- 1Department of Radiology, The First Affiliated Hospital of Wenzhou Medical University, Wenzhou, China
- 2Department of Radiology, The Second Affiliated Hospital Zhejiang University School of Medicine, Hangzhou, China
- 3School of Laboratory Medicine and Life Sciences, Wenzhou Medical University, Wenzhou, China
- 4School of Medicine, Indiana University, Indianapolis, IN, United States
- 5Department of Psychiatry, The First Affiliated Hospital of Wenzhou Medical University, Wenzhou, China
- 6Key Laboratory of Diagnosis and Treatment of Severe Hepato-Pancreatic Diseases of Zhejiang Province, Zhejiang Provincial Top Key Discipline in Surgery, The First Affiliated Hospital of Wenzhou Medical University, Wenzhou, China
Objective: To analyze the correlation between susceptibility single nucleotide polymorphisms (SNPs) and the severity of clinical symptoms in children with attention deficit hyperactivity disorder (ADHD), so as to supplement the clinical significance of gene polymorphism and increase our understanding of the association between genetic mutations and ADHD phenotypes.
Methods: 193 children with ADHD were included in our study from February 2017 to February 2020 in the Children’s ADHD Clinic of the author’s medical institution. 23 ADHD susceptibility SNPs were selected based on the literature, and multiple polymerase chain reaction (PCR) targeted capture sequencing technology was used for gene analysis. A series of ADHD-related questionnaires were used to reflect the severity of the disease, and the correlation between the SNPs of specific sites and the severity of clinical symptoms was evaluated. R software was used to search for independent risk factors by multivariate logistic regression and the “corplot” package was used for correlation analysis.
Results: Among the 23 SNP loci of ADHD children, no mutation was detected in 6 loci, and 2 loci did not conform to Hardy-Weinberg equilibrium. Of the remaining 15 loci, there were 9 SNPs, rs2652511 (SLC6A3 locus), rs1410739 (OBI1-AS1 locus), rs3768046 (TIE1 locus), rs223508 (MANBA locus), rs2906457 (ST3GAL3 locus), rs4916723 (LINC00461 locus), rs9677504 (SPAG16 locus), rs1427829 (intron) and rs11210892 (intron), correlated with the severity of clinical symptoms of ADHD. Specifically, rs1410739 (OBI1-AS1 locus) was found to simultaneously affect conduct problems, control ability and abstract thinking ability of children with ADHD.
Conclusion: There were 9 SNPs significantly correlated with the severity of clinical symptoms in children with ADHD, and the rs1410739 (OBI1-AS1 locus) may provide a new direction for ADHD research. Our study builds on previous susceptibility research and further investigates the impact of a single SNP on the severity of clinical symptoms of ADHD. This can help improve the diagnosis, prognosis and treatment of ADHD.
Introduction
Attention deficit hyperactivity disorder (ADHD) is a common neurodevelopmental disorder that affects between 2 and 7% of children worldwide (1, 2), and a growing body of literature supports the notion that the disease persists into adulthood in most cases (3). It seriously impairs the individual’s ability to function in academic, career and social environments, and has adverse effects on individuals, families and society as a whole (4, 5). Clinically, ADHD is characterized by a considerable degree of hyperactivity, impulsivity and inattention (6). Previous studies have shown that ADHD is usually persistent and significantly impairs health, with an increased risk of poor overall outcomes (7). Adolescents with ADHD are at risk for other psychiatric disorders in childhood, adolescence, and adulthood, including mood, anxiety, and substance use disorders, and may even lead to criminal behavior (6). Therefore, studies on the etiology and pathophysiological mechanism of ADHD have been of great scientific concern.
Some epidemiological and clinical studies have demonstrated that genetic and environmental risk factors influence the structural and functional ability of brain networks involved in behavior and cognition in the etiology of ADHD (7). Studies of families, twins and foster children suggest that ADHD is familial, in which up to 80% of the different phenotypes can be explained by genetic variation (8). In addition, there is considerable genetic overlap between ADHD and other psychiatric disorders, such as antisocial personality disorder/behavior, cognitive disorders, autism spectrum disorders, schizophrenia, bipolar disorder, and major depressive disorder (9–15). A recent cross-trait meta-analysis identified pleiotropic genomic loci responsible for ADHD, autism spectrum disorder, and intelligence. The research also found that ADHD was associated with inheriting a reduced set of low-intelligence alleles (16). Thus, genetic factors can be seen to play an important role in the development of the disease, and both common and rare genetic variants are associated with the risk of ADHD (17, 18).
Previous studies have shown that the risk of a common genetic variant of ADHD, also known as single nucleotide polymorphism (SNP) heritability, is also associated with depression, behavioral problems, schizophrenia, persistent measures of ADHD symptoms and other neurodevelopmental disorders (7). Recently, the SNP panel has been widely used in population genetics studies (19, 20). Here, based on previous susceptibility studies, we plan to further analyze the effect of SNPs on the clinical severity of ADHD.
Recently, a combined sample of 55,374 individuals from an international collaboration for genome-wide association study (GWAS) was used to identify the first genome-wide salient loci for ADHD. This genome-wide meta-analysis provided the results of 12 ADHD-associated loci and their genome-wide significant index variations (7). 11 of these loci were selected for this study based on the form of the SNPs (rs11420276, rs1222063, rs9677504, rs4858241, rs28411770, rs4916723, rs74760947, rs11591402, rs1427829, rs281324, and rs212178). On this basis, in order to strengthen the clinical diagnosis of ADHD in the case of extreme expression of one or more heritable quantitative traits, 12 other previously reported ADHD susceptibility SNPs were included according to the literature (rs3768046, rs1199039, rs11210892, rs12741964, rs2906457, rs1410739, rs223508, rs429699, rs27048, rs2652511, rs11564750, and rs10044618) (21–24). Since ADHD susceptibility SNPs were focused on, the whole gene detection was not conducted, but susceptibility loci were chosen based on previous findings: ADHD-related loci were selected via GWAS and transcriptome-wide association study (TWAS) (7, 21); at the same time, because dopamine-related genes have been considered as candidate genes for ADHD heritability (25, 26), ADHD susceptibility SNPs were also screened and selected at dopamine-related loci (22–24). For example, rs27048 and rs429699 have been reported to be genetic markers of ADHD-inattention subtype (ADHD-I) (23), and rs2652511 was found to be significantly related to ADHD-combined subtype (ADHD-C) (24). Then the amplification and extension products of twenty-three SNP sites were designed and made into a panel to detect the mutation of gene loci, so as to evaluate the influence of SNPs on the severity of clinical symptoms of ADHD.
Materials and methods
After enrollment and exclusion criteria, different ADHD-related questionnaires, such as Conners parent symptom questionnaire, Stroop color-word test, Wisconsin card sorting test, et al., were used for assessment. The indicators from the questionnaires were then used to reflect the severity of different clinical symptoms of ADHD. DNA was extracted from venous blood for genotyping and bioinformatics analysis to obtain mutations in SNPs. Then the influence of SNPs on the severity of clinical symptoms of ADHD can be evaluated by correlational analysis between the indicators in the questionnaire results and the mutation status of SNPs. The workflow is shown in Figure 1.
Participants
A total of 193 children with ADHD were included in our study from February 2017 to February 2020 in the Children’s ADHD Clinic of the author’s medical institution, including 163 males and 32 females, aged 6–15 (8.87 ± 2.17) years. Inclusion criteria: (1) meet the diagnostic criteria for ADHD according to the Diagnostic and Statistical Manual of Mental Disorders – the Fifth Edition (DSM-V); (2) complete questionnaire data; (3) complete magnetic resonance imaging (MRI) data, including sequences of T1-weighted imaging (T1WI), T2-weighted imaging and diffusion-weighted imaging (DWI), which showed no organic lesions. Exclusion criteria: (1) intellectual disability, i.e., full-scale IQ (FIQ) < 70 according to the Chinese Wechsler Intelligence Scale for Children (C-WISC); (2) serious physical diseases, such as epilepsy, congenital abnormalities, cerebrovascular diseases and autoimmune diseases; (3) other psychiatric disorders, such as autism spectrum disorders, schizophrenia, bipolar disorder, major depression, alcohol and substance use disorders; (4) drug abuse or dependence; (5) incomplete MRI data or MRI showed organic lesions; (6) family history of mental illness. The study, including all objectives and experimental procedures, has been approved by the Ethics Committee of Clinical Research in the First Affiliated Hospital of Wenzhou Medical University (Ethics Audit Number: KY2018-162).
Clinical data collection
Clinical data were collected through electronic medical records. Gender, age, date of birth, grade of schooling, mode of delivery, past medical history and family history of mental illness were recorded.
Variables and data sources
Chinese Wechsler intelligence scale for children
The C-WISC (27) was used to evaluate the IQ of children. The measurement results of the scale were expressed by verbal IQ (VIQ), performance IQ (PIQ) and full-scale IQ (FIQ), which were used to illustrate children’s cognitive ability. Higher IQ scores reflect better overall cognitive ability. According to their FIQ score, they were stratified into six grades: “A” means FIQ ≥ 130, “B” means 120 ≤ FIQ < 130, “C” means 110 ≤ FIQ < 120, “D” means 90 ≤ FIQ < 110, “E” means 80 ≤ FIQ < 90, “F” means 70 ≤ FIQ < 80; they would be listed by “FIQ (Grade)” in the results.
Conners parent symptom questionnaire
The Conners parent symptom questionnaire (PSQ) was used to assess the symptoms in children with ADHD. The scale consisted of 48 items and 6 subscales, which reflect 6 clinical phenotypes of ADHD, respectively, including conduct problems, psychosomatic disorders, anxiety, learning problems, hyperactivity/impulsivity and hyperactivity indices. Each score is based on a scale of four from 0 to 3: “0” means no exception; “1” means occasionally a little or slightly; “2” means frequent or more severe; “3” means very common or very serious. A Z-score was calculated by adding up the scores and dividing it by the number of items. Each phenotype was graded based on gender and PSQ score: “Y” indicates the presence of the phenotype, and “N” indicates the opposite (28, 29).
Stroop color-word test
The Stroop color-word test was used to assess control capabilities. The test mainly measures the ability of perceptual switching, selective attention and the ability to inhibit habitual response patterns, and is sensitive to the plasticity of mental control and response in executive function (30, 31). In the Stroop color-word test, 126 words were randomly arranged in 14 × 9 (rows × columns). The test consisted of three parts: part A named the color blocks (red, blue, green, or yellow); part B involved reading words of color printed in black (“red,” “blue,” “green,” and “yellow”); part C asked for naming the color of the printed word, which was inconsistent with the word itself (for example, the word “red” was printed in blue). There was a 60-s break after each part, and a 100 ms-long “+” was presented before the next part began. The participants were asked to respond accurately, and as quickly as possible by pressing the appropriate button. Stimuli were presented one by one. Reaction time (RT) and errors were recorded. The Stroop intervention score (IG) was obtained by formula C − [(A * B)/(A + B)]. The higher the IG, the more severe the cognitive control deficit. If the participants felt tired during the task, the rest time was extended. All participants completed the test (32–34).
Wisconsin card sorting test
The Wisconsin card sorting test (WCST) was used to assess the ability of abstract thinking. It consisted of 128 cards made up of different colors (red, yellow, green, and blue), shapes (triangle, cross, circle, and pentacle), and the number of shapes. The four templates were 1 red triangle, 2 pentacles, 3 yellow crosses, and 4 blue circles. In this research, the indexes used to evaluate cognitive function were: total test number, correct response number, wrong response number, persistent wrong response number, non-persistent wrong response number and completed classification number (35, 36).
Integrated visual and auditory continuous performance test
The Integrated visual and auditory continuous performance test (IVA-CPT) was used to evaluate the degree of dysfunctions in response control, attention and audiovisual integration in children. Persistent operation test software (US, Braintrain, IVA-CPT 3.0) was used to test the enrolled subjects. The IVA database automatically recorded the response control quotient, attention quotient and 29 other quotient indexes. During this study, the auditory control quotient, auditory attention quotient, visual control quotient, visual attention quotient, comprehensive control quotient and comprehensive attention quotient were selected to assess persistent attention deficits in children with ADHD (37–39).
Genotyping
DNA was extracted from venous blood by the Phenol-Chloroform method. Multiple polymerase chain reaction (PCR) targeted capture sequencing technology was used for gene analysis. For each coding sequence of the selected gene locus, a 120 bp probe sequence was designed from the first base in the direction of 5′–3′ according to the principle of sequence reverse complementation, and there was a 60 bp overlap between each two adjacent probe sequences. Sequences of CAAGCAGAAGACGGCATACGAGAT and GTGACTGGAGTTCAGACGTG were added to the 5′ and 3′ ends of each probe sequence, respectively; in situ synthesis of oligonucleotides was carried out on a large scale on a chip; the oligonucleotides on the chip were eluted with ammonia water and dissolved in water to form an oligonucleotide mixture. And through PCR, forward and reverse primers (Supplementary Table 1) with the 5′-end biotin-labeled oligonucleotide mixture were amplified to form a DNA probe library with biotin-labeled ADHD-related genes. The multi-PCR library construction and acquisition process is shown in Figure 2.
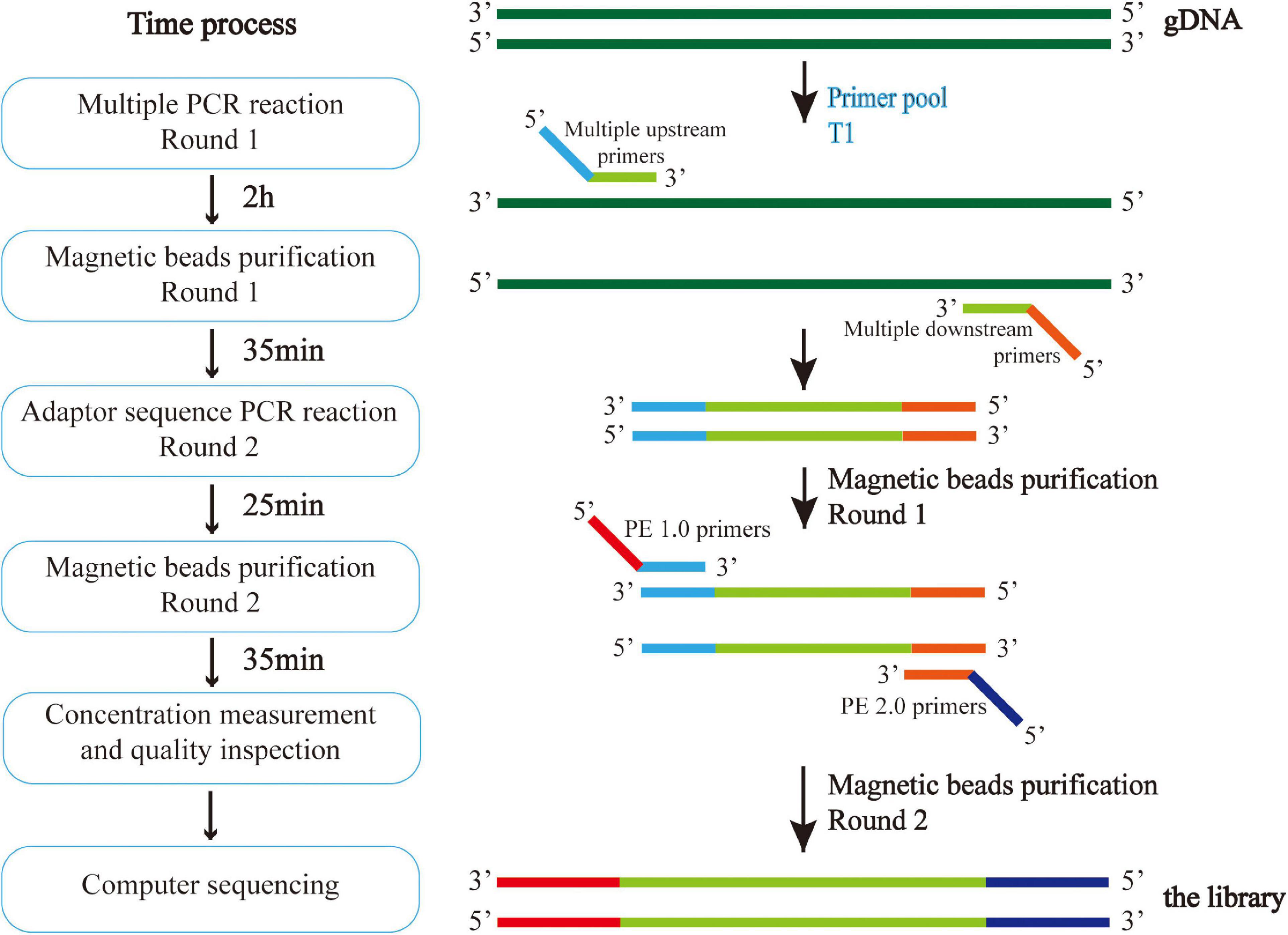
Figure 2. Multiple PCR technology was used to amplify multiple target regions of genomic DNA at the same time to obtain the amplicon. Then, the second generation sequencing connector was added to both sides of the amplicon by PCR to obtain the amplicon library, and the second generation sequencing was carried out to obtain the sequence information of the target region. The experiment was conducted in strict accordance with the library construction process.
Bioinformatics analysis
After the original Sequenced Reads were obtained, the information analysis process was carried out by referring to the genome (GRCh38/HG38), including sequencing data quality assessment and variation detection. The former mainly carries out statistics on data volume, base quality, comparison rate, coverage rate, capture rate, uniformity and other indicators, and evaluates whether the database sequencing meets the standards. If it meets the standards, subsequent analyses will be conducted. The latter, compares high-quality sequences to the human reference genome, detects the variation information in the sample, and also makes statistics and annotations of the detected variation. The multiplex PCR bioinformatics analysis process is shown in Figure 3.
Statistics
The double-check method was adopted for data entry. SPSS statistic (Version 24.0)1 was used to test the genetic balance fit degree of the Hardy–Weinberg law on population data. Correlation analysis was conducted between the indicators in the questionnaire results and the mutation of SNPs. R software (Version 4.0)2 was used to search for independent risk factors by multivariate logistic regression and the “corrplot” package was used for correlation analysis. A correlation was considered positive if the value of the correlation coefficient (rho) was >0, moderate positive if the rho was >0.2, weak negative if the rho was −0.2 ∼ 0, and moderate negative if the rho was ≤−0.2.
Results
Coincidence test of Hardy–Weinberg equilibrium
Sequencing and information analysis were performed on 23 loci of ADHD children, and no mutations were detected in 6 loci (rs11420276, rs12741964, rs11591402, rs28411770, rs11564750, and rs74760947). The coincidence test of Hardy–Weinberg equilibrium was performed on 17 loci with mutations. The results showed that the observations of 15 loci fit well with the expectations, conforming to Hardy–Weinberg equilibrium (P > 0.05), which was representative of the population, suggesting that the population in this study was genetically balanced. However, the genotype frequency distribution of rs1222063 and rs10044618 was not consistent with the Hardy–Weinberg equilibrium test (P < 0.05), and was therefore, not representative of the population. The results are shown in Table 1.
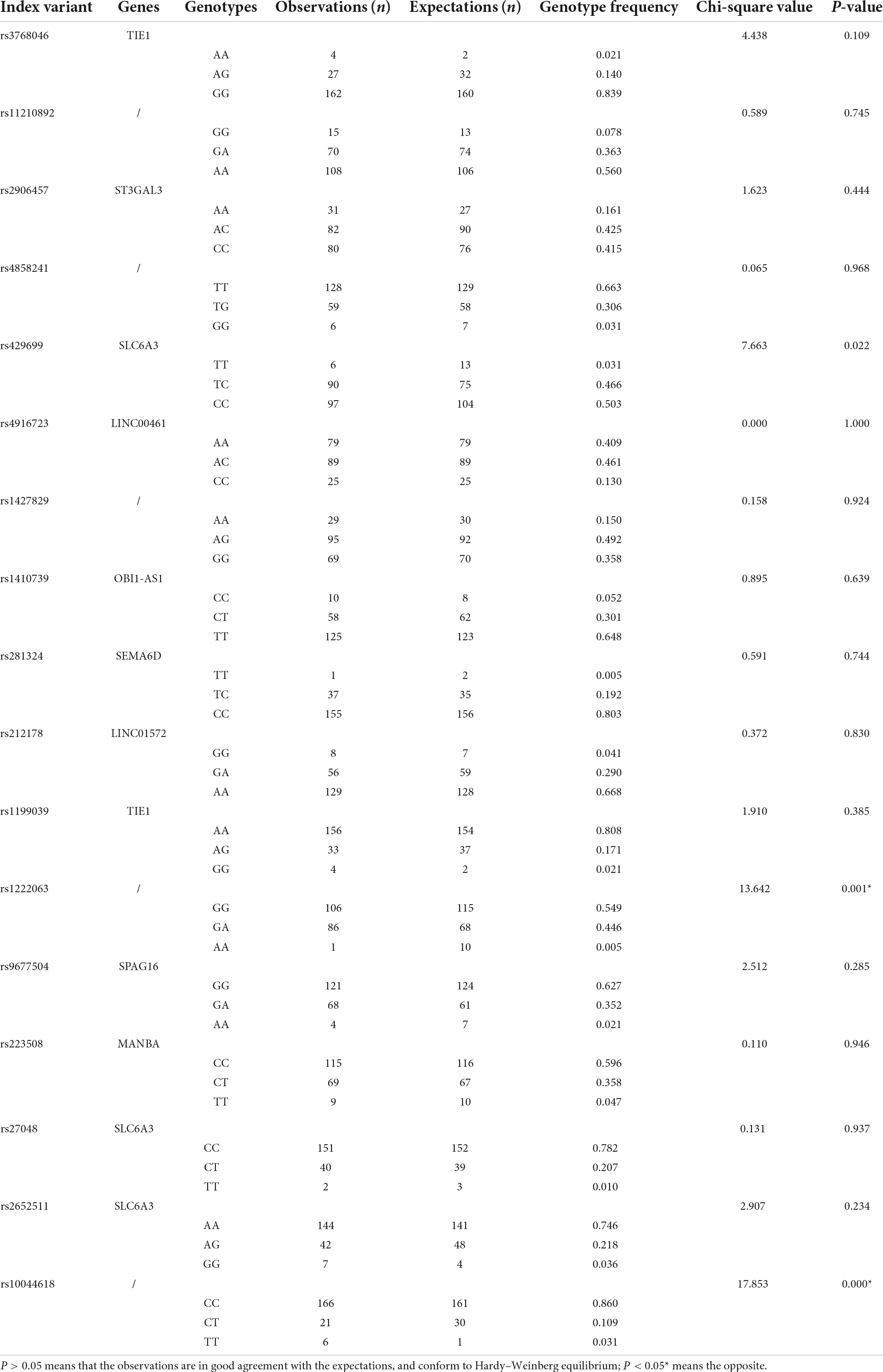
Table 1. Coincidence test of Hardy–Weinberg equilibrium of 17 attention deficit hyperactivity disorder (ADHD)-related loci in 193 children with ADHD.
Correlation analysis between single nucleotide polymorphisms and the severity of clinical symptoms of attention deficit hyperactivity disorder
The 15 SNPs obtained in the previous step and the clinical symptom characteristics of ADHD were analyzed, including 30 items from C-WISC, PSQ, Stroop color-word test, WCST and IVA-CPT; from this, the heatmap was made. The results showed that one SNP (rs9677504) and the psychosomatic disorders of PSQ had a moderate-negative correlation (the rho was −0.20), and a moderate-positive correlation with the persistent error number of WCST (the rho was 0.23); one SNP (rs223508) had a moderate-negative correlation with verbal IQ (the rho was −0.21), a moderate-negative correlation with correct numbers of WCST (the rho was −0.20), and a moderate-negative correlation with error numbers of WCST (the rho was −0.20); another SNP (rs2652511) had a moderate-positive correlation with anxiety (Grade) of PSQ (the rho was 0.24). Some SNPs, rs1427829 and rs223508, showed relatively high correlation coefficients with ADHD clinical symptoms (the rho >0.10); they had the largest number of items, 11 and 15, respectively. One SNP (rs212178) had a low correlation with the clinical symptoms and characteristics of ADHD, and there was no correlation coefficient of more than 0.10. The results are shown in Figure 4, and the specific correlation coefficients can be seen in Supplementary Table 2.
Multivariate analysis results of the influence of single nucleotide polymorphisms on IQ differences of children with attention deficit hyperactivity disorder
As shown in Supplementary Table 3, multivariate analysis showed that one SNP (rs223508) was an independent risk factor for the decrease of VIQ (OR = 0.98, P = 0.006) and FIQ (OR = 0.98, P = 0.020) of ADHD children.
Multivariate analysis results of the influence of single nucleotide polymorphisms on various clinical phenotypes of attention deficit hyperactivity disorder
Based on the results of the PSQ questionnaire, 5 SNPs have an impact on various clinical phenotypes of ADHD. Multivariate analysis showed that one SNP (rs1427829) was an independent risk factor for anxiety (OR = 4.89, P < 0.001) and anxiety (Grade) (P = 0.020), and similarly, one SNP (rs2652511) was an independent risk factor for anxiety (OR = 2.41, P = 0.024) and anxiety (Grade) (P = 0.003). It was also found that two SNPs were independent risk factors for conduct problem (rs2906457, OR = 2.08, P = 0.046; rs2906457, OR = 2.71, P = 0.044); one SNP (rs9677504) was an independent protective factor for psychosomatic disorders (OR = 0.27, P = 0.002). The results are shown in Table 2.
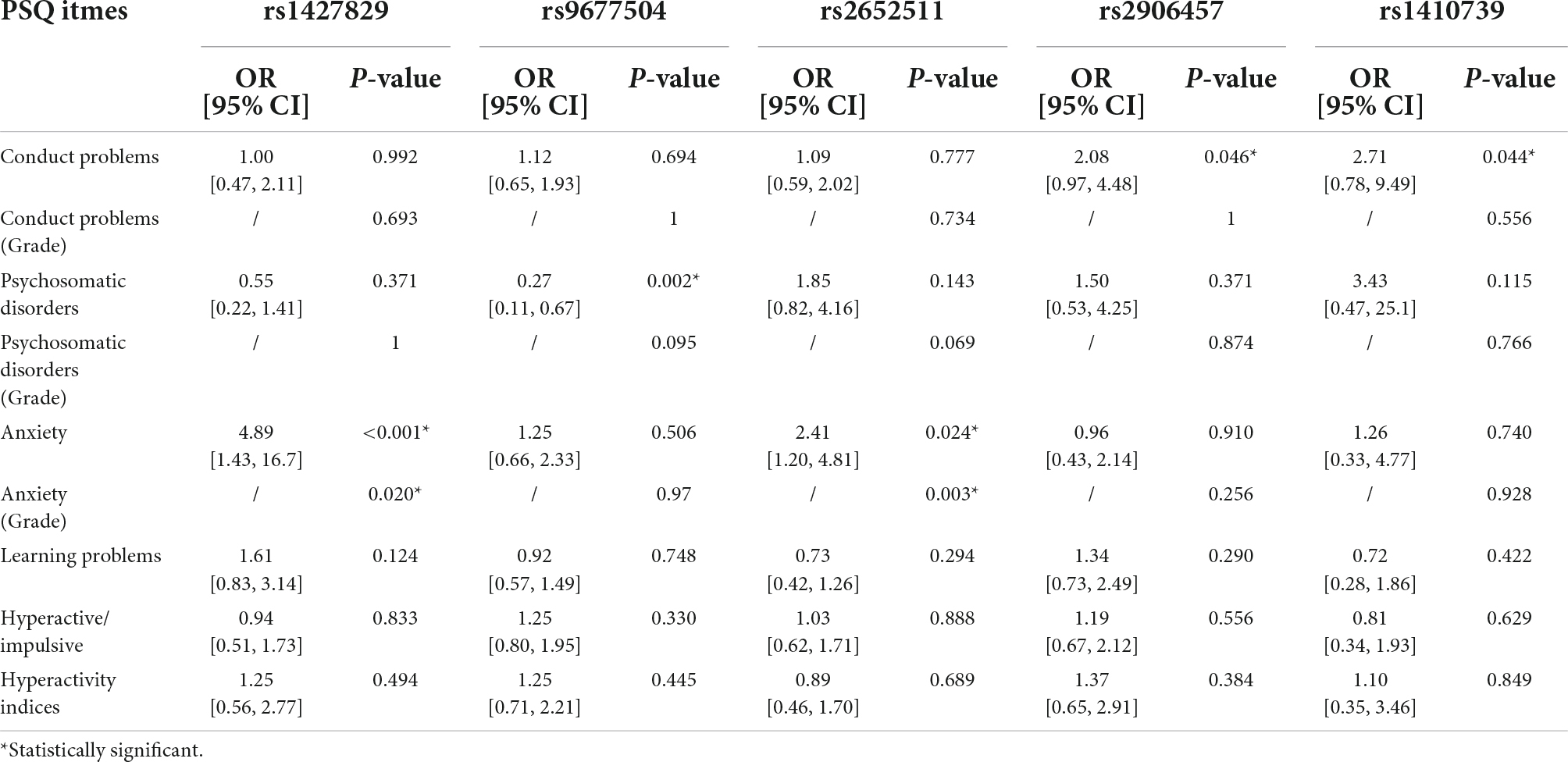
Table 2. Multivariate analysis results of the influence of single nucleotide polymorphisms (SNPs) on various clinical phenotypes of ADHD.
Multivariate analysis results of the influence of single nucleotide polymorphisms on control capabilities of children with attention deficit hyperactivity disorder
Combined with the results of the Stroop color-word test and multivariate analysis, as shown in Supplementary Table 4, one SNP (rs1410739) was found to be an independent risk factor affecting the control ability of ADHD children, which was embodied in the correct number of test items (OR = 1.03, P = 0.019). The SNP had no effect on the reaction time of test items (OR = 1.00, P = 0.040).
Multivariate analysis results of the influence of single nucleotide polymorphisms on the ability for abstract thinking of children with attention deficit hyperactivity disorder
According to the test results of WCST, 6 SNPs had an impact on the abstract thinking ability of children with ADHD. The results of multivariate analysis showed that subjects with rs9677504 (OR = 0.95, P = 0.035) or rs3768046 (OR = 0.96, P = 0.030) mutations were less likely to have an increase in the non-persistent error number, and subjects with rs1427829 mutation had an increased likelihood of persistent errors (OR = 1.05, P = 0.038). One SNP (rs1410739) was found to be associated with the correct classification results (P = 0.049). There were two SNPs, rs11210892 and rs223508, that were found to share some similar and significant results; with those two mutations, children with ADHD were more likely to have an increase in the error number and persistent error number, and less likely to have an increase in the correct number, as shown in Table 3. It can be inferred that rs9677504, rs1427829, rs1427829 and rs1427829 are independent risk factors for ADHD symptom aggravation, and rs3768046 is an independent protective factor.
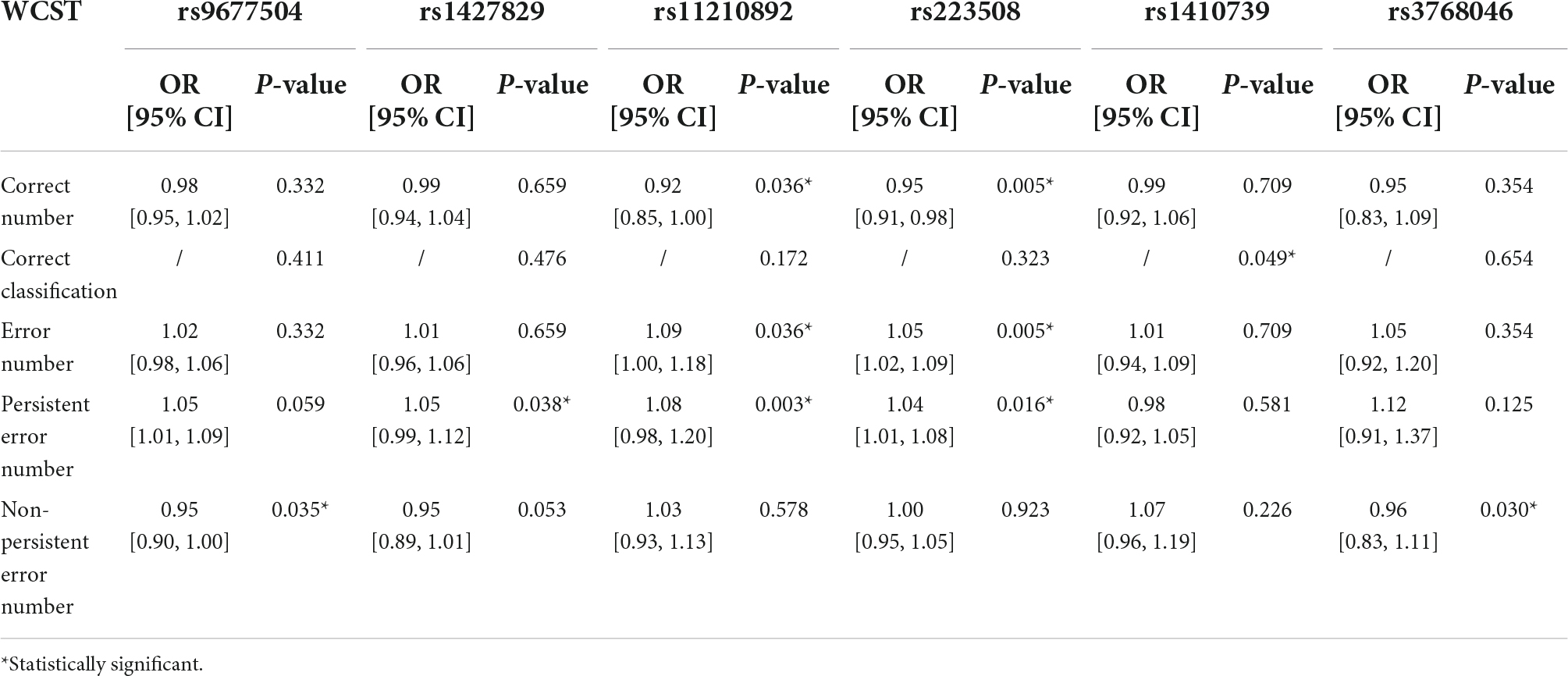
Table 3. Multivariate analysis results of the influence of SNPs on the ability for abstract thinking of children with ADHD.
Multivariate analysis results of the influence of single nucleotide polymorphisms on persistent attention deficits of children with attention deficit hyperactivity disorder
The IVA-CPT is a tool to reflect the degree of children’s response control, attention and audiovisual integration dysfunction. According to the test results, we conducted a multivariate analysis, and as shown in Supplementary Table 5, the results showed that one SNP (rs4916723) was an independent risk factor for the decrease of visual attention quotient (OR = 0.99, P = 0.036) in ADHD children.
Discussion
Previous studies have confirmed that the occurrence of ADHD is affected by multiple genes. In this research, various ADHD-related questionnaires were used to reflect the severity of the disease, and the correlation between the single nucleotide polymorphism of specific sites and the severity of clinical symptoms was then analyzed. The results presented that the SNPs at different loci have an impact on the severity of clinical symptoms of ADHD, a supplement to the field of genetic research on ADHD. This indicates a role for genetic testing in the evaluation of children with ADHD. Additionally, when the same SNP shows statistical differences in several questionnaires or several sub-items of the same questionnaire, the evaluation significance of the odds ratio of different questionnaires and items is consistent, which confirms the reliability of our study.
Based on the strong evidence that the dopaminergic neurotransmission system is involved in ADHD, the gene encoding dopamine transporter (DAT) on human chromosome 5 (SLC6A3 gene; also known as DAT1) has been proposed as a candidate gene for ADHD (25, 26). SLC6A3 may alter human DA transporter protein (hDAT) density, DA reuptake activity, and the dynamics of DA neurotransmission, participating in the pathophysiology of the central and peripheral nervous systems (40). Studies on the genetic basis of individual differences in attention suggest that SLC6A3 polymorphism is associated with executive attention (41, 42). Recent studies have also shown that the SLC6A3 genotype affects attention/cognitive function (26). Our results showed that rs2652511 (SLC6A3 locus) was an independent risk factor for the anxiety symptom in children with ADHD, which is new information on SLC6A3 gene research. It should be noted that there was no significant difference in this SNP on the questionnaire reflecting attention, which may be caused by the fact that our study was conducted on the symptoms of children with ADHD, or may be caused by probable reasons that the SLC6A3 gene maybe works in the form of multi-gene combination and it requires further study in the future.
Among the 9 loci with significantly different results in multivariate analysis, rs1410739 (OBI1-AS1 locus) affected three items from the questionnaire, including PSQ, STROOP, and WCST, respectively, corresponding to conduct problems, control ability and abstract thinking ability of children with ADHD, and all were independent risk factors. This may indicate a meaningful SNP locus that can simultaneously affect multiple ADHD symptoms was identified. Further it would provide a basis for future studies on ADHD and SNP. The SNP rs1410739 (locus in OBI-AS1, which is also regarded as RNF219-AS1) was found to be significantly associated with ADHD in the recent TWAS based on ADHD GWAS (21), however, the mechanism has not been effectively explained, so it is reasonable to discuss results at the level of the OBI1-AS1 gene. Although the exact function of OBI1-AS1 remains unclear, several studies have suggested a regulatory role for the hybridization of natural antisense RNAs with endogenous mRNAs (43). Recent studies have reported that RNF219-AS1 was involved in the pathophysiology of ADHD (44), and that OBI1-AS1 has a potential role in glutamate receptor signaling and synaptic responses (45). Previous studies have linked genetic variations in the ion glutamate receptor to ADHD risk (46). Several human genetic studies and animal studies also reported that genetic variations in the metabotropic glutamate receptor (mGluRs) subtype III may be associated with ADHD (47–54). OBI1-AS1 expression has also been reported to be associated with astrocyte content, and some studies in recent years have attributed the pathophysiology of depression and mood disorders to astrocytes (45, 55–57). These can aid in the interpretation of our results to some extent, but our findings must be viewed with caution and be seen as preliminary. This research will need to be supported by further research.
In our study, rs3768046 (TIE1 locus) was found to influence the abstract thinking ability of children with ADHD. The latest study reported that rs3768046 may change the expression of TIE1 by affecting the binding sites of transcription factors, and the expression level of TIE1 in the blood samples of patients was significantly higher than that of the control group, suggesting that TIE1 is a susceptibility gene for ADHD. The study also indicated that the G allele of ra3768046 was associated with increased susceptibility to ADHD in Chinese Han children (58). Previous studies have indicated that TIE1 plays a key role in normal vascular development and function by forming a heteromeric complex with another TIE receptor, TIE2 (59–62). The overexpression of TIE1 may lead to the destruction of the TIE2 signaling system, thus affecting the normal development and function of the vascular system, leading to neuroinflammation and the destruction of the blood–brain barrier, etc., and then damaging ADHD-related organs and systems. Abnormal perfusion may be one of the pathological bases of TIE1’s effect on ADHD (58, 63, 64).
Another SNP, rs223508 (MANBA locus), was found to be an independent risk factor to influence VIQ, IQ and abstract thinking ability via multivariate analysis. The protein β-mannosidase encoded by MANBA is a member of the glycosylhydrolase 2 family and acts as the final exon of β-glycosidase in the N-linked glycoprotein oligosaccharide catabolism pathway (65). MANBA is mainly confined to the midbrain and hindbrain, including the cerebellar cortex, medulla and pons. The expression level of MANBA is very low at all stages of human brain development (66), and its activity decreases with age (67). Up to date, there are few studies on MANBA and ADHD, and the mechanism is not clear. Recent studies have found that the gene-regulated expression of MANBA in the cerebellum is significantly associated with the risk of ADHD (68), and the expression of MANBA is significantly up-regulated in patients (58). In contrast, other studies have shown that increased levels of β-mannosidase are associated with a reduced risk of ADHD (69). More research is needed to resolve these controversies.
We also found that rs2906457 (ST3GAL3 locus) had an effect on the severity of conduct problems in children with ADHD, and that it was its independent risk factor. ST3GAL3 is expressed in a variety of tissues, including neurons, and encodes a membrane protein (ST3Gal III) that adds sialic acid to the end of glycolipids or glycoproteins, a process that has an important impact on brain function, and St3gal3 single gene deletion mice have reduced motor coordination, cognitive impairment and behavioral hyperactivity (70, 71). Haploid deficiency of ST3GAL3 leads to sex-dependent changes in markers of cognitive, behavioral, and brain plasticity (72). Since the human brain is particularly rich in sialic acid containing glycolipids (gangliosides), ST3GAL3 may also play a role in human brain development (73). It has been indicated that gangliosides regulate calcium homeostasis and signal transduction in neurons (74). Recent human gene analysis has indicated that increased transcription of ST3GAL3 is significantly associated with ADHD, and common gene variants in ST3GAL3 are also associated with education level (75–77). Our results further confirmed the functional correlation between ST3GAL3 and ADHD.
The SNP rs4916723 (LINC00461 locus) was found to affect response control, attention, and audiovisual integration. This SNP is located in the gene LINC00461, which is mainly expressed in the brain and plays a key role in the regulation of brain function (78–80). LINC00461 is one of the most pleiotropic genome-wide risk genes for major psychiatric traits, and it has been found to be associated with five psychiatric disorders at the same time, including ADHD, depression, anxiety disorder, schizophrenia and the personality trait of neuroticism (81–83). The latest study also reports LINC00461 as a novel risk gene for ASD and confirms that the LINC00462-MEF2C gene cluster is one of the most potent genomic contributors to major psychotic traits (84). Moreover, a recent GWAS study said that LINC00461 correlates with education and is associated with reduced brain size, cortical morphology abnormalities, and hippocampal mossy fiber morphology abnormalities (76). Knockout of LINC00461 lineal homolog expression in mouse embryos showed impaired neuronal migration, further supporting its role in the neurodevelopmental hypothesis of most major psychiatric disorders (81). Previous studies also indicated that some lncRNAs in the cytoplasm may bind to miRNAs to inhibit their activity, thereby regulating the expression of target genes (85). It is worth mentioning that MIR9-2, which encodes neuron-specific miRNA miR-9, is located within the loci LINC00461. Knockdown of LINC00461 also greatly inhibits the expression level of Mir-9 (78, 86), while overexpression of miR-9 has been shown to be sufficient to ameliorate neural migration defects (87).
What’s more, we found that rs1427829 (intron) could influence the severity of anxiety symptoms and abstract thinking ability in children with ADHD, also, rs11210892 (intron) had an effect on abstract thinking ability in children with ADHD. They have previously been found to be significantly associated with the development of ADHD in multiple replication mete-analyses (7, 21). Besides, we found that rs9677504 (SPAG16 locus) affects the severity of psychosomatic disorders and abstract thinking ability. Previous studies have shown that SPAG16 is associated with osteoporosis (88), primary ciliary motility disorder (PCD) (89), multiple sclerosis (90, 91), and reduced male fertility due to arsenism and fluorosis (92, 93). However, the mechanism of these three SNPs, with respect to their impact on the onset and severity of ADHD symptoms, has not been explained yet.
In addition, the use of “with or without” grading of PSQ projects according to the literature was verified to a certain extent. Table 2 shows that “Anxiety (Grade)” and “Anxiety,” which have significant results in children with ADHD, are always consistent. When accounting for possible genetic differences between populations, our study is also a validation of these SNPs in a sample of Chinese children.
There are several limitations of our work that should be considered. First, our sample size is relatively small, so it needs to be expanded in the future in order to provide more reliable results. Second, our susceptibility SNPs were screened according to the results of previous research including GWAS, TWAS and et al., which may cause that not all ADHD-related SNPs were included in our study. Third, we focused only on children with ADHD and did not perform a comparative analysis with healthy controls. Fourth, although our research has achieved certain results, the underlying mechanism of SNPs is still unclear, which requires further cell and animal experiments to explore and verify. In the future, we plan to conduct further studies on the clinical phenotypes and neural mechanisms of ADHD in combination with more clinical data from these children.
Data availability statement
The original contributions presented in this study are publicly available. This data can be found here: NCBI: PRJNA873257.
Ethics statement
The studies involving human participants were reviewed and approved by the Ethics Committee of Clinical Research in The First Affiliated Hospital of Wenzhou Medical University. Written informed consent to participate in this study was provided by the participants’ legal guardian/next of kin.
Author contributions
YX and MW: conceptualization. YX, JT, AQ, XL, RZ, SC, CY, and BC: acquisition of the data for the work. YX and SL: analysis of data for the work. YX, SL, and AQ: interpretation of data for the work. YX, SL, and PV: manuscript writing. MW and AQ: funding acquisition. All authors contributed to the article and approved the submitted version.
Funding
This work was supported by the Key Laboratory of Intelligent Medical Imaging of Wenzhou (No. 2021HZSY0057), Key Laboratory of Alzheimer’s Disease of Zhejiang Province, Institute of Aging, Wenzhou Medical University, Wenzhou, Zhejiang, China, and the Wenzhou Municipal Science and Technology Bureau (No. Y20210231).
Conflict of interest
The authors declare that the research was conducted in the absence of any commercial or financial relationships that could be construed as a potential conflict of interest.
Publisher’s note
All claims expressed in this article are solely those of the authors and do not necessarily represent those of their affiliated organizations, or those of the publisher, the editors and the reviewers. Any product that may be evaluated in this article, or claim that may be made by its manufacturer, is not guaranteed or endorsed by the publisher.
Supplementary material
The Supplementary Material for this article can be found online at: https://www.frontiersin.org/articles/10.3389/fpsyt.2022.1003542/full#supplementary-material
Footnotes
References
1. Sayal K, Prasad V, Daley D, Ford T, Coghill D. ADHD in children and young people: prevalence, care pathways, and service provision. Lancet Psychiatry. (2018) 5:175–86. doi: 10.1016/S2215-0366(17)30167-0
2. Fontana BD, Franscescon F, Rosemberg DB, Norton WHJ, Kalueff AV, Parker MO. Zebrafish models for attention deficit hyperactivity disorder (ADHD). Neurosci Biobehav Rev. (2019) 100:9–18. doi: 10.1016/j.neubiorev.2019.02.009
3. Abramovitch A, Dar R, Mittelman A, Wilhelm S. Comorbidity between attention deficit/hyperactivity disorder and obsessive-compulsive disorder across the lifespan: a systematic and critical review. Harv Rev Psychiatry. (2015) 23:245–62. doi: 10.1097/HRP.0000000000000050
4. DuPaul GJ, Jitendra AK, Volpe RJ, Tresco KE, Lutz JG, Vile Junod RE, et al. Consultation-based academic interventions for children with ADHD: effects on reading and mathematics achievement. J Abnorm Child Psychol. (2006) 34:635–48. doi: 10.1007/s10802-006-9046-7
5. Wehmeier PM, Schacht A, Barkley RA. Social and emotional impairment in children and adolescents with ADHD and the impact on quality of life. J Adolesc Health. (2010) 46:209–17. doi: 10.1016/j.jadohealth.2009.09.009
6. Spencer TJ, Biederman J, Mick E. Attention-deficit/hyperactivity disorder: diagnosis, lifespan, comorbidities, and neurobiology. J Pediatr Psychol. (2007) 32:631–42. doi: 10.1093/jpepsy/jsm005
7. Demontis D, Walters RK, Martin J, Mattheisen M, Als TD, Agerbo E, et al. Discovery of the first genome-wide significant risk loci for attention deficit/hyperactivity disorder. Nat Genet. (2019) 51:63–75. doi: 10.1038/s41588-018-0269-7
8. Faraone SV, Larsson H. Genetics of attention deficit hyperactivity disorder. Mol Psychiatry. (2019) 24:562–75. doi: 10.1038/s41380-018-0070-0
9. Christiansen H, Chen W, Oades RD, Asherson P, Taylor EA, Lasky-Su J, et al. Co-transmission of conduct problems with attention-deficit/hyperactivity disorder: familial evidence for a distinct disorder. J Neural Transm. (2008) 115:163–75. doi: 10.1007/s00702-007-0837-y
10. Kuntsi J, Pinto R, Price TS, van der Meere JJ, Frazier-Wood AC, Asherson P. The aration of ADHD inattention and hyperactivity-impulsivity symptoms: pathways from genetic effects to cognitive impairments and symptoms. J Abnorm Child Psychol. (2014) 42:127–36. doi: 10.1007/s10802-013-9771-7
11. Rommelse NN, Franke B, Geurts HM, Hartman CA, Buitelaar JK. Shared heritability of attention-deficit/hyperactivity disorder and autism spectrum disorder. Eur Child Adolesc Psychiatry. (2010) 19:281–95. doi: 10.1007/s00787-010-0092-x
12. Ghirardi L, Brikell I, Kuja-Halkola R, Freitag CM, Franke B, Asherson P, et al. The familial co-aggregation of ASD and ADHD: a register-based cohort study. Mol Psychiatry. (2018) 23:257–62. doi: 10.1038/mp.2017.17
13. Larsson H, Rydén E, Boman M, Långström N, Lichtenstein P, Landén M. Risk of bipolar disorder and schizophrenia in relatives of people with attention-deficit hyperactivity disorder. Br J Psychiatry. (2013) 203:103–6. doi: 10.1192/bjp.bp.112.120808
14. Faraone SV, Biederman J, Wozniak J. Examining the comorbidity between attention deficit hyperactivity disorder and bipolar I disorder: a meta-analysis of family genetic studies. Am J Psychiatry. (2012) 169:1256–66. doi: 10.1176/appi.ajp.2012.12010087
15. Faraone SV, Biederman J. Do attention deficit hyperactivity disorder and major depression share familial risk factors? J Nerv Ment Dis. (1997) 185:533–41. doi: 10.1097/00005053-199709000-00001
16. Rao S, Baranova A, Yao Y, Wang J, Zhang F. Genetic relationships between attention-deficit/hyperactivity disorder, autism spectrum disorder, and intelligence. Neuropsychobiology. (2022) 28:1–13. doi: 10.1159/000525411
17. Lo HS, Wang Z, Hu Y, Yang HH, Gere S, Buetow KH, et al. Allelic variation in gene expression is common in the human genome. Genome Res. (2003) 13:1855–62. doi: 10.1101/gr.1006603
18. Martin J, O’Donovan MC, Thapar A, Langley K, Williams N. The relative contribution of common and rare genetic variants to ADHD. Transl Psychiatry. (2015) 5:e506. doi: 10.1038/tp.2015.5
19. Alexander DH, Novembre J, Lange K. Fast model-based estimation of ancestry in unrelated individuals. Genome Res. (2009) 19:1655–64. doi: 10.1101/gr.094052.109
20. Shi R, Zhang Y, Wang YC, Huang T, Lu GC, Yue T, et al. The evaluation of genomic homozygosity for Xinjiang inbred population by SNP panels. Yi Chuan. (2020) 42:493–505. doi: 10.16288/j.yczz.20-071
21. Liao C, Laporte AD, Spiegelman D, Akçimen F, Joober R, Dion PA, et al. Transcriptome-wide association study of attention deficit hyperactivity disorder identifies associated genes and phenotypes. Nat Commun. (2019) 10:4450. doi: 10.1038/s41467-019-12450-9
22. Doyle C, Brookes K, Simpson J, Park J, Scott S, Coghill DR, et al. Replication of an association of a promoter polymorphism of the dopamine transporter gene and attention deficit hyperactivity disorder. Neurosci Lett. (2009) 462:179–81. doi: 10.1016/j.neulet.2009.06.084
23. Shang CY, Gau SS, Liu CM, Hwu HG. Association between the dopamine transporter gene and the inattentive subtype of attention deficit hyperactivity disorder in Taiwan. Prog Neuropsychopharmacol Biol Psychiatry. (2011) 35:421–8. doi: 10.1016/j.pnpbp.2010.08.016
24. Brookes KJ, Xu X, Anney R, Franke B, Zhou K, Chen W, et al. Association of ADHD with genetic variants in the 5’-region of the dopamine transporter gene: evidence for allelic heterogeneity. Am J Med Genet B Neuropsychiatr Genet. (2008) 147B:1519–23. doi: 10.1002/ajmg.b.30782
25. Faraone SV, Perlis RH, Doyle AE, Smoller JW, Goralnick JJ, Holmgren MA, et al. Molecular genetics of attention-deficit/hyperactivity disorder. Biol Psychiatry. (2005) 57:1313–23. doi: 10.1016/j.biopsych.2004.11.024
26. Kuc K, Bielecki M, Racicka-Pawlukiewicz E, Czerwinski MB, Cybulska-Klosowicz A. The SLC6A3 gene polymorphism is related to the development of attentional functions but not to ADHD. Sci Rep. (2020) 10:6176. doi: 10.1038/s41598-020-63296-x
27. Gong YX, Cai TS. The Wechsler intelligence scale for children in China (C-WISC). Chin J Clin Psychol. (1994) 2:1–6. doi: 10.16128/j.cnki.1005-3611.1994.01.001
28. Su LY, Li XR, Huang CX, Luo XR, Zhang JS. Norms of the Conners parent symptom questionnaire in Chinese urban children. Chin J Clin Psychol. (2001) 9:241–52. doi: 10.16128/j.cnki.1005-3611.2001.04.001
29. Goyette CH, Conners CK, Ulrich RF. Normative data on revised Conners parent and teacher rating scales. J Abnorm Child Psychol. (1978) 6:221–36. doi: 10.1007/BF00919127
30. Stroop JR. Studies of interference in serial verbal reactions. J Exp Psychol Gen. (1992) 121:15–23. doi: 10.1037/0096-3445.121.1.15
31. Yu HT, Zhou FC, Bo QJ, Chi Y, Wang CY. Analysis of the factors related to cognitive function in bipolar disorder depression. Chin J Med. (2020) 55:917–9. doi: 10.3969/j.issn.1008-1070.2020.08.031
32. Dahdah MN, Bennett M, Prajapati P, Parsons TD, Sullivan E, Driver S. Application of virtual environments in a multi-disciplinary day neurorehabilitation program to improve executive functioning using the Stroop task. NeuroRehabilitation. (2017) 41:721–34. doi: 10.3233/NRE-172183
33. Golden CJ. A Manual for the Clinical and Experimental use of the Stroop Color And Word Test. Wood Dale, IL: Stoelting Company (1978).
34. Zhu Y, Liu L, Yang D, Ji H, Huang T, Xue L, et al. Cognitive control and emotional response in attention-deficit/hyperactivity disorder comorbidity with disruptive, impulse-control, and conduct disorders. BMC Psychiatry. (2021) 21:232. doi: 10.1186/s12888-021-03221-2
35. Alloway TP, Gathercole SE, Willis C, Adams AM. A structural analysis of working memory and related cognitive skills in young children. J Exp Child Psychol. (2004) 87:85–106. doi: 10.1016/j.jecp.2003.10.002
36. Zhu XZ, Wang XY, Huang Y, Yao SQ, Tang HB. A comparative study of wisconsin card sorting test in individuals with different degrees of alexithymia. Chin J Clin Psychol. (2006) 14:131–2. doi: 10.16128/j.cnki.1005-3611.2006.02.008
37. Lu JP, Yang ZW, Shu MY, Liu HL, Wang JT, Fan WD. Comparative study on integrated continuous performance test in ADHD children. Chin J Clin Psychol. (2004) 12:264–5. doi: 10.16128/j.cnki.1005-3611.2004.03.015
38. Jiang L, Su Y, Zhang W, Liu X, Zhang HS, Mu Y. Case-control study of attention deficit hyperactivity disorder diagnosis with integrated visual and auditory continuous performance test and DSM-IV. Chin. J. Child Health Care. (2004) 5:388–90. doi: 10.3969/j.issn.1008-6579.2004.05.007
39. Tian GQ, Gan JG. Continuous performance test detecting of continuous attention function. Chin J Clin Neurosci. (2009) 17:653–6. doi: 10.3969/j.issn.1008-0678.2009.06.019
40. Reith MEA, Kortagere S, Wiers CE, Sun H, Kurian MA, Galli A, et al. The dopamine transporter gene SLC6A3: multidisease risks. Mol Psychiatry. (2022) 27:1031–46.
41. Posner MI, Rothbart MK, Sheese BE. Attention genes. Dev Sci. (2007) 10:24–9. doi: 10.1111/j.1467-7687.2007.00559.x
42. Fossella J, Sommer T, Fan J, Wu Y, Swanson JM, Pfaff DW, et al. Assessing the molecular genetics of attention networks. BMC Neurosci. (2002) 3:14. doi: 10.1186/1471-2202-3-14
43. Káňová E, Tkáčová Z, Bhide K, Kulkarni A, Jiménez-Munguía I, Mertinková P, et al. Transcriptome analysis of human brain microvascular endothelial cells response to Neisseria meningitidis and its antigen MafA using RNA-seq. Sci Rep. (2019) 9:18763. doi: 10.1038/s41598-019-55409-y
44. Fu GH, Chen W, Li HM, Wang YF, Liu L, Qian QJ. A potential association of RNF219-AS1 with ADHD: evidence from categorical analysis of clinical phenotypes and from quantitative exploration of executive function and white matter microstructure endophenotypes. CNS Neurosci Ther. (2021) 27:603–16. doi: 10.1111/cns.13629
45. Mamivand A, Bayat S, Maghrouni A, Shabani S, Khoshnevisan A, Saffar H, et al. Data mining of bulk and single-cell RNA sequencing introduces OBI1-AS1 as an astrocyte ker with possible role in glioma recurrence and progression. Clin Epigenetics. (2022) 14:35. doi: 10.1186/s13148-022-01260-4
46. Zhang Q, Huang X, Chen XZ, Li SY, Yao T, Wu J. Association of gene variations in ionotropic glutamate receptor and attention-deficit/hyperactivity disorder in the Chinese population: a two-stage case-control study. J Atten Disord. (2021) 25:1362–73. doi: 10.1177/1087054720905089
47. Zhang Q, Chen X, Li S, Yao T, Wu J. Association between the group III metabotropic glutamate receptor gene polymorphisms and attention-deficit/hyperactivity disorder and functional exploration of risk loci. J Psychiatr Res. (2021) 132:65–71. doi: 10.1016/j.jpsychires.2020.09.035
48. Akutagava-Martins GC, Salatino-Oliveira A, Genro JP, Contini V, Polanczyk G, Zeni C, et al. Glutamatergic copy number variants and their role in attention-deficit/hyperactivity disorder. Am J Med Genet B Neuropsychiatr Genet. (2014) 165B:502–9. doi: 10.1002/ajmg.b.32253
49. Davis MJ, Iancu OD, Acher FC, Stewart BM, Eiwaz MA, Duvoisin RM, et al. Role of mGluR4 in acquisition of fear learning and memory. Neuropharmacology. (2013) 66:365–72. doi: 10.1016/j.neuropharm.2012.07.038
50. Elia J, Glessner JT, Wang K, Takahashi N, Shtir CJ, Hadley D, et al. Genome-wide copy number variation study associates metabotropic glutamate receptor gene networks with attention deficit hyperactivity disorder. Nat Genet. (2011) 44:78–84. doi: 10.1038/ng.1013
51. Gerlai R, Adams B, Fitch T, Chaney S, Baez M. Performance deficits of mGluR8 knockout mice in learning tasks: the effects of null mutation and the background genotype. Neuropharmacology. (2002) 43:235–49. doi: 10.1016/s0028-3908(02)00078-3
52. Gerlai R, Roder JC, Hampson DR. Altered spatial learning and memory in mice lacking the mGluR4 subtype of metabotropic glutamate receptor. Behav Neurosci. (1998) 112:525–32. doi: 10.1037//0735-7044.112.3.525
53. Hölscher C, Schmid S, Pilz PK, Sansig G, van der Putten H, Plappert CF. Lack of the metabotropic glutamate receptor subtype 7 selectively modulates Theta rhythm and working memory. Learn Mem. (2005) 12:450–5. doi: 10.1101/lm.98305
54. Noroozi R, Taheri M, Omrani MD, Ghafouri-Fard S. Glutamate receptor metabotropic 7 (GRM7) gene polymorphisms in mood disorders and attention deficit hyperactive disorder. Neurochem Int. (2019) 129:104483. doi: 10.1016/j.neuint.2019.104483
55. Zhou X, Xiao Q, Xie L, Yang F, Wang L, Tu J. Astrocyte, a promising target for mood disorder interventions. Front Mol Neurosci. (2019) 12:136. doi: 10.3389/fnmol.2019.00136
56. Cavaccini A, Durkee C, Kofuji P, Tonini R, Araque A. Astrocyte signaling gates long-term depression at corticostriatal synapses of the direct pathway. J Neurosci. (2020) 40:5757–68. doi: 10.1523/JNEUROSCI.2369-19.2020
57. Navarrete M, Cuartero MI, Palenzuela R, Draffin JE, Konomi A, Serra I, et al. Astrocytic p38α MAPK drives NMDA receptor-dependent long-term depression and modulates long-term memory. Nat Commun. (2019) 10:2968. doi: 10.1038/s41467-019-10830-9
58. Chen X, Yao T, Cai J, Zhang Q, Li S, Li H, et al. A el cis-regulatory variant modulating TIE1 expression associated with attention deficit hyperactivity disorder in Han Chinese children. J Affect Disord. (2022) 300:179–88. doi: 10.1016/j.jad.2021.12.066
59. Augustin HG, Koh GY, Thurston G, Alitalo K. Control of vascular morphogenesis and homeostasis through the angiopoietin-Tie system. Nat Rev Mol Cell Biol. (2009) 10:165–77. doi: 10.1038/nrm2639
60. Saharinen P, Kerkelä K, Ekman N, Marron M, Brindle N, Lee GM, et al. Multiple angiopoietin recombinant proteins activate the Tie1 receptor tyrosine kinase and promote its interaction with Tie2. J Cell Biol. (2005) 169:239–43. doi: 10.1083/jcb.200411105
61. Savant S, La Porta S, Budnik A, Busch K, Hu J, Tisch N, et al. The orphan receptor tie1 controls angiogenesis and vascular remodeling by differentially regulating Tie2 in tip and stalk cells. Cell Rep. (2015) 12:1761–73. doi: 10.1016/j.celrep.2015.08.024
62. Seegar TC, Eller B, Tzvetkova-Robev D, Kolev MV, Henderson SC, Nikolov DB, et al. Tie1-Tie2 interactions mediate functional differences between angiopoietin ligands. Mol Cell. (2010) 37:643–55. doi: 10.1016/j.molcel.2010.02.007
63. Spalletta G, Pasini A, Pau F, Guido G, Menghini L, Caltagirone C. Prefrontal blood flow dysregulation in drug naive ADHD children without structural abnormalities. J Neural Transm. (2001) 108:1203–16. doi: 10.1007/s007020170010
64. Tan YW, Liu L, Wang YF, Li HM, Pan MR, Zhao MJ, et al. Alterations of cerebral perfusion and functional brain connectivity in medication-naïve male adults with attention-deficit/hyperactivity disorder. CNS Neurosci Ther. (2020) 26:197–206. doi: 10.1111/cns.13185
65. Gytz H, Liang J, Liang Y, Gorelik A, Illes K, Nagar B. The structure of mammalian β-mannosidase provides insight into β-mannosidosis and nystagmus. FEBS J. (2019) 286:1319–31.
66. Yu P, Cui Y, Cai W, Wu H, Xiao X, Shao Q, et al. Lysosomal storage disease in the brain: mutations of the β-mannosidase gene identified in autosomal dominant nystagmus. Genet Med. (2015) 17:971–9. doi: 10.1038/gim.2015.10
67. Cooper A, Hatton C, Sardharwalla IB. Acid beta-mannosidase of human plasma: influence of age and sex on enzyme activity. J Inherit Metab Dis. (1987) 10:229–33. doi: 10.1007/BF01800067
68. Fernàndez-Castillo N, Cabana-Domínguez J, Kappel DB, Torrico B, Weber H, Lesch KP, et al. Exploring the contribution to ADHD of genes involved in mendelian disorders presenting with hyperactivity and/or inattention. Genes. (2021) 13:93. doi: 10.3390/genes13010093
69. Yang J, He X, Qian L, Zhao B, Fan Y, Gao F, et al. Association between plasma proteome and childhood neurodevelopmental disorders: a two-sample Mendelian randomization analysis. EBioMedicine. (2022) 78:103948. doi: 10.1016/j.ebiom.2022.103948
70. Yoo SW, Motari MG, Susuki K, Prendergast J, Mountney A, Hurtado A, et al. Sialylation regulates brain structure and function. FASEB J. (2015) 29:3040–53. doi: 10.1096/fj.15-270983
71. Schnaar RL, Gerardy-Schahn R, Hildebrandt H. Sialic acids in the brain: gangliosides and polysialic acid in nervous system development, stability, disease, and regeneration. Physiol Rev. (2014) 94:461–518. doi: 10.1152/physrev.00033.2013
72. Rivero O, Alhama-Riba J, Ku HP, Fischer M, Ortega G, Álmos P, et al. Haploinsufficiency of the attention-deficit/hyperactivity disorder risk gene St3gal3 in mice causes alterations in cognition and expression of genes involved in myelination and sialylation. Front Genet. (2021) 12:688488. doi: 10.3389/fgene.2021.688488
73. Wang B. Molecular mechanism underlying sialic acid as an essential nutrient for brain development and cognition. Adv Nutr. (2012) 3:465S–72S. doi: 10.3945/an.112.001875
74. Wu G, Xie X, Lu ZH, Ledeen RW. Cerebellar neurons lacking complex gangliosides degenerate in the presence of depolarizing levels of potassium. Proc Natl Acad Sci U.S.A. (2001) 98:307–12. doi: 10.1073/pnas.98.1.307
75. Hall LS, Pain O, O’Brien HE, Anney R, Walters JTR, Owen MJ, et al. Cis-effects on gene expression in the human prenatal brain associated with genetic risk for neuropsychiatric disorders. Mol Psychiatry. (2021) 26:2082–8. doi: 10.1038/s41380-020-0743-3
76. Okbay A, Beauchamp JP, Fontana MA, Lee JJ, Pers TH, Rietveld CA, et al. Genome-wide association study identifies 74 loci associated with educational attainment. Nature. (2016) 533:539–42. doi: 10.1038/nature17671
77. Walton E, Pingault JB, Cecil CA, Gaunt TR, Relton CL, Mill J, et al. Epigenetic profiling of ADHD symptoms trajectories: a prospective, methylome-wide study. Mol Psychiatry. (2017) 22:250–6. doi: 10.1038/mp.2016.85
78. Smith RM, Webb A, Papp AC, Newman LC, Handelman SK, Suhy A, et al. Whole transcriptome RNA-Seq allelic expression in human brain. BMC Genomics. (2013) 14:571. doi: 10.1186/1471-2164-14-571
79. Deguchi S, Katsushima K, Hatanaka A, Shinjo K, Ohka F, Wakabayashi T, et al. Oncogenic effects of evolutionarily conserved noncoding RNA ECONEXIN on gliomagenesis. Oncogene. (2017) 36:4629–40. doi: 10.1038/onc.2017.88
80. Fagerberg L, Hallström BM, Oksvold P, Kampf C, Djureinovic D, Odeberg J, et al. Analysis of the human tissue-specific expression by genome-wide integration of transcriptomics and antibody-based proteomics. Mol Cell Proteomics. (2014) 13:397–406. doi: 10.1074/mcp.M113.035600
81. Liu S, Rao S, Xu Y, Li J, Huang H, Zhang X, et al. Identifying common genome-wide risk genes for major psychiatric traits. Hum Genet. (2020) 139:185–98. doi: 10.1007/s00439-019-02096-4
82. Wu Y, Cao H, Baranova A, Huang H, Li S, Cai L, et al. Multi-trait analysis for genome-wide association study of five psychiatric disorders. Transl Psychiatry. (2020) 10:209. doi: 10.1038/s41398-020-00902-6
83. Rao S, Tian L, Cao H, Baranova A, Zhang F. Involvement of the long intergenic non-coding RNA LINC00461 in schizophrenia. BMC Psychiatry. (2022) 22:59. doi: 10.1186/s12888-022-03718-4
84. Baranoa A, Wang J, Cao H, Chen JH, Chen J, Chen M, et al. Shared genetics between autism spectrum disorder and attention-deficit/hyperactivity disorder and their association with extraversion. Psychiatry Res. (2022) 314:114679. doi: 10.1016/j.psychres.2022.114679
85. Tay Y, Rinn J, Pandolfi PP. The multilayered complexity of ceRNA crosstalk and competition. Nature. (2014) 505:344–52. doi: 10.1038/nature12986
86. Tovo-Rodrigues L, Quinte GC, Brum CB, Ghisleni G, Bastos CR, Oliveira IO, et al. The role of MIR9-2 in shared susceptibility of psychiatric disorders during childhood: a population-based birth cohort study. Genes. (2019) 10:626. doi: 10.3390/genes10080626
87. Topol A, Zhu S, Hartley BJ, English J, Hauberg ME, Tran N, et al. Dysregulation of miRNA-9 in a subset of schizophrenia patient-derived neural progenitor cells. Cell Rep. (2016) 15:1024–36. doi: 10.1016/j.celrep.2016.03.090
88. Liu A, Liu Y, Su KJ, Greenbaum J, Bai Y, Tian Q, et al. A transcriptome-wide association study to detect el genes for volumetric bone mineral density. Bone. (2021) 153:116106. doi: 10.1016/j.bone.2021.116106
89. Stevanoic N, Skakic A, Minic P, Sovtic A, Stojiljkovic M, Pavlovic S, et al. Identification and classification of el genetic variants: en route to the diagnosis of Pri y Ciliary Dyskinesia. Int J Mol Sci. (2021) 22:8821. doi: 10.3390/ijms22168821
90. Fraussen J, de Bock L, Somers V. B cells and antibodies in progressive multiple sclerosis: contribution to neurodegeneration and progression. Autoimmun Rev. (2016) 15:896–9. doi: 10.1016/j.autrev.2016.07.008
91. de Bock L, Fraussen J, Villar LM, Álvarez-Cerme?o JC, Van Wijmeersch B, van Pesch V, et al. Anti-SPAG16 antibodies in pri y progressive multiple sclerosis are associated with an elevated progression index. Eur J Neurol. (2016) 23:722–8. doi: 10.1111/ene.12925
92. Han Y, Liang C, Yu Y, Manthari RK, Cheng C, Tan Y, et al. Chronic arsenic exposure lowered sperm motility via impairing ultra-microstructure and key proteins expressions of sperm acrosome and flagellum formation during spermiogenesis in male mice. Sci Total Environ. (2020) 734:139233. doi: 10.1016/j.scitotenv.2020.139233
Keywords: attention deficit hyperactivity disorder (ADHD), single nucleotide polymorphism (SNP), GWAS, TWAS, panel, severity of clinical symptoms
Citation: Xu Y, Lin S, Tao J, Liu X, Zhou R, Chen S, Vyas P, Yang C, Chen B, Qian A and Wang M (2022) Correlation research of susceptibility single nucleotide polymorphisms and the severity of clinical symptoms in attention deficit hyperactivity disorder. Front. Psychiatry 13:1003542. doi: 10.3389/fpsyt.2022.1003542
Received: 27 July 2022; Accepted: 01 September 2022;
Published: 23 September 2022.
Edited by:
Fuquan Zhang, Nanjing Medical University, ChinaReviewed by:
Hongbao Cao, George Mason University, United StatesJitendra Kumar Sinha, GloNeuro Academy, India
Copyright © 2022 Xu, Lin, Tao, Liu, Zhou, Chen, Vyas, Yang, Chen, Qian and Wang. This is an open-access article distributed under the terms of the Creative Commons Attribution License (CC BY). The use, distribution or reproduction in other forums is permitted, provided the original author(s) and the copyright owner(s) are credited and that the original publication in this journal is cited, in accordance with accepted academic practice. No use, distribution or reproduction is permitted which does not comply with these terms.
*Correspondence: Andan Qian, YW5kYW4xMTI3QDE2My5jb20=; Meihao Wang, d3p3bWhAd211LmVkdS5jbg==
†These authors have contributed equally to this work