- 1Clinical Psychopharmacology Division, Peking University Institute of Mental Health (Sixth Hospital) & National Clinical Research Center for Mental Disorders/Key Laboratory of Mental Health, Ministry of Health (Peking University), Beijing, China
- 2Beijing Suicide Research and Prevention Center, Beijing Huilongguan Hospital, Beijing, China
- 3CAS Key Laboratory of Behavioral Science, Institute of Psychology, Beijing, China
- 4The 984th Hospital of People's Liberation Army of China, Beijing, China
- 5Department of Radiology, 306 Hospital of People's Liberation Army, Beijing, China
Background: Only less than 40% of patients with Major depressive disorder (MDD) can achieve remission after several weeks of initial antidepressant treatment. Predicting whether the prescribed treatment is effective in the following course may help clinicians modify the treatment regimen in time, and reduce the staggering burden for patients and society. However, there are not yet reliable markers based on neurobiological change after a treatment regimen steadily applied, for predicting clinical treatment outcome. The striatal circuits often exhibit abnormality for MDD patients, and are implicated in antidepressant treatments.
Methods: Nineteen first-episode drug-naive MDD patients (nine females, mean age was 30 years old) were recruited to undergo clinical symptom assessment and resting state fMRI scanning at baseline, after 2 and 8 weeks of treatment with duloxetine. A seed-based analysis was used to obtain functional connectivity (FC) maps of six sub-regions of the stratum, then we explored the relationship of 2-week changes of striatal FC with clinical symptom improvement after 8-week duloxetine treatment.
Results: The results revealed that 2-week FC changes of the striatal cognitive and affective subdivisions with the frontoparietal regions positively correlated with 8-week symptom improvement. We also found that early FC changes between the striatal motor subdivision and the motor-related cortical regions negatively correlated with later symptom improvement.
Conclusions: These findings suggest that change of the FC of the cortical-striatal circuits at the early stage of treatment is critical for later remission of MDD. Furthermore, the association between the FC change and symptom improvement may have significant implication for clinical practice to regard neural changes as reference for evaluating how antidepressant treatment works.
Introduction
Major depressive disorder (MDD) is a common and disabling psychiatric disorder, with multi-dimensional clinical symptoms involving emotion, cognition, psychomotor, and somatic domains (1). Though antidepressant medication is used as first-line treatment for MDD, only less than 40% of patients achieve remission with initial treatment (2, 3). As suggested by current treatment guidelines, treatment regimen should be changed if there has not been a partial response after 4 to 6 weeks (1, 4), which make non-respondent patients endure prolonged ineffective treatment and largely increase disease burden. Hence, it is of great value to identify indicators of response to a given antidepressant treatment early in the treatment process. However, the neural mechanism underlying the predictor of treatment outcome is unclear. Several lines of clinical evidence have shown that early improvement of clinical symptoms within the first 2 weeks of antidepressant treatment can partly predict later treatment outcome (5, 6), highlighting 2-week as a key time frame in determining long-term clinical therapeutic effect. In addition, from a clinical perspective, the antidepressant treatment may take 2 weeks to have a stable effect on the brain after dose titration to the target treatment dose or reaching steady state.
Functional magnetic resonance imaging (fMRI) have provided a powerful tool to investigate the changes of brain activity and functional networks of MDD patients during treatment, which may help to identify some reliable and objective predictors of treatment outcome (7). In the domain of neuroimaging, neural systems supporting emotion processing and regulation, and reward seeking are found dysfunctional in MDD, which mainly can be conceptualized as emotion network and reward network (8). Reward network focusing on ventral striatum is modulated by dopamine (9), and related to anhedonia, which is one of core MDD symptoms (10). The most consistent finds from reward system in MDD patients is hypo-activation of striatal regions during the whole reward processing, such as salience, anticipation, feedback, and learning (11). Neuroimaging studies also investigated the neural predictors of treatment outcome and found reward-related function is predictive of treatment outcome. Previous research focused on pretreatment prognostic measures of treatment response to antidepressant agents. The most prominent findings showed that higher anterior cingulate activity before treatment was predictive of positive response to treatment of MDD (12, 13). A meta-analysis study indicates that increased pretreatment rostral anterior cingulate cortex activity is a reliable marker of treatment response (14). Nonetheless, there is little research on early biomarkers during the treatment that can be used to predict later response to antidepressant agents. One study revealed that greater reduction of activation to fearful facial expressions across the anterior cingulate, insula, amygdala, and thalamus after 1-week Selective and Serotonin Reuptake Inhibitors (SSRI) treatment was associated with 6-week clinical response for MDD patients (15).
We mainly focused on 2-week neural changes of the striatum-based circuits since the deficits of these circuits have been constantly linked to MDD (16, 17). As a key node of the reward circuit, striatum has extensive interconnections with the prefrontal cortex, and engages in complex interactions among dopaminergic, serotoninergic, and glutamatergic systems in function (18), making it a critical therapeutic target of antidepressants. Previous studies have shown that striatal activation and cortico-striatal functional connectivity (FC) during reward processing could be altered after different antidepressant treatments (19, 20). Besides, pre-treatment striatal activity and FC is associated with clinical symptom improvement after treatment (21, 22). The striatum has been proposed to functionally divide into several subregions, supporting affective, cognitive, and motor processing, respectively (23), and MDD patients often experience difficulties in these domains (24). As one of core MDD symptoms, anhedonia may result from deficits of the brain's reward system (25, 26), which were associated with abnormality of the striatum-based circuits (17, 25, 27). Psychomotor retardation, as a classic clinical symptom of MDD (28), was associated with decrease in the fronto-striatal connectivity and decrease in striatal volume (29, 30). These findings highlight that the striatal circuits may be key to prognosis and treatment response of MDD.
Abnormal communication among functional brain networks has been found in MDD, mainly focused on the default mode network (DMN) related to the rumination, frontoparietal network (FPN) related to cognitive regulation, and somatosensory network (SMN) referring to psychomotor symptoms of MDD (31), suggesting that investigating MDD from the perspective of unified networks of neural dysfunction may yield an integrative framework to understand this disorder. Based on previous researches, we hypothesized that the change of communication of striatum with DMN, FPN, and SMN at early stage of treatment would decide the enduring clinical symptom improvement of MDD patients.
In the current study, we investigated the resting-state FC changes of striatum within 2-week treatment as an imaging biomarker of clinical symptom improvement after 8-week treatment, given that the dopamine neurotransmission in the striatum and its function were impaired in MDD, but could possibly be changed by the treatment (32, 33). We administered duloxetine to first-episode drug-naïve MDD patients, since duloxetine is a dual-action antidepressant, which increases norepinephrine (NE) and serotonin (5-HT).
Materials and Methods
Subjects
Thirty-four first-episode drug-naive MDD patients (18 females; mean age was 30 years old) were recruited to undergo clinical symptom assessment at baseline, after 2 and 8 weeks of treatment with duloxetine. They also underwent resting-state fMRI scanning at baseline and after 2 weeks of the treatment. These patients were diagnosed by qualified psychiatrists using the Mini International Neuropsychiatric Interview (MINI) (34), a short structured interview developed to derive diagnoses according to the Diagnostic and Statistical Manual of Mental Disorders, 4th edition (DSM-IV). Inclusion criteria included an acute depressive episode, total score on the 24-item Hamilton Rating Scale for Depression (HRSD24) (35) ≥ 20, and illness duration ≤ 24 months. MDD patients with comorbid Axis I disorders, Axis II personality disorders, or intellectual disability were excluded. Other exclusion criteria consisted of serious medical or neurological illness, a history of significant head trauma, substance dependence or abuse within the last year, current or previous use of psychotropic drugs, a history of electroconvulsive therapy (ECT), acutely suicidal or homicidal, current pregnancy or breastfeeding, or any contraindications to an MRI scan.
Among the initially recruited 34 patients at baseline, eight withdrew the informed consent due to not local residents and not convenient to come back for follow-up, and seven discontinued medication due to the side effect or some personal reasons. A total of 19 patients who finished all MRI scanning and clinical assessment were finally included in this study (see Figure S1). This study was carried out in accordance with the recommendation of the Ethics Committee of the Sixth Hospital (Institute of Mental Health) of Peking University with written informed consent from all subjects. All subjects gave written informed consent in accordance with the Declaration of Helsinki. The protocol was approved by the Ethics Committee of the Sixth Hospital (Institute of Mental Health) of Peking University.
Antidepressant Treatment and Clinical Assessment
All the 19 patients included in the present study received duloxetine treatment for 8 weeks, without taking any other psychotropic medication or psychotherapies during this period. They were treated initially with oral duloxetine at a dose of 30–60 mg/day; then the dose was increased to 60–90 mg/day within the first two weeks, and continued at this level until they finished the 8-week study. The final dose was 60 mg/day for 17 patients, and 90 mg/day for 2 patients. The dose adjustment was accorded to the clinical judgment of the psychiatrist and the patient's consent and findings in our study are not driven by dose effects. (see Table S2). Clinical ratings were administered at baseline, the end of 2nd week, and the end of 8th week, using HRSD24.
fMRI Data Acquisition
Brain imaging was performed on a 3.0 T scanner (Siemens Magnetom Trio; Siemens Medical Solutions, Erlangen, Germany) in the 306th hospital of People's Liberation Army of China. For resting-state scanning, subjects were instructed to keep their eyes closed, to remain still without head movement, and not to fall asleep or think of anything in particular. The resting-state functional images were collected with a gradient-echo echo-planar imaging sequence with the following parameters: repetition time (TR), 2,000 msec; echo time (TE), 30 msec; flip angle, 90°; matrix, 64×64; field of view, 210×210 mm2; slice thickness/gap, 4.0 mm/0.8 mm; and 30 axial slices covering the whole brain. 210 functional volumes were acquired in 7 min. After the functional MRI scanning, high-spatial-resolution structural images were acquired for each subject with the T1-weighted magnetization-prepared rapidly acquired gradient-echo (MPRAGE) sequence (TR, 2,300 msec; TE, 3.01 msec; matrix, 256×256; spatial resolution, 1×1×1 mm3; flip angle, 9°; thickness, 1 mm; 176 sagittal slices) to achieve better normalization.
Image Data Preprocessing
The fMRI images were preprocessed with the Data Processing Assistant for Resting-State fMRI (DPARSF, http://rfmri.org/DPARSF) (36), which is based on SPM12 (http://www.fil.ion.ucl.ac.uk/spm). After removing the first 10 volumes, the remaining 200 volumes were corrected for different slice acquisition timing and head motion. Then, the nuisance signals were regressed out, including signal associated with 24 Friston head-motion parameters, signal from the cerebrospinal fluid and white matter, global signal, and linear signal trend. Derived images were co-registered with the corresponding structural images which were segmented and normalized to the Montreal Neurological Institute (MNI) space using the DARTEL. The functional images were then normalized to the MNI space with the warped parameters, and resampled to 3 × 3 × 3 mm cubic voxels. The transformed images were then band-pass filtered (0.01–0.1 Hz) and spatially smoothed with a full width at half maximum of 6 mm. Subjects with large head displacement (max > 3mm) or head rotation (max > 3 degree) were excluded for further analyses. None of the patients were excluded due to large head motion. Since it has been shown that head motion might result in artefactual inter-individual difference in resting-state metrics (37, 38), we adopted a more strict setting of data preprocessing to control the effect of head motion. In brief, beyond regressing out the nuisance signals associated with 24 Friston head-motion parameters, we further removed or “scrubbed’ bad time points with large head motion. The bad time points were defined as those whose frame-wise displacement (FD) was larger than 0.2 mm (see Supplementary Material). On average, it scrubbed 43.89 ± 36.89 frames of functional images for both baseline and 2-week scans (see Supplementary Material).
Functional Connectivity Analysis
The striatum is proposed to divide into 12 representative subregions, which are defined bilaterally in the dorsal caudate (DC) (x = ± 13, y = 15, z = 9), superior ventral striatum (VSs) (x = ± 10, y = 15, z = 0), inferior ventral striatum (VSi)/nucleus accumbens (x = ± 9, y = 9, z = -8), dorsal rostral putamen (DRP) (x = ± 25, y = 8, z = 6), dorsal caudal putamen (DCP) (x = ± 28, y = 1, z = 3), and ventral rostral putamen (VRP) (x = ± 20, y = 12, z = -3), as reported in a study by Di Martino et al. (23). These regions are proposed to be differentially involved in executive control (DC, VSs, and VRP), motor (DRP and DCP), and affective processing (VSs and VSi) (23).
We used a seed-based approach to compute FC of the 12 subregions of the striatum. These subregions were regarded as regions of interest (ROI), and a sphere with 4 mm radius was created for each ROI that covered 7 voxels. For FC computation, the mean BOLD time series for each ROI was extracted, and correlated with the BOLD time series of each voxel within the brain using Pearson's Correlation. Then the derived correlation coefficient was transformed to Fisher's Z score for statistical analyses. For each subject, 12 whole-brain voxel-wise striatal FC maps were created.
Statistical Analysis
FC alterations (ΔFC) from baseline to 2-week after treatment were calculated for each subject and for each ROI: ΔFC = FC2W – FCbaseline. The treatment efficacy was evaluated using rate of reduction in HRSD scores: ΔHRSD = (HRSDbaseline – HRSD8W)/HRSDbaseline × 100%. Correlation analyses between the ΔFC and ΔHRSD were subsequently performed across the brain, with age, gender, and education level as covariates. We combined Bonferroni correction and Gaussian random field (GRF) theory for multiple comparison correction. Bonferroni correction corrected for multiple comparisons for the 12 ROIs, resulting in p < 0.0042 (i.e. equal to 0.05/12). GRF theory corrected for multiple comparisons across voxels of the brain. Here we performed two-tailed GRF correction, with voxel p < 0.001 and cluster p < 0.0042 (i.e., voxel p < 0.0005 and cluster p < 0.0021 for each tail). To exclude the possibility that the observed association was driven by any outlier, we also performed robust regression test which could decrease the effect of outlier on the association between two variables. The robust regression tests were performed on extracted ROI mean values, using the function of “robustfit” in Matlab. Furthermore, in order to provide evidence that our results were not an artefact caused by head motion, the functional connectivity analysis and the statistical analysis were rerun with the scrubbed data.
Results
Sample Characteristics
The HRSD24 scores decreased significantly in MDD patients after treatment over 2 and 8 weeks (all ps < 0.001, Table 1). For the 19 MDD patients who completed the whole 8-week treatment and assessment, all but two of them showed a clinical response to duloxetine treatment at the end of 8th week, defined as 50% or more reduction of the HRSD24 score from baseline; and 15 of them achieved clinical remission with a HRSD24 score of 8 or below.
Positive Correlation of 2-Week ΔFC With Symptom Improvement
The present results revealed that greater ΔFC of striatal subdivisions with brain regions in the FPN and DMN after 2-week treatment was associated with better clinical improvement at 8-week.
As shown in Figures 1A, C and Table 2, the ΔFC of the left DC with right middle frontal gyrus (MFG) in the FPN was positively related to treatment response at 8-week (r = 0.94). The ΔFC of the left VSs with right MFG and right inferior parietal lobule (IPL) in the FPN was positively related to treatment response at 8-week as well (r = 0.91 for right MFG and r = 0.87 for the right IPL). The significant clusters of right MFG for the two caudate subdivisions involved in executive control processing (i.e. left DC and left VSs) were overlapped (in orange intersected by blue and green in Figure 1), indicative of consistency of the findings. Moreover, for both the right DRP and right VRP, the ΔFC with right angular gyrus (AG) in DMN was positively related to treatment response at 8-week (r = 0.92 for right DRP – right AG, and r = 0.89 for right VRP – right AG; Figure 1 and Table 2). The significant clusters of the right AG were also overlapped (in orange intersected by purple and red), for the two putamen subdivisions (i.e. right DRP and right VRP). Robust regression test also demonstrated that these associations were not affected by any outlier effect (all p < 0.05, FDR corrected). Moreover, the correlations remained significant when the dose level over initial two weeks was added as another covariate, suggesting the results were not driven by dose effects (see Supplementary Material).
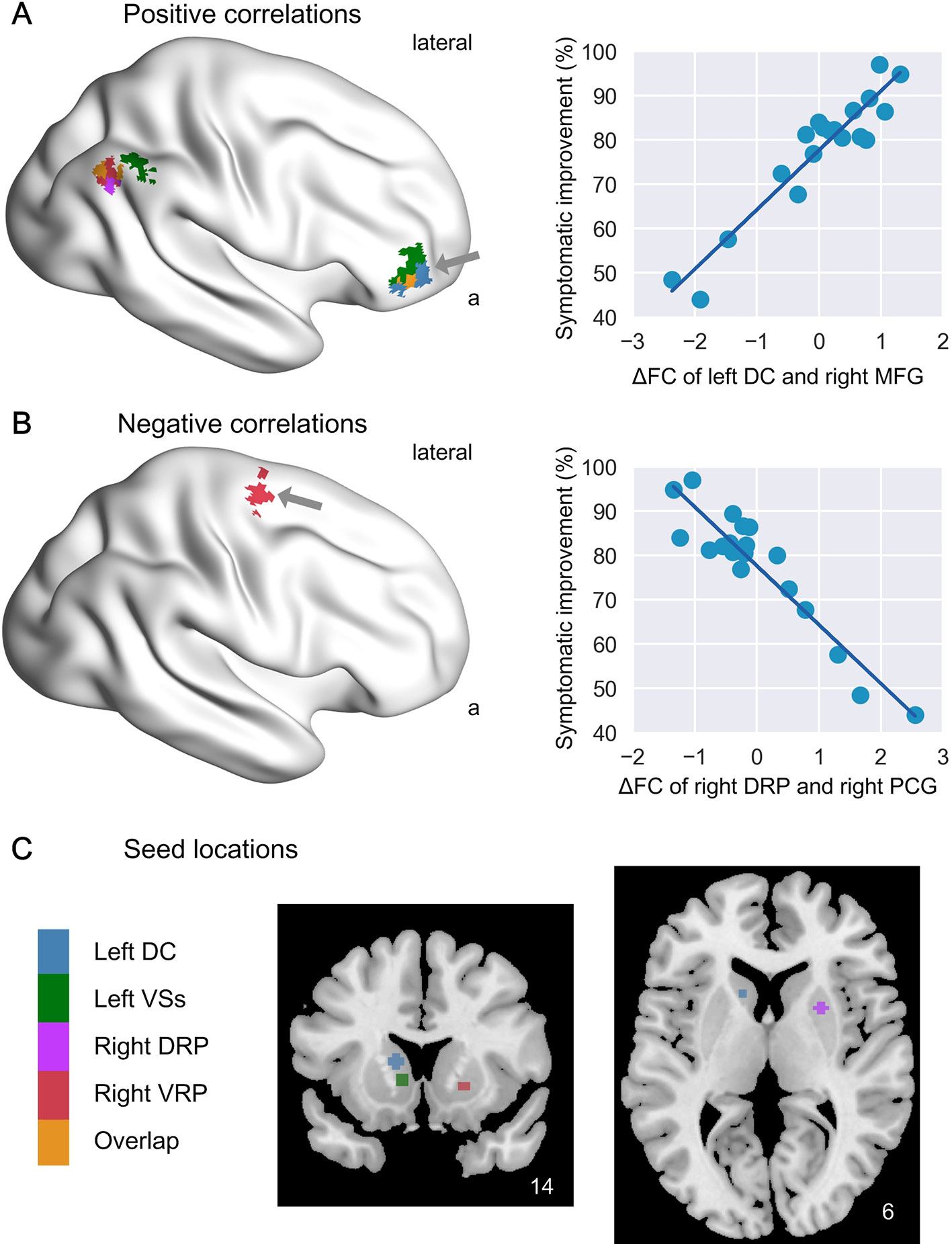
Figure 1 Association of 2-week ΔFC of striatum with 8-week symptom improvement. The image shows the brain regions whose 2-week functional connectivity alterations with different striatal subdivisions (in color) were positively (A) and negatively (B) correlated with 8-week symptom improvement, and shows the seed locations of the striatal subdivisions (C). On the right are scatters showing examples of positive association (ΔFC of left DC and right MFG, indicated by a grey arrow) and negative association (ΔFC of right DRP and right PCG). DC, dorsal caudate; VSs, superior ventral striatum; DRP, dorsal rostral putamen; VRP, ventral rostral putamen; ΔFC, functional connectivity alteration.
Negative Correlation of 2-Week ΔFC With Symptom Improvement
By contrast, greater ΔFC of striatal subdivisions with brain regions in the SMN regions after 2-week treatment was associated with worse clinical improvement at 8-week.
As shown in Figure 1 and Table 2, the ΔFC of the right DRP with right precentral gyrus (PrCG) in SMN was negatively related to treatment response at 8-week (r = -0.93). This association of ΔFC and treatment response was not driven by the effect of outlier, as evidenced by robust regression test (p < 0.001). Notably, the negative correlation happened for the cortico-striatal circuit involved in motor processing, while the positive correlation for the circuits involved in high-order cognitive processing (Figure 2).
Validation With Scrubbed Data
In order to further exclude possible confounding effect caused by head motion, we also rerun the data preprocessing with scrubbing which deleted the bad time points with large head motion (frame-wise displacement larger than 0.2 mm). The results of the analyses on scrubbed data almost remained the same as those reported above (Table S1), indicating that our findings were not susceptible to artefactual effect induced by head motion.
Discussion
The cognitive neuropsychological model of drug action proposed by Harmer et al. (39) indicates that the antidepressants affects neural substrates earlier than they change behavior, mood, and social reinforcement in MDD patients. Some research found caudomedial nucleus accumben contains equally high levels of both noradrenaline and dopamine (33), meanwhile duloxetine is a dual-action antidepressant and we suppose it may influence the reward network through increasing norepinephrine of striatum. In addition, the authors further suggest that early neural changes ultimately contribute to later symptomatic improvement (39). Our study was in accord with this model by showing that the early change of the striatum-based circuits after treatment was related to later clinically-observed treatment effects. Interestingly, the positive correlation was observed for the striatal connectivity with the FPN and DMN, which were considered as high-order brain regions involved in cognitive control and emotion processing, while the negative correlation for the striatal connectivity with the SMN considered as low-order primary brain regions (see Figure 2).
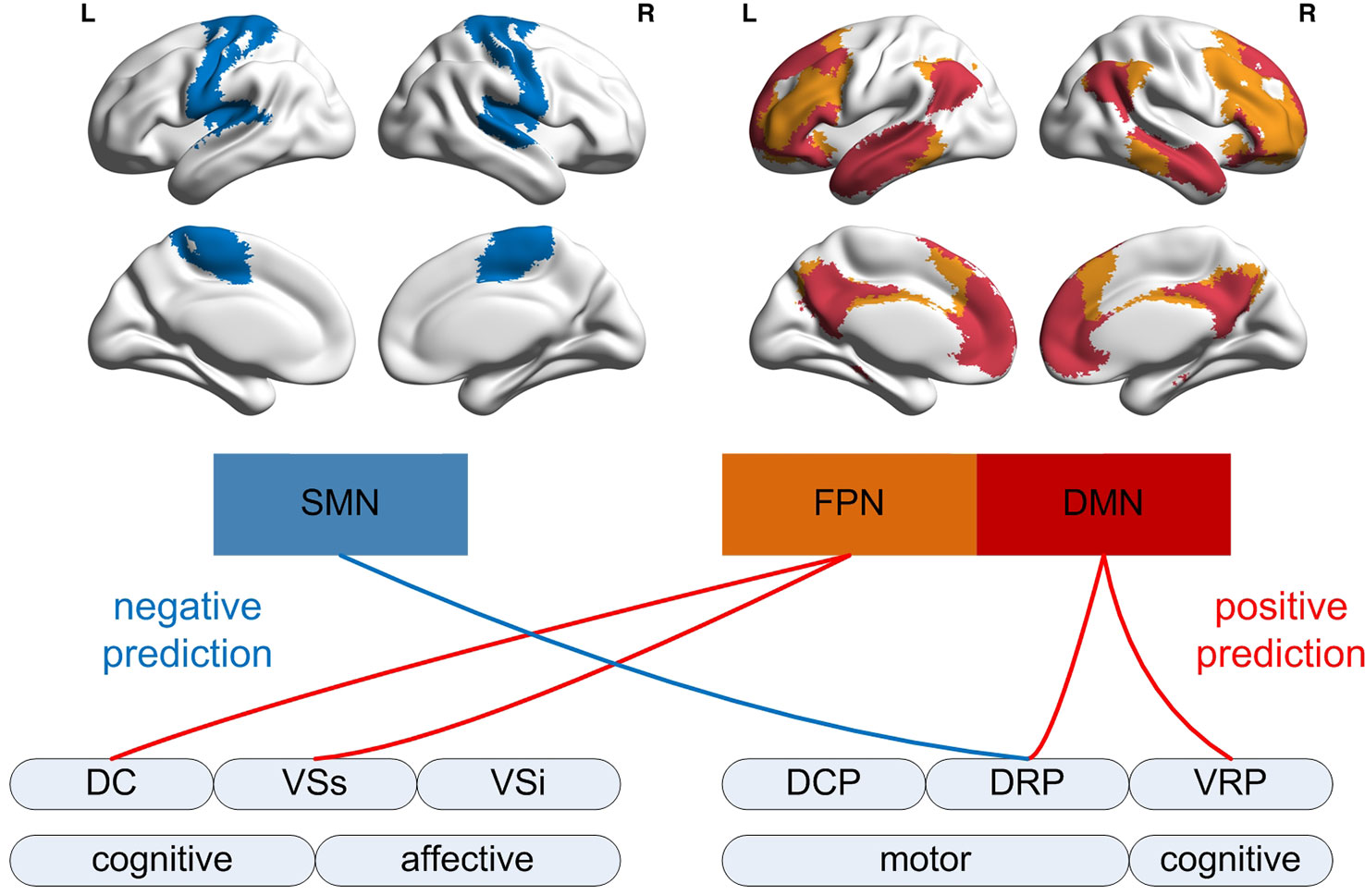
Figure 2 Association of 2-week ΔFC within the network with 8-week symptom improvement. The schematic image shows positive and negative association of functional connectivity changes between different striatal subdivisions with the regions in cortical networks defined by Yeo et al. (40). The positive association mainly involves the striatal subdivision and cortical networks for high-order cognitive processing, while the negative association mainly involves those for visual and motor processing.
Positive Association of ΔFC With Symptom Improvement
The positive association of FC changes with symptom improvement was mainly observed between the left caudate (DC and VSs) and the frontoparietal regions (MFG and IPL), indicating that the more the striatal FC was increased after 2 weeks of treatment with duloxetine, the better the treatment outcome would be after 8 weeks of treatment with duloxetine. The DC and VSs are proposed to be the cognitive subdivision of the striatum, which mainly connects to the cortical regions responsible for cognitive/executive control processing (23). The right MFG and IPL are the core regions of the frontoparietal control network (41), and located within the FPN supporting the cognitive control, defined by Yeo and colleagues (40). A previous study using meta-analysis of resting-state FC has demonstrated aberrant cortico-striatal resting-state FC within the FPN in patients with MDD (31). MDD patients are reliably acknowledged to present significant deficits of executive functions which are related to illness severity and cannot be accounted by motor slowing (42). Poor ability of cognitive control for MDD patients is predictive of their poor response to treatment (43). Our study further indicated that the importance of coordination of the striatum and FPN in action mechanism of the antidepressant. Increased communication between the stratum and FPN after 2-week antidepressant treatment may be beneficial to the cognitive bias and executive function, and then contributes to subsequent mood improvement in daily life (39). Future studies could enroll health controls to further certificate whether normalization of FC of the stratum and FPN after short-term antidepressant treatment can predict the long-term treatment efficacy.
The VSs is also proposed to be the affective/emotion subdivision of the striatum, which is in charge of emotional processing (23). Previous evidence showed decreased activation in the ventral striatum and attenuated FC between ventral striatum with the right MFG during emotion processing in MDD patients (44). The ventral striatum and dorsolateral prefrontal cortex form a neural circuit for affective control and regulation (45), and the deficit of this circuit may contribute to anhedonia, a core feature of MDD (17, 25). Top-down regulation from the PFC has been shown to trigger dopamine release to striatum (46), and dopamine signaling play a key role on mediating reward-related behaviors (47, 48).
Previous researches indeed showed that the abnormally reduced FC of the affective control circuit could be increased by long period of antidepressant treatment, accompanied by clinical symptom improvement (19, 20). Our study further found that the FC changes of the affective control circuit at early stage of treatment, even before the onset of mood change, was positively related to later treatment outcome. MDD patients, especially patients with anhedonia are inclined to positive blockade, which decreases positive emotion experienced during a pleasant event (49). In consistent with cognitive neuropsychological model of antidepressant drug action (39), we speculate that cooperation of affective control circuit may enhance the balance of positive to negative emotional processing, improving the MDD patients' capacity to interpret emotional information in a positive way, which may will be helpful for translating neural changes into clinically symptom improvement in future.
The positive association of FC changes with symptom improvement was also observed between the putamen (right DRP and right VRP) and the DMN regions (AG). The right DRP and right VRP are proposed to be the cognitive and motor subdivision of the putamen, respectively, while the putamen was proposed to be involved in reward-related processing, especially reinforcement learning. Greater cooperation between putamen and AG reflects better mediation of external demands on working memory associated with reward-related reinforcement learning (50). Our study showed antidepressant could change the communication of the putamen and DMN at the early stage of treatment, which may benefit to reward function (51), thus improving subsequent depressive symptoms improvement in a long time.
Negative Association of ΔFC With Symptom Improvement
Our findings further revealed negative association of FC changes between the striatal motor sub-division (DRP) with the SMN (right PrCG). The DRP in the striatum is involved in various motor-related functions through abundant connections with the motor regions (23), while the PrCG is considered as the primary motor area for movement execution (52). MDD is characterized by psychomotor retardation, which is reflected as slow thought and reduced physical movement (53). Un-medicated MDD patients have been shown to respond slower in simple motor tasks, suggesting deficit in their motor system (24, 54). Previous studies on medicated MDD patients has linked psychomotor retardation to increased activity of the motor-related regions and the striatum (55), but to decrease in the connectivity between these regions (30), which may result from the antidepressant effect. In our study, the negative assoication reflected that decrease in the striatal-SMN FC involved in motor execution after 2-week treatment with duloxetine was related to MDD remission, which is consistent with the finding by Liberg and Rahm (30). We speculated that changing interaction of striatal and SMN at early stage of treatment was important for enduring symptomatic improvement. Future studies are needed to clarify the abnormality of motor-related circuits associated with MDD.
Implication for Clinical Practice
The current findings provide empirical evidence supporting potential clinical practices that antidepressant aiming to potentiate 5-HT and NE transmission can change the striatal-based circuits at an early stage, and striatal FC changes within 2-week treatment may be an indicator of clinical improvement in long term. MDD patients with greater level of cognition and execution function can achieve better clinical improvement at 8-week. Without inclusion of a control group, the present study could not determine whether 2-week treatment exerted such normalized effects. It will also be necessary for future studies to have a placebo control group or a different MDD group taking other treatment regimen, to clarify the specific drug-induced mechanism underlying predictive effects of early striatum-based FC changes on later clinical improvement.
One limitation of this study was the relatively high rate of drop out of patients (15/34), which is unavoidable in clinical trial. We cannot generalize our findings to the drop-out patients, who probably do not benefit from the treatment. Another related limitation is the relatively small sample size for this study. Future studies are needed to verify the results with a larger sample size. In addition, future studies could assess whether the early neural changes can be used to predict response at an individual in a larger cohort of patients.
In conclusion, the current study revealed that neural changes of the striatal circuits after 2-week antidepressant treatment was associated with later treatment outcome for patients with MDD. The positive association was manifested for functional connectivity between multiple brain networks, especially the FPN and DMN involved in cognitive and affective control, while the negative association mainly focused on the SMN concerned with motor processing.
Data Availability Statement
The raw data supporting the conclusions of this article will be made available by the authors, without undue reservation, to any qualified researcher.
Ethics Statement
This study was carried out in accordance with the recommendation of the Ethics Committee of the Sixth Hospital (Institute of Mental Health) of Peking University with written informed consent from all subjects. All subjects gave written informed consent in accordance with the Declaration of Helsinki. The protocol was approved by the Ethics Committee of the Sixth Hospital (Institute of Mental Health) of Peking University. Written informed consent for participation was not required for this study in accordance with the national legislation and the institutional requirements.
Author Contributions
JA and LL wrote the paper. LW, Y-AS, and YW enrolled the patients. KL and YZ carried out the MRI scans. QK gave suggestions on the paper. TS and CY designed the study.
Funding
We are thankful for the funding from the National Natural Science Foundation of China (No. 81630031; 81671774), Beijing Municipal Science and Technology Project (Z171100000117016), the Capital Foundation of Medicine Research and Development (2016-1-4111), National Key R&D Program of China (2017YFC1309902), and the Hundred Talents Program of the Chinese Academy of Sciences.
Conflict of Interest
The authors declare that the research was conducted in the absence of any commercial or financial relationships that could be construed as a potential conflict of interest.
Acknowledgments
We thank Chen Chao, Fan Xiaodong, Qiao Hong, Liu Chaozhon for collecting the data for us.
Supplementary Material
The Supplementary Material for this article can be found online at: https://www.frontiersin.org/articles/10.3389/fpsyt.2019.00884/full#supplementary-material
References
1. APA. Diagnostic and statistical manual of mental disorders. 4th ed. Washington, DC: Author (2000).
2. Gaynes BN, Warden D, Trivedi MH, Wisniewski SR, Fava M, Rush AJ. What did STAR*D teach us? Results from a large-scale, practical, clinical trial for patients with depression. Psychiatr Serv (2009) 60(11):1439–45. doi: 10.1176/ps.2009.60.11.1439
3. Holtzheimer PE, Mayberg HS. Stuck in a rut: rethinking depression and its treatment. Trends Neurosci (2011) 34(1):1–9. doi: 10.1016/j.tins.2010.10.004
4. Bauer M. Updated WFSBP guidelines for the biological treatment of unipolar depressive disorders in primary care. World J Biol Psychiatry (2007) 8(2):66. doi: 10.1080/15622970701308413
5. Nierenberg AA. Predictors of response to antidepressants general principles and clinical implications. Psychiatr Clin North Am (2003) 26(2):345–52 doi: 10.1016/S0193-953X(02)00105-3
6. Szegedi A, Jansen WT, van Willigenburg AP, van der Meulen E, Stassen HH, Thase ME. Early improvement in the first 2 weeks as a predictor of treatment outcome in patients with major depressive disorder: a meta-analysis including 6562 patients. J Clin Psychiatry (2009) 70(3):344–53. doi: 10.4088/jcp.07m03780
7. Fonseka TM, MacQueen GM, Kennedy SH. Neuroimaging biomarkers as predictors of treatment outcome in major depressive disorder. J Affect Disord (2017) 233:21–35. doi: 10.1016/j.jad.2017.10.049
8. Kupfer DJ, Frank E, Phillips ML. Major depressive disorder: new clinical, neurobiological, and treatment perspectives. Lancet (2012) 379:1045–55. doi: 10.1016/S0140-6736(11)60602-8
9. Schultz W. Behavioral dopamine signals. Trends Neurosci (2007) 30:203–10. doi: 10.1016/j.tins.2007.03.007
10. Epstein J, Pan H, Kocsis JH, Yang Y, Butler T, Chusid J, et al. Lack of ventral striatal response to positive stimuli in depressed versus normal subjects. Am J Psychiatry (2006) 163:1784–90. doi: 10.1176/ajp.2006.163.10.1784
11. Smoski MJ, Felder J, Bizzell J, Green SR, Ernst M, Lynch TR, et al. fMRI of alterations in reward selection, anticipation, and feedback in major depressive disorder. J Affect Disord (2009) 118:69–78. doi: 10.1016/j.jad.2009.01.034
12. Chen CH, Ridler K, Suckling J, Williams S, Fu CH, Merlo-Pich E, et al. Brain imaging correlates of depressive symptom severity and predictors of symptom improvement after antidepressant treatment. Biol Psychiatry (2007) 62:407–14. doi: 10.1016/j.biopsych.2006.09.018
13. Phillips ML, Chase HW, Sheline YI, Etkin A, Almeida JR, Deckersbach T, et al. Identifying predictors, moderators, and mediators of antidepressant response in major depressive disorder: neuroimaging approaches. Am J Psychiatry (2015) 172:124–38. doi: 10.1176/appi.ajp.2014.14010076
14. Pizzagalli DA. Frontocingulate dysfunction in depression: toward biomarkers of treatment response. Neuropsychopharmacology (2011) 36:183–206. doi: 10.1038/npp.2010.166
15. Godlewska BR, Browning M, Norbury R, Cowen PJ, Harmer CJ. Early changes in emotional processing as a marker of clinical response to SSRI treatment in depression. Transl Psychiatry (2016) 6(11):e957. doi: 10.1038/tp.2016.130
16. Furman DJ, Hamilton JP, Gotlib IH. Frontostriatal functional connectivity in major depressive disorder. Biol Mood Anxiety Disord (2011) 1(1):11. doi: 10.1186/2045-5380-1-11
17. Gabbay V, Ely BA, Li Q, Bangaru SD, Panzer AM, Alonso CM, et al. Striatum-based circuitry of adolescent depression and anhedonia. J Am Acad Child Adolesc Psychiatry (2013) 52(6):628–641 e613. doi: 10.1016/j.jaac.2013.04.003
18. Sturm V, Lenartz D, Koulousakis A, Treuer H, Herholz K, Klein JC, et al. The nucleus accumbens: a target for deep brain stimulation in obsessive-compulsive- and anxiety-disorders. J Chem Neuroanat (2003) 26(4):293–9. doi: 10.1016/j.jchemneu.2003.09.003
19. Heller AS, Johnstone T, Light SN, Peterson MJ, Kolden GG, Kalin NH, et al. Relationships between changes in sustained fronto-striatal connectivity and positive affect in major depression resulting from antidepressant treatment. Am J Psychiatry (2013) 170(2):197–206. doi: 10.1176/appi.ajp.2012.12010014
20. Admon R, Kaiser RH, Dillon DG, Beltzer M, Goer F, Olson DP, et al. Dopaminergic enhancement of striatal response to reward in major depression. Am J Psychiatry (2017) 174(4):378–86. doi: 10.1176/appi.ajp.2016.16010111
21. Forbes EE, Olino TM, Ryan ND, Birmaher B, Axelson D, Moyles DL, et al. Reward-related brain function as a predictor of treatment response in adolescents with major depressive disorder. Cognit Affect Behav Neurosci (2010) 10(1):107–18. doi: 10.3758/CABN.10.1.107
22. Walsh E, Carl H, Eisenlohr-Moul T, Minkel J, Crowther A, Moore T, et al. Attenuation of frontostriatal connectivity during reward processing predicts response to psychotherapy in major depressive disorder. Neuropsychopharmacology (2017) 42 (4):831–43. doi: 10.1038/npp.2016.179
23. Di Martino A, Scheres A, Margulies DS, Kelly AM, Uddin LQ, Shehzad Z, et al. Functional connectivity of human striatum: a resting state FMRI study. Cereb Cortex (2008) 18(12):2735–47. doi: 10.1093/cercor/bhn041
24. Pier MP, Hulstijn W, Sabbe BG. Differential patterns of psychomotor functioning in unmedicated melancholic and nonmelancholic depressed patients. J Psychiatr Res (2004) 38(4):425–35. doi: 10.1016/j.jpsychires.2003.11.008
25. Der-Avakian A, Markou A. The neurobiology of anhedonia and other reward-related deficits. Trends Neurosci (2012) 35(1):68–77. doi: 10.1016/j.tins.2011.11.005
26. Pizzagalli DA. Depression, stress, and anhedonia: toward a synthesis and integrated model. Annu Rev Clin Psychol (2014) 10:393–423. doi: 10.1146/annurev-clinpsy-050212-185606
27. Pizzagalli DA, Oakes TR, Fox AS, Chung MK, Larson CL, Abercrombie HC, et al. Functional but not structural subgenual prefrontal cortex abnormalities in melancholia. Mol Psychiatry (2004) 9(4):325, 393–405. doi: 10.1038/sj.mp.4001469
28. Bennabi D, Vandel P, Papaxanthis C, Pozzo T, Haffen E. Psychomotor retardation in depression: a systematic review of diagnostic, pathophysiologic, and therapeutic implications. BioMed Res Int (2013) 2013:158746. doi: 10.1155/2013/158746
29. Liberg B, Klauser P, Harding IH, Adler M, Rahm C, Lundberg J, et al. Functional and structural alterations in the cingulate motor area relate to decreased fronto-striatal coupling in major depressive disorder with psychomotor disturbances. Front Psychiatry (2014) 5:176. doi: 10.3389/fpsyt.2014.00176
30. Liberg B, Rahm C. The functional anatomy of psychomotor disturbances in major depressive disorder. Front Psychiatry (2015) 6:34. doi: 10.3389/fpsyt.2015.00034
31. Kaiser RH, Andrews-Hanna JR, Wager TD, Pizzagalli DA. Large-Scale network dysfunction in major depressive disorder: a meta-analysis of resting-state functional connectivity. JAMA Psychiatry (2015) 72(6):603–11. doi: 10.1001/jamapsychiatry.2015.0071
32. Chalon SA, Granier LA, Vandenhende FR, Bieck PR, Bymaster FP, Joliat MJ, et al. Duloxetine increases serotonin and norepinephrine availability in healthy subjects: a double-blind, controlled study. Neuropsychopharmacology (2003) 28(9):1685–93. doi: 10.1038/sj.npp.1300209
33. Tong J, Hornykiewicz O, Kish SJ. Identification of a noradrenaline-rich subdivision of the human nucleus accumbens. J Neurochem (2006) 96(2):349–54. doi: 10.1111/j.1471-4159.2005.03546.x
34. Sheehan DV, Lecrubier Y, Sheehan KH, Amorim P, Janavs J, Weiller E, et al. The Mini-International Neuropsychiatric Interview (M.I.N.I.): the development and validation of a structured diagnostic psychiatric interview for DSM-IV and ICD-10. J Clin Psychiatry (1998) 59 Suppl 20:22–33;quiz 34-57.
35. Hamilton M. Development of a rating scale for primary depressive illness. Br J Soc Clin Psychol (1967) 6(4):278–96. doi: 10.1111/j.2044-8260.1967.tb00530.x
36. Yan CG, Wang XD, Zuo XN, Zang YF. DPABI: Data Processing & Analysis for (Resting-State) Brain Imaging. Neuroinformatics (2016) 14(3):339–51. doi: 10.1007/s12021-016-9299-4
37. Power JD, Barnes KA, Snyder AZ, Schlaggar BL, Petersen SE. Spurious but systematic correlations in functional connectivity MRI networks arise from subject motion. Neuroimage (2012) 59(3):2142–54. doi: 10.1016/j.neuroimage.2011.10.018
38. Power JD, Mitra A, Laumann TO, Snyder AZ, Schlaggar BL, Petersen SE. Methods to detect, characterize, and remove motion artifact in resting state fMRI. Neuroimage (2014) 84:320–41. doi: 10.1016/j.neuroimage.2013.08.048
39. Harmer CJ, Goodwin GM, Cowen PJ. Why do antidepressants take so long to work? A cognitive neuropsychological model of antidepressant drug action. Br J Psychiatry (2009) 195(2):102–8. doi: 10.1192/bjp.bp.108.051193
40. Yeo BT, Krienen FM, Sepulcre J, Sabuncu MR, Lashkari D, Hollinshead M, et al. The organization of the human cerebral cortex estimated by intrinsic functional connectivity. J Neurophysiol (2011) 106:1125–65. doi: 10.1152/jn.00338.2011
41. Vincent JL, Kahn I, Snyder AZ, Raichle ME, Buckner RL. Evidence for a frontoparietal control system revealed by intrinsic functional connectivity. J Neurophysiol (2008) 100(6):3328–42. doi: 10.1152/jn.90355.2008
42. Snyder HR. Major depressive disorder is associated with broad impairments on neuropsychological measures of executive function: a meta-analysis and review. Psychol Bull (2013) 139(1):81–132. doi: 10.1037/a0028727
43. Gotlib IH, Joormann J. Cognition and depression: current status and future directions. Annu Rev Clin Psychol (2010) 6:285–312. doi: 10.1146/annurev.clinpsy.121208.131305
44. Heller AS, Johnstone T, Shackman AJ, Light SN, Peterson MJ, Kolden GG, et al. Reduced capacity to sustain positive emotion in major depression reflects diminished maintenance of fronto-striatal brain activation. Proc Natl Acad Sci U. S. A. (2009) 106(52):22445–50. doi: 10.1073/pnas.0910651106
45. Wager TD, Davidson ML, Hughes BL, Lindquist MA, Ochsner KN. Prefrontal-subcortical pathways mediating successful emotion regulation. Neuron (2008) 59(6):1037–50. doi: 10.1016/j.neuron.2008.09.006
46. Del Arco A, Mora F. Prefrontal cortex-nucleus accumbens interaction: in vivo modulation by dopamine and glutamate in the prefrontal cortex. Pharmacol Biochem Behav (2008) 90(2):226–35. doi: 10.1016/j.pbb.2008.04.011
47. Wise RA. Dopamine, learning and motivation. Nat Rev Neurosci (2004) 5(6):483–94. doi: 10.1038/nrn1406
48. Berridge KC. The debate over dopamine's role in reward: the case for incentive salience. Psychopharmacol (Berl) (2007) 191(3):391–431. doi: 10.1007/s00213-006-0578-x
50. Hearne L, Cocchi L, Zalesky A, Mattingley JB. Interactions between default mode and control networks as a function of increasing cognitive reasoning complexity. Hum Brain Mapp (2015) 36(7):2719–31. doi: 10.1002/hbm.22802
51. Pizzagalli DA, Jahn AL, O'Shea JP. Toward an objective characterization of an anhedonic phenotype: a signal-detection approach. Biol Psychiatry (2005) 57(4):319–27. doi: 10.1016/j.biopsych.2004.11.026
52. Meier JD, Aflalo TN, Kastner S, Graziano MS. Complex organization of human primary motor cortex: a high-resolution fMRI study. J Neurophysiol (2008) 100(4):1800–12. doi: 10.1152/jn.90531.2008
53. Buyukdura JS, McClintock SM, Croarkin PE. Psychomotor retardation in depression: biological underpinnings, measurement, and treatment. Prog Neuropsychopharmacol Biol Psychiatry (2011) 35(2):395–409. doi: 10.1016/j.pnpbp.2010.10.019
54. Sabbe B, Hulstijn W, van Hoof J, Tuynman-Qua HG, Zitman F. Retardation in depression: assessment by means of simple motor tasks. J Affect Disord (1999) 55(1):39–44. doi: 10.1016/s0165-0327(98)00087-1
Keywords: major depressive disorder, antidepressant, striatum, prediction, clinical improvement
Citation: An J, Li L, Wang L, Su Y-A, Wang Y, Li K, Zeng Y, Kong Q, Yan C and Si T (2019) Striatal Functional Connectivity Alterations After Two-Week Antidepressant Treatment Associated to Enduring Clinical Improvement in Major Depressive Disorder. Front. Psychiatry 10:884. doi: 10.3389/fpsyt.2019.00884
Received: 20 May 2019; Accepted: 11 November 2019;
Published: 06 December 2019.
Edited by:
Wenbin Guo, Central South University, ChinaReviewed by:
Luke Norman, University of Michigan, United StatesChien-Han Lai, National Yang-Ming University, Taiwan
Copyright © 2019 An, Li, Wang, Su, Wang, Li, Zeng, Kong, Yan and Si. This is an open-access article distributed under the terms of the Creative Commons Attribution License (CC BY). The use, distribution or reproduction in other forums is permitted, provided the original author(s) and the copyright owner(s) are credited and that the original publication in this journal is cited, in accordance with accepted academic practice. No use, distribution or reproduction is permitted which does not comply with these terms.
*Correspondence: Chaogan Yan, eWFuY2dAcHN5Y2guYWMuY24=; Tianmei Si, c2kudGlhbi1tZWlAMTYzLmNvbQ==
†These authors have contributed equally to this work