-being in older men and women. Biol Psychol (2014) 103:158–66. doi:10.1016/j.biopsycho.2014.08.015
36. Carrier N, Saland SK, Duclot F, He H, Mercer R, Kabbaj M. The anxiolytic and antidepressant-like effects of testosterone and estrogen in gonadectomized male rats. Biol Psychiatry (2015) 78(4):259–69. doi:10.1016/j.biopsych.2014.12.024
37. Kumari S, Mittal A, Dabur R. Moderate alcohol consumption in chronic form enhances the synthesis of cholesterol and C-21 steroid hormones, while treatment with Tinospora cordifolia modulate these events in men. Steroids (2016) 114:68–77. doi:10.1016/j.steroids.2016.03.016
38. Blaine SK, Milivojevic V, Fox H, Sinha R. Alcohol effects on stress pathways: impact on craving and relapse risk. Can J Psychiatry (2016) 61:145–53. doi:10.1177/0706743716632512
39. Lovallo WR. Cortisol secretion patterns in addiction and addiction risk. Int J Psychophysiol (2006) 59:195–202. doi:10.1016/j.ijpsycho.2005.10.007
40. Emanuele MA, Emanuele N. Alcohol and the male reproductive system. Alcohol Res Health (2001) 25:282–7.
41. Cornelius JR, Salloum IM, Mezzich J, Cornelius MD, Fabrega H Jr, Ehler JG, et al. Disproportionate suicidality in patients with comorbid major depression and alcoholism. Am J Psychiatry (1995) 152:358–64. doi:10.1176/ajp.152.3.358
42. Beato M. Gene regulation by steroid hormones. Gene Expr. (1993). 27 p. Available from: http://link.springer.com/chapter/10.1007/978-1-4684-6811-3_3
43. Schneider G, Zitzmann M, Gromoll J, Ladwig KH, Berger K. The relation between sex hormone levels, the androgen receptor CAGn-polymorphism and depression and mortality in older men in a community study. Psychoneuroendocrinology (2013) 38:2083–90. doi:10.1016/j.psyneuen.2013.03.016
44. Vermeersch H, T’Sjoen G, Kaufman JM, Vincke J, Van Houtte M. Testosterone, androgen receptor gene CAG repeat length, mood and behaviour in adolescent males. Eur J Endocrinol (2010) 163:319–28. doi:10.1530/EJE-10-0090
45. Schneider G, Nienhaus K, Gromoll J, Heuft G, Nieschlag E, Zitzmann M. Depressive symptoms in men aged 50 years and older and their relationship to genetic androgen receptor polymorphism and sex hormone levels in three different samples. Am J Geriatr Psychiatry (2011) 19:274–83. doi:10.1097/JGP.0b013e3181e70c22
46. Seidman SN, Araujo AB, Roose SP, McKinlay JB. Testosterone level, androgen receptor polymorphism, and depressive symptoms in middle-aged men. Biol Psychiatry (2001) 50:371–6. doi:10.1016/S0006-3223(01)01148-9
47. Lenz B, Jacob C, Frieling H, Jacobi A, Hillemacher T, Muschler M, et al. Polymorphism of the long polyglutamine tract in the human androgen receptor influences craving of men in alcohol withdrawal. Psychoneuroendocrinology (2009) 34:968–71. doi:10.1016/j.psyneuen.2009.01.009
48. Notaras M, Hill R, van den Buuse M. The BDNF gene Val66Met polymorphism as a modifier of psychiatric disorder susceptibility: progress and controversy. Mol Psychiatry (2015) 20:916–30. doi:10.1038/mp.2015.27
49. Yu H, Wang DD, Wang Y, Liu T, Lee FS, Chen Z-Y. Variant brain-derived neurotrophic factor Val66Met polymorphism alters vulnerability to stress and response to antidepressants. J Neurosci (2012) 32:4092–101. doi:10.1523/JNEUROSCI.5048-11.2012
50. Schmidt HD, Duman RS. Peripheral BDNF produces antidepressant-like effects in cellular and behavioral models. Neuropsychopharmacology (2010) 35:2378–91. doi:10.1038/npp.2010.114
51. Pezawas L, Verchinski BA, Mattay VS, Callicott JH, Kolachana BS, Straub RE, et al. The brain-derived neurotrophic factor val66met polymorphism and variation in human cortical morphology. J Neurosci (2004) 24:10099–102. doi:10.1523/JNEUROSCI.2680-04.2004
52. Soliman F, Glatt CE, Bath KG, Levita L, Jones RM, Pattwell SS, et al. A genetic variant BDNF polymorphism alters extinction learning in both mouse and human. Science (2010) 327:863–6. doi:10.1126/science.1181886
53. Wang S, Freeman MR, Sathish V, Thompson MA, Pabelick CM, Prakash YS. Sex steroids influence brain-derived neurotropic factor secretion from human airway smooth muscle cells. J Cell Physiol (2015) 231(7):1586–92. doi:10.1002/jcp.25254
54. Pompili M, Serafini G, Innamorati M, Venturini P, Fusar-Poli P, Sher L, et al. Agomelatine, a novel intriguing antidepressant option enhancing neuroplasticity: a critical review. World J Biol Psychiatry (2013) 14:412–31. doi:10.3109/15622975.2013.765593
55. Gyekis JP, Yu W, Dong S, Wang H, Qian J, Kota P, et al. No association of genetic variants in BDNF with major depression: a meta- and gene-based analysis. Am J Med Genet B Neuropsychiatr Genet (2013) 162:61–70. doi:10.1002/ajmg.b.32122
56. Sun N, Yang C, Liu Z, Li X, Xu Y, Zhang K. Effects of polymorphisms of serotonin transporter promoter (5-HTTLPR) and brain derived neurotrophic factor gene (G196A rs6265) on the risk of major depressive disorder in the Chinese Han population. Eur Rev Med Pharmacol Sci (2016) 20:1852–9.
57. Ambrus L, Lindqvist D, Träskman-Bendz L, Westrin Å. Hypothalamic–pituitary–adrenal axis hyperactivity is associated with decreased brain derived neurotrophic factor in female suicide attempters. Nord J Psychiatry (2016) 9488:1–7. doi:10.1080/08039488.2016.1184310
58. Huang MC, Chen CH, Chen CH, Liu SC, Ho CJ, Shen WW, et al. Alterations of serum brain-derived neurotrophic factor levels in early alcohol withdrawal. Alcohol Alcohol (2007) 43:241–5. doi:10.1093/alcalc/agm172
59. Heberlein A, Muschler M, Wilhelm J, Frieling H, Lenz B, Gröschl M, et al. BDNF and GDNF serum levels in alcohol-dependent patients during withdrawal. Prog Neuropsychopharmacol Biol Psychiatry (2010) 34:1060–4. doi:10.1016/j.pnpbp.2010.05.025
60. Oo KZ, Aung YK, Jenkins MA, Win AK. Associations of 5HTTLPR polymorphism with major depressive disorder and alcohol dependence: a systematic review and meta-analysis. Aust N Z J Psychiatry (2016) 50(9):842–57. doi:10.1177/0004867416637920
61. Anttila S, Huuhka K, Huuhka M, Rontu R, Hurme M, Leinonen E, et al. Interaction between 5-HT1A and BDNF genotypes increases the risk of treatment-resistant depression. J Neural Transm (2007) 114:1065–8. doi:10.1007/s00702-007-0705-9
62. Josephs RA, Telch MJ, Hixon JG, Evans JJ, Lee H, Knopik VS, et al. Genetic and hormonal sensitivity to threat: testing a serotonin transporter genotype. Psychoneuroendocrinology (2012) 37:752–61. doi:10.1016/j.psyneuen.2011.09.006
63. Mirkovic B, Laurent C, Podlipski M-A, Frebourg T, Cohen D, Gerardin P. Genetic association studies of suicidal behavior: a review of the past 10 years, progress, limitations, and future directions. Front Psychiatry (2016) 7:158. doi:10.3389/fpsyt.2016.00158
64. Szczepankiewicz A, Leszczyńska-Rodziewicz A, Pawlak J, Narozna B, Rajewska-Rager A, Wilkosc M, et al. FKBP5 polymorphism is associated with major depression but not with bipolar disorder. J Affect Disord (2014) 164:33–7. doi:10.1016/j.jad.2014.04.002
65. Stechschulte LA, Sanchez ER. FKBP51 – a selective modulator of glucocorticoid and androgen sensitivity. Curr Opin Pharmacol (2011) 11:332–7. doi:10.1016/j.coph.2011.04.012
66. O’Leary JC, Dharia S, Blair LJ, Brady S, Johnson AG, Peters M, et al. A new anti-depressive strategy for the elderly: ablation of FKBP5/FKBP51. PLoS One (2011) 6(9):e24840. doi:10.1371/journal.pone.0024840
67. Delic D, Grosser C, Dkhil M, Al-Quraishy S, Wunderlich F. Testosterone-induced upregulation of miRNAs in the female mouse liver. Steroids (2010) 75:998–1004. doi:10.1016/j.steroids.2010.06.010
68. Szczepankiewicz A, Leszczyńska-Rodziewicz A, Pawlak J, Rajewska-Rager A, Dmitrzak-Weglarz M, Wilkosc M, et al. Glucocorticoid receptor polymorphism is associated with major depression and predominance of depression in the course of bipolar disorder. J Affect Disord (2011) 134:138–44. doi:10.1016/j.jad.2011.06.020
69. Gałecka E, Szemraj J, Bieńkiewicz M, Majsterek I, Przybyłowska-Sygut K, Gałecki P, et al. Single nucleotide polymorphisms of NR3C1 gene and recurrent depressive disorder in population of Poland. Mol Biol Rep (2013) 40:1693–9. doi:10.1007/s11033-012-2220-9
70. Hardeveld F, Spijker J, Peyrot WJ, de Graaf R, Hendriks SM, Nolen WA, et al. Glucocorticoid and mineralocorticoid receptor polymorphisms and recurrence of major depressive disorder. Psychoneuroendocrinology (2015) 55:154–63. doi:10.1016/j.psyneuen.2015.02.013
71. Leszczyńska-Rodziewicz A, Szczepankiewicz A, Pawlak J, Dmitrzak-Weglarz M, Hauser J. Association, haplotype, and gene-gene interactions of the HPA axis genes with suicidal behaviour in affective disorders. ScientificWorldJournal (2013) 2013:207361. doi:10.1155/2013/207361
72. Zheng Y, Albert D, McMahon RJ, Dodge K, Dick D. Glucocorticoid receptor (NR3C1) gene polymorphism moderate intervention effects on the developmental trajectory of African-American adolescent alcohol abuse. Prev Sci (2016) 1–11. doi:10.1007/s11121-016-0726-4
73. Qiu B, Luczak SE, Wall TL, Kirchhoff AM, Xu Y, Eng MY, et al. The FKBP5 gene affects alcohol drinking in knockout mice and is implicated in alcohol drinking in humans. Int J Mol Sci (2016) 17(8):1271–88. doi:10.3390/ijms17081271
74. Cai C, Yuan X, Balk SP. Androgen receptor epigenetics. Transl Androl Urol (2013) 2:148–57. doi:10.3978/j.issn.2223-4683.2013.09.02
75. Massie CE, Mills IG, Lynch AG. The importance of DNA methylation in prostate cancer development. J Steroid Biochem Mol Biol (2016) 16:1–15. doi:10.1016/j.jsbmb.2016.04.009
76. Guintivano J, Arad M, Gould TD, Payne JL, Kaminsky ZA. Antenatal prediction of postpartum depression with blood DNA methylation biomarkers. Mol Psychiatry (2014) 19:560–7. doi:10.1038/mp.2013.62
77. Na K-S, Won E, Kang J, Chang HS, Yoon H-K, Tae WS, et al. Brain-derived neurotrophic factor promoter methylation and cortical thickness in recurrent major depressive disorder. Sci Rep (2016) 6:21089. doi:10.1038/srep21089
78. Xu X, Ji H, Liu G, Wang Q, Liu H, Shen W, et al. A significant association between BDNF promoter methylation and the risk of drug addiction. Gene (2016) 584:54–9. doi:10.1016/j.gene.2016.03.010
79. Hranilovic D, Stefulj J, Schwab S, Borrmann-Hassenbach M, Albus M, Jernej B, et al. Serotonin transporter promoter and intron 2 polymorphisms: relationship between allelic variants and gene expression. Biol Psychiatry (2004) 55:1090–4. doi:10.1016/j.biopsych.2004.01.029
80. Kinnally EL, Capitanio JP, Leibel R, Deng L, Leduc C, Haghighi F, et al. Epigenetic regulation of serotonin transporter expression and behavior in infant Rhesus macaques. Genes Brain Behav (2010) 9:575–82. doi:10.1111/j.1601-183X.2010.00588.x
81. Iga J, Watanabe S, Numata S, Umehara H, Nishi A, Kinoshita M, et al. Association study of polymorphism in the serotonin transporter gene promoter, methylation profiles, and expression in patients with major depressive disorder. Hum Psychopharmacol (2016) 31:193–9. doi:10.1002/hup.2527
82. Park BL, Lee B, Jung KH, Jung MH, Park BL, Chai YG, et al. Epigenetic changes of serotonin transporter in the patients with alcohol dependence: methylation of an serotonin transporter promoter CpG island. Psychiatry Investig (2011) 8:130–3. doi:10.4306/pi.2011.8.2.130
83. Lopizzo N, Bocchio Chiavetto L, Cattane N, Plazzotta G, Tarazi FI, Pariante CM, et al. Gene–environment interaction in major depression: focus on experience-dependent biological systems. Front Psychiatry (2015) 6:68. doi:10.3389/fpsyt.2015.00068
84. Roy A, Gorodetsky E, Yuan Q, Goldman D, Enoch MA. Interaction of FKBP5, a stress-related gene, with childhood trauma increases the risk for attempting suicide. Neuropsychopharmacology (2010) 35:1674–83. doi:10.1038/npp.2009.236
85. Hohne N, Poidinger M, Merz F, Pfister H, Bruckl T, Zimmermann P, et al. FKBP5 genotype-dependent DNA methylation and mRNA regulation after psychosocial stress in remitted depression and healthy controls. Int J Neuropsychopharmacol (2015) 18:yu087–087. doi:10.1093/ijnp/pyu087
86. Conradt E, Lester BM, Appleton AA, Armstrong DA, Marsit CJ. The roles of DNA methylation of NR3C1 and 11β-HSD2 and exposure to maternal mood disorder in utero on newborn neurobehavior. Epigenetics (2013) 8:1321–9. doi:10.4161/epi.26634
87. Bockmühl Y, Patchev AV, Madejska A, Hoffmann A, Sousa JC, Sousa N, et al. Methylation at the CpG island shore region upregulates Nr3c1 promoter activity after early-life stress. Epigenetics (2015) 10:247–57. doi:10.1080/15592294.2015.1017199
88. Yehuda R, Daskalakis NP, Desarnaud F, Makotkine I, Lehrner AL, Koch E, et al. Epigenetic biomarkers as predictors and correlates of symptom improvement following psychotherapy in combat veterans with PTSD. Front Psychiatry (2013) 4:118. doi:10.3389/fpsyt.2013.00118
89. Wingo AP, Almli LM, Stevens JJ, Klengel T, Uddin M, Li Y, et al. DICER1 and microRNA regulation in post-traumatic stress disorder with comorbid depression. Nat Commun (2015) 6:10106. doi:10.1038/ncomms10106
90. Kaminsky Z, Wilcox HC, Eaton WW, Van Eck K, Kilaru V, Jovanovic T, et al. Epigenetic and genetic variation at SKA2 predict suicidal behavior and post-traumatic stress disorder. Transl Psychiatry (2015) 5:e627. doi:10.1038/tp.2015.105
91. Ciufolini S, Dazzan P, Kempton MJ, Pariante C, Mondelli V. HPA axis response to social stress is attenuated in schizophrenia but normal in depression: evidence from a meta-analysis of existing studies. Neurosci Biobehav Rev (2015) 47:359–68. doi:10.1016/j.neubiorev.2014.09.004
92. Burke HM, Davis MC, Otte C, Mohr DC. Depression and cortisol responses to psychological stress: a meta-analysis. Psychoneuroendocrinology (2005) 30:846–56. doi:10.1016/j.psyneuen.2005.02.010
93. Kudielka BM, Hellhammer J, Hellhammer DH, Wolf OT, Pirke KM, Varadi E, et al. Sex differences in endocrine and psychological responses to psychosocial stress in healthy elderly subjects and the impact of a 2-week dehydroepiandrosterone treatment. J Clin Endocrinol Metab (1998) 83:1756–61. doi:10.1210/jcem.83.5.4758
94. Sher L. Combined dexamethasone suppression-corticotropin-releasing hormone stimulation test in studies of depression, alcoholism, and suicidal behavior. ScientificWorldJournal (2006) 6:1398–404. doi:10.1100/tsw.2006.251
95. Pfennig A, Kunzel HE, Kern N, Ising M, Majer M, Fuchs B, et al. Hypothalamus-pituitary-adrenal system regulation and suicidal behavior in depression. Biol Psychiatry (2005) 57:336–42. doi:10.1016/j.biopsych.2004.11.017
96. Lennartsson A-K, Kushnir MM, Bergquist J, Billig H, Jonsdottir IH. Sex steroid levels temporarily increase in response to acute psychosocial stress in healthy men and women. Int J Psychophysiol (2012) 84:246–53. doi:10.1016/j.ijpsycho.2012.03.001
97. Schoofs D, Wolf OT. Are salivary gonadal steroid concentrations influenced by acute psychosocial stress? A study using the Trier Social Stress Test (TSST). Int J Psychophysiol (2011) 80:36–43. doi:10.1016/j.ijpsycho.2011.01.008
98. Lennartsson AK, Sjörs A, Jonsdottir IH. Indication of attenuated DHEA-s response during acute psychosocial stress in patients with clinical burnout. J Psychosom Res (2015) 79:107–11. doi:10.1016/j.jpsychores.2015.05.011
99. Lennartsson AK, Theorell T, Kushnir MM, Bergquist J, Jonsdottir IH. Perceived stress at work is associated with attenuated DHEA-S response during acute psychosocial stress. Psychoneuroendocrinology (2013) 38:1650–7. doi:10.1016/j.psyneuen.2013.01.010
100. Collu R, Gibb W, Ducharme JR. Role of catecholamines in the inhibitory effect of immobilization stress on testosterone secretion in rats. Biol Reprod (1984) 30:416–22. doi:10.1095/biolreprod30.2.416
101. Almeida SA, Petenusci SO, Franci JA, Rosa E, Silva AA, Lamano Carvalho TL. Chronic immobilization-induced stress increases plasma testosterone and delays testicular maturation in pubertal rats. Andrologia (2000) 32:7–11. doi:10.1111/j.1439-0272.2000.tb02858.x
102. Martinez-Mota L, Ulloa RE, Herrera-Perez J, Chavira R, Fernandez-Guasti A. Sex and age differences in the impact of the forced swimming test on the levels of steroid hormones. Physiol Behav (2011) 104:900–5. doi:10.1016/j.physbeh.2011.05.027
103. Daskalakis NP, Bagot RC, Parker KJ, Vinkers CH, de Kloet ER. The three-hit concept of vulnerability and resilience: toward understanding adaptation to early-life adversity outcome. Psychoneuroendocrinology (2013) 38:1858–73. doi:10.1016/j.psyneuen.2013.06.008
104. Rice SM, Fallon BJ, Aucote HM, Möller-Leimkühler AM. Development and preliminary validation of the male depression risk scale: furthering the assessment of depression in men. J Affect Disord (2013) 151:950–8. doi:10.1016/j.jad.2013.08.013
Keywords: male depression, alcohol use disorder, suicidal behavior, steroid secretion, polymorphism, methylation, stress reactivity, vulnerability
Citation: Walther A, Rice T, Kufert Y and Ehlert U (2017) Neuroendocrinology of a Male-Specific Pattern for Depression Linked to Alcohol Use Disorder and Suicidal Behavior. Front. Psychiatry 7:206. doi: 10.3389/fpsyt.2016.00206
Received: 12 October 2016; Accepted: 15 December 2016;
Published: 03 January 2017
Edited by:
Paul Stokes, King’s College London, UKReviewed by:
Casimiro Cabrera Abreu, Queen’s University and Providence Care, CanadaGianluca Serafini, University of Genoa, Italy
Nicola Kalk, King’s College London, UK
Copyright: © 2017 Walther, Rice, Kufert and Ehlert. This is an open-access article distributed under the terms of the Creative Commons Attribution License (CC BY). The use, distribution or reproduction in other forums is permitted, provided the original author(s) or licensor are credited and that the original publication in this journal is cited, in accordance with accepted academic practice. No use, distribution or reproduction is permitted which does not comply with these terms.
*Correspondence: Ulrike Ehlert, dS5laGxlcnRAcHN5Y2hvbG9naWUudXpoLmNo