- 1ENCORE Expertise Centre for Neurodevelopmental Disorders, Erasmus University Medical Center, Rotterdam, Netherlands
- 2Department of Child- and Adolescent Psychiatry and Psychology, Erasmus University Medical Center, Rotterdam, Netherlands
- 3Child Brain Center, Erasmus University Medical Center, Rotterdam, Netherlands
- 4Department of General Paediatrics, Erasmus MC, Rotterdam, Netherlands
- 5Clinical Psychology and Experimental Psychopathology Unit, Department of Psychology, Rijksuniversiteit Groningen, Groningen, Netherlands
- 6Yulius Mental Health, Dordrecht, Netherlands
- 7Jonx Autism Team Northern-Netherlands, Lentis Mental Health, Groningen, Netherlands
Objective: The etiology of autism spectrum disorder (ASD) remains unclear, due to genetic heterogeneity and heterogeneity in symptoms across individuals. This study compares ASD symptomatology between monogenetic syndromes with a high ASD prevalence, in order to reveal syndrome specific vulnerabilities and to clarify how genetic variations affect ASD symptom presentation.
Methods: We assessed ASD symptom severity in children and young adults (aged 0-28 years) with Fragile X Syndrome (FXS, n = 60), Angelman Syndrome (AS, n = 91), Neurofibromatosis Type 1 (NF1, n = 279) and Tuberous Sclerosis Complex (TSC, n = 110), using the Autism Diagnostic Observation Schedule and Social Responsiveness Scale. Assessments were part of routine clinical care at the ENCORE expertise center in Rotterdam, the Netherlands. First, we compared the syndrome groups on the ASD classification prevalence and ASD severity scores. Then, we compared individuals in our syndrome groups with an ASD classification to a non-syndromic ASD group (nsASD, n = 335), on both ASD severity scores and ASD symptom profiles. Severity scores were compared using MANCOVAs with IQ and gender as covariates.
Results: Overall, ASD severity scores were highest for the FXS group and lowest for the NF1 group. Compared to nsASD, individuals with an ASD classification in our syndrome groups showed less problems on the instruments' social domains. We found a relative strength in the AS group on the social cognition, communication and motivation domains and a relative challenge in creativity; a relative strength of the NF1 group on the restricted interests and repetitive behavior scale; and a relative challenge in the FXS and TSC groups on the restricted interests and repetitive behavior domain.
Conclusion: The syndrome-specific strengths and challenges we found provide a frame of reference to evaluate an individual's symptoms relative to the larger syndromic population and to guide treatment decisions. Our findings support the need for personalized care and a dimensional, symptom-based diagnostic approach, in contrast to a dichotomous ASD diagnosis used as a prerequisite for access to healthcare services. Similarities in ASD symptom profiles between AS and FXS, and between NF1 and TSC may reflect similarities in their neurobiology. Deep phenotyping studies are required to link neurobiological markers to ASD symptomatology.
Introduction
Autism spectrum disorder (ASD) is a heterogeneous neurodevelopmental disorder defined by impairments in social communication, restricted or repetitive behaviors or interests, and hyper- or hyposensitivity to sensory stimuli. ASD occurs in around 1.7% of the general population (1). Despite the rapid discovery of genes related to ASD, and the high heritability estimates (64–91%), the exact etiology of ASD remains unclear (2, 3). Studying ASD symptoms in genetically homogenous groups could clarify the pathway from genes to behavior. Genetic syndromes with high ASD prevalence rates include Fragile X Syndrome (FXS), Angelman syndrome (AS), Tuberous Sclerosis Complex (TSC) and Neurofibromatosis type 1 (NF1) (4). Despite their unique genetic variation, these syndromes show similarities in their neurodevelopmental pathways. These syndromes are all affected by alterations in the mechanistic target of rapamycin (mTOR) pathway (5–10), which has also been related to non-syndromic ASD (nsASD) (10, 11). FXS and AS are similar in that both syndromes show atypical DNA methylation that results in increased levels of Activity-Regulated Cytoskeleton-associated protein (Arc), causing reduced synaptic plasticity and disruptions in cerebral development which often lead to intellectual disability (8). TSC and NF1 are similar in that they are both affected by genetic variations that inactivate tumor-suppressor genes. This inactivation leads to an over-activation of mTOR, which increases the risk of tumors in the nervous system that may affect brain development and function (7, 8). In these syndromes, a unique spectrum of ASD symptoms seems to be present (12–17). If differences or similarities in the affected pathways are also reflected by differences or similarities in ASD symptom presentation, this might help identify factors that contribute to the development of specific ASD symptoms, or the development of ASD in general. As a step toward linking ASD symptoms to specific neurobiological pathways, several studies have been conducted to describe ASD symptomatology in monogenetic neurodevelopmental disorders in detail.
FXS affects ~1 in 4,000 males and 1 in 8,000 females (18, 19), and is one of the leading inherited causes of autism and developmental delay (4). FXS is caused by an CGG repeat expansion in the Fragile X Mental Retardation 1 (FMR1) gene, which leads to reduced synaptic plasticity, complications with dendritic development and problems with neurogenesis (20–24). Due to the X-linked nature of FXS, males are generally more severely affected than females (males: 20 < IQ < 70 (25), females: 70 < IQ < 90) (26, 27). It is estimated that about 15 to 36% of people with a full Fragile X Syndrome mutation meet the clinical criteria for an assessment-based ASD classification (4, 28–30). Even without meeting all the criteria of ASD, the majority of people with FXS express behavior related to autism (30–33). Studies have shown that, compared to nsASD, individuals with FXS and ASD show less impairment on social and communication domains (32–35), more social anxiety (16), more problems with restricted and repetitive behavior, and less compulsive and ritualistic behavior (32–35).
Angelman Syndrome (AS) can be caused by different genetic variations that affect the expression of the UBE3A gene in the chromosomal region 15q11-q13 (36). AS is characterized by cognitive impairments, lack of speech, motor dysfunction, and epilepsy (37). The intellectual development of individuals with AS usually does not exceed a mental age of 24 to 30 months, regardless of their chronological age (38, 39). The prevalence of assessment-based ASD classifications in AS ranges from 20 to 80% in the literature (4, 40–42). While several studies have found that genetic variation within chromosomal region 15q11-q13 is independently associated with ASD (43), a meta-analysis revealed no such relation. Therefore, the precise effect of UBE3A variations on the development of ASD remains unclear (44). Compared to people with nsASD, people with AS and ASD display significantly less impairment in areas such as social smile, facial expressions directed to others, shared enjoyment in interaction, response to name and unusual interests or repetitive behavior (40). Compared to FXS, individuals with AS appear to be more sociable (12), and while both syndromes have altered sensory processing, their response to sensory stimuli is not similar (13).
TSC is caused by genetic variations in either the TSC1 or TSC2 gene, leading to mTOR overstimulation (45). This activation induces cellular and tissue dysplasia, causing tumorigenesis that can affect multiple organs (45, 46). Since the central nervous system is almost always afflicted, epilepsy and neuropsychiatric disorders are often seen (46, 47). While approximately half of individuals with TSC score within the normal range of cognitive ability, the other half shows mild to severe (14.5%, 25 ≤ IQ < 70) or profound (30.5%, IQ < 25) intellectual disability (48). The estimated prevalence of assessment-based ASD classifications in TSC ranges from 35 to 60% (4, 17, 47, 49, 50), of which the severity may be influenced by presence of epilepsy (49). Jeste et al. showed that impairments in social communication in children with TSC do not differ from those in children with nsASD (17). Recently, molecular target therapy with mTOR inhibitors has demonstrated a reduction of epilepsy symptoms in people with TSC (51, 52), and the reduction of autism symptoms in animal models (53, 54).
In NF1, a genetic variation in the NF1 gene causes a neurofibromin deficiency, which inhibits the cell cycle and cell differentiation, and enables unrestricted cell growth (55). Tumor growth in NF1 can cause various neurologic, cardiovascular, gastrointestinal, endocrinal and orthopedic complications (56–60). However, the most common challenge for children with NF1 are learning and behavioral problems (61–63). The average IQ of individuals with NF1 lies around 90, with 6 to 7% having an IQ lower than 70 (15, 64). The prevalence of assessment-based ASD classifications in NF1 ranges from 10 to 39% (4, 15, 49, 65). Some studies have found that NF1 has a unique ASD phenotype with better eye contact, less repetitive behavior and more severe autistic mannerisms compared to individuals with nsASD (66, 67). However, others have shown that NF1 shows a symptom profile similar to that in both nsASD and TSC (49).
In summary, FXS, TSC, AS and NF1 have a high prevalence of ASD symptoms and are caused by unique and well-described genetic variations, making them ideal candidates to study genotype-phenotype relationships in ASD. A direct comparison of these syndromes, in which ASD symptoms are assessed in the same clinical setting and with the same diagnostic instruments, has not yet been done. The main aim of this large cohort study is to identify differences and similarities in ASD symptom severity between these monogenetic developmental disorders, as well as compared to a non-syndromic ASD group. Based on earlier studies, we expect the FXS group to display the most severe symptoms, especially on the domains of stereotypic behavior and limited interests, while children with TSC and NF1 will show less severity on these domains. In contrast, we expect less severe symptoms for the FXS and AS groups in social interaction. Based on the similarities in the neurobiological pathways between FXS and AS, and between NF1 and TSC, respectively, we will explore if and how these similarities may be reflected in the ASD symptom profiles of these groups.
Besides linking genetic pathways to behavior, the ASD symptom profiles in these syndrome groups could help us understand and value the information gathered with diagnostic instruments that are developed using non-syndromic norm groups. This may add to the discussion of whether a categorical approach in ASD diagnostics is appropriate in syndromic ASD as well as non-syndromic ASD, or whether a symptom-based approach is more suitable (68). A forced fit between symptom presentation and scoring procedures may result in a loss of clinically important information or the under- or over diagnosis of ASD within these groups, which may directly impact clinical decision making and an individual's access to health services (33). Additionally, as treatment of ASD symptoms requires a personalized stepped-care approach (69), the syndrome specific symptom profiles might reveal syndrome-specific targets for treatment and intervention.
Methods
Participants
We included four groups of children and young adults (aged 0–28 years) with syndromes that have high ASD prevalence: FXS, AS, NF1, and TSC, see Figure 1. ASD symptoms and cognitive functioning were assessed as part of routine clinical care that is performed in all children seen at the ENCORE expertise center for genetic neurocognitive developmental disorders within the Erasmus MC Sophia Children's Hospital in Rotterdam, the Netherlands. All children with these syndromes were included, regardless of their ASD symptomatology.
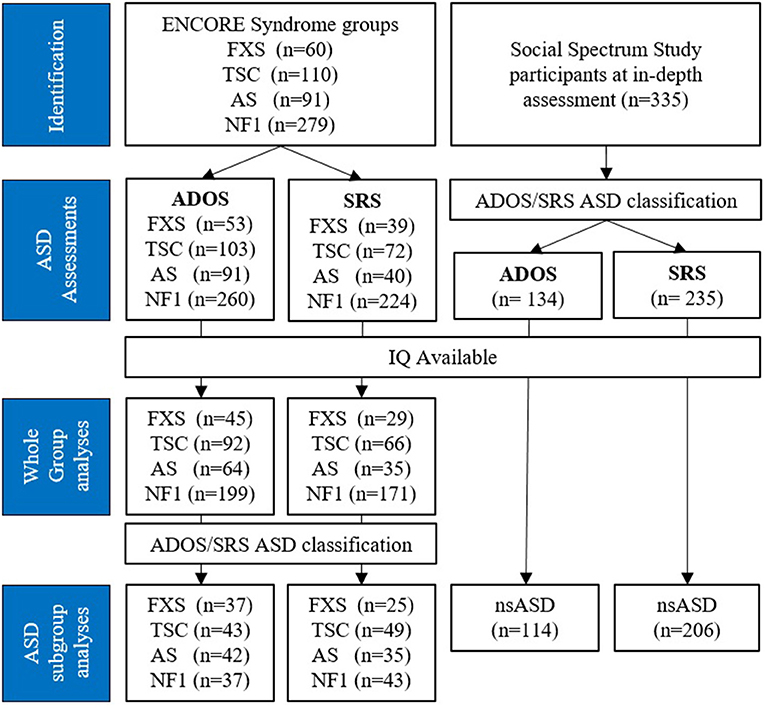
Figure 1. Flow chart of inclusion process. ASD, Autism Spectrum Disorder; FXS, Fragile X Syndrome; TSC, Tuberous Sclerosis Complex; AS, Angelman Syndrome; NF1, Neurofibromatosis type 1; nsASD, non-syndromic ASD; ADOS, Autism Diagnostic Observation Schedule; SRS, Social Responsiveness Scale.
In addition, we included an nsASD group as a frame of reference. For this group, we used data collected as part of the Social Spectrum Study, a clinical cohort study that aimed to identify children at risk for ASD by screening children who had been referred to six large child and adolescent mental health services in the South-West of the Netherlands. A detailed description of the sample characteristics and data collection procedure of this group can be found in the study design paper of the Social Spectrum Study (70). We included children that participated in the in-depth assessment (T1), which consisted of all children with an ASD classification on the Social Responsiveness Scale (SRS) (71) at initial screening (T0) (N = 235) and a random selection of children that screened negative at T0 (N = 100). From this group, we included the children with an ASD classification according to the Autism Diagnostic Observation Schedule (ADOS) (72) and/or the SRS in our subgroup analyses. None of these children were diagnosed with a genetic syndrome.
Measures
Autism Spectrum Symptoms
To assess ASD symptom severity, two diagnostic instruments were used: the ADOS and the SRS. Both instruments provide valuable insight into a child's behavior and are therefore often used together in clinical practice, which is also the case for children seen at ENCORE.
ADOS
The ADOS uses a semi-structured schedule of activities to allow researchers or clinicians to observe an individual's behavior in areas associated with a diagnosis of ASD: social communication, reciprocal social interactions and restricted and repetitive behavior. To suit an individual's developmental level and language level, one of five modules is selected. Items vary across the modules. The sensitivity of the ADOS-2 is between 72 and 97% and the specificity is between 19 and 94%, depending on the module used (73).
Data was collected by certified clinicians, using the ADOS-G (3.6%) (72) and ADOS-2 (96.4%) (74). ADOS-G scores were converted to ADOS-2 scores via the manual (74). Calibrated severity scores (CSS) are computed for the total score, and for the domains Social Affect (SA) and Restricted and Repetitive Behavior (RRB). CSS correct for chronological-age and range between 1 and 10, with higher scores indicating more severe ASD symptoms. For children younger or older than the available norm groups we calculated scores based on the nearest available norm group. Based on the Total CSS, individuals classify as “non-spectrum” (scores 1–3), “ASD” (scores 4–5) or “Autism” (scores >5) (75, 76). A Total CSS > 3 was used as a cut-off for the ASD classification used in our analyses. It is important to note however, that the RRB CSS, scored 1 to 10, are converted from the raw RRB subscale scores that range from 0 to 7. The raw scores are converted to a 1–10 scale by making scores 2, 3, and 4 impossible to obtain. While this improves the ease of interpretation relative to the other CSS scores, it is problematic for data analysis (14). Therefore, we used the original CSS-RRB scores on a 0–7 scale in our analyses.
The ADOS groups items into five subscales (language and communication, reciprocate social interaction, creativity/play, restricted and repetitive behavior, and other behavior), but does not provide normed subscale scores. For our ASD profile analyses, we computed a “Weighted Subscale Score” (WSS) for each subscale that accounts for the differences in the number of items between both the subscales and the ADOS modules. The WSS were calculated by dividing the individual's total score per subscale through the subscale's maximum score, resulting in a score between 0 and 1, with higher scores representing higher autism severity. Because Subscale E includes 3 items that measure different constructs, we did not calculate a weighted score for this subscale.
SRS
In this 65-item ASD screening questionnaire, parents or caregivers rate their child's behavior over the past 6 months on a 4-point Likert scale, with higher scores indicating higher symptom severity. Each item belongs to one of five subscales: Social Awareness, Social Cognition, Social Communication, Social Motivation, and Autistic Mannerisms. Age- and gender-normed T-scores can be computed for the total score (SRSTOT) and for the domains “Social Communication and Interaction” (SRSSCI, consisting of the first four subscales) and “Restricted Interests and Repetitive Behavior” (SRSRRB, consisting of the Autistic Mannerisms subscale). In addition, T-scores can be calculated for all subscales. Based on the T-scores, ASD symptom severity is interpreted as non-clinical (T < 60), mild (60 ≤ T ≥ 75) or severe (T > 75). An SRSTOT T-score > = 60 was used as a cut-off for the ASD classification used in our analyses. The sensitivity of the SRS-2 is 93%, with a specificity of 91% (38). Our sample included data from both the SRS and SRS-2. As items do not differ between the SRS and the SRS-2, all cases were classified using the SRS-2 classification methods, regardless of the questionnaire used. For children younger or older than the available norm groups we calculated scores based on the nearest available norm group.
Cognitive Functioning
Children with developmental delay are more likely to score in the clinical range of both the ADOS (77) and SRS (78). As developmental levels differ between the syndrome groups, we included intellectual functioning as a covariate in the group comparisons. Cognitive functioning was assessed using an age- or developmental level appropriate instrument. These instruments include the Wechsler preschool and primary scale of intelligence (WPPSI-III-NL) (79), the Wechsler intelligence scale for children [WISC-III-NL (80) or WISC-V-NL (81)], the Wechsler Adult Intelligence Scale (WAIS-III) (82), the Wechsler Non Verbal scale of Ability (WNV) (83), the Bayley Scales of Infant and Toddler Development third edition (Bayley-III) (84) and the Snijders-Oomen Non-verbal intelligence test (SON) (85). In some cases, the chronological age of the individual was higher than the available norm groups (e.g., when an 18-year-old with developmental delay was assessed using the WISC). In these cases, no full-scale IQ could be calculated and a developmental quotient (DQ) was computed instead (DQ = estimated developmental age/chronological age × 100, with M = 100, SD = 15).
Procedure
For the syndrome groups we included individuals with a complete ADOS and/or SRS assessment between May 2009 to March 2021. In case complete data from multiple time points was available for an individual, we selected the most recent time point. This resulted in 507 cases for the ADOS and 375 cases for the SRS. The nsASD group data consisted of 134 ADOS assessments and 235 SRS questionnaires, collected between May 2011 and December 2013.
Statistical Analysis
As each instrument has a unique focus, separate analyses were performed for the ADOS and the SRS data. First, we compared the syndrome groups on the prevalence of ADOS and SRS ASD classifications using Chi square tests. Next, we compared the main ASD severity scores (ADOS: CSSTOT, CSSSA, and CSSRRB; SRS: TTOT, TSCI, TRRB) between the syndrome groups using MANCOVA's with developmental level (IQ/DQ) and gender as covariates. We first did this for the syndrome groups as a whole. Then, we compared the syndrome groups again while only including individuals with an ASD classification on the ADOS or SRS, respectively, in order to include all individuals with a clinical score on that particular instrument irrespective of their classification status on the other instrument, with the nsASD group added as reference. We did this to reduce the expected within-group variability in ASD severity. Finally, for our ASD profile analysis, we also compared the ASD subgroups on subscales of the ADOS and SRS.
Data analyses were performed in IBM SPSS Statistics version 25 (86). We use an alpha level of 0.05 for all analyses and applied a Bonferroni correction for multiple comparisons in our post-hoc comparisons. In all tables the uncorrected-mean-scores, standard deviations, F-statistics, Bonferroni-corrected-p-values and the effect sizes (partial η2) are provided. Without a suitable alternative, MANCOVA's were used despite the skewed data (see Supplementary Figure 1) and correlation between the covariates and the predictor, so our results should be interpreted cautiously.
Missing Data
A non-response analysis for missing IQ/DQ scores in the syndrome groups revealed no differences in ADOS or SRS ASD severity scores between individuals with and without available IQ/DQ scores. For the nsASD group however, severity scores were higher for those without an available IQ/DQ score than for those with an IQ/DQ score for the ADOS [t(266) = −2.087, p = 0.038]. Because we expected the effect of IQ/DQ to be substantial in the syndrome groups we included IQ as a covariate nonetheless.
Results
Descriptives
Sample characteristics are presented in Table 1. We found significant differences in ASD classification prevalence between the syndrome groups for both the ADOS and the SRS. According to both instruments, approximately a quarter of individuals in the NF1 group received an ASD classification, which was lower compared to the FXS, TSC, and AS groups in which at least half of individuals received an ASD classification. The ASD classification prevalence was highest in the FXS and AS groups. An overview of ASD classifications in the syndrome groups is provided in Supplementary Figure 2.
Cross-Syndrome Comparisons of Symptom Severity Scores
ADOS
ASD severity scores of the whole-group cross-syndrome comparison are presented in Figure 2A and Table 2. We found a significant main effect of group for the ADOS CSS scores collectively and individually. Post hoc pairwise comparisons revealed that for the total CSS, autism severity was higher in the FXS group compared to all other groups, and higher in the TSC group compared to the AS group. For the social affect domain, the FXS and TSC groups had higher severity scores than the AS and NF1 groups. Lastly, the FXS group had higher severity scores on the restricted and repetitive behavior domain compared to all other groups.
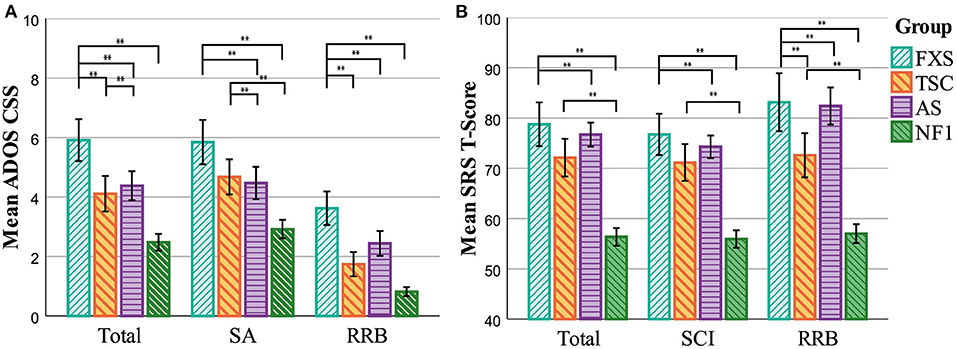
Figure 2. ASD severity scores per syndrome group. (A) Means represent the ADOS scores of the whole group, regardless of the presence of an ADOS ASD classification. (B) Means represent the SRS T-scores of the whole group, regardless of the presence of an SRS ASD classification. FXS, Fragile X Syndrome; TSC, Tuberous Sclerosis Complex; AS, Angelman Syndrome; NF1, Neurofibromatosis Type 1; CSS, Calibrated Severity Score; SA, Social Affect; RRB, Restricted (Interests) and Repetitive Behavior; SCI, Social Communication and Interaction. The bars represent the uncorrected mean scores of the groups. Significant group differences of the MANCOVA post hoc comparisons are presented. Error bars represent 95% CI, **p < 0.01, Bonferroni corrected.
SRS
The comparison of SRS severity scores also revealed a significant effect of group for the collective and individual T-scores, see Figure 2B and Table 2. Similar to the results of the ADOS, post hoc pairwise comparisons showed that the FXS group had higher severity scores than the NF1 and AS groups for all subscales, and higher severity scores compared to all other groups on the Restricted Interests and Repetitive Behavior domain. While for the ADOS the TSC group had higher ASD severity scores compared to the NF1 group specifically on the Social Affect domain, for the SRS the TSC group had higher severity scores compared to the NF1 group on all severity scores.
Cross-Syndrome Comparisons of Symptom Severity Scores in Individuals With an ASD Classification
ADOS
When including only individuals with an ASD classification on the ADOS, a group comparison of the ADOS severity scores again showed a main effect of Group for the CSS scores collectively and individually (see Figure 3A and Table 3). Post hoc pairwise comparisons showed that for both the Total and Social Affect CSS, severity scores in the individuals with an ASD classification in the FXS, TSC, and NF1 groups did not differ significantly from the severity scores of the nsASD group. Only individuals with an ADOS ASD classification in the AS group had lower severity scores compared to the nsASD group, as well as compared to all other syndrome groups. For Restricted and Repetitive Behavior, the severity scores were higher for individuals with an ADOS ASD classification in the FXS group compared to the nsASD group, as well as the AS and NF1 groups.
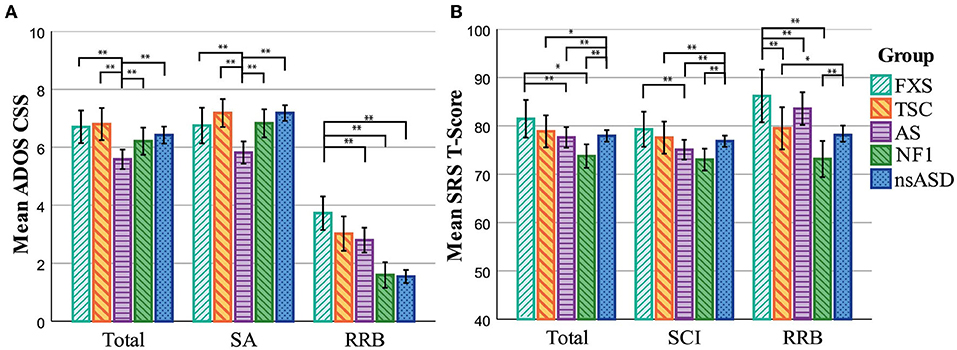
Figure 3. Cross-syndrome comparisons of symptom severity scores in individuals with an ASD classification. (A) Means represent the ADOS scores. (B) Means represent the SRS T-scores. FXS, Fragile X Syndrome; TSC, Tuberous Sclerosis Complex; AS, Angelman Syndrome; NF1, Neurofibromatosis Type 1; nsASD, non-syndromic ASD; CSS, Calibrated Severity Score; SA, Social Affect; RRB, Restricted (Interests) and Repetitive Behavior; SCI, Social Communication and Interaction. The bars represent the uncorrected mean scores of the groups. Significant group differences of the MANCOVA post hoc comparisons are presented. Error bars represent 95% CI, *p < 0.05, **p < 0.01, Bonferroni corrected.
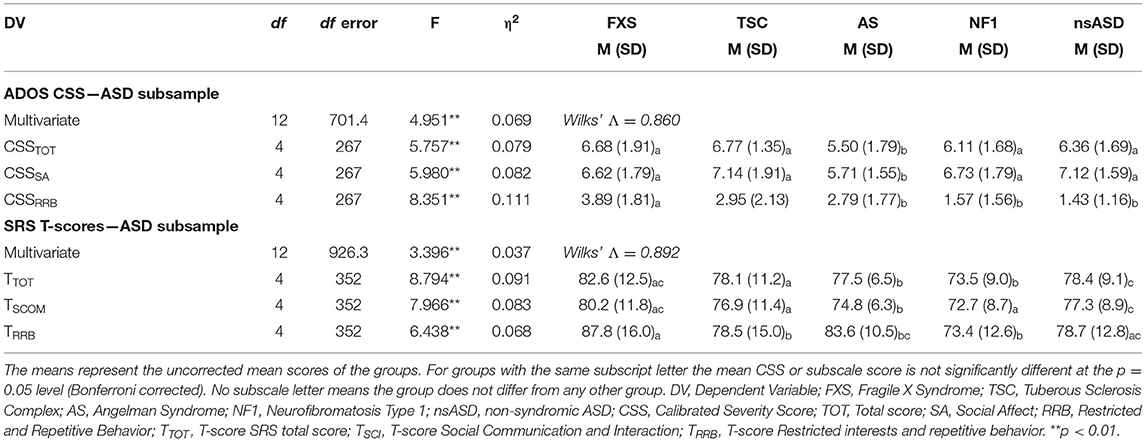
Table 3. Cross-syndrome comparisons of symptom severity scores in individuals with an ASD classification.
SRS
The group comparison of SRS severity scores in individuals with an SRS ASD classification again showed a significant main effect of Group for the collective and individual T-scores (see Table 3 and Figure 3B). While for the ADOS only the AS group severity scores were lower compared to the nsASD group on the total and Social Affect severity scores, the SRS post hoc comparisons showed that the nsASD group had higher severity scores compared to individuals with an ASD classification in the TSC, AS and NF1 groups on these two subscales. Also unlike the ADOS, there was no difference in severity scores between individuals with an ASD classification in the FXS group and the nsASD group on the SRS Restricted Interests and Repetitive Behavior domain. The nsASD group did show higher severity scores on the SRS Restricted Interests and Repetitive Behavior domain compared to individuals with an SRS ASD classification in the NF1 and TSC groups.
When we compare the syndrome groups to each other, individuals with an ASD classification in the FXS group had higher severity scores on the total score compared to individuals with an ASD classification in the AS and NF1 groups, higher scores compared to individuals with an ASD classification in the AS group on the Social Communication and Interaction domain, and higher severity scores compared to all other syndrome groups on the Restricted Interests and Repetitive Behavior domain. Unlike for the ADOS, we did not find differences in SRS severity scores between individuals with an ASD classification in the TSC, AS and NF1 groups.
Cross-Syndrome Symptom Profile Analysis in Individuals With an ASD Classification
ADOS
The results of the cross-syndrome comparison of ASD symptom profiles are presented in Figure 4 and Supplementary Table 1. Individuals with an ASD classification on the ADOS in the FXS and AS group had similar profiles for all subscales except creativity and play, for which the AS group had higher severity scores than the FXS group, as well as the NF1 group. The profiles of individuals with an ADOS ASD classification in the NF1 and TSC groups were similar to each other, as well as to the profile of the nsASD group, for all subscales.
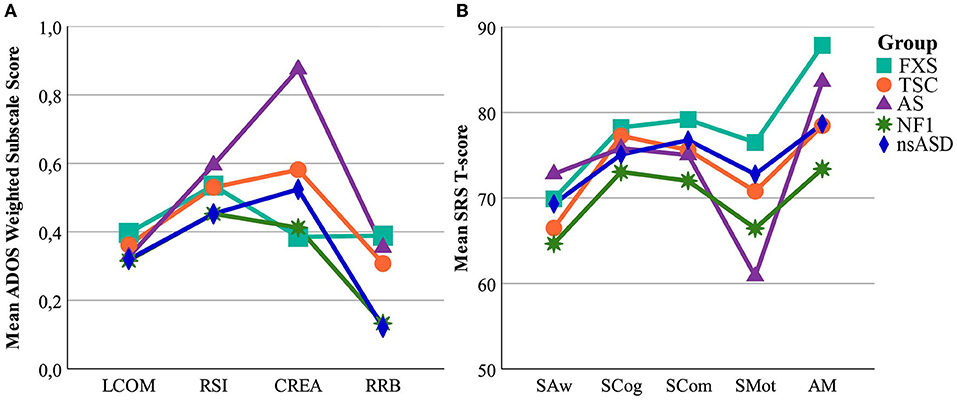
Figure 4. ASD symptom severity profile per syndrome group in the ASD subsample. (A) Means represent ADOS Weighted Subscale Scores. (B) Means represent SRS subscale T-scores. FXS, Fragile X Syndrome; TSC, Tuberous Sclerosis Complex; AS, Angelman Syndrome; NF1, Neurofibromatosis Type 1; nsASD, non-syndromic Autism Spectrum Disorder; WSS, Weighted subscale score; LCOM, Language and communication; RSI, Reciprocal Social Interaction; CREA, Creativity and Play subscale; RRB, Restricted and Repetitive Behavior; SAw, Social Awareness; SCog, Social Cognition; SCom, Social Communication; SM, Social Motivation; AM, Autistic mannerisms.
SRS
Contrary to the ADOS, the profiles of individuals with an ASD classification on the SRS in the FXS and AS groups showed little similarity for the SRS, as the FXS group showed higher severity scores compared to the AS group on all subscales except Social Awareness. Instead, the profile of the FXS group was similar to that of the nsASD group on all subscales. The AS group on the other hand showed lower severity scores compared to the nsASD group on the Social Cognition, Social Communication and Social Motivation subscales. Similar to the ADOS, individuals with an ASD classification in the NF1 and TSC groups shared a similar ASD profile for all subscales of the SRS, but their profiles were not similar to that of the nsASD group.
Discussion
The main aim of this study was to identify differences and similarities in ASD symptomatology between monogenetic syndromes with high ASD prevalence—FXS, TSC, NF1, and AS—that may reveal how different genetic variations affect ASD symptom severity.
ASD Classification Prevalence
In line with the literature, for both instruments, the ASD classification prevalence was highest in the FXS group and lowest in the NF1 group. The prevalence of ADOS and SRS ASD classifications we found for the FXS group (around 80–90%), and the AS group (70–90%) fell on the high end of the range found in the literature. Overall, ASD classification prevalence seemed to be higher for the SRS compared to the ADOS. This difference was especially high for the TSC (26% difference) and AS groups (29% difference), although we did not test this statistically as the samples contained different individuals. The difference in ASD classification prevalence between the ADOS and SRS is likely the result of the fundamental differences between the instruments. First, as a screening instrument the SRS is required to be highly sensitive by design. Secondly, the caregiver's perspective provided by the SRS may be more subjective. Thirdly, the setting and time period that is covered by these instruments may affect the scores, as some children may have learned to present more socially desirable behavior in unfamiliar surroundings than they would do in the comfort of their own home. Alternatively, they may experience more anxiety in the presence of an unfamiliar individual.
An important factor that may explain the higher prevalence of ADOS and SRS ASD classifications in children with FXS and AS is their developmental level. Generally, individuals with FXS and AS experience more severe intellectual disability or developmental delay compared to NF1. Cognitive impairment is known to affect scores on ASD screening instruments (75), and has been related to reduced specificity of the ADOS, especially for young children (73). Cognitive impairment additionally impacts whether individuals qualify for a DSM-5 classification, which impacts their access to health care services (33). The DSM-5 requires that difficulties in social communication must be lower than could be expected based on the developmental level of the individual, so that the deficiencies in social skills cannot be attributed to developmental delay. Therefore, it is likely that difficulties in social communication in individuals with FXS and AS are more frequently attributed to developmental delay rather than to the presence of ASD. This means that children with intellectual disability or developmental delay can score in the clinical range on screening or diagnostic instruments but not qualify for a formal DSM-5 ASD classification, even though they may experience the same symptom severity. At the same time, children with intellectual disability or developmental delay who qualify for a formal DSM-5 ASD classification are likely to show more severe symptoms compared to children with a formal DSM-5 ASD classification without intellectual disability or developmental delay. Even though we controlled for IQ/DQ in our analyses, it remains unclear whether the symptoms we see in our sample are beyond their expected developmental level.
Given the wide variety of symptom profiles that exist, in both syndromic and non-syndromic populations, some researchers have raised the question whether an all-or-nothing ASD classification should be replaced by a multimodal symptom-based assessment of health care needs (68). Based on our findings, we would argue that, at least in children with a syndromic form of ASD, a symptom-based approach might be favorable. This would ensure that individuals who experience ASD symptoms are eligible for treatment of these symptoms regardless of their diagnostic status. However, further research is required to better understand the effect of developmental delay on social skills measured by ASD screening instruments. While an all-or-nothing diagnosis is still the standard, the differences between these instruments in our study highlight the importance of a multimodal assessment of ASD symptoms to diagnose ASD reliably in these children.
Cross-Syndrome Comparisons of Symptom Severity Scores
In line with our hypothesis, and in accordance with the ASD classification prevalence, ASD symptom severity overall was highest in the FXS group and lowest in the NF1 group when we compared the main subscales of the ADOS and SRS. Also in line with our expectations, the scores of the TSC and AS groups mostly fell between the FXS and NF1 scores. The TSC group showed higher symptom severity compared to the AS and NF1 group for the ADOS social affect domain, and higher scores than the NF1 group on the restricted and repetitive behavior domain of the SRS. Based on the similarities in the underlying pathways of FXS and AS, and of TSC and NF1, we hypothesized that we might also find similarities in symptom presentation between these groups. Contrary to our expectations, the overall ASD severity scores of the TSC group seemed to be more similar to those of the FXS group whereas the AS group scores appeared more similar to those of the NF1 group.
Cross-Syndrome Comparisons of Symptom Severity Scores in Individuals With an ASD Classification
By comparing the syndrome groups only including individuals with an instrument-based ASD classification we reduced the within-group variability and we were able to compare our syndrome groups to a non-syndromic ASD group. The severity scores of the syndrome groups differed from those of the non-syndromic ASD group on several domains. Especially on the SRS, the syndrome groups showed less ASD symptom severity compared to non-syndromic ASD in the social domain. On the restricted and repetitive behavior domains on the other hand, the nsASD severity scores were lower than those of the FXS group, and higher than those of the TSC and NF1 groups. Despite some slight differences, the results of the subgroup comparisons were broadly similar for the ADOS and the SRS. On the main scores of the ADOS and SRS, the scores that determine whether someone qualifies for an ASD classification, the syndrome groups seem to perform relatively well in terms of social interaction and communication compared to the non-syndromic ASD group. As we discussed earlier, the DSM-5 requires that social communication difficulties are more impaired than would be expected based on the developmental level of an individual. Our findings suggest that a combination of severe developmental delay and relatively good social communication skills may prevent individuals with these syndromes from receiving an ASD classification or even a clinical diagnosis, which influences their access to health care services.
Cross-Syndrome Symptom Profile Analysis in Individuals With an ASD Classification
Our comparison of the ASD symptom profiles revealed several syndrome-specific strengths and challenges. While the FXS group scored highest overall, our results demonstrated a specific relative challenge for this group in the restricted interests and repetitive behavior domain. On the other end of the spectrum, the NF1 group had the least severe ASD symptoms overall, and had a specific relative strength in the restricted and repetitive behavior domain. The low severity scores in NF1 could cause ASD symptoms to be more easily overlooked in clinical practice in this group compared to the other syndrome groups. The TSC group also showed more challenges in the restricted and repetitive behavior domain, but their scores in this domain were lower than the FXS group and the nsASD group. For the AS group we found a relative strength on the social communication and social motivation domains, and a relative weakness in the reciprocal social interaction and in the creativity and play domains of the ADOS. Despite the fact that for all children with AS the lowest module was selected, this effect is likely due to a discrepancy between the developmental level of the AS group and the demands that some ADOS items put on the children. Therefore, in clinical practice, all scores that require a certain cognitive level must be interpreted cautiously for individuals with severe developmental delay.
While the genetic variations in FXS and AS, and in NF1 and TSC, respectively, affect neurodevelopmental pathways in a similar manner, the syndromes are fundamentally different in their origin as well as their phenotypes. Therefore, it would be highly unlikely that these syndromes would show an identical pattern of ASD symptoms on all subscales. Despite several differences, the syndrome pairs did show similarities on a majority of the ASD symptom subscales. To further explore the relationship between the observed similarities in the ASD profiles and the genetic pathways affected in these syndromes deep phenotyping studies are recommended that, for example, include (neural) biomarkers.
Strengths and Limitations
A strength of our study is that our sample was relatively free of selection bias. All assessments were carried out in the context of regular clinical care and all children were assessed regardless of whether ASD was suspected. This was supported by the fact that we did not find a difference in ASD severity between the children with and without an IQ assessment. Nevertheless, a selection bias may still exist for the FXS group. As the somatic problems are often mild or absent in FXS—as opposed to NF1, TSC and AS—it is possible that only children with more severe (behavioral) problems choose to visit the ENCORE center of expertise.
Even though our sample is relatively large for a cross-disorder comparison between rare disorders, and the sample is relatively free of bias, we could not control for neurobiological variability within the syndrome groups (e.g., the deletion status in AS, the mutation type in TSC, the locations of neuronal tubers or abnormal tissue growth in NF1 and TSC, or the genetic mosaicism and gender differences related to the X-linked nature of FXS). We also did not account for other comorbidity within our sample, such as epilepsy, ADHD or anxiety disorders. While ASD, social anxiety and ADHD are distinct neurodevelopmental conditions, their symptoms do overlap and therefore comorbidity of ADHD and/or anxiety with ASD may influence scores on both the ADOS and the SRS. Especially in FXS, social anxiety is very common, in children with and without an ASD diagnosis (87). Future in-depth studies should explore these potentially contributing factors in these individual syndromes and study their effect on the development and presentation of ASD symptoms. In the subgroup analyses the sample sizes were even smaller as we included only individuals with an ADOS or SRS ASD classification. We chose to use an instrument-based classification for both the SRS and ADOS separately, instead of DSM-5 criteria, or a combined ADOS and SRS classification, for our subgroup selection because we wanted to examine the broad spectrum of ASD symptoms regardless of whether individuals qualified for a DSM-5 classification, or whether they qualified on both instruments. Although conclusions on the presence of clinical ASD diagnoses within these syndrome groups could not be drawn with this approach, it enabled us to examine both clinical and sub-clinical symptoms present in these syndromes. While a reduction of sample sizes in our subgroup analyses was expected, given the prevalence of ASD in these syndromes, the results of the subgroup analyses should be interpreted cautiously. Therefore, replication of this study in a larger sample, while accounting for syndrome specific features, would be necessary to validate the results of this study. This may be achieved by combining data from large natural history studies, such as the FORWARD study on FXS (88) and the TSC Natural History Database (TSC Alliance) for example. Data from the FORWARD study shows that 87% of children with FXS would classify as having ASD on the SRS, irrespective of ASD diagnosis (89), which supports the findings of this study. However, because ASD is not as prevalent in all syndromes, ASD screening is not standard practice for all syndromes, so selection bias should be accounted for.
Regarding gender differences, males in general are more likely to receive an ASD diagnosis than females (90). In addition, we know that FXS is more prevalent in males, and that males with FXS are more severely affected, in terms of developmental level as well as ASD symptomatology. Therefore, we added gender as a covariate in our analyses. The proportion of males vs. females in our FXS group was high at ~75%. Because the prevalence of ASD in males is higher than in females, in FXS (33) as well as in general (33, 91), including more males may have resulted in a higher ASD classification prevalence in our FXS sample compared to other studies, and a lower developmental level. Even though our sample size was relatively large for a cross-disorder comparison between rare disorders, the number of included females was not sufficient for stratification into gender subgroups. From studies in non-syndromic ASD as well as syndrome groups, we know that gender affects the pattern of ASD symptom presentation (90). Future studies should stratify their data in order to reveal the impact of gender on the presentation of ASD characteristics in males vs. females.
Another limitation of this study is the difference in developmental level between our groups, especially between the syndrome groups and the nsASD group. As our FXS group contained more males than expected, and the IQ of males with FXS is generally lower than that of females with FXS, the IQ of our FXS group may be relatively low compared to more balanced samples. It is known that the developmental level of children and young adults can influence the measurement of ASD symptom severity (77, 78). The ADOS allows clinicians to choose a module based on the developmental level of the participant, which is estimated based on the level of spoken language. However, the cognitive profiles of syndrome groups also show syndrome specific strengths and challenges. Individuals with similar levels of spoken language may vary greatly in other areas of cognitive and motor development. There are no specific norms available for the ADOS for samples with developmental delay or complex behavioral problems. In our study we compared symptom severity in the syndrome groups to a non-syndromic ASD sample in order to better interpret the group differences that we have found. However, it is important to keep in mind that the developmental level of this non-syndromic ASD sample was higher than that of our syndrome groups. We have attempted to reduce the effect of developmental levels on our results by adding IQ or DQ scores as a covariate in our analyses, but as IQ/DQ levels are likely to vary within and between our groups, our results should be interpreted cautiously.
Conclusion
The syndrome-specific strengths and challenges we found in FXS, TSC, AS, and NF1 provide a frame of reference to evaluate an individual's symptom severity relative to the syndromic population as a whole and to guide treatment decisions. Based on the overall ASD symptom profile, clinicians should closely monitor the development of ASD-symptoms, taking into account the syndrome-specific strengths and weaknesses within the ASD profile when selecting treatment methods. Similarities in ASD symptom profiles between AS and FXS, and between NF1 and TSC may be caused by similarities in their underlying neurobiological pathways. Deep phenotyping studies are required to link symptom patterns to specific neurobiological pathways more directly. Additionally, the variation in symptom severity within our sample also highlights the need to investigate patterns of ASD symptom severity within syndromes, which might reveal subgroups with a more homogeneous symptom presentation.
Encore Expertise Group
Rianne Oostenbrink1, 2, 3, Karen C.G.B. Bindels-de Heus1, 3, Marie-Claire Y. de Wit1, 4, Henriëtte A. Mol1, 3 and Ype Elgersma1, 5.
1ENCORE Expertise Center for Neurodevelopmental Disorders, Erasmus MC University Medical Center, Rotterdam, Netherlands
2Full Member of the European Reference Network on Genetic Tumor Risk Syndromes (ERN GENTURIS)—Project ID No 739547
3Department of General Pediatrics, Erasmus MC, Rotterdam, Netherlands
4Department of Neurology, Erasmus MC, Rotterdam, Netherlands
5Department of Clinical Genetics, Erasmus MC, Rotterdam, Netherlands
Data Availability Statement
The original contributions presented in the study are included in the article/Supplementary Materials, further inquiries can be directed to the corresponding author/s.
Ethics Statement
The studies involving human participants were reviewed and approved by Medical Ethics Committee of the Erasmus University Medical Center, Rotterdam, Netherlands (MEC-2015-203 and MEC-2011-078). Written informed consent to participate in this study was provided by the participants' legal guardian/next of kin.
Author Contributions
BD, LH, PN, JL, AR, the ENCORE Expertise Group, SM, and GD were involved in the design of the ENCORE database and supervised data collection. ES, KL, and DH gathered and prepared the dataset. KG-L provided the data of the Social Spectrum Study. KL performed the analyses. SM, GD, and MH were involved in the planning and supervision of the project. KL and ES prepared the manuscript in consultation with SM, BD, and GD. All authors discussed the results and contributed to the final manuscript.
Funding
This research was financially supported by the Sophia Children's Hospital Fund (Rotterdam, Netherlands) under grant numbers S16-14 and B14-02. This research was supported (not financially) by the European Reference Network on Genetic Tumor Risk Syndromes (ERN GENTURIS) – Project ID No 739547. ERN GENTURIS is partly co-funded by the European Union within the framework of the Third Health Programme ERN-2016-Framework Partnership Agreement 2017–2021.
Conflict of Interest
The authors declare that the research was conducted in the absence of any commercial or financial relationships that could be construed as a potential conflict of interest.
Publisher's Note
All claims expressed in this article are solely those of the authors and do not necessarily represent those of their affiliated organizations, or those of the publisher, the editors and the reviewers. Any product that may be evaluated in this article, or claim that may be made by its manufacturer, is not guaranteed or endorsed by the publisher.
Acknowledgments
The authors thank all clinicians and staff members of the ENCORE expertise center and the department of child- and adolescent psychiatry/psychology at the Erasmus MC involved in data collection. We also thank the student team involved in structuring the database, and Anoek Melaard and Jesse de Kok specifically for organizing the ADOS and SRS datasets. We also thank the participants and researchers of the Social Spectrum Study for allowing us to use their data as a frame of reference in interpreting our results. The authors of this publication are members of the European Reference Network on Rare Congenital Malformations and Rare Intellectual Disability ERN-ITHACA [EU Framework Partnership Agreement ID: 3HP-HP-FPA ERN-01-2016/739516].
Supplementary Material
The Supplementary Material for this article can be found online at: https://www.frontiersin.org/articles/10.3389/fpsyt.2022.852208/full#supplementary-material
References
1. Baio J, Wiggins L, Christensen DL, Maenner MJ, Daniels J, Warren Z, et al. Prevalence of Autism spectrum disorder among children aged 8 years - autism and developmental disabilities monitoring network, 11 sites, United States, 2014. MMWR Surveill Summ. (2018) 67:1–23. doi: 10.15585/mmwr.ss6706a1
2. Rodriguez-Fontenla C, Carracedo A. UTMOST, a single and cross-tissue TWAS (Transcriptome Wide Association Study), reveals new ASD (Autism Spectrum Disorder) associated genes. Transl Psychiatry. (2021) 11:256. doi: 10.1038/s41398-021-01378-8
3. Tick B, Bolton P, Happé F, Rutter M, Rijsdijk F. Heritability of autism spectrum disorders: a meta-analysis of twin studies. J Child Psychol Psychiatry. (2016) 57:585–95. doi: 10.1111/jcpp.12499
4. Richards C, Jones C, Groves L, Moss J, Oliver C. Prevalence of autism spectrum disorder phenomenology in genetic disorders: a systematic review and meta-analysis. Lancet Psychiat. (2015) 2:909–16. doi: 10.1016/S2215-0366(15)00376-4
5. Henske EP, Józwiak S, Kingswood JC, Sampson JR, Thiele EA. Tuberous sclerosis complex. Nat Rev Dis Primers. (2016) 2:16035. doi: 10.1038/nrdp.2016.35
6. Bagni C, Zukin RS. A synaptic perspective of fragile X syndrome and autism spectrum disorders. Neuron. (2019) 101:1070–88. doi: 10.1016/j.neuron.2019.02.041
7. Lodish MB, Stratakis CA. Endocrine tumours in neurofibromatosis type 1, tuberous sclerosis and related syndromes. Best Pract Res Clin Endocrinol Metab. (2010) 24:439–49. doi: 10.1016/j.beem.2010.02.002
8. Millan MJ. An epigenetic framework for neurodevelopmental disorders: from pathogenesis to potential therapy. Neuropharmacology. (2013) 68:2–82. doi: 10.1016/j.neuropharm.2012.11.015
9. Maranga C, Fernandes TG, Bekman E, da Rocha ST. Angelman syndrome: a journey through the brain. FEBS J. (2020) 287:2154–75. doi: 10.1111/febs.15258
10. Kelleher RJ, Bear MF. The autistic neuron: troubled translation? Cell. (2008) 135:401–6. doi: 10.1016/j.cell.2008.10.017
11. Fahnestock M, Nicolini C. Bridging the gap between genes and behavior: brain-derived neurotrophic factor and the mTOR pathway in idiopathic autism. Autism Open Access. (2015) 5:2. doi: 10.4172/2165-7890.1000143
12. Moss J, Nelson L, Powis L, Waite J, Richards C, Oliver C. A comparative study of sociability in Angelman, Cornelia de Lange, fragile X, down and Rubinstein Taybi syndromes and autism spectrum disorder. Am J Intellect Dev Disabil. (2016) 121:465–86. doi: 10.1352/1944-7558-121.6.465
13. Heald M, Adams D, Oliver C. Profiles of atypical sensory processing in Angelman, Cornelia de Lange and Fragile X syndromes. J Intellect Disabil Res. (2020) 64:117–30. doi: 10.1111/jir.12702
14. Abbeduto L, Thurman AJ, McDuffie A, Klusek J, Feigles RT, Ted Brown W, et al. ASD comorbidity in fragile X syndrome: symptom profile and predictors of symptom severity in adolescent and young adult males. J Autism Dev Disord. (2019) 49:960–77. doi: 10.1007/s10803-018-3796-2
15. Eijk S, Mous SE, Dieleman GC, Dierckx B, Rietman AB, de Nijs PFA, et al. Autism spectrum disorder in an unselected cohort of children with neurofibromatosis type 1 (NF1). J Autism Dev Disord. (2018) 48:2278–85. doi: 10.1007/s10803-018-3478-0
16. Niu M, Han Y, Dy ABC, Du J, Jin H, Qin J, et al. Autism symptoms in fragile X syndrome. J Child Neurol. (2017) 32:903–9. doi: 10.1177/0883073817712875
17. Jeste SS, Varcin KJ, Hellemann GS, Gulsrud AC, Bhatt R, Kasari C, et al. Symptom profiles of autism spectrum disorder in tuberous sclerosis complex. Neurology. (2016) 87:766–72. doi: 10.1212/WNL.0000000000003002
18. Turner G, Webb T, Wake S, Robinson H. Prevalence of fragile X syndrome. Am J Med Genet. (1996) 64:196–7. doi: 10.1002/(SICI)1096-8628(19960712)64:1<196::AID-AJMG35>3.0.CO;2-G
19. Hagerman PJ. The fragile X prevalence paradox. J Med Genet. (2008) 45:498–9. doi: 10.1136/jmg.2008.059055
20. Pieretti M, Zhang FP, Fu YH, Warren ST, Oostra BA, Caskey CT, et al. Absence of expression of the FMR-1 gene in fragile X syndrome. Cell. (1991) 66:817–22. doi: 10.1016/0092-8674(91)90125-I
21. Bagni C, Greenough WT. From mRNP trafficking to spine dysmorphogenesis: the roots of fragile X syndrome. Nat Rev Neurosci. (2005) 6:376–87. doi: 10.1038/nrn1667
22. Choi CH, Schoenfeld BP, Weisz ED, Bell AJ, Chambers DB, Hinchey J, et al. PDE-4 inhibition rescues aberrant synaptic plasticity in Drosophila and mouse models of fragile X syndrome. J Neurosci. (2015) 35:396–408. doi: 10.1523/JNEUROSCI.1356-12.2015
23. Zhang Z, Marro SG, Zhang Y, Arendt KL, Patzke C, Zhou B, et al. The fragile X mutation impairs homeostatic plasticity in human neurons by blocking synaptic retinoic acid signaling. Sci Transl Med. (2018) 10:eaar4338. doi: 10.1126/scitranslmed.aar4338
24. Halevy T, Czech C, Benvenisty N. Molecular mechanisms regulating the defects in fragile X syndrome neurons derived from human pluripotent stem cells. Stem Cell Rep. (2015) 4:37–46. doi: 10.1016/j.stemcr.2014.10.015
25. Huddleston LB, Visootsak J, Sherman SL. Cognitive aspects of fragile X syndrome. Wiley Interdiscipl Rev Cogn Sci. (2014) 5:501–8. doi: 10.1002/wcs.1296
26. Loesch DZ, Huggins RM, Hagerman RJ. Phenotypic variation and FMRP levels in fragile X. Ment Retard Dev Disabil Res Rev. (2004) 10:31–41. doi: 10.1002/mrdd.20006
27. Gallagher A, Hallahan B. Fragile X-associated disorders: a clinical overview. J Neurol. (2012) 259:401–13. doi: 10.1007/s00415-011-6161-3
28. Rogers SJ, Wehner EA, Hagerman R. The behavioral phenotype in fragile X: symptoms of autism in very young children with fragile x syndrome, idiopathic autism, and other developmental disorders. J Dev Behav Pediatr. (2001) 22:409–17. doi: 10.1097/00004703-200112000-00008
29. Hagerman RJ, Jackson Iii AW, Levitas A, Rimland B, Braden M, Opitz JM, et al. An analysis of autism in fifty males with the fragile X syndrome. Am J Med Genet. (1986) 23:359–74. doi: 10.1002/ajmg.1320230128
30. Marlborough M, Welham A, Jones C, Reckless S, Moss J. Autism spectrum disorder in females with fragile X syndrome: a systematic review and meta-analysis of prevalence. J Neurodev Disord. (2021) 13:28. doi: 10.1186/s11689-021-09362-5
31. Bailey DB Jr., Hatton DD, Mesibov G, Ament N, Skinner M. Early development, temperament, and functional impairment in autism and fragile X syndrome. J Autism Dev Disord. (2000) 30:49–59. doi: 10.1023/A:1005412111706
32. McDuffie A, Thurman AJ, Hagerman RJ, Abbeduto L. Symptoms of autism in males with fragile X syndrome: a comparison to nonsyndromic ASD using current ADI-R scores. J Autism Dev Disord. (2015) 45:1925–37. doi: 10.1007/s10803-013-2013-6
33. Wheeler AC, Mussey J, Villagomez A, Bishop E, Raspa M, Edwards A, et al. DSM-5 changes and the prevalence of parent-reported autism spectrum symptoms in Fragile X syndrome. J Autism Dev Disord. (2015) 45:816–29. doi: 10.1007/s10803-014-2246-z
34. Smith LE, Barker ET, Seltzer MM, Abbeduto L, Greenberg JS. Behavioral phenotype of fragile X syndrome in adolescence and adulthood. Am J Intellect Dev Disabil. (2012) 117:1–17. doi: 10.1352/1944-7558-117.1.1
35. Wolff JJ, Bodfish JW, Hazlett HC, Lightbody AA, Reiss AL, Piven J. Evidence of a distinct behavioral phenotype in young boys with fragile X syndrome and autism. J Am Acad Child Adolesc Psychiatry. (2012) 51:1324–32. doi: 10.1016/j.jaac.2012.09.001
36. Kishino T, Lalande M, Wagstaff J. UBE3A/E6-AP mutations cause Angelman syndrome. Nat Genet. (1997) 15:70–3. doi: 10.1038/ng0197-70
37. Williams CA, Beaudet AL, Clayton-Smith J, Knoll JH, Kyllerman M, Laan LA, et al. Angelman syndrome 2005: updated consensus for diagnostic criteria. Am J Med Genet A. (2006) 140:413–8. doi: 10.1002/ajmg.a.31074
38. Micheletti S, Palestra F, Martelli P, Accorsi P, Galli J, Giordano L, et al. Neurodevelopmental profile in Angelman syndrome: more than low intelligence quotient. Italian J Pediatr. (2016) 42:91. doi: 10.1186/s13052-016-0301-4
39. Peters SU, Goddard-Finegold J, Beaudet AL, Madduri N, Turcich M, Bacino CA. Cognitive and adaptive behavior profiles of children with Angelman syndrome. Am J Med Genet Part A. (2004) 128:110–3. doi: 10.1002/ajmg.a.30065
40. Trillingsgaard A, JR OS. Autism in Angelman syndrome: an exploration of comorbidity. Autism. (2004) 8:163–74. doi: 10.1177/1362361304042720
41. Bonati MT, Russo S, Finelli P, Valsecchi MR, Cogliati F, Cavalleri F, et al. Evaluation of autism traits in Angelman syndrome: a resource to unfold autism genes. Neurogenetics. (2007) 8:169–78. doi: 10.1007/s10048-007-0086-0
42. Sahoo T, Peters SU, Madduri NS, Glaze DG, German JR, Bird LM, et al. Microarray based comparative genomic hybridization testing in deletion bearing patients with Angelman syndrome: genotype-phenotype correlations. J Med Genet. (2006) 43:512–6. doi: 10.1136/jmg.2005.036913
43. Cook EH Jr., Lindgren V, Leventhal BL, Courchesne R, Lincoln A, et al. Autism or atypical autism in maternally but not paternally derived proximal 15q duplication. Am J Hum Genet. (1997) 60:928–34.
44. Mahdavi M, Kheirollahi M, Riahi R, Khorvash F, Khorrami M, Mirsafaie M. Meta-analysis of the association between GABA receptor polymorphisms and Autism Spectrum Disorder (ASD). J Mol Neurosci. (2018) 65:1–9. doi: 10.1007/s12031-018-1073-7
45. Feliciano DM. The neurodevelopmental pathogenesis of Tuberous Sclerosis Complex (TSC). Front Neuroanat. (2020) 14:39. doi: 10.3389/fnana.2020.00039
46. Northrup H, Krueger DA, International Tuberous Sclerosis Complex Consensus G. Tuberous sclerosis complex diagnostic criteria update: recommendations of the 2012. International Tuberous Sclerosis Complex Consensus Conference. Pediatr Neurol. (2013) 49:243–54. doi: 10.1016/j.pediatrneurol.2013.08.001
47. Curatolo P, Cusmai R, Cortesi F, Chiron C, Jambaque I, Dulac O. Neuropsychiatric aspects of tuberous sclerosis. Ann N Y Acad Sci. (1991) 615:8–16. doi: 10.1111/j.1749-6632.1991.tb37743.x
48. Joinson C, O'Callaghan FJ, Osborne JP, Martyn C, Harris T, Bolton PF. Learning disability and epilepsy in an epidemiological sample of individuals with tuberous sclerosis complex. Psychol Med. (2003) 33:335–44. doi: 10.1017/S0033291702007092
49. Van Eeghen AM, Pulsifer MB, Merker VL, Neumeyer AM, Van Eeghen EE, Thibert RL, et al. Understanding relationships between autism, intelligence, and epilepsy: a cross-disorder approach. Dev Med Child Neurol. (2013) 55:146–53. doi: 10.1111/dmcn.12044
50. de Vries PJ, Hunt A, Bolton PF. The psychopathologies of children and adolescents with tuberous sclerosis complex (TSC). Eur Child Adolesc Psychiatry. (2007) 16:16–24. doi: 10.1007/s00787-006-0570-3
51. Mizuguchi M, Ikeda H, Kagitani-Shimono K, Yoshinaga H, Suzuki Y, Aoki M, et al. Everolimus for epilepsy and autism spectrum disorder in tuberous sclerosis complex: EXIST-3 substudy in Japan. Brain Dev. (2019) 41:1–10. doi: 10.1016/j.braindev.2018.07.003
52. French JA, Lawson JA, Yapici Z, Ikeda H, Polster T, Nabbout R, et al. Adjunctive everolimus therapy for treatment-resistant focal-onset seizures associated with tuberous sclerosis (EXIST-3): a phase 3, randomised, double-blind, placebo-controlled study. Lancet. (2016) 388:2153–63. doi: 10.1016/S0140-6736(16)31419-2
53. Sato A, Kasai S, Kobayashi T, Takamatsu Y, Hino O, Ikeda K, et al. Rapamycin reverses impaired social interaction in mouse models of tuberous sclerosis complex. Nat Commun. (2012) 3:1292. doi: 10.1038/ncomms2295
54. Petrasek T, Vojtechova I, Klovrza O, Tuckova K, Vejmola C, Rak J, et al. mTOR inhibitor improves autistic-like behaviors related to Tsc2 haploinsufficiency but not following developmental status epilepticus. J Neurodev Disord. (2021) 13:14. doi: 10.1186/s11689-021-09357-2
55. Rauen KA. The RASopathies. Annu Rev Genomics Hum Genet. (2013) 14:355–69. doi: 10.1146/annurev-genom-091212-153523
56. Tonsgard JH. Clinical manifestations and management of neurofibromatosis type 1. Semin Pediatr Neurol. (2006) 13:2–7. doi: 10.1016/j.spen.2006.01.005
57. Diwakar G, Zhang D, Jiang S, Hornyak TJ. Neurofibromin as a regulator of melanocyte development and differentiation. J Cell Sci. (2008) 121(Pt. 2):167–77. doi: 10.1242/jcs.013912
58. Stevenson DA, Zhou H, Ashrafi S, Messiaen LM, Carey JC, D'Astous JL, et al. Double inactivation of NF1 in tibial pseudarthrosis. Am J Hum Genet. (2006) 79:143–8. doi: 10.1086/504441
59. Molosh AI, Johnson PL, Spence JP, Arendt D, Federici LM, Bernabe C, et al. Social learning and amygdala disruptions in Nf1 mice are rescued by blocking p21-activated kinase. Nat Neurosci. (2014) 17:1583–90. doi: 10.1038/nn.3822
60. Bajenaru ML, Hernandez MR, Perry A, Zhu Y, Parada LF, Garbow JR, et al. Optic nerve glioma in mice requires astrocyte Nf1 gene inactivation and Nf1 brain heterozygosity. Cancer Res. (2003) 63:8573–7. Available online at: https://aacrjournals.org/cancerres/article/63/24/8573/510900/Optic-Nerve-Glioma-in-Mice-Requires-Astrocyte-Nf1
61. Garg S, Lehtonen A, Huson SM, Emsley R, Trump D, Evans DG, et al. Autism and other psychiatric comorbidity in neurofibromatosis type 1: evidence from a population-based study. Dev Med Child Neurol. (2013) 55:139–45. doi: 10.1111/dmcn.12043
62. Walsh KS, Vélez JI, Kardel PG, Imas DM, Muenke M, Packer RJ, et al. Symptomatology of autism spectrum disorder in a population with neurofibromatosis type 1. Dev Med Child Neurol. (2013) 55:131–8. doi: 10.1111/dmcn.12038
63. Torres Nupan MM, Velez Van Meerbeke A, López Cabra CA, Herrera Gomez PM. Cognitive and behavioral disorders in children with neurofibromatosis type 1. Front Pediatr. (2017) 5:227. doi: 10.3389/fped.2017.00227
64. Ottenhoff MJ, Rietman AB, Mous SE, Plasschaert E, Gawehns D, Brems H, et al. Examination of the genetic factors underlying the cognitive variability associated with neurofibromatosis type 1. Genet Med. (2020) 22:889–97. doi: 10.1038/s41436-020-0752-2
65. Morris SM, Acosta MT, Garg S, Green J, Huson S, Legius E, et al. Disease burden and symptom structure of autism in neurofibromatosis type 1: a study of the international NF1-ASD Consortium Team (INFACT). JAMA Psychiatry. (2016) 73:1276–84. doi: 10.1001/jamapsychiatry.2016.2600
66. Chisholm AK, Anderson VA, Pride NA, Malarbi S, North KN, Payne JM. Social function and autism spectrum disorder in children and adults with neurofibromatosis type 1: a systematic review and meta-analysis. Neuropsychol Rev. (2018) 28:317–40. doi: 10.1007/s11065-018-9380-x
67. Garg S, Plasschaert E, Descheemaeker MJ, Huson S, Borghgraef M, Vogels A, et al. Autism spectrum disorder profile in neurofibromatosis type I. J Autism Dev Disord. (2015) 45:1649–57. doi: 10.1007/s10803-014-2321-5
68. Müller RA, Amaral DG. Time to give up on autism spectrum disorder? Autism Res. (2017) 10:10–4. doi: 10.1002/aur.1746
69. Lord C, Charman T, Havdahl A, Carbone P, Anagnostou E, Boyd B, et al. The Lancet Commission on the future of care and clinical research in autism. Lancet. (2021) 399:P271–334. doi: 10.1016/S0140-6736(21)01541-5
70. Duvekot J, Hoopen LW, Slappendel G, van der Ende J, Verhulst FC, van der Sijde A, et al. Design and cohort characteristics of the social spectrum study: a multicenter study of the autism spectrum among clinically referred children. J Autism Dev Disord. (2017) 47:33–48. doi: 10.1007/s10803-016-2919-x
71. Constantino JN, Gruber CP. Social Responsiveness Scale Second Edition (SRS-2): Manual. Torrance, CA: Western Psychological Services (WPS) (2012).
72. Lord C, Rutter M, Dilavore P, Risi S. The Autism Diagnostic Observation Schedule (ADOS). Los Angeles, CA: Western Psychological Corporation (1999).
73. Gotham K, Risi S, Pickles A, Lord C. The Autism Diagnostic Observation Schedule: revised algorithms for improved diagnostic validity. J Autism Dev Disord. (2007) 37:613–27. doi: 10.1007/s10803-006-0280-1
74. Lord C, Rutter M, DiLavore PC, Risi S, Gotham K, Bishop S. Autism Diagnostic Observation Schedule. 2nd ed. Torrance, CA: Western Psychological Services (2012).
75. Hus V, Gotham K, Lord C. Standardizing ADOS domain scores: separating severity of social affect and restricted and repetitive behaviors. J Autism Dev Disord. (2014) 44:2400–12. doi: 10.1007/s10803-012-1719-1
76. Hus V, Lord C. The autism diagnostic observation schedule, module 4: revised algorithm and standardized severity scores. J Autism Dev Disord. (2014) 44:1996–2012. doi: 10.1007/s10803-014-2080-3
77. Molloy CA, Murray DS, Akers R, Mitchell T, Manning-Courtney P. Use of the Autism Diagnostic Observation Schedule (ADOS) in a clinical setting. Autism. (2011) 15:143–62. doi: 10.1177/1362361310379241
78. Hus V, Bishop S, Gotham K, Huerta M, Lord C. Factors influencing scores on the social responsiveness scale. J Child Psychol Psychiatry. (2013) 54:216–24. doi: 10.1111/j.1469-7610.2012.02589.x
79. Wahlstrom D, Breaux KC, Zhu J, Weiss LG. The Wechsler Preschool and Primary Scale of Intelligence—Third Edition, the Wechsler Intelligence Scale for Children—Fourth Edition, and the Wechsler Individual Achievement Test—Third Edition. Contemporary Intellectual Assessment: Theories, Tests, and Issues. 3rd ed. New York, NY: The Guilford Press (2012). p. 224–48.
80. Wechsler D. Weschler Intelligence Scale for Children: Third Edition Manual. San Antonio, TX: The Psychological Corporation (1991).
82. Wechsler DPC. WAIS-III: Administration and Scoring Manual: Wechsler Adult Intelligence Scale. San Antonio, TX: Psychological Corporation (1997).
84. Bayley N. Bayley Scales of Infant and Toddler Development. 3rd ed. San Antonio, TX: Harcourt Assessment (2006).
85. Tellegen PJ, Laros JA. Snijders-Oomen Niet-verbale Intelligentietest: Verantwoording. Göttingen: Hogrefe (2011).
87. Cordeiro L, Ballinger E, Hagerman R, Hessl D. Clinical assessment of DSM-IV anxiety disorders in fragile X syndrome: prevalence and characterization. J Neurodev Disord. (2011) 3:57–67. doi: 10.1007/s11689-010-9067-y
88. Sherman SL, Kidd SA, Riley C, Berry-Kravis E, Andrews HF, Miller RM, et al. FORWARD: a registry and longitudinal clinical database to study fragile X syndrome. Pediatrics. (2017) 139(Suppl. 3):S183–93. doi: 10.1542/peds.2016-1159E
89. Kidd SA, Berry-Kravis E, Choo TH, Chen C, Esler A, Hoffmann A, et al. Improving the diagnosis of autism spectrum disorder in fragile X syndrome by adapting the social communication questionnaire and the social responsiveness scale-2. J Autism Dev Disord. (2020) 50:3276–95. doi: 10.1007/s10803-019-04148-0
90. Duvekot J, van der Ende J, Verhulst FC, Slappendel G, van Daalen E, Maras A, et al. Factors influencing the probability of a diagnosis of autism spectrum disorder in girls versus boys. Autism. (2017) 21:646–58. doi: 10.1177/1362361316672178
Keywords: Fragile X Syndrome, Angelman Syndrome, Tuberous Sclerosis Complex, Neurofibromatosis Type 1, autism spectrum disorder, autistic traits
Citation: Lubbers K, Stijl EM, Dierckx B, Hagenaar DA, ten Hoopen LW, Legerstee JS, de Nijs PFA, Rietman AB, Greaves-Lord K, Hillegers MHJ, Dieleman GC, Mous SE and ENCORE Expertise Center (2022) Autism Symptoms in Children and Young Adults With Fragile X Syndrome, Angelman Syndrome, Tuberous Sclerosis Complex, and Neurofibromatosis Type 1: A Cross-Syndrome Comparison. Front. Psychiatry 13:852208. doi: 10.3389/fpsyt.2022.852208
Received: 10 January 2022; Accepted: 26 April 2022;
Published: 16 May 2022.
Edited by:
Kate Anne Woodcock, University of Birmingham, United KingdomReviewed by:
Walter Erwin Kaufmann, Emory University, United StatesMaija Liisa Castrén, University of Helsinki, Finland
Copyright © 2022 Lubbers, Stijl, Dierckx, Hagenaar, ten Hoopen, Legerstee, de Nijs, Rietman, Greaves-Lord, Hillegers, Dieleman, Mous and ENCORE Expertise Center. This is an open-access article distributed under the terms of the Creative Commons Attribution License (CC BY). The use, distribution or reproduction in other forums is permitted, provided the original author(s) and the copyright owner(s) are credited and that the original publication in this journal is cited, in accordance with accepted academic practice. No use, distribution or reproduction is permitted which does not comply with these terms.
*Correspondence: Sabine E. Mous, cy5tb3VzQGVyYXNtdXNtYy5ubA==
†These authors have contributed equally to this work and share last authorship