- 1The Second Affiliated Hospital of Xinxiang Medical University, Xinxiang, China
- 2Xinxiang Key Laboratory of Multimodal Brain Imaging, Xinxiang, China
- 3School of Psychology, Xinxiang Medical University, Xinxiang, China
- 4Henan Key Lab of Biological Psychiatry of Xinxiang Medical University, Xinxiang, China
- 5International Joint Research Laboratory for Psychiatry and Neuroscience of Henan, Xinxiang, China
Background: Altered resting-state functional connectivity of the cerebellum in obsessive-compulsive disorder (OCD) has been previously reported. However, the previous study investigating cerebellar–cerebral functional connectivity relied on a priori–defined seeds from specific networks. In this study, we aimed to explore the connectivity alterations of the cerebellum in OCD under resting-state conditions with a hypothesis-free approach.
Methods: Thirty patients with OCD and 26 healthy controls (HCs) underwent functional magnetic resonance imaging (fMRI) scanning at resting state. Regional cerebral function was evaluated by measuring the fraction of amplitude of low-frequency fluctuation (fALFF). Regions with mean fALFF (mfALFF) alterations were used as seeds in seed correlation analysis (SCA). An independent samples t test was used to compare the differences in mfALFF and functional connection (FC) between the two groups. Pearson correlation analysis was performed to identify the association between functional neural correlates and OCD symptom severity evaluated using the Yale-Brown Obsessive Compulsive Scale (Y-BOCS).
Results: Compared with the HC group, the OCD group showed significantly increased mfALFF values in bilateral cerebellar. The results of FC analysis showed weakened connectivity among the left Crus II, lobule VIII, and right striatum and between the right lobule VIII and the right striatum, and cingulate in the OCD group compared with the HC group. Some of the abovementioned results were associated with symptom severity.
Conclusions: OCD patients showed abnormal spontaneous cerebellar activity and weakened functional connectivity between the cerebellum and the cortico-striato-thalamo-cortical (CSTC) circuit (striatum and cingulate), suggesting that the cerebellum may play an essential role in the pathophysiology of OCD.
Introduction
Obsessive-compulsive disorder (OCD) is a common psychiatric condition, with a lifetime prevalence of 1% to 3% in the general population (1, 2). The core symptoms of OCD are obsessions and compulsions. OCD patients also experience deterioration of cognitive functions, such as attention, memory, decision-making ability, and inhibitory control (3, 4).
Multiple lines of evidence indicate that cortico-striato-thalamo-cortical (CSTC) circuit dysfunction is the core pathophysiological feature of OCD (5). In the CSTC circuit, the direct pathway projects from the prefrontal cortex to the striatum, then to the thalamus, and back to the prefrontal cortex (6, 7). Hyperactivity of the direct pathway is thought to lead to hyperactivation of the orbitofrontal cortex. Thus, excessive concern about danger, health, or injury mediated by the orbitofrontal cortex may lead to sustained conscious attention to perceived threats in OCD patients, leading to compulsive behavior aimed at eliminating perceived threats. The results of functional imaging studies support the above views. The most consistent findings in functional imaging studies of OCD pertain to abnormally increased activation of the lateral prefrontal cortex, including the orbitofrontal cortex and anterior cingulate (8, 9). Similarly, hyperactivity in the striatum and thalamus has been reported in OCD patients (10). However, recent studies have shown that OCD patients have structural and functional abnormalities in the cerebellum (11, 12), including increased cerebellar volume (12) and enhanced spontaneous cerebellar activity under resting state (13). The cerebellum is commonly associated with motor regulation, but recent experimental evidence suggests that it may also play a key role in cognition and emotion (14). Current evidence suggests that separate cerebellar regions are connected with different cerebral areas to form multiple cognitive circuits with topographic functions (15, 16). These functions include attention, language, working memory, visuospatial processing, and decision-making (14), which have been reported to be deficient in OCD patients (17, 18). This information is inevitably reminiscent of the role of cerebellar–cerebral functional connectivity in OCD.
The amplitude of low-frequency fluctuations (ALFFs) for the regional blood oxygen level-dependent (BOLD) signal, which is a method to investigate the regional spontaneous activity by calculating the square root of the power spectrum in the frequency range (19, 20), has been widely used in neuroimaging studies (21–23). The fractional ALFF (fALFF) approach (24) is an improved ALFF method, which measures the ratio of power spectrum of low-frequency range to that of the whole frequency range. Non-specific signal components could be effectively suppressed by this technology, and the sensitivity and specificity in examining regional spontaneous brain activity could be significantly improved. At present, this method has been successfully applied to the study of brain function (25–27). In addition, fALFF is also used in the selection of seed in functional connection analysis (28).
Seed correlation analysis (SCA) is a method of seed activation based on computed correlations with other areas of the brain that have similar temporal pathways, providing relatively precise and specific FC. This method has proven to be effective in revealing features of the brain connection network in psychiatric diseases and has been widely adopted (29, 30). In general, the seed can be selected based on a previous hypothesis or a collection of points based on prior fMRI studies. Currently, cerebellar–cerebral functional connectivity studies based on previous seed hypotheses have been reported, and they found that the cerebellum had reduced functional connections to a wide range of cortical regions, including parts of the frontal, temporal, occipital, and parietal lobes (31). However, there are still few studies on the cerebellar functional connections of OCD, and in view of the heterogeneity of experimental samples, a study with a hypothesis-free approach is necessary.
In the present study, we used a multiple-algorithm analysis in combination with fALFF and FC to explore the alterations in the resting-state functional connectivity of the cerebellum in OCD.
Materials and Methods
Subjects
Thirty right-handed adult patients with OCD were recruited from the Second Affiliated Hospital of Xinxiang Medical University. All patients were diagnosed by two psychiatrists according to the Diagnostic and Statistical Manual of Mental Disorders, 4th Edition (DSM-IV) criteria. Of the 30 OCD patients, 5 were drug-naïve and 25 were medication-free (patients who had discontinued medication for at least 2 weeks were considered medication-free); the medication-free patients mainly had received treatment with selective serotonin reuptake inhibitors, with the obsessive-compulsive symptom still existing or having relapsed when the cohort was recruited. None of the OCD patients had any other psychiatric disorder that met axis I of the DSM-IV. Twenty-six healthy controls (HCs) matched for age, gender, handedness, and education level were recruited as the control group. All HCs were screened using the Structured Clinical Interview for DSM-IV Axis I Disorders (SCID-I) to ensure that there was no history of other psychiatric disorders that met the criteria of axis I of the DSM-IV. The exclusion criteria for all participants included serious head injury, a history of drug or alcohol abuse, serious physical illness, and contraindications to MRI. All participants received a complete description of the study, and written informed consent was obtained. This study was approved by the Ethics Committee of the Second Affiliated Hospital of Xinxiang Medical University and complied with the Helsinki Declaration.
Clinical Measures
OCD patients were assessed using the Yale-Brown Obsessive-Compulsive Scale (Y-BOCS), and all patients were required to have a Y-BOCS total score of ≥16.
MRI Date Acquisition
Images were acquired with a 3 T MRI system (Siemens Magnet Verio) equipped with an eight-channel phased-array head coil. All subjects were told to relax and close their eyes during the scan. Foam padding and earplugs were used to reduce head motion and scanner noise. Resting-state BOLD images of the whole brain were acquired by using a gradient-echo echo-planar imaging sequence with the following parameters: time repetition (TR) = 2,000 ms, time echo (TE) = 30 ms, flip angle = 90°, matrix size = 64×64, field of view (FOV) = 220 mm, slices = 33, slice thickness = 4 mm, and time point = 240.
Image Processing and Analysis
Date Processing
The preprocessing of the BOLD images was conducted using the Data Processing Assistant for Resting-State fMRI (DPARSF, http://rfmri.org/DPARSF), which is based on Statistical Parametric Mapping (SPM, https://www.fil.ion.ucl.ac.uk/spm/)and the toolbox for Data Processing & Analysis of Brain Imaging (DPABI, http://rfmri.org/DPABI). The images of the first 10 time points were removed to allow the signal to reach equilibrium. The remaining 230 points were corrected for slice timing and realigned for head motion correction. Subjects with more than 2 mm of maximal translation and 2° of maximal rotation were excluded. Previous studies have shown that a slight head movement can affect experimental results (32, 33). We also calculated framewise displacement (FD Jenkinson), which indexes the volume-to-volume changes in head position (34). There were no subjects with FD Jenkinson >0.2 mm, and there were no significant group differences in FD Jenkinson (t = 0.427, p = 0.675) between the OCD patients (0.057 ± 0.034) and controls (0.054 ± 0.027). Next, the motion-corrected functional volumes were spatially normalized to the MNI space and were resampled to 3 × 3 × 3 mm3 using the normalization parameters estimated during unified segmentation. The obtained images were smoothed with a 4-mm full-width at half-maximum (FWHM) Gaussian kernel. Further preprocessing included temporal bandpass filtering (0.01–0.08 Hz) and linear detrending.
Statistical Analysis
The mfALFF was calculated using DPARSF. We transformed the time series into the frequency domain to obtain the power spectrum. After calculating the square root of each frequency in the power spectrum, we obtained the mean square root across a low-frequency range (0.01–0.08 Hz), which was regarded as the ALFF. fALFF is the ratio of the power of each frequency at the low-frequency range to that of the entire frequency range (24). Finally, the resulting spatial fALFF maps were then normalized with each voxel divided by the whole-brain fALFF mean, providing mfALFF spatial maps.
The mfALFF values of the whole brain of the two groups were compared with a two-sample t test using statistical parametric mapping SPM8. Although the age, gender, and years of education of the subjects in the two groups were matched, respectively, we still use them as covariables in the horizontal regression analysis of the group. Finally, we corrected statistical maps for Gaussian random field (GRF) correction to a significance level of p < 0.05, combining the voxel threshold p < 0.001 and cluster size >12 voxels.
The regions of cerebellar with changed mfALFF value were selected as seed regions for seed-based functional connectivity analysis using DPARSF. Temporary series of all voxels in each seed region were extracted and then averaged for each individual. Pearson correlation coefficients were computed between the seeds and the voxels of the whole brain to create the correlation maps for each seed and each participant. All correlation maps were transformed to z-value FC maps by applying Fisher’s r-to-z conversion (35) according to the equation:
where r is the Pearson correlation coefficient, and z is approximately a normal distribution.
Cerebellar–cerebral functional connectivity was compared between the two groups using two-sample t-test. The significance level was set at voxel p < 0.005 with GRF cluster correction of p < 0.05 (cluster size > 102 voxels).
The mfALFF values, z-fc values, and Y-BOCS scores of the OCD patients were analyzed by partial correlation analysis with age, gender, and years of education as the nuisance covariates. The significance level was set at p < 0.05/n after Bonferroni correction.
Results
Participants
After data collection, a total of 56 subjects were enrolled in the study, including 30 OCD patients and 26 controls. The demographic and clinical characteristics of the subjects are summarized in Table 1. There were no significant difference between the OCD group and the control group in age, gender, or years of education.
fALFF Analysis
Compared to the control group, the values of mfALFF were increased in the left Crus II, the left lobule VIII, and the right lobule VIII of the cerebellum under resting state in the OCD group (see details in Table 2 and Figure 1).
Seed-Based Functional Analysis
mfALFF-difference regions of the cerebellum were selected as seeds for seed-based functional connectivity analysis. The results of FC analysis showed weakened connectivity among the left Crus II, lobule VIII, and right striatum and between the right lobule VIII, and the right striatum and cingulate in the OCD group compared with the HC group (see details in Table 3 and Figure 2). Under the more rigorous correction (voxel threshold p < 0.001), there was no difference between the two groups.
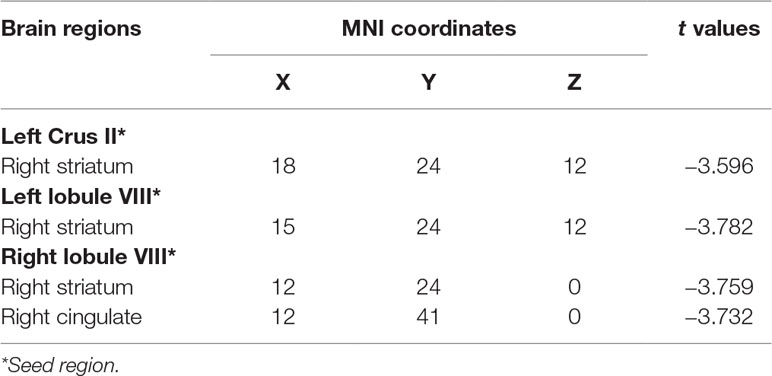
Table 3 Brain regions showing decreased cerebellar–cerebral FC in the OCD group compared with the HC group.
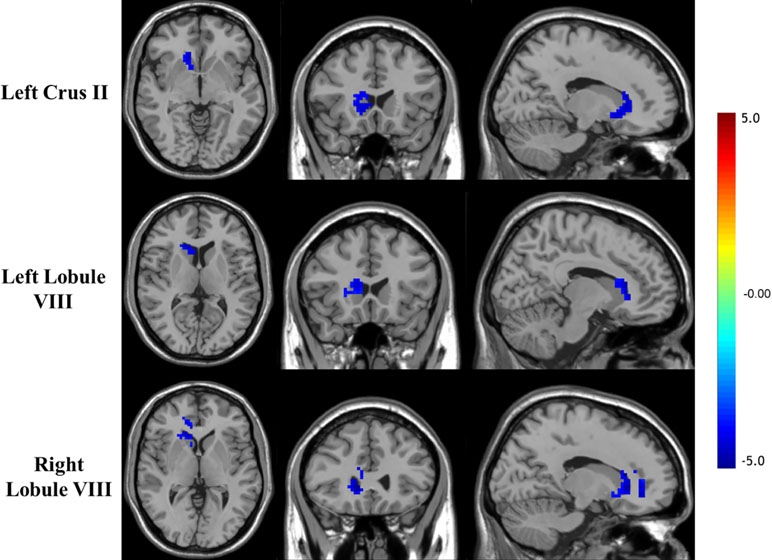
Figure 2 Brain regions showing decreased FC with the cerebellar seed in OCD patients (p < 0.05, GRF corrected).
Correlation Analysis
In OCD patients, the values of mfALFF in the right Crus II region of the cerebellum were positively correlated with the total score of Y-BOCS (see details in Figure 3). And after Bonferroni correction, the FC between the left Crus II and right striatum was negatively correlated with Y-BOCS total scores (see details in Figure 4).
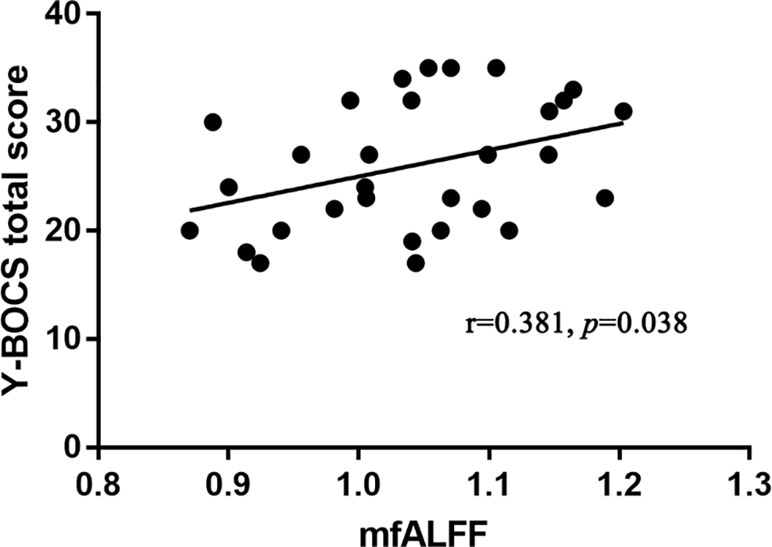
Figure 3 Significant positive correlation of mfALFF values in the left Crus II with the severity of obsessive-compulsive symptom in OCD patients (p < 0.05, Pearson correlation, uncorrected).
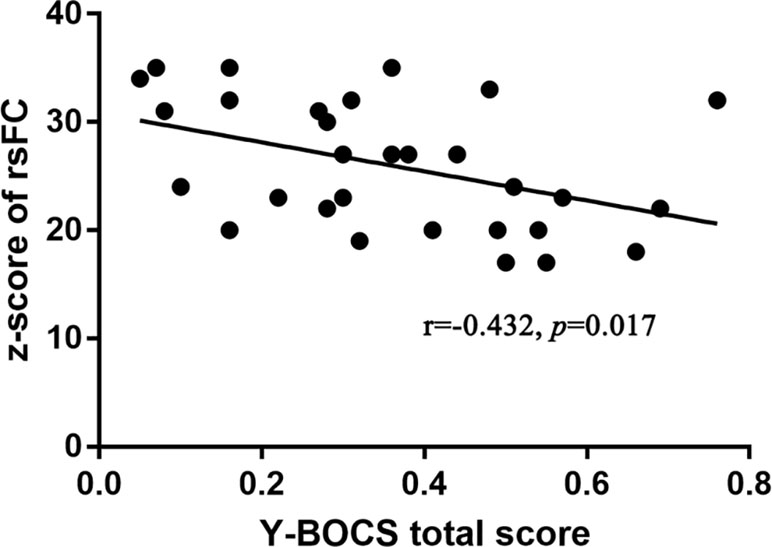
Figure 4 Significant positive correlation of FC between left Crus II and right striatum with the severity of obsessive-compulsive symptom in OCD patients (p < 0.05, Pearson correlation, Bonferroni corrected).
Discussion
In this study, we combined fALFF and FC to investigate the functional and connectivity alterations of the cerebellum in OCD. We found significantly increased mfALFF values in the bilateral posterior cerebellar lobes in the OCD patients, including the left Crus II, left lobule VIII, and right lobule VIII, consistent with the areas of the cerebellum that previous studies have suggested are involved in cognitive function. Furthermore, these cerebellar regions showed weakened functional connection with the striatum, suggesting that abnormal connection between the cerebellum and striatum may be involved in the neuropathology of OCD. In addition, some of the results above were associated with symptom severity.
Cerebellum and OCD
Various studies have shown that people with OCD have an abnormal cerebellum presenting as abnormalities in structure, function, and blood flow (36–39). In addition, studies have also reported cases of OCD caused by cerebellar organic damage (40, 41). However, the focus on the role of the cerebellum in mental illness stems from its contribution to cognitive function. In motor regulation, the cerebellum is responsible for the expected forward control and error-based supervised learning in motor control. That is, the cerebellum, as a forward controller, predicts the consequences of the upcoming actions, compares and adjusts the errors with reality, and communicates the processed information to the corresponding cerebral cortex (42). Recent studies have shown that the cerebellum may regulate cognitive processes in a similar way, that is, as a forward controller to predict the consequences of cognitive activities and psychological expectations, then correcting its errors and conveying them to the cerebral cortex to help the cortex complete the correct cognitive activities (43). Interestingly, previous studies have shown that OCD patients have dysfunctional forward model mechanisms (44). In this study, the Crus II and lobule VIII regions we identified were in the posterior portion of the cerebellum and are involved in cognition (14), which to some extent confirmed this hypothesis. Previous studies have shown that the Crus II and lobule VIII regions are associated with executive function, whereas lobule VIII is involved in working memory (45, 46). Functional abnormalities in the above regions may be the cause of executive function and working memory deficits in OCD patients. However, some previous studies have reported different results. A recent rs-fMRI study showed decreased fALFF in the right cerebellum in treatment-naive OCD patients (47). In another study, female patients with OCD also had reduced fALFF in the cerebellum (48). The reasons for these differences may include sample heterogeneity, such as differences in patient gender composition and treatment status. Moreover, different data processing methods, such as differences in the FWHM during the smoothing process, may also be the cause of the difference in results. In addition, studies about the functional topography of the human cerebellum suggest that different regions of the cerebellum are involved in different functional domains, which may also be the cause of inconsistent results.
Cerebellum and the CSTC Circuit
Earlier research may have been limited by the heterogeneity of OCD and the different research methods used. In previous studies, the results of abnormal cerebellar regions in OCD patients were not consistent, which caused difficulties in the selection of seed regions. A previous study investigating cerebellar–cerebral functional connectivity has relied on a priori–defined seeds from specific networks. In other words, seeds were selected based on different samples from the previous experiment. In view of the present evidence suggesting the heterogeneity of OCD (49), these seeds may not be suitable for different subjects. In this study, the fALFF method was first used to locate abnormal cerebellar regions in patients with OCD, and then the mfALFF value difference region was used as seed regions for whole-brain functional connectivity analysis. In this method, we did not make prior assumptions about the selection of seed regions, and this seed-based FC analysis approach may have advantages over studies based on a priori–defined seeds and provide more reasonable and persuasive findings regarding the pathogenesis of OCD. We found that the cerebellar regions with increased activity in OCD patients had decreased functional connectivity with the components in the CSTC circuit, including the striatum and cingulate. Additionally, decreased functional connectivity between the left Crus II and right striatum was negatively correlated with the severity of obsessive-compulsive symptoms. This is different from previous findings by Xu et al. (31). Differences in the above research methods may account for this result. Interestingly, our findings are more relevant for correlating the cerebellum with the CSTC circuit. Recent research shows that the cerebellum has extensive communication with regions in the CSTC circuit, such as the striatum and thalamus, and the communication was associated with nonmotor function (50–52). The striatum has always been suggested to be involved in habit formation and goal-directed action, and striatum dysfunction is an important cause of OCD (53–55). Alterations of the striatum have also been reported in both structural and functional neuroimaging studies in OCD (12, 56). Previous studies have shown bidirectional anatomical and functional connections between the cerebellum and the striatum (52, 57), and the abnormal functional connections may lead to some cognitive impairment, such as in reward and executive control (58–60). We hypothesized that decreased functional connectivity between the cerebellum and the striatum might affect striatum function, resulting in the inability of the striatum to effectively inhibit the thalamus. The hyperactive thalamus then leads to excessive activation of the frontal cortex, causing dysfunction in executive control and reward.
The cingulate is also an important part of the CSTC circuit, which is mainly related to activity inhibition, decision-making, and emotion regulation (61). Other studies have shown that the cingulate gyrus is closely related to attention (62). In previous studies, OCD patients showed decreased gray matter (GM) and hyperactivity in the cingulate (63–65). Due to the weakened connection between the cerebellum and cingulate, OCD patients may not be able to effectively transmit feedforward information to the anterior cingulate cortex, which makes it difficult for OCD patients to effectively inhibit forced movements and transfer their attention from compulsive thoughts, behaviors, or fears.
However, there are still some limitations in this study. On the one hand, the sample size of this study was small. On the other hand, we did not find any abnormal connections between brain regions and seed regions in OCD patients under a more rigorous correction (voxel threshold p < 0.001). The reason may be the lack of statistical effectiveness due to the small sample size. This study also lacked direct evidence regarding the relationship between abnormal neural correlates and cognitive deficits in OCD patients. We also did not perform a longitudinal comparison before and after treatment. Therefore, we still need supplementary information in future studies to provide further evidence for the role of the cerebellum in the pathogenesis of OCD.
Conclusion
In summary, this study demonstrated abnormal spontaneous cerebellar activity and weakened functional connectivity between the cerebellum and the CSTC circuit in OCD patients, and some of the abovementioned results were associated with symptom severity. Our findings provide additional evidence that the cerebellum may play an essential role, and alteration of functional connections between the cerebellum and the CSTC circuit may be involved in the pathophysiology of OCD.
Ethics Statement
In this study, all participants received a complete description of the study and written informed consent was obtained. This study was approved by the Ethics Committee of the Second Affiliated Hospital of Xinxiang Medical University, and complied with the Helsinki Declaration.
Author Contributions
HZ and BW were involved in data collection, analysis, interpretation of results, and writing of the manuscript. KL was involved in data collection and preprocessing of the data. JZ, XL, QZ, YY, and XW were involved in subject recruitment and data collection. LL, MZ, and HZ were involved in study conception, design, manuscript editing, and intellectual content, and supervised all aspects of the study.
Funding
The research was supported by NSFC-Henan mutual funds (U1704190), the National Nature Science Foundation of China (31600927, 81071091, 81671330, and 81571315), Innovation Scientists and Technicians Troop Construction Projects of Henan Province (174100510024), the Program for Innovative Research Team in Science and Technology in University of Henan Province (18IRTSTHN025), the Open Program of the Institute of Mental (2016PN-KFKT-10 and 2016PN-KFKT-28), the Open Program of the Henan Biological Psychiatry Key Laboratory (ZDSYS2014006 and ZDSYS2015002), High Scientific and Technological Research Fund of Xinxiang Medical University (2017ZDCG-04), Henan Science and Technology Program foundation and frontier project (162300410246), and the Natural Science Research Plan of the Department of Education of Henan province (12A320018).
Conflict of Interest Statement
The authors declare that the research was conducted in the absence of any commercial or financial relationships that could be construed as a potential conflict of interest.
Acknowledgments
The authors would like to thank all the study participants and their families for participating in the study. The authors also thank the doctors (Bo Liu, En Xu) for their help in enrolling the patients.
References
1. Ruscio AM, Stein DJ, Chiu WT, Kessler RC. The epidemiology of obsessive-compulsive disorder in the National Comorbidity Survey Replication. Mol Psychiatry (2010) 15(1):53–63. doi: 10.1038/mp.2008.94
2. Adam Y, Meinlschmidt G, Gloster AT, Lieb R. Obsessive-compulsive disorder in the community: 12-month prevalence, comorbidity and impairment. Soc Psychiatry Psychiatr Epidemiol (2012) 47(3):339–49. doi: 10.1007/s00127-010-0337-5
3. Benzina N, Mallet L, Burguiere E, N’Diaye K, Pelissolo A. Cognitive dysfunction in obsessive-compulsive disorder. Curr Psychiatry Rep (2016) 18(9):80. doi: 10.1007/s11920-016-0720-3
4. Hirschtritt ME, Bloch MH, Mathews CA. Obsessive-compulsive disorder: advances in diagnosis and treatment. JAMA (2017) 317(13):1358–67. doi: 10.1001/jama.2017.2200
5. Graybiel AM, Rauch SL. Toward a neurobiology of obsessive-compulsive disorder. Neuron (2000) 28(2):343–7. doi: 10.1016/S0896-6273(00)00113-6
6. Alexander GE, DeLong MR, Strick PL. Parallel organization of functionally segregated circuits linking basal ganglia and cortex. Annu Rev Neurosci (1986) 9:357–81. doi: 10.1146/annurev.ne.09.030186.002041
7. Pauls DL, Abramovitch A, Rauch SL, Geller DA. Obsessive-compulsive disorder: an integrative genetic and neurobiological perspective. Nat Rev Neurosci (2014) 15(6):410–24. doi: 10.1038/nrn3746
8. Menzies L, Chamberlain SR, Laird AR, Thelen SM, Sahakian BJ, Bullmore ET. Integrating evidence from neuroimaging and neuropsychological studies of obsessive-compulsive disorder: the orbitofronto-striatal model revisited. Neurosci Biobehav Rev (2008) 32(3):525–49. doi: 10.1016/j.neubiorev.2007.09.005
9. Yuqi C, Jian X, Binbin N, Chunrong L, Tao Y, Haijun L, et al. Abnormal resting-state activities and functional connectivities of the anterior and the posterior cortexes in medication-naïve patients with obsessive-compulsive disorder. PLoS One (2013) 8(6):e67478. doi: 10.1371/journal.pone.0067478
10. Saxena S, Brody AL, Maidment KM, Smith EC, Zohrabi N, Katz E, et al. Cerebral glucose metabolism in obsessive-compulsive hoarding. Am J Psychiatry (2004) 161(6):1038–48. doi: 10.1176/appi.ajp.161.6.1038
11. Narayanaswamy JC, Jose D, Kalmady SV, Agarwal SM, Venkatasubramanian G, Janardhan Reddy YC. Cerebellar volume deficits in medication-naive obsessive compulsive disorder. Psychiatry Res Neuroimaging (2016) 254:164–8. doi: 10.1016/j.pscychresns.2016.07.005
12. Hu X, Du M, Chen L, Li L, Zhou M, Zhang L, et al. Meta-analytic investigations of common and distinct grey matter alterations in youths and adults with obsessive-compulsive disorder. Neurosci Biobehav Rev (2017) 78:91–103. doi: 10.1016/j.neubiorev.2017.04.012
13. Tang W, Zhu Q, Gong X, Zhu C, Wang Y, Chen S. Cortico-striato-thalamo-cortical circuit abnormalities in obsessive-compulsive disorder: a voxel-based morphometric and fMRI study of the whole brain. Behav Brain Res (2016) 313:17–22. doi: 10.1016/j.bbr.2016.07.004
14. D’Angelo E, Casali S. Seeking a unified framework for cerebellar function and dysfunction: from circuit operations to cognition. Front Neural Circuits (2012) 6:116. doi: 10.3389/fncir.2012.00116
15. Habas C, Kamdar N, Nguyen D, Prater K, Beckmann CF, Menon V, et al. Distinct cerebellar contributions to intrinsic connectivity networks. J Neurosci (2009) 29(26):8586–94. doi: 10.1523/JNEUROSCI.1868-09.2009
16. Guell X, Schmahmann JD, Gabrieli J, Ghosh SS. Functional gradients of the cerebellum. Elife (2018) 7:e36652. doi: 10.7554/eLife.36652
17. Abramovitch A, Abramowitz JS, Mittelman A. The neuropsychology of adult obsessive-compulsive disorder: a meta-analysis. Clin Psychol Rev (2013) 33(8):1163–71. doi: 10.1016/j.cpr.2013.09.004
18. Bragdon LB, Gibb BE, Coles ME. Does neuropsychological performance in OCD relate to different symptoms? A meta analysis comparing the symmetry and obsessing dimensions. Depress Anxiety (2018) 35(8):761–74. doi: 10.1002/da.22785
19. Zang YF, He Y, Zhu CZ, Cao QJ, Sui MQ, Liang M, et al. Altered baseline brain activity in children with ADHD revealed by resting-state functional MRI. Brain Dev (2007) 29(2):83–91. doi: 10.1016/j.braindev.2006.07.002
20. Biswal B, Yetkin FZ, Haughton VM, Hyde JS. Functional connectivity in the motor cortex of resting human brain using echo-planar MRI. Magn Reson Med (2010) 34(4):537–41. doi: 10.1002/mrm.1910340409
21. Guo WB, Liu F, Xue ZM, Xu XJ, Wu RR, Ma CQ, et al. Alterations of the amplitude of low-frequency fluctuations in treatment-resistant and treatment-response depression: a resting-state fMRI study. Prog Neuropsychopharmacol Biol Psychiatry (2012) 37(1):153–60. doi: 10.1016/j.pnpbp.2012.01.011
22. Zhu Y, Fan Q, Zhang H, Qiu J, Tan L, Xiao Z, et al. Altered intrinsic insular activity predicts symptom severity in unmedicated obsessive-compulsive disorder patients: a resting state functional magnetic resonance imaging study. BMC Psychiatry (2016) 16(1):104. doi: 10.1186/s12888-016-0806-9
23. Wang P, Yang J, Yin Z, Duan J, Zhang R, Sun J, et al. Amplitude of low-frequency fluctuation (ALFF) may be associated with cognitive impairment in schizophrenia: a correlation study. BMC Psychiatry (2019) 19(1):30. doi: 10.1186/s12888-018-1992-4
24. Zou QH, Zhu CZ, Yang Y, Zuo XN, Long XY, Cao QJ, et al. An improved approach to detection of amplitude of low-frequency fluctuation (ALFF) for resting-state fMRI: fractional ALFF. J Neurosci Methods (2008) 172(1):137–41. doi: 10.1016/j.jneumeth.2008.04.012
25. Liu F, Guo W, Liu L, Long Z, Ma C, Xue Z, et al. Abnormal amplitude low-frequency oscillations in medication-naive, first-episode patients with major depressive disorder: a resting-state fMRI study. J Affect Disord (2013) 146(3):401–6. doi: 10.1016/j.jad.2012.10.001
26. Fryer SL, Roach BJ, Ford JM, Turner JA, van Erp TG, Voyvodic J, et al. Relating Intrinsic low-frequency bold cortical oscillations to cognition in schizophrenia. Neuropsychopharmacology (2015) 40(12):2705–14. doi: 10.1038/npp.2015.119
27. Yang X, Hu X, Tang W, Li B, Yang Y, Gong Q, et al. Intrinsic brain abnormalities in drug-naive patients with obsessive-compulsive disorder: a resting-state functional MRI study. J Affect Disord (2019) 245:861–8. doi: 10.1016/j.jad.2018.11.080
28. Wenbin G, Liuyu Y, Zhikun Z, Jian Z, Huafu C, Changqing X. Is there a cerebellar compensatory effort in first-episode, treatment-naive major depressive disorder at rest? Progr Neuropsychopharmacol Biol Psychiatry (2013) 46(10):13–8. doi: 10.1016/j.pnpbp.2013.06.009
29. Wang Y, Zhong S, Chen G, Liu T, Zhao L, Sun Y, et al. Altered cerebellar functional connectivity in remitted bipolar disorder: a resting-state functional magnetic resonance imaging study. Austral NZ J Psychiatry (2017) 52(10):962–71. doi: 10.1177/0004867417745996
30. Wu L, Caprihan A, Bustillo J, Mayer A, Calhoun V. An approach to directly link ICA and seed-based functional connectivity: application to schizophrenia. Neuroimage (2018) 179:448–70. doi: 10.1016/j.neuroimage.2018.06.024
31. Xu T, Zhao Q, Wang P, Fan Q, Chen J, Zhang H, et al. Altered resting-state cerebellar-cerebral functional connectivity in obsessive-compulsive disorder. Psychol Med (2019) 49(7):1156–65. 1–10. doi: 10.1017/S0033291718001915
32. Power JD, Barnes KA, Snyder AZ, Schlaggar BL, Petersen SE. Spurious but systematic correlations in functional connectivity MRI networks arise from subject motion. Neuroimage (2012) 59(3):2142–54. doi: 10.1016/j.neuroimage.2011.10.018
33. Yan CG, Craddock RC, He Y, Milham MP. Addressing head motion dependencies for small-world topologies in functional connectomics. Front Hum Neurosci (2013) 7:910. doi: 10.3389/fnhum.2013.00910
34. Mark J, Peter B, Michael B, Stephen S. Improved optimization for the robust and accurate linear registration and motion correction of brain images. Neuroimage (2002) 17(2):825–41. doi: 10.1016/S1053-8119(02)91132-8
35. Vincent JL, Patel GH, Fox MD, Snyder AZ, Baker JT, Van Essen DC, et al. Intrinsic functional architecture in the anaesthetized monkey brain. Nature (2007) 447 :83–6. doi: 10.1038/nature05758
36. Busatto GF, Buchpiguel CA, Zamignani DR, Garrido GE, Glabus MF, Rosario-Campos MC, et al. Regional cerebral blood flow abnormalities in early-onset obsessive-compulsive disorder: an exploratory SPECT study. J Am Acad Child Adolesc Psychiatry (2001) 40(3):347–54. doi: 10.1097/00004583-200103000-00015
37. Zarei M, Mataix-Cols D, Heyman I, Hough M, Doherty J, Burge L, et al. Changes in gray matter volume and white matter microstructure in adolescents with obsessive-compulsive disorder. Biol Psychiatry (2011) 70(11):1083–90. doi: 10.1016/j.biopsych.2011.06.032
38. Hartmann T, Vandborg S, Rosenberg R, Sorensen L, Videbech P. Increased fractional anisotropy in cerebellum in obsessive-compulsive disorder. Acta Neuropsychiatr (2016) 28(3):141–8. doi: 10.1017/neu.2015.57
39. Moon CM, Jeong GW. Associations of neurofunctional, morphometric and metabolic abnormalities with clinical symptom severity and recognition deficit in obsessive-compulsive disorder. J Affect Disord (2018) 227:603–12. doi: 10.1016/j.jad.2017.11.059
40. Tonna M, Ottoni R, Ossola P, De Panfilis C, Marchesi C. Late-onset obsessive-compulsive disorder associated with left cerebellar lesion. Cerebellum (2014) 13(4):531–5. doi: 10.1007/s12311-014-0561-8
41. Takeuchi N, Kato E, Kanemoto K. Obsessive-compulsive disorder associated with posterior cranial fossa meningioma. Case Rep Psychiatry (2017) 2017:8164537. doi: 10.1155/2017/8164537
42. Pisotta I, Molinari M. Cerebellar contribution to feedforward control of locomotion. Front Hum Neurosci (2014) 8:475. doi: 10.3389/fnhum.2014.00475
43. Ito M. Control of mental activities by internal models in the cerebellum. Nat Rev Neurosci (2008) 9(4):304–13. doi: 10.1038/nrn2332
44. Gentsch A, Schutz-Bosbach S, Endrass T, Kathmann N. Dysfunctional forward model mechanisms and aberrant sense of agency in obsessive-compulsive disorder. Biol Psychiatry (2012) 71(7):652–9. doi: 10.1016/j.biopsych.2011.12.022
45. Balsters JH, Laird AR, Fox PT, Eickhoff SB. Bridging the gap between functional and anatomical features of cortico-cerebellar circuits using meta-analytic connectivity modeling. Hum Brain Mapp (2014) 35(7):3152–69. doi: 10.1002/hbm.22392
46. E KH, Chen SH, Ho MH, Desmond JE. A meta-analysis of cerebellar contributions to higher cognition from PET and fMRI studies. Hum Brain Mapp (2014) 35(2):593–615. doi: 10.1002/hbm.22194
47. Qiu L, Fu X, Wang S, Tang Q, Chen X, Cheng L, et al. Abnormal regional spontaneous neuronal activity associated with symptom severity in treatment-naive patients with obsessive-compulsive disorder revealed by resting-state functional MRI. Neurosci Lett (2017) 640:99–104. doi: 10.1016/j.neulet.2017.01.024
48. Meng Z, Zhang Z, Fan Q, Li Y. Altered fractional amplitude of low frequency fluctuations in unmedicated female patients with obsessive-compulsive disorder. Conf Proc IEEE Eng Med Biol Soc (2018) 2018:1144–7. doi: 10.1109/EMBC.2018.8512490
49. Dougherty DD, Brennan BP, Stewart SE, Wilhelm S, Widge AS, Rauch SL. Neuroscientifically informed formulation and treatment planning for patients with obsessive-compulsive disorder: a review. JAMA Psychiatry (2018) 75(10):1081–7. doi: 10.1001/jamapsychiatry.2018.0930
50. Bostan AC, Dum RP, Strick PL. Cerebellar networks with the cerebral cortex and basal ganglia. Trends Cogn Sci (2013) 17(5):241–54. doi: 10.1016/j.tics.2013.03.003
51. Chen CH, Fremont R, Arteaga-Bracho EE, Khodakhah K. Short latency cerebellar modulation of the basal ganglia. Nat Neurosci (2014) 17(12):1767–75. doi: 10.1038/nn.3868
52. Bostan AC, Strick PL. The basal ganglia and the cerebellum: nodes in an integrated network. Nat Rev Neurosci (2018) 19(6):338–50. doi: 10.1038/s41583-018-0002-7
53. Burton AC, Nakamura K, Roesch MR. From ventral-medial to dorsal-lateral striatum: neural correlates of reward-guided decision-making. Neurobiol Learn Mem (2015) 117(Sp. Iss. SI):51–9. doi: 10.1016/j.nlm.2014.05.003
54. Vaghi MM, Vértes PE, Kitzbichler MG, Apergis-Schoute AM, Flier FEVD, Fineberg NA, et al. Specific fronto-striatal circuits for impaired cognitive flexibility and goal-directed planning in obsessive-compulsive disorder: evidence from resting-state functional connectivity. Biol Psychiatry (2016) 81(8):708–17. doi: 10.1016/j.biopsych.2016.08.009
55. Malvaez M, Greenfield VY, Matheos DP, Angelillis NA, Murphy MD, Kennedy PJ, et al. Habits are negatively regulated by histone deacetylase 3 in the dorsal striatum. Biol Psychiatry (2018) 84(5):383–92. doi: 10.1016/j.biopsych.2018.01.025
56. Wia H, Clair AH, Fernandez-Vidal S, Gholipour B, Morgiève M, Mallet L. Altered anatomical connections of associative and limbic cortico-basal-ganglia circuits in obsessive-compulsive disorder. Eur Psychiatry (2018) 51(5):1–8. doi: 10.1016/j.eurpsy.2018.01.005
57. Bostan AC, Dum RP, Strick PL. The basal ganglia communicate with the cerebellum. Proc Natl Acad Sci U S A (2010) 107(18):8452–6. doi: 10.1073/pnas.1000496107
58. Koehler S, Ovadiacaro S, vander Meer E, Villringer A, Heinz A, Romanczukseiferth N, et al. Increased functional connectivity between prefrontal cortex and reward system in pathological gambling. PLoS One (2013) 8(12):e84565. doi: 10.1371/journal.pone.0084565
59. Brunamonti E, Chiricozzi FR, Clausi S, Olivito G, Giusti MA, Molinari M, et al. Cerebellar damage impairs executive control and monitoring of movement generation. PLoS One (2014) 9(1):e85997. doi: 10.1371/journal.pone.0085997
60. Xiao L, Bornmann C, Hatstattburklé L. Regulation of striatal cells and goal-directed behavior by cerebellar outputs. Nat Comm (2018) 9:3133. doi: 10.1038/s41467-018-05565-y
61. Seeley WW, Menon V, Schatzberg AF, Keller J, Glover GH, Kenna H, et al. Dissociable intrinsic connectivity networks for salience processing and executive control. J Neurosci (2007) 27(9):2349–56. doi: 10.1523/JNEUROSCI.5587-06.2007
62. Wen X, Liu Y, Yao L, Ding M. Top-down regulation of default mode activity in spatial visual attention. J Neurosci (2013) 33(15):6444. doi: 10.1523/JNEUROSCI.4939-12.2013
63. Kate Dimond F, Welsh RC, Gehring WJ, Abelson JL, Himle JA, Israel L, et al. Error-related hyperactivity of the anterior cingulate cortex in obsessive-compulsive disorder. Biol Psychiatry (2005) 57(3):287–94. doi: 10.1016/j.biopsych.2004.10.038
64. Jingming H, Wenjing W, Yun L, Jian W, Daiquan Z, Junwei G, et al. Localization of cerebral functional deficits in patients with obsessive-compulsive disorder: a resting-state fMRI study. J Affect Disord (2012) 138(3):313–21. doi: 10.1016/j.jad.2012.01.022
Keywords: obsessive-compulsive disorder, cerebellum, cortico-striato-thalamo-cortical circuit, functional magnetic resonance imaging, FC
Citation: Zhang H, Wang B, Li K, Wang X, Li X, Zhu J, Zhao Q, Yang Y, Lv L, Zhang M and Zhang H (2019) Altered Functional Connectivity Between the Cerebellum and the Cortico-Striato-Thalamo-Cortical Circuit in Obsessive-Compulsive Disorder. Front. Psychiatry 10:522. doi: 10.3389/fpsyt.2019.00522
Received: 27 December 2018; Accepted: 02 July 2019;
Published: 24 July 2019.
Edited by:
Feng Liu, Tianjin Medical University General Hospital, ChinaReviewed by:
Hao Yan, Peking University, ChinaYiqun Guo, Chongqing University of Posts and Telecommunications, China
Copyright © 2019 Zhang, Wang, Li, Wang, Li, Zhu, Zhao, Yang, Lv, Zhang and Zhang. This is an open-access article distributed under the terms of the Creative Commons Attribution License (CC BY). The use, distribution or reproduction in other forums is permitted, provided the original author(s) and the copyright owner(s) are credited and that the original publication in this journal is cited, in accordance with accepted academic practice. No use, distribution or reproduction is permitted which does not comply with these terms.
*Correspondence: Haisan Zhang, emhzMzg2QDE2My5jb20=; Hongxing Zhang, emh4MTY2NjY2QDE2My5jb20=
†These authors have contributed equally to this work.