- 1Faculty of Rehabilitation Medicine, University of Alberta, Edmonton, AB, Canada
- 2Alberta Health Services, Edmonton, AB, Canada
- 3Neuroscience and Mental Health Institute, University of Alberta, Edmonton, AB, Canada
- 4Department of Occupational Therapy, University of Alberta, Edmonton, AB, Canada
- 5Faculty of Kinesiology, Sport, and Recreation, University of Alberta, Edmonton, AB, Canada
- 6Department of Psychiatry, Faculty of Medicine and Dentistry, University of Alberta, Edmonton, AB, Canada
- 7Department of Communication Sciences and Disorders, University of Alberta, Edmonton, AB, Canada
Background: Patients with depression and/or anxiety are commonly seen in inpatient geriatric settings. Both disorders are associated with an increased risk of cognitive impairments, notably in executive functioning. Transcranial direct current stimulation (tDCS), a type of non-invasive brain stimulation, involves the administration of a low-dose electrical current to induce neuromodulation, which ultimately may act on downstream cognitive processing.
Objective: The purpose of this study was to determine the effects of tDCS on executive functioning in geriatric inpatients with symptoms of depression and/or anxiety.
Design: Pilot Randomized Controlled Trial.
Setting: Specialized geriatric wards in a tertiary rehabilitation hospital.
Methods: Thirty older-aged adults were recruited, of which twenty completed ten-to-fifteen sessions of 1.5 mA anodal or sham tDCS over the left dorsolateral prefrontal cortex. Cognitive assessments were administered at baseline and following the tDCS protocol; analyses examined the effects of tDCS on cognitive performance between groups (anodal or sham tDCS).
Results: tDCS was found to increase inhibitory processing and cognitive flexibility in the anodal tDCS group, with significant changes on the Stroop test and Trail Making Test-Part B. No significant changes were observed on measures of attention or working memory.
Discussion: These results provide preliminary evidence that tDCS-induced neuromodulation may selectively improve cognitive processing in older adults with symptoms of depression and/or anxiety.
Clinical Trials Registration: www.clinicaltrials.gov, NCT04558177
Introduction
Older adults admitted for rehabilitation often present with concomitant depression and/or anxiety (1–3). Multi-morbidity as well as cognitive impairment may be synergistically coupled with depression and anxiety in older adults (4, 5). Additionally, depression and anxiety are often comorbid psychiatric disorders (6, 7). In older adults, the severity of depression often increases when an anxiety disorder comorbidity is present (8, 9). Furthermore, depression and/or anxiety may impair processes related to successful rehabilitation and are associated with increased length of hospital stay, increased utilization of inpatient resources, and a higher rate of inpatient mortality (2, 10, 11).
Executive functioning (EF) is a key mediating factor associated with functional status in older adults (12). EF is often discussed in terms of three subdomains required to perform daily activities: inhibition, working memory, and cognitive flexibility (13). Although EF naturally declines across normal cognitive ageing (14–16), numerous underlying etiologies including mild cognitive impairment (MCI) and dementia can impair EF beyond the extent seen across normal cognitive ageing (17). Cognitive changes in EF have been observed in older adults with neurocognitive and psychiatric disorders, including depression, anxiety, MCI, and dementia (18–26). Therefore, interventions aimed at improving EF may lead to improved functional outcomes in older adults [See Karr and colleagues (27) for a review].
Experimental use of transcranial direct current stimulation (tDCS) has been increasingly explored as a cognitive enhancement technique, including within older adult populations (28–30). tDCS is an emerging method of non-invasive brain stimulation, where neuromodulation is achieved by altering neuronal polarities through the administration of a low-dose electrical current applied across the scalp to target brain structures. Although transcranial magnetic stimulation (TMS) has been approved for clinical applications such as treatment-resistant depression in an increasing number of countries, tDCS approval currently remains primarily for research purposes in most nations (31).
Previous studies have demonstrated that tDCS can result in improvements in cognition, as well as modulating symptoms associated with depression, MCI, and dementia (32–34). Several of these studies explored the effects of tDCS on the dorsolateral prefrontal cortex (DLPFC) (32, 34). The DLPFC has also been established as a neural region involved in mediating cognitive processes underlying EF, including attention (35), cognitive flexibility [the ability to switch across multiple different concepts in a context-dependent manner; (36, 37)] and higher-order cognition (38, 39). Further, neuroimaging has consistently demonstrated that the DLPFC is implicated in depression and anxiety (40–45).
The purpose of this pilot study was to explore the effects of tDCS on cognitive performance in older adult inpatients with symptoms of depression and/or anxiety within an inpatient geriatric rehabilitation clinical care setting. The following research question was of interest: What are the effects of multi-session anodal tDCS over the left DLPFC on EF in older adult inpatients with symptoms of depression and/or anxiety compared to those receiving sham stimulation? It was hypothesized that anodal tDCS would augment performance across executive functioning processes compared to sham stimulation.
Materials and methods
To answer the research question, a double-blinded parallel, sham-controlled, single-centre randomized control trial was conducted at the Glenrose Rehabilitation Hospital (Alberta Health Services, Edmonton, Canada). Ethics was approved by the University of Alberta Research Ethics Board (Pro00078317). The study protocol was registered with the National Institute of Health (NCT04558177).
Participants
A subset of patients taking part in a larger study examining the effects of tDCS in geriatric depression and anxiety were recruited to undergo additional cognitive assessments before and after tDCS stimulation. Convenience sampling was utilized; participants were recruited from Specialized Geriatric Rehabilitation inpatient wards at the Glenrose Rehabilitation Hospital (Alberta Health Services, Edmonton, Canada). All patients underwent depression and anxiety screenings upon admission using the Geriatric Depression Scale [GDS; (46)] and Geriatric Anxiety Inventory [GAI; (47)] These screening tests were administered by Occupational Therapists on the geriatric wards. Inclusion criteria were defined as: being over the age of 65 years old, GDS ≥ 5, GAI ≥ 8, proficiency in English, ability to provide informed consent, and the absence of dementia. If eligible for participation, patients were referred to the research team by the Occupational Therapists or Physicians for recruitment. Signed informed consent was obtained from patients who agreed to participate in the study or their respective powers of attorney. Exclusion criteria included: active infection, implanted medical devices (e.g., cardiac pacemakers, deep brain stimulators), history of seizures, metallic implants in the head, or history of severe neurological illness. Participation in the study was in addition to usual routine clinical care, and participants did not receive compensation.
Clinical care
Multi-disciplinary clinical care varied across patients; however, all patients received a combination of geriatric-oriented physical and occupational therapy, in addition to medical and nursing care. As routine clinical care remained the primary focus for these patients, tDCS sessions were occasionally skipped if required to accommodate standard patient care. Therefore, not all individuals were able to complete all fifteen tDCS sessions; participants who completed more than 10 tDCS sessions were included for analysis.
Cognitive assessments
Participants underwent paper-based cognitive assessments administered by the primary author, with a battery of tasks largely assessing EF, including: inhibitory control, working memory, attention, processing speed, and cognitive flexibility (see Table 1 for an overview of the cognitive battery administered). The cognitive battery was administered in the same order across participants. Instructions and practice trials of the assessments were given to ensure participant comprehension; errors were immediately corrected during the trial runs. To minimize potential physiological and circadian confounds relating to cognitive fatigue, cognitive assessments and tDCS sessions were administered between 15:00–17:30 daily based on the participant's availability around their clinical care routine. Cognitive testing and tDCS sessions were delivered in participants' hospital rooms with distractions minimized (e.g., lights on, television off, door closed); attention and comprehension of the cognitive tasks were ensured by completing practice trials.
tDCS randomization & parameters
To maintain double-blinding, six HDCStim tDCS devices (Newronika, Italy) were programmed to deliver anodal (n = 3) or sham (n = 3) stimulation by an individual not involved in the study. Participants, researchers, and clinicians remained blinded to the intervention. Recruited participants were allocated a specific tDCS device for the study in a 1:1 allocation ratio. Simple randomization was performed, dependent on the programming of the tDCS device to deliver an active anodal or sham stimulation.
tDCS sessions were delivered daily, based on the participants' availability around routine clinical care, with participants receiving 10–15 consecutive sessions (including weekends). tDCS parameters were based on previously established safety parameters [refer to (52)]. tDCS sessions were administered by the primary author as well as three other research assistants, who all received training from the same rehabilitation engineer familiar with tDCS. Electrodes were placed in 5 cm × 7 cm (35 cm2) electrode sponges and saturated with 10 ml 0.9% NaCl solution and secured to the scalp using a snuggly fitting hairnet. Using the 10:20 EEG system (53), the anode was placed over F3 (the left DLPFC) and the cathode over the contralateral (right) supraorbital region, in line with Liao and colleagues (54); refer to Figure 1. A 1.5 mA current was applied for 20 min per session. The current was ramped over 1 min until reaching 1.5 mA (52). Participants were free to participate in any task during tDCS sessions; most participants remained in bed or watched television.
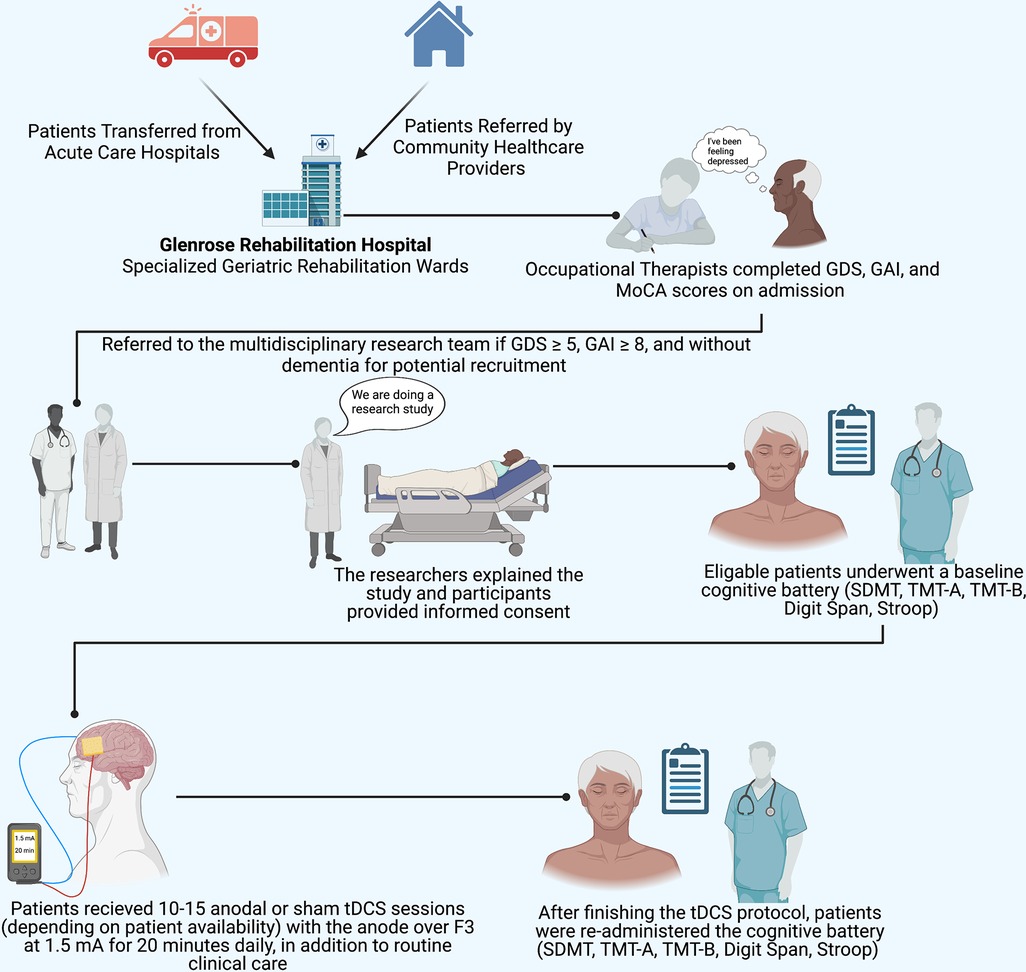
Figure 1. Implemented protocol. The study protocol implemented illustrating participant recruitment, consent, assessment, and tDCS procedures. SDMT, symbol digit modalities test; TMT-A, Trail Making Test Part A; TMT-B, Trail Making Test Part B; MoCA, Montreal Cognitive Assessment. tDCS electrode placement: the anode (red) was placed over F3, the cathode (blue) was placed over the right supraorbital region. Figure 1 was created with Biorender.com.
The sham group received 1.5 mA of electrical stimulation for one minute: ramping up over 15 s, steady for 30 s and ramping down for 15 s. This blinding technique involves the replication of a cutaneous electrical sensation used to mask participants' group allocation (52, 55). Once the data collection period was completed, stimulator assignment and group allocation (anodal, sham) were revealed by the individual outside of the study.
Analyses
The primary outcome was examining the effects of tDCS on cognitive performance in older adults with symptoms of depression or anxiety. Differences between pre-test and post-test scores were calculated for all cognitive assessments. Analysis methods were based on a previous randomized control trial using multi-session tDCS using a similar sample size of older adults with MCI (54). A two-way mixed ANOVA was performed in SPSS (Version 27, IBM Corp. Armonk, NY) on change scores, with time as a within-subjects factor and treatment condition (anodal or sham) as a between-subject factor. An alpha of 0.05 was set to determine significance, with a Bonferroni correction for multiple comparisons. Significant main effects and main interactions reported in the two-way mixed ANOVA were followed up using both paired and independent t-tests to determine any significant differences. Analysis was conducted based on the tDCS group assignment.
Results
Participants
Twenty eligible participants were included in the final sample (n = 10 in each group), consistent with similar sample sizes of older adults with multi-session tDCS interventions in MCI and depression (54, 56). Participant recruitment and data collection were stopped early because of the emergence of SARS-CoV-2. The recruitment process is highlighted in Supplementary Figure S1. Admitting diagnoses included: decreased functional mobility and weakness, falls, cognitive decline, depression, anxiety, cancer, congestive heart failure, chronic obstructive pulmonary disease, myocardial infarction, and cerebrovascular accidents.
No significant differences were found between those who received 10–14 tDCS sessions and those who completed all 15 sessions, as well as on age, education, baseline MoCA, GDS, and GAI scores. Hence, we combined the results of those receiving 10–15 tDCS sessions and report them together, respective to their tDCS group allocation. An independent t-test found no significant differences between the active and sham groups on the variables of age, years of education, baseline MoCA, GDS, and GAI (all p's > 0.05; Refer to Table 2). An overview of obtained results is presented in Figure 2.
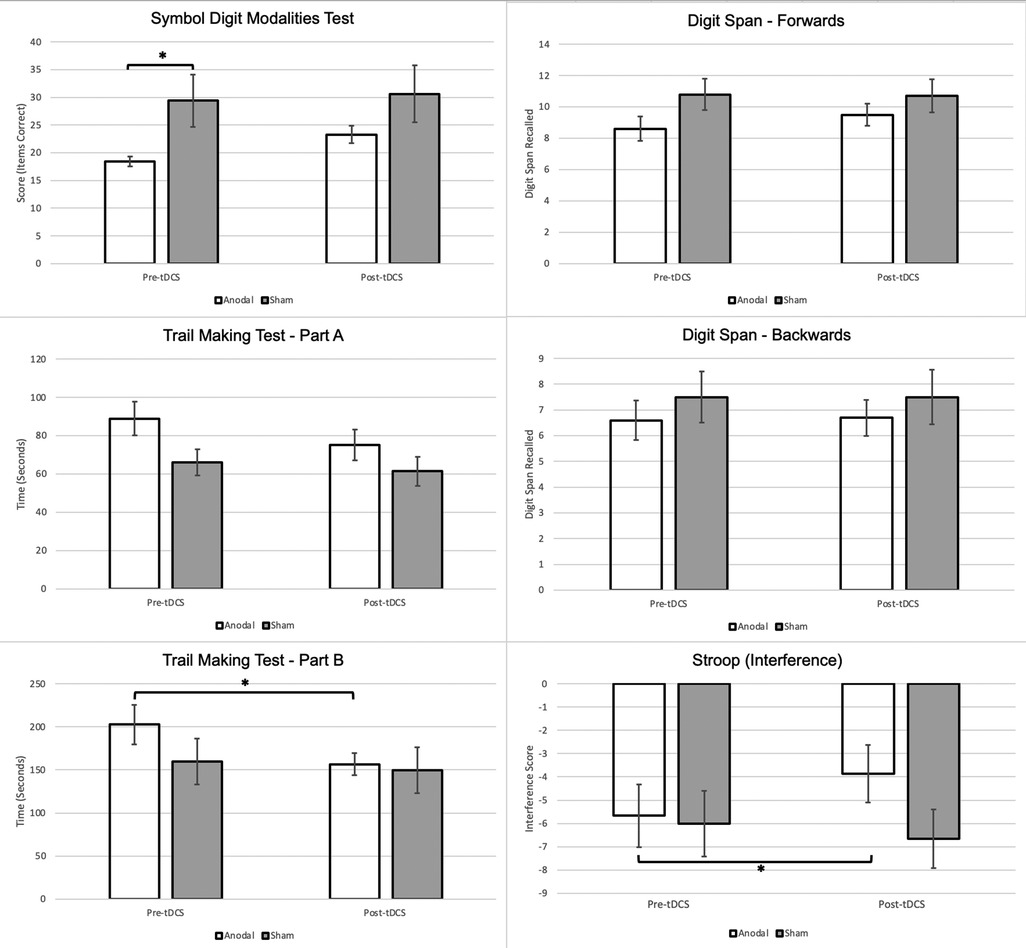
Figure 2. Changes in cognitive performance between anodal & sham stimulation groups. Note: * is significant at p < 0.05. Stroop Interference: Negative interference suggests a pathological impairment of inhibition, lower scores indicate greater impairment (60).
Symbol digit modalities test (SDMT)
A non-significant main effect of time [F (1,18) = 3.031, p = 0.099, ηp2 = 0.144] and condition [F (1,18) = 0.53, p = 0.475, ηp2 = 0.029] was found on the SDMT, as well as a non-significant time × condition (anodal or sham) interaction [F (1,18) = 2.82, p = 0.110, ηp2 = 0.136]. Paired t-tests demonstrate a significantly higher post-tDCS SDMT score when compared to the baseline scores within the anodal group only, however, no significant group differences were found on the independent t-test.
Trail making test part A (TMT-A)
Although there was no main effect of condition on TMT-A [F (1,18) = 2.83, p = 0.110, ηp2 = 0.136], there was a significant effect of time [F (1,18) = 15.96, p < 0.001, ηp2 = 0.470]. The time × condition interaction for TMT-A was found to be non-significant [F (1,18) = 3.95, p = 0.062, ηp2 = 0.180]. Paired t-tests demonstrated significant improvement in TMT-A times within both groups, and an independent t-test found that the change from baseline to post-tDCS was significantly greater in the anodal group relative to the sham group.
Trail making test part B (TMT-B)
No significant main effect of condition on TMT-B completion time was found [F (1,18) = 0.64, p = 0.435, ηp2 = 0.034]. The main effect of time on TMT-B completion time was significant [F (1,18) = 10.70, p = 0.004, ηp2 = 0.373]. The time × condition interaction was determined to be significant [F (1,18) = 4.44, p = 0.049, ηp2 = 0.198]. The paired t-test found that only the anodal group demonstrated a significant difference in TMT-B time between baseline and post-tDCS. The independent t-test demonstrated a significant improvement in TMT-B scores within the anodal group.
Digit span forward
No significant main effects of time [F (1,18) = 2.23, p = 0.152, ηp2 = 0.110] and condition [F (1,18) = 1.80, p = 0.197, ηp2 = 0.091] were observed. Interactions between time and condition on digit forward scores [F (1,18) = 3.49, p = 0.078, ηp2 = 0.162] were also non-significant.
Digit span backwards
No significant main effect of time [F (1,18) = 0.11, p = 0.747, ηp2 = 0.006] or main effect of condition [F (1,18) = 0.64, p = 0.435, ηp2 = 0.034] was found. Like digit span forwards, there was no significant interaction between time and condition [F (1,18) = 0.11, p = 0.747, ηp2 = 0.006]. In addition, no significant within-group or between-group differences were noted across t-tests.
Stroop interference
A non-significant main effect of time [F (1,18) = 2.93, p = 0.104, ηp2 = 0.140] and a non-significant main effect of condition [F (1,18) = 3.95, p = 0.062, ηp2 = 0.180] was found. A significant time × condition interaction was found on the two-way ANOVA [F (1,18) = 4.77 ηp2, p = 0.042, ηp2 = 0.209]; significant increases in Stroop interference scores were found in the paired t-test only within the anodal group. In addition, there was a significant difference between the changes in interference score from pre-tDCS to post-tDCS between the active and sham groups, with the active group demonstrating a greater mean change.
Discussion
In this study, 20 older adult inpatients with self-reported symptoms of depression and/or anxiety received 10–15 anodal or sham tDCS sessions delivered over the left DLPFC. We report that the tDCS-protocol provided over the left DLPFC selectively augmented cognitive processing. In line with our hypothesis, we note significant changes in tests involved in higher cognitive processes (i.e., the TMT-B and Stroop tasks). However, the effectiveness of tDCS on the domains of attention and working memory was minimal.
The time required to complete the TMT-B decreased in the anodal group, suggestive of increased cognitive flexibility and interference processing. It is generally agreed that the TMT-B has a higher sensitivity to central EF and cognitive flexibility and task-switching compared to the TMT-A (64, 65). Increased performance on the Stroop task was also evident, suggesting a potential increase in inhibition capacity. tDCS may have modulated the ability to minimize interfering distractors, resulting in more accurate and rapid processing of presented stimuli. In addition, we report null tDCS effects on the other DLPFC-associated cognitive processes of working memory and attention, assessed by the SDMT, TMT-A, and the digit span tests. Cognitive flexibility, working memory, and inhibition are processes highly involved in EF (13), and necessary in higher-order cognitive processing. Thus, tDCS-induced neuromodulation may have invoked a selective-synergistic interaction in EF domains of cognitive flexibility and interference, increasing performance on tests of higher-order cognition.
These results corroborate previously reported findings. In a similar study design targeting Parkinson's Disease, Doruk and colleagues (66) report significant improvements on the TMT-B without changes on the TMT-A, with the maintenance of these findings extending to one-month post-stimulation. Bystad and colleagues (67) further report significant gains on the TMT-B without improvements in the TMT-A only within a young adult group. In addition, Loftus and colleagues (68) report reaction time improvements on the Stroop task in young adults after receiving anodal DLPFC stimulation resulting in inhibitory control enhancement; our results continue to support inhibition control enhancement in older aged adults. However, the extent of tDCS effects on cognitive enhancement may vary across the lifespan, which to date remains largely unknown.
We report null tDCS-induced cognitive effects on working memory and attention. These findings are in line with Kumar and colleagues (69) who report a lack of tDCS effects on working memory and global cognition in older adults with depression. Nonetheless, contrasting results are reported by Nissim and colleagues (70) who reported significant changes in working memory as well as functional connectivity after a two-week tDCS protocol paired with cognitive training in healthy older adults. Again, the pairing of tDCS with working memory training has also been demonstrated to increase digit span performance in older adults (71). Although tDCS alone has been demonstrated to selectively modulate working memory within older adults with higher levels of education (72), dual tDCS-cognitive training paradigms may optimize effects on working memory and attention which requires further investigation.
Taken together, these results further contribute to the proposed roles of the DLPFC in higher-order cognition and behaviour (73, 74). However, the role of the DLPFC in lower-order cognition remains unclear. Other frontal lobe structures, including the ventrolateral prefrontal cortex, have been proposed to be engaged in lower-order cognitive processing (75). In addition, these results provide additional evidence that tDCS may selectively increase EF in older adults. Future research is needed to determine who may be optimal candidates for tDCS therapy for EF augmentation, and the effects of tDCS on prefrontal networks.
Limitations and future directions
Across the literature, varying study designs, cognitive protocols, populations of interest, and tDCS parameters exist. The effects of tDCS may be task-specific, cognitive-domain specific, age, and etiology dependent, with varying montages resulting in varying neuro-cognitive modulatory effects. Furthermore, additional factors including multi-morbidity, level of education, and pharmacological agents may all impact neurological and cognitive modulation, which was not accounted for in this study. Taken together, the generalizability of the obtained results to other clinical populations, age groups, tDCS montages, and cognitive domains should be interpreted with caution.
The tDCS montage applied in this study (anode over the left DLPFC; cathode over the contralateral supraorbital region) was similar to a separate randomized control trial using tDCS in older adults with MCI (54). However, previous tDCS modelling highlighted the potential of deeper cortical and larger white matter network activation using an extracephalic return electrode (76), which in turn may alter the efficacy of tDCS and the obtained results. In addition, morphological changes and cerebral atrophy present in MCI may impact tDCS current vectors and electrical field densities (77). Additionally, no significant differences were found between those completing 10–15 tDCS sessions. Thus, the effect of tDCS-intervention frequency and intensity on EF performance in older-adult inpatients should be investigated in future studies.
The purpose of this study was to investigate whether tDCS influenced cognition within a sample of older adult inpatients with symptoms of depression and/or anxiety. Within this study, participants had an overall mean MoCA of 22.75, which may be indicative of MCI. Furthermore, cognition may have been impaired due to the confounds related to depression and anxiety. With this study designed as a pilot, our sample may limit the overall power, however, conducting this study within the hospital context contributes towards ecological validity and generalizability of tDCS applications in real-world clinical settings with an interdisciplinary approach.
In this study, we report no major adverse effects from tDCS stimulation. tDCS was found to be well-tolerated by the older-adult participants and did not significantly interfere with routine clinical care. Mild side effects including a tingling sensation under the electrodes, as well as slight discomfort from the snuggly fitted hairnet, were reported by some individuals; these side effects are consistent with previous studies (78).
Unique considerations exist when recruiting patients from an inpatient geriatric rehabilitation setting for a tDCS study, including coordination of treatments with routine clinical care, family visits, legal factors, personal values, and comfort. These factors should be taken into consideration and weighed in terms of the feasibility of future studies. Additionally, with a pragmatic design, real-world conditions were present which may have impacted the results. For instance, during the tDCS sessions, participants were free to sleep, read, or watch television during their downtime; it is possible that the activities completed during the tDCS interventions may have impacted cognitive performance, which should be examined in future studies. Further, test-retest reliability was not assessed, which we recognize as a limitation. Additionally, future studies should incorporate larger sample sizes, consider the pairing of tDCS with cognitive or behavioural training, explore other tDCS montages, include a maintenance period, incorporate neuroimaging modalities, consider adding other executive functioning assessments, and explore effects in other cognitive disorders associated with ageing including MCI and dementia. In addition, future studies are encouraged to consider ecological validity to extend the generalizability of cognitive augmentation into real-world settings.
Conclusion
In this study, multi-session tDCS over the left DLPFC appears to invoke beneficial cognitive augmentation within the domains of inhibition processing, processing speed, and cognitive flexibility in older adult inpatients with symptoms of depression or anxiety. This evidence supports that anodal tDCS-invoked neuromodulation may extend into cognitive modulation, including executive functioning. By examining the effects of anodal tDCS within a geriatric sample, we contribute to the ongoing investigation of non-invasive brain stimulation targeting cognitive decline in older adults. Future studies should continue to investigate tDCS in normal and pathological cognitive ageing, in addition to targeting the optimization of protocols, as well as determining ideal candidates for tDCS interventions. The results of tDCS research are important for assessing its efficacy and practicality for clinical and therapeutic use within geriatric rehabilitation settings.
Data availability statement
The raw data supporting the conclusions of this article will be made available by the authors, without undue reservation.
Ethics statement
The studies involving human participants were reviewed and approved by University of Alberta Research Ethics Board. The patients/participants provided their written informed consent to participate in this study.
Author contributions
MF, EK, AL, JR, and HK contributed to the conception and design of the study. MF aided in the clinical implementation and data collection. MF and SV and performed the statistical analysis. HK, DR, and MKW provided clinical leadership to physicians and occupational therapists throughout the study. MF, EK, and AL wrote the first draft of the manuscript. SV, JR, SB, HK, DR, and MKW wrote sections of the manuscript. All authors contributed to the article and approved the submitted version.
Funding
This work was supported by the Centre for Aging + Brain Health Innovation and the Glenrose Rehabilitation Hospital Foundation.
Acknowledgments
We would like to acknowledge and thank all of the Occupational Therapists and Physicians on units 3D and 4C at the Glenrose Rehabilitation Hospital, as well as the Glenrose Geriatric Research Team. Special thanks go to Terry Blois, the lead Rehabilitation Engineer, as well as Brendon Restall, and Hosein Bahari.
Conflict of interest
The authors declare that the research was conducted in the absence of any commercial or financial relationships that could be construed as a potential conflict of interest.
Publisher's note
All claims expressed in this article are solely those of the authors and do not necessarily represent those of their affiliated organizations, or those of the publisher, the editors and the reviewers. Any product that may be evaluated in this article, or claim that may be made by its manufacturer, is not guaranteed or endorsed by the publisher.
Supplementary material
The Supplementary Material for this article can be found online at: https://www.frontiersin.org/articles/10.3389/fresc.2022.997531/full#supplementary-material.
References
1. Shah DC, Evans M, King D. Prevalence of mental illness in a rehabilitation unit for older adults. Postgrad Med J. (2000) 76:153–6. doi: 10.1136/pmj.76.893.153
2. Sayers SL, Hanrahan N, Kutney A, Clarke SP, Reis BF, Riegel B. Psychiatric comorbidity and greater hospitalization risk, longer length of stay, and higher hospitalization costs in older adults with heart failure. J Am Geriatr Soc. (2007) 55:1585–91. doi: 10.1111/j.1532-5415.2007.01368.x
3. Yohannes AM, Baldwin RC, Connolly MJ. Prevalence of depression and anxiety symptoms in elderly patients admitted in post-acute intermediate care. Int J Geriatr Psychiatry. (2008) 23:1141–7. doi: 10.1002/gps.2041
4. Read JR, Sharpe L, Modini M, Dear BF. Multimorbidity and depression: a systematic review and meta-analysis. J Affect Disord. (2017) 221:36–46. doi: 10.1016/j.jad.2017.06.009
5. Gould CE, O’Hara R, Goldstein MK, Beaudreau SA. Multimorbidity is associated with anxiety in older adults in the health and retirement study. Int J Geriatr Psychiatry. (2016) 31:1105–15. doi: 10.1002/gps.4532
6. Kalin NH. The critical relationship between anxiety and depression. Am J Psychiatry. (2020) 177:365–7. doi: 10.1176/appi.ajp.2020.20030305
7. Beekman ATF, De Beurs E, Van Balkom AJLM, Deeg DJH, Van Dyck R, Van Tilburg W. Anxiety and depression in later life: co-occurrence and communality of risk factors. Am J Psychiatry. (2000) 157:89–95. doi: 10.1176/ajp.157.1.89
8. Fiske A, Wetherell JL, Gatz M. Depression in older adults. Annu Rev Clin Psychol. (2009) 5:363–89. doi: 10.1146/annurev.clinpsy.032408.153621
9. Andreescu C, Lenze EJ, Dew MA, Begley AE, Mulsant BH, Dombrovski AY, et al. Effect of comorbid anxiety on treatment response and relapse risk in late-life depression: controlled study. Br J Psychiatry. (2007) 190:344–9. doi: 10.1192/bjp.bp.106.027169
10. Prina AM, Deeg D, Brayne C, Beekman A, Huisman M. The association between depressive symptoms and non-psychiatric hospitalisation in older adults. PLoS One. (2012) 7:e34821. doi: 10.1371/journal.pone.0034821
11. Sugawara N, Metoki N, Hagii J, Saito S, Shiroto H, Tomita T, et al. Effect of depressive symptoms on the length of hospital stay among patients hospitalized for acute stroke in Japan. Neuropsychiatr Dis Treat. (2015) 11:2551–6. doi: 10.2147/NDT.S91303
12. Grigsby J, Kaye K, Baxter J, Shetterly SM, Hamman RF. Executive cognitive abilities and functional status among community- dwelling older persons in the San Luis Valley health and aging study. J Am Geriatr Soc. (1998) 46:590–6. doi: 10.1111/j.1532-5415.1998.tb01075.x
13. Diamond A. Executive functions. Annu Rev Psychol. (2013) 64:135–68. doi: 10.1146/annurev-psych-113011-143750
14. Etienne V, Marin-Lamellet C, Laurent B. Évolution du contrôle exécutif au cours du vieillissement Normal. Rev Neurol (Paris). (2008) 164:1010–7. doi: 10.1016/j.neurol.2008.03.021
15. Harada CN, Natelson Love MC, Triebel KL. Normal Cognitive aging. Clin Geriatr Med. (2013) 29:737–52. doi: 10.1016/j.cger.2013.07.002
16. Fjell AM, Sneve MH, Grydeland H, Storsve AB, Walhovd KB. The disconnected brain and executive function decline in aging. Cereb Cortex. (2017) 27:2303–17. doi: 10.1093/cercor/bhw082
17. Kirova AM, Bays RB, Lagalwar S. Working memory and executive function decline across normal aging, mild cognitive impairment, and Alzheimer’s disease. Biomed Res Int. (2015) 2015:1–9. doi: 10.1155/2015/748212
18. Lockwood KA, Alexopoulos GS, Van Gorp WG. Executive dysfunction in geriatric depression. Am J Psychiatry. (2002) 159:1119–26. doi: 10.1176/appi.ajp.159.7.1119
19. Alexopoulos GS, Raue PJ, Kiosses DN, MacKin RS, Kanellopoulos D, McCulloch C, et al. Problem-solving therapy and supportive therapy in older adults with major depression and executive dysfunction: effect on disability. Arch Gen Psychiatry. (2011) 68:33–41. doi: 10.1001/archgenpsychiatry.2010.177
20. Pantzar A, Laukka EJ, Atti AR, Fastbom J, Fratiglioni L, Bäckman L. Cognitive deficits in unipolar old-age depression: a population-based study. Psychol Med. (2014) 44:937–47. doi: 10.1017/S0033291713001736
21. Brandt J, Aretouli E, Neijstrom E, Samek J, Manning K, Albert MS, et al. Selectivity of executive function deficits in mild cognitive impairment. Neuropsychology. (2009) 23:607–18. doi: 10.1037/a0015851
22. Duong A, Whitehead V, Hanratty K, Chertkow H. The nature of lexico-semantic processing deficits in mild cognitive impairment. Neuropsychologia. (2006) 44:1928–35. doi: 10.1016/j.neuropsychologia.2006.01.034
23. Clark LR, Schiehser DM, Weissberger GH, Salmon DP, Delis DC, Bondi MW. Specific measures of executive function predict cognitive decline in older adults. J Int Neuropsychol Soc. (2012) 18:118–27. doi: 10.1017/S1355617711001524
24. Guarino A, Favieri F, Boncompagni I, Agostini F, Cantone M, Casagrande M. Executive functions in Alzheimer disease: a systematic review. Front Aging Neurosci. (2019) 10:437. doi: 10.3389/fnagi.2018.00437
25. Kassem AM, Ganguli M, Yaffe K, Hanlon JT, Lopez OL, Wilson JW, et al. Anxiety symptoms and risk of cognitive decline in older community-dwelling men. Int Psychogeriatrics. (2017) 29:1137–45. doi: 10.1017/S104161021700045X
26. Yochim BP, Mueller AE, Segal DL. Late life anxiety is associated with decreased memory and executive functioning in community dwelling older adults. J Anxiety Disord. (2013) 27:567–75. doi: 10.1016/j.janxdis.2012.10.010
27. Karr JE, Areshenkoff CN, Rast P, Garcia-Barrera MA. An empirical comparison of the therapeutic benefits of physical exercise and cognitive training on the executive functions of older adults: a meta-analysis of controlled trials. Neuropsychology. (2014) 28:829–45. doi: 10.1037/neu0000101
28. Huo L, Zhu X, Zheng Z, Ma J, Ma Z, Gui W, et al. Effects of transcranial direct current stimulation on episodic memory in older adults: a meta-analysis. J Gerontol Ser B Psychol Sci Soc Sci. (2021) 76:692–702. doi: 10.1093/geronb/gbz130
29. Indahlastari A, Hardcastle C, Albizu A, Alvarez-Alvarado S, Boutzoukas EM, Evangelista ND, et al. A systematic review and meta-analysis of transcranial direct current stimulation to remediate age-related cognitive decline in healthy older adults. Neuropsychiatr Dis Treat. (2021) 17:971–90. doi: 10.2147/NDT.S259499
30. Lee JH, Lee TL, Kang N. Transcranial direct current stimulation decreased cognition-related reaction time in older adults: a systematic review and meta-analysis. Ageing Res Rev. (2021) 70:101377. doi: 10.1016/j.arr.2021.101377
31. Fregni F, Nitsche MA, Loo CK, Brunoni AR, Marangolo P, Leite J, et al. Regulatory considerations for the clinical and research use of transcranial direct current stimulation (tDCS): review and recommendations from an expert panel. Clin Res Regul Aff. (2015) 32:22–35. doi: 10.3109/10601333.2015.980944
32. Kalu UG, Sexton CE, Loo CK, Ebmeier KP. Transcranial direct current stimulation in the treatment of major depression: a meta-analysis. Psychol Med. (2012) 42:1791–800. doi: 10.1017/S0033291711003059
33. Meinzer M, Lindenberg R, Phan MT, Ulm L, Volk C, Flöel A. Transcranial direct current stimulation in mild cognitive impairment: behavioral effects and neural mechanisms. Alzheimer’s Dement. (2015) 11:1032–40. doi: 10.1016/j.jalz.2014.07.159
34. Cruz Gonzalez P, Fong KNK, Chung RCK, Ting KH, Law LLF, Brown T. Can transcranial direct-current stimulation alone or combined with cognitive training be used as a clinical intervention to improve cognitive functioning in persons with mild cognitive impairment and dementia? A systematic review and meta-analysis. Front Hum Neurosci. (2018) 12:1–14. doi: 10.3389/fnhum.2018.00416
35. Kane MJ, Engle RW. The role of prefrontal cortex in working-memory capacity, executive attention, and general fluid intelligence: an individual-differences perspective. Psychon Bull Rev. (2002) 9:637–71. doi: 10.3758/BF03196323
36. Kim C, Johnson NF, Cilles SE, Gold BT. Common and distinct mechanisms of cognitive flexibility in prefrontal cortex. J Neurosci. (2011) 31:4771–9. doi: 10.1523/JNEUROSCI.5923-10.2011
37. Uddin LQ. Cognitive and behavioural flexibility: neural mechanisms and clinical considerations. Nat Rev Neurosci. (2021) 22:167–79. doi: 10.1038/s41583-021-00428-w
38. MacPherson SE, Phillips LH, Della Sala S. Age, executive function, and social decision making: a dorsolateral prefrontal theory of cognitive aging. Psychol Aging. (2002) 17:598–609. doi: 10.1037/0882-7974.17.4.598
39. Tremblay S, Lepage JF, Latulipe-Loiselle A, Fregni F, Pascual-Leone A, Théoret H. The uncertain outcome of prefrontal tDCS. Brain Stimul. (2014) 7:773–83. doi: 10.1016/j.brs.2014.10.003
40. Kennedy SH, Javanmard M, Vaccarino FJ. A review of functional neuroimaging in mood disorders: positron emission tomography and depression. Can J Psychiatry. (1997) 42:467–75. doi: 10.1177/070674379704200502
41. Grimm S, Beck J, Schuepbach D, Hell D, Boesiger P, Bermpohl F, et al. Imbalance between left and right dorsolateral prefrontal Cortex in Major depression is linked to negative emotional judgment: an fMRI study in severe Major depressive disorder. Biol Psychiatry. (2008) 63:369–76. doi: 10.1016/j.biopsych.2007.05.033
42. Chang CC, Yu SC, McQuoid DR, Messer DF, Taylor WD, Singh K, et al. Reduction of dorsolateral prefrontal cortex gray matter in late-life depression. Psychiatry Res Neuroimaging. (2011) 193:1–6. doi: 10.1016/j.pscychresns.2011.01.003
43. Moon CM, Kang HK, Jeong GW. Metabolic change in the right dorsolateral prefrontal cortex and its correlation with symptom severity in patients with generalized anxiety disorder: proton magnetic resonance spectroscopy at 3 tesla. Psychiatry Clin Neurosci. (2015) 69:422–30. doi: 10.1111/pcn.12279
44. Balderston NL, Vytal KE, O’Connell K, Torrisi S, Letkiewicz A, Ernst M, et al. Anxiety patients show reduced working memory related dlPFC activation during safety and threat. Depress Anxiety. (2017) 34:25–36. doi: 10.1002/da.22518
45. Meyer BM, Rabl U, Huemer J, Bartova L, Kalcher K, Provenzano J, et al. Prefrontal networks dynamically related to recovery from major depressive disorder: a longitudinal pharmacological fMRI study. Transl Psychiatry. (2019) 9:1–10. doi: 10.1038/s41398-019-0395-8
46. Sheikh JI, Yesavage JA. Geriatric depression scale (GDS): recent evidence and development of a shorter version. Clin Gerontol. (1986) 5:165–73. doi: 10.1300/J018V05N01_09
47. Pachana NA, Byrne GJ, Siddle H, Koloski N, Harley E, Arnold E. Development and validation of the geriatric anxiety inventory. Int Psychogeriatrics. (2007) 19:103–14. doi: 10.1017/S1041610206003504
48. Smith A. Symbol digit modalities test: Manual. Los Angeles, CA: Western Psychological Services (1982).
49. Reitan RM. The relation of the trail making test to organic brain damage. J Consult Psychol. (1955) 19:393–4. doi: 10.1037/h0044509
50. Wechsler D. Wechsler adult intelligence scale—fourth edition: administration and scoring manual. San Antonio, TX: Pearson (2008).
51. Stroop JR. Studies of interference in serial verbal reactions. J Exp Psychol. (1935) 18:643–62. doi: 10.1037/h0054651
52. Thair H, Holloway AL, Newport R, Smith AD. Transcranial direct current stimulation (tDCS): a Beginner’s guide for design and implementation. Front Neurosci. (2017) 11:1–13. doi: 10.3389/fnins.2017.00641
53. Klem GH, Lüders HO, Jasper HH, Elger C. The ten-twenty electrode system of the international federation. The international federation of clinical neurophysiology. Electroencephalogr Clin Neurophysiol Suppl. (1999) 52:3–6. PMID: 10590970
54. Liao Y-Y, Liu M-N, Wang H-C, Walsh V, Lau CI. Combining transcranial direct current stimulation with tai chi to improve dual-task gait performance in older adults with mild cognitive impairment: a randomized controlled trial. Front Aging Neurosci. (2021) 13:888. doi: 10.3389/fnagi.2021.766649
55. Ambrus G, Al-Moyed H, Chaieb L, Sarp L, Antal A, Paulus W. The fade-in—short stimulation—fade out approach to sham tDCS—reliable at 1 mA for naïve and experienced subjects, but not investigators. Brain Stimul. (2012) 5:499–504. doi: 10.1016/j.brs.2011.12.001
56. Brooks H, Oughli HA, Kamel L, Subramanian S, Morgan G, Blumberger DM, et al. Enhancing cognition in older persons with depression or anxiety with a combination of mindfulness-based stress reduction (MBSR) and transcranial direct current stimulation (tDCS): results of a pilot randomized clinical trial. Mindfulness (N Y). (2021) 12:3047–59. doi: 10.1007/s12671-021-01764-9
57. Kiely KM, Butterworth P, Watson N, Wooden M. The symbol digit modalities test: normative data from a large nationally representative sample of Australians. Arch Clin Neuropsychol. (2014) 29:767–75. doi: 10.1093/arclin/acu055
58. Tombaugh TN. Trail making test A and B: normative data stratified by age and education. Arch Clin Neuropsychol. (2004) 19:203–14. doi: 10.1016/S0887-6177(03)00039-8
59. Grégoire J, Van der Linden M. Effect of age on forward and backward digit spans. Aging, Neuropsychol Cogn. (1997) 4:140–9. doi: 10.1080/13825589708256642
60. Scarpina F, Tagini S. The stroop color and word test. Front Psychol. (2017) 8:557. doi: 10.3389/fpsyg.2017.00557
61. Conradsson M, Rosendahla E, Littbranda H, Gustafson Y, Olofsson B, Lövheim H. Usefulness of the geriatric depression scale 15-item version among very old people with and without cognitive impairment. Aging Ment Health. (2013) 17:638. doi: 10.1080/13607863.2012.758231
62. Nasreddine ZS, Phillips NA, Bédirian V, Charbonneau S, Whitehead V, Collin I, et al. The Montreal Cognitive Assessment, MoCA: a brief screening tool for mild cognitive impairment. J Am Geriatr Soc. (2005) 53:695–9. doi: 10.1111/j.1532-5415.2005.53221.x
63. Bilotta C, Bowling A, Nicolini P, Casè A, Pina G, Rossi SV, et al. Older People’s Quality of Life (OPQOL) scores and adverse health outcomes at a one-year follow-up. A prospective cohort study on older outpatients living in the community in Italy. Health Qual Life Outcomes. (2011) 9:1–10. doi: 10.1186/1477-7525-9-72
64. Arbuthnott K, Frank J. Executive control in set switching: residual switch cost and task-set inhibition. Can J Exp Psychol. (2000) 54:33–41. doi: 10.1037/h0087328
65. McMorris T. History of research into the acute exercise-cognition interaction: a cognitive psychology approach. In: McMorris T, editor. Exercise-cognition interaction: Neuroscience perspectives. London, England: Academic Press (2016). p. 1–28.
66. Doruk D, Gray Z, Bravo GL, Pascual-Leone A, Fregni F. Effects of tDCS on executive function in Parkinson’s disease. Neurosci Lett. (2014) 582:27–31. doi: 10.1016/j.neulet.2014.08.043
67. Bystad M, Storø B, Gundersen N, Wiik IL, Nordvang L, Grønli O, et al. Can accelerated transcranial direct current stimulation improve memory functions? An experimental, placebo-controlled study. Heliyon. (2020) 6:e05132. doi: 10.1016/j.heliyon.2020.e05132
68. Loftus AM, Yalcin O, Baughman FD, Vanman EJ, Hagger MS. The impact of transcranial direct current stimulation on inhibitory control in young adults. Brain Behav. (2015) 5:332. doi: 10.1002/brb3.332
69. Kumar S, Batist J, Ghazala Z, Zomorrodi RM, Brooks H, Goodman M, et al. Effects of bilateral transcranial direct current stimulation on working memory and global cognition in older patients with remitted major depression: a pilot randomized clinical trial. Int J Geriatr Psychiatry. (2020) 35:1233–42. doi: 10.1002/gps.5361
70. Nissim NR, O’Shea A, Indahlastari A, Telles R, Richards L, Porges E, et al. Effects of in-scanner bilateral frontal tDCS on functional connectivity of the working memory network in older adults. Front Aging Neurosci. (2019) 111–10. doi: 10.3389/fnagi.2019.00051
71. Jones KT, Gözenman F, Berryhill ME. The strategy and motivational influences on the beneficial effect of neurostimulation: a tDCS and fNIRS study. Neuroimage. (2015) 105:238–47. doi: 10.1016/j.neuroimage.2014.11.012
72. Berryhill ME, Jones KT. tDCS selectively improves working memory in older adults with more education. Neurosci Lett. (2012) 521:148–51. doi: 10.1016/j.neulet.2012.05.074
73. Krawczyk DC. Contributions of the prefrontal cortex to the neural basis of human decision making. Neurosci Biobehav Rev. (2002) 26:631–64. doi: 10.1016/S0149-7634(02)00021-0
74. Vendetti MS, Bunge SA. Evolutionary and developmental changes in the lateral frontoparietal network: a little goes a long way for higher-level cognition. Neuron. (2014) 84:906–17. doi: 10.1016/j.neuron.2014.09.035
75. Goto K, Hoshi Y, Sata M, Kawahara M, Takahashi M, Murohashi H. Role of the prefrontal cortex in the cognitive control of reaching movements: near-infrared spectroscopy study. J Biomed Opt. (2011) 16:127003. doi: 10.1117/1.3658757
76. Noetscher GM, Yanamadala J, Makarov SN, Pascual-Leone A. Comparison of cephalic and extracephalic montages for transcranial direct current stimulation-a numerical study. IEEE Trans Biomed Eng. (2014) 61:2488–98. doi: 10.1109/TBME.2014.2322774
77. Mahdavi S, Towhidkhah F. Computational human head models of tDCS: influence of brain atrophy on current density distribution. Brain Stimul. (2018) 11:104–7. doi: 10.1016/j.brs.2017.09.013
Keywords: transcranial direct current simulation, executive functioning, depression, anxiety, geriatric, rehabilitation, mental health, neuromodualtion
Citation: Figeys M, Villarey S, Leung AWS, Raso J, Buchan S, Kammerer H, Rawani D, Kohls-Wiebe M and Kim ES (2022) tDCS over the left prefrontal Cortex improves mental flexibility and inhibition in geriatric inpatients with symptoms of depression or anxiety: A pilot randomized controlled trial. Front. Rehabilit. Sci. 3:997531. doi: 10.3389/fresc.2022.997531
Received: 19 July 2022; Accepted: 4 October 2022;
Published: 25 October 2022.
Edited by:
Sarah Donkers, University of Saskatchewan, CanadaReviewed by:
Dhaval Solanki, University of Rhode Island, United StatesAnthony P. Salvatore, University of Louisiana at Lafayette, United States
© 2022 Figeys, Villarey, Leung, Raso, Buchan, Kammerer, Rawani, Kohls-Wiebe and Kim. This is an open-access article distributed under the terms of the Creative Commons Attribution License (CC BY). The use, distribution or reproduction in other forums is permitted, provided the original author(s) and the copyright owner(s) are credited and that the original publication in this journal is cited, in accordance with accepted academic practice. No use, distribution or reproduction is permitted which does not comply with these terms.
*Correspondence: Mathieu Figeys ZmlnZXlzQHVhbGJlcnRhLmNh
Specialty Section: This article was submitted to Interventions for Rehabilitation, a section of the journal Frontiers in Rehabilitation Sciences