- 1Institute of Biological Sciences, Federal University of Goiás, Goiania, GO, Brazil
- 2Department of Physiology, Institute of Biomedical Sciences, Federal University of Uberlândia, Uberlândia, Brazil
- 3Department of Physiology and Pathology, School of Dentistry, São Paulo State University (UNESP), Araraquara, Brazil
- 4Graduate Program in Pharmaceutical Sciences, Campus Arthur Wesley Archibald, Evangelical University of Goiás, Anápolis, Brazil
Post-partum depression (PPD) with varying clinical manifestations affecting new parents remains underdiagnosed and poorly treated. This minireview revisits the pharmacotherapy, and relevant etiological basis, capable of advancing preclinical research frameworks. Maternal tasks accompanied by numerous behavioral readouts demand modeling different paradigms that reflect the complex and heterogenous nature of PPD. Hence, effective PPD-like characterization in animals towards the discovery of pharmacological intervention demands research that deepens our understanding of the roles of hormonal and non-hormonal components and mediators of this psychiatric disorder.
1. Introduction
The development of psychological disorders is associated with demanding physical and psychological changes during pregnancy and the postpartum period (1). Childbirth often increases the risk of mental disorders as well as higher rates of hospitalization and psychiatric treatment (2, 3). The global impact of post-partum depression (PPD) has been estimated around 20% of women at peripartum – during pregnancy or post-partum period (4). The plethora of clinical manifestations in patients with PPD includes emotional tension, mood swing, guilt, agitation, insomnia, irritability, anxiety, depressive disorder, and confusion characterized by withdrawal, intrusiveness, hostility, and non-response to infant’s cues (5, 6). The infant may later suffer from the impairment of the cognitive performance, executive function, intelligence, and language development (7, 8). Poor maternal–infant bonding, impaired development, infanticide, child neglect, and negative neurodevelopment and behavior, as well as suicide tendencies, may result from untreated PPD (5, 9, 10). Stressful life events, history of psychiatric illness, drug abuse, low levels of social or partner support, unexpected pregnancy or complications, and genetic susceptibility are among PPD’s risk factors (11, 12).
A population-based cohort study has revealed the first-time experience of psychiatric disorder in many women with PPD (13). High rates of manic-depressive, obsessive–compulsive, post-traumatic stress, eating, bipolar and pain disorders often increase the recurrence risk of the post-partum episode (14–16). Complex clinical manifestations or comorbidity of PPD with other disorders pose challenges to the accurate assessment of its prevalence. A set of cross-cultural variables, differences in the perception of mental health and its stigma, differences in environmental and socioeconomic contexts remain compounding factors (17, 18).
Historically, mental illnesses associated with childbirth were considered a discrete disease entity, a toxic-confusional or delirious picture, puerperal disorders, post-partum psychoses, baby blues, puerperal fever, or milk fever (19–23). The PPD was first described with the expression “if the womb is too moist, the brain is filled with water, and the moisture running over the eyes, compels them to involuntarily shed tears” in the 13th century or thereabout (24). With years of conceptual descriptions marked by little consensual breakthroughs on the criterion for PPD, the fifth edition-text revision of the Diagnostic and Statistical Manual of Mental Disorders (DSM-V-TR) considers PPD as a major depressive disorder (MDD) of peripartum onset. Distinguishing between MDD and PDD is difficult, for example, about 80% of PPD cases are relapses of MDD; however, particularly in PPD there are gonadal hormonal changes that accompany delivery - dramatic elevation of hormones during pregnancy that drop dramatically after delivery (25). The symptoms of PPD are often identified within the first 4 weeks of delivery up to 12 months (26, 27). Early detection, proper diagnosis, and treatment are key to preventing symptom exacerbation (28). Low mood in the early postpartum period which is largely deemed “normal” with 50–80% of new mothers often makes early intervention against PPD elusive (29, 30).
Hence, leveraging etiological factors of PPD and emotional burden exacerbation to preclinical cues could engender early intervention and benefit at-risk mothers. In this manner, this minireview revisits available pharmacological interventions, etiological hypotheses of PPD, key mediators, parameters, and preclinical framework for effective screening of drugs.
2. Pharmacological approaches and implications
Diverse mediators of PPD that are involved in complex pathophysiological changes provide support for hormonal and non-hormonal pharmacological interventions. The current use of typical antidepressants to treat PPD suffers from limited evidence of safety and efficacy coupled with suboptimal outcomes (31, 32). Psychotherapy or antidepressants was recommended by the American Psychiatric Association as first-line treatment for mild-to-moderate PPD (33). The monoamine-based medication which sometimes comes with the risk of teratogenicity, neonatal toxicity, and/or long-term developmental impact is widely used as the first-line treatment (Table 1). Some of them are selective serotonin reuptake inhibitors (SSRIs), serotonin and norepinephrine reuptake inhibitors (SNRIs), and tricyclic antidepressants (TCA). The worrisome infant exposure to these conventional antidepressants throughout perinatal and breastfeeding periods (40–42) should ideally limit their administration in patients. According to Deligiannidis et al. in 2021, none of these monoaminergic antidepressants have specific indications for PPD (43).
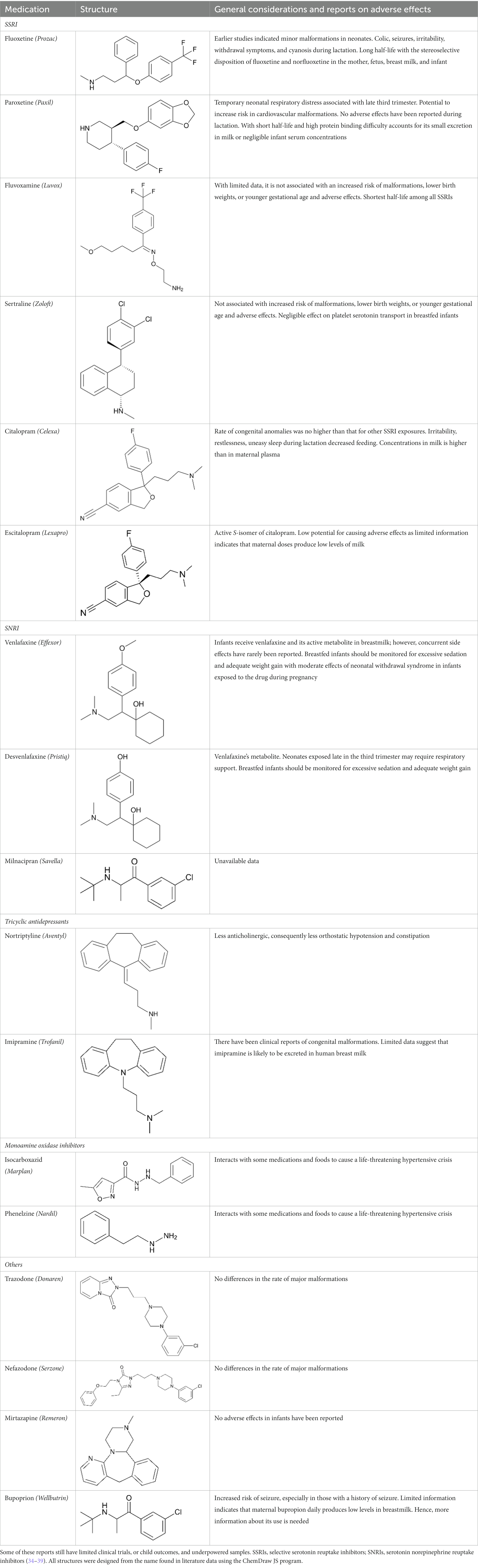
Table 1. General considerations and reports on antidepressant-induced adverse effects during pregnancy and lactation.
The etiological implication of the GABAergic system (44) through allopregnanolone [3α, 5α-tetrahydroprogesterone; a modulator of γ-aminobutyric acid (GABA) A receptor] provides additional insights into the drug development program for PPD. Negative feedback involving this neuroactive steroid rapidly suppresses neuronal excitability and HPA axis responses to postnatal stress to restore homeostasis (45, 46). Besides allopregnanolone, 3α, 5β-tetrahydro progesterone (pregnanolone), 5α, 3α-tetrahydrodeoxycorticosterone, pregnenolone, progesterone, and deoxycorticosterone are important neuroactives of pharmacological interest. The hypothesis supporting neurosteroids and GABAergic transmission in PPD favors clinical applications of synthetic allopregnanolone (brexanolone) and the development of zuranolone now in the third phase of the clinical trial (1). Brexanolone induces a positive allosteric modulation of GABA receptors. The activation of these receptors prior to chloride ion influx reduced the depression score. In addition, possible impairment of episodic memory has been reported in some women receiving intravenous brexanolone (47). Reducing GABA signaling has been suggested to precede exacerbated glutamatergic transmission that contributes to depressive phenotype. The approval of ketamine, a non-competitive NMDA receptor antagonist, for treatment-resistant depressive patients can support the idea of a promising effect on PPD (48, 49). Furthermore, the neurosteroid-induced GABA A receptor (GABAAR) modulation of the hypothalamic–pituitary-gonadal axis (50) provides a clue on the reduction in the plasma levels of luteinizing and follicle-stimulating hormone without estradiol or progesterone alteration. Hence, direct activation of GABA receptors plays important role in hormonal oscillations and regulations.
Cortisol, corticotropin-releasing hormone (CRH), adrenocorticotropic hormone (ACTH), oxytocin, prolactin, testosterone, and/or estradiol provide important pharmacological clues. Transdermal estrogen and estradiol are considered the effective treatment of depressive episodes in women with PPD or perimenopause. As the outcome of estradiol treatment raised expectations (51, 52), a transdermal estrogen for severe PPD treatment elicited more rapid improvement in depressive symptoms using the Edinburg Postnatal Depression Scale (53). However, the effectiveness and acceptability of these two treatment protocols vary among patients. The administration and abrupt withdrawal of a high dose of estradiol and progesterone during ovarian suppression in euthymic non-pregnant women increased depressive symptoms in women with a history of PPD (54). The leuprolide-induced hypogonadal post-partum hormonal changes simulation was reversed among 62% of the women with a history of PPD by supraphysiological doses of estradiol and progesterone (55). Altogether, these results reiterate how relevant hormonal targets are key to the understanding of meaningful pharmacological intervention in drug screening and development programs.
3. Etiological understanding and relevant mediators
The behavioral cues and neurobiological aspects suggestive of depressive signs, sleep, and cognitive disruptions, and hormonal and non-hormonal alterations are important components of PPD (Figure 1). Some of these etiological components of PPD and infant suffering are the outcome of maternal exposure to stress. For instance, stress-induced reduction in circulating allopregnanolone at birth could in turn reduce its production in the neonate’s brain with far-reaching childhood adversity and multigenerational consequences (56). The stressful and intense demands of caring for a newborn are often accompanied by psychological, social, and biological factors (26, 57). Qualitative and quantitative analysis of hormonal and non-hormonal input to PPD mediated by stress is pertinent (Figure 2) as stress adaptation and resilience instinct affect the perception of uncontrollable/unpredictable events from the ones that are or become controllable/predictable. Hypothetically, stress-induced suppression of the inherent rewarding component of the maternal-newborn buffering bond could drive depression and behavioral deficits because of the disruptions of the maternal resilience and recovery system. The psychosocial hypothesis supports PPD being an endpoint of excessive worry and abrupt perinatal changes in the immunity and physiological state emanating from adapting to the maternal role and life with a new infant (57, 58). Psychosocial predictors impacting women’s resilience and mental health recovery seem to be associated with genetic components as supported by family and twin studies (59–61). These studies among others identified relevant genes that are associated with depressive episodes (60–62).
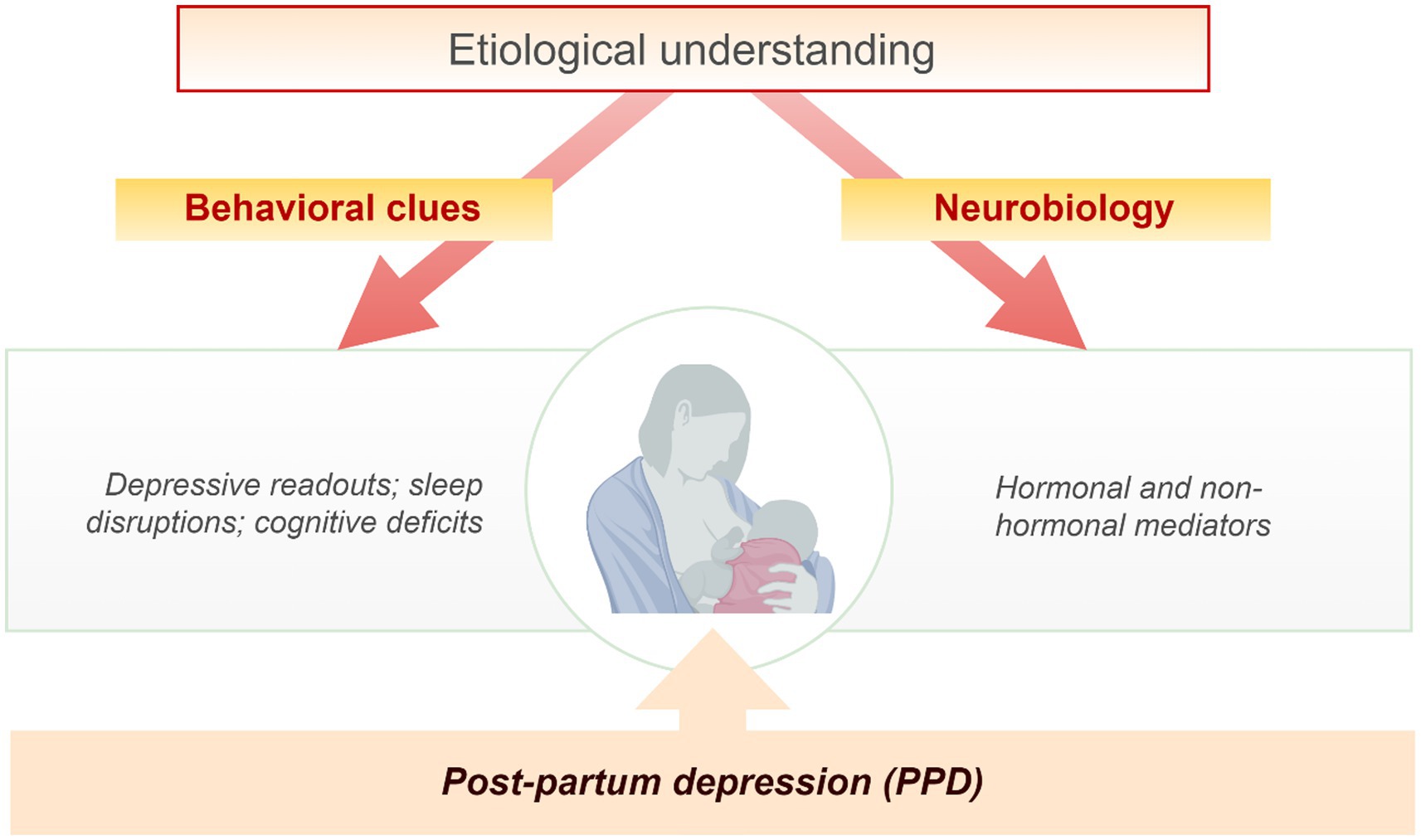
Figure 1. Etiological understanding of post-partum depression (PPD). The behavioral clues and neurobiological aspects suggestive of depressive signs, sleep, and cognitive disruptions, hormonal and non-hormonal alterations are important components of PPD etiology.
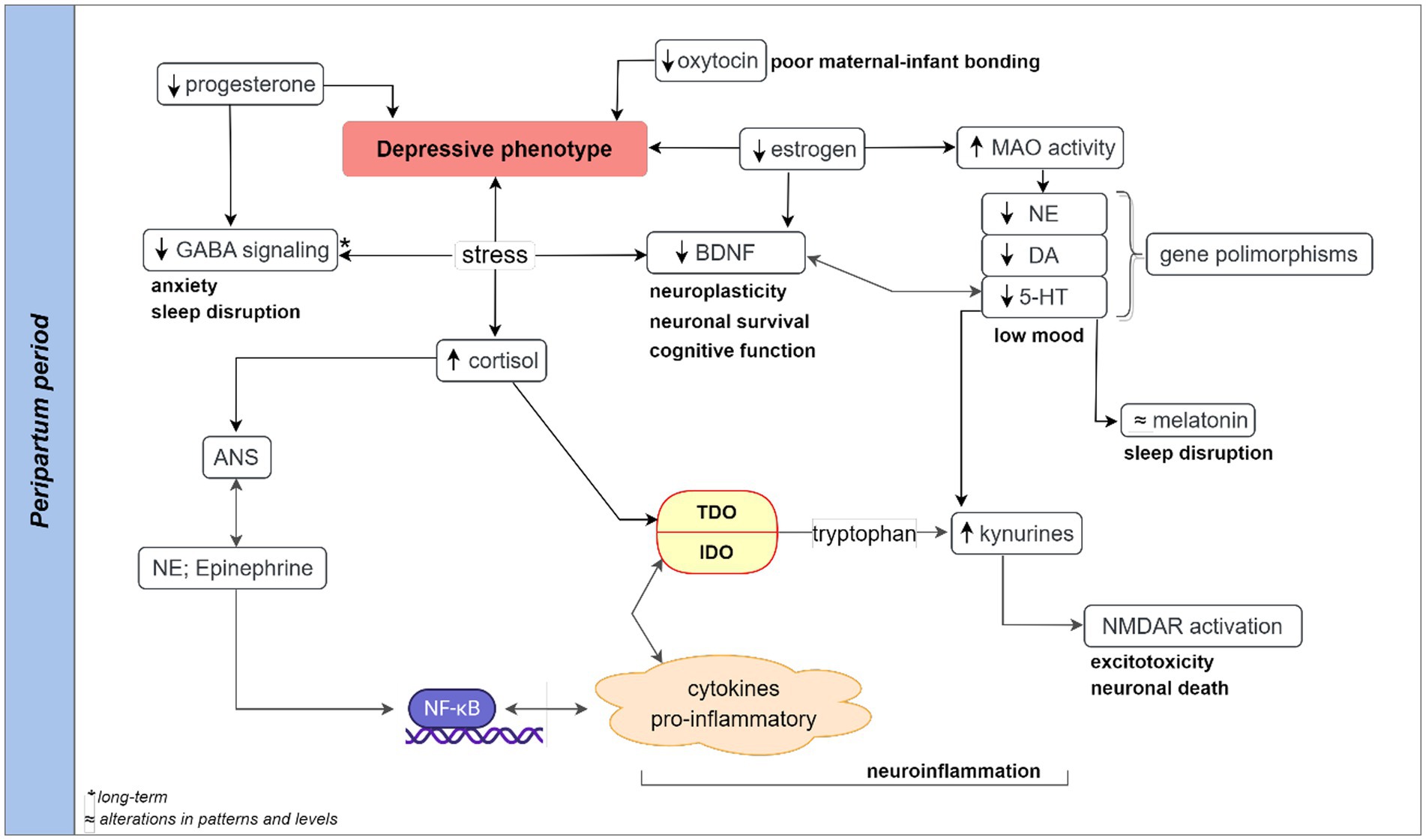
Figure 2. Hypothetical pathways contributing to peripartum depressive readouts. Stress-induced activation of the HPA axis could lead to a chain of events involving cortisol release and autonomic nervous system (ANS) release of catecholamines. Both HPA and ANS are highly coordinated and interconnected with the overall impact on neuroendocrine (reduced levels of progesterone, oxytocin, and estrogen), monoamines (norepinephrine, serotonin, and dopamine), and neuroinflammation (increased level of inflammatory cytokines) at the peripartum period. Long-term stress and reduced progesterone may lead to decreased GABAergic signaling, contributing to peripartum sleep disruption and anxiety. A high level of stress-induced immune-inflammatory activity could shift tryptophan-serotonin-melatonin metabolism to metabolites-driven kynurenine pathway. The downregulation of serotonin and melatonin synthesis coupled with the upregulation of kynurenic and quinolinic acid contribute to the depressive phenotype. The alterations in the tryptophan-kynurenine pathway can lead to excessive activation of N-Methyl-D-Aspartate receptor, inflammation, oxidative stress responses, and excitotoxicity leading to cellular damage, and depressive readouts in postpartum depression. A monoamine-lowering process with genetic polymorphism or a dramatic elevation of MAO-A following estrogen decline could potentiate stress-induced BDNF reductions increase the risk of neuronal damage and low mood. 5-HT, serotonin; ANS, autonomous nervous system; BDNF, brain-derived neurotrophic factor; DA, dopamine; GABA, gamma aminobutyric acid; HPA, hypothalamic–pituitary–adrenal; IDO, indoleamine 2,3-dioxygenase enzyme; MAO, Monoamine oxidase enzyme; NE, norepinephrine; NF-κB, nuclear factor kappa B; NMDAR, N-Methyl-D-Aspartate receptor; TDO, tryptophan 2,3-dioxygenase enzyme.
Genetic variants in hormones, transporters, receptors, and metabolic enzymes contributing to behavioral changes have been detected and analyzed during the post-partum period. Genetic alterations in estradiol, oxytocin, glucocorticoid, and CRH, estrogen receptors, and maternal gene expression across multiple brain regions with altered immune transcriptomic landscape and activation were associated with PPD (63–68). The variants of the serotonin transporter (SLC6A4; SERT), dopa decarboxylase, and protein kinase C beta play roles in stressful life events, depression, and PPD onset (69–72). High expression and polymorphism of SERT that are more reactive to environmental stressors (5-HTTLPR and STIN2-VNTR), 5-HT2A receptor, tryptophan hydroxylase (TPH), neurotrophic factors, Val158Met COMT or MAO-A, as well as tryptophan depletion, could contribute to a net decrease in brain 5-HT bioavailability prior to depressive phenotype (69–73).
Physiological adaptations required for gestation undoubtedly involve neuroendocrine fluctuations at different perinatal phases (74) and may converge to set the mother’s mental health state (75, 76). This may be part of a bidirectional feedback loop settled on some hormonal axes during the peripartum period that results in persistent anxiety and depression in some women (77, 78). Over the years depressive episodes have increased research hypotheses on monoaminergic neurotransmission. Monoamines seem to be affected by hormonal changes, as revealed by the substantial modification in monoamine oxidase kinetics through a short postpartum timeframe. Robust and accelerated degradation of neurotransmitters in PPD (79) supports monoamine involvement. Estrogen has a neuroprotective role, reduces inflammatory responses, also, can improve serotonin function (25). The monoamine-lowering process with a dramatic elevation of MAO-A and simultaneous estrogen decline during the first week of postpartum (79, 80), as well as tryptophan depletion or tyrosine hydroxylase inhibition (81) are all connected to low mood and stress-induced immune-inflammatory (proinflammatory cytokines) components of peripartum changes leading to PPD (Figure 2). For instance, a report showed varying peripartum tryptophan with lower plasma concentration in the second and third trimesters as compared to the first trimester, and gradual postpartum restoration after 6 weeks (82).
Additionally, sleep disruptions and cognitive decline involving complex endocrinal and psychological interplays are important etiological manifestations in PPD patients (83–89). Cognitive dysfunction may be an early symptom of a depressive episode (89, 90). The sleep initiation, maintenance, and circadian rhythm prior to depressive manifestations and/or cognitive deficits implicate alterations in melatonin levels (91, 92), estrogen, and progesterone (90, 93–101). Besides, a high level of stress-induced immune-inflammatory activity could shift tryptophan-serotonin-melatonin metabolism to a metabolites-driven kynurenine pathway (102, 103). This is followed by a significant reduction of serotonin, a substrate for the synthesis of melatonin precursor N-acetylserotonin, at mid-late pregnancy and the early postpartum period (104). The downregulation of serotonin and melatonin synthesis coupled with the upregulation of kynurenic and quinolinic acid contribute to depressive phenotype (105, 106). The alterations in the tryptophan-kynurenine pathway can lead to excessive activation of NMDARs, lipid peroxidation, autoimmune, inflammation, and oxidative stress responses underlining cellular damage, and PPD (5, 107–112).
Complex interactions of kynurenine, gut microbiota, and inflammatory and hormonal mediators (113) provide additional important targets in PPD. Although both indoleamine 2,3-dioxygenase (IDO) and tryptophan 2,3-dioxygenase (TDO) convert tryptophan into kynurenine, the IDO-induced tryptophan conversion contributes to maternal-fetal immune tolerance in placental villi (protecting the fetus from maternal T-lymphocyte and natural killer cell attack during pregnancy) (114) unlike TDO that is primarily induced by the stress hormone. The IDO is induced by gamma-interferon (INF-γ), and other pro-inflammatory cytokines (5). The involvement of gut microbiota in tryptophan and serotonin metabolism provides additional insight into the role of kynurenic acid in PPD. The release of pro-inflammatory cytokines could be exacerbated by the vicious cycle of the stress-induced release of catecholamine (norepinephrine and epinephrine) and activation of the nuclear factor-kappa-beta (NF-kB) cascade (Figure 2).
The modification of autonomic and neuroendocrine reactivity (115) in response to infant cries and odors may drive maternal hyper-reaction (116). While a study associated increases in stress hormone levels with the modification of a mother’s mental health (117), an alteration in the hypothalamic–pituitary–adrenal (HPA) axis around the third month of pregnancy could boost positive feedback involving placental CRH (118). The abrupt withdrawal of placental CRH a couple of days from delivery towards the re-establishment of the maternal HPA axis could contribute to the PPD phenotype (119). Therefore, peripartum HPA screening may pave the way for new PPD pharmacotherapy interventions.
In addition, a 3-4-fold increase in basal serum oxytocin (implicated in well-being and social interaction) during pregnancy (120) prior to pulsatile increase during labor (121) seems to support the pathophysiological role of the central oxytocinergic target. The sudden reductions in oxytocin levels following the delivery in PPD (122) constitute an important hypothetical mediator. The report showed that higher oxytocin levels induced by serotonin-targeted antidepressant drugs improved cognitive performance (123) and parenting (124). This finding supports cross-communication between oxytocinergic, and serotoninergic pathways often impact other systems. The synergistic effects of the serotonin system and brain-derived neurotrophic factor (BDNF) modulate neuronal development and plasticity (125). Of note, N-acetylserotonin mimics BDNF activating TrkB (126). Hence, a decrease in serotonin may lower TrkB activation. Stress-induced BDNF reductions might cause neuronal damage, which would in turn heighten biological vulnerability to PPD behavioral phenotypes (127).
According to the neurotrophic hypothesis, the altered activity of neurotrophins (NTs) or their receptors plays a well-defined role in depression (128–130). The nerve growth factor (NGF) and BDNF which regulates neuronal growth and survival (128, 131) play important roles during pregnancy in the process of placental angiogenesis and maturation (132, 133). After delivery, NTs continue to perform functions in both the baby and the mother. Reduction in the level of these growth factors seems to contribute to PPD. Although lower serum BDNF levels after delivery were reported in women with PPD as compared to others without PPD (134, 135), an author suggested that a marked decrease in BDNF serum levels before and after childbirth alone did not sufficiently predict PPD (104).
Throughout the perinatal period, considerable declines in BDNF occur from the first to the third trimester prior to post-partum increase (136). Decreasing levels throughout pregnancy may, in part, reflect the use of maternal BDNF by the placenta and fetus (137). An inverse relationship between BDNF and depressive symptoms as observed in the third trimester suggests that late pregnancy is the period of greatest vulnerability to BDNF-induced depression (136). Altogether, some of these reports perhaps reflect the etiological complexity of PPD.
4. Update on preclinical assessments of PPD in vivo
As pharmacological treatment of PPD is still shrouded by limited therapeutic evidence of available antidepressants, rodent models of are fundamental to drug research and effective understanding of the neurobiology of this disorder. The assessment of PPD in animals constitutes a great challenge considering the concept of the validity of pharmacological intervention producing a similar effect in both humans and animals (predictive), the similarity of the behavioral readout or phenotype of animals to the disease phenotype being assessed in human (face validity) as well as the correlation between the underlying genetic or cellular mechanisms that result in psychological dysfunction in the animal model and in the human population (construct validity) as earlier reported (138).
In laboratory animals, pregnancy, childbirth, and lactation often induce profound physiological, neuroendocrine, and behavioral changes. The neurobiological advancement and pharmacological screening of new drugs demand improved profiling of PPD-like readouts with appropriate investigational tools in animal settings (40). A manifold of temporal peripartum cellular and molecular machinery underlining tension, mood swings, irritability, anxiety, and depression make preclinical profiling of behavioral manifestations to be herculean. Comparative assessments of behavior before and after pregnancy particularly maternal interactions with the newborn are important. The highly validated forced swimming, tail suspension, open field (139), elevated plus maze, light–dark box, and sucrose preference tests are among the important set-up for behavioral profiling.
The development of depressive-like responses and its correlation with the reductions in the level of norepinephrine, dopamine, serotonin, and intermediates in the striatum (care-giving), hippocampus (cognitive function), and hypothalamus (maternal care & eating behavior) in female BALB/c during the pregnancy and post-partum periods was used to establish PPD-like behavior (139). As PPD may be associated with alterations in the level of neurotransmitters, preclinical screening of drug targeting normalization of these alterations may be a promising candidate for the treatment of PPD. In previous studies, the withdrawal of estradiol and progesterone resulted in an increase in immobility and a decrease in forced swimming (140, 141). According to Frye and Walf, intra-amygdala administration of progesterone increased the entries in the central area of the open field, increased the time spent in the open arms of the elevated plus-maze, and decreased the freezing time after foot shock (antiaversive or antianxiety-like effect) (142). All these experimental manipulations could alter neuronal ensembles and impact maternal tasks.
The hormonal oscillations (progesterone, estradiol, prolactin, oxytocin among others) that reorganize the brain make drug injections into different brain structures an interesting preclinical procedure for behavioral profiling of despair, anhedonia, anxiety, and negative affect. Chemical injections and experimental lesions of different brain regions (medial preoptic area, ventral tegmental area, nucleus accumbens, and arcuate nucleus) in rodents could abolish the onset of maternal behaviors, motivation network, nursing behaviors, lactation (143, 144). These experimental interventions that disrupt endocrine and neurotransmitters communication such as monoamines are important preclinical strategies. Monoamine dysregulation especially in mesocorticolimbic structures contributes to parenting deficits and maternal care (145). Parenting tasks as measured with lactations, dams’ latency to approach the pups, total dam-pup time spent in contact measured as indices of maternal behavior, licking/grooming, active nursing, nest building, self-grooming, pups out of the nest, climbing/digging, pup retrieval, handling, crouching over pups could provide complementary cues to depressive-like behavior. Disruption of maternal tasks could provide insight into postpartum-related emotional disturbances. Lactating animals and humans have a diminished physiologic reactivity to stressors (146), increased calmness, and nurturing behavior that maintains milk quality and quantity (147). Different behavioral repertoires directed toward pups are exhibited by lactating animals with suppressed HPA responses (148). After lactation stress responses or HPA reactivity are downregulated as cortisol, glucose, ACTH, and prolactin levels return to normal (148, 149). Grooming and rearing behaviors during lactation which are indicative of increased HPA activity could be measured indirectly through stress-induced anxiety and depressive phenotypes.
As estrogen directly stimulates CRH production prior to ACTH secretion (150), its low levels during lactation may therefore suppress the hypothalamic release of CRH (146). Hence, phasic hormonal (prolactin, oxytocin, estrogen, and progesterone) levels during pregnancy, at birth, and after lactation (150) could provide important cues to behavioral changes. A high level of oxytocin being maintained throughout lactation and emotional stress may be an important parameter for measuring the antistress nature of lactation. The oxytocin-induced amnesia, anxiolysis, sedation, antinociception, lower blood pressure, decreased corticosteroid levels, and increased vagal activity (34, 146) imply systemic effects with the involvement of a wide range of mediators. An additional intervention like immobilization (stressor) of these lactating animals that elevated plasma catecholamines (epinephrine and norepinephrine) (35, 146) could be explored in preclinical settings and that could explain the role of the autonomic nervous system in PPD as shown in the hypothetic Figure 2.
The analysis of maternal behavior, impulsive responses, and consummatory behavior could correlate with hormonal oscillations. The estrogen and oxytocin modulation have been associated with maternal licking, grooming, pup gathering, and nest building in rodents (36). As estrogen levels decrease, impulsive behavior increases (37). An increase in food intake and a decrease in locomotor activity in certain female rodents following ovariectomy and the subsequent depletion of estradiol and progesterone leading to weight gain support hormonal regulation of eating behavior (38). Cyclic elevations in estradiol could modulate dopamine levels in the prefrontal cortex prior to changes in impulsive behavior. Progesterone suppression of depression-like behaviors, motor, and cognitive deficits have been associated with neuroprotection that is independent of changes in general motor coordination, pain threshold, or plasma corticosterone levels (39, 151, 152).
Altogether, drug stimulation or blockade of different brain regions as well as knockout models, lesion, or tissue removal could provide important neurobiological clues towards the screening of drug candidates. The knockout mice model with Gabrd gene (encoding GABAAR δ subunit) silencing was explored to establish abnormal peripartum GABAAR-induced neuroplasticity and tonic inhibition (40, 153). These mice exhibited depressive-and anxiety-like phenotypes in the postpartum period complementing the increased depressive symptoms following post-partum inhibition of progesterone metabolism (44). The perinatal cognitive impairment (90), and reduced memory processing speed deficits during late pregnancy and after childbirth could be detected using step down passive avoidance test. Ovariectomized rodents instrumented with a single bipolar stimulating electrode directed into the lateral hypothalamus could be used to study anhedonia among other behavioral manifestations. Additionally, the brain-gut-microbiome axis or pre-pregnancy exposure to stress (154), whole-cell patch-clamp recording change in synaptic receptor function, pools, and plasticity could provide meaningful mechanistic data. Considering the temporal nature of peripartum changes, neuroimaging, and real-time monitoring represent promising technology for examining patterns of hormonal and neurochemical changes that discriminate PPD from physiological changes or other depressive-like episodes.
5. Final considerations
Despite the current knowledge of PPD, neurobiological understanding that can augment new drug screening and treatment is still at infancy. The etiological perspectives which reveal a wide range of PPD mediators partly explain the pharmacological approaches showing significant variation in the treatment response and remission in women with PPD. Reports provide consistent evidence of complex temporal changes in gene–environment, hormonal, non-hormonal, and stress-driven mediators. PPD is etiologically different from other depressive episodes which supports the necessity of specific treatment (155). The efficacy of the conventional antidepressant drugs may be largely enhanced with appropriate adjuvant treatments or evidence-based psychotherapies. Despite the recent support for GABAergic target therapy, the reports on brexanolone or progesterone’s effects in PPD patients are still few and somehow inconclusive (156). Although the dosing regimen of brexanolone takes into consideration the serum allopregnanolone concentrations at the end of pregnancy (157, 158), the long-term impact on endogenous hormonal release or effects remains largely unclear.
The dearth of extensive analysis of peripartum phasic hormonal and non-hormonal changes leading to depressive readouts in PPD still limits the scope of pharmacological interventions. The determination of the early mediators of PPD and validated screening tools for PPD are key to early intervention, and screening of new drugs. The panacea to the myriads of controversy that are associated with the study of psychiatric disorders in animals (159, 160) includes analyses of the underlying factors of behavioral changes using a new and well-validated animal model.
An increase in immobility time that indicate depressive-like readout in rodents in the most widely used forced swimming and tail suspension tests (161, 162) could turn out to be an adaptive coping response to stress rather than behavioral despair (163). In the case of drug treatment (amphetamine), an increase in the swimming time could be an indication of CNS stimulation rather than antidepressant effects. Hence, complementary assessments of different dimensions of the depressive state of PPD as characterized by complex, heterogeneous, and multiple factors (154) could eliminate false positive or erroneous interpretations of findings. Robust assessment of the key neurobiological pathways and mediators of PPD could help in the discovery of standard pharmacological intervention.
Author contributions
JF, EC, and GP: conceptualization, design, and methodology. LM, CM, CC, MD, DR, MF-N, and EC: writing – original draft preparation, reviewing and editing. LM and JF: software, preparation, and editing of figures. All authors contributed to the article and approved the submitted version.
Acknowledgments
FUNADESP, CNPQ, CAPES, FAPEG, and UFG for research support while acknowledging free access to BioRender.com and draw.io for the creation of figures.
Conflict of interest
The authors declare that the research was conducted in the absence of any commercial or financial relationships that could be construed as a potential conflict of interest.
Publisher’s note
All claims expressed in this article are solely those of the authors and do not necessarily represent those of their affiliated organizations, or those of the publisher, the editors and the reviewers. Any product that may be evaluated in this article, or claim that may be made by its manufacturer, is not guaranteed or endorsed by the publisher.
References
1. Žigová, L, Massarová, P, Vranecová, K, Hrubá, O, Adamičková, A, and Gažová, A. Neuroactive steroids - new possibilities in the treatment of postpartum depression. Ceska Slov Farm. (2022) 71:142–50. doi: 10.5817/CSF2022-4-140
2. Munk-Olsen, T, and Agerbo, E. Does childbirth cause psychiatric disorders? A population-based study paralleling a natural experiment. Epidemiology. (2015) 26:79–84. doi: 10.1097/EDE.0000000000000193
3. Sūdžiūtė, K, Murauskienė, G, Jarienė, K, Jaras, A, Minkauskienė, M, Adomaitienė, V, et al. Pre-existing mental health disorders affect pregnancy and neonatal outcomes: a retrospective cohort study. BMC Pregnancy Childbirth. (2020) 20:419. doi: 10.1186/s12884-020-03094-5
4. Hahn-Holbrook, J, Cornwell-Hinrichs, T, and Anaya, I. Economic and health predictors of national postpartum depression prevalence: a systematic review, meta-analysis, and meta-regression of 291 studies from 56 countries. Front Psych. (2017) 8:248. doi: 10.3389/fpsyt.2017.00248
5. Duan, KM, Ma, JH, Wang, SY, Huang, ZD, Zhou, YY, and Yu, HE. The role of tryptophan metabolism in postpartum depression. Metab Brain Dis. (2018) 33:647–60. doi: 10.1007/s11011-017-0178-y
6. Engqvist, I, Ferszt, G, Åhlin, A, and Nilsson, K. Psychiatric nurses descriptions of women with postpartum psychosis and nurses' responses—an exploratory study in Sweden. Issues Ment Health Nurs. (2009) 30:23–30. doi: 10.1080/01612840802498268
7. Mothes, L, Kristensen, CH, Grassi-Oliveira, R, Fonseca, RP, Argimon, IIL, and Irigaray, TQ. Childhood maltreatment and executive functions in adolescents. Child Adolesc Ment Health. (2015) 20:56–62. doi: 10.1111/camh.12068
8. Su, Y, D'Arcy, C, Yuan, S, and Meng, X. How does childhood maltreatment influence ensuing cognitive functioning among people with the exposure of childhood maltreatment? A systematic review of prospective cohort studies. J Affect Disord. (2019) 252:278–93. doi: 10.1016/j.jad.2019.04.026
9. Goodman, SH, Rouse, MH, Connell, AM, Broth, MR, Hall, CM, and Heyward, D. Maternal depression and child psychopathology: a meta-analytic review. Clin Child Fam Psychol Rev. (2011) 14:1–27. doi: 10.1007/s10567-010-0080-1
10. Madigan, S, Oatley, H, Racine, N, Fearon, RMP, Schumacher, L, Akbari, E, et al. A meta-analysis of maternal prenatal depression and anxiety on child socio-emotional development. J Am Acad Child Adolesc Psychiatry. (2018) 57:645–57.e8. doi: 10.1016/j.jaac.2018.06.012
11. Mallikarjun, PK, and Oyebode, F. Prevention of postnatal depression. J R Soc Promot Heal. (2005) 125:221–6. doi: 10.1177/146642400512500514
12. Wald, MF, Muzyk, AJ, and Clark, D. Bipolar depression: pregnancy, postpartum, and lactation. Psychiatr Clin North Am. (2016) 39:57–74. doi: 10.1016/j.psc.2015.10.002
13. Rasmussen, M-LH, Strøm, M, Wohlfahrt, J, Videbech, P, and Melbye, M. Risk, treatment duration, and recurrence risk of postpartum affective disorder in women with no prior psychiatric history: a population-based cohort study. PLoS Med. (2017) 14:e1002392. doi: 10.1371/journal.pmed.1002392
14. Lim, S, Hill, B, Teede, HJ, Moran, LJ, and O'Reilly, S. An evaluation of the impact of lifestyle interventions on body weight in postpartum women: a systematic review and meta-analysis. Obes Rev. (2020) 21:e12990. doi: 10.1111/obr.12990
15. Meltzer-Brody, S, Howard, L, Bergink, V, Vigod, S, Jones, I, and Munk-Olsen, T. Postpartum psychiatric disorders. Nat Rev Dis Primers. (2018) 4:18022. doi: 10.1038/nrdp.2018.22
16. Kadrmas, A, Winokur, G, and Crowe, R. Postpartum manias. Br J Psychiatry. (1979) 135:551–4. doi: 10.1192/bjp.135.6.551
17. Halbreich, U, and Karkun, S. Cross-cultural and social diversity of prevalence of postpartum depression and depressive symptoms. J Affect Disord. (2006) 91:97–111. doi: 10.1016/j.jad.2005.12.051
18. Corwin, EJ, Kohen, R, Jarrett, M, and Stafford, B. The heritability of postpartum depression. Biol Res Nurs. (2010) 12:73–83. doi: 10.1177/1099800410362112
20. Esquirol, E. Mental Maladies: A Treatise on Insanity (translated by EK hunt). Philadelphia, Lea amp: Blanchard (1845).
21. Marcé, LV. Traité de la Folie des Femmes Encientes, de Nouvelles. Paris, Baillière: Accouchées et de Nourrices (1858).
22. Coble, PA, and Day, NL. The epidemiology of mental and emotional disorders during pregnancy and the postpartum period In: RL Cohen, editor. Psychiatric Consultation in Childbirth Settings. Boston, MA: Springer (1988).
23. Hamilton, JA. The identity of postpartum psychosis In: IF Brockington and R Kumar, editors. Motherhood and Mental Illness. London: Academic Press (1982). 1–17.
24. Brockington, I. A historical perspective on the psychiatry of motherhood In: A Riecher-Rossler, editor. Perinatal Stress, Mood and Anxiety Disorders. Basel: Karger (2005). 1–5.
25. Worthen, RJ, and Eleonore, B. Inflammatory and neurodegenerative pathophysiology implicated in postpartum depression. Neurobiol Dis. (2022) 165:105646. doi: 10.1016/j.nbd.2022.105646
26. Hutchens, BF, and Kearney, J. Risk factors for postpartum depression: an umbrella review. J Midwifery Womens Health. (2020) 65:96–108. doi: 10.1111/jmwh.13067
27. Stewart, DE, and Vigod, S. Postpartum Depression. NEJM. (2016) 375:2177–86. doi: 10.1056/NEJMcp1607649
28. Pope, CJ, Sharma, V, and Mazmanian, D. Bipolar disorder in the postpartum period: management strategies and future directions. Womens Health (Lond). (2014) 10:359–71. doi: 10.2217/whe.14.33
29. O'Hara, MW, and McCabe, JE. Postpartum depression: current status and future directions. Annu Rev Clin Psychol. (2013) 9:379–407. doi: 10.1146/annurev-clinpsy-050212-185612
30. Galea, LA, and Frokjaer, VG. Perinatal depression: embracing variability toward better treatment and outcomes. Neuron. (2019) 102:13–6. doi: 10.1016/j.neuron.2019.02.023
31. Zhang, Q, Dai, X, and Li, W. Comparative efficacy and acceptability of pharmacotherapies for postpartum depression: a systematic review and network meta-analysis. Front Pharmacol. (2022) 13:950004. doi: 10.3389/fphar.2022.950004
32. Kaufman, Y, Carlini, SV, and Deligiannidis, KM. Advances in pharmacotherapy for postpartum depression: a structured review of standard-of-care antidepressants and novel neuroactive steroid antidepressants. Ther Adv Psychopharmacol. (2022) 12:20451253211065859. doi: 10.1177/20451253211065859
33. Yonkers, KA, Wisner, KL, Stewart, DE, Oberlander, TF, Dell, DL, Stotland, N, et al. The management of depression during pregnancy: a report from the American Psychiatric Association and the American College of Obstetricians and Gynecologists. Gen Hosp Psychiatry. (2009) 31:403–13. doi: 10.1016/j.genhosppsych.2009.04.003
34. Carter, CS, and Altemus, M. Integrative functions of lactational hormones in social behavior and stress management. Ann N Y Acad Sci. (1997) 807:164–74. doi: 10.1111/j.1749-6632.1997.tb51918.x
35. Higuchi, T, Negoro, H, and Arita, J. Reduced responses of prolactin and catecholamine to stress in the lactating rat. J Endocrinol. (1989) 122:495–8. doi: 10.1677/joe.0.1220495
36. Champagne, F, Diorio, J, Sharma, S, and Meaney, MJ. Naturally occurring variations in maternal behavior in the rat are associated with differences in estrogen-inducible central oxytocin receptors. Proc Natl Acad Sci U S A. (2001) 98:12736–41. doi: 10.1073/pnas.221224598
37. Smith, CT, Sierra, Y, Oppler, SH, and Boettiger, CA. Ovarian cycle effects on immediate reward selection bias in humans: a role for estradiol. J Neurosci. (2014) 34:5468–76. doi: 10.1523/JNEUROSCI.0014-14.2014
38. Witte, MM, Resuehr, D, Chandler, AR, Mehle, AK, and Overton, JM. Female mice and rats exhibit species-specifc metabolic and behavioral responses to ovariectomy. Gen Comp Endocrinol. (2010) 166:520–8. doi: 10.1016/j.ygcen.2010.01.006
39. Casas, S, Garcia, S, Cabrera, R, Nanfaro, F, Escudero, C, and Yunes, R. Progesterone prevents depression-like behavior in a model of Parkinson's disease induced by 6-hydroxydopamine in male rats. Pharmacol Biochem Behav. (2011) 99:614–8. doi: 10.1016/j.pbb.2011.06.012
40. Frieder, A, Fersh, M, Hainline, R, and Deligiannidis, KM. Pharmacotherapy of postpartum depression: current approaches and novel drug development. CNS Drugs. (2019) 33:265–82. doi: 10.1007/s40263-019-00605-7
41. Newport, DJ, Wilcox, MM, and Stowe, ZN. Antidepressants during pregnancy and lactation: defining exposure and treatment issues. Semin Perinatol. (2001) 25:177–90. doi: 10.1053/sper.2001.24901
42. Molyneaux, E, Howard, LM, McGeown, H, Karia, AM, and Trevillion, K. Antidepressant treatment for postnatal depression. Cochrane Database Syst Rev. (2014) 9:CD002018. doi: 10.1002/14651858.CD002018.pub2
43. Deligiannidis, KM, Meltzer-Brody, S, Gunduz-Bruce, H, Doherty, J, Jonas, J, Li, S, et al. Effect of Zuranolone vs placebo in postpartum depression: a randomized clinical trial. JAMA Psychiat. (2021) 78:951–9. doi: 10.1001/jamapsychiatry.2021.1559
44. Verbe, J, Dubertret, C, El-Hage, W, Bonnet-Brilhault, F, and Duriez, P. Approche GABAergique de la dépression du post-partum: une revue critique translationnelle [GABAergic approach of postpartum depression: a translational review of literature]. Encéphale. (2020) 46:123–34. doi: 10.1016/j.encep.2019.09.007
45. Brunton, PJ. Neuroactive steroids and stress axis regulation: pregnancy and beyond. J Steroid Biochem Mol Biol. (2016) 160:160–8. doi: 10.1016/j.jsbmb.2015.08.003
46. Paul, SM, and Purdy, RH. Neuroactive steroids. FASEB J. (1992) 6:2311–22. doi: 10.1096/fasebj.6.6.1347506
47. Kash, K, Bächström, T, Nilsson, L-G, and Sundström-Poromaa, I. Allopregnanolone impairs episodic memory in healthy women. Psychopharmacology. (2008) 199:161–8. doi: 10.1007/s00213-008-1150-7
48. García-Baos, A, Gallego-Landin, I, Ferreres-Álvarez, I, Puig-Reyne, X, Castro-Zavala, A, Valverde, O, et al. Effects of fast-acting antidepressant drugs on a postpartum depression mice model. Biomed Pharmacother. (2022) 154:113598. doi: 10.1016/j.biopha.2022.113598
49. Chen-Li, D, Lui, LMW, Rosenblat, JD, Lipsitz, O, Teopiz, KM, Ho, R, et al. Ketamine as potential treatment for postpartum depression: a narrative review. Ann Clin Psychiatry. (2022) 34:264–74. doi: 10.12788/acp.0082
50. Timby, E, Hedstrom, H, Bachstrom, T, Sundstrom-Poromaa, I, Nyberg, S, and Bixo, M. Allopregnanolone, a GABAA receptor agonist, decreases gonadotropin levels in women. A preliminary study. Gynecol Endocrinol. (2011) 27:1087–93. doi: 10.3109/09513590.2010.540603
51. Wisner, KL, Sit, DK, Mc Shea, MC, Rizzo, DM, Zoretich, RA, Hughes, CL, et al. Onset timing, thoughts of self-harm, and diagnoses in postpartum women with screen-positive depression findings. JAMA Psychiat. (2013) 70:490–8. doi: 10.1001/jamapsychiatry.2013.87
52. Segre, LS, O’Hara, MW, Arndt, S, and Stuart, S. The prevalence of postpartum depression: the relative significance of three social status indices. Soc Psychiatry Psychiatr Epidemiol. (2007) 42:316–21. doi: 10.1007/s00127-007-0168-1
53. Gregoire, AJ, Kumar, R, Everitt, B, Henderson, AF, and Studd, JW. Transdermal oestrogen for treatment of severe postnatal depression. Lancet. (1996) 347:930–3. doi: 10.1016/s0140-6736(96)91414-2
54. Mershy, JP, and Janczewstì, CE. Adverse childhood experiences and postpartum depression in home visiting programs: prevalence, association, and mediating mechanisms. Matern Child Health J. (2018) 22:1051–8. doi: 10.1007/s10995-018-2488-z
55. Centers for Disease Control and Prevention (CDC). Prevalence of self-reported postpartum depressive symptoms--17 states, 2004-2005. MMWR Morb Mortal Wkly Rep. (2008) 57:361–6.
56. Cory-Slechta, DA. Chapter nine-enduring behavioral and brain impacts of prenatal stress and childhood adversity and their potential multigenerational consequences. Adv Neurotoxicol. (2018) 2:265–300. doi: 10.1016/bs.ant.2018.03.007
57. Yim, IS, Tanner Stapleton, LR, Guardino, CM, Hahn-Holbrook, J, and Dunkel, SC. Biological and psychosocial predictors of postpartum depression: systematic review and call for integration. Annu Rev Clin Psychol. (2015) 11:99–137. doi: 10.1146/annurev-clinpsy-101414-020426
58. Liu, H, Wang, LL, Zhao, SJ, Kwak-Kim, J, Mor, G, and Liao, AH. Why are pregnant women susceptible to COVID-19? An immunological viewpoint. J Reprod Immunol. (2020) 139:103122. doi: 10.1016/j.jri.2020.103122
59. Guintivano, J, Arad, M, Gould, TD, Payne, JL, and Kaminsky, ZA. Antenatal prediction of postpartum depression with blood DNA methylation biomarkers. Mol Psychiatry. (2014) 19:560–7. doi: 10.1038/mp.2013.62
60. Couto, TC, Brancaglion, MYM, Alvim-Soares, A, Moreira, L, Garcia, FD, Nicolato, R, et al. Postpartum depression: a systematic review of the genetics involved. World J Psychiatry. (2015) 5:103–11. doi: 10.5498/wjp.v5.i1.103
61. Forty, L, Jones, L, Macgregor, S, Caesar, S, Cooper, C, Hough, A, et al. Familiality of postpartum depression in unipolar disorder: results of a family study. Am J Psychiatry. (2006) 163:1549–53. doi: 10.1176/ajp.2006.163.9.1549
62. Treloar, SA, Martin, NG, Bucholz, KK, Madden, PA, and Heath, AC. Genetic influences on post-natal depressive symptoms: findings from an Australian twin sample. Psychol Med. (1999) 29:645–54. doi: 10.1017/S0033291799008387
63. Mehta, D, Grewen, K, Pearson, B, Wani, S, Wallace, L, Henders, AK, et al. Genome-wide gene expression changes in postpartum depression point towards an altered immune landscape. Transl Psychiatry. (2021) 11:155. doi: 10.1038/s41398-021-01270-5
64. Gutiérrez-Zotes, A, Díaz-Peña, R, Costas, J, Martorell, L, Gelabert, E, Sans, T, et al. Interaction between the functional SNP rs 2070951 in NR3C2 gene and high levels of plasma corticotropin-releasing hormone associates to postpartum depression. Arch Womens Ment Health. (2020) 23:413–20. doi: 10.1007/s00737-019-00989-x
65. Zoubovsky, SP, Hoseus, S, Tumukuntala, S, Schulkin, JO, Williams, MT, Vorhees, CV, et al. Chronic psychosocial stress during pregnancy affects maternal behavior and neuroendocrine function and modulates hypothalamic CRH and nuclear steroid receptor expression. Transl Psychiatry. (2020) 10:6. doi: 10.1038/s41398-020-0704-2
66. Choi, D, Tsuchiya, KJ, and Takei, N. Interaction effect of oxytocin receptor (OXTR) rs 53576 genotype and maternal postpartum depression on child behavioural problems. Sci Rep. (2019) 9:7685. doi: 10.1038/s41598-019-44175-6
67. Mehta, D, Newport, D, Frishman, G, Kraus, L, Rex-Haffner, M, Ritchie, JC, et al. Early predictive biomarkers for postpartum depression point to a role for estrogen receptor signaling. Psychol Med. (2014) 44:2309–22. doi: 10.1017/S0033291713003231
68. Landsman, A, Aidelman, R, Smith, Y, Boyko, M, and Greenberger, C. Distinctive gene expression profile in women with history of postpartum depression. Genomics. (2017) 109:1–8. doi: 10.1016/j.ygeno.2016.10.005
69. Binder, EB, Newport, DJ, Zach, EB, Smith, AK, Deveau, TC, Altshuler, LL, et al. A serotonin transporter gene polymorphism predicts peripartum depressive symptoms in an at-risk psychiatric cohort. J Psychiatr Res. (2010) 44:640–6. doi: 10.1016/j.jpsychires.2009.12.001
70. Mitchell, C, Notterman, D, Brooks-Gunn, J, Hobcraft, J, Garfinkel, I, Jaeger, K, et al. Role of mother's genes and environment in postpartum depression. Proc Natl Acad Sci U S A. (2011) 108:8189–93. doi: 10.1073/pnas.1014129108
71. Mehta, D, Quast, C, Fasching, PA, Seifert, A, Voigt, F, Beckmann, MW, et al. The 5-HTTLPR polymorphism modulates the influence on environmental stressors on peripartum depression symptoms. J Affect Disord. (2012) 136:1192–7. doi: 10.1016/j.jad.2011.11.042
72. Pinheiro, RT, Coelho, FM, Silva, RA, Pinheiro, KAT, Oses, JP, Quevedo, LA, et al. Association of a serotonin transporter gene polymorphism (5-HTTLPR) and stressful life events with postpartum depressive symptoms: a population-based study. J Psychosom Obstet Gynaecol. (2013) 34:29–33. doi: 10.3109/0167482X.2012.759555
73. Sun, HS, Tsai, HW, Ko, HC, Chang, FM, and Yeh, TL. Association of tryptophan hydroxylase gene polymorphism with depression, anxiety and comorbid depression and anxiety in a population-based sample of postpartum Taiwanese women. Genes Brain Behav. (2004) 3:328–36. doi: 10.1111/j.1601-183X.2004.00085.x
74. Hoffiz, YC, Castillo-Ruiz, A, Hall, MAL, Hite, TA, Gray, JM, Cisternas, CD, et al. Birth elicits a conserved neuroendocrine response with implications for perinatal osmoregulation and neuronal cell death. Sci Rep. (2021) 11:2335. doi: 10.1038/s41598-021-81511-1
75. Schiller, CE, Meltzer-Brody, S, and Rubinow, DR. The role of reproductive hormones in postpartum depression. CNS Spectr. (2015) 20:48–59. doi: 10.1017/S1092852914000480
76. Bloch, M, Daly, RC, and Rubinow, DR. Endocrine factors in the etiology of postpartum depression. Compr Ther. (2003) 44:234–46. doi: 10.1016/S0010-440X(03)00034-8
77. Martini, J, Wittich, J, Petzoldt, J, Winkel, S, Einsle, F, Siegert, J, et al. Maternal anxiety disorders prior to conception, psychopathology during pregnancy and early infants' development: a prospective-longitudinal study. Arch Womens Ment Health. (2013) 16:549–60. doi: 10.1007/s00737-013-0376-5
78. Martini, J, Petzoldt, J, Einsle, F, Beesdo-Baum, K, Höfler, M, and Wittchen, HU. Risk factors and course patterns of anxiety and depressive disorders during pregnancy and after delivery: a prospective-longitudinal study. J Affect Disord. (2015) 175:385–95. doi: 10.1016/j.jad.2015.01.012
79. Sacher, J, Wilson, AA, Houle, S, Rusjan, P, Hassan, S, Bloomfield, PM, et al. Elevated brain monoamine oxidase a binding in the early postpartum period. Arch Gen Psychiatry. (2010) 67:468–74. doi: 10.1001/archgenpsychiatry.2010.32
80. Sacher, J, Rekkas, PV, Wilson, AA, Houle, S, Romano, L, Hamidi, J, et al. Relationship of monoamine oxidase-a distribution volume to postpartum depression and postpartum crying. Neuropsychopharmacology. (2015) 40:429–35. doi: 10.1038/npp.2014.190
81. Hasler, G, Fromm, S, Carlson, PJ, Luckenbaugh, DA, Waldeck, T, Geraci, M, et al. Neural response to catecholamine depletion in unmedicated subjects with major depressive disorder in remission and healthy subjects. Arch Gen Psychiatry. (2008) 65:521–31. doi: 10.1001/archpsyc.65.5.521
82. Schröcksnadel, K, Wirleitner, B, Winkler, C, and Fuchs, D. Monitoring tryptophan metabolism in chronic immune activation. Clin Chim Acta. (2006) 364:82–90. doi: 10.1016/j.cca.2005.06.013
83. Keenan, PA, Yaldoo, DT, Stress, ME, Fuerst, DR, and Ginsburg, KA. Explicit memory in pregnant women. Am J Obstet Gyneco. (1998) 179:731–7. doi: 10.1016/s0002-9378(98)70073-0
84. Macbeth, AH, and Luine, VN. Changes in anxiety and cognition due to reproductive experience: a review of data from rodent and human mothers. Neurosci Biobehav Rev. (2010) 34:452–67. doi: 10.1016/j.neubiorev.2009.08.011
85. Henry, JF, and Sherwin, BB. Hormones and cognitive functioning during late pregnancy and postpartum: a longitudinal study. Behav Neurosci. (2012) 126:73–85. doi: 10.1037/a0025540
86. Logan, DM, Hill, KR, Jones, R, Holt-Lunstad, J, and Larson, MJ. How do memory and attention change with pregnancy and childbirth? A controlled longitudinal examination of neuropsychological functioning in pregnant and postpartum women. J Clin Exp Neuropsychol. (2014) 36:528–39. doi: 10.1080/13803395.2014.912614
87. Hampson, E, Phillips, SD, Duff-Canning, SJ, Evans, KL, Merrill, M, Pinsonneault, JK, et al. Working memory in pregnant women: relation to estrogen and antepartum depression. Horm Behav. (2015) 74:218–27. doi: 10.1016/j.yhbeh.2015.07.006
88. Hoekzema, E, Barba-Müller, E, Pozzobon, C, Picado, M, Lucco, F, García García, D, et al. Pregnancy leads to long-lasting changes in human brain structure. Nat Neurosci. (2017) 20:287–96. doi: 10.1038/nn.4458
89. Mazor, E, Sheiner, E, Wainstock, T, Attias, M, and Walfisch, A. The association between depressive state and maternal cognitive function in postpartum women. Am J Perinatol. (2019) 36:285–90. doi: 10.1055/s-0038-1667376
90. Zheng, JX, Chen, YC, Chen, H, Jiang, L, Bo, F, Feng, Y, et al. Disrupted spontaneous neural activity related to cognitive impairment in postpartum women. Front Psychol. (2018) 9:624. doi: 10.3389/fpsyg.2018.00624
91. Thomas, KA, and Burr, RL. Melatonin level and pattern in postpartum versus nonpregnant nulliparous women. Journal of obstetric, gynecologic, and neonatal nursing. JOGNN. (2006) 35:608–15. doi: 10.1111/j.1552-6909.2006.00082.x
92. Sharkey, KM, Pearlstein, TB, and Carskadon, MA. Circadian phase shifts and mood across the perinatal period in women with a history of major depressive disorder: a preliminary communication. J Affect Disord. (2013) 150:1103–8. doi: 10.1016/j.jad.2013.04.046
93. Murray, G, and Harvey, A. Circadian rhythms and sleep in bipolar disorder. Bipolar Disord. (2010) 12:459–72. doi: 10.1111/j.1399-5618.2010.00843.x
94. Lewis, KJ, Foster, RG, and Jones, IR. Is sleep disruption a trigger for postpartum psychosis? Br J Psychiatry. (2016) 208:409–11. doi: 10.1192/bjp.bp.115.166314
95. Krawczak, EM, Minuzzi, L, Hidalgo, MP, and Frey, BN. Do changes in subjective sleep and biological rhythms predict worsening in postpartum depressive symptoms? A prospective study across the perinatal period. Arch Womens Ment Health. (2016) 19:591–8. doi: 10.1007/s00737-016-0612-x
96. Lee, KA, Zaffke, ME, and McEnany, G. Parity and sleep patterns during and after pregnancy. Obstet Gynecol. (2000) 95:14–8. doi: 10.1016/s0029-7844(99)00486-x
97. Grant, MM, Thase, ME, and Sweeney, JA. Cognitive disturbance in outpatient depressed younger adults: evidence of modest impairment. Biol Psychiatry. (2001) 50:35–43. doi: 10.1016/s0006-3223(00)01072-6
98. Okun, ML. Disturbed sleep and postpartum depression. Curr Psychiatr Ther. (2016) 18:66. doi: 10.1007/s11920-016-0705-2
99. Richardson, L, and Adams, S. Cognitive deficits in patients with depression. J Nurse Pract. (2018) 14:437–443.e3. doi: 10.1016/j.nurpra.2018.03.006
100. Perini, G, Ramusino, MC, Sinforiani, E, Bernini, S, Petrachi, R, and Costa, A. Cognitive impairment in depression: recent advances and novel treatments. Neuropsychiatr Dis Treat. (2019) 15:1249–58. doi: 10.2147/NDT.S199746
101. Ji, Y, Li, W, Liu, B, Liu, J, Ju, Y, Wang, M, et al. Clinical characteristics of cognitive deficits in major depressive disorder: a 6-month prospective study. Arch Clin Psychiatry. (2020) 47:101–5. doi: 10.1590/0101-60830000000241
102. Anderson, G, Berk, M, and Maes, M. Biological phenotypes underpin the physio-somatic symptoms of somatization, depression, and chronic fatigue syndrome. Acta Psychiatr Scand. (2014) 129:83–97. doi: 10.1111/acps.12182
103. Roomruangwong, C, Kanchanatawan, B, Sirivichayakul, S, Anderson, G, Carvalho, AF, Duleu, S, et al. IgA/IgM responses to tryptophan and tryptophan catabolites (trycats) are differently associated with prenatal depression, physio-somatic symptoms at the end of term and premenstrual syndrome. Mol Neurobiol. (2017) 54:3038–49. doi: 10.1007/s12035-016-9877-3
104. Lommatzsch, M, Hornych, K, Zingler, C, Schuff-Werner, P, Höppner, J, and Virchow, JC. Maternal serum concentrations of BDNF and depression in the perinatal period. Psychoneuroendocrinology. (2006) 31:388–94. doi: 10.1016/j.psyneuen.2005.09.003
105. O’Mahony, SM, Clarke, G, Borre, YE, Dinan, TG, and Cryan, JF. Serotonin, tryptophan metabolism and the brain-gut-microbiome axis. Behav Brain Res. (2015) 277:32–48. doi: 10.1016/j.bbr.2014.07.027
106. Oxenkrug, G. Serotonin-kynurenine hypothesis of depression: historical overview and recent developments. Curr Drug Targets. (2013) 14:514–21. doi: 10.2174/1389450111314050002
107. Mithaiwala, MN, Santana-Coelho, D, Porter, GA, and O’Connor, JC. Neuroinflammation and the kynurenine pathway in CNS disease: molecular mechanisms and therapeutic implications. Cells. (2021) 10:1548. doi: 10.3390/cells10061548
108. Krupa, A, and Kowalska, I. The kynurenine pathway-new linkage between innate and adaptive immunity in autoimmune endocrinopathies. Int J Mol Sci. (2021) 22:9879. doi: 10.3390/ijms22189879
109. Sas, K, Szabó, E, and Vécsei, L. Mitochondria, oxidative stress and the kynurenine system, with a focus on ageing and neuroprotection. Molecules. (2018) 23:191. doi: 10.3390/molecules23010191
110. Achtyes, E, Keaton, SA, Smart, L, Burmeister, AR, Heilman, PL, Krzyzanowski, S, et al. Inflammation and kynurenine pathway dysregulation in post-partum women with severe and suicidal depression. Brain Behav Immun. (2020) 83:239–47. doi: 10.1016/j.bbi.2019.10.017
111. Veen, C, Myint, AM, Burgerhout, KM, Schwarz, MJ, Schütze, G, Kushner, SA, et al. Tryptophan pathway alterations in the postpartum period and in acute postpartum psychosis and depression. J Affect Disord. (2016) 189:298–305. doi: 10.1016/j.jad.2015.09.064
112. Sha, Q, Madaj, Z, Keaton, S, Galvis, MLE, Smart, L, Krzyzanowski, S, et al. Cytokines and tryptophan metabolites can predict depressive symptoms in pregnancy. Transl Psychiatry. (2022) 12:35. doi: 10.1038/s41398-022-01801-8
113. Kimmel, MC, Cox, E, Schiller, C, Gettes, E, and Meltzer-Brody, S. Pharmacologic treatment of perinatal depression. Obstet Gynecol Clin N Am. (2018) 45:419–40. doi: 10.1016/j.ogc.2018.04.007
114. Sedlmayr, P, Blaschitz, A, and Stocker, R. The role of placental tryptophan catabolism. Front Immunol. (2014) 5:230. doi: 10.3389/fimmu.2014.00230
115. Brunton, PJ, and Russell, JA. Neuroendocrine control of maternal stress responses and fetal programming by stress in pregnancy. Prog Neuro-Psychopharmacol Biol Psychiatry. (2011) 35:1178–91. doi: 10.1016/j.pnpbp.2010.12.023
116. Poggi-Davis, E, and Sandman, CA. Prenatal exposure to stress and stress hormones influences child development. Infants Young Child. (2006) 19:246–59. doi: 10.1097/00001163-200607000-00008
117. Seth, S, Lewis, AJ, and Galbally, M. Perinatal maternal depression and cortisol function in pregnancy and the postpartum period: a systematic literature review. BMC Pregnancy Childbirth. (2016) 16:124. doi: 10.1186/s12884-016-0915-y
118. Glynn, LM, Davis, EP, and Sandman, CA. New insights into the role of perinatal HPA-axis dysregulation in postpartum depression. Neuropeptides. (2013) 47:363–70. doi: 10.1016/j.npep.2013.10.007
119. Wang, J, Yun, Q, Ma, SF, Song, HR, Guo, MN, and Zhang, WN. Inhibition of expression of glucocorticoids receptors may contribute to postpartum depression. Biochem Biophys Res Commun. (2020) 523:159–64. doi: 10.1016/j.bbrc.2019.12.040
120. De Geest, K, Thiery, M, Piron-Possuyt, G, Vanden Driessche, R, and Driessche, RV. Plasma oxytocin in human pregnancy and parturition. J Perinat Med. (1985) 13:3–13. doi: 10.1515/jpme.1985.13.1.3
121. Uvnäs-Moberg, K, Ekström-Bergström, A, Berg, M, Buckley, S, Pajalic, Z, Hadjigeorgiou, E, et al. Maternal plasma levels of oxytocin during physiological childbirth – a systematic review with implications for uterine contractions and central actions of oxytocin. BMC Pregnancy Childbirth. (2019) 19:285. doi: 10.1186/s12884-019-2365-9
122. Prevost, M, Zelkowitz, P, Tulandi, T, Hayton, B, Feeley, N, Carter, CS, et al. Oxytocin in pregnancy and the postpartum: relations to labor and its management. Front Public Health. (2014) 2:1. doi: 10.3389/fpubh.2014.00001
123. Donadon, MF, Martin-Santos, R, and Osório, F. Oxytocin effects on the cognition of women with postpartum depression: a randomized, placebo-controlled clinical trial. Prog Neuro-Psychopharmacol Biol Psychiatry. (2021) 111:110098. doi: 10.1016/j.pnpbp.2020.110098
124. Galbally, M, Watson, SJ, Keelan, JA, Spigset, O, and Lewis, A. The relationship between oxytocin blood concentrations and antidepressants over pregnancy and the postpartum. Prog Neuro-Psychopharmacol Biol Psychiatry. (2021) 109:110218. doi: 10.1016/j.pnpbp.2020.110218
125. Martinowich, K, and Lu, B. Interaction between BDNF and serotonin: role in mood disorders. Neuropsychopharmacology. (2008) 33:73–83. doi: 10.1038/sj.npp.1301571
126. Jang, SW, Liu, X, Pradoldej, S, Tosini, G, Chang, Q, Iuvone, PM, et al. N-acetylserotonin activates Trk B receptor in a circadian rhythm. Proc Natl Acad Sci U S A. (2010) 107:3876–81. doi: 10.1073/pnas.0912531107
127. Duman, RS, and Monteggia, LM. A neurotrophic model for stress-related mood disorders. Biol Psychiatry. (2006) 59:1116–27. doi: 10.1016/j.biopsych.2006.02.013
128. Ivanisevic, L, and Saragovi, H. Chapter 224 - neurotrophins In: A Kastin, editor. Handbook of Biologically Active Peptides. 2nd ed. Amsterdam: Academic Press (2013). 1639–46.
129. Wiener, CD, de Mello, SF, Pedrotti, FM, Bittencourt, G, de Oliveira, JF, Lopez, MM, et al. Serum levels of nerve growth factor (NGF) in patients with major depression disorder and suicide risk. J Affect Disord. (2015) 15:245–8. doi: 10.1016/j.jad.2015.05.067
130. Pedrotti Moreira, F, Borges, CJ, Wiener, CD, da Silva, PM, Portela, LV, Lara, DR, et al. Serum brain-derived neurotrophic factor levels in subjects with major depressive disorder with previous suicide attempt: a population-based study. Psychiatry Res. (2018) 262:500–4. doi: 10.1016/j.psychres.2017.09.033
131. Huang, EJ, and Reichardt, LF. Neurotrophins: roles in neuronal development and function. Annu Rev Neurosci. (2001) 24:677–736. doi: 10.1146/annurev.neuro.24.1.677
132. Sahay, AS, Sundrani, DP, and Joshi, SR. Neurotrophins: Role in Placental Growth and Development. Vitam Horm. (2017) 104:243–61. doi: 10.1016/bs.vh.2016.11.002
133. Kawamura, K, Kawamura, N, Sato, W, Fukuda, J, Kumagai, J, and Tanaka, T. Brain-derived neurotrophic factor promotes implantation and subsequent placental development by stimulating trophoblast cell growth and survival. Endocrinology. (2009) 150:3774–82. doi: 10.1210/en.2009-0213
134. Gazal, M, Motta, LS, Wiener, CD, Fernandes, JC, Quevedo, LA, Jansen, K, et al. Brain-derived neurotrophic factor in post-partum depressive mothers. Neurochem Res. (2012) 37:583–7. doi: 10.1007/s11064-011-0647-3
135. Gao, X, Wang, J, Yao, H, Cai, Y, and Cheng, R. Serum BDNF concentration after delivery is associated with development of postpartum depression: a 3-month follow up study. J Affect Disord. (2016) 200:25–30. doi: 10.1016/j.jad.2016.04.002
136. Christian, LM, Mitchell, AM, Gillespie, SL, and Palettas, M. Serum brain-derived neurotrophic factor (BDNF) across pregnancy and postpartum: associations with race, depressive symptoms, and low birth weight. Psychoneuroendocrinology. (2016) 74:69–76. doi: 10.1016/j.psyneuen.2016.08.025
137. Kodomari, I, Wada, E, Nakamura, S, and Wada, K. Maternal supply of BDNF tomouse fetal brain through the placenta. Neurochem Int. (2009) 54:95–8. doi: 10.1016/j.neuint.2008.11.005
138. Belzung, C, and Lemoine, M. Criteria of validity for animal models of psychiatric disorders: focus on anxiety disorders and depression. Biol Mood Anxiety Disord. (2011) 1:9. doi: 10.1186/2045-5380-1-9
139. Avraham, Y, Hants, Y, Vorobeiv, L, Staum, M, Abu Ahmad, W, Mankuta, D, et al. Brain neurotransmitters in an animal model with postpartum depressive-like behavior. Behav Brain Res. (2017) 326:307–21. doi: 10.1016/j.bbr.2017.01.013
140. Galea, LA, Wide, JK, and Barr, AM. Estradiol alleviates depressive-like symptoms in a novel animal model of post-partum depression. Behav Brain Res. (2001) 122:1–9. doi: 10.1016/s0166-4328(01)00170-x
141. Stoffel, EC, and Craft, RM. Ovarian hormone withdrawal-induced "depression" in female rats. Physiol Behav. (2004) 83:505–13. doi: 10.1016/j.physbeh.2004.08.033
142. Frye, CA, and Walf, AA. Estrogen and/or progesterone administered systemically or to the amygdala can have anxiety-, fear-, and pain-reducing effects in ovariectomized rats. Behav Neurosci. (2004) 118:306–13. doi: 10.1037/0735-7044.118.2.306
143. Keller, M, Vandenberg, LN, and Charlier, TD. The parental brain and behavior: a target for endocrine disruption. Front Neuroendocrinol. (2019) 54:100765. doi: 10.1016/j.yfrne.2019.100765
144. Crowley, WR. Neuroendocrine regulation of lactation and milk production. Compr Physiol. (2015) 5:255–91. doi: 10.1002/cphy.c140029
145. Winokur, SB, Lopes, KL, Moparthi, Y, and Pereira, M. Depression-related disturbances in rat maternal behavior are associated with altered monoamine levels within mesocorticolimbic structures. J Neuroendocrinol. (2019) 31:e12766. doi: 10.1111/jne.12766
146. Groer, MW, Davis, MW, and Hemphill, J. Postpartum stress: current concepts and the possible protective role of breastfeeding. JOGNN. (2002) 31:411–7. doi: 10.1111/j.1552-6909.2002.tb00063.x
147. Altemus, M, Deuster, PA, Galliven, E, Carter, CS, and Gold, PW. Suppression of hypothalmic-pituitary-adrenal axis responses to stress in lactating women. J Clin Endocrinol Metab. (1995) 80:2954–9. doi: 10.1210/jcem.80.10.7559880
148. Windle, RJ, Wood, S, Shanks, N, Perks, P, Conde, GL, da Costa, APC, et al. Endocrine and behavioural responses to noise stress: comparison of virgin and lactating female rats during non-disrupted maternal activity. J Neuroendocrinol. (1997) 9:407–14. doi: 10.1046/j.1365-2826.1997.00587.x
149. Fischer, D, Patchev, VK, Hellbach, S, and Hassan, AH, Almeida OF. Lactation as a model for naturally reversible hypercortisolism plasticity in the mechanisms governing hypothalamo-pituitary-adrenocortical activity in rats. J Clin Invest. (1995) 96:1208–15. doi: 10.1172/JCI118153
150. Magiakou, MA, Mastorakos, G, Webster, E, and Chrousos, GP. The hypothalamic-pituitary-adrenal axis and the female reproductive system. Ann N Y Acad Sci. (1997) 816:42–56. doi: 10.1111/j.1749-6632.1997.tb52128.x
151. Frye, CA, and Walf, AA. Progesterone reduces depression-like behavior in a murine model of Alzheimer's disease. Age (Dordr). (2009) 31:143–53. doi: 10.1007/s11357-009-9091-6
152. Li, Y, Raaby, KF, Sanchez, C, and Gulinello, M. Serotonergic receptor mechanisms underlying antidepressant-like action in the progesterone withdrawal model of hormonally induced depression in rats. Behav Brain Res. (2013) 256:520–8. doi: 10.1016/j.bbr.2013.09.002
153. Maguire, J, and Mody, I. GABA (a) R plasticity during pregnancy: relevance to postpartum depression. Neuron. (2008) 59:207–13. doi: 10.1016/j.neuron.2008.06.019
154. Mir, FR, Pollano, A, and Rivarola, MA. Animal models of postpartum depression revisited. Psychoneuroendocrinology. (2022) 136:105590. doi: 10.1016/j.psyneuen.2021.105590
155. Batt, MM, Duffy, KA, Novick, AM, Metcalf, CA, and Epperson, CN. Is postpartum depression different from depression occurring outside of the perinatal period? A review of the evidence. FOCUS (Am Psychiatr Publ). (2020) 18:106–19. doi: 10.1176/appi.focus.20190045
156. Barak, Y, and Glue, P. Progesterone loading as a strategy for treating postpartum depression. Hum Psychopharmacol. (2020) 35:e2731. doi: 10.1002/hup.2731
157. Kanes, SJ, Colquhoun, H, Doherty, J, Raines, S, Hoffmann, E, Rubinow, DR, et al. Open-label, proof-ofconcept study of brexanolone in the treatment of severe postpartum depression. Hum Psychopharmacol. (2017) 32:e2576. doi: 10.1002/hup.2576
158. Luisi, S, Petraglia, F, Benedetto, C, Nappi, RE, Bernardi, F, Fadalti, M, et al. Serum allopregnanolone levels in pregnant women: changes during pregnancy, at delivery, and in hypertensive patients. J Clin Endocrinol Metab. (2000) 85:2429–33. doi: 10.1210/jcem.85.7.6675
159. Nestler, EJ, and Hyman, SE. Animal models of neuropsychiatric disorders. Nat Neurosci. (2010) 13:1161–9. doi: 10.1038/nn.2647
160. Gururajan, A, Reif, A, Cryan, JF, and Slattery, DA. The future of rodent models in depression research. Nat Rev Neurosci. (2019) 20:686–701. doi: 10.1038/s41583-019-0221-6
161. Fajemiroye, J, Polepally, P, Chaurasiya, N, Tekwani, BL, Zjawiony, JK, and Costa, EA. Oleanolic acid acrylate elicits antidepressant-like effect mediated by 5-HT1A receptor. Sci Rep. (2015) 5:11582. doi: 10.1038/srep11582
162. Fajemiroye, JO, Galdino, PM, Florentino, IF, da Rocha, FF, Ghedini, PC, Polepally, PR, et al. Plurality of anxiety and depression alteration mechanism by oleanolic acid. J Psychopharmacol. (2014) 28:923–34. doi: 10.1177/0269881114536789
Keywords: depression, pharmacological targets, preclinical assessments, behavioral models, post-partum
Citation: Moreira LKS, Moreira CVL, Custódio CHX, Dias MLP, Rosa DA, Ferreira-Neto ML, Colombari E, Costa EA, Fajemiroye JO and Pedrino GR (2023) Post-partum depression: From clinical understanding to preclinical assessments. Front. Psychiatry 14:1173635. doi: 10.3389/fpsyt.2023.1173635
Edited by:
Tasuku Hashimoto, International University of Health and Welfare (IUHW), JapanReviewed by:
David Do Carmo Malvar, Federal Rural University of Rio de Janeiro, BrazilCopyright © 2023 Moreira, Moreira, Custódio, Dias, Rosa, Ferreira-Neto, Colombari, Costa, Fajemiroye and Pedrino. This is an open-access article distributed under the terms of the Creative Commons Attribution License (CC BY). The use, distribution or reproduction in other forums is permitted, provided the original author(s) and the copyright owner(s) are credited and that the original publication in this journal is cited, in accordance with accepted academic practice. No use, distribution or reproduction is permitted which does not comply with these terms.
*Correspondence: James O. Fajemiroye, amFtZXNmYWplbWlyb3llQHVmZy5icg==
†These authors share last authorship