- 1SpineX Inc., Los Angeles, CA, United States
- 2International Collaboration on Repair Discoveries (ICORD), Department of Medicine, University of British Columbia, Vancouver, BC, Canada
- 3GirshinPT, Rancho Cucamunga, CA, United States
- 4Rancho Research Institute, Downey, CA, United States
- 5USC Neurorestoration Center, University of Southern California, Los Angeles, CA, United States
- 6Institut Guttmann, Hospital de Neurorehabilitació, Institut Universitari Adscrit a la Universitat Autònoma de Barcelona, Barcelona, Spain
Motor dysfunction in individuals with cerebral palsy (CP) such as the inability to initiate voluntary movements, walking with compensatory movement patterns, and debilitating spasticity is due to the aberrant neural connectivity between the brain and spinal cord. We tested the efficacy of noninvasive spinal cord neuromodulation (SCiP™, SpineX Inc.) with activity-based neurorehabilitation therapy (ABNT) in improving the sensorimotor function in six children with CP. Children received 8 weeks of either SCiP™ or sham therapy with ABNT (n = 3 per group). At the end of 8 weeks, all participants received 8 weeks of SCiP™ therapy with ABNT. Follow up assessments were done at week 26 (10 weeks after the last therapy session). Sensorimotor function was measured by the Gross Motor Function Measure 88 (GMFM88) test. We observed minimal change in sham group (mean 6% improvement), however, eight weeks of SCiP™ therapy with ABNT resulted in statistically and clinically relevant improvement in GMFM88 scores (mean 23% increase from baseline). We also observed reduced scores on the modified Ashworth scale only with SCiP™ therapy (−11% vs. +5.53% with sham). Similar improvements were observed in sham group but only after the cross over to SCiP™ therapy group at the end of the first eight weeks. Finally, sixteen weeks of SCiP™ therapy with ABNT resulted in further improvement of GMFM88 score. The improvement in GMFM88 scores were maintained at week 26 (10 weeks after the end of therapy), suggesting a sustained effect of SCiP™ therapy.
Introduction
Cerebral palsy (CP) is the most common childhood motor disorder affecting 2–4 children in every 1,000 births (1–3). The affected children present with a wide range of functional disorders including inability to move voluntarily, maintain balance and posture, spasticity and abnormal sensation during early development that often worsen with age (4, 5). The primary standard of care (SoC) is physical therapy (PT) (6), potentially with subsequent medication and/or surgery to manage pain and reduce spasticity (7). For children with significant spasticity, SoC often includes selective dorsal root rhizotomy (SDR) (8) and intramuscular injections of OnabotulinumtoxinA (9). While these treatments reduce spasticity, they are invasive, may diminish muscle function, and have minimal effect on voluntary sensorimotor function. For instance, 3 months of standard PT resulted in 5.7 points increase in GMFM88 (10); and intramuscular OnabotulinumtoxinA injections result in 1.7–2.2 points increase in GMFM88 after 1–2 months (7). However, CP children that underwent SDR surgery showed a 6.5 points increase in GMFM66 at 4 months (8). More importantly, the GMFM66 decreased by 20 points 17 years post-surgery (11).
Over the last decade, we and others have extensively shown the therapeutic promise of noninvasive spinal cord neuromodulation in spinal cord injury (12–18). We have previously demonstrated the acute (19) and chronic effects (20) of spinal cord neuromodulation on improvements in sensorimotor function in children with CP. However, the effect of activity-based neurorehabilitation therapy (ABNT) alone compared to spinal neuromodulation with ABNT remains unknown. We hypothesized that children with CP who undergo SCiP™ therapy with ABNT will show greater levels of sensorimotor function improvement as assessed by GMFM88 score, compared to children with CP who undergo inactive sham neuromodulation with ABNT. To test this hypothesis, we performed a single blinded, sham-controlled, one-sided crossover study to investigate the impact of noninvasive spinal neuromodulation with ABNT to improve sensorimotor function in children with CP.
Methods
Six participants diagnosed with CP (GMFCS level I (n = 1), level II (n = 1), level III (n = 1) and level V (n = 3), aged 20 months–8 years) were enrolled in the study (Table 1). The participants demographics and baseline characteristics are described in Table 1. Participants were randomly assigned to either treatment or sham group (n = 3 each). Sham group received 8 weeks of ABNT with sham therapy (2 mA for 1 min followed by 0 mA for 60 min) whereas the treatment group received 8 weeks of ABNT with therapeutic SCiP™ therapy delivered using our proprietary SCiP™ device (SpineX Inc., Los Angeles, CA) (20). The spinal neuromodulation consists of delayed biphasic waveform formed with a carrier pulse (10 KHz) with a 1 µs delay between the two phases (positive and negative). The delayed biphasic carrier (10 KHz) was combined with a low frequency (30 Hz) burst with a pulse width of 1 ms. Neuromodulation was applied using two adhesive electrodes placed between C5–6 and T11–12 vertebral levels serving as the cathodes (1.25″ in diameter), and two adhesive electrodes over bilateral iliac crests as anodes (3 × 5″). A visible motor contraction of any muscle or any involuntary movement induced by the stimulation, identified by the therapist was used to determine thresholds for the two sites (C5–6: 18–22 mA, and T11–12: 16–20 mA). The neuromodulation intensity was initially set at 20% below the threshold for each site. The intensities over the C5–6 spine ranged between 12 and 18 mA and over the T11–12 ranged between 10 and 16 mA depending on the activity being performed by the participant. During activities involving sitting, rolling, etc., the therapist lowered the amplitudes by 2–4 mA prior to initiation of the activity. Whereas, during standing and stepping, the therapist increased the intensities by 1–2 mA prior to initiation of the activity. During the course of a given activity, the intensities would be modulated ±2 mA based on observed functional performance of the child.
Details of ABNT sessions are provided in Table 1. The treatment was administered by a trained pediatric physical therapist. The participants (and parents) were blinded to the randomization group. At the end of 8 weeks, the sham group crossed into the therapeutic group and received 8 weeks of SCiP therapy with ABNT. The treatment group continued SCiP™ therapy with ABNT for another 8 weeks (i.e., total 16 weeks; Figure 1A). Voluntary sensorimotor function was measured as the primary outcome using Gross Motor Function Measure 88 (GMFM88) and muscle tone (spasticity) was measured using the Modified Ashworth Scale as the secondary outcome (22), at baseline, 8 weeks, and 16 weeks (10). Ten weeks after the last therapeutic session, three participants were reassessed for the primary outcome. Primary end point assessment was based on improvement in GMFM88 scores at 8 weeks compared to baseline.
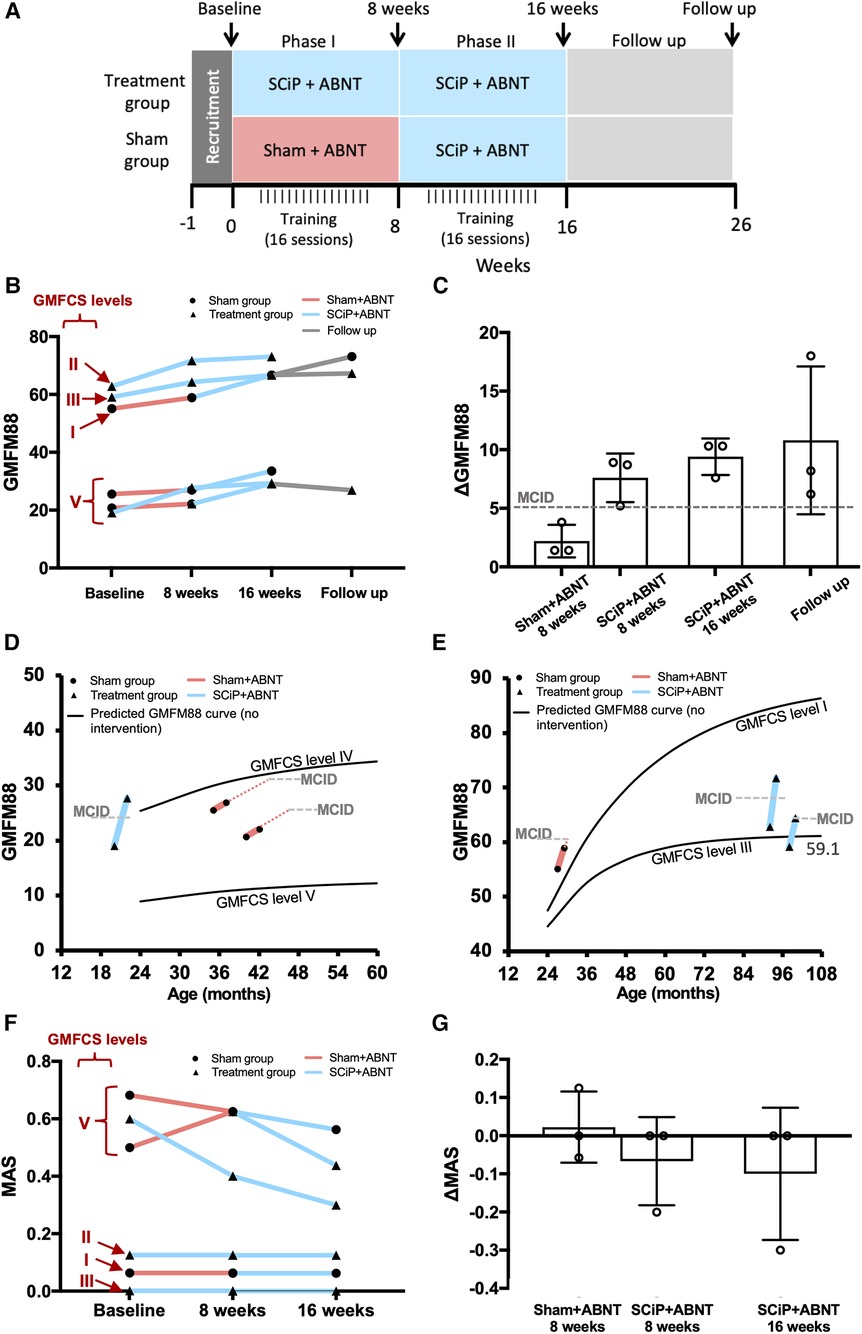
Figure 1. (A) Experimental design and timeline of events. (B) GMFM88 scores at baseline, week 8, week 16 and follow up. 3 participants started with SCiP™ + ABNT (blue) vs. 3 with sham + ABNT (red). Sham group crossed over to SCiP™ at week 8 and all participants received SCiPTM from week 8 to 16, followed by 10 weeks of no intervention (grey). (C) Mean ± SD change from baseline in GMFM88 scores after 8 weeks of sham, 8 weeks of SCiP™, 16 weeks of SCiP™ and follow up (n = 3 each). (D,E) Comparison of GMFM88 scores at the end of 8 weeks (primary efficacy endpoint) between sham (red) and SCiP™ (blue) groups with reference to a validated predicted model of change in GMFM scores without an intervention, matched for age and GMFCS level (21). (F) spasticity scores (MAS) at baseline, week 8 and week 16 for sham (red) and SCiP™ (blue) groups. (G) Mean ± SD change in spasticity scores suggest that children in therapeutic group had lower spasticity at the end of 8 and 16 weeks, compared to baseline.
Results
Eight weeks of SCiP™ therapy resulted in an increase (mean ± SD) in GMFM88 scores by 7.6 ± 2.08 points (minimal clinically important difference; MCID = 5 points) (23), compared to a 2.2 ± 1.38 points increase in the sham group (Figures 1B,C). Further, when the participants from sham arm crossed over and received 8 weeks of therapeutic SCiP™, their GMFM88 increased by 7.13 ± 0.6, equivalent to the treatment group. The participants originally randomized to treatment group continued SCiP™ therapy for another 8 weeks and achieved the ΔGMFM88 score of 9.4 ± 1.5 at week 16 compared to baseline. Interestingly, three participants (1 from treatment group and 2 from sham group) that were reassessed at 26 weeks (i.e., 10 weeks after last SCiP™ therapy session and no further intervention) showed a ΔGMFM88 score of 10.8 ± 6.3 compared to baseline, suggesting a sustained effect of SCiP™ therapy with ABNT. All participants receiving SCiP™ therapy qualified as responders at the primary efficacy endpoint (i.e., ΔGMFM88 > 5 points at 8 weeks), and showed an accelerated functional improvement, compared to the predicted GMFM88 model curve matched for age and GMFCS level (Figures 1D,E) (21). Qualitative observations by the physical therapist and parents suggested meaningful functional improvements in response to SCiP™ therapy, during and post treatment. Table 1 describes the qualitative results for each participant, along with notable feedback from parents. Eight weeks of SCiP™ therapy with ABNT reduced spasticity compared to the sham therapy with ABNT group (ΔMAS −0.06 ± 0.1 SCiP™ vs. +0.02 ± 0.09 sham). Continuation of SCiP™ therapy with ABNT for additional 8 weeks further reduced spasticity score (ΔMAS −0.1 ± 0.1). None of the participants demonstrated an increase in spasticity in response to SCiP™ (Figures 1F,G). No adverse events were reported during the course of SCiP™ therapy with ABNT.
Discussion
To our knowledge SCiP™ therapy with ABNT is the first intervention to show a significant clinical improvement in sensorimotor function in children with CP within a short period of 8 weeks and be able to sustain the improvement for an extended period of time (10 weeks). Our preliminary findings demonstrate greater improvement in sensorimotor function relative to the available standard of care treatment options, reduced spasticity and increased participation in activities of daily living with SCiP™ therapy with ABNT. Although the exact mechanistic understanding of the proposed combination therapy of SCiP™ with ABNT is incomplete, insights can be gained from studies with spinal cord injury and other forms of paralysis. We hypothesize that spinal neuromodulation (SCiP™) transforms the targeted spinal-supraspinal neural networks into an activated state of plasticity, which are made functionally more competent using activity dependent guidance (ABNT), obtained from proprioception (24). The two key findings of this study are (a) the recovery in voluntary motor function even in the absence of active spinal neuromodulation, and (b) the persistence of improved function during the follow up period. While the present study did not directly test the evidence for putative neural plasticity, it has been previously documented in studies investigating neuromodulation-mediated recovery in the spinal cord injury population (25–28). However, since CP and spinal cord injury have distinct pathophysiologies, the mechanism of action responsible for neuromodulation driven changes in sensorimotor function remains unknown. Despite the lack of mechanistic evidence, our initial findings suggest that noninvasive neuromodulation (i.e., SCiP™ therapy) can be a viable option to improve sensorimotor function in CP, and warrants a comprehensive investigation using a randomized control trial with a larger sample size.
Data availability statement
The original contributions presented in the study are included in the article, further inquiries can be directed to the corresponding author.
Ethics statement
The studies involving human participants were reviewed and approved by Advarra IRB. Written informed consent to participate in this study was provided by the participants’ legal guardian/next of kin.
Author contributions
PG, KG and VE: Study design. PG, KG and YS: Study execution. RS, PG and KG: Data analysis. All authors contributed to the article and approved the submitted version.
Funding
The authors acknowledge the funding support from BEL13VE in Miracles Jack Jablonski Foundation, Consortium for Technology & Innovation in Pediatrics, Brain Recovery Project (BRP), Cerebral Palsy Alliance (Australia) and Cerebral Palsy Alliance Research Foundation (USA).
Conflict of interest
PG and VE have Shareholder interest in SpineX Inc. VE has shareholder interest in Onward Medical.
The remaining authors declare that the research was conducted in the absence of any commercial or financial relationships that could be construed as a potential conflict of interest.
Publisher's note
All claims expressed in this article are solely those of the authors and do not necessarily represent those of their affiliated organizations, or those of the publisher, the editors and the reviewers. Any product that may be evaluated in this article, or claim that may be made by its manufacturer, is not guaranteed or endorsed by the publisher.
References
1. Oskoui M, Coutinho F, Dykeman J, Jette N, Pringsheim T. An update on the prevalence of cerebral palsy: a systematic review and meta-analysis. Dev Med Child Neurol. (2013) 55(6):509–19. doi: 10.1111/dmcn.12080
2. Maenner MJ, Blumberg SJ, Kogan MD, Christensen D, Yeargin-Allsopp M, Schieve LA. Prevalence of cerebral palsy and intellectual disability among children identified in two U.S. national surveys, 2011–2013. Ann Epidemiol. (2016) 26(3):222–6. doi: 10.1016/j.annepidem.2016.01.001
3. Christensen D, Van Naarden Braun K, Doernberg NS, Maenner MJ, Arneson CL, Durkin MS, et al. Prevalence of cerebral palsy, co-occurring autism spectrum disorders, and motor functioning—autism and developmental disabilities monitoring network, USA, 2008. Dev Med Child Neurol. (2014) 56(1):59–65. doi: 10.1111/dmcn.12268
4. Graham HK, Rosenbaum P, Paneth N, Dan B, Lin JP, Damiano DL, et al. Cerebral palsy. Nat Rev Dis Primers. (2016) 2:15082. doi: 10.1038/nrdp.2015.82
5. Gajdosik CG, Cicirello N. Secondary conditions of the musculoskeletal system in adolescents and adults with cerebral palsy. Phys Occup Ther Pediatr. (2001) 21(4):49–68. doi: 10.1300/j006v21n04_04
6. Damiano DL. Rehabilitative therapies in cerebral palsy: the good, the not as good, and the possible. J Child Neurol. (2009) 24(9):1200–4. doi: 10.1177/0883073809337919
7. Choi JY, Kim SK, Park ES. The effect of Botulinum toxin injections on gross motor function for lower limb spasticity in children with cerebral palsy. Toxins (Basel). 2019;11(11). doi: 10.3390/toxins11110651
8. Lundkvist Josenby A, Jarnlo GB, Gummesson C, Nordmark E Longitudinal construct validity of the GMFM-88 total score and goal total score and the GMFM-66 score in a 5-year follow-up study. Phys Ther. (2009) 89(4):342–50. doi: 10.2522/ptj.20080037
9. Heinen F, Desloovere K, Schroeder AS, Berweck S, Borggraefe I, Campenhout AV, et al. The updated European consensus 2009 on the use of Botulinum toxin for children with cerebral palsy. Eur J Paediatr Neurol. (Jan 2010) 14(1):45–66. doi: 10.1016/j.ejpn.2009.09.005
10. Ko J, Kim M. Reliability and responsiveness of the gross motor function measure-88 in children with cerebral palsy. Phys Ther. (Mar 2013) 93(3):393–400. doi: 10.2522/ptj.20110374
11. Tedroff K, Lowing K, Astrom E. A prospective cohort study investigating gross motor function, pain, and health-related quality of life 17 years after selective dorsal rhizotomy in cerebral palsy. Dev Med Child Neurol. (2015) 57(5):484–90. doi: 10.1111/dmcn.12665
12. Minassian K, Bayart A, Lackner P, Binder H, Freundl B, Hofstoetter US. Rare phenomena of central rhythm and pattern generation in a case of complete spinal cord injury. Nat Commun. (2023) 14(1):3276. doi: 10.1038/s41467-023-39034-y
13. Keller A, Singh G, Sommerfeld JH, King M, Parikh P, Ugiliweneza B, et al. Noninvasive spinal stimulation safely enables upright posture in children with spinal cord injury. Nat Commun. (2021) 12(1):5850. doi: 10.1038/s41467-021-26026-z
14. Gad P, Gerasimenko Y, Zdunowski S, Turner A, Sayenko D, Lu DC, et al. Weight bearing over-ground stepping in an exoskeleton with non-invasive spinal cord neuromodulation after motor complete paraplegia. Front Neurosci. (2017) 11:333. doi: 10.3389/fnins.2017.00333
15. Sachdeva R, Nightingale TE, Pawar K, Kalimullina T, Mesa A, Marwaha A, et al. Noninvasive neuroprosthesis promotes cardiovascular recovery after spinal cord injury. Neurotherapeutics. (2021) 18(2):1244–56. doi: 10.1007/s13311-021-01034-5
16. Gerasimenko YP, Lu DC, Modaber M, Zdunowski S, Gad P, Sayenko DG, et al. Noninvasive reactivation of motor descending control after paralysis. J Neurotrauma. (2015) 32(24):1968–80. doi: 10.1089/neu.2015.4008
17. Lee S, Gad P, Edgerton VR. Non-invasive spinal cord stimulation to improve hand function in SCI. J Spinal Cord Med. (2017) 40(5):592–3. American Academy of Spinal Cord Injury Professionals Educational Conference 2017, ASCIP 2017. United States. doi: 10.1080/10790268.2017.1351703
18. Zhang F, Momeni K, Ramanujam A, Ravi M, Carnahan J, Kirshblum S, et al. Cervical spinal cord transcutaneous stimulation improves upper extremity and hand function in people with complete tetraplegia: a case study. IEEE Trans Neural Syst Rehabil Eng. (2020) 28(12):3167–74. doi: 10.1109/TNSRE.2020.3048592
19. Gad P, Hastings S, Zhong H, Seth G, Kandhari S, Edgerton VR. Transcutaneous spinal neuromodulation reorganizes neural networks in patients with cerebral palsy. Neurother. (2021) 18(3):1953–62. doi: 10.1007/s13311-021-01087-6
20. Hastings S, Zhong H, Feinstein R, Zelczer G, Mitrovich C, Gad P, et al. A pilot study combining noninvasive spinal neuromodulation and activity-based neurorehabilitation therapy in children with cerebral palsy. Nat Commun. (2022) 13(1):5660. doi: 10.1038/s41467-022-33208-w
21. Palisano RJ, Hanna SE, Rosenbaum PL, Russell DJ, Walter SD, Wood EP, et al. Validation of a model of gross motor function for children with cerebral palsy. Phys Ther. (2000) 80(10):974–85. doi: 10.1093/ptj/80.10.974
22. Bohannon RW, Smith MB. Interrater reliability of a modified ashworth scale of muscle spasticity. Phys Ther. (1987) 67(2):206–7. doi: 10.1093/ptj/67.2.206
23. Storm FA, Petrarca M, Beretta E, Strazzer S, Piccinini L, Maghini C, et al. Minimum clinically important difference of gross motor function and gait endurance in children with motor impairment: a comparison of distribution-based approaches. Biomed Res Int. (2020) 2020:2794036. doi: 10.1155/2020/2794036
24. Edgerton VR, Hastings S, Gad PN. Engaging spinal networks to mitigate supraspinal dysfunction after CP. Front Neurosci. (2021) 15:643463. doi: 10.3389/fnins.2021.643463
25. Anderson MA, Squair JW, Gautier M, Hutson TH, Kathe C, Quentin Barraud Q, et al. Natural and targeted circuit reorganization after spinal cord injury. Nat Neurosci. (2022) 25(12):1584–96. doi: 10.1038/s41593-022-01196-1
26. Samejima S, Henderson R, Pradarelli J, Mondello SE, Moritz CT. Activity-dependent plasticity and spinal cord stimulation for motor recovery following spinal cord injury. Exp Neurol. (2022) 357:114178. doi: 10.1016/j.expneurol.2022.114178
27. Gonzalez-Rothi EJ, Streeter KA, Hanna MH, Stamas AC, Reier PJ, Baekey DM, et al. High-frequency epidural stimulation across the respiratory cycle evokes phrenic short-term potentiation after incomplete cervical spinal cord injury. J Neurophysiol. (2017) 118(4):2344–57. doi: 10.1152/jn.00913.2016
Keywords: spinal cord neuromodulation, noninvasive stimulation, cerebral palsy, sensorimotor function, spasticity
Citation: Sachdeva R, Girshin K, Shirkhani Y, Gad P and Edgerton VR (2023) Combining spinal neuromodulation and activity based neurorehabilitation therapy improves sensorimotor function in cerebral palsy. Front. Rehabil. Sci. 4:1216281. doi: 10.3389/fresc.2023.1216281
Received: 3 May 2023; Accepted: 10 July 2023;
Published: 26 July 2023.
Edited by:
Ping Zhou, University of Health and Rehabilitation Sciences, ChinaReviewed by:
Gustavo Balbinot, University Health Network (UHN), CanadaSamit Chakrabarty, University of Leeds, United Kingdom
© 2023 Sachdeva, Girshin, Shirkhani, Gad and Edgerton. This is an open-access article distributed under the terms of the Creative Commons Attribution License (CC BY). The use, distribution or reproduction in other forums is permitted, provided the original author(s) and the copyright owner(s) are credited and that the original publication in this journal is cited, in accordance with accepted academic practice. No use, distribution or reproduction is permitted which does not comply with these terms.
*Correspondence: Rahul Sachdeva cmFodWxAc3BpbmV4LmNv