- 1CNRS, Aquitaine Institute for Cognitive and Integrative Neuroscience, INCIA, UMR 5287, Université de Bordeaux, Bordeaux, France
- 2Laboratoire EA 4136 – Handicap Activité Cognition Santé HACS, Collège Science de la Sante, Institut Universitaire des Sciences de la Réadaptation, Université de Bordeaux, Bordeaux, France
- 3Centre Hospitalier Charles-Perrens, Pôle Universitaire de Psychiatrie de l’Enfant et de l’Adolescent, Bordeaux, France
- 4Department of Neurology, University of Louisville, Louisville, KY, United States
Autism spectrum disorder (ASD) is a neurodevelopmental disorder, which affects 1 in 44 children and may cause severe disabilities. Besides socio-communicational difficulties and repetitive behaviors, ASD also presents as atypical sensorimotor function and pain reactivity. While chronic pain is a frequent co-morbidity in autism, pain management in this population is often insufficient because of difficulties in pain evaluation, worsening their prognosis and perhaps driving higher mortality rates. Previous observations have tended to oversimplify the experience of pain in autism as being insensitive to painful stimuli. Various findings in the past 15 years have challenged and complicated this dogma. However, a relatively small number of studies investigates the physiological correlates of pain reactivity in ASD. We explore the possibility that atypical pain perception in people with ASD is mediated by alterations in pain perception, transmission, expression and modulation, and through interactions between these processes. These complex interactions may account for the great variability and sometimes contradictory findings from the studies. A growing body of evidence is challenging the idea of alterations in pain processing in ASD due to a single factor, and calls for an integrative view. We propose a model of the pain cycle that includes the interplay between the molecular and neurophysiological pathways of pain processing and it conscious appraisal that may interfere with pain reactivity and coping in autism. The role of social factors in pain-induced response is also discussed. Pain assessment in clinical care is mostly based on subjective rather than objective measures. This review clarifies the strong need for a consistent methodology, and describes innovative tools to cope with the heterogeneity of pain expression in ASD, enabling individualized assessment. Multiple measures, including self-reporting, informant reporting, clinician-assessed, and purely physiological metrics may provide more consistent results. An integrative view on the regulation of the pain cycle offers a more robust framework to characterize the experience of pain in autism.
Introduction
“On the one hand, some people with autism can tolerate extreme heat, cold or pressure and seem relatively insensitive to pain. On the other hand, they may experience intense pain from idiosyncratic sources but struggle to communicate it.” Citation from Spectrum Autism Research News (1).
Autism Spectrum Disorder (ASD) is a neurodevelopmental disorder, affecting around one birth out of 150 worldwide (2). According to new report from Centers for Disease Control and Prevention, the incidence is as high as 1 in 44 children, leading to a prevalence estimated at 2.3% (3), a male-to-female ratio around 3:1 (4). ASD significantly decreases the person’s educational, social and employment opportunities. Among other comorbidities, pain is frequent, sometimes undiagnosed or diagnosed with delay in children with ASD (5–8). Children with autism are about twice as likely as their typical peers to experience chronic or recurrent pain (9). Various co-morbidities in ASD, such as epilepsy (10, 11), joint hypermobility-related disorders (12), gastrointestinal disorders (13), anxiety (14), and sleep problems (15) may be additive sources of pain. Individuals with ASD face significant inequities in healthcare, despite their high rate of medical comorbidities. They tend to have poorer health outcomes (16) and higher mortality rates (10, 17, 18) than their neurotypical peers. Aside from other core symptoms, such as socio-communicational difficulties, repetitive activities and/or interests and atypical sensory modulation, some individuals may present high levels of Self-Injury Behaviors (SIB; 19) which may be related to pain reactivity in ASD (20, 21).
The paradox of pain perception in ASD has attracted the attention of researchers for many years. Atypical pain perception, expression and difficulties in pain assessment in people with ASD have been described (22–27). On one hand, some studies report a decrease or absence of pain reactivity in daily life mostly based on reports from self or others and clinical observations (22, 28). On the other hand, various results show equal or greater responsiveness to painful stimuli in experimental conditions (29–31). Atypical pain sensations, like allodynia (extreme sensitivity to usual non-painful tactile stimulation causing intensive pain), paradoxical heat sensation (gentle cooling perceived as hot or burning), and hypoesthesia (decreased pain sensitivity) are also reported for ASD (32). Various hypotheses attempted to explain observed alterations. However, none of these hypothesis by themselves were able to reconcile these apparently contradictory findings.
The International Association for the Study of Pain defines pain as “an unpleasant sensory and emotional experience associated with, or resembling that associated with, actual or potential tissue damage” (33). On one hand, pain is a universal “alert sign,” considered to be an instinct to prevent chronic and repetitive injuries. On the other hand, pain is a subjective, personal, and multifaceted construction that may be modulated by various biological, psychological, and social (cultural and contextual) factors. It may be provoked by physical, emotional, or social triggers (e.g., evoked by the observation of the suffering of others; 34). Pain regulation is essential for successful adaptation to life events, development of social interactions skills and empathy (35, 36) and has been implicated in social difficulties in autism (37).
The physiological mechanisms and both peripheral and central pathways of pain processing are well documented. Following activation of specific nociceptors, pain signals are transmitted via the peripheral nervous system (PNS) to the central nervous system (CNS), composed of the spinal cord and the brain. The CNS is responsible for processing, integrating, and interpreting the information sent from the PNS, ultimately elaborating into a complex sensory experience (Supplementary Table 1). The subcortical and cortical centers in the brain coordinate all the motor responses produced to diminish or avoid painful input. Both thresholds and intensity of pain perception may be modulated by internal mechanisms. Endogenous pain modulation includes the “endogenous analgesia” which refers to the pain-inhibiting pathway originating in the brainstem and terminating in the spinal cord. Another mechanism, “descending pain modulation system,” consists of a large network in the brain which regulates pain sensory input to the CNS and behavioral reactivity. The internal mechanisms can change the meaning of pain based in previous exposure and pain expectancy, its influence on our emotional state, and its relevance to our survival. However, these processes may be affected by mood, neurological disorders, environmental and genetic factors (36, 38, 39).
Whatever the medical condition or the noxious input, individuals can use a large number of cognitive or behavioral strategies for coping with pain. These reactions include cognitive self-instruction; visual imagery and distraction; body relaxation training; seeking for social support or, alternatively, withdrawing from social contact; worrying or even catastrophizing; each of which can either decrease or increase pain sensation (40). Therefore, pain perception and pain responses form dynamic feedback loops (41–43). Interruptions in this “pain cycle” (Figure 1) may cause long-term plasticity in pain perception and regulation mechanisms which can be responsible for increased or decreased pain sensitivity, or even allodynia (44).
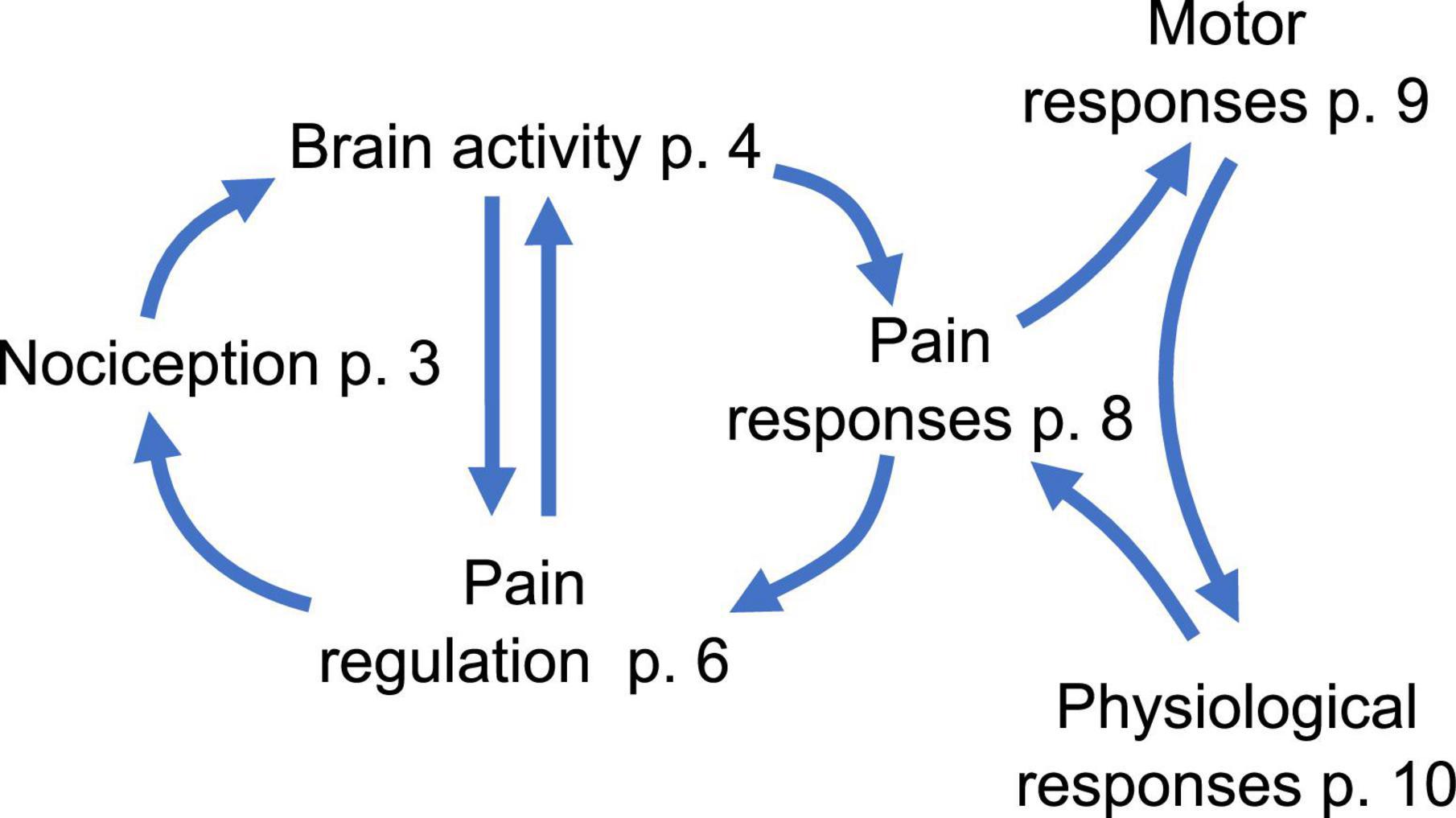
Figure 1. The simplified view of the cycle of pain (for illustrative purposes) with the numbers of corresponding pages of the paper.
When one considers the perspectives of pain research in ASD, one may ask what would be the origins of altered pain sensitivity and reactivity in ASD? In other words, where and when did the circle of pain start to go wrong, and for whom? Another important question, what should be done to overcome that issue?
To address this, we will review in the second section, the recent literature on pain processing and regulation in individuals with ASD, from initial stages to brain response in the “pain matrix.” We will propose that altered cognitive and emotional control of pain may also contribute to the altered pain response in ASD, in line with the chronic pain disorders hypothesis (42).
In the third section, pain responses in individuals with ASD and clinical methods for pain assessment (self-reports and reports by others, physiological measures, body responses) will be synthesized, highlighting the need for objective and multimodal approaches. The significance of an individualized approach for pain assessment and management will be highlighted.
Thereafter, the altered pathophysiological pathways and brain circuits involved in the emergence of ASD will be reviewed and discussed with regard to their possible involvement in the pain regulation. We will conclude by proposing an integrative view of the pain cycle, which, along with existing mechanisms of pain alterations in ASD, defines key targets for further pain research in ASD.
Introduction Highlights. Individuals with ASD demonstrate a wide range of alterations in pain reactivity. However, the question of pain perception in autism is understudied. Processing of pain includes several stages with feed-back and feed-forward interactions, forming “a pain modulation circle.” Reactivity to and coping with pain in autism may be altered because of various interruptions of this cycle; these can be configured differently in each individual with ASD.
Neurophysiological Processing of Pain in Autism Spectrum Disorder
Initial Stages of Pain Signaling: From Nociceptors to the Central Axis
While pain perception arises from the combination of different somatosensory modalities (45), processing of a painful stimulus starts from nociceptors (Supplementary Table 1), which are specialized for perception of painful stimuli of different modalities, detecting potential thermal, mechanical, and chemical tissue damage. The signal is transmitted to the CNS via more rapid, myelinated Aδ (A-delta) fiber axons responsible for acute pain transmission and slow, non-myelinated C-fibers (small fibers). The combination of these signals creates two phases of pain perception; fast and intense, followed by diffuse and dull pain (36). In individuals with ASD, atypical small fiber density was recently described (46, 47). These alterations were associated with autistic symptoms, and may also contribute to hypoesthesia (already explained above) and allodynia in autism (47). However, increased pain and touch sensitivity were observed specifically in areas innervated by C-fibers, providing a candidate mechanism for atypical avoiding behaviors or hypersensitivity in ASD. Another hypothesis could be a pathological activation of silent nociceptors, which are normally unresponsive to noxious stimuli, but become responsive to mechanical stimulation (30).
The initial stages of noxious signaling are usually characterized by the so-called nociceptive “thresholds,” i.e., the lowest intensity at which noxious stimuli are perceived to be painful. The thresholds may be different depending on whether noxious stimuli are thermal, mechanical, or due to compression. Studies in individuals with ASD have reported contradictory findings on pain thresholds depending of the type of stimuli (24, 25, 29, 31, 32, 48). No difference between neurotypical subjects and individuals with ASD was reported for electrocutaneous pain (48) and thermal pain (14, 49) thresholds. Lower thresholds (i.e., higher sensitivity) were observed for pressure-induced pain (29, 30) and heat-induced pain (31). By contrast, subjects with ASD displayed hyposensitivity to pinprick-induced mechanical pain (32).
In addition to these inconsistent results, individuals with ASD may display hypersensitivity to stimuli usually considered painless. These responses may be accompanied by absence of reactivity to potentially hazardous and noxious stimuli (1). Such stimulus overselectivity, when an individual responds only to a limited category/amount of incoming sensory information, was not confirmed on group level, however, (50). Furthermore, sensory processing patterns are known to change with time, depending on the context in which they are estimated (51). Both hyper- and hyposensitivity to the same type of stimuli may exist in the same person with ASD, challenging the suggestion that only the PNS is implicated in the observed alterations (52). In addition, the results of such psychophysical evaluation of pain detection and discrimination may be confounded by other factors like attentional resources, levels of anxiety or task performance capacities which may be different in autism.
At the level of the spinal cord, complex interactions between excitatory and inhibitory interneurons have been shown to modulate pain signal transduction. According to the “gate control” theory (53), concurrent activation of large sensory afferents from the skin (Aβ-fibers) could suppress transmission in small unmyelinated C-fiber afferents and therefore block pain perception. As a consequence, tactile stimulation of a painful area may relieve pain (54).
The relation between self-stimulation and self-injuring behavior and response to pain in autism has been discussed (20). A study measured SIBs and pain signs in non-verbal individuals with ASD (55) revealed increased behavioral signs of pain in adults with chronic self-injury. That suggests that the SIBs might be a coping strategy to manage chronic pain. Children with ASD showed a significantly reduced pain sensitivity and increased tactile sensitivity after somatosensory- directed therapeutic manipulations (with touch, proprioception, vibration, and stereognosis; 56); this effect was not find in the control group. The authors suggested that repetitive somatosensory distraction, by an increase of affective-motivational input affects pain processing and alleviates pain sensation, in line with findings in various chronic pain conditions (57–59). Although the effects of these therapeutic manipulations may be ascribed to central pain processing and top-down regulation, normalization and integrity of peripheral sensory perception likely also contributes. However, the causal relations between SIB and altered pain perception in ASD is far from understood.
Initial stages of neurophysiological processing of pain in ASD highlights. The evidence for alterations in pain transduction at the level of peripheral receptors in ASD is limited to reports of decreased nociceptor density. That question needs to be more robustly corroborated, given that pain thresholds in individuals with ASD and the control groups weren’t different in most studies. However, peripheral nerve alterations may partly explain sensory dysfunctions like allodynia – painful atypical response to touch, and hypoesthesia – hyposensitivity to injurious stimuli, but also paradoxical heat sensation documented in the battery of sensory tests in autism.
Atypical Brain Activity for Pain Processing in Autism Spectrum Disorder
The Pain Matrix: First Level of Response “The Nociceptive Matrix” (Thalamus, Somatosensory Cortex)
The Thalamus
The thalamus, a regulatory hub for sensory inputs from different modalities, is involved in pain processing (60). It can be reorganized following chronic pain, altering maps of noxious and innocuous stimulation and leading to the perception of innocuous stimuli as nociceptive (61). In individuals with ASD, both structural and functional deviations in the thalamus have been found (62–66; Figure 2). The available studies on the resting state or anatomical connectivity yielded heterogeneous results. Both hypo-(64, 67) and hyperconnectivity (65, 68) between thalamus and cortical areas were demonstrated in ASD. During an aversive sensory stimulation, thalamocortical connectivity was attenuated, while connectivity between thalamus and subcortical areas (putamen, hippocampus, and amygdala) was, in contrast, enhanced in individuals with ASD when compared to controls (69). This hyper-connectivity in response to aversive stimuli could indicate a lack of cortical inhibition. Additionally, connectivity between thalamus and amygdala is thought to play a role in directing attention to emotionally salient information. This suggests that modified thalamus connectivity may be responsible for over-attribution of “pain” salience to benign tactile stimuli (allodynia).
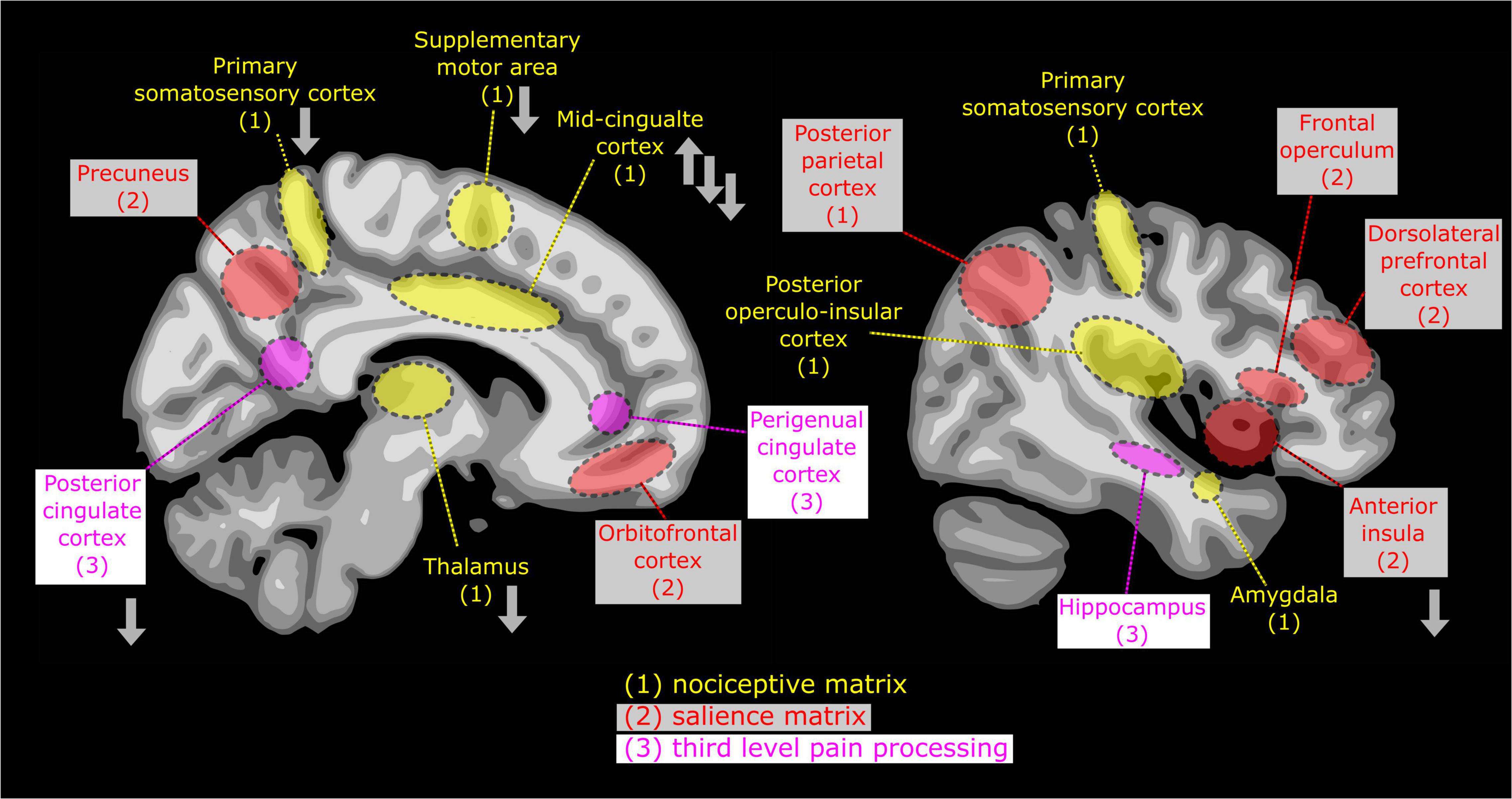
Figure 2. Basic view of ascending pain processing, delineated on two brain sections, with emphasis on early, sub-conscious (1, “nociceptive matrix”: the posterior operculo-insular cortex, the primary sensory areas, p-mid-cingulate cortex, supplementary motor area and the amygdala) and conscious pain perception (2, “salience matrix”: the anterior cingulate cortex, the anterior insula, posterior parietal, prefrontal and orbitofrontal cortices), and (3, “areas of third-order brain activation”: the hippocampus and the anterior and posterior cingulate). Nociceptive cortical processing is initiated in parallel in sensory, motor and limbic areas. Some activation may last longer than voluntary motor reaction. Based on brain response dynamics and models described in Bushnell et al. (42), Garcia-Larrea and Bastuji (70). The alterations in pain-induced responses in the brain of individuals with ASD are shown with gray arrows according to Failla et al. (62), Chien et al. (71), and Gu et al. (72).
In a recent study investigating different stages of pain-induced brain responses, Failla et al. (62) showed that processing of noxious stimuli in the brain differs between subjects with ASD and neurotypicals, with regard to the duration and type of pain. In this study, adults with ASD showed significantly less brain activation in the thalamus compared with controls during sustained pain stimulation (62). In contrast, acute brain responses to pain were similar across groups. That reorganization of thalamocortical and thalamosubcortical pain-processing pathways may be responsible for reduced pain awareness due to attenuated thalamocortical signaling. In addition, increased unspecified distress due to upregulated signaling between thalamus and sub-cortical structures noxious may result in possible perception of neutral stimuli as in ASD.
The Somatosensory Cortex
The functional and structural modifications in one of the earliest brain areas activated by noxious stimuli, the primary somatosensory cortex, S1 have been detected in subjects with ASD (73, 74) along with altered sensitivity profiles (32, 75). In addition, reduced gray matter volume in somatosensory brain areas and possible disruptions in thalamocortical white matter fiber pathways were associated with higher SIB scores in autism (76). In the above-mentioned study (62) sustained painful stimulation (heat stimuli administered to a right lateral calf), was associated with significantly less activation in the left S1 and bilateral secondary somatosensory cortices S2 in the group of participants with ASD than in their neurotypical peers. Again, brain activation during the initial stage of stimulation was not different across these groups.
The Pain Matrix: Salient Encoding of Pain Stimuli “The Salience Matrix” (The Cingulate Cortex, Prefrontral Cortex and Insula), and Their Interactions With Third Order Areas
Activation of “salience matrix” regions is not specific to painful stimulation. These circuits may be activated by any sensory stimulus that rises above the sensory threshold. Such co-activation adds the “salience” meaning of noxious stimuli and triggers attentional control of pain-evoked response (70). The coordinated response within the sensory-specific and second-order networks (Supplementary Table 1 and Figure 2) is crucial to create links between pre-conscious nociception and conscious pain (36, 43).
The cingulate cortex: Besides its role in the attentional component of pain stimulus processing (Supplementary Table 1), the cingulate cortex plays a role in the brain response related to negative affect and goal-directed behavior, resulting in “urgency to act” (77). The alterations in cingulate cortex function have already been documented in ASD (78, 79). Decreased engagement of the anterior cingulate cortex in participants with autism was also observed in the above-mentioned study of sustained pain responses (62). In addition, during a heat-induced pain, both amplitudes and latencies of evoked potentials in the cingulate gyrus were found to be lower in adults with ASD compared to controls, while pain scores were not significantly different between groups (71). Such results suggest that the cingulate cortex seems to be less implicated in general, but may have a specific role at the latest stages of pain processing in ASD, contributing to the motor hyporesponsiveness of individuals with autism.
The insula is involved both in the initial perceptual stage and in the cognitive encoding of pain (80). Structural and functional changes in the insula, as well as alterations in connectivity, were observed in autism, as reviewed in Caria and de Falco (81), Uddin and Menon (82). Decreased insula activation in participants with autism was also observed during sustained pain (62). The insula is considered as a hub for interoception, i.e., representation of our own internal word, (83, 84); self-awareness and bodily self-consciousness (85); which have generally been reported as atypical in population with ASD (86–92). Disrupted engagement of the insula during pain processing may be responsible for altered pain awareness and/or cognitive pain representation (49).
The (pre-)frontal cortex participates in top-down cognitive and anti-nociceptive short- and long-term controls over pain perception and reaction (93, 94). Less prefrontal cortex engagement may be related to reduced antinociceptive feedback (Supplementary Table 1 and Figure 2). Even if pain-related prefrontal cortex activation does not seem to differ between individuals with and without ASD (62), it is negatively correlated with levels of perceived pain in participants with ASD. Another recent results reported a reduced prefrontal cortex response to painful stimulation in participants with ASD (95).
The reciprocal connections between prefrontal cortex, hippocampus, and amygdala are known to be implicated in different stages of pain processing (96). It has been reported that noxious stimuli modulate prefrontal-hippocampal connectivity and impact behavioral performance (97). Chronic pain causes neuroplastic changes in these regions of the brain, resulting in aversive states and memory deficits (98, 99). In ASD, structural and functional alterations have been observed in the hippocampus-prefrontal cortex-amygdala network (100–102). The interruption of maturation of these brain areas caused by chronic pain in early life has been proposed as a predisposing factor for ASD (103). Taking into account recent theories about reorganization of pain processing pathways during the transition from acute to chronic pain proposed by De Ridder et al. (104), one may speculate that such a reorganization during perinatal development could explain alterations observed in ASD. Further research on this topic may provide a more unified account of how sensory and social peculiarities impact together the perception and processing of pain in subjects with ASD (37).
Default mode network (DMN) may also be related to pain processing in autism. According to recent models (104), chronic pain results in reorganization of pain networks in the brain and also implicates the DMN (the medial prefrontal cortex, posterior cingulate cortex, inferior parietal cortex and the precuneus; 105–107). That leads to integration of pain into body schema and changes in its appraisal. Little is known about DMN activity during pain processing in subjects with autism, but numerous alterations of DMN activity and in its connections with other brain areas have been described in subjects with ASD (108).
By referring to the model of chronic pain, we may suggest reorganization of thalamocortical and thalamosubcortical pain-processing pathways in ASD. That plasticity could be partly responsible for reduced pain awareness due to attenuated thalamocortical signaling. Second, reduced activation of the second-order brain areas may lead to deviant pain consciousness and hypo- or delayed activation of structures implicated in goal directed behaviors and motor responses. Finally, increased unspecified distress due to upregulated signaling between thalamus and sub-cortical structures, might lead to perception of neutral stimuli as nociceptive.
The “pain matrix” in ASD highlights. Results of neuroimaging studies of pain processing in ASD are limited but suggest atypical brain activation patterns. Decreased activation in most of the areas of the pain matrix, starting from earliest cortical and sub cortical structures, during sustained pain stimulation in contrast to acute pain, supports the hypothesis of “feedback default” or “atypical top-down pain regulation” in ASD. Possible re-organization of pain processing pathways in early stages of development in ASD may be responsible for such alterations. Taken together, available information on central pain processing in ASD may explain the paradoxical dissociation between (near to-) intact low-level perceptive abilities in pain detection tasks and altered salience and awareness of pain and its affective components.
Pain Perception Modulation in Autism Spectrum Disorder
The Descending Pain Control Pathways
The descending pain modulatory system involves connections between the (pre-)frontal cortex, the thalamus, the periaqueductal gray (PAG) and the amygdala (Supplementary Table 1 and Figure 2). Among these brain structures, the amygdala appears as a key component of top-down regulation. The amygdala allocates attention and assigns emotional value – either positive or negative – to sensory information, thus leading to adaptive behavioral and affective responses and also contributing to emotional memory. Under short-term aversive conditions, such as stress or fear, amygdala activation induces hypoalgesia. Contrary to this, during chronic pain caused by various medical conditions, long-lasting functional plasticity of amygdala activity is related to enhanced nociceptive responses, including hyperalgesia, aversive behavioral reactions and anxiety-like states (109, 110). The amygdala has received considerable attention in ASD studies (111). For example, the amygdala in individuals with ASD show both microscopic (112) and macroscopic structural regional abnormalities. Recently, in a large sample of 1,571 participants with ASD, increased thickness in the frontal cortex and reduced subcortical amygdala volumes were reported (113) as well as connectivity atypicalities (114). In line with the so-called “weak amygdala’s emotional modulation hypothesis in ASD” (115), such deviant regulation may yield atypical behavioral and social/psychological responses (116). Reduced connectivity between the prefrontal cortex and the amygdala during unpleasant stimulus processing was recently observed in children with ASD (117). That suggests that alterations in the interconnectivity of these structures may play a role in the blunted behavioral responses to pain in autism. Importantly, the amygdala is activated by pain as early as the first-order cortical areas (118). Thus if prefrontal networks are unable to exert inhibitory modulation, it may remain over-activated, producing a cascade of autonomic and behavioral reactions.
The descending pain modulation pathways can be both facilitatory as well as inhibitory. Facilitatory pathways are the ones which enhance pain perception, while inhibitory pathways suppress pain perception. Endogeneous opioids are released at synapses at multiple points in the PNS to block the ascending pain transmission signal. Moreover, during chronic pain, these pathways are plastic, resulting in pain sensitization or other perceptual alterations such as allodynia or nocebo (119). Alterations of the balance between descending controls, both excitatory and inhibitory, are involved in some dysfunctional and chronic pain states such as fibromyalgia (120–122) that has been associated with higher rate of autistic traits (123).
Of the endogenous pain modulatory mechanisms, the Diffuse Noxious Inhibitory Control (DNIC), is essential and often been described as “pain inhibits pain.” That occurs when response to a painful stimulus is inhibited by another, often spatially distant, noxious stimulus. The DNIC is predominantly studied in humans using the psychophysical paradigm of Conditioned Pain Modulation (CPM; 124, 125). The most commonly investigated test stimuli are pressure pain threshold (PPT) and cold water immersion is the most frequently studied conditioning stimulus (126). In ASD, recent results suggested a preserved CPM effect. The measures of PPTs increase significantly after a cold conditioning stimulus in both adults with and without ASD (32, 127). Nevertheless, as the pain scores were very variable in individuals with ASD, with a greater range of extreme scores than in control group, results must be interpreted with caution (127).
Anticipation of Painful Stimuli
Brain activity patterns accompanying pain anticipation and processing were studied in high functioning adults with ASD and matched neurotypical individuals (72). Stronger activation in the anterior cingulate cortex was observed during the anticipatory phase in the group of participants with autism, while brain responses during painful stimulation were not different from neurotypical peers, in line with (62). The increased anticipatory brain response was paralleled by augmented behavioral sensitivity to anticipated (expected) pain in individuals with autism. This may seem to be contrary to previous observations of decreased response to sustained pain stimuli. This may be explained by the fact that in Gu et al. (72), contrary to Failla et al. (62) a cued paradigm was used to force participants to attend to incoming noxious stimuli and concentrate on the sensory aspects of stimulation. It should be noted that in this study, participants with ASD pre-selected a lower level of painful stimulation than healthy individuals, consistent with their hypothesized nociceptive hypersensitivity. The idea being developed here is that one may explain one of the paradoxes of pain perception in autism: expected pain perception is enhanced due to stronger attentional engagement.
Pain Resonance and Empathy
A growing body of evidence suggests that the ability to understand and feel compassion for another’s pain, so called “empathy for pain” (128) is underpinned by the same neural structures that are involved in the direct experience of pain. Observing someone experiencing pain induces brain response in the insula, anterior cingulate cortex, the brainstem and the cerebellum, but also emotional and physiological responses similar to those caused by a direct experience of pain (129–131). Empathy for pain is hypothesized to be based on the subject’s own experience of pain. It may be related to vicarious pain perception, the sensation of pain on one’s own body while observing the pain of others (132). Results from studies of vicarious pain perception and empathy for pain in ASD are contradictory. Some studies reported no difference from typical peers in behavioral and neuronal signatures of empathy for pain, while others suggest atypical brain activations and reactions in ASD (29, 109, 133–135).
Observation of someone’s injured hand vs. non-injured hand (from a first-person perspective) induced strong and consistent activations of cingulate, somatosensory and insular cortices both in participants in control and ASD groups (109). These responses are accompanied by the same level of pupil dilation, an estimator of physiological arousal. Similarly, no group difference was found in the activation of the insula during a task based on painful stimulation applied to both the other’s hand and one’s own (48). Similar results were obtained from the observation of facial expressions of others experiencing painful stimuli (134, 135); both neurotypical subjects and participants with ASD exhibited activation in regions involved in pain processing (the insula, somatosensory cortex, supplementary motor area, periaqueductal gray, and prefrontal cortex), in affective reaction (orbito-frontal cortex, the amygdala) and in face and body part processing (fusiform face area, extrastriate body areas). These results would suggest that typical brain processes involved in shared representations of pain or emotional contagion are intact in autism.
In contrast, other results revealed atypical brain activations and physiological and behavioral responses to vicarious pain in ASD. In the same study cited above (135), videos of someone’s limbs in painful vs. non-painful situations caused less activation in the inferior frontal gyrus, the precentral gyrus, the frontal orbital cortex and the medial prefrontal cortex in adults with ASD compared to neurotypical controls. As these regions are known as important parts of motor resonance and empathy for pain network (136) their hyporesponsiveness may contribute to emotional resonance alterations in autism.
In the study of Gu et al. (133), subjects observe images of someone’s hands or feet (from first-person perspective) in painful or non-painful situations. In that study subjects with autism were less able to discern pain in others, but demonstrated higher activation in the anterior insula and extrastriatal body areas, and lower activation of right prefrontal cortex compared to controls. In addition, participants with ASD had augmented empathetic pain-related skin conductance response. That response correlated with brain activation evoked by images, suggesting higher level of physiological arousal and sympathetic nervous system engagement, compared to control participants.
In general, empathic responses are described as non-typical in ASD (137). Although general empathy and empathy for pain are distinct, they may share common neurological and physiological mechanisms. It has been reported that in subjects with ASD and their neurotypical peers, lower empathy is correlated with greater anterior cingulate cortex and insula activation in a pain anticipation task (72). Consistent with this, empathy scores are negatively correlated with insula activation only in participants with ASD during vicarious pain perception (133). In addition to autonomic hyperarousal evoked by these stimuli, it is plausible that alterations in the regulation loop between central and peripheral responses during subjective and vicarious pain experiences may be related to empathy modulation in ASD. It is also possible that difficulties in recognizing and reporting on one’s own (and others’) emotions, referred to as alexithymia, may be more responsible for observed alterations in empathy than core autistic features themselves (48, 110). For example, it has been shown that higher levels of alexithymia is related to increased arousal and diminished habituation to negative emotional stimuli (138). However, this conjecture remains unresolved (139).
The role of embodiment, e.g., the integration of sensations from one’s own bodily experience (in the present as well as in the past) in the construction of conscious perception in order to understand our own experience, and the experiences of others (140) in ASD is discussed (92). Embodied cognition is based on the same neurophysiological mechanisms which are activated during observation of stimulation applied to others as during perception by self (141–143). When participants observed someone’s body parts being injured, participants with ASD had increased activation in the somatosensory cortex (S1/S2) and decreased activation in the medial prefrontal cortex comparatively to controls (29). In addition, only participants with ASD demonstrated that S1/S2 overactivation evoked by vicarious pain was associated with lower pain pressure thresholds (e.g., higher pain sensitivity) and, paradoxically, to reduced unpleasantness rating scores. These results suggest that atypical coupling between embodiment and atypical sensory processing influences perception of vicarious pain in ASD. Consistent with these findings, reduced embodiment reaction in response to emotional stimuli is demonstrated in autism (144). Interestingly, production of an empathic response to other’s pain requires directed attention from individuals with ASD (145), which is attenuated when the individual is distracted.
The attentional demands required to detect others’ pain may partially explain discrepancies in insular cortex responses in subjects with ASD: overactivation of the insula demonstrated in Gu et al. (133) or inhibition of its activity shown in Fan et al. (29). While in the former study the images of different type (pain/no pain) were counterbalanced and randomized, Fan et al. presented stimuli in blocks of consecutive events, creating the possibility of priming the subject to the valence (pain/no pain) of the upcoming stimulus. This latter observation may be congruent with lower insula activation for sustained pain stimulation observed in Failla et al. (62). Taking into account the integrative role of the insula in the perception of the self, these observations may demonstrate alterations in upper-level integrative processes in ASD during vicarious pain perception.
In summary, results from the limited number of studies on brain reactivity to vicarious pain in ASD need to be replicated to clarify the patterns of activation of each area of the pain matrix, compared to typical activation. Previous discrepancies concerning somatosensory or insula activities during empathy for pain may arise from differences in experimental paradigms, as already described in similar studies of neurotypical development (146). Nonetheless, these studies identified potential specific alterations in brain activity, similar to those reported in studies of direct pain stimulation, especially in the (pre-)frontal brain areas. This suggests a possibility of shared origins between atypical responses to pain stimuli and difficulties in empathy (at least for pain) in ASD. According to embodiment theories, altered perception of pain in oneself may be related to anomalous reactions to signs of pain, and may be related to other socio-cognitive deviations in ASD, such as attenuated distinction between positive vs. negative emotions (124).
Social Touch and Down-Regulation of Pain in Autism
The social component of pain modulation must not be underestimated in the global processing of pain, particularly in ASD. The observation of someone’s pain induces a need for action in observers, an urge to provide help or reassure the person in distress. One of the most common ways to respond to someone’s pain, to reassure and calm, is through the so called “social,” or “soft,” “interpersonal,” or “affective” touch, a specific type of tactile stimulation provided by others, promoting pleasant feelings, approach-related behaviors and reductions in pain (125). It has been reported that brain correlates of soft touch-induced analgesia, included modification of pain-related activation in “nociceptive” brain areas as well as secondary (“salience”) and third-order pain perception areas, and in sub-cortical regions such as amygdala, hypothalamus, and PAG (125). Affective touch signals are conveyed to the brain via the specific variety of type C nerve fibers, so called C-tactile, CT fibers (127). CT fibers stimulation activates the insula, but not somatosensory areas S1 and S2 and underlies positive affective aspects of touch (147). However, these types of interactions are often reported as stressful by people with ASD, provoking unpleasant feelings or touch-aversion behavior (148).
Recent studies demonstrated that brain correlates of social touch are modified in ASD (149–151). These alterations in brain activation were correlated with the autism severity (150). Moreover, while activation of the insula and other socio–emotional brain regions were reduced, social touch evoked atypical hyper-reactivity in primary somatosensory cortex (151), consistent with increased tactile sensitivity in areas innervated by CT-fibers (30). This may explain the unpleasantness of social touch and interruption of the mechanism of soft touch-induced analgesia in autism.
Social touch promotes communication through oxytocin-dependent mechanisms (152). Oxytocin, a neuropeptide receiving increased attention because of its prosocial effects, has potential in social interactions improvement in autism (153, 154). The regulatory role of oxytocin on pain processing may be found on various levels from peripheral antinociception to high-level emotional pain, pain anticipation and pain memory (155). Some studies demonstrated that oxytocin treatment potentiated social brain connectivity and behavioral improvements in subjects with ASD (156, 157) and reduced heat pain intensity ratings and amygdala activation during painful stimulation (158, 159). This suggests the therapeutic potential of oxytocin in modulation of pain down-regulation by others in autism.
Formation of adequate top-down regulation of pain requires conscious appraisal of it emerging from coordinated co-activation of numerous brain areas. Decoupling between first and second order brain areas of the pain matrix (for example, under anesthesia) leads to nociceptive matrix-elicited physiological and hormonal responses (160, 161), but absence of down-regulation from upper brain areas (162). Such blunted reactions may have long-term consequences such as anxiety, pain sensitization, depression, or post-traumatic stress disorder (70, 163). In ASD, an increase in SIB (21, 28) or other-injurious (164) behavior after painful procedures, along with increased physiological and hormonal responses (165) could be considered consequences of such dysregulation.
To conclude, a body of evidence suggests atypical neuronal processing of pain in ASD. Although some recent results indicate possible alterations in nociceptors (46) or suggest possible excitatory/inhibitory imbalance in the spinal cord level (5), most of the findings focus on atypical brain processing. Together with evidence of hypoactivation in areas of the second and third order brain areas, more consistently in (pre-)frontal cortex, scientific findings support the hypothesis of a “feed-back defect” or “altered top-down regulation” in pain processing, in ASD (62). In addition, the crucial role of attentional engagement in resulting pain-evoked response may be proposed. In many studies these alterations correlated with participants’ assessment of direct pain, linking central mechanism dysfunction with atypical expression of pain in ASD. This section emphasizes the linkages between deviations in social regulation and pain perception in ASD.
Pain perception modulation in ASD highlights. Pain processing in the brain relies on coordinated activation of multiple brain areas. Reorganization of that response may decrease or increase levels of perceived pain. Affective and cognitive aspects of pain perception, as well as in vicarious pain experience and down-regulation of pain response by social touch seem to be modified in people with ASD. Observed alterations should be taken into account when considering the role of social reciprocity in pain regulation in autism.
Atypical Pain Reactions in Autism Spectrum Disorder
Emotional expressions (bodily, verbal, and facial) have an important social function in communicating one’s own feelings to others to receive adequate responses. Pain is a complex socio-communicative experience which includes biological, physiological and cognitive but also social determinants. Expressions of pain can be enhanced or suppressed by the presence of others and social context, as well as by the personal history of reassurance and support, in reference to the “social communication model of pain” (166). Displays of pain command the attention of observers and provoke reflexive behaviors that facilitate down−regulation by various mechanisms of distraction, reassurance or social referencing (36). Alternatively, individuals with autism may mask or camouflage their feelings (167), which may be associated with adverse mental health outcomes. Alterations of any of the top-down modulation steps including clear pain communication (Figure 1) may lead to aggravation of painful experience (168).
Different levels of pain communication exist. They include more or less volitionally regulated facial and body expressions, vocalizations, withdrawal from the source of the pain and also visible physiological signs of distress, like increased pupil diameter, sweating, pale skin, increased heart and respiratory rate, mediated by specific changes in hormonal and neurochemical responses (36). It is still debated (24, 27) whether atypical patterns of pain expression in ASD are mostly attributed to alterations in pain processing and regulation itself, or in the motor response realization, as alterations in motor skills are frequent in autism (169).
Challenges of pain assessment in ASD. Historically, hypo-responsiveness to painful stimuli was included as one of the symptoms of ASD as “apparent indifference to pain” (19), also largely reported by clinicians and relatives of the patient (22, 25, 170). During evaluation of every-day pain reactivity, among several types of behaviors rated as “very often occurred” after a painful incident in people with ASD, some were similar to individuals without ASD (“seeking comfort,” “crying”) and some were atypical (“being difficult to distract,” “jumping around,” “agitated,” and “fidgety”; 171). However, despite the higher incidence of pain-evoking accidents (172), missing, delayed or aberrant reactions to painful stimuli are often reported in daily observation of subjects with ASD.
Observer-rated pain assessment may provide a long-term profile, and thus is well-suited for chronic pain conditions. Various scales have been specifically adapted for pain assessment in populations with communication and cognitive alterations, as a function of the age and global mental functioning of the person. We can cite the short-form McGill Pain Questionnaire, SF-MPQ (49, 173); the Non-communicating Children’s Pain Checklist, NCCPC-R (174, 175), the Pre-Linguistic Behavioral Pain Reactivity Scale, PL-BPRS (21, 165); the Faces, Legs, Activity, Cry and Consolability – Revised (FLACC-R) or the Faces Pain Scale-Revised and a Numeric Rating Scale (176). These scales are focused on behavioral signs, extracted from body language and facial expression, in particular on visible signs of agitation, stress and discomfort. Importantly, in ASD many signs of emotions including stress, anxiety, discomfort, but also pain are considered as “idiosyncratic.” Thus these methods of pain evaluation in individuals with ASD, who are known to express atypical responses, should be applied with caution. Parents and observers must be familiar with the “common behavior” of the person, in order to catch the new “signal” and to recognize it as the response to pain (24, 26, 177).
Self-assessment of pain gives direct, but subjective information and requires familiarization with the scales. Tools that obtain quantified self-reports of pain intensity usually allow the individual to state a number or point to a face that correlates with a number, such as the Wong-Baker Faces Pain Rating Scale (178) or the Pain-O-Meter, a plastic tool with a moveable marker (179). Self-evaluation could be difficult in an acute pain situation, even more so if the person has intellectual and conceptual difficulties and cannot follow instructions for self-reporting or has limited understanding of concepts necessary for tool utilization. Ely et al. promoted an alternate interactive approach to pain assessment in ASD based on individualized consideration and estimation of pain assessment methods. Utilizing the help and knowledge of parents appeared to be essential in identifying the presence of pain and accurately estimating its intensity (177). More research must be completed to advance knowledge and practice for assessing the pain of the individual with ASD. Researchers developing new tools should recruit parents for assistance.
Global motor response. Based on the use of these various scales, some studies have reported a reduced behavioral reactivity to pain in children with autism, whether at home (parental assessment), in institutions or during a venipuncture (professional assessment; 165, 180, 181), but hyper-reactivity was also suggested in similar experimental conditions as well as in other clinical settings, such as during dental cleaning (182, 183). In addition to behavioral observations with traditional scales, delayed or aberrant reactions to painful stimuli are often reported in daily observations by parents and clinical reports (171). These are: lack of protective body position or withdrawal reactions (7); a wide range of deviant pain-evoked responses like: hyperactivity, paradoxical laughs, aggressiveness, stereotyped behaviors and SIB (20, 22, 165, 184); a general expression of discomfort without localization of the source of pain, as well as other variations (171).
Very few tools to capture atypical signs that are not listed in traditional scales have been tested or adapted for individuals with autism. Only one adaptative scale: the “Simplified Pain Evaluation Scale for Dyscommunicative Autism Spectrum Disorders: ESDDA” has been proposed, but it currently lacks psychometric validation (185). The development of reliable and validated assessment tools remains challenging, because of the high heterogeneity of ASD clinical features but also because pain is defined as a highly subjective experience that varies among individuals in general.
Facial expression of pain in ASD. Facial expressions of pain are a crucial component of social signaling (186–188), even in non-communicative or unconscious patients (189).
Facial emotion recognition and the ability to convey emotion through facial expression has been a topic of interest in autism research for more than three decades. In people with ASD, facial expressions are often perceived as awkward or atypical for a review, see (190). The idea that people with ASD spontaneously express less frequent and shorter emotional expressions and of lower quality (less accurate and intense) than their neurotypical peers is widely accepted (191, 192), described as “amimia” in clinical reports (191). Many screening and diagnostic tools for ASD include the items: “range of facial expression” or “inappropriate facial expressions,” as one of the early signs of ASD (e.g., the Social Communication Questionnaire, Modified Checklist for Autism in Toddlers, Childhood Autism Rating Scale, Autism Diagnostic Interview).
Studies examining pain expression in autism have generally documented mixed results that have failed to provide a consensus. For example, during a venipuncture, some authors reported more facial expressions in children with ASD than in their neurotypical peers (175) whereas no differences were identified in another sample (193). To note, the existing methodologies based on manual scoring of facial expression are strongly dependent on the abilities of the observer (194–196). To minimize the impact of subjective bias and context, several studies were designed to explore facial expressions accompanying pain through standardized conditions and tools and to compare observational rating scores with physiological markers of pain perception during routine clinical procedures, such as venipuncture (165, 175, 193, 195) or dental procedures (183).
Nader et al. (175) has analyzed facial expressions in children using the most frequently used tool in traditional research on facial emotion expressions: the Child Facial Action Coding System for children (FACS) during a venipuncture. The study demonstrated that participants with ASD had greater facial response during the needle insertion phase than the children in the control group. However, other results indicated a lack of concordance between the observer and parental scores of pain evaluations (193). In addition to this, facial reactivity was compared with the motor and physiological responses (heart rate) in children with ASD, children with developmental delay and neurotypical controls.
Only children with ASD demonstrated elevated facial and motor reactivity after the end of the venipuncture, and authors indicated that the age-dependent decrement of pain-induced facial reactions was not observed in this group (193). To avoid observer-related subjectivity, automated facial emotion recognition technologies based on FACS were developed and tested in experimental settings (197–205), however, they need to be adapted to individual features of a person with ASD. To our knowledge, currently no experimental design has been proposed to test and refine algorithmic detection of pain in individuals with ASD.
Physiological correlates of pain perception in ASD. The experience of pain evokes numerous physiological responses driven by the sympathetic and parasympathetic nervous system (206–208). The acute experience of pain causes an increase in heart and respiratory rate, skin conductance response, blood pressure, and pupil diameter, all features of sympathetic nervous system activation. Painful stimuli also provoke heart rate variability changes, mostly an increase in low frequency vs. high frequency ratio (209), muscle tension and a decrease in skin temperature see for review (210). Most of the existing results reported similar physiological responses in individuals with and without ASD, with regard to skin conductance responses (72) and heart rate (193). Only one study indicated increased heart rate in children with ASD compared to children without ASD, after a venipuncture (165). After venipuncture, the low-functioning autism participants demonstrated increased rates of injurious behavior directed toward others (164). Importantly, prediction models based on the integration of various physiological signals (electrodermal activity and cardiovascular activity) with motor response measured by accelerometry may be applied to anticipate the behavioral outcome in non-verbal patients with autism (211), and, potentially, to prevent negative affect and pain after manipulation.
Several limitations, related to the medical manipulation itself, should be mentioned. As venipuncture or dental care include not only painful stimulation, but also different manipulations of the subject’s hand or mouth (a tourniquet placement, numerous touches to find the vein, disinfection of the skin, or mixed sensory stimulations – lights, odors…), these procedures may generate certain level of stress. Taking in mind high unpleasantness of touch or odors for some individuals with ASD and possible impact of other psychological issues (increased anxiety, for example), these stressors may obscure the response to pain. Therefore, it would be optimal to disentangle several factors of such manipulation to characterize the specific pain-induced reactions.
To conclude, more research is needed to address the broad heterogeneity of pain expression in ASD. Experimental and clinical assessment of pain expression in ASD should be addressed by a multimodal approach, combining data from self-assessed pain, as well as the assessment of pain by an observer, with an effort to adapt and validate the traditional scales. In addition, pain reactions should be assessed via the automated analysis of facial expressions and body movements, as well as skin temperature and conductance; vocalizations, and other physiological signals, to create a complete profile of pain-evoked response (212, 213). Moreover, during studies that exploit the analysis of pain-evoked responses in clinical settings, efforts should be made to minimize anxiety and distress due to novelty and anticipation of pain as it may undesirably magnify pain perception in individuals with ASD (168). Paradoxically, in a recent study (175), the children who were the most reactive to pain were those who were described as the least sensitive and reactive to pain by the parents. To explain these results, the authors propose that the expression of pain differs according to the type of pain (care-related acute pain, daily acute, or chronic pains). While participants with ASD would have atypical or reduced behaviors in an everyday situation, they would express themselves in an exaggerated way during a care situation (175). Thus, in the future, preference should be given to non-invasive or more naturalistic methods with minimum manipulations or novelty.
To achieve this goal, new technologies provide some innovative approaches (190, 211). The use of wearable devices allows to assess a range of physiological and behavioral reactions in individuals with ASD (214); however, to date, these studies were not aimed to evaluate pain-evoked responses. Neuro-feedback based strategies, which would join individual neurophysiological correlates of pain experience with simultaneously recorded expressions of pain, may provide a pathway for people with ASD to express their feelings and solicit reflexive behaviors from social partners.
Atypical pain reactions in ASD highlights. Production of an adaptive reaction to a painful event is indispensable to receive adequate social support. In individuals with ASD, reactivity to pain is often changed, leading to the absence of proper pain management and corruption of pain cycle functioning. The dissociation between central and autonomic responses to pain may be a cause of alterations of pain processing in ASD. The need for an integrative evaluation of pain responses utilizing modern real-life technologies is necessary to address the individualized needs of each patient.
Mechanisms and Perspectives
Summary of Common Mechanisms, Involved in Both Autism Spectrum Disorder and Pain Regulation
Many neurochemical pathways involved in determining ASD are also known to participate to pain processing in typical development (see; 215). The pain regulation pathways are predominantly noradrenergic and serotonergic, but implicate other neuromodulators including dopamine and opioids, as reviewed in Yam et al. (35). Here we will discuss the role of some neurotransmitters in ASD in relation to the regulation of pain.
Opioids and Gut Hormones
Endorphins are important components of endogenous pain control (35, 208, 216). Peripherally, beta-endorphins produce analgesia by binding to opioid receptors. At the level of CNS, mu-opioid receptors block pain processing and activate descending pain control circuits including the amygdala and PAG (216). The “Brain Opioid Theory of Social Attachment” (BOTSA), in which social and affiliative behaviors were proposed to depend on endogenous opioid peptide levels, has been based on similarities between social attachment and drug addiction (217). Many symptoms of ASD seems to resemble behaviors induced in animals or humans by opioid administration, including: reduced socialization, repetitive stereotypies, motor hyperactivity and especially insensitivity to pain. This has led to the neurochemical opioid hypothesis in ASD, based on evidence from animal models and human studies and from clinical trials with opioid antagonists (naloxone and naltrexone), in the early 90s. Blood and cerebrospinal fluid studies have reported that beta-endorphin levels were altered in individuals with ASD (218–222). In children with ASD, compared to neurotypical children, increased opioid levels were found after exposure to painful manipulation (venipuncture), which were correlated with autism severity (165). However, these results failed to be replicated, that may been ascribed to measures biases (165, 219–222).
After years of disinterest, the role of opioids in ASD could be updated. In light of recent views on the opioid antagonists effect (223) and findings on modifications in particular opioid receptors in subjects with ASD (224), imbalance in the opioid system may be proposed as one of the mechanisms of pain sensitization, hyperalgesia and increasing of pain vulnerability (225, 226). It has been hypothesized that chronic pain conditions in individuals with ASD could be responsible for SIB, which would have a calming effect through opioid release (24). SIB may be related both to enhanced expressions of pain (227), and to reduced pain sensitivity (228). In neurodevelopmental disorders, SIB has been proposed to represent a nociceptive coping behavior, mediated by altered nociception, allodynia or hyperalgesia and neuroinflammatory mechanisms similar to those in chronic neuropathic pain disorders (229). In a recent review of 10 clinical trials testing opioid antagonists and specific drugs in children with ASD, the authors concluded that only in a sub-group with elevated endorphin levels, naltrexone was effective in improving SIB, hyperactivity and restlessness (223).
Endogenous opioids are implicated not only in pain downregulation, but also play a role in many high-level processes like regulation of social behavior. Thus, imbalance of opioid signaling in ASD may be implicated in altered pain perception and SIB as an internal pathophysiological mechanism of opioid tone regulation, but also may contribute to social symptoms. However, the nature of the relationship between self-harm, endogenous opioids and pain processing is not clear yet.
An alternative supposition has been formulated by Jonhson et al. (230, 231), elaborating the links between opioids and ASD. The authors proposed that autism may be related to a genetic predisposition in sub-groups of individuals, triggered by administration of exogenous opioid hormone/medication at birth or during labor that interferes with the natural fetal opioid balance. However, this hypothesis hasn’t been documented in a large cohort of babies born to opioid-addicted mothers (232). To note, pre-conception opioid prescription was associated with 2.43 times more for the odds of ASD compared to mothers without prescriptions (233).
Recently, the relation between opioids and gastrointestinal disorders, which is often comorbid in autism, has been discussed (234–236). The gastrointestinal tract problems in ASD include impairments in bowel mucosa function, selective permeability, gut immune response, potentially creating a source of chronic irritation and visceral pain in these patients (237). Increased gastrointestinal tract symptoms are significantly associated with post-stress cortisol concentrations in ASD (238). Gut hormones may play an important role in such relations (239). Among them, ghrelin, a peptide hormone regulating appetitive behavior, reward, stress and anxiety response (240) is decreased in children with autism (241). Ghrelin may also be involved in pain modulation, as central ghrelin injections increased beta endorphin concentrations in PAG and reduced behavioral pain responses (242). Taken together, these observations underpin possible interactions between co-occurrence of chronic pain, SIB and gut dysfunctions, pointing toward a role of endogenous opioids and stress hormones in dysregulated pain perception in ASD.
The Gamma-Aminobutyric Acid Role
The excitatory-inhibitory misbalance in the neuronal system Is implicated in the pathophysiology of pain (243, 244). Gamma-aminobutyric acid (GABA) is known as the main inhibitory neurotransmitter in the adult brain. However, during prenatal stage, GABAergic regulation possesses excitatory role (245, 246). In typical development, GABAergic system switches from excitatory to inhibitory function. The “GABA” hypothesis suggests that early dysfunction of the GABAergic signaling disables normal excitatory/inhibitory switch and is responsible for ASD-related symptoms (247–250). This suggestion is in line with model of “developmental heterochrony,” according to which, autism-related traits may be explained by delays or truncation of typical development (251). In support of this, various studies in ASD have reported reduced GABA concentration, specifically in visual, auditory, somatosensory areas, both in children and adults (252–254).
Recent findings demonstrated that lack of GABA transmission via deficiency in long-term potentiation and learning mechanisms impacts the ability of the amygdala to form fear memory (255) and may be responsible for autistic-like features. Improvement in ASD symptoms related to Bumetanide therapy (a drug promoting of GABA shift from excitation toward inhibition) in young children (<6 years old) was associated with normalization of GABA/Glutamate ratios specifically in the insula cortex (256). This may relate GABA dysfunction and the role of negative affect in socio-emotional learning of pain perception. Two other recent studies analyzed the relationship between GABA levels in several brain regions and multiple aspects of sensory processing alterations in ASD. The first study revealed that GABA concentration in the sensorimotor cortex of adults with ASD was lower than in neurotypical adults. In addition, GABA levels were positively correlated with self-reported tactile hypersensitivity in adults with ASD, but not in neurotypical adults (257). In line with these findings, another study in adults with ASD revealed a negative association between left ventral pre-motor cortex GABA concentration and the severity of sensory hyper-responsiveness scores on the Adolescent/Adult Sensory Profile (258). To our knowledge, no study has examined whether GABA concentrations in the brain were associated with altered pain experiences in people with ASD. Future work should evaluate GABA levels in the brain pain matrix as a significant biomarker and therapeutic target for autistic sensory processing disorder and specifically pain processing.
The Role of the Endocannabinoid System
In direct relation with GABA neurotransmission, cannabinoid receptors are present in brain and peripheral neurons, the vagus nerve, gastrointestinal lining, immune cells and skin (259). The ECS participates in brain development, synaptic plasticity, sensory processing and integration, stress regulation and neuromodulation (260). This system modulates social interactions, anxiety and social reward (261). In the context of dysregulation of pain perception, cannabinoid receptors have also been found to play a role in sensory transmission and pain integration (262). Endocannabinoid system dysregulation has been proposed as a pathophysiological mechanism of autism (263, 264). Decreased levels of endocannabinoids were found in children with ASD (265); see for systematic review (266, 267) to link various symptoms in ASD (inflammation, microglia dysfunction, and social symptoms; 268). Three recent papers published by the same team (269–271) focused on the modulation of the brain’s excitatory and inhibitory systems in adults with ASD and neurotypical controls, after a single dose of 600 mg of cannabinoids. This treatment increased levels of GABA in the dorsomedial prefrontal cortex of controls, but decreased GABA in that region of participants with ASD. In light of these findings, new avenues of therapeutic intervention in the treatment of autism have emerged, on one hand from empirical experience of patients and members of their families, and on the other hand, leveraging genetic and metabolic research. In relation to the above-mentioned gut-brain axis dysfunction in ASD, the potential for the endocannabinoids in alleviating gastrointestinal (272) and behavioral disorders in autism (263, 273–275) along with pain management (262) should be explored. However, the precise mechanism of action of cannabinoids in ASD is far from complete. For example, the two main active components, tetrahydrocannabinol and cannabidiol, interact divergently with cannabinoid receptors. The former activates the receptors, whereas the latter seems to block them (260). However, both components were found to improve behaviors in autism (273, 276), indicating needs to future investigations.
Thus, numerous alterations of neurochemical pathways implicated in autism (Table 1) may provide a rich background for integrating numerous behavioral and neurophysiological alterations documented in pain perception, as well as in the development of strategies for pain treatment.
Global Sensory Processing Alterations
Sensory atypicalities are recognized as diagnostic criteria in ASD (19). 90% of individuals with ASD have atypical sensory experiences and regulation, described as both hyper- and hypo-reactivity, with altered responses to tactile or auditory stimulations (75). This topic has been the focus of intensive research over the last 10 years, including studies on pain perception, leading to the “hypothesis of sensory dysfunction” as a cause of altered pain sensitivity in ASD (14, 30, 32). These anomalies affect all the sensory channels of the child and the adult (see section “Initial Stages of Pain Signaling: From Nociceptors to the Central Axis”) and complicate the integration and cognitive processing of stimuli, making each pain percept unique and poorly integrated in a general concept of the self.
Altered Central Pain Processing and Modified Regulation of Pain Responses
A growing body of evidence delineating alterations of central (both cortical and subcortical) and peripheral pain transduction, transmission and processing are challenging the idea of impaired pain processing due to a single factor, and calls for an integrative model. To summarize the findings regarding the central brain responses and altered pain reactivity in ASD (see section “Neurophysiological Processing of Pain in Autism Spectrum Disorder”), we propose the integrated model of the pain cycle in autism (Figure 3). Within this framework, evidence for altered self-awareness and interoception, and reciprocal relations between altered top-down regulation of pain processing and pain coping in autism may lead to inadequate and deviant pain reactivity. By analogy with other chronic pain conditions, we speculate that altered pain processing in the brain of individuals within the spectrum results from reorganization of pain pathways. This suggestion is supported by recent findings from neuroimaging studies (62, 71, 95). However, more research is needed to delineate causal dependencies between these diverse processes, and to demonstrate how pre-conscious nociception relates to conscious pain perception in subjects with autism, given their self-awareness and interoceptive abilities. Although the basic processes of pain down-regulation seem to be unchanged, the capacity for conscious appraisal of pain and the communication of pain to others might be limited in ASD, especially in early life, when imbalances in physiological and hormonal responses lead to dysregulation of pain management, and probably to long-term consequences like increases in anxiety and depression (168).
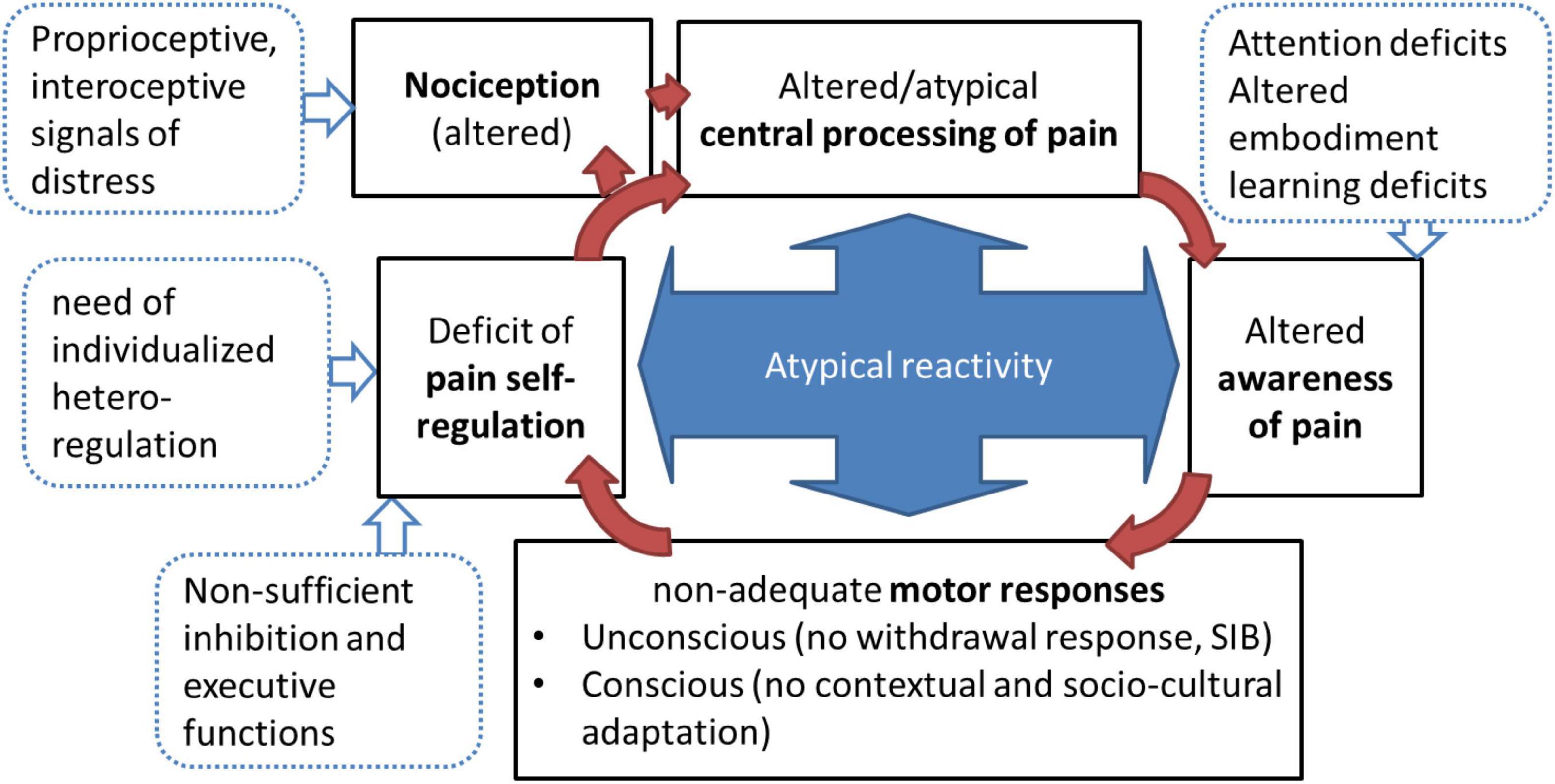
Figure 3. The pain cycle in ASD with possible impacts from suggested mechanisms, modified integrated model, adapted from Dubois et al. (23), Rattaz et al. (24), and (277).
Socio-Communicative Issues
Communicational model of pain highlights the social role of pain expression and intrapersonal reciprocity in down-regulation of pain (277). Observed dissociation between enhanced physiological and biological stress responses and behavioral reactions in autism (28, 165) suggest that, at least for some individuals, the neurochemical and brain pathways are preserved but pain communication is altered (8, 177, 181). Based on the various findings reported above (see section “Atypical Pain Reactions in Autism Spectrum Disorder”) atypical motor response and altered reciprocity may inhibit the observer’s empathy, raising a “double-empathy issue” (278) that was highlighted in recent publications (23, 24). As in most cases, people with ASD have no intellectual disability (279) and may use alternative strategies of social adaptation (280), the potential of specific cognitive-behavioral therapy (281) for pain understanding in autism should be explored.
Perspectives
Do core autism symptoms (sensorial and social) initially cause altered pain perception and expression, which are then worsened by comorbidities and atypical social modulation experiences? Or does pain processing in both neurotypical and ASD individuals share common vulnerabilities that lead to association of ASD symptoms and pain dysregulation? Would it be possible to compensate or prevent alteration of the pain modulation as early as ASD is diagnosed? General heritability of ASD is approximately 80% (282). Among the numerous genes associated with ASD, some have also recently been implicated in pain processing (283, 284). These genes are involved in various cytomolecular mechanisms controlling of C-fiber excitability thresholds, or glutamate pathways (27) and induce alteration in pain processing, in both animal and human models (5) if muted. However, findings in animal models of autism report wide divergence of pain perception and responses (27), pointing out the possible impact of epigenetic factors on relevant genetic alterations of ASD. To note, most animal models are monogenic mutation models, in contrast with human. Additional research is needed to better understand the common genetic and biomolecular pathways (283, 284), and propose innovative and preventative therapeutics.
Pain processing develops with age, starting during gestation, and matures with social experiences throughout life (285, 286). Given that ASD is considered a developmental condition, future research need to address this developmental aspect, proposing longitudinal studies, including early therapeutics and preventative strategies.
Social development interacts with pain management. Positive social experiences impact pain modulation and may alleviate pain perception. Oppositely, negative social experiences as isolation, bullying, and social rejection among others, may aggravate perceived pain. Such “psychological” pain itself produces similar brain response in pain matrix as does physical pain (287, 288) and have been associated with SIB in ASD (289). That bidirectional aspect of social interactions on pain perception and expression in ASD should be more explored.
Mechanisms and perspectives highlights. Numerous mechanisms involved in ASD pathogenesis have been proposed to explain the alterations in pain processing. Among them, alterations in neurotransmitters and neuropeptides (opioids, endocannabinoids, GABA), in global sensory processing, in brain response, together with socio-communicational issues are thought to be associated differently in individuals with ASD contributing to both autism core symptoms and pain dysregulation. In an attempt to integrate these mechanisms, we propose our all-inclusive model of the pain cycle in autism. However, a number of open questions still remains, requiring an integrative approach to resolving the paradox of pain in ASD.
Conclusion
The research of pain in ASD is complex. Recent findings in alterations of brain pathways, summarized as an integrated model of the pain cycle in autism (Figure 3), suggest more than nociceptor and neuronal dysfunctions and implicate altered self-awareness and interoception, which interact with top-down regulatory pathways for processing and coping with pain. Both peripheral and central deviations in pain signal processing are documented in autism. The high variability in pain-related responses in this population makes the group-based approach challenging. To date, other- and self-rated pain assessments aren’t sufficient to characterize the specificity of pain-related processes in autism and to drive interventions. New technologies, already used in emotion recognition research in ASD, joined with continuous physiological activity screening, may provide more quantitative and integrative approaches to specify what is atypical in expressions of pain in people with ASD. Future directions may address the role of these various alterations at different stages of pain signaling and regulation in ASD symptomatology and may yield promising candidates for global therapy, social improvements and behavioral amelioration. The importance of adequate, objective and multiple approaches for the assessment of pain in individuals with autism is essential not only for health outcomes but also to prevent the worsening of social disorders. A better understanding of the complexity and individuality of pain regulation may resolve the paradox of pain in autism, leading to the development of individualized pain management strategies, and with novel therapeutic approaches.
Author Contributions
OB: conceptualization and writing – original draft. VB: visualization and writing – review and editing. AP, MB, and NM: writing – review and editing. J-RC: funding acquisition, supervision, and writing – review and editing. AA: funding acquisition, supervision, conceptualization, and writing – review and editing. All authors contributed to the article and approved the submitted version.
Funding
This work was supported by John Bost foundation grant to AA and J-RC.
Conflict of Interest
The authors declare that the research was conducted in the absence of any commercial or financial relationships that could be construed as a potential conflict of interest.
Publisher’s Note
All claims expressed in this article are solely those of the authors and do not necessarily represent those of their affiliated organizations, or those of the publisher, the editors and the reviewers. Any product that may be evaluated in this article, or claim that may be made by its manufacturer, is not guaranteed or endorsed by the publisher.
Supplementary Material
The Supplementary Material for this article can be found online at: https://www.frontiersin.org/articles/10.3389/fpsyt.2022.910824/full#supplementary-material
References
1. Spectrum Autism Research News. Unseen Agony: Dismantling Autism’s House of Pain. (2015). Available online at: https://www.spectrumnews.org/features/deep-dive/unseen-agony-dismantling-autisms-house-of-pain/ (accessed January 19, 2021)
2. Elsabbagh M, Divan G, Koh Y-J, Kim YS, Kauchali S, Marcín C, et al. Global Prevalence of autism and other pervasive developmental disorders. Autism Res. (2012) 5:160–79. doi: 10.1002/aur.239
3. Maenner MJ. Prevalence and Characteristics of autism spectrum disorder among children aged 8 years — autism and developmental disabilities monitoring network, 11 Sites, United States, 2018. MMWR Surveill Summ. (2021) 70:1–16. doi: 10.15585/mmwr.ss7011a1
4. Loomes R, Hull L, Mandy WPL. what is the male-to-female ratio in autism spectrum disorder? A systematic review and meta-analysis. J Am Acad Child Adolesc Psychiatry. (2017) 56:466–74. doi: 10.1016/j.jaac.2017.03.013
5. Brown CO, Uy J, Singh KK. A mini-review: bridging the gap between autism spectrum disorder and pain comorbidities. Can J Pain. (2020) 4:37–44. doi: 10.1080/24740527.2020.1775486
6. Lipsker CW, Bölte S, Hirvikoski T, Lekander M, Holmström L, Wicksell RK. Prevalence of autism traits and attention-deficit hyperactivity disorder symptoms in a clinical sample of children and adolescents with chronic pain. J Pain Res. (2018) 11:2827–36. doi: 10.2147/JPR.S177534
7. Bursch B, Ingman K, Vitti L, Hyman P, Zeltzer LK. Chronic pain in individuals with previously undiagnosed autistic spectrum disorders. J Pain. (2004) 5:290–5. doi: 10.1016/j.jpain.2004.04.004
8. Clarke C. Autism spectrum disorder and amplified pain. Case Rep Psychiatry. (2015) 2015:e930874. doi: 10.1155/2015/930874
9. Whitney DG, Shapiro DN. National prevalence of pain among children and adolescents with autism spectrum disorders. JAMA Pediatr. (2019) 173:1203–5. doi: 10.1001/jamapediatrics.2019.3826
10. Woolfenden S, Sarkozy V, Ridley G, Coory M, Williams K. A systematic review of two outcomes in autism spectrum disorder – epilepsy and mortality. Dev Med Child Neurol. (2012) 54:306–12. doi: 10.1111/j.1469-8749.2012.04223.x
11. Lukmanji S, Manji SA, Kadhim S, Sauro KM, Wirrell EC, Kwon C-S, et al. The co-occurrence of epilepsy and autism: a systematic review. Epilepsy Behav. (2019) 98:238–48. doi: 10.1016/j.yebeh.2019.07.037
12. Baeza-Velasco C, Cohen D, Hamonet C, Vlamynck E, Diaz L, Cravero C, et al. Autism, joint hypermobility-related disorders and pain. Front Psychiatry. (2018) 9:656. doi: 10.3389/fpsyt.2018.00656
13. Bjørklund G, Pivina L, Dadar M, Meguid NA, Semenova Y, Anwar M, et al. Gastrointestinal alterations in autism spectrum disorder: what do we know? Neurosci Biobehav Rev. (2020) 118:111–20. doi: 10.1016/j.neubiorev.2020.06.033
14. Failla MD, Gerdes MB, Williams ZJ, Moore DJ, Cascio CJ. Increased pain sensitivity and pain-related anxiety in individuals with autism. Pain Rep. (2020) 5:e861. doi: 10.1097/PR9.0000000000000861
15. Tudor ME, Walsh CE, Mulder EC, Lerner MD. Pain as a predictor of sleep problems in youth with autism spectrum disorders. Autism Int J Res Pract. (2015) 19:292–300. doi: 10.1177/1362361313518994
16. Cashin A, Buckley T, Trollor JN, Lennox N. A scoping review of what is known of the physical health of adults with autism spectrum disorder. J Intellect Disabil. (2018) 22:96–108. doi: 10.1177/1744629516665242
17. Hirvikoski T, Mittendorfer-Rutz E, Boman M, Larsson H, Lichtenstein P, Bölte S. Premature mortality in autism spectrum disorder. Br J Psychiatry J Ment Sci. (2016) 208:232–8. doi: 10.1192/bjp.bp.114.160192
18. Hawkes N. People with autism die 16 years earlier on average, says charity. BMJ. (2016) 352:i1615. doi: 10.1136/bmj.i1615
19. American Psychiatric Association. Diagnostic and Statistical Manual of Mental Disorders: DSM-5. Virginia, VA: American Psychiatric Association (2013).
20. Summers J, Shahrami A, Cali S, D’Mello C, Kako M, Palikucin-Reljin A, et al. Self-injury in autism spectrum disorder and intellectual disability: exploring the role of reactivity to pain and sensory input. Brain Sci. (2017) 7:140. doi: 10.3390/brainsci7110140
21. Tordjman S, Antoine C, Cohen DJ, Gauvain-Piquard A, Carlier M, Roubertoux P, et al. [Study of the relationships between self-injurious behavior and pain reactivity in infantile autism]. L’Encephale. (1999) 25:122–34.
22. Allely CS. Pain sensitivity and observer perception of pain in individuals with autistic spectrum disorder. Sci World J. (2013) 2013:916178. doi: 10.1155/2013/916178
23. Dubois A, Rattaz C, Pry R, Baghdadli A. Autisme et douleur – analyse bibliographique. Pain Res Manag J Can Pain Soc. (2010) 15:245–53. doi: 10.1155/2010/749275
24. Rattaz C, Dubois A, Baghdadli A. How do people with autism spectrum disorders (ASD) experience pain? In: A Battaglia editor. An Introduction to Pain and its Relation to Nervous System Disorders. New York, NY: John Wiley & Sons, Ltd (2016). p. 295–315. doi: 10.1002/9781118455968.ch12
25. Moore DJ. Acute pain experience in individuals with autism spectrum disorders: a review. Autism Int J Res Pract. (2015) 19:387–99. doi: 10.1177/1362361314527839
26. Knoll AKI, McMurtry CM, Chambers C. Pain in children with autism spectrum disorder: experience, expression, and assessment. Pediatr Pain Lett. (2013) 15:23–8.
27. Glaunec LR-L, Inquimbert P, Hugel S, Schlichter R, Bossu J-L. Nociception, douleur et autisme. Méd Sci. (2021) 37:141–51. doi: 10.1051/medsci/2020280
28. Tordjman S, Anderson GM, Charrier A, Oriol C, Kermarrec S, Canitano R, et al. Relationships between self-injurious behaviors, pain reactivity, and β-endorphin in children and adolescents with autism. J Clin Psychiatry. (2018) 79:16m10889. doi: 10.4088/JCP.16m10889
29. Fan Y-T, Chen C, Chen S-C, Decety J, Cheng Y. Empathic arousal and social understanding in individuals with autism: evidence from fMRI and ERP measurements. Soc Cogn Affect Neurosci. (2014) 9:1203–13. doi: 10.1093/scan/nst101
30. Riquelme I, Hatem SM, Montoya P. Abnormal pressure pain, touch sensitivity, proprioception, and manual dexterity in children with autism spectrum disorders. Neural Plast. (2016) 2016:1723401. doi: 10.1155/2016/1723401
31. Cascio C, McGlone F, Folger S, Tannan V, Baranek G, Pelphrey KA, et al. Tactile perception in adults with autism: a multidimensional psychophysical study. J Autism Dev Disord. (2008) 38:127–37. doi: 10.1007/s10803-007-0370-8
32. Vaughan S, McGlone F, Poole H, Moore DJA. Quantitative sensory testing approach to pain in autism spectrum disorders. J Autism Dev Disord. (2020) 50:1607–20. doi: 10.1007/s10803-019-03918-0
33. Raja SN, Carr DB, Cohen M, Finnerup NB, Flor H, Gibson S, et al. The revised international association for the study of pain definition of pain: concepts, challenges, and compromises. Pain. (2020) 161:1976–82. doi: 10.1097/j.pain.0000000000001939
34. Orr PM, Shank BC, Black AC. The role of pain classification systems in pain management. Crit Care Nurs Clin North Am. (2017) 29:407–18. doi: 10.1016/j.cnc.2017.08.002
35. Yam MF, Loh YC, Tan CS, Adam SK, Manan NA, Basir R. General pathways of pain sensation and the major neurotransmitters involved in pain regulation. Int J Mol Sci. (2018) 19:2164. doi: 10.3390/ijms19082164
36. Garland EL. Pain processing in the human nervous system: a selective review of nociceptive and biobehavioral pathways. Prim Care. (2012) 39:561–71. doi: 10.1016/j.pop.2012.06.013
37. Fitzgibbon B, Segrave R, Fitzgerald P, Enticott P. Can studies of pain help to bridge the gap between sensory and social impairments in autism? Front Hum Neurosci. (2013) 7:103. doi: 10.3389/fnhum.2013.00103
38. Ossipov MH, Morimura K, Porreca F. Descending pain modulation and chronification of pain. Curr Opin Support Palliat Care. (2014) 8:143–51. doi: 10.1097/SPC.0000000000000055
39. Apkarian AV, Bushnell MC, Treede R-D, Zubieta J-K. Human brain mechanisms of pain perception and regulation in health and disease. Eur J Pain Lond Engl. (2005) 9:463–84. doi: 10.1016/j.ejpain.2004.11.001
40. Fraga MM, Terreri MT, Azevedo RT, Hilário MOE, Len CA. PAIN PERCEPTION AND PAIN COPING MECHANISMS IN CHILDREN AND ADOLESCENTS WITH JUVENILE FIBROMYALGIA AND POLYARTICULAR JUVENILE IDIOPATHIC ARTHRITIS. Rev Paul Pediatr. (2019) 37:11–9. doi: 10.1590/1984-0462/;2019;37;1;00006
41. Jepma M, Koban L, van Doorn J, Jones M, Wager TD. Behavioural and neural evidence for self-reinforcing expectancy effects on pain. Nat Hum Behav. (2018) 2:838–55. doi: 10.1038/s41562-018-0455-8
42. Bushnell MC, Ceko M, Low LA. Cognitive and emotional control of pain and its disruption in chronic pain. Nat Rev Neurosci. (2013) 14:502–11. doi: 10.1038/nrn3516
43. Baliki MN, Apkarian AV. Nociception, pain, negative moods, and behavior selection. Neuron. (2015) 87:474–91. doi: 10.1016/j.neuron.2015.06.005
44. He Y, Kim P. Allodynia: StatPearls [Internet]. Treasure Island (FL): StatPearls Publishing (2020).
45. Hill RZ, Bautista DM. Getting in touch with mechanical pain mechanisms. Trends Neurosci. (2020) 43:311–25. doi: 10.1016/j.tins.2020.03.004
46. Chien Y-L, Chao C-C, Wu S-W, Hsueh H-W, Chiu Y-N, Tsai W-C, et al. Small fiber pathology in autism and clinical implications. Neurology. (2020) 95:e2697–706. doi: 10.1212/WNL.0000000000010932
47. Silva L, Schalock M. First skin biopsy reports in children with autism show loss of C-tactile fibers. J Neurol Disord. (2016) 4:262. doi: 10.4172/2329-6895.1000262
48. Bird G, Silani G, Brindley R, White S, Frith U, Singer T. Empathic brain responses in insula are modulated by levels of alexithymia but not autism. Brain J Neurol. (2010) 133:1515–25. doi: 10.1093/brain/awq060
49. Yasuda Y, Hashimoto R, Nakae A, Kang H, Ohi K, Yamamori H, et al. Sensory cognitive abnormalities of pain in autism spectrum disorder: a case–control study. Ann Gen Psychiatry. (2016) 15:8. doi: 10.1186/s12991-016-0095-1
50. Dube WV, Farber RS, Mueller MR, Grant E, Lorin L, Deutsch CK. STIMULUS OVERSELECTIVITY IN AUTISM, DOWN SYNDROME, AND TYPICAL DEVELOPMENT. Am J Intellect Dev Disabil. (2016) 121:219–35. doi: 10.1352/1944-7558-121.3.219
51. Brown NB, Dunn W. Relationship between context and sensory processing in children with autism. Am J Occup Ther. (2010) 64:474–83. doi: 10.5014/ajot.2010.09077
52. Tuttle AH, Bartsch VB, Zylka MJ. The troubled touch of autism. Cell. (2016) 166:273–4. doi: 10.1016/j.cell.2016.06.054
53. Melzack R, Wall PD. Pain mechanisms: a new theory. Science. (1965) 150:971–9. doi: 10.1126/science.150.3699.971
54. Steeds CE. The anatomy and physiology of pain. Surg Oxf Int Ed. (2016) 34:55–9. doi: 10.1016/j.mpsur.2015.11.005
55. Symons FJ, Harper VN, McGrath PJ, Breau LM, Bodfish JW. Evidence of increased non-verbal behavioral signs of pain in adults with neurodevelopmental disorders and chronic self-injury. Res Dev Disabil. (2009) 30:521–8. doi: 10.1016/j.ridd.2008.07.012
56. Riquelme I, Hatem SM, Montoya P. Reduction of pain sensitivity after somatosensory therapy in children with autism spectrum disorders. J Abnorm Child Psychol. (2018) 46:1731–40. doi: 10.1007/s10802-017-0390-6
57. Dietz FR, Compton SP. Outcomes of a simple treatment for complex regional pain syndrome type I in children. Iowa Orthop J. (2015) 35:175–80.
58. Gay CW, Robinson ME, George SZ, Perlstein WM, Bishop MD. Immediate changes after manual therapy in resting-state functional connectivity as measured by functional magnetic resonance imaging in participants with induced low back pain. J Manipulative Physiol Ther. (2014) 37:614–27. doi: 10.1016/j.jmpt.2014.09.001
59. Staud R, Robinson ME, Goldman CT, Price DD. Attenuation of experimental pain by vibro-tactile stimulation in patients with chronic local or widespread musculoskeletal pain. Eur J Pain Lond Engl. (2011) 15:836–42. doi: 10.1016/j.ejpain.2011.01.011
60. Lenz FA, Weiss N, Ohara S, Lawson C, Greenspan JD. Chapter 6 The role of the thalamus in pain. In: M Hallett, LH Phillips, DL Schomer, JM Massey editors. Supplements to Clinical Neurophysiology. Advances in Clinical Neurophysiology. Amsterdam: Elsevier (2004). p. 50–61. doi: 10.1016/S1567-424X(09)70342-3
61. Anderson WS, Ohara S, Lawson HC, Treede R-D, Lenz FA. Plasticity of pain-related neuronal activity in the human thalamus. In: AR Møller editor. Progress in Brain Research. Reprogramming of the Brain. Amsterdam: Elsevier (2006). p. 353–64. doi: 10.1016/S0079-6123(06)57021-9
62. Failla MD, Moana-Filho EJ, Essick GK, Baranek GT, Rogers BP, Cascio CJ. Initially intact neural responses to pain in autism are diminished during sustained pain. Autism Int J Res Pract. (2018) 22:669–83. doi: 10.1177/1362361317696043
63. Schuetze M, Park MTM, Cho IY, MacMaster FP, Chakravarty MM, Bray SL. Morphological alterations in the thalamus, striatum, and pallidum in autism spectrum disorder. Neuropsychopharmacology. (2016) 41:2627–37. doi: 10.1038/npp.2016.64
64. Nair A, Treiber JM, Shukla DK, Shih P, Müller R-A. Impaired thalamocortical connectivity in autism spectrum disorder: a study of functional and anatomical connectivity. Brain. (2013) 136:1942–55. doi: 10.1093/brain/awt079
65. Woodward ND, Giraldo-Chica M, Rogers B, Cascio CJ. Thalamocortical dysconnectivity in autism spectrum disorder: an analysis of the Autism Brain Imaging Data Exchange. Biol Psychiatry Cogn Neurosci Neuroimaging. (2017) 2:76–84. doi: 10.1016/j.bpsc.2016.09.002
66. Tamura R, Kitamura H, Endo T, Hasegawa N, Someya T. Reduced thalamic volume observed across different subgroups of autism spectrum disorders. Psychiatry Res. (2010) 184:186–8. doi: 10.1016/j.pscychresns.2010.07.001
67. Cheon K-A, Kim Y-S, Oh S-H, Park S-Y, Yoon H-W, Herrington J, et al. Involvement of the anterior thalamic radiation in boys with high functioning autism spectrum disorders: a diffusion tensor imaging study. Brain Res. (2011) 1417:77–86. doi: 10.1016/j.brainres.2011.08.020
68. Iidaka T, Kogata T, Mano Y, Komeda H. Thalamocortical hyperconnectivity and amygdala-cortical hypoconnectivity in male patients with autism spectrum disorder. Front Psychiatry. (2019) 10:252. doi: 10.3389/fpsyt.2019.00252
69. Green SA, Hernandez L, Bookheimer SY, Dapretto M. Reduced modulation of thalamocortical connectivity during exposure to sensory stimuli in ASD. Autism Res. (2017) 10:801–9. doi: 10.1002/aur.1726
70. Garcia-Larrea L, Bastuji H. Pain and consciousness. Prog Neuropsychopharmacol Biol Psychiatry. (2018) 87:193–9. doi: 10.1016/j.pnpbp.2017.10.007
71. Chien Y-L, Wu S-W, Chu C-P, Hsieh S-T, Chao C-C, Gau SS-F. Attenuated contact heat-evoked potentials associated with sensory and social-emotional symptoms in individuals with autism spectrum disorder. Sci Rep. (2017) 7:36887. doi: 10.1038/srep36887
72. Gu X, Zhou TJ, Anagnostou E, Soorya L, Kolevzon A, Hof PR, et al. Heightened brain response to pain anticipation in high-functioning adults with autism spectrum disorder. Eur J Neurosci. (2018) 47:592–601. doi: 10.1111/ejn.13598
73. Marco EJ, Khatibi K, Hill SS, Siegel B, Arroyo MS, Dowling AF, et al. Children with autism show reduced somatosensory response: an MEG study. Autism Res. (2012) 5:340–51. doi: 10.1002/aur.1247
74. Khan S, Michmizos K, Tommerdahl M, Ganesan S, Kitzbichler MG, Zetino M, et al. Somatosensory cortex functional connectivity abnormalities in autism show opposite trends, depending on direction and spatial scale. Brain. (2015) 138:1394–409. doi: 10.1093/brain/awv043
75. Balasco L, Provenzano G, Bozzi Y. Sensory abnormalities in autism spectrum disorders: a focus on the tactile domain, from genetic mouse models to the clinic. Front Psychiatry. (2019) 10:1016. doi: 10.3389/fpsyt.2019.01016
76. Duerden EG, Card D, Roberts SW, Mak-Fan KM, Chakravarty MM, Lerch JP, et al. Self-injurious behaviours are associated with alterations in the somatosensory system in children with autism spectrum disorder. Brain Struct Funct (2014) 219:1251–61. doi: 10.1007/s00429-013-0562-2
77. Shackman AJ, Salomons TV, Slagter HA, Fox AS, Winter JJ, Davidson RJ. The integration of negative affect, pain and cognitive control in the cingulate cortex. Nat Rev Neurosci. (2011) 12:154–67. doi: 10.1038/nrn2994
78. Thakkar KN, Polli FE, Joseph RM, Tuch DS, Hadjikhani N, Barton JJS, et al. Response monitoring, repetitive behaviour and anterior cingulate abnormalities in autism spectrum disorders (ASD). Brain J Neurol. (2008) 131:2464–78. doi: 10.1093/brain/awn099
79. Laidi C, Boisgontier J, de Pierrefeu A, Duchesnay E, Hotier S, d’Albis M-A, et al. Decreased cortical thickness in the anterior cingulate cortex in adults with autism. J Autism Dev Disord. (2019) 49:1402–9. doi: 10.1007/s10803-018-3807-3
80. Lu C, Yang T, Zhao H, Zhang M, Meng F, Fu H, et al. Insular cortex is critical for the perception, modulation, and chronification of pain. Neurosci Bull. (2016) 32:191–201. doi: 10.1007/s12264-016-0016-y
81. Caria A, de Falco S. Anterior insular cortex regulation in autism spectrum disorders. Front Behav Neurosci. (2015) 9:38. doi: 10.3389/fnbeh.2015.00038
82. Uddin LQ, Menon V. The anterior insula in autism: under-connected and under-examined. Neurosci Biobehav Rev.(2009) 33:1198–203. doi: 10.1016/j.neubiorev.2009.06.002
83. Chen WG, Schloesser D, Arensdorf AM, Simmons JM, Cui C, Valentino R, et al. The emerging science of interoception: sensing, integrating, interpreting, and regulating signals within the self. Trends Neurosci. (2021) 44:3–16. doi: 10.1016/j.tins.2020.10.007
84. Craig ADB. How do you feel–now? The anterior insula and human awareness. Nat Rev Neurosci. (2009) 10:59–70. doi: 10.1038/nrn2555
85. Blanke O, Slater M, Serino A. Behavioral, neural, and computational principles of bodily self-consciousness. Neuron. (2015) 88:145–66. doi: 10.1016/j.neuron.2015.09.029
86. DuBois D, Ameis SH, Lai M-C, Casanova MF, Desarkar P. Interoception in autism spectrum disorder: a review. Int J Dev Neurosci. (2016) 52:104–11. doi: 10.1016/j.ijdevneu.2016.05.001
87. Noel J-P, Cascio CJ, Wallace MT, Park S. The spatial self in schizophrenia and autism spectrum disorder. Schizophr Res. (2017) 179:8–12. doi: 10.1016/j.schres.2016.09.021
88. Mul C-L, Cardini F, Stagg SD, Sadeghi Esfahlani S, Kiourtsoglou D, Cardellicchio P, et al. Altered bodily self-consciousness and peripersonal space in autism. Autism Int J Res Pract. (2019) 23:2055–67. doi: 10.1177/1362361319838950
89. Huang AX, Hughes TL, Sutton LR, Lawrence M, Chen X, Ji Z, et al. Understanding the self in individuals with autism spectrum disorders (ASD): a review of literature. Front Psychol. (2017) 8:1422. doi: 10.3389/fpsyg.2017.01422
90. Toichi M, Kamio Y, Okada T, Sakihama M, Youngstrom EA, Findling RL, et al. Lack of self-consciousness in autism. Am J Psychiatry. (2002) 159:1422–4. doi: 10.1176/appi.ajp.159.8.1422
91. Carmody DP, Lewis M. Self representation in children with and without autism spectrum disorders. Child Psychiatry Hum Dev. (2012) 43:227–37. doi: 10.1007/s10578-011-0261-2
92. Tordjman S, Celume MP, Denis L, Motillon T, Keromnes G. Reframing schizophrenia and autism as bodily self-consciousness disorders leading to a deficit of theory of mind and empathy with social communication impairments. Neurosci Biobehav Rev. (2019) 103:401–13. doi: 10.1016/j.neubiorev.2019.04.007
93. Ong W-Y, Stohler CS, Herr DR. Role of the prefrontal cortex in pain processing. Mol Neurobiol. (2019) 56:1137–66. doi: 10.1007/s12035-018-1130-9
94. Mordeniz C. Pain perception within consciousness. Interdiscip J Neurosci Quantum Phys. (2016) 14:439–46. doi: 10.14704/nq.2016.14.2.957
95. Schudlo LC, Anagnostou E, Chau T, Doyle-Thomas K. Investigating sensory response to physical discomfort in children with autism spectrum disorder using near-infrared spectroscopy. PLoS One. (2021) 16:e0257029. doi: 10.1371/journal.pone.0257029
96. Spedding M, Chattarji S, Spedding C, Jay TM. Brain circuits at risk in psychiatric diseases and pharmacological pathways. Therapies. (2021) 76:75–86. doi: 10.1016/j.therap.2020.12.005
97. Cardoso-Cruz H, Paiva P, Monteiro C, Galhardo V. Bidirectional optogenetic modulation of prefrontal-hippocampal connectivity in pain-related working memory deficits. Sci Rep (2019) 9:10980. doi: 10.1038/s41598-019-47555-0
98. Mokhtari T, Tu Y, Hu L. Involvement of the hippocampus in chronic pain and depression. Brain Sci Adv. (2019) 5:288–98. doi: 10.26599/BSA.2019.9050025
99. Tortora D, Severino M, Di Biase C, Malova M, Parodi A, Minghetti D, et al. Early pain exposure in very preterm neonates. Front Neurosci. (2019) 13:899. doi: 10.3389/fnins.2019.00899
100. Blatt GJ. The Neuropathology of Autism. Scientifica. (2012) 2012:e703675. doi: 10.6064/2012/703675
101. Fetit R, Hillary RF, Price DJ, Lawrie SM. The neuropathology of autism: a systematic review of post-mortem studies of autism and related disorders. Neurosci Biobehav Rev. (2021) 129:35–62. doi: 10.1016/j.neubiorev.2021.07.014
102. Banker SM, Gu X, Schiller D, Foss-Feig JH. Hippocampal contributions to social and cognitive deficits in autism spectrum disorder. Trends Neurosci. (2021) 44:793–807. doi: 10.1016/j.tins.2021.08.005
103. Lee JH, Espinera AR, Chen D, Choi K-E, Caslin AY, Won S, et al. Neonatal inflammatory pain and systemic inflammatory responses as possible environmental factors in the development of autism spectrum disorder of juvenile rats. J Neuroinflamm. (2016) 13:109. doi: 10.1186/s12974-016-0575-x
104. De Ridder D, Vanneste S, Smith M, Adhia D. Pain and the triple network model. Front Neurol. (2022) 13:757241. doi: 10.3389/fneur.2022.757241
105. Baliki MN, Geha PY, Apkarian AV, Chialvo DR. Beyond feeling: chronic pain hurts the brain, disrupting the default-mode network dynamics. J Neurosci. (2008) 28:1398–403. doi: 10.1523/JNEUROSCI.4123-07.2008
106. Baliki MN, Mansour AR, Baria AT, Apkarian AV. Functional reorganization of the default mode network across chronic pain conditions. PLoS One. (2014) 9:e106133. doi: 10.1371/journal.pone.0106133
107. Alshelh Z, Marciszewski KK, Akhter R, Di Pietro F, Mills EP, Vickers ER, et al. Disruption of default mode network dynamics in acute and chronic pain states. Neuroimage Clin. (2017) 17:222–31. doi: 10.1016/j.nicl.2017.10.019
108. Padmanabhan A, Lynch CJ, Schaer M, Menon V. The Default Mode Network in Autism. Biol Psychiatry Cogn Neurosci Neuroimaging (2017) 2:476–86. doi: 10.1016/j.bpsc.2017.04.004
109. Wiech K, Tracey I. The influence of negative emotions on pain: behavioral effects and neural mechanisms. Neuroimage. (2009) 47:987–94. doi: 10.1016/j.neuroimage.2009.05.059
110. Veinante P, Yalcin I, Barrot M. The amygdala between sensation and affect: a role in pain. J Mol Psychiatry. (2013) 1:9. doi: 10.1186/2049-9256-1-9
111. Weston CSE. Four social brain regions, their dysfunctions, and sequelae, extensively explain autism spectrum disorder symptomatology. Brain Sci. (2019) 9:130. doi: 10.3390/brainsci9060130
112. Schumann CM, Amaral DG. Stereological analysis of amygdala neuron number in autism. J Neurosci. (2006) 26:7674–9. doi: 10.1523/JNEUROSCI.1285-06.2006
113. van Rooij D, Anagnostou E, Arango C, Auzias G, Behrmann M, Busatto GF, et al. Cortical and subcortical brain morphometry differences between patients with autism spectrum disorder and healthy individuals across the lifespan: results from the ENIGMA ASD working group. Am J Psychiatry. (2018) 175:359–69. doi: 10.1176/appi.ajp.2017.17010100
114. Gibbard CR, Ren J, Skuse DH, Clayden JD, Clark CA. Structural connectivity of the amygdala in young adults with autism spectrum disorder. Hum Brain Mapp. (2018) 39:1270–82. doi: 10.1002/hbm.23915
115. Sato W, Uono S, Kochiyama T. Neurocognitive mechanisms underlying social atypicalities in autism: weak amygdala’s emotional modulation hypothesis. Front Psychiatry. (2020) 11:864. doi: 10.3389/fpsyt.2020.00864
116. Swartz JR, Wiggins JL, Carrasco M, Lord C, Monk CS. Amygdala habituation and prefrontal functional connectivity in youth with autism spectrum disorders. J Am Acad Child Adolesc Psychiatry. (2013) 52:84–93. doi: 10.1016/j.jaac.2012.10.012
117. Ibrahim K, Eilbott JA, Ventola P, He G, Pelphrey KA, McCarthy G, et al. Reduced amygdala-prefrontal functional connectivity in children with autism spectrum disorder and co-occurring disruptive behavior. Biol Psychiatry Cogn Neurosci Neuroimaging. (2019) 4:1031–41. doi: 10.1016/j.bpsc.2019.01.009
118. Neugebauer V. Amygdala pain mechanisms. Handb Exp Pharmacol. (2015) 227:261–84. doi: 10.1007/978-3-662-46450-2_13
119. Bannister K, Dickenson AH. The plasticity of descending controls in pain: translational probing. J Physiol. (2017) 595:4159–66. doi: 10.1113/JP274165
120. Staud R, Vierck CJ, Cannon RL, Mauderli AP, Price DD. Abnormal sensitization and temporal summation of second pain (wind-up) in patients with fibromyalgia syndrome. Pain. (2001) 91:165–75. doi: 10.1016/s0304-3959(00)00432-2
121. Perrot S, Dickenson AH, Bennett RM. Fibromyalgia: harmonizing science with clinical practice considerations. Pain Pract. (2008) 8:177–89. doi: 10.1111/j.1533-2500.2008.00190.x
122. Gwilym SE, Keltner JR, Warnaby CE, Carr AJ, Chizh B, Chessell I, et al. Psychophysical and functional imaging evidence supporting the presence of central sensitization in a cohort of osteoarthritis patients. Arthritis Rheum. (2009) 61:1226–34. doi: 10.1002/art.24837
123. Carmassi C, Cordone A, Ciapparelli A, Bertelloni CA, Barberi FM, Foghi C, et al. Adult autism subthreshold spectrum correlates to post-traumatic stress disorder spectrum in patients with fibromyalgia. Clin Exp Rheumatol. (2021) 39(Suppl. 130):20–6. doi: 10.55563/clinexprheumatol/nivxh9
124. Le Bars D, Dickenson AH, Besson J-M. Diffuse noxious inhibitory controls (DNIC). II. Lack of effect on non-convergent neurones, supraspinal involvement and theoretical implications. Pain. (1979) 6:305–27. doi: 10.1016/0304-3959(79)90050-2
125. Yarnitsky D. Conditioned pain modulation (the diffuse noxious inhibitory control-like effect): its relevance for acute and chronic pain states. Curr Opin Anaesthesiol. (2010) 23:611–5. doi: 10.1097/ACO.0b013e32833c348b
126. Kennedy DL, Kemp HI, Ridout D, Yarnitsky D, Rice ASC. Reliability of conditioned pain modulation: a systematic review. Pain. (2016) 157:2410–9. doi: 10.1097/j.pain.0000000000000689
127. Dubois A, Boudjarane M, Le Fur-Bonnabesse A, Dion A, L’heveder G, Quinio B, et al. Pain modulation mechanisms in ASD adults. J Autism Dev Disord. (2020) 50:2931–40. doi: 10.1007/s10803-019-04361-x
128. Decety J, Lamm C. Human empathy through the lens of social neuroscience. Sci World J. (2006) 6:1146–63. doi: 10.1100/tsw.2006.221
129. Li Y, Zhang T, Li W, Zhang J, Jin Z, Li L. Linking brain structure and activation in anterior insula cortex to explain the trait empathy for pain. Hum Brain Mapp. (2019) 41:1030–42. doi: 10.1002/hbm.24858
130. Singer T, Seymour B, O’Doherty J, Kaube H, Dolan RJ, Frith CD. Empathy for pain involves the affective but not sensory components of pain. Science. (2004) 303:1157–62. doi: 10.1126/science.1093535
131. Botvinick M, Jha AP, Bylsma LM, Fabian SA, Solomon PE, Prkachin KM. Viewing facial expressions of pain engages cortical areas involved in the direct experience of pain. Neuroimage. (2005) 25:312–9. doi: 10.1016/j.neuroimage.2004.11.043
132. Fitzgibbon BM, Giummarra MJ, Georgiou-Karistianis N, Enticott PG, Bradshaw JL. Shared pain: from empathy to synaesthesia. Neurosci Biobehav Rev. (2010) 34:500–12. doi: 10.1016/j.neubiorev.2009.10.007
133. Gu X, Eilam-Stock T, Zhou T, Anagnostou E, Kolevzon A, Soorya L, et al. Autonomic and brain responses associated with empathy deficits in autism spectrum disorder. Hum Brain Mapp. (2015) 36:3323–38. doi: 10.1002/hbm.22840
134. Hadjikhani N, Zürcher NR, Rogier O, Hippolyte L, Lemonnier E, Ruest T, et al. Emotional contagion for pain is intact in autism spectrum disorders. Transl Psychiatry. (2014) 4:e343–343. doi: 10.1038/tp.2013.113
135. Lassalle A, Zürcher NR, Hippolyte L, Billstedt E, Porro CA, Benuzzi F, et al. Effect of visual stimuli of pain on empathy brain network in people with and without Autism Spectrum Disorder. Eur J Neurosci. (2018) 48:2333–42. doi: 10.1111/ejn.14138
136. Naor N, Rohr C, Schaare LH, Limbachia C, Shamay-Tsoory S, Okon-Singer H. The neural networks underlying reappraisal of empathy for pain. Soc Cogn Affect Neurosci. (2020) 15:733–44. doi: 10.1093/scan/nsaa094
137. Harmsen IE. Empathy in autism spectrum disorder. J Autism Dev Disord. (2019) 49:3939–55. doi: 10.1007/s10803-019-04087-w
138. Bogdanov VB, Bogdanova OV, Gorlov DS, Gorgo YP, Dirckx JJJ, Makarchuk MY, et al. Alexithymia and empathy predict changes in autonomic arousal during affective stimulation. Cogn Behav Neurol. (2013) 26:121–32. doi: 10.1097/WNN.0000000000000002
139. Shah P, Livingston LA, Callan MJ, Player L. Trait autism is a better predictor of empathy than alexithymia. J Autism Dev Disord. (2019) 49:3956–64. doi: 10.1007/s10803-019-04080-3
140. Arzy S, Overney LS, Landis T, Blanke O. Neural mechanisms of embodiment: asomatognosia due to premotor cortex damage. Arch Neurol. (2006) 63:1022–5. doi: 10.1001/archneur.63.7.1022
141. Pulvermüller F. How neurons make meaning: brain mechanisms for embodied and abstract-symbolic semantics. Trends Cogn Sci. (2013) 17:458–70. doi: 10.1016/j.tics.2013.06.004
142. Neal DT, Chartrand TL. Embodied emotion perception: amplifying and dampening facial feedback modulates emotion perception accuracy. Soc Psychol Personal Sci. (2011) 2:673–8. doi: 10.1177/1948550611406138
143. Dijkstra K, Post L. Mechanisms of embodiment. Front Psychol. (2015) 6:1525. doi: 10.3389/fpsyg.2015.01525
144. Fanghella M, Gaigg SB, Candidi M, Forster B, Calvo-Merino B. Somatosensory evoked potentials reveal reduced embodiment of emotions in autism. J Neurosci. (2022) 42:2298–312. doi: 10.1523/JNEUROSCI.0706-21.2022
145. Meng J, Shen L, Li Z, Peng W. Top-down effects on empathy for pain in adults with autistic traits. Sci Rep. (2019) 9:8022. doi: 10.1038/s41598-019-44400-2
146. Cheng Y, Chen C, Decety J. How situational context impacts empathic responses and brain activation patterns. Front Behav Neurosci. (2017) 11:165. doi: 10.3389/fnbeh.2017.00165
147. Ackerley R. C-tactile (CT) afferents: evidence of their function from microneurography studies in humans. Curr Opin Behav Sci. (2022) 43:95–100. doi: 10.1016/j.cobeha.2021.08.012
148. Richer J. The social-avoidance behaviour of autistic children. Anim Behav. (1976) 24:898–906. doi: 10.1016/s0003-3472(76)80020-6
149. Lee Masson H, Pillet I, Amelynck S, Van De Plas S, Hendriks M, Op de Beeck H, et al. Intact neural representations of affective meaning of touch but lack of embodied resonance in autism: a multi-voxel pattern analysis study. Mol Autism. (2019) 10:39. doi: 10.1186/s13229-019-0294-0
150. Voos AC, Pelphrey KA, Kaiser MD. Autistic traits are associated with diminished neural response to affective touch. Soc Cogn Affect Neurosci. (2013) 8:378–86. doi: 10.1093/scan/nss009
151. Kaiser MD, Yang DY-J, Voos AC, Bennett RH, Gordon I, Pretzsch C, et al. Brain mechanisms for processing affective (and Nonaffective) touch are atypical in autism. Cereb Cortex. (2016) 26:2705–14. doi: 10.1093/cercor/bhv125
152. Yu H, Miao W, Ji E, Huang S, Jin S, Zhu X, et al. Social touch-like tactile stimulation activates a tachykinin 1-oxytocin pathway to promote social interactions. Neuron. (2022) 110: 1051–1067.e7. doi: 10.1016/j.neuron.2021.12.022
153. Yamasue H, Okada T, Munesue T, Kuroda M, Fujioka T, Uno Y, et al. Effect of intranasal oxytocin on the core social symptoms of autism spectrum disorder: a randomized clinical trial. Mol Psychiatry. (2018) 25:1849–58. doi: 10.1038/s41380-018-0097-2
154. Yamasue H, Domes G. Oxytocin and Autism Spectrum Disorders. Curr Top Behav Neurosci. (2018) 35:449–65. doi: 10.1007/7854_2017_24
155. Poisbeau P, Grinevich V, Charlet A. Oxytocin signaling in pain: cellular, circuit, system, and behavioral levels. Curr Top Behav Neurosci. (2018) 35:193–211. doi: 10.1007/7854_2017_14
156. Bernaerts S, Boets B, Steyaert J, Wenderoth N, Alaerts K. Oxytocin treatment attenuates amygdala activity in autism: a treatment-mechanism study with long-term follow-up. Transl Psychiatry. (2020) 10:1–12. doi: 10.1038/s41398-020-01069-w
157. Gordon I, Jack A, Pretzsch CM, Vander Wyk B, Leckman JF, Feldman R, et al. Intranasal oxytocin enhances connectivity in the neural circuitry supporting social motivation and social perception in children with autism. Sci Rep. (2016) 6:35054. doi: 10.1038/srep35054
158. Zunhammer M, Geis S, Busch V, Greenlee MW, Eichhammer P. Effects of intranasal oxytocin on thermal pain in healthy men: a randomized functional magnetic resonance imaging study. Psychosom Med. (2015) 77:156–66. doi: 10.1097/PSY.0000000000000142
159. Singer T, Snozzi R, Bird G, Petrovic P, Silani G, Heinrichs M, et al. Effects of oxytocin and prosocial behavior on brain responses to direct and vicariously experienced pain. Emot Wash DC. (2008) 8:781–91. doi: 10.1037/a0014195
160. Kassubek J, Juengling FD, Els T, Spreer J, Herpers M, Krause T, et al. Activation of a residual cortical network during painful stimulation in long-term postanoxic vegetative state: a 15O–H2O PET study. J Neurol Sci. (2003) 212:85–91. doi: 10.1016/S0022-510X(03)00106-0
161. Laureys S, Faymonville ME, Peigneux P, Damas P, Lambermont B, Del Fiore G, et al. Cortical processing of noxious somatosensory stimuli in the persistent vegetative state. Neuroimage. (2002) 17:732–41.
162. Boly M, Faymonville M-E, Schnakers C, Peigneux P, Lambermont B, Phillips C, et al. Perception of pain in the minimally conscious state with PET activation: an observational study. Lancet Neurol. (2008) 7:1013–20. doi: 10.1016/S1474-4422(08)70219-9
163. Bienvenu OJ, Colantuoni E, Mendez-Tellez PA, Shanholtz C, Dennison-Himmelfarb CR, Pronovost PJ, et al. Cooccurrence of and remission from general anxiety, depression, and posttraumatic stress disorder symptoms after acute lung injury: a 2-year longitudinal study. Crit Care Med. (2015) 43:642–53. doi: 10.1097/CCM.0000000000000752
164. Bronsard G, Botbol M, Tordjman S. Aggression in low functioning children and adolescents with autistic disorder. PLoS One. (2010) 5:e14358. doi: 10.1371/journal.pone.0014358
165. Tordjman S, Anderson GM, Botbol M, Brailly-Tabard S, Perez-Diaz F, Graignic R, et al. Pain reactivity and plasma beta-endorphin in children and adolescents with autistic disorder. PLoS One. (2009) 4:e5289. doi: 10.1371/journal.pone.0005289
166. Craig KD. Social communication model of pain. Pain. (2015) 156:1198–9. doi: 10.1097/j.pain.0000000000000185
167. Cook J, Hull L, Crane L, Mandy W. Camouflaging in autism: a systematic review. Clin Psychol Rev. (2021) 89:102080. doi: 10.1016/j.cpr.2021.102080
168. Garcia-Villamisar D, Moore D, Garcia-Martínez M. Internalizing symptoms mediate the relation between acute pain and autism in adults. J Autism Dev Disord. (2019) 49:270–8. doi: 10.1007/s10803-018-3765-9
169. Ohara R, Kanejima Y, Kitamura M, Izawa K. Association between social skills and motor skills in individuals with autism spectrum disorder: a systematic review. Eur J Investig Health Psychol Educ. (2019) 10:276–96. doi: 10.3390/ejihpe10010022
170. Biersdorff KK. Incidence of significantly altered pain experience among individuals with developmental disabilities. Am J Ment Retard AJMR. (1994) 98:619–31.
171. Dubois A, Michelon C, Rattaz C, Zabalia M, Baghdadli A. Daily living pain assessment in children with autism: exploratory study. Res Dev Disabil. (2017) 62:238–46. doi: 10.1016/j.ridd.2017.01.003
172. Lee L, Ra H, Chang JJ, Connors Sl. Increased risk of injury in children with developmental disabilities. Res Dev Disabil. (2007) 29:247–55. doi: 10.1016/j.ridd.2007.05.002
173. Melzack R. The short-form McGill Pain Questionnaire. Pain. (1987) 30:191–7. doi: 10.1016/0304-3959(87)91074-8
174. McGrath PJ, Rosmus C, Canfield C, Campbell MA, Hennigar A. Behaviours caregivers use to determine pain in non-verbal, cognitively impaired individuals. Dev Med Child Neurol. (1998) 40:340–3. doi: 10.1111/j.1469-8749.1998.tb15386.x
175. Nader R, Oberlander TF, Chambers CT, Craig KD. Expression of pain in children with autism. Clin J Pain. (2004) 20:88–97. doi: 10.1097/00002508-200403000-00005
176. Bandstra NF, Johnson SA, Filliter JH, Chambers CT. Self-reported and parent-reported pain for common painful events in high-functioning children and adolescents with autism spectrum disorder. Clin J Pain. (2012) 28:715–21. doi: 10.1097/AJP.0b013e318243ecf6
177. Ely E, Chen-Lim ML, Carpenter KM, Wallhauser E, Friedlaender E. Pain assessment of children with autism spectrum disorders. J Dev Behav Pediatr JDBP. (2016) 37:53–61. doi: 10.1097/DBP.0000000000000240
178. Wong DL, Baker CM. Pain in children: comparison of assessment scales. Pediatr Nurs. (1988) 14:9–17.
179. Gaston-Johansson F. Measurement of pain: the psychometric properties of the pain-O-meter, a simple, inexpensive pain assessment tool that could change health care practices. J Pain Symptom Manag. (1996) 12:172–81. doi: 10.1016/0885-3924(96)00128-5
180. Militerni R, Bravaccio C, Falco C, Puglisi-Allegra S, Pascucci T, Fico C. Pain reactivity in children with autistic disorder. J Headache Pain. (2000) 1:53–6. doi: 10.1007/s101940050011
181. Gilbert-MacLeod CA, Craig KD, Rocha EM, Mathias MD. Everyday pain responses in children with and without developmental delays. J Pediatr Psychol. (2000) 25:301–8. doi: 10.1093/jpepsy/25.5.301
182. Pernon E, Rattaz C. Les modes d’expression de la douleur chez l’enfant autiste: étude comparée. Devenir. (2003) 15:263–77.
183. Daughters H, Palermo T, Koh J. Procedural pain and distress in children with autism– a pilot study. J Pain. (2007) 8:S31. doi: 10.1016/j.jpain.2007.02.124
184. Breau LM, Camfield CS, Symons FJ, Bodfish JW, Mackay A, Finley GA, et al. Relation between pain and self-injurious behavior in nonverbal children with severe cognitive impairments. J Pediatr. (2003) 142:498–503. doi: 10.1067/mpd.2003.163
185. EPS Barthélémy Durand. ESDDA: Echelle Simplifiée d’Evaluation de la Douleur chez les Personnes Dyscommunicantes Avec Troubles du Spectre de l’Autisme. Étampes: EPS Barthélémy Durand (2017).
186. Prkachin KM. Assessing pain by facial expression: facial expression as nexus. Pain Res Manag J Can Pain Soc. (2009) 14:53–8. doi: 10.1155/2009/542964
187. Craig K, Prkachin K, Grunau R. The facial expression of pain. In: DC Turk, R Melzack editors. Handbook Pain Assessment. New York, NY: Guilford (2001). p. 153–69.
188. Rojo R, Prados-Frutos JC, López-Valverde A. Pain assessment using the facial action coding system. A systematic review. Med Clin (Barc). (2014) 145:350–5. doi: 10.1016/j.medcli.2014.08.010
189. Arif-Rahu M, Grap MJ. Facial expression and pain in the critically ill non-communicative patient: state of science review. Intensive Crit Care Nurs. (2010) 26:343–52. doi: 10.1016/j.iccn.2010.08.007
190. Briot K, Pizano A, Bouvard M, Amestoy A. New technologies as promising tools for assessing facial emotion expressions impairments in ASD: a systematic review. Front Psychiatry. (2021) 12:634756. doi: 10.3389/fpsyt.2021.634756
191. Trevisan DA, Hoskyn M, Birmingham E. Facial expression production in autism: a meta-analysis. Autism Res. (2018) 11:1586–601. doi: 10.1002/aur.2037
192. Keating CT, Cook JL. Facial expression production and recognition in autism spectrum disorders: a shifting landscape. Child Adolesc Psychiatr Clin N Am. (2020) 29:557–71. doi: 10.1016/j.chc.2020.02.006
193. Rattaz C, Dubois A, Michelon C, Viellard M, Poinso F, Baghdadli A. How do children with autism spectrum disorders express pain? A comparison with developmentally delayed and typically developing children. Pain. (2013) 154:2007–13. doi: 10.1016/j.pain.2013.06.011
194. Prkachin KM, Mass H, Mercer SR. Effects of exposure on perception of pain expression. Pain. (2004) 111:8–12. doi: 10.1016/j.pain.2004.03.027
195. Messmer RL, Nader R, Craig KD. Brief report: judging pain intensity in children with autism undergoing venepuncture: the influence of facial activity. J Autism Dev Disord. (2008) 38:1391–4. doi: 10.1007/s10803-007-0511-0
196. Deyo KS, Prkachin KM, Mercer SR. Development of sensitivity to facial expression of pain. Pain. (2004) 107:16–21. doi: 10.1016/S0304-3959(03)00263-X
197. Mieronkoski R, Syrjälä E, Jiang M, Rahmani A, Pahikkala T, Liljeberg P, et al. Developing a pain intensity prediction model using facial expression: a feasibility study with electromyography. PLoS One. (2020) 15:e0235545. doi: 10.1371/journal.pone.0235545
198. Stöckli S, Schulte-Mecklenbeck M, Borer S, Samson A. Facial expression analysis with AFFDEX and FACET: a validation study. Behav Res Methods. (2017) 50:1446–60. doi: 10.3758/s13428-017-0996-1
199. Chen Z, Ansari R, Wilkie DJ. Automated detection of pain from facial expressions: a rule-based approach using AAM. Proc SPIE Int Soc Opt Eng. (2012) 8314:83143O. doi: 10.1117/12.912537
200. Ahn SJ-G, Bailenson J, Fox J, Jabon M. Using automated facial expression analysis for emotion and behavior prediction. In: K Doveling, C von Scheve, E Konijn editors. The Routledge Handbook of Emotions and Mass Media. New York, NY: Routledge (2010). p. 349–69.
201. Dzedzickis A, Kaklauskas A, Bucinskas V. Human emotion recognition: review of sensors and methods. Sensors. (2020) 20:592. doi: 10.3390/s20030592
202. Bangerter A, Chatterjee M, Manfredonia J, Manyakov N, Ness S, Boice M, et al. Automated recognition of spontaneous facial expression in individuals with autism spectrum disorder: parsing response variability. Mol Autism. (2020) 11:31. doi: 10.1186/s13229-020-00327-4
203. Coco MD, Leo M, Carcagnì P, Spagnolo P, Mazzeo PL, Bernava M, et al. A Computer vision based approach for understanding emotional involvements in children with autism spectrum disorders. Proceedings of the 2017 IEEE International Conference on Computer Vision Workshops (ICCVW). Venice: (2017). p. 1401–7. doi: 10.1109/ICCVW.2017.166
204. Owada K, Kojima M, Yassin W, Kuroda M, Kawakubo Y, Kuwabara H, et al. Computer-analyzed facial expression as a surrogate marker for autism spectrum social core symptoms. PLoS One. (2018) 13:e0190442. doi: 10.1371/journal.pone.0190442
205. Dawes TR, Eden-Green B, Rosten C, Giles J, Governo R, Marcelline F, et al. Objectively measuring pain using facial expression: is the technology finally ready? Pain Manag. (2018) 8:105–13. doi: 10.2217/pmt-2017-0049
206. Burton AR, Fazalbhoy A, Macefield VG. Sympathetic responses to noxious stimulation of muscle and skin. Front Neurol. (2016) 7:109. doi: 10.3389/fneur.2016.00109
207. Ledowski T. Objective monitoring of nociception: a review of current commercial solutions. Br J Anaesth. (2019) 123:e312–21. doi: 10.1016/j.bja.2019.03.024
208. Korving H, Sterkenburg PS, Barakova EI, Feijs LMG. Physiological measures of acute and chronic pain within different subject groups: a systematic review. Pain Res Manag. (2020) 2020:9249465. doi: 10.1155/2020/9249465
209. Koenig J, Jarczok MN, Ellis RJ, Hillecke TK, Thayer JF. Heart rate variability and experimentally induced pain in healthy adults: a systematic review. Eur J Pain. (2014) 18:301–14. doi: 10.1002/j.1532-2149.2013.00379.x
210. Kyle BN, McNeil DW. Autonomic arousal and experimentally induced pain: a critical review of the literature. Pain Res Manag. (2014) 19:159–67. doi: 10.1155/2014/536859
211. Goodwin MS, Mazefsky CA, Ioannidis S, Erdogmus D, Siegel M. Predicting aggression to others in youth with autism using a wearable biosensor. Autism Res. (2019) 12:1286–96. doi: 10.1002/aur.2151
212. Gruss S, Geiger M, Werner P, Wilhelm O, Traue HC, Al-Hamadi A, et al. Multi-modal signals for analyzing pain responses to thermal and electrical stimuli. J Vis Exp. (2019) 146:e59057. doi: 10.3791/59057
213. Poria S, Cambria E, Bajpai R, Hussain A. A review of affective computing: from unimodal analysis to multimodal fusion. Inf Fusion. (2017) 37:98–125. doi: 10.1016/j.inffus.2017.02.003
214. Black MH, Milbourn B, Chen NTM, McGarry S, Wali F, Ho ASV, et al. The use of wearable technology to measure and support abilities, disabilities and functional skills in autistic youth: a scoping review. Scand J Child Adolesc Psychiatry Psychol. (2020) 8:48–69. doi: 10.21307/sjcapp-2020-006
215. Marotta R, Risoleo MC, Messina G, Parisi L, Carotenuto M, Vetri L, et al. The neurochemistry of autism. Brain Sci. (2020) 10:163. doi: 10.3390/brainsci10030163
216. Sprouse-Blum AS, Smith G, Sugai D, Parsa FD. Understanding endorphins and their importance in pain management. Hawaii Med J. (2010) 69:70–1.
217. Panksepp J. A neurochemical theory of autism. Trends Neurosci. (1979) 2:174–7. doi: 10.1016/0166-2236(79)90071-7
218. Campbell M, Anderson LT, Small AM, Adams P, Gonzalez NM, Ernst M. Naltrexone in autistic children: behavioral symptoms and attentional learning. J Am Acad Child Adolesc Psychiatry. (1993) 32:1283–91. doi: 10.1097/00004583-199311000-00024
219. Leboyer M, Bouvard MP, Recasens C, Philippe A, Guilloud-Bataille M, Bondoux D, et al. Difference between plasma N– and C-terminally directed beta-endorphin immunoreactivity in infantile autism. Am J Psychiatry. (1994) 151:1797–801. doi: 10.1176/ajp.151.12.1797
220. Bouvard MP, Leboyer M, Launay JM, Recasens C, Plumet MH, Waller-Perotte D, et al. Low-dose naltrexone effects on plasma chemistries and clinical symptoms in autism: a double-blind, placebo-controlled study. Psychiatry Res. (1995) 58:191–201. doi: 10.1016/0165-1781(95)02601-r
221. Nagamitsu S. CSF beta-endorphin levels in pediatric neurologic disorders. Kurume Med J. (1993) 40:233–41. doi: 10.2739/kurumemedj.40.233
222. Weizman R, Gil-Ad I, Dick J, Tyano S, Szekely GA, Laron Z. Low plasma immunoreactive beta-endorphin levels in autism. J Am Acad Child Adolesc Psychiatry. (1988) 27:430–3. doi: 10.1097/00004583-198807000-00009
223. Roy A, Roy M, Deb S, Unwin G, Roy A. Are opioid antagonists effective in attenuating the core symptoms of autism spectrum conditions in children: a systematic review. J Intellect Disabil Res JIDR. (2015) 59:293–306. doi: 10.1111/jir.12122
224. Pellissier LP, Gandía J, Laboute T, Becker JAJ, Le Merrer J. μ opioid receptor, social behaviour and autism spectrum disorder: reward matters. Br J Pharmacol. (2018) 175:2750–69. doi: 10.1111/bph.13808
225. Le Roy C, Laboureyras E, Gavello-Baudy S, Chateauraynaud J, Laulin J-P, Simonnet G. Endogenous opioids released during non-nociceptive environmental stress induce latent pain sensitization Via a NMDA-dependent process. J Pain. (2011) 12:1069–79. doi: 10.1016/j.jpain.2011.04.011
226. Simonnet G. Physiologie de la douleur et de l’hyperalgésie ou de la nociception à la contagion émotionnelle de la douleur. Douleur Anal. (2016) 29:37–47. doi: 10.1007/s11724-016-0452-5
227. Courtemanche AB, Black WR, Reese RM. The relationship between pain, self-injury, and other problem behaviors in young children with autism and other developmental disabilities. Am J Intellect Dev Disabil. (2016) 121:194–203. doi: 10.1352/1944-7558-121.3.194
228. Halayem S, Charfi N, Touati M, Mrabet A, Bouden A. Sensitivity to pain in autistic spectrum disorders: its links with self-gressivity. Tunis Med. (2018) 96:501–4.
229. Symons FJ. Self-injurious behavior in neurodevelopmental disorders: relevance of nociceptive and immune mechanisms. Neurosci Biobehav Rev. (2011) 35:1266–74. doi: 10.1016/j.neubiorev.2011.01.002
230. Johnson B, Ulberg S, Shivale S, Donaldson J, Milczarski B, Faraone SV. Fibromyalgia, autism, and opioid addiction as natural and induced disorders of the endogenous opioid hormonal system. Discov Med. (2014) 18:209–20.
231. Anugu V, Ringhisen J, Johnson B. Autism case report: cause and treatment of “high opioid tone”. Autism. Front Psychol. (2021) 12:657952. doi: 10.3389/fpsyg.2021.657952
232. Azuine RE, Ji Y, Chang H-Y, Kim Y, Ji H, DiBari J, et al. Prenatal risk factors and perinatal and postnatal outcomes associated with maternal opioid exposure in an urban, low-income, multiethnic US population. JAMA Netw Open. (2019) 2:e196405. doi: 10.1001/jamanetworkopen.2019.6405
233. Rubenstein E, Young JC, Croen LA, DiGuiseppi C, Dowling NF, Lee L-C, et al. Brief report: maternal opioid prescription from preconception through pregnancy and the odds of autism spectrum disorder and autism features in children. J Autism Dev Disord. (2019) 49:376–82. doi: 10.1007/s10803-018-3721-8
234. Lázaro CP, Pondé MP, Rodrigues LEA. Opioid peptides and gastrointestinal symptoms in autism spectrum disorders. Rev Bras Psiquiatr Sao Paulo Braz 1999. (2016) 38:243–6. doi: 10.1590/1516-4446-2015-1777
235. Rueda-Ruzafa L, Cruz F, Cardona D, Hone AJ, Molina-Torres G, Sánchez-Labraca N, et al. Opioid system influences gut-brain axis: dysbiosis and related alterations. Pharmacol Res. (2020) 159:104928. doi: 10.1016/j.phrs.2020.104928
236. Seo M, Anderson G. Gut-amygdala interactions in autism spectrum disorders: developmental roles via regulating mitochondria, exosomes, immunity and microRNAs. Curr Pharm Des. (2019) 25:4344–56. doi: 10.2174/1381612825666191105102545
237. Madra M, Ringel R, Margolis KG. Gastrointestinal issues and autism spectrum disorder. Psychiatr Clin North Am. (2021) 44:69–81. doi: 10.1016/j.psc.2020.11.006
238. Ferguson BJ, Marler S, Altstein LL, Lee EB, Mazurek MO, McLaughlin A, et al. Associations between cytokines, endocrine stress response, and gastrointestinal symptoms in autism spectrum disorder. Brain Behav Immun. (2016) 58:57–62. doi: 10.1016/j.bbi.2016.05.009
239. Qi X-R, Zhang L. The potential role of gut peptide hormones in autism spectrum disorder. Front Cell Neurosci. (2020) 14:73. doi: 10.3389/fncel.2020.00073
240. Bali A, Jaggi AS. An integrative review on role and mechanisms of ghrelin in stress, anxiety and depression. Curr Drug Targets. (2016) 17:495–507. doi: 10.2174/1389450116666150518095650
241. Al-Zaid FS, Alhader AA, Al-Ayadhi LY. Altered ghrelin levels in boys with autism: a novel finding associated with hormonal dysregulation. Sci Rep. (2014) 4:6478. doi: 10.1038/srep06478
242. Pirzadeh S, Sajedianfard J, Aloisi AM, Ashrafi M. Effects of intracerebroventricular and intra-arcuate nucleus injection of ghrelin on pain behavioral responses and met-enkephalin and β-endorphin concentrations in the periaqueductal gray area in rats. Int J Mol Sci. (2019) 20:2475. doi: 10.3390/ijms20102475
243. Harris RE, Clauw DJ. Imaging central neurochemical alterations in chronic pain with proton magnetic resonance spectroscopy. Neurosci Lett. (2012) 520:192–6. doi: 10.1016/j.neulet.2012.03.042
244. Peek AL, Rebbeck T, Puts NA, Watson J, Aguila M-ER, Leaver AM. Brain GABA and glutamate levels across pain conditions: a systematic literature review and meta-analysis of 1H-MRS studies using the MRS-Q quality assessment tool. Neuroimage. (2020) 210:116532. doi: 10.1016/j.neuroimage.2020.116532
245. Ganguly K, Schinder AF, Wong ST, Poo MGABA. Itself promotes the developmental switch of neuronal GABAergic responses from excitation to inhibition. Cell. (2001) 105:521–32. doi: 10.1016/S0092-8674(01)00341-5
246. Wu C, Sun D. GABA receptors in brain development, function, and injury. Metab Brain Dis. (2015) 30:367–79. doi: 10.1007/s11011-014-9560-1
247. Pizzarelli R, Cherubini E. Alterations of GABAergic signaling in autism spectrum disorders. Neural Plast. (2011) 2011:297153. doi: 10.1155/2011/297153
248. Inui T, Kumagaya S, Myowa-Yamakoshi M. Neurodevelopmental hypothesis about the etiology of autism spectrum disorders. Front Hum Neurosci. (2017) 11:354. doi: 10.3389/fnhum.2017.00354
249. Yizhar O, Fenno LE, Prigge M, Schneider F, Davidson TJ, O’Shea DJ, et al. Neocortical excitation/inhibition balance in information processing and social dysfunction. Nature. (2011) 477:171–8. doi: 10.1038/nature10360
250. Horder J, Petrinovic MM, Mendez MA, Bruns A, Takumi T, Spooren W, et al. Glutamate and GABA in autism spectrum disorder—a translational magnetic resonance spectroscopy study in man and rodent models. Transl Psychiatry. (2018) 8:1–11. doi: 10.1038/s41398-018-0155-1
251. Crespi B. Developmental heterochrony and the evolution of autistic perception, cognition and behavior. BMC Med. (2013) 11:119. doi: 10.1186/1741-7015-11-119
252. Gaetz W, Bloy L, Wang DJ, Port RG, Blaskey L, Levy SE, et al. GABA estimation in the brains of children on the autism spectrum: measurement precision and regional cortical variation. Neuroimage. (2014) 86:1–9. doi: 10.1016/j.neuroimage.2013.05.068
253. Puts NAJ, Wodka EL, Harris AD, Crocetti D, Tommerdahl M, Mostofsky SH, et al. Reduced GABA and altered somatosensory function in children with autism spectrum disorder. Autism Res. (2017) 10:608–19. doi: 10.1002/aur.1691
254. Robertson CE, Ratai E-M, Kanwisher N. Reduced GABAergic action in the autistic brain. Curr Biol CB. (2016) 26:80–5. doi: 10.1016/j.cub.2015.11.019
255. Nakamura T, Sakaue F, Nasu-Nishimura Y, Takeda Y, Matsuura K, Akiyama T. The autism-related protein PX-RICS mediates GABAergic synaptic plasticity in hippocampal neurons and emotional learning in mice. eBioMedicine. (2018) 34:189–200. doi: 10.1016/j.ebiom.2018.07.011
256. Zhang L, Huang C-C, Dai Y, Luo Q, Ji Y, Wang K, et al. Symptom improvement in children with autism spectrum disorder following bumetanide administration is associated with decreased GABA/glutamate ratios. Transl Psychiatry. (2020) 10:9. doi: 10.1038/s41398-020-0692-2
257. Sapey-Triomphe L-A, Lamberton F, Sonié S, Mattout J, Schmitz C. Tactile hypersensitivity and GABA concentration in the sensorimotor cortex of adults with autism. Autism Res Off J Int Soc Autism Res. (2019) 12:562–75. doi: 10.1002/aur.2073
258. Umesawa Y, Atsumi T, Chakrabarty M, Fukatsu R, Ide MGABA. Concentration in the left ventral premotor cortex associates with sensory hyper-responsiveness in autism spectrum disorders without intellectual disability. Front Neurosci. (2020) 14:482. doi: 10.3389/fnins.2020.00482
259. Zou S, Kumar U. Cannabinoid receptors and the endocannabinoid system: signaling and function in the central nervous system. Int J Mol Sci. (2018) 19:833. doi: 10.3390/ijms19030833
260. Elphick MR, Egertová M. The neurobiology and evolution of cannabinoid signalling. Philos Trans R Soc Lond B Biol Sci. (2001) 356:381–408. doi: 10.1098/rstb.2000.0787
261. Wei D, Allsop S, Tye K, Piomelli D. Endocannabinoid signaling in the control of social behavior. Trends Neurosci. (2017) 40:385–96. doi: 10.1016/j.tins.2017.04.005
262. Guerrero-Alba R, Barragán-Iglesias P, González-Hernández A, Valdez-Moráles EE, Granados-Soto V, Condés-Lara M, et al. Some prospective alternatives for treating pain: the endocannabinoid system and its putative receptors GPR18 and GPR55. Front Pharmacol. (2018) 9:1496. doi: 10.3389/fphar.2018.01496
263. Carbone E, Manduca A, Cacchione C, Vicari S, Trezza V. Healing autism spectrum disorder with cannabinoids: a neuroinflammatory story. Neurosci Biobehav Rev. (2021) 121:128–43. doi: 10.1016/j.neubiorev.2020.12.009
264. Krueger DD, Brose N. Evidence for a common endocannabinoid-related pathomechanism in autism spectrum disorders. Neuron. (2013) 78:408–10. doi: 10.1016/j.neuron.2013.04.030
265. Aran A, Eylon M, Harel M, Polianski L, Nemirovski A, Tepper S, et al. Lower circulating endocannabinoid levels in children with autism spectrum disorder. Mol Autism. (2019) 10:2. doi: 10.1186/s13229-019-0256-6
266. Fusar-Poli L, Cavone V, Tinacci S, Concas I, Petralia A, Signorelli MS, et al. Cannabinoids for people with ASD: a systematic review of published and ongoing studies. Brain Sci. (2020) 10:E572. doi: 10.3390/brainsci10090572
267. Schultz S, Siniscalco D, Schultz S, Siniscalco D. Endocannabinoid system involvement in autism spectrum disorder: an overview with potential therapeutic applications. AIMS Mol Sci. (2019) 6:27–37. doi: 10.3934/molsci.2019.1.27
268. Su T, Yan Y, Li Q, Ye J, Pei L. Endocannabinoid system unlocks the puzzle of autism treatment via microglia. Front Psychiatry. (2021) 12:734837. doi: 10.3389/fpsyt.2021.734837
269. Pretzsch CM, Freyberg J, Voinescu B, Lythgoe D, Horder J, Mendez MA, et al. Effects of cannabidiol on brain excitation and inhibition systems; a randomised placebo-controlled single dose trial during magnetic resonance spectroscopy in adults with and without autism spectrum disorder. Neuropsychopharmacology. (2019) 44:1398–405. doi: 10.1038/s41386-019-0333-8
270. Pretzsch CM, Voinescu B, Lythgoe D, Horder J, Mendez MA, Wichers R, et al. Effects of cannabidivarin (CBDV) on brain excitation and inhibition systems in adults with and without autism spectrum disorder (ASD): a single dose trial during magnetic resonance spectroscopy. Transl Psychiatry. (2019) 9:313. doi: 10.1038/s41398-019-0654-8
271. Pretzsch CM, Voinescu B, Mendez MA, Wichers R, Ajram L, Ivin G, et al. The effect of cannabidiol (CBD) on low-frequency activity and functional connectivity in the brain of adults with and without autism spectrum disorder (ASD). J Psychopharmacol Oxf Engl. (2019) 33:1141–8. doi: 10.1177/0269881119858306
272. Wright KL, Duncan M, Sharkey KA. Cannabinoid CB2 receptors in the gastrointestinal tract: a regulatory system in states of inflammation. Br J Pharmacol. (2008) 153:263–70. doi: 10.1038/sj.bjp.0707486
273. Aran A, Cassuto H, Lubotzky A. Cannabidiol based medical cannabis in children with autism– a retrospective feasibility study (P3.318). Neurology. (2018) 90:200. doi: 10.1007/s10803-018-3808-2
274. Aran A, Harel M, Cassuto H, Polyansky L, Schnapp A, Wattad N, et al. Cannabinoid treatment for autism: a proof-of-concept randomized trial. Mol Autism. (2021) 12:6. doi: 10.1186/s13229-021-00420-2
275. Barchel D, Stolar O, De-Haan T, Ziv-Baran T, Saban N, Fuchs DO, et al. Oral cannabidiol use in children with autism spectrum disorder to treat related symptoms and co-morbidities. Front Pharmacol. (2018) 9:1521. doi: 10.3389/fphar.2018.01521
276. Bar-Lev Schleider L, Mechoulam R, Saban N, Meiri G, Novack V. Real life experience of medical cannabis treatment in autism: analysis of safety and efficacy. Sci Rep. (2019) 9:200. doi: 10.1038/s41598-018-37570-y
277. Hadjistavropoulos T, Craig KD. A theoretical framework for understanding self-report and observational measures of pain: a communications model. Behav Res Ther. (2002) 40:551–70. doi: 10.1016/S0005-7967(01)00072-9
278. Mitchell P, Sheppard E, Cassidy S. Autism and the double empathy problem: implications for development and mental health. Br J Dev Psychol. (2021) 39:1–18. doi: 10.1111/bjdp.12350
279. Katusic MZ, Myers SM, Weaver AL, Voigt RG. IQ in autism spectrum disorder: a population-based birth cohort study. Pediatrics. (2021) 148:e2020049899. doi: 10.1542/peds.2020-049899
280. Wright B, Spikins P, Pearson H. Should autism spectrum conditions be characterised in a more positive way in our modern world? Medicina (Mex). (2020) 56:233. doi: 10.3390/medicina56050233
281. Wood JJ, Drahota A, Sze K, Har K, Chiu A, Langer DA. Cognitive behavioral therapy for anxiety in children with autism spectrum disorders: a randomized, controlled trial. J Child Psychol Psychiatry. (2009) 50:224–34. doi: 10.1111/j.1469-7610.2008.01948.x
282. Bai D, Yip BHK, Windham GC, Sourander A, Francis R, Yoffe R, et al. Association of genetic and environmental factors with autism in a 5-country cohort. JAMA Psychiatry. (2019) 76:1035–43. doi: 10.1001/jamapsychiatry.2019.1411
283. Sener EF, Sahin MC, Taheri S, Bayramov KK, Marasli MK, Zararsiz G, et al. A preliminary study of the genes related to aggression and insensitivity to pain in autism spectrum disorders. Psychiatry Clin Psychopharmacol. (2017) 27:24–9. doi: 10.1080/24750573.2017.1293248
284. Sener EF, Taheri S, Sahin MC, Bayramov KK, Marasli MK, Zararsiz G, et al. Altered global mRNA expressions of pain and aggression related genes in the blood of children with autism spectrum disorders. J Mol Neurosci MN. (2019) 67:89–96. doi: 10.1007/s12031-018-1213-0
285. Simons SHP, Tibboel D. Pain perception development and maturation. Semin Fetal Neonatal Med. (2006) 11:227–31. doi: 10.1016/j.siny.2006.02.010
286. Fitzgerald M, Beggs S. The neurobiology of pain: developmental aspects. Neuroscientist. (2001) 7:246–57. doi: 10.1177/107385840100700309
287. Kross E, Berman MG, Mischel W, Smith EE, Wager TD. Social rejection shares somatosensory representations with physical pain. Proc Natl Acad Sci USA. (2011) 108:6270–5. doi: 10.1073/pnas.1102693108
288. Eisenberger NI. The pain of social disconnection: examining the shared neural underpinnings of physical and social pain. Nat Rev Neurosci. (2012) 13:421–34. doi: 10.1038/nrn3231
Keywords: pain, autism, perception, reaction, evaluation, expression
Citation: Bogdanova OV, Bogdanov VB, Pizano A, Bouvard M, Cazalets J-R, Mellen N and Amestoy A (2022) The Current View on the Paradox of Pain in Autism Spectrum Disorders. Front. Psychiatry 13:910824. doi: 10.3389/fpsyt.2022.910824
Received: 01 April 2022; Accepted: 17 June 2022;
Published: 22 July 2022.
Edited by:
Khaled Saad, Assiut University Hospital, EgyptReviewed by:
Catherine Caldwell-Harris, Boston University, United StatesIslam Aud, Al-Azhar University, Egypt
Copyright © 2022 Bogdanova, Bogdanov, Pizano, Bouvard, Cazalets, Mellen and Amestoy. This is an open-access article distributed under the terms of the Creative Commons Attribution License (CC BY). The use, distribution or reproduction in other forums is permitted, provided the original author(s) and the copyright owner(s) are credited and that the original publication in this journal is cited, in accordance with accepted academic practice. No use, distribution or reproduction is permitted which does not comply with these terms.
*Correspondence: Olena V. Bogdanova, olena.bogdanova@u-bordeaux.fr