- School of Civil Engineering, University of Leeds, Leeds, United Kingdom
Despite the relatively benign characteristics of construction and demolition waste, its mismanagement can result in considerable harm to human health for 200 million workers and those who live and work in proximity to construction and demolition activities. The high number of workers classified as informal, results in a large unregulated and vulnerable workforce at a high risk of exposure to hazards. We focused a systematic scoping review (PRISMA-ScR) on evidence associating construction and demolition waste with hazards and risks in low- and middle-income countries. We reviewed more than 3,000 publications, narrowed to 49 key sources. Hazard-pathway-receptor scenarios/combinations were formulated, enabling indicative ranking and comparison of the relative harm caused to different groups. Though the evidential basis is sparse, there is a strong indication that the combustible fraction of construction and demolition waste is disposed of by open burning in many low- and middle-income countries, including increasing quantities of high chloride-content PVC; risking exposure to dioxins and related compounds. A long-standing and well-known hazard, asbestos, continues to represent a health threat throughout the world, claiming 250,000 lives per annum despite being banned in most countries. In the coming decades, it is anticipated that more than half of all deaths from asbestos will take place in India, where it is still sold. Comparatively, the highest risks from construction and demolition waste exist in low- and middle-income countries where attention to risk mitigation and control is needed.
Introduction
Construction and demolition waste (CDW) receives considerably less attention in the literature compared to municipal solid waste (MSW), despite being a huge global contributor toward total solid waste generation (estimated 36% wt.) (Wilson et al., 2015). Partly, this can be explained because many of the constituents of CDW are comparatively benign. CDW is characterized (on a weight basis) by comparatively high-density materials such as concrete, bricks, metals, soil and gypsum, as well as plastics, along with of a range of composites and assemblies of items and other materials (Supplementary Section S.5.2). Because it is often generated during commercial (non-domestic) activities, any negative effects on the environment or public health emerge away from the public eye.
CDW is defined as the material generated during: construction of new buildings; renovation of old structures; partial deconstruction of buildings; and during full building demolition. Both construction and demolition wastes share characteristics in that they are comprised of materials that were intended for a similar purpose, however, the mode of generation is often quite different and the materials themselves are subject to quite different conditions during the use-phase. Construction waste could be considered as more easily controlled and separated compared to demolition waste, as its constituents are yet to be bonded into complex assemblies and structures, and are therefore more easily identifiable and separable. For this reason, in high income countries (HICs), CDW has been managed with increasing resource recovery and wider circularity over recent decades (Ginga et al., 2020).
Historically, the construction and demolition sector has had a poor record for injury and deaths (Sirrs, 2016). Globally, this trend appears to be ongoing, according to data published by the International Labour Organization (2020a), indicating that 20% of all workplace fatalities reported to its database were in the construction industry, more than double the proportion of people working in the sector (8.6%) (Mella and Savage, 2018). Possibly close to a quarter of a billion people work in the construction sector, of which as much as 80% can come from the informal economy in low- and middle-income countries (LIMICs) and 16% in high income countries (Jewell et al., 2005). In fact, in countries such as India, ~96–97% of the workforce is estimated to be informal (Mella and Savage, 2018). The high inferred accident and fatality rate and the level of informality in this sector may have profound consequences for the health, safety and wellbeing for construction and demolition workers. According to strong anecdotal evidence, informal workers are less likely to operate with safe systems of work, less likely to have medical insurance and often work without personal protective equipment; leaving them with a much higher vulnerability to exposure from chemical and particle exposure (e.g., asbestos) and accidents (Ferronato and Torretta, 2019).
A posteriori evidence suggests that understanding the types of activity that result in injuries or fatalities and the type of accident itself is crucial to developing safe systems of work to mitigate the probability of them occurring in the future. As CDW management is a subset of the construction sector, accident and safety data are rarely reported separately. Several narrowly scoped reviews address demolition safety, such as by Ertaş and Erdogan (2017), who investigated accident data in the UK and Australia; and by Gürcanli and Müngen (2013), who carried out a similar study analyzing prosecution records in Turkey. Several recent efforts that are partly related to the safety topic include Chen et al. (2021), who carried out analysis of the environmental effects of CDW; and Molla et al. (2021), who investigated chemicals of concern in CDW. A large body of evidence also exists regarding the occupational and public health implications of the management of specific CDW components; the most prominent being asbestos, which has claimed many thousands of lives since its commercialization in the early 20th century and which is expected to continue to do so for several more decades (Driscoll et al., 2005; Odgerel et al., 2017; Furuya et al., 2018; Stevulova et al., 2020). However, there are no reviews on CDW management and its safety in the public domain that is disaggregated from the wider topic of construction and demolition.
In response to this research gap and its relevance due to the large and inherently vulnerable global workforce and the large quantities of CDW being generated, we carry out here a systematic review, taking a material flow systems approach (Figure 1). We bring together, for the first time, a wide range of literature, indicating occupational and public health risks from CDW. The review is organized into three activity-based generic “challenges”: (1) Handling and physical processing; (2) Land disposal; and (3): Thermal deconstruction and processing. The intention of these groupings is to help the reader link identified safety challenges with practiced CDW management activities.
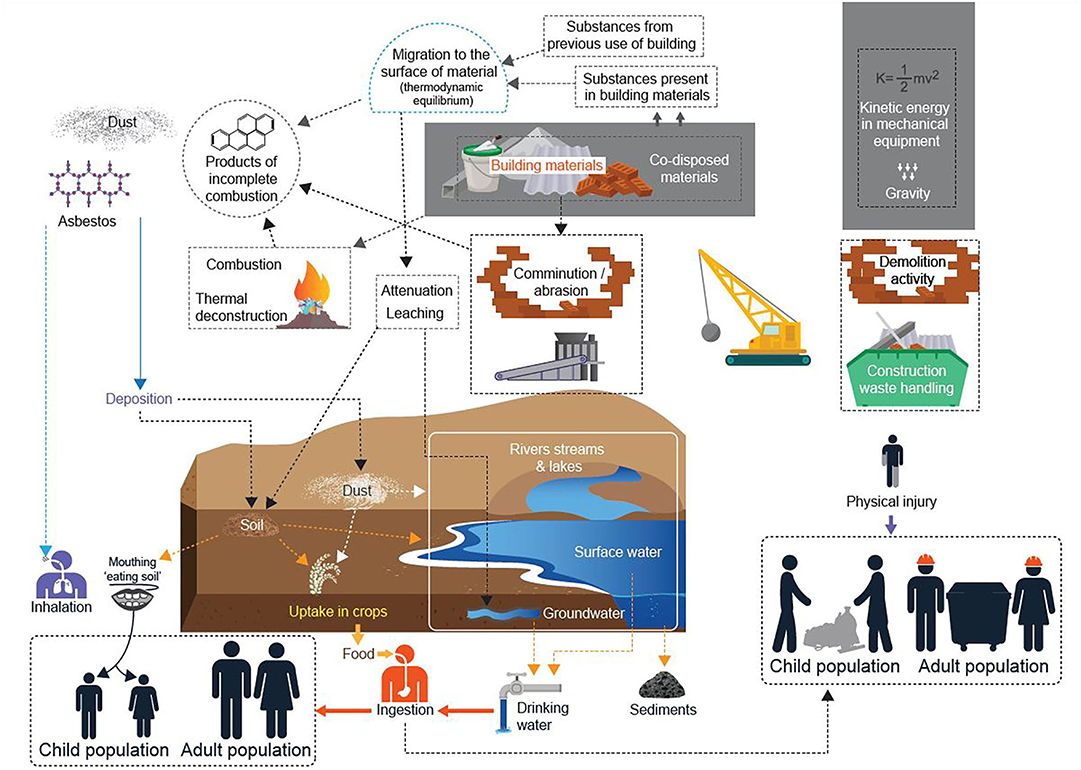
Figure 1. Summary of the main hazards associated with construction and demolition waste (CDW) and the pathways through which they may result in exposure to receptors.
For each challenge, we arrange individual risks into hazard-pathway-receptor combinations and semi-quantitatively assign risk scores to indicate and rank the relative harm of activities within the sector. The focus is on LIMICs, where well-resourced, independent environmental regulation is not always guaranteed. However, the dearth of research in LIMICs means that many of the papers reviewed are taken from the HIC context. We exclude the management of CDW from disasters, war and conflict, as these very specific sub-cases warrant a specialist review (shown outside the CDW system boundary in Supplementary Figure S5). In addition, we do not address risks from the wider construction activities, but focus only on those that relate to CDW itself. The preprint of this paper is available at https://doi.org/10.31224/osf.io/5tpbz (Cook and Velis, 2022).
Methods
Systematic Review
We carried out a systematic scoping review following PRISMA-ScR guidelines (Peters et al., 2020) and using the preferred reporting items listed by Tricco et al. (2018) (Supplementary Section S2) to explore the following research questions (RQ):
• RQ1: What evidence exists to indicate risk to public and occupational safety posed by CDW?
• RQ2: What are the comparative risks to public and occupational safety that arise from the management of CDW?
One-at-a-time sensitivity analysis (Hamby, 1995) was used to optimize Boolean search terms (Supplementary Section S.3.1) in order to retrieve the maximum number of relevant articles, whilst reducing the non-relevant from Scopus, Web of Science and Google Scholar. Sources were selected for inclusion according to the criteria in Supplementary Section S.3.2 (Supplementary Table S2). Snowball and citation searching (Cooper et al., 2018) was used to obtain further relevant information that had not been revealed during the systematic search. Several further relevant sources were searched in more detail, such as Health Safety Executive (2020b); International Labour Organization (2020b); The World Bank (2020); World Health Organization (2020), (HSE). A summary of the results of search decisions is illustrated in Supplementary Section S.3.3 (Supplementary Figure S1) and geographical provenance of the first author's affiliated institution is shown in Supplementary Section S.3.4 (Supplementary Figure S2).
Risk Based Approach
A semi-quantitative risk assessment process detailed in Supplementary Section S.3.5 (Supplementary Table S4) was used to characterize risks associated with CDW based on an approach adapted from Hunter et al. (2003); World Health Organization (2012); Kaya et al. (2018) and Burns et al. (2019); the adaptation was first described by Cook et al. (2020) and elaborated by Velis and Cook (2021). Combinations of hazards, pathways, and receptors were identified from the literature and arranged on the basis of realistically experienced scenarios as described by Cook et al. (2020); enabling the preparation of source-pathway-receptor flow diagrams as illustrated in (Figure 1). Each hazard-pathway-receptor combination was indicatively assigned a risk score based on the likelihood of it occurring and potential severity to different receptors (Supplementary Section S.3.5 and Supplementary Table S5). Scores were assessed separately for HICs and LIMICs. This process was not intended to quantitatively assess risk, but to indicate and rank the relative risks to prioritize future research agenda; the combined and ranked results are shown in Supplementary Section S.4 (Supplementary Table S6).
Challenge 1: Handling and Physical Processing of Construction and Demolition Waste
Context
The hazard-pathway-receptor (H-P-R) linkages necessary for potential hazards to be actualized on construction and demolition sites as a consequence of handling and physical processing are shown in Supplementary Figure S6. Broadly these are delineated here by: hazards that exist from materials or substances in construction waste or the previous use of buildings, and physical accidents that take place during deconstruction (demolition) and/or waste removal activities.
Accidents Involving Construction and Demolition Waste
According to data from 2016, 20% of all workplace fatalities occurred in the construction and demolition sector (International Labour Organization, 2020a) which has been reported to employ 8.6% of all workers (Mella and Savage, 2018).
Eurostat (2020) reports accidents requiring more than 3 days absence from work (hereafter “>3 day accidents”) and fatalities for European Union member states (European Union, 2008b). Very little change was reported for the number of accidents and fatalities between 2014 and 2017, with ~3,000 >3 day accidents per 100,000 people and around 5-6 fatalities reported for the construction related NACE economic categories (Supplementary Figure S7A). Accidents and fatalities involving CDW are not well reported. Though not obliged to do so (European Commission, 2009), some member states provide more granular, “Phase III” information for “bulk waste” (no definition is provided for this term) (Eurostat, 2010). As shown in Supplementary Figure S7B, ~5 were reported each year between 2014 and 2017 and no fatalities were reported in 2016 or 2017.
Accidents or near misses that do not result in significant injury are not included in the statistics (European Commission, 2009) and it is likely that many accidents go unreported (Eurostat, 2019), for instance by self-employed people; uninsured people (in states where the data originate from the insurance industry); and public sector, mining and fishing workers, who may be covered under specific insurance schemes that do not necessarily submit data (European Commission, 2009). It is also suggested here that several types of accident involving waste are likely to be omitted from this category and included in others. For instance, exposure to asbestos waste and demolition are grouped under the title “construction” rather than being reported under distinct sub-categories.
Accidents During the Demolition Phase
Demolition work is often expected to be faster and less costly than construction work and hence, sometimes results in shortcuts being taken at the expense of occupational health and safety (Ertaş and Erdogan, 2017). Nonetheless, this section reveals that there is little specific data to evidence the risk of accidents resulting from demolition; a premise supported by at least two other authors (Zaharuddin et al., 2009; Ertaş and Erdogan, 2017; Takahashi, 2019). Hence, the level of risk exposure to accidents from this important waste activity is poorly understood.
Three sources of information indicate the number of injuries and fatalities from demolition activities as a proportion of all activities (Table 1). European Commission (2009) reported a specific Phase III sub-category of “Demolition” for the EU15 in 2005. As a proportion of injuries from all sectors, it indicated that 0.16% of all >3 day injuries and workplace fatalities combined occur in the demolition sector compared to 0.4% in Australia, as reported by Zaharuddin et al. (2009). In the EU15, the demolition sector reported a higher proportion (0.71%) of fatalities in comparison to the >3 day injuries. The only other data point that evidences fatalities in the demolition sector is reported by Maeda et al. (2003) who observed fatalities to be higher than European Commission (2009) by a factor of 10 in a single city in Japan between 1996 and 2001. There is insufficient evidence from the two studies to explain this large disparity. However, there may be a variety of factors specific to the local conditions in Japan, such as corporate attitudes toward safety; differing regulatory framework; or possibly the influence of a single company's record. Although the Maeda et al. (2003) dataset is small in comparison to the European Commission dataset, other studies (Evans, 2014) have suggested that fatality data are unlikely to be under-reported unlike accidents, which may not be. In any case, such a high comparative fatality rate warrants further investigation in Japan and other parts of the world to understand the proportion of demolition sector injuries and fatalities.
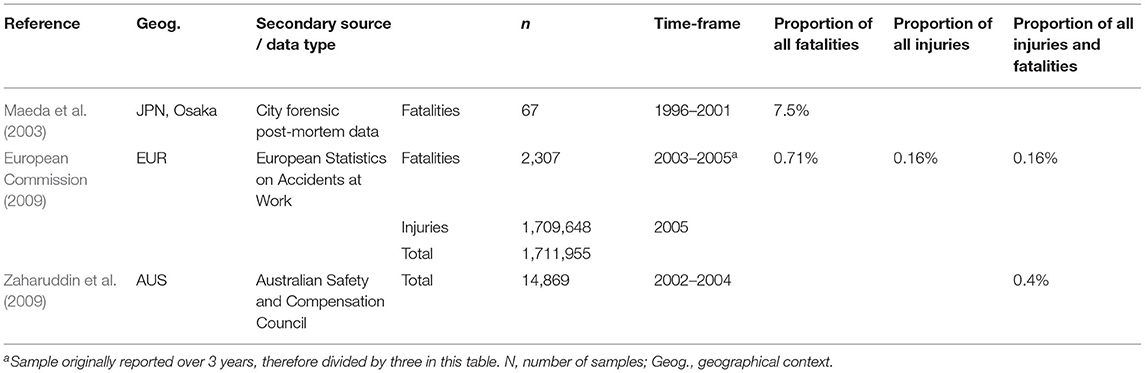
Table 1. Injuries and fatalities from demolition activities as a proportion of injuries and fatalities from all sectors.
Accident data for the demolition sector as a proportion of construction and demolition as a whole are more numerous than data reported as a proportion of all accidents, with five sources identified (Table 2). Whereas, the proportions of fatalities and >3 day injuries by the European Commission (2009) in Table 2 are broadly proportional to those reported in Table 1, the overall proportion of accidents and fatalities from demolition reported by Zaharuddin et al. (2009) are nearly six times higher. The Zaharuddin et al. (2009) dataset is smaller, and from an earlier timeframe, and the UK has greatly improved its health and safety record for the construction and demolition sector since, as evidenced from the later and larger UK dataset reported by Ertaş and Erdogan (2017), indicating the accident rate has halved.
The data reported by Takahashi (2019) of demolition fatalities over a 5 year period in Japan (Table 2), have some similarity with the data reported by Maeda et al. (2003) for Osaka, Japan (Table 1), in that the fatality rate of the sector is ~6 times higher than the European Commission and nearly twice the proportion reported by Gürcanli and Müngen (2013) in their study of eye-witness court testimonies in Turkey. For instance, recent analysis by Shim et al. (2022) of risk on construction sites in Japan showed the fatality rate to be almost six times great than the UK.
Takahashi (2019) noted that it was common practice among demolition workers in Japan to cut a hole in the floor of a building under deconstruction to pass valuable scrap metals through for recycling and that the technique for demolishing walls was to manually weaken the bottom before mechanically pushing walls over with a mechanical plant. While limited evidence was revealed to indicate the underlying causality of accidents, the testimony by Takahashi (2019) ought to provide the basis for further investigation of attitudes toward safety in specific cultures. Japan is an HIC, and intuitively ought to possess the resources necessary to train and equip its workforce to carry out potentially hazardous activities under a safe system of work. There is one suggestion that the strict hierarchy in Japan is a hindrance to health and safety implementation (Shoji and Egawa, 2006). Speculatively, if conditions are as hazardous as the Japan data suggests, then it is conceivable that many LIMICs with less rigid regulatory frameworks and less ingrained health and safety culture may also have a poor accident and fatality record.
In studies from both the USA (Ertaş and Erdogan, 2017) and Japan (Takahashi, 2019), demolition workers were most likely to suffer a fatality as a result of a fall from height or a building collapse (Table 2). Zaharuddin et al. (2009) reported a similar proportion for demolition workers in the UK, with ~53% of fatal and non-fatal accidents being caused by collapse and 28% caused by a fall. Whereas, the data for these four accident types shows some congruence, other accident types reported in Table 2 are less consistent, making the data challenging to compare.
Analysis by Gürcanli and Müngen (2013) of eye-witness accounts of accidents over 36 years in the Turkish construction sector provides some indication of the types of demolition activity that resulted in injuries and fatalities over that period (Supplementary Table S9). Compared to other reports, the injury and fatality data appear low in absolute number terms, with less than one fatality and slightly more than one injury over the 36 year period, compared to Japan which reports ~21 fatalities per year between 2010 and 2014 (Takahashi, 2019) (Table 2).
Gürcanli and Müngen (2013) cautioned that official Turkish statistics are unreliable indicators of accidents because they only report injuries and fatalities for which a conviction was successful. Moreover, they noted that Turkey has no specific health and safety legislation, suggesting that the lack of regulatory framework would result in an accident rate that far exceeded other countries where a framework exists. If there is a societal aspiration to reduce accidents across the demolition sector, then the data shown in this section highlight the need for a more harmonized global system of reporting, without which cost-effective interventions cannot be targeted where most needed.
Asbestos
Asbestos is the generalized term used to describe a group of six main types (Supplementary Table S10) of naturally occurring fibrous silicate minerals that have been used in a variety of commercial and industrial applications for many thousands of years (Furuya et al., 2018).
After it was first commercially extracted in Quebec in 1876 (Henderson and Leigh, 2012), asbestos production rose sharply following the Second World War, reaching its peak in 1980 (Supplementary Figure S8), after which concerns over its safety resulted in successive bans of specific asbestos applications and materials across Europe and in the US (Kazan-Allen, 2019a); although there is still no outright asbestos ban in the US (Arachi et al., 2021). Both amphiboles (crocidolite and amosite) were effectively banned by the mid-1980s in most western countries and chrysotile asbestos has been banned in many countries since. According to Flanagan (2020), reliable data on global asbestos production and consumption have not been published since 2017 when Russia supplied nearly two thirds of the 1.1 Mt consumed worldwide, while Brazil produced around 12% (Supplementary Figure S9). But there is an apparent continuing downward trend in production, and in 2020 Brazil closed its last mine extracting asbestos.
Asbestos was still consumed in 39 countries in 2017, with India, China and Russia representing over 60% of global consumption (Supplementary Figure S10). Although the data since 2010 indicates a general reduction in consumption in Russia and China, continued use is apparent in India (Supplementary Figure S11), where there are no restrictions on its production and consumption (Jadhav and Gawde, 2019). Specific data are not available, but it has been reported that in India almost all asbestos is used in cement bonded sheet material (Burki, 2010), and the International Chrysotile Association ((nd)) reports a similar picture elsewhere. Other applications continue, including: insulating protective equipment for fire-fighting and brakes for automobiles (Frank, 2006; Henderson and Leigh, 2012; Ogunseitan, 2015).
Asbestos fibers are mineral and do not volatilize so they only represent a hazard when they have been weathered or otherwise abraded from the material after which solid particles can easily aerosolize (i.e., become suspended in the atmosphere) and be potentially inhaled (IARC Working Group on the Evaluation of Carcinogenic Risks to Humans, 2012). In the lungs, the barbed asbestos particles become lodged, causing inflammation and scarring over time, and resulting in several diseases. The link between mesothelioma, a malignant cancer of the pleura, and both occupational and non-occupational exposure to asbestos was established in the 1960s (Henderson and Leigh, 2012) and since then, occupational asbestos exposure has been the subject of more than 100 cohort studies and several reviews (Concha-Barrientos et al., 2004); the World Health Organization estimates that ~125 million living people have been exposed (Spasiano and Pirozzi, 2017).
Estimates of the number of deaths caused by asbestos exposure vary. Although it is linked to several diseases, including lung cancer, ovarian cancer and kidney cancer (Frank and Joshi, 2014), the death rate for mesothelioma is a strong indicator as virtually all are thought to be a result of asbestos exposure (Driscoll et al., 2005; Stayner et al., 2013). The World Health Organization (2019) Mortality Database provides a record of reported cases of mesothelioma from countries that submit data. Delgermaa et al. (2011) analyzed the database entries from 1994 to 2008, finding generally low levels of reporting. For instance, in 1995 just four countries submitted data, rising to 75 in 2003 and 100 by 2007. As shown in Supplementary Table S11, cases were almost three times higher between 2001 and 2008 compared to 1994 to 2000. Almost 88% of cases reported were in high income countries, with negligible numbers reported in low income countries. This is partly explained by the number of countries that submitted data; clearly much greater in the HICs. However, mesothelioma is still a comparatively rare condition that is not always easy to diagnose; often requiring cumulative experience which can take many years for the medical profession to accumulate (Odgerel et al., 2017). Therefore, it is likely that many cases in LIMICs are not classified as mesothelioma and are consequently not reported rather than the low numbers of cases being a reflection of safe working practices around asbestos (Li et al., 2014). Moreover, the median latency (period from exposure to death) for mesothelioma has been estimated at nearly 23 years (Frost, 2013) which means that many cases will not arise in countries with lower life expectancy.
Taking the arithmetic mean of the global deaths reported between 2001 and 2008 (Supplementary Table S11), indicates 8,748 annual cases of mesothelioma each year, considerably lower than a previous estimate of 43,000 per annum estimated in 2005 by Driscoll et al. (2005). To estimate the unreported cases, Odgerel et al. (2017) used the World Health Organization (2019) Mortality Database to model deaths from mesothelioma in the countries that either did not report or appeared to underreport. The study based the estimates on the historical use of asbestos in each country, the level of employment in the construction sector, and the continental average. For example, in Figure 2 the continental adjusted data are compared with the reported data, revealing huge underreporting in Asia and Africa.
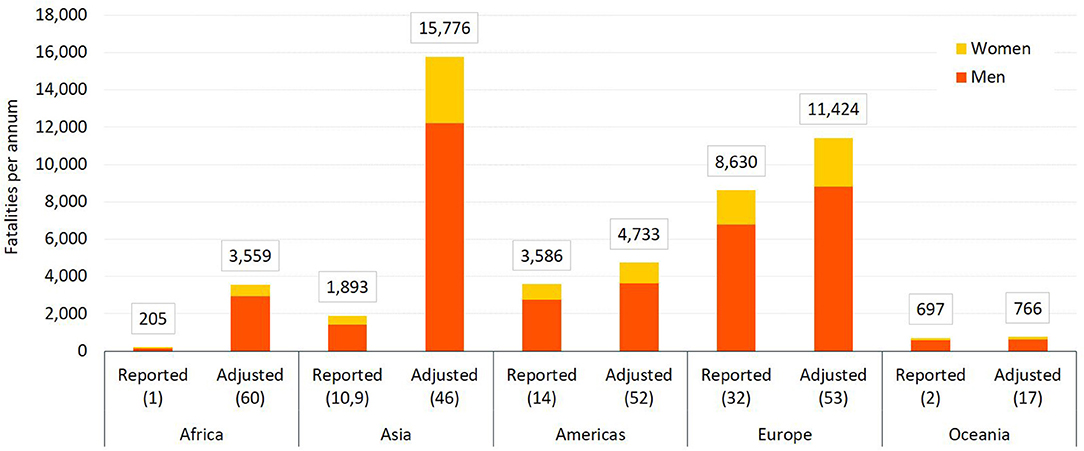
Figure 2. Global mesothelioma deaths region reported to the World Health Organization (2019) alongside adjusted data calculated by Odgerel et al. (2017). Numbers in boxes at the top of bars represent the total of fatalities per category. Numbers in brackets represent the number of countries reporting deaths within each region.
As with the reported data, the proportion of people dying from mesothelioma in the estimated (adjusted) data who were women was ~23%, a likely reflection of the number of men working in construction compared to women worldwide (Figure 2). When stratified by the World Bank income category for countries (The World Bank, (nd)), the differences between the reported deaths and modeled deaths are stark (Supplementary Figure S12), with virtually all reporting taking place in HICs and almost none in lower middle income countries (LMCs) or low income countries (LICs).
Odgerel et al. (2017) proffered their “asbestos use” adjustment as the most reliable estimate of the three adjustments; with an annual average death rate of 38,400, it was fairly close to the 43,000 estimated by Driscoll et al. (2005) a decade earlier. However, although mesothelioma deaths are a reliable indicator, they are only one of several diseases that are attributable to asbestos exposure. Various estimates have been suggested for the total number of deaths from all asbestos related diseases, ranging from 90,000 to 112,000 (Henderson and Leigh, 2012; Furuya et al., 2018). Estimates by Furuya et al. (2018) (Figure 3) suggested that the real figure may be as much as 255,000 deaths (243,223 to 260,029) of which 233,000 deaths (222,322 to 242,802) are occupational, with the greatest contribution from lung cancer, particularly in HICs. Other diseases made a comparatively small contribution to global mortality from occupational exposure to asbestos, with ~2,000 in each of UMCs and LMCs, and <300 in LICs.
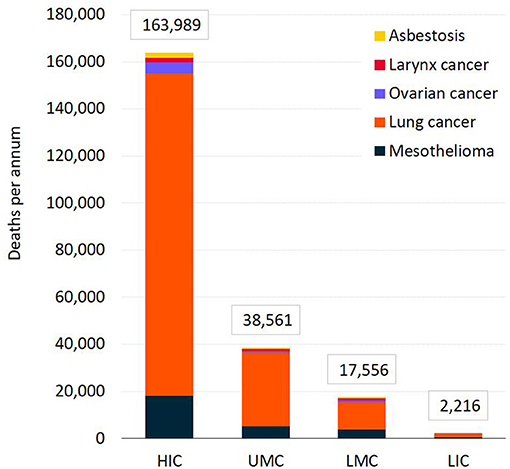
Figure 3. Global deaths from all diseases as a result of occupational exposure to asbestos; data from Furuya et al. (2018). Numbers in boxes at the top of bars represent the total of deaths per category. HIC, high-income country; UMC, upper-middle-income country; LMC, lower-middle-income country; LIC, low-income country.
As of July 2019, 67 countries have banned asbestos (Kazan-Allen, 2019b), yet it is likely that the pandemic of asbestos related deaths is likely to continue to increase in the future despite apparent reductions in some countries, such as Sweden and the Netherlands which were some of the early countries to ban asbestos in the 1970s (Stayner et al., 2013). The analysis by Furuya et al. (2018) indicated that the current death rate from asbestos may continue in future, at least at the same rate, acknowledging that the lack of data in LIMICs makes these kind of predictions highly uncertain.
Nonetheless, while countries such as India continue to permit unabated consumption of asbestos, it is likely that the death rate from asbestos exposure will continue to rise (Frank and Joshi, 2014), especially as life expectancy increases (Singh and Ladusingh, 2013) and exposed populations survive the latency period for mesothelioma (Frost, 2013). It has been predicted that of the 1.25 million people who are expected to suffer from asbestos related cancer in the coming years, more than half will be in India (Jadhav and Gawde, 2019).
Other Particulate Matter
Collectively, construction and demolition activities are an important source of particulate matter (PM) emissions (Font et al., 2014). In London (UK), for instance, construction and demolition activities were estimated to contribute to 1.4% of total PM10 emissions in 2010 (Font et al., 2014) and a study of over 80 sites across the city by Fuller and Green (2004) between 1999 and 2001 found that construction and demolition activities contributed to mean daily concentrations of >50 μg.m−3 at 25% of the sites observed each year.
This review has a specific focus on “construction waste” and “demolition waste and activities,” therefore “construction activities” that do not involve waste are excluded. This presents a challenge in this section because most studies of PM emissions present data that is aggregated together with “construction,” “demolition” and “construction waste.” Furthermore, because this section focuses on mechanical (non-thermal) emissions of PM, the scope is narrowed further to focus on emissions that arise when materials, such as ceramics, undergo mechanical attrition and aerosolisation. Here, we will discuss “dust”, defined variously as PM that is <75 or <100 μm in diameter (World Health Organization, 1999).
Two studies in the UK (Stacey et al., 2011) and Iran (Normohammadi et al., 2016) reported concentrations of total dust and respirable silica (a sub-category of dust) in and around demolition sites (Table 3). The first, Stacey et al. (2011) visited 13 construction and demolition sites in the UK and found that the concentrations of respirable dust were not significantly different from background samples, except for the demolition activity, which showed a significantly different concentration (p < 0.001). Silica dust exposure is an increasing public and occupational health issue and is known to cause silicosis, a fibrotic disease of the lung (Leung et al., 2012) as well as being linked to lung cancer, pulmonary tuberculosis and other diseases as well as an indicative, but less studied, link with cardio-vascular diseases (Chen et al., 2012). Nonetheless, although the time weighted average concentrations of respirable silica reported by Stacey et al. (2011) were higher during demolition activities, they were still far below the recently imposed absolute limit of 100 μg.m−3 stipulated in Directive (EU) 2017/2398 (European Union, 2009).
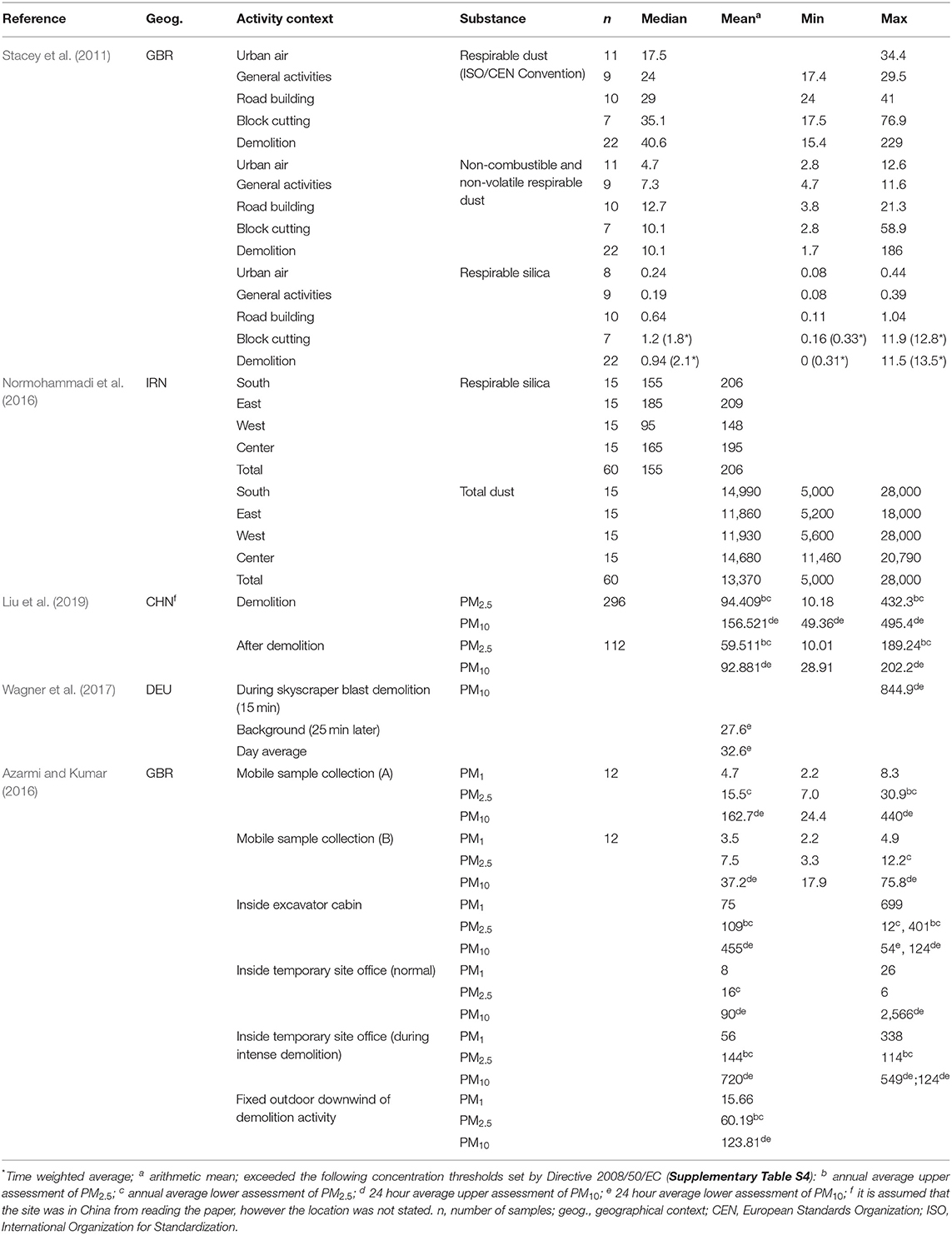
Table 3. Concentrations of particulate matter (PM) in air around construction and demolition activities (μg.m−3).
The The Air Quality Standards Regulations (2010) in the UK, require that PM <10 μm (PM10) concentrations must not exceed 50 μg.m−3 more than 35 times per year or an annual mean of 40 μg.m−3. Although the PM10 was not measured specifically, two of the concentrations for total dust measured by Stacey et al. (2011) were higher than 50 μg.m−3, for block cutting and demolition (Table 3). However, the majority were below the 50 μg.m−3 threshold and although the average for the demolition site for respirable dust (defined as the portion of PM that is capable of reaching the alveoli—gas exchange sacs in the lungs) was slightly higher than the mean average concentration limit in The Air Quality Standards Regulations (2010), the activity did not last for a year and therefore would not exceed the threshold.
The mean and median concentrations of respirable silica measured by Normohammadi et al. (2016) at a demolition site in Tehran, were ~100 and 200 times higher, respectively, than those observed by Stacey et al. (2011) (Table 3). All the mean concentrations identified by Normohammadi et al. exceeded the absolute limit of 100 μg.m−3 stipulated in Directive (EU) 2017/2398 (European Union, 2009), indicating that exposure to workers near these activities was possibly negatively affecting their health.
Three further studies measured concentrations of PM in and around demolition sites in China, Germany and the UK (Table 3), with many concentrations exceeding the threshold concentrations in Directive 2008/50/EC (European Union, 2008a) (Supplementary Table S8). Liu et al. (2019) measured concentrations of PM10 and PM <2.5 μm (PM2.5) during and following the demolition of a teaching building, finding elevated levels of both particle size profiles during the demolition process. Levels remained high after the demolition activities, indicating a very high background concentration in the area that exceeds the thresholds in Directive 2008/50/EC. Wagner et al. (2017) also found a considerable difference between PM levels during and after blast demolition of a skyscraper in Frankfurt, Germany, with maximum concentrations nearly 16 times greater than the limit value of 50 μg.m−3. Both Liu et al. (2019) and Wagner et al. (2017) reported that atmospheric PM concentrations returned to background levels when demolition was not taking place, indicating that the PM generated were either easily dispersed or deposited to the land. In the case of Wagner et al. (2017), the PM cleared within 25 min, meaning that the daily average concentration only slightly exceeded the 24 h lower threshold (25 μg.m−3) for PM10 stated in the Directive 2008/50/EC.
The study by Azarmi and Kumar (2016) took place over seven days and involved measuring concentrations in a variety of locations near to the demolition of a building, including static sampling sites; inside a static portable office; inside an excavator and also mobile sampling around the site (Table 3). Both the downwind and one of the mobile samplers showed levels of PM10 that exceeded the 50 μg.m−3 limit, as well as the 24 h upper and lower assessment thresholds. The levels in the excavator were 6.5 times higher than the fixed outdoor sampler exceeding the 50 μg.m−3 threshold by nine times. Even more concerning were the concentrations at the temporary site office that reached levels of more than 14 times the threshold limit during a period of intense demolition. Speculatively, office environments are often considered relatively safe spaces on construction and demolition sites and respiratory protection equipment was rarely worn inside prior to the SARS-CoV-2 pandemic. Azarmi and Kumar (2016) showed that the concentrations were higher inside the office than anywhere else on the site, inferring a potential need for engineering controls or procedures to prevent the ingress of PM or to ventilate buildings.
The relative contribution of demolition activities compared to construction and excavation activities was investigated by Arocho et al. (2014) who monitored air concentrations around two road resurfacing projects in the US (Supplementary Figure S13). The study showed a significant contribution from the demolition phase; accounting for 35 and 45% of PM in the two studies. While these data are highly specific to two projects in a US context, they provide a useful indication of emissions that can be used by health and safety risk planners to mitigate potentially harmful concentrations of atmospheric matter produced by their projects.
To assist future occupational safety planners further with the proactive management of risk, several authors have derived emissions factors for PMs emitted from various construction and demolition processes; data that are scarce in the literature (Azarmi and Kumar, 2016). Both Kumar and Morawska (2014) and Kumar et al. (2012) reported particle count from simulated concrete recycling and demolition processes, respectively. The data are not presented here, but the studies focus on “ultra-fine” particles which the authors assert may pose significant health and safety hazards, and are a likely subject of further research. Azarmi and Kumar (2016) provided more accessible emissions factors for PM10, PM2.5, and PM1.0 based on their observations of the UK demolition site (reported in Table 3), providing indicative values on the basis of μg PM per floor space demolished per second (Supplementary Table S12).
Two studies used particle emission characterization to calculate occupational and public health risk. Normohammadi et al. (2016) used the concentrations of silica identified in dusts sampled in Tehran to calculate lifetime excess cancer cases from occupational exposure over 45 years (Supplementary Table S13). The study found that the concentrations identified in Table 3 would result in an average of 50 excess cancer deaths per 1,000 workers exposed. The study also calculated that the cumulative effect of silica exposure to workers in the study over 45 years would result in a further 22.64 deaths per 1,000 people due to silicosis (data not shown) based on a cohort study of silicosis mortality by t Mannetje et al. (2002).
High alkalinity of PM is also likely to be a source of risk to the health of people exposed to airborne dust from CDW according to Landrigan Philip et al. (2004) who associated high pH in dusts from the World Trade Center collapse with increased risk of asthma, persistent cough and bronchial hyper-reactivity. Both Chen and Thurston (2002) and McGee John et al. (2003) observed high alkalinity in World Trade Center collapse dusts as a consequence of concrete and gypsum content, reporting pH>10 and pH 8.9–10.0 (aqueous extracts of PM2.5), respectively. noted that alkalinity decreased with particle size, to the degree that PM2.5 were generally neutral. Research into the impact of PM from CDW primarily focusses on the finer, respirable fractions, larger inhalable fractions tend to receive less attention (Lippmann et al., 2015). In a critical review of the health effects of dust from the disaster, Lippmann et al. (2015) argued that the massive load of highly alkaline dusts caused extensive damage to epithelial cell function in exposed individuals, resulting in both pulmonary and gastroesophageal damage.
Substances From Previous Use of Industrial Premises
The previous two sections summarize evidence for emissions produced during the physical handling and processing of construction and demolition waste, relating to the actual materials used to construct buildings. However, there are also substances that arise from the previous use of the buildings. This section briefly summarizes two examples where waste from two demolished manufacturing facilities in South-western Sweden (Van Praagh and Modin, 2016) and Northern China (Huang et al., 2016) has been contaminated with pesticides at a time when possibly the occupiers were ignorant of the risks and for which such legacy contamination remains a challenge for those undertaking clean-up work (Table 4).
Van Praagh and Modin (2016) found that although the concentrations of phenoxy acids, chlorophenols and chlorocresols were higher than Swedish soil guidelines for residential and industrial properties, they were far below the concentrations necessary to be classed as hazardous waste. Leaching from the concrete occurred at a rate greater than inorganic substances and therefore the recycling of this concrete should be discouraged according to the study.
Huang et al. (2016) analyzed construction and demolition waste from a disused pesticide production facility in northern China that had been closed for a decade (Table 4). The study identified 11 organophosphorus compounds and Cypermethrin, a type of pyrethroid insecticide. Several of the organophosphorus pesticides were found in extremely high concentrations amongst the CDW. For instance, the arithmetic mean concentration of Phorate was 16,868 mg.kg−1 CDW and for Parathion it was 6,521 mg.kg−1 CDW. The level of potential human exposure to both of these agents is dependent on the human activity in those areas but given their potential toxicity the risk of lethal human exposure is potentially high. Phorate bio concentrates in the human body (Chawla et al., 2018) and the median lethal dose (LD50) for oral ingestion is 2–4 mg.kg−1 mammalian body weight (Dar et al., 2022). Parathion has a median lethal dose (LD50) for oral ingestion of ~2–4 mg.kg−1 mammalian body weight (PubChem, 1998) and has been used in chemical warfare (Soltaninejad and Shadnia, 2014).
Challenge 2: Land Disposal of Construction and Demolition Waste
Context
The majority of CDW by mass is composed of materials that are engineered to be biologically inert (for the timescales considered) such as ceramics, plastics, and metals (Section S.5.2). Although most of these materials are comparatively harmless to biota, some plastics contain substances that can migrate to the surface and into surrounding media such as water or soil (Molla et al., 2021). For instance, polybrominated diphenyl ethers (PBDEs) that have been added to plastics to retard combustion are known to migrate from their host polymer to the surface from where they can be washed away by rainwater and into the surrounding land, ground, or surface water (Duan et al., 2016). Biological matter (primarily timber) also exists in CDW and has the potential to decompose, releasing gasses, chemical and biological residues that are created while being consumed by micro-biota (Vodyanitskii, 2016). Though CDW is classified as “inert” in many geographical jurisdictions (Butti et al., 2018), or as having a low potential impact on the environment (Cerminara and Cossu, 2018), in reality, as we show here, it includes heterogeneously distributed components (materials and substances) that may result in harmful emissions under certain conditions.
Leachate From Construction and Demolition Waste
Leachate from landfill sites has been regulated in many HICs, for at least the last 50 years, for instance the Deposit of Poisonous Waste Act (1972) in the UK prohibited waste being deposited on land where it is ‘liable to give rise to an environmental hazard'. More recent legislation includes specific thresholds for monthly surface discharge of leachate according to its physico-chemical properties (European Union, 1999; United States Environmental Protection Agency, 2000), and contemporary sanitary landfills incorporate sophisticated leachate capture and treatment (Christensen et al., 2011). In LIMICs, especially scenarios where open dumps are the main disposal method, leachate is often not controlled at all and may be at risk of interacting with sensitive receptors in the vicinity or further afield through water transport (Wilson et al., 2015).
Studies of leachate are either field based, or simulated in the laboratory, the latter of which is often carried out to determine whether waste is suitable for disposal prior to actually doing so. López and Lobo (2014) sampled leachate at a CDW Spanish landfill site over a 5 year period that accepted mainly wood (31.5%), aggregates (28%), fine inert material (14.5%), plastics (6.7%), and inert building material (5%) along with many other materials produced as a consequence of construction and demolition activities. Concentrations of most substances in the leachate were mostly within limits for inert waste acceptance set by Directive 1999/31/EC (European Union, 2002) (Landfill Directive) (Table 5). While the Directive limits are intended to establish waste acceptance and are not designed for comparison with field analysis, they provide a useful comparison alongside other primary research for reference. Some elements showed higher concentrations than those set in the Directive. For instance, Pb was historically used in paints, coatings, and is still used in flashing and caulks. Mean concentrations of Pb were much higher than the levels in studies by Townsend et al., Weber et al., and Melendez, 6.5 times higher than the Directive limit, but lower than the United States Environmental Protection Agency (USEPA) study.
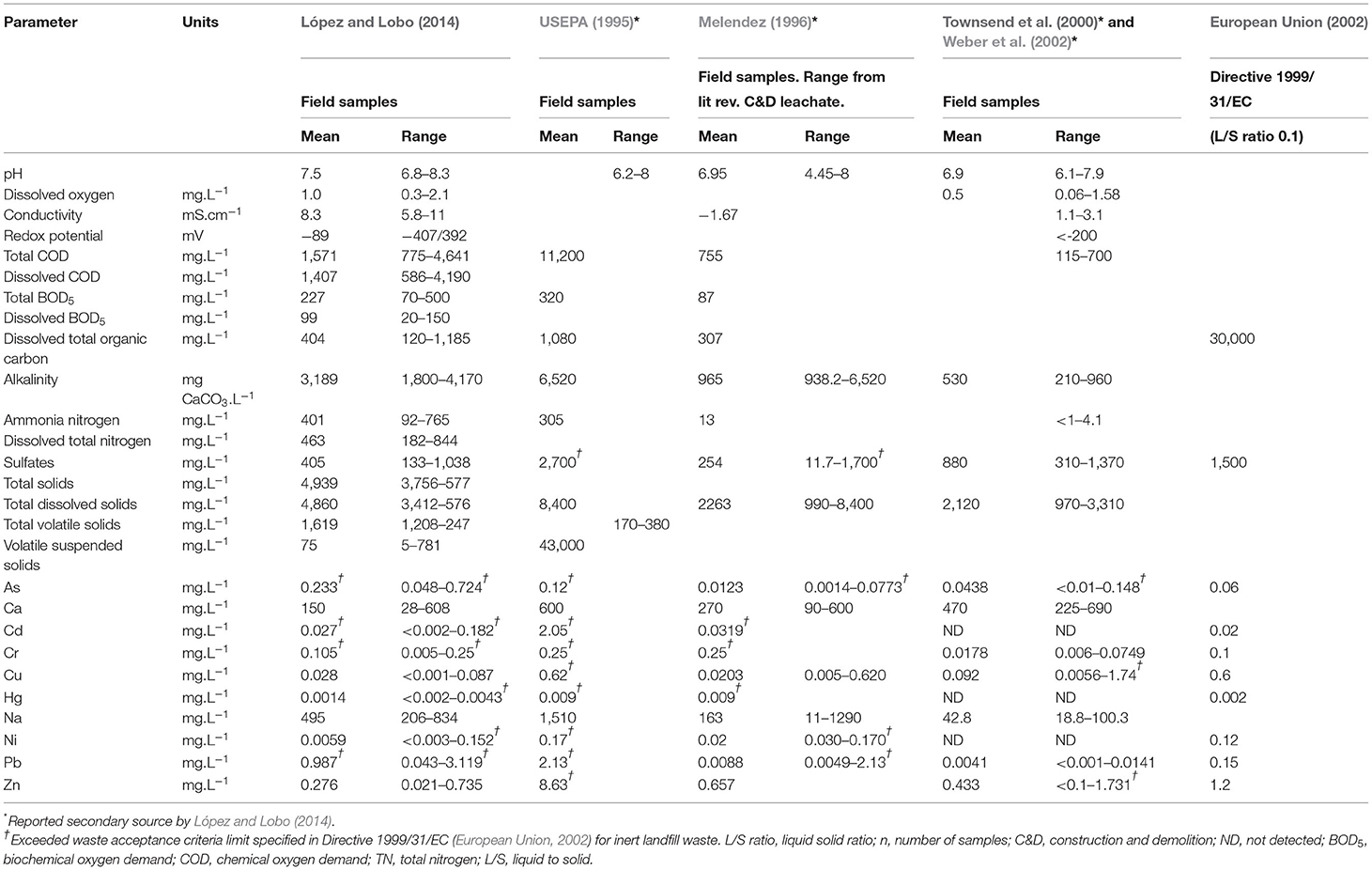
Table 5. Leachate quality from field samples collected from CDW landfill sites; field sample test from primary research by López and Lobo (2014) alongside compared values from other studies reported by the same author.
Concentrations of As and Cd were also high in comparison to the other sites (Table 5) and to the levels set by Directive 1999/31/EC (European Union, 2002), the former of which has been used historically in wood treatments. Alkalinity was within the 7.4–8.3 range as befits this type of material where dissolution of carbonates present in the CDW takes place. Ammoniacal-nitrogen levels were generally higher than the other studies compared, likely because of the very high levels of wood waste being accepted at the site.
In laboratory based samples, virtually all of the concentrations were well within limits set by Directive 1999/31/EC (European Union, 2002) (Supplementary Table S14). An exception is the levels of Pb determined by Devia and Suryo (2017) which were more than four times greater in some samples. The sampling took place in Indonesia and the author attributes the high levels to paint on chip plaster. Research by Saca et al. (2017) of waste obtained from a demolished steel plant investigated biologically inert CDW such as concrete, bricks and ceramics, which are materials that are unlikely to contain large quantities of hazardous materials. In all cases, the concentrations determined by Saca et al. (2017) were low. Unsurprisingly, the concrete batches showed higher pH due to carbonate dissolution, whereas the brick waste was neutral. Sulfate concentrations were variable and not congruent with the material type. The likely source of sulfate ions is gypsum plasterboard and therefore it is likely that the concentrations relate to material adhered to the surface or otherwise included in the samples from a demolished factory.
Whereas, the characteristics presented in Supplementary Table S14 refer to data obtained from percolation tests, two other tests are common for determining leachate over 6 or 24 h using a liquid to solid ratios (L/S) of 2 and 10 L.kg−1, respectively. Saca et al. (2017) performed these tests on demolition waste from a steel plant in addition to those whose results are presented in Supplementary Table S14.
The concentrations of substances assessed by both Puthussery et al. (2017) and Saca et al. (2017) were also well below limits set by Directive 1999/31/EC (European Union, 2002) (Supplementary Table S15). One exception is chlorides, which were slightly higher in one test, indicating that the material may not be suitable for recycling as the chloride ions can threaten the stability of structures by leading to corrosion of steel reinforcing materials. The phenol index was also higher in four of the samples and Saca et al. (2017) postulated that these concentrations were related to the previous use of the building where phenolic compounds are used in steel production.
In summary, the concentrations of elements and other parameters identified in both the field sampling (Table 5) and the lab sampling (Supplementary Tables S14, S15) were low in most cases compared to the limits set by Directive 1999/31/EC (European Union, 2002) and also other sites. However, only studies of surrounding soil, surface and underground waste can determine the potential exposure to receptors in proximity to land disposal. Furthermore, the limits set by Directive 1999/31/EC relate to well-managed European landfills that have undergone careful site selection and risks assessment to determine the risk of leachate contamination to the surrounding area and groundwater sources. In the context of LIMICs where such rigor may not have been applied, CDW may pose a more significant risk to the environment and health of the local populous. Further studies should focus on understanding the impact of CDW on environmental compartments in LIMICs to determine the credibility of these risks.
Wood
Wood used in construction is often treated with biocidal agents such as fungicides, preservatives, creosote, paint, varnish, oils, glues, resins, and stains (Environment Agency, 2017). A large number of biocides have been used historically, several of which contain potentially hazardous and carcinogenic ingredients, such as pentachlorophenol, an organic chlorinated compound (Freeman et al., 2006) creosote, a tarry black substance containing a complex mix of polycyclic aromatic hydrocarbons (Freeman et al., 2006); and chromated copper arsenate (CCA), a highly effective, waterborne biocide (Morrell, 2006).
Preservatives in timber are released through several mechanisms that involve: biodegradation of the wood itself; transformation of the wood, and/or preservatives by biological and thermal activity; and desorption by thermodynamic equilibrium (Schiopu and Tiruta-Barna, 2012). Once they have reached the surface of the wood, they may be volatilized, leached into surrounding liquids or attenuated into soil. Many studies into the environmental and health impacts of treated wood focus on the release and exposure of preservatives during the use phase, whereas there is comparatively scant information on the after-use phase (Schiopu and Tiruta-Barna, 2012).
Koyano et al. (2019) analyzed samples of demolition and recycled timber for the presence of four wood preservatives that are now known to be persistent organic pollutants, comparing concentrations to limits suggested by the Basel Convention Secretariat (Table 6). Although the Basel Convention concerns the transboundary movements of waste, the Secretariat publishes guidelines that defines whether waste has been managed responsibly, so called “environmentally sound management” (Secretariat of the Basel Convention, (nd)). To assist with determining whether waste contains concentrations of persistent organic pollutants (POPs), it publishes a threshold below which the content is considered “low” and hence different treatment practices may be applied. These low POP content limits provide a useful benchmark for determining the potentially hazardous concentrations of waste. Koyano et al. (2019) found low levels of POP wood treatments in all samples of wood compared to the Basel Convention limits of: chlordanes 50 mg.kg−1 (Secretariat of the Basel Convention, 2017c); pentachlorophenol 100 mg.kg−1 (Secretariat of the Basel Convention, 2017a); and polychloronaphthalenes 10 mg.kg−1 (Secretariat of the Basel Convention, 2017b). Of course, the samples analyzed by Koyano are highly specific to one area in Japan and further research would be required to determine whether these concentrations are representative of other contexts.
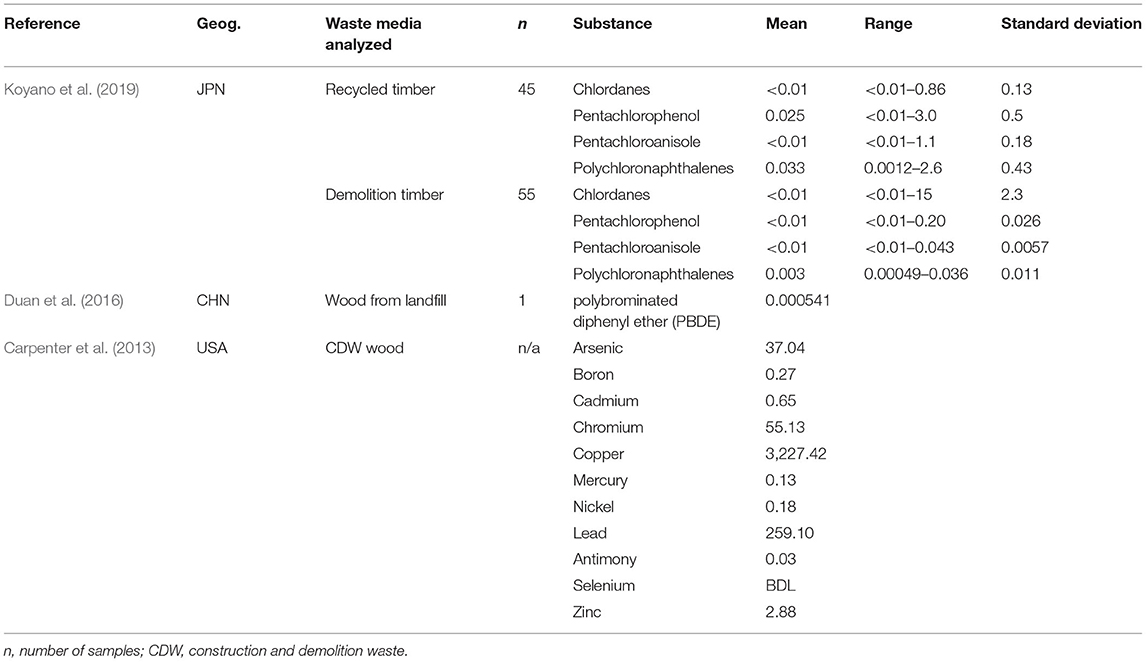
Table 6. Concentrations of elements, polybrominated diphenyl ethers, and selected wood preservatives (mg.kg−1 dry wt.).
Two other authors determined concentrations of potentially hazardous substances (Table 6). Duan et al. (2016) observed concentrations of brominated flame retardants were six orders of magnitude lower than the Basel Convention's recommended Low POP Content of 1,000 mg.kg−1 (for sum of hexa-BDE, hepta-BDE, penta-BDE and tetra-BDE). Carpenter et al. (2013) reported concentrations of various elements in CDW wood from a variety of literature sources, however no commentary is provided as these levels were reported as emission factors.
Leaching tests of engineered timber mulch carried out by Gaskin et al. (2005) showed little difference compared to non-treated varieties and levels of all substances were low enough to conclude that engineered timber studies is entirely suitable to be used as mulch. Jambeck et al. (2008) studied the leachability of As, Cr and Cu from CDW containing 10% (wt.) timber treated with chromated copper arsenate preservative (Supplementary Table S17). The study found that though the concentrations of Cu were not different to the control, that Cr and As levels were significantly (α=0.05, p <0.001) higher, indicating the need for vigilance in CDW landfills where leachate is not captured for treatment and where attenuation may risk contaminating sensitive receptors.
Gypsum
Calcium sulfate dihydrate, otherwise known as “gypsum,” is a soft mineral used in fertilizer, Portland cement, plaster and drywall plasterboard (Colman et al., 2020). Global mine production has grown steadily from ~10 million tons in 1940 to 160 million tons in 2010 and this sustained growth rate is expected to continue in the near future alongside global population growth (Asakura, 2013; U. S. Geological Survey, 2020). In demolished buildings where it has been used to coat internal walls, gypsum is often liberally distributed throughout the waste, existing as fragments and dust between 17 and 27% (wt.) of CDW (Townsend et al., 2000). Once it has been mixed, it is challenging to separate and if the concrete is to be recycled to The British Standards Institution (BS 8500-2:2015+A2:2019) for instance, the gypsum content must be reduced to <1% (wt.) with an acid soluble sulfate content <0.8%. Often this is done via a comminution and size screening process or manual separation (Asakura, 2013) and some novel methods have also been suggested (Montero et al., 2010), but source separation is often the most economical method (Rodríguez-Quijano et al., 2015; Jiménez-Rivero and García-Navarro, 2017).
In situ, plasterboard (drywall) and rendered plaster are generally stable, and exist in buildings for many hundreds of years. However, in landfills or dumpsites, sulfate ions are leached from the gypsum when they become solubilized, and in combination with carbon (organic matter), water and a lack of oxygen (anaerobic environment) the conditions are created to allow sulfate reducing bacteria to flourish and produce hydrogen sulfide (H2S) (Townsend et al., 2000).
H2S is colorless, smells of rotten eggs, and can be hazardous to human health if inhaled at sufficient quantity. H2S can cause: eye and lung irritation (20 to 200 ppm); pulmonary edema (250 to 500 ppm); serious damage to eyes, unconsciousness, amnesia and death after 4–8 h (500 ppm) (Guidotti, 1996). The concentrations necessary to cause a fatality have been reported at 1,000 ppm (Guidotti, 1996; Asakura, 2013) and 2,000 ppm (Townsend et al., 2000) and there are incidences where landfill operators have been killed after being overcome with H2S fumes (Kitazaki et al., 2014). The Health Safety Executive (2020a) in the UK, sets an 8-h time-weighted average workplace exposure limit of 5 ppm and a 15 min exposure limit of 10 ppm.
In a non-academic report, Townsend et al. (2000) investigated H2S production from drywall gypsum plasterboard in landfills in the US. This spurred two academic studies by Lee et al. (2006) and Yang et al. (2006), who determined concentrations of H2S generated from CDW leachate samples in field and simulated studies CDW samples in the laboratory, respectively (Table 7). The subsurface probes and landfill gas samples in the field studies observed average concentrations that breached the UK HSE long-term workplace exposure limits at nine of the 10 sites investigated, and the short-term exposure limit at seven. The average ambient concentrations were generally low in the study by Lee et al., indicating generally low risk to workers at the site with the exception of two sites where concentrations exceeded the limit of detection (>50 ppm) for the ambient sampling equipment. Both the sites that showed a very high limit disposed of fines from CDW recycling plants, which are known to contain higher than average concentrations of gypsum drywall fragments that are generally more friable and easily fall through the grate openings of ballistic separation equipment.
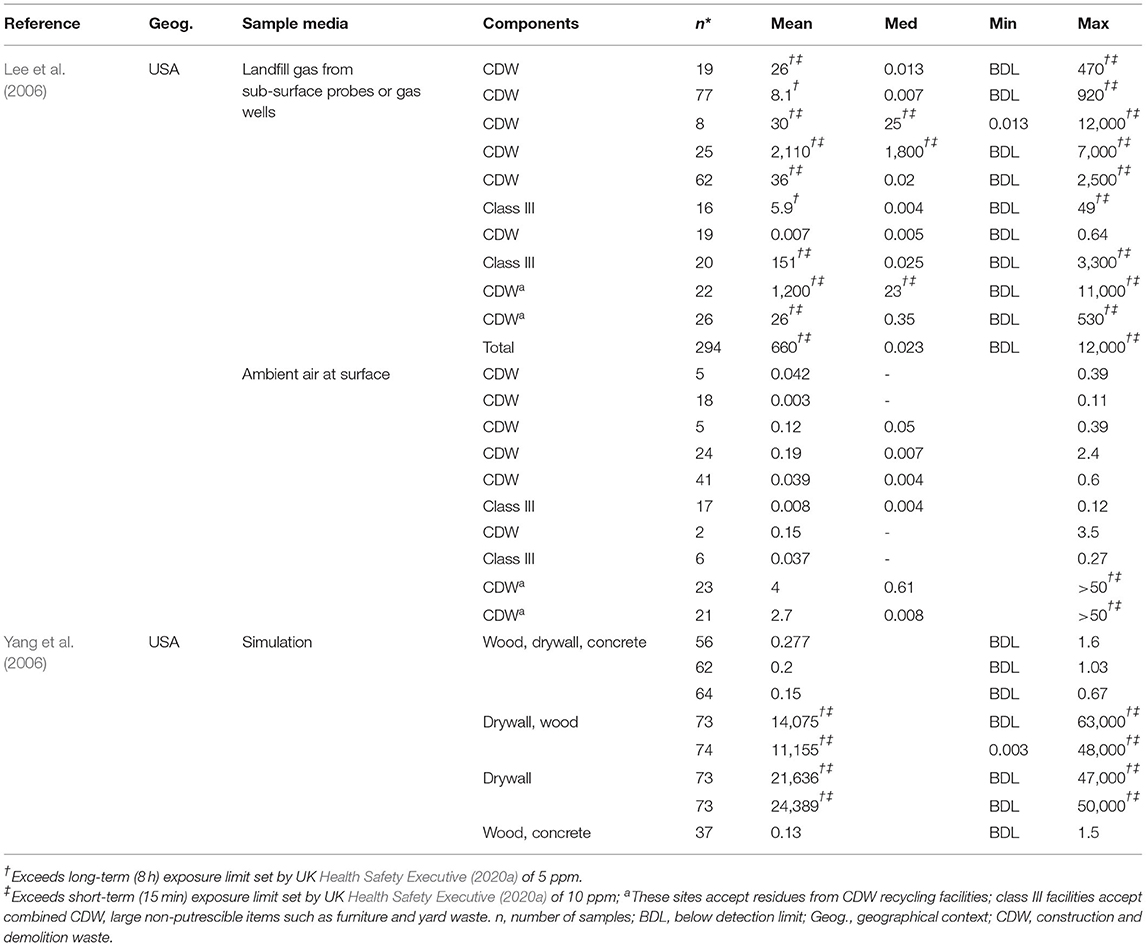
Table 7. Concentrations of hydrogen sulfide (H2S) produced by construction and demolition waste (CDW) in field sampled and simulated experiments.
The gas samples generated from simulated CDW studied by Yang et al. (2006) showed very high concentrations of H2S in four of the eight samples investigated. The four samples that included concrete showed much lower overall decomposition, and subsequent studies (Xu et al., 2011) have indicated that the concrete has an inhibiting effect on H2S production due to its alkalinity. Sulfate reducing bacteria require a source of carbon, and despite the wood content in the concrete sample, H2S production remained low. The samples that did not contain concrete produced high concentrations of H2S including the purely drywall sample, which obtained enough carbon from the paper lining (typically 10% of the drywall mass) (Yang et al., 2006).
Modern, well-managed landfill operators deposit gypsum in separate cells and capture and manage the landfill gas generated. Although no evidence was forthcoming, it is conceivable that CDW disposal practices in LIMICs are less rigorous, and that an increasing quantity of gypsum may be co-disposed with MSW in the future. As some landfill site and dumpsites in LIMICs are not restricted effectively from public access, H2S generation could pose an increasing threat to human health and even cause further fatalities if management practices are not improved.
Hexabromocyclododecane (HBCD)
As one of the most widely used brominated flame retardants, hexabromocyclododecane (HBCD), is mainly used in expanded polystyrene insulation, an increasingly prevalent component of CDW (Nie et al., 2015). In 2011 production was at 31,000 tons worldwide; however, it has decreased in recent years as its persistence in the natural environment and potentially harmful health effects on humans and animals have become established and alternatives developed to perform the same function. HBCD is listed in Annex A of the Stockholm Convention (Secretariat of the Stockholm Convention, (nd)), which means that parties to the convention must take steps to eliminate it from production and consumption; as well as Annex C, which obliges parties to control unintended release of the substance into the environment. As HBCD has been used in insulating material, it is likely to be in use for many decades and will therefore continue to arise in CDW.
Similarly to HBCD, PBDEs include congeners that are persistent organic pollutants and cause harm to fauna. Duan et al. (2016) sampled CDW collected from a recycling facility in China to determine HBCD and PBDE concentrations, finding the highest in samples of polyurethane foam and sponge for both compounds compared to other samples by orders of magnitude (Table 8). Drage et al. (2018) sampled expanded polystyrene and extruded polystyrene insulation found in construction waste in Ireland, finding high concentrations of HBCD in the extruded polystyrene sample and extremely high concentrations in the expanded polystyrene sample.
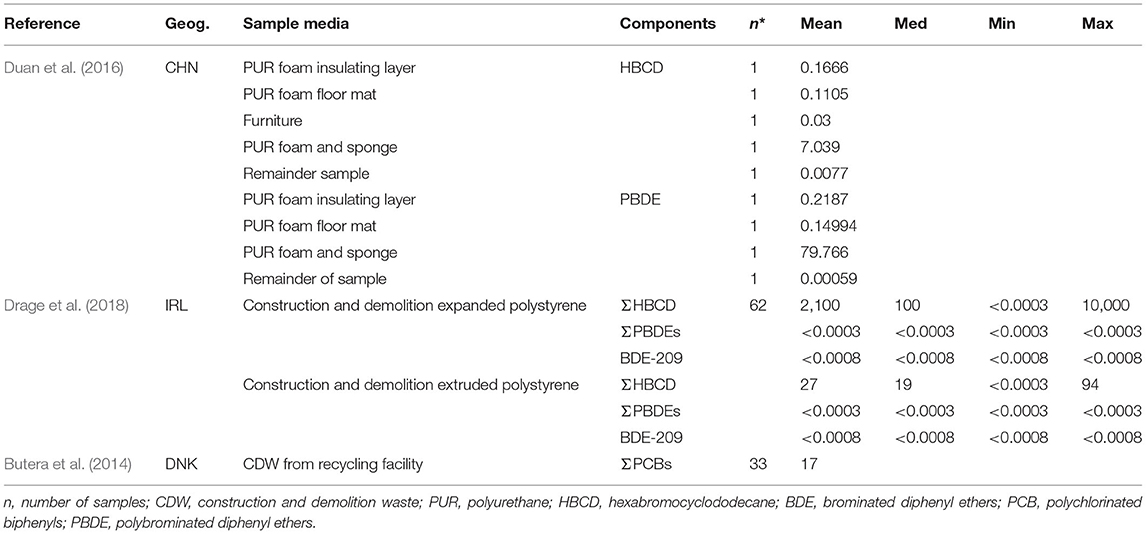
Table 8. Concentrations of selected brominated flame retardants, and polychlorinated biphenyls (PCBs) in construction and demolition waste (CDW) (mg.kg−1 total solids).
While concentrations of brominated flame retardants and PCBs in leachate and groundwater were not identified in proximity to CDW activities in this study, the concentrations identified in Table 8 provide an indication that these substances exist in considerable quantity. As Nie et al. (2015) highlighted, the prevalence of these substances and their persistence in the value chain means that considerable attention will need to be paid toward managing these products safely in the future, particularly when it comes to land disposal. Furthermore, assuming the recycling of CDW becomes more common in the coming decades, there will be a greater need to identify products containing polychlorinated dibenzo-p-dioxins, HBCDs, PBDEs and PCBs and divert them to other forms of treatment for complete destruction.
Challenge 3: Thermal Deconstruction, Open Burning and Thermal Processing of Construction and Demolition Waste
Context
Several thermal processes take place on construction and demolition sites. Materials may be combusted in the open (open burning) as a means of waste disposal, resulting in uncontrolled emissions of substances within materials and also those that are formed and transformed when substances and materials interact during combustion and various temperatures. Other thermal processes involve more incidental emission of substances. For instance steelwork on a surface coated in lead paint, or paint de-coating with a heat gun. These processes, the emissions that result and the pathways through which these emissions may reach receptors are illustrated in the conceptual diagram in Supplementary Figure S14.
Lead Release During Deconstruction Activities
The dangers of lead (Pb) exposure have been known for thousands of years (Scholz et al., 2002). In CDW, Pb occurs in soldered plumbing, but mainly in paints and coatings where Pb has been added to accelerate drying, increase durability, maintain a fresh appearance and resist moisture. Though Pb is still used in road markings, its potential hazardousness has seen the substance phased out of use in recent decades, however it still exists almost ubiquitously throughout the built environment. For instance, Turner and Solman (2016) analyzed paint sampled (n = 272) from multiple public buildings, road markings, street furniture, children's playgrounds, and residential buildings in Plymouth, UK, finding it was present in 221 (81%) of the samples with a mean concentration of 29,300 μg.g−1 and a median of 4,180 μg.g−1. In 1998, Jacobs (1998) reported that in the US, more than 90,000 bridges were painted in lead-based coatings and ~83% of residential homes constructed before 1980.
These findings indicate the prevalence of Pb almost everywhere people live, however there is some evidence that it is still being used in contemporary construction. For instance Gottesfeld et al. (2013) analyzed 61 samples of paint in Cameroon and found that 66% contained concentrations of Pb that exceeded United States Environmental Protection Agency (USEPA) 90 ppm total Pb with a median content of 2,150 across the samples (range: <21–500,000 ppm). While there is considerable awareness of the dangers of Pb exposure in HICs, and in many LIMICs, construction and demolition workers in LIMICs may have less awareness and have limited access to safe systems of work and specifically protective equipment to protect them from the potential hazards posed by Pb when it is heated and volatilized during deconstruction activities.
Jacobs (1998) reported a range of Pb concentrations in workplaces in the US from secondary sources, showing a large range of concentrations reported (Table 9), many of which exceeded the Health Safety Executive (2009) occupational exposure ceiling limit of 150 μg.m−3 (“lead other than lead alkyls”). Scholz et al. (2002) also found similarly high limits in a study of paint workers who removed Pb paint during refurbishment activities. In another study by Lange and Thomulka (2000) much lower concentrations were identified in a study of workers who implemented US Occupational Safety and Health Administration procedures in their work, indicating that they were effective at reducing their exposure.
Blood concentrations of workers involved in deconstruction activities were determined by three authors (Table 10). Fischbein et al. (1978) found concentrations of Pb in the blood of steel deconstruction workers to be higher in some cases than the HSE maximum limit set at 600 μg.L−1 blood. Centers for Disease Control Prevention (1989) found very high concentrations in the blood of workers deconstructing a steel bridge, noting that the paintwork on the bridge contained 30% Pb (wt.). Four of the workers in that study had to undergo chelation therapy to recover from the experience. Concentrations observed by Jacobs (1998) were substantially lower than those identified by Fischbein et al. (1978) and Centers for Disease Control Prevention (1989), well within the HSE's maximum safe limit; possibly indicating that safe systems of work for Pb related activities had improved by the turn of the century.
Most of the studies reviewed in this section relate to HIC examples from several decades ago. Workplace safety has improved considerably in HICs since these studies took place and awareness of the dangers of Pb at work has increased to the level where many workers have safety systems of work in place to protect them from harmful exposure. However, in LIMICs, as with many hazards, such safety measures may not have been implemented with the same stringency, therefore resulting in ongoing and considerable risk to those engaged in thermal deconstruction of steel structures and in the removal of paint.
Open Burning of Construction and Demolition Waste
The combustible fraction of CDW is a potential source of fuel for heat or cooking in LIMICs. If fuel is not required, then alongside dumping and storage, combustion is a common disposal option (Nie et al., 2015) as it can rapidly reduce the volume and mass of waste, discharging the problem to the atmosphere. The prevalence of open burning activity is not well-reported, but surveys of Nigerian construction workers indicate 2.9% (n = 243) (Ogunmakinde et al., 2019), and 16% (n = 75) (Wahab and Lawal, 2011) of construction practitioners engaged in open burning activities as a method of disposal. Furthermore, construction wood that is sold for reuse as suggested by Dania et al. (2007) is often burned as fuel, though the prevalence was not stated.
Combustible components of CDW include: wood, plastics, foam insulation, plastics, yard waste. Lemieux et al. (2004) inferred that open burning of CDW is likely to be a prevalent activity; but, suggested that there is little evidence to support its prevalence or impact. Instead, they referred to a study by Carroll (2001) that characterizes polychlorinated dibenzo-p-dioxins and polychlorinated dibenzofuran (hereafter dioxins and related compounds—DRCs) emissions from house fires as the composition of the material has some congruence with CDW. Carroll (2001) provided a comprehensive review of emission factors for various wood products, demolition and construction wastes and plastics used in construction such as polyvinyl chloride (PVC) piping (Supplementary Table S17). PVC is used increasingly on construction sites and a priori data suggests that it may occur increasingly in demolished buildings as its use becomes more prevalent. The chlorine content in PVC means that production of DRCs is considerably higher (for example, 3,500 μg I-TEQ.t−1 in soot phase) than other combustible components of CDW (for example, waste wood 26–173 μg I-TEQ.t−1 in vapor phase).
When wood that has been treated with preservatives is combusted, the potential exists for some chemical species to be produced in addition to those already created because of combustion of the wood itself. For instance, pentachlorophenol, an organochlorinated compound used in many pressure treated timber products since the late 1950s contained DRCs formed at the time of production (Supplementary Table S19).
All chlorinated hydrocarbons have the potential to produce DRCs when combusted, including untreated timber (Zhang et al., 2022). If combustion is controlled, for instance in modern incinerators, dioxin production is limited by maintaining optimum temperatures to reduce formation and increase the potential for destruction. Emissions cleaning technology is able to capture the majority of DRCs before the remaining (circa 1%) are released to the atmosphere where they are diluted into the environment. However, in open burning no such controls exist, and although temperatures in some parts of the fire may be sufficient (for example, >850°C) (Wielgosiński, 2011) to reduce formation, other parts will facilitate conditions ideal for DRC formation and release (Tame et al., 2007).
CCA is another important wood preservative that entered the global market in the 1940s and became the most globally prevalent preservative used in wood treatment during the 1970s (Wasson et al., 2005). CCA has a high content of three potentially toxic elements Cr, Cu and As which are emitted into the ash and air during combustion. Wasson et al. (2005) characterized emissions from combustion of wood treated with several CCA formulations, finding very large concentrations of As, Cr and Cu in the fly ash (Supplementary Table S20).
Emission factors for As, Cr and Cu were also calculated by Wasson et al. (2005) and are presented in Supplementary Table S21, however the emissions of DRCs reported in the same study were “unremarkable” with mean concentrations of 1.7 ng TEQ.kg−1; indicating that CCA treatment may not contribute substantially to DRC formation.
Data on the open burning of CDW are extremely limited. It is a recommendation of this report that considerable additional work is carried out to determine the prevalence of this activity and also to determine the relative emissions of different material composition to assist with the improved compilation of a global inventory.
Risk Characterization and Discussion
Handling and Physical Processing of Construction and Demolition Waste
Asbestos, a longstanding, potentially lethal, and prolific material, continues to cause the occupational deaths of ~90,000–250,000 people every year, mainly in HICs where its historical use was most prevalent. On this basis our risk assessment indicates that the occupational risk in HICs is medium-high, despite stringent safe system of work being in place in most contexts (Table 11). Nonetheless, substantial stocks of asbestos that exist throughout the global built environment mean that exposure to asbestos will continue to be a considerable cause of death and ill-health over the coming decades, as engineered structures are demolished when they reach their end of life. Despite this sustained loss of life, India continues to allow asbestos consumption and consequently, it is anticipated that around half of asbestos deaths may occur there in the coming decades. We scored the risk of asbestos exposure as very high in LIMIC contexts, partially because of lack of awareness and control but also due to the largely informal nature of the workforce. Though asbestos is a prolific killer, we consider the risk to local populations as low in all contexts. Despite the obvious danger from asbestos particles, even when not controlled, the exposure is unlikely to be sustained for prolonged periods.
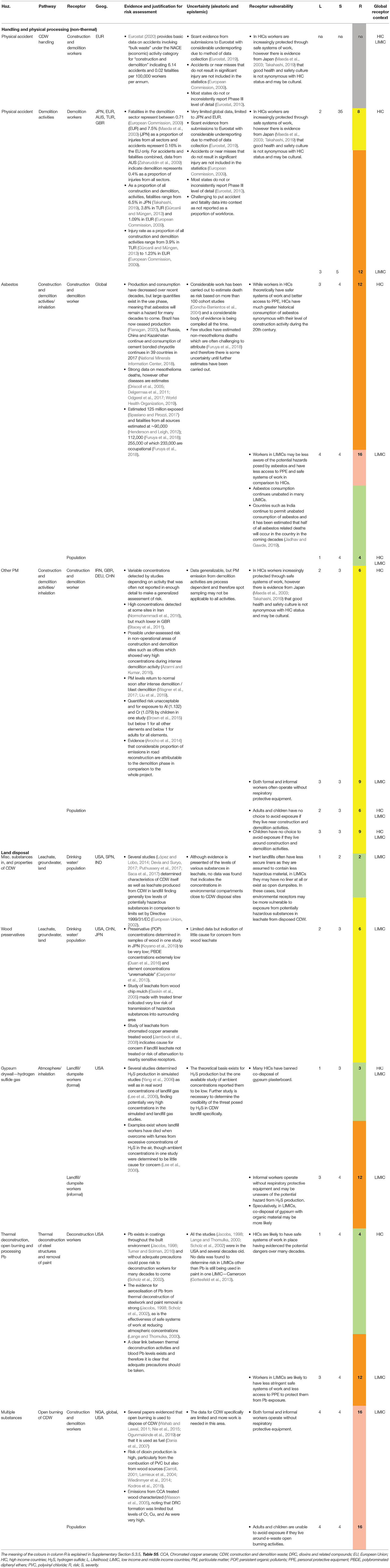
Table 11. Risk characterization summary for handling and physical processing (non-thermal) of construction and demolition waste (CDW).
Non-asbestos related PM is also an important hazard, though it scored medium low in all categories. One potentially overlooked risk is exposure to people working in portable offices who were exposed to extremely high levels of PM from demolition activities in one study. This is important, because workers in offices are less likely to wear protective equipment as it is often assumed that they work in a safe area.
The risk of physical accident in LIMICs was scored medium high as there is evidence for a much higher accident rate. The core assumption driving this score, is that safe systems of work are generally less stringent in LIMICs and businesses engaged in construction and demolition have less access to monetary resources to reduce accidents.
The risks from substances resulting from the previous use of a building were not assessed. These were included to indicate the harm, but there is little evidence to suggest the prevalence of hazards or risks, though further investigation of this theme may be warranted.
Land Disposal of Construction and Demolition Waste
Proportionally (by weight), CDW is mainly composed of biologically inert and non-hazardous material, resulting in generally low to medium risks from CDW when disposed of on land (Table 11). Some exceptions are the inclusion of gypsum plasterboard that can produce hydrogen sulfide gas when co-disposed with small amounts of biological material; providing a source of carbon for sulfate reducing bacteria to consume and produce the gas. Some wood preservatives may also pose a risk and one author cautions vigilance in scenarios where CDW is disposed in unlined and unmonitored landfills where it is assumed that the contents are generally inert and pose little threat to the surrounding environment. This is particularly important for LIMICs where less stringent governance and monitoring may be implemented. HBCD was not assessed as it was considered to present a negligible risk based on the evidence.
Thermal Deconstruction, Open Burning and Processing of Construction and Demolition Waste
Whereas, only a small proportion (wt.) of CDW materials are combustible, several substances of concern may be released in open, uncontrolled fires that is thought to be used as a common method of disposal in countries where MSW mismanagement is reported to be high. However, until the activity prevalence can be determined, it is challenging to assess the magnitude of these emissions, and hence, potential harm to human health. Our very high scores for these hazard-pathway-receptor combinations (Table 11) are based on the assumption that the open burning of CDW is at least as prevalent as the rate of household burning activities, which are reported to be as high as 50% of all MSW (Velis and Cook, 2021). Combined with that assumption is the risk that waste plastics, particularly PVC, may be burned as a form of disposal, resulting in the emission of dioxins and related compounds. Given the mass of CDW generated worldwide, and the very high risk potential, it is recommended that more data are gathered to determine the prevalence of this threat to human health.
Exposure to lead was scored low in HICs mainly because the dangers are well-established and safe systems of work have been in place, often for many decades. In LIMICs the score was medium high as although the dangers are known, the governance, enforcement and access to resources required to reduce exposure may not be in place; acknowledging that no evidence was found to determine direct lead exposure from CDW in LIMICs in this study.
Discussion
Our assessment of risk, based on conceptualized, hazard-pathway-receptor combinations, highlights a disparity between the Global North where workers enjoy predominantly safe systems of work to protect them and their surrounding populations from exposure to hazards, and the Global South where such regimes are inconsistently implemented. We propose that two central characteristics of the construction and demolition sector contribute to these heightened risks in the Global South context: (1) A high rate of informality in the construction and demolition sector worldwide (200 million informal workers, 80% of the workforce) (Jewell et al., 2005) (Supplementary Section S.5.4); and (2) Fewer resources (financial and technical) are allocated to providing independent and effective environmental and safety regulation (LaDou et al., 2018). These two factors create an environment in which construction and demolition site operators have few reasons to implement safe systems of work (Boadu et al., 2020), let alone to investigate or keep safety monitoring records that can be used to identify unsafe practice (Ahmed et al., 2018). This dearth of evidence is exemplified by the lack of data on the number of injuries and fatalities specifically on demolition sites, given that these workplaces are intuitively high-risk (Supplementary Section S.3.3).
The high rate of informality in the construction and demolition sector presents several challenges: (1) Data collection by informal workers is unlikely to be commonplace, as these people are mostly independent operators, there are few reasons for them to keep records (separate to the issue regarding operator records) (Forastieri, 2014; Darbi et al., 2018); (2) Informal workers often operate independently and without safe systems of work including protective equipment, exposing them to greater risk (Boadu et al., 2020); (3) When informal workers sustain injury or fall ill, they may not return to work, biasing data collection on the basis of survivorship.
Conclusions and Outlook
As we demonstrate in this PRISMA-ScR systematic scoping review, some construction and demolition processes result in the transformation, physical movement and emission of materials and substances from CDW, thus creating pathways through which humans can be exposed to hazards. Alongside available accident data, we have arranged the diverse and often incongruous evidence base for these processes according to three groups, described here as “challenges” based on the types of activity involved, and more broadly, the emission type and pathways through which hazards may be realized (RQ1), these are: (1) handling and physical processing; (2) land disposal; and (3) thermal deconstruction, open burning and thermal processing.
We used this evidence to develop scenarios—hazard-pathway-receptor linkage combinations—and through doing so were able to assign semi-quantitative risk scores to each so that they could be compared and ranked to enable focus on those which result in the greatest risk exposure. Inherently, virtually all construction activities involve working with or around CDW and therefore the majority of construction workers will undergo frequent exposure to many of the risks associated with it. Yet, with the exception of asbestos, specific data on the occupational risks from CDW are largely absent and the majority of our analysis is conjecture based on the limited or inferred peripheral data. The risks from asbestos are well-documented and supported by several complex and ambitious global burden of disease studies. Though we observed that the methodological approaches (not assessed systematically in this review) in many of the studies on non-asbestos related topics were not well-documented, limiting our ability to infer aleatoric and epistemic uncertainty. Many of the sources reviewed were case studies from which we extrapolated meaning, but some lacked sufficient context to be generalizable across wider socio-economic conditions. This lack of robustly reported research into solid waste and human health in countries where risks are likely to be higher, can be expected to encourage the continuation of elevated risk practices.
As a consequence of this information paucity, the global burden of disease from CDW related activities remains uncertain and we recommend future studies focus attention on improving understanding of this topic. In particular, high potential risks from the open burning of CDW will continue to be unquantifiable without data on the magnitude of the activity. Surveys combined with on-the-ground observations to determine prevalence combined with modeling would add substantial and much needed evidence to an almost empty research discipline.
The large number of workers engaged in the construction and demolition sector, nearly a quarter of a billion, means that any residual risk affects a substantial population. The high rate of informality reduces efforts to control those risks to populations that may already have compromised health and who are often least prepared or empowered to manage their own exposure. Historically, risk reduction for informal construction and demolition workers has been addressed, either through integration, or outright formalization in many HICs. For instance in the UK, The Construction (Design Management) Regulations (1994) compelled coordination between construction site contractors, which meant that even if workers were effectively informal, that they had to be controlled under a single safety regime.
Although the implementation of health and safety regulation is a critical component of reducing the risk of harm from CDW related activities, if not supported by a well-resourced and independent environmental regulator then it will have limited efficacy. Moreover, if the resources to implement safe systems of work are insufficient amongst construction and demolition sector participants, then the regulator will have few options than to close down construction activities altogether. We did not review or suggest specific policy responses in detail in this review, however a priori data suggest that some authorities may continue to prioritize the need to build infrastructure above worker safety. The lack of documentation of risk in the LIMIC context suggests then health and safety challenges around CDW may continue to constitute a major challenge in the foreseeable future unless full cost for all stakeholders are covered, enabling a sector–wide health and safety culture to be developed.
Data Availability Statement
All data underlying the results are available as part of the article and Supplementary Information and there are no additional no source data.
Author Contributions
EC: conceptualization, data curation, formal analysis, investigation, methodology, project administration, resources, validation, visualization, writing—original draft, and writing—review and editing. CV: conceptualization, data curation, formal analysis, funding acquisition, investigation, methodology, project administration, resources, software, supervision, validation, visualization, writing—original draft, and writing—review and editing. LB: writing—review and editing and validation. All authors contributed to the article and approved the submitted version.
Funding
This work was made possible by the Engineering X Safer End of Engineered Life programme which was funded by Lloyd's Register Foundation. Engineering X is an international collaboration, founded by the Royal Academy of Engineering and Lloyd's Register Foundation, that brings together some of the world's leading problem-solvers to address the great challenges of our age.
Conflict of Interest
The authors declare that the research was conducted in the absence of any commercial or financial relationships that could be construed as a potential conflict of interest.
Publisher's Note
All claims expressed in this article are solely those of the authors and do not necessarily represent those of their affiliated organizations, or those of the publisher, the editors and the reviewers. Any product that may be evaluated in this article, or claim that may be made by its manufacturer, is not guaranteed or endorsed by the publisher.
Acknowledgments
We are grateful to the Technical Advisory Board of the Engineering X Safer End of Engineered Life programme, of the Royal Academy of Engineering for their steering and insightful feedback, especially on early versions of this research and manuscript. We thank the Programme Board, chaired by Professor William Powrie FREng & the Academy staff, especially Hazel Ingham and Shaarad Sharma who provided support throughout the process. Ad hoc advice, guidance and criticism was provided by multiple stakeholder representatives, as listed in the relevant Engineering X report. We are grateful Nick Rigas (D-Waste), for the presentation of infographics and to Dr. Godwin Akpeimeh for preliminary data collection. The research communicated and opinions expressed here are authors' alone. A preprint of this paper is available at https://doi.org/10.31224/osf.io/5tpbz.
Supplementary Material
The Supplementary Material for this article can be found online at: https://www.frontiersin.org/articles/10.3389/frsus.2022.924926/full#supplementary-material
Abbreviations
BDE, brominated diphenyl ether; BDL, below detection limit; CCA, chromated copper arsenate; CDW, construction and demolition waste; conc., concentration; DRCs, dioxins and related compounds; H2S, hydrogen sulfide; HBCD, hexabromocyclododecane; HBCD, hexabromocyclododecane; HI, hazard index; HIC, high income countries; HSE, Health and Safety Executive; ind., industrial; L/S, liquid to solid ratio; LIC, low income countries; LIMIC, low income and middle income countries; LMC, lower middle income countries; MSW, municipal solid waste; Mt, million metric tons; n, number of samples; PBDEs, polybrominated diphenyl ethers; PCB, polychlorinated biphenyls; PM, particulate matter; PM0.1, particulate matter <0.1 μm; PM10, particulate matter <10 μm; PM2.5, particulate matter <2.5 μm; POP, persistent organic pollutants; PPE, personal protective equipment; ppm, parts per million; PUR, polyurethane; PVC, polyvinyl chloride; res., residential; RQ, research question; SGV, soil guideline values; t, metric tons (1,000 kg); UK, United Kingdom; UMC, upper middle income countries; US, United States; USD, United States dollars; USEPA, United States Environmental Protection Agency; VSS, volatile suspended solids; wt., weight (i.e., on a weight reporting basis).
References
Ahmed, I., Shaukat, M. Z., Usman, A., Nawaz, M. M., and Nazir, M. S. (2018). Occupational health and safety issues in the informal economic segment of Pakistan: a survey of construction sites. Int. J. Occup. Saf. Ergon. 24, 240–250. doi: 10.1080/10803548.2017.1366145
Arachi, D., Furuya, S., David, A., Mangwiro, A., Chimed-Ochir, O., Lee, K., et al. (2021). Development of the “National Asbestos Profile” to eliminate asbestos-related diseases in 195 countries. Int. J. Environ. Res. Public Health 18, 1804. doi: 10.3390/ijerph18041804
Arocho, I., Rasdorf, W., and Hummer, J. (2014). “Methodology to forecast the emissions from construction equipment for a transportation construction project,” in Construction Research Congress 2014 (Atlanta, GA: American Society of Civil Engineers).
Asakura, H. (2013). “Removing gypsum from construction and demolition waste (C&DW),” in Handbook of Recycled Concrete and Demolition Waste, eds F. Pacheco-Torgal, V. W. Y. Tam, J. A. Labrincha, Y. Ding, and J. de Brito. (Cambridge, UK: Woodhead Publishing), 479–499. doi: 10.1533/9780857096906.4.479
Azarmi, F., and Kumar, P. (2016). Ambient exposure to coarse and fine particle emissions from building demolition. Atmos. Environ. 137, 62–79. doi: 10.1016/j.atmosenv.2016.04.029
Boadu, E. F., Wang, C. C., and Sunindijo, R. Y. (2020). Characteristics of the construction industry in developing countries and its implications for health and safety: an exploratory study in Ghana. Int. J. Environ. Res. Public Health 17, 4110. doi: 10.3390/ijerph17114110
Brown, A., Barrett, J. E. S., Robinson, H., and Potgieter-Vermaak, S. (2015). Risk assessment of exposure to particulate output of a demolition site. Environ. Geochem. Health 37, 675–687. doi: 10.1007/s10653-015-9747-3
Burki, T. (2010). Health experts concerned over India's asbestos industry. Lancet 375, 626–627. doi: 10.1016/S0140-6736(10)60251-6
Burns, C. J., LaKind, J. S., Mattison, D. R., Alcala, C. S., Branch, F., Castillo, J., et al. (2019). A matrix for bridging the epidemiology and risk assessment gap. Glob. Epidemiol. 1, 100005. doi: 10.1016/j.gloepi.2019.100005
Butera, S., Christensen, T. H., and Astrup, T. F. (2014). Composition and leaching of construction and demolition waste: inorganic elements and organic compounds. J. Hazard. Mater. 276, 302–311. doi: 10.1016/j.jhazmat.2014.05.033
Butti, L., Peres, F., and Lops, C. (2018). “Chapter 1.3 - Legal Framework of Landfilling in Different Areas of the World,” in Solid Waste Landfilling, eds. R. Cossu and R. Stegmann (Amsterdam: Elsevier), 41–50. doi: 10.1016/B978-0-12-407721-8.00003-6
Carpenter, A., Jambeck, J. R., Gardner, K., and Weitz, K. (2013). Life cycle assessment of end-of-life management options for construction and demolition debris. J. Indus. Ecol. 17, 396–406. doi: 10.1111/j.1530-9290.2012.00568.x
Carroll, W. F. (2001). The relative contribution of wood and poly(vinyl chloride) to emissions of PCDD and PCDF from house fires. Chemosphere 45, 1173–1180. doi: 10.1016/S0045-6535(01)00003-0
Centers for Disease Control and Prevention. (1989). “Lead poisoning in bridge demolition workers - Massachusetts,” in Morbidity and Mortality Weekly Report (MMWR) (Washington, DC: Centers for Disease Control and Prevention (CDC)).
Cerminara, G., and Cossu, R. (2018). “1.2 - Waste Input to Landfills,” in Solid Waste Landfilling, eds. R. Cossu and R. Stegmann (Amsterdam: Elsevier), 15–39. doi: 10.1016/B978-0-12-407721-8.00002-4
Chawla, P., Kaushik, R., Shiva Swaraj, V. J., and Kumar, N. (2018). Organophosphorus pesticides residues in food and their colorimetric detection. Environ. Nanotechnol. Monit. Manag. 10, 292–307. doi: 10.1016/j.enmm.2018.07.013
Chen, K., Wang, J., Yu, B., Wu, H., and Zhang, J. (2021). Critical evaluation of construction and demolition waste and associated environmental impacts: a scientometric analysis. J. Clean. Prod. 287, 125071. doi: 10.1016/j.jclepro.2020.125071
Chen, L. C., and Thurston, G. (2002). World Trade Center cough. Lancet 360, s37–s38. doi: 10.1016/S0140-6736(02)11814-9
Chen, W., Liu, Y., Wang, H., Hnizdo, E., Sun, Y., Su, L., et al. (2012). Long-term exposure to silica dust and risk of total and cause-specific mortality in Chinese workers: a cohort study. PLoS Med. 9, e1001206–e1001206. doi: 10.1371/journal.pmed.1001206
Christensen, T. H., Manfredi, S., Kjeldsen, P., and Wallace, R. B. (2011). “Landfilling: bottom lining and leachate collection,” in Solid Waste Technology and Management, ed T. H. Christensen (Chichester: Wiley), 800–829. doi: 10.1002/9780470666883.ch51
Colman, C., Bulteel, D., Rémond, S., Zhao, Z., and Courard, L. (2020). Valorization of fine recycled aggregates contaminated with gypsum residues: characterization and evaluation of the risk for secondary ettringite formation. Materials 13, 4866. doi: 10.3390/ma13214866
Concha-Barrientos, M., Nelson, D. I., Driscoll, T., Steenland, N. K., Punnett, L., Fingerhut, M. A., et al. (2004). “Selected occupational risk factors,” in Comparative Quantification of Health Risks Global and Regional Burden of Disease Attributable to Selected Major Risk Factors, eds M. Ezzati, A. D. Lopez, A. Rodgers and C. J. L. Murray (Geneva: World Health Organization), 1651–1801.
Cook, E., and Velis, C. A. (2022). Construction and demolition waste management: A systematic review of risks to occupational and public health. engrXiv [Preprint]. doi: 10.31224/osf.io/5tpbz
Cook, E., Velis, C. A., and Derks, M. (2020). Plastic waste reprocessing for circular economy: A systematic review of risks to occupational and public health from legacy substances and extrusion. engrXiv [Preprint]. doi: 10.31224/osf.io/yxb5u
Cooper, C., Booth, A., Varley-Campbell, J., Britten, N., and Garside, R. (2018). Defining the process to literature searching in systematic reviews: a literature review of guidance and supporting studies. BMC Med. Res. Methodol. 18, 85. doi: 10.1186/s12874-018-0545-3
Dania, A. A., Kehinde, J. O., and Bala, K. (2007). “A study of construction material waste management practices by construction firms in Nigeria,” in The Third Scottish Conference for Postgraduate Researchers of the Built and Natural Environment (Glasgow: Glasgow Caledonian University).
Dar, M. A., Baba, Z. A., and Kaushik, G. (2022). A review on phorate persistence, toxicity and remediation by bacterial communities. Pedosphere 32, 171–183. doi: 10.1016/S1002-0160(21)60043-7
Darbi, W. P. K., Hall, C. M., and Knott, P. (2018). The informal sector: a review and agenda for management research. Int. J. Manag. Rev. 20, 301–324. doi: 10.1111/ijmr.12131
Delgermaa, V., Takahashi, K., Park, E.-K., Le, G. V., Hara, T., and Sorahan, T. (2011). Global mesothelioma deaths reported to the World Health Organization between 1994 and 2008. Bull. World Health Organiz. 89, 716C−724C. doi: 10.2471/BLT.11.086678
Devia, Y. P., and Suryo, E. A. (2017). “Leachate of demolition waste,” in 6th International Conference of Euro Asia Civil Engineering Forum, eds J. W. Park, H. A. Lie, H. Hardjasaputra, and P. Thayaalan (Seoul: E D P Sciences). doi: 10.1051/matecconf/201713808002
Drage, D. S., Sharkey, M., Abdallah, M. A. E., Berresheim, H., and Harrad, S. (2018). Brominated flame retardants in Irish waste polymers: concentrations, legislative compliance, and treatment options. Sci. Total Environ. 625, 1535–1543. doi: 10.1016/j.scitotenv.2018.01.076
Driscoll, T., Nelson, D. I., Steenland, K., Leigh, J., Concha-Barrientos, M., Fingerhut, M., et al. (2005). The global burden of disease due to occupational carcinogens. Am. J. Ind. Med. 48, 419–431. doi: 10.1002/ajim.20209
Duan, H., Yu, D., Zuo, J., Yang, B., Zhang, Y., and Niu, Y. (2016). Characterization of brominated flame retardants in construction and demolition waste components: HBCD and PBDEs. Sci. Total Environ. 572, 77–85. doi: 10.1016/j.scitotenv.2016.07.165
Ertaş, H., and Erdogan, A. S. (2017). An analysis of occupational accidents in demolition work. Civil Eng. Archit. 5, 37–51. doi: 10.13189/cea.2017.050201
European Union (1999). Council Directive 1999/31/EC of 26 April 1999 on the landfill of waste. 1999/31/EC.
European Union (2002). “Council Decision of 19 December 2002 establishing criteria and procedures for the acceptance of waste at landfills pursuant to Article 16 of and Annex II to Directive 1999/31/EC”, in: L11, p.27-49. Official Journal of the European Communities.
European Union (2008a). “Directive 2008/50/EC of the European Parliament and of the Council of 21 May 2008 on ambient air quality and cleaner air for Europe”, in: L152, P. 169-212. Official Journal of the European Union.
European Union (2008b). “Regulation (EC) No 1338/2008 of The European Parliament and of The Council of 16 December 2008 on Community statistics on public health and health and safety at work”, in: L 354/70,–No match found−70–81.
European Union (2009). “Directive (EU) 2017/2398 of the European Parliament and of the Council of 12 December 2017 amending Directive 2004/37/EC on the protection of workers from the risks related to exposure to carcinogens or mutagens at work (Text with EEA relevance)”, in: L345. p. 87–95.
Eurostat (2010). Accidents at work (ESAW) - until 2007 (hsw_acc7_work): Reference Metadata in Euro SDMX Metadata Structure (ESMS). Luxembourg: Eurostat, the statistical office of the European Union. Available online at: https://ec.europa.eu/eurostat/cache/metadata/en/hsw_acc7_work_esms.htm (accessed May 26, 2020).
Eurostat (2019). Accidents at work statistics. Eurostat. Available online at: https://ec.europa.eu/eurostat/statistics-explained/index.php/Accidents_at_work_statistics#Accidents_2010_to_2017_.E2.80.94_absolute_changes_in_incidence_rates (accessed May 27, 2020).
Eurostat (2020). Your Key to European Statistics. Luxembourg: European Commission. Available online at: https://ec.europa.eu/eurostat (accessed June 2, 2020).
Evans, L. (2014). Traffic fatality reductions: United States compared with 25 other countries. Am. J. Public Health. 104, 1501–1507. doi: 10.2105/AJPH.2014.301922
Ferronato, N., and Torretta, V. (2019). Waste mismanagement in developing countries: a review of global issues. Int. J. Environ. Res. Public Health 16, 1060. doi: 10.3390/ijerph16061060
Fischbein, A., Daum, S. M., Davidow, B., Slavin, G., Alvares, A. P., Sassa, S., et al. (1978). Lead hazard among ironworkers. Dismantling lead-painted elevated subway line in New York City. N. Y. State J. Med. 78, 1250–1259.
Font, A., Baker, T., Mudway, I. S., Purdie, E., Dunster, C., and Fuller, G. W. (2014). Degradation in urban air quality from construction activity and increased traffic arising from a road widening scheme. Sci. Total Environ. 497–498, 123–132. doi: 10.1016/j.scitotenv.2014.07.060
Frank, A. L. (2006). “The history of the extraction and use of asbestos,” in Asbestos?Risk Assessment, Epidemiology, and Health Effects, eds. R. F. Dodson and S. P. Hammar (Portland, OR: Taylor and Francis Group), 1–7. doi: 10.1201/9781420038149.ch1
Frank, A. L., and Joshi, T. (2014). The global spread of asbestos. Ann. Glob. Health 80, 257–262. doi: 10.1016/j.aogh.2014.09.016
Freeman, M. H., Nicholas, D. D., and Schultz, T. P. (2006). “Nonarsenical wood protection: alternatives for chromated copper arsenate, creosote and pentachlorophenol,” in Environmental Impacts of Treated Wood, 1st Edn., eds T. G. Townsend and H. Solo-Gabriele (Boca Raton, FL: Taylor and Francis). doi: 10.1201/9781420006216.ch2
Frost, G. (2013). The latency period of mesothelioma among a cohort of British asbestos workers (1978-2005). Br. J. Cancer 109, 1965–1973. doi: 10.1038/bjc.2013.514
Fuller, G. W., and Green, D. (2004). The impact of local fugitive PM10 from building works and road works on the assessment of the European Union Limit Value. Atmos. Environ. 38, 4993–5002. doi: 10.1016/j.atmosenv.2004.06.024
Furuya, S., Chimed-Ochir, O., Takahashi, K., David, A., and Takala, J. (2018). Global asbestos disaster. Int. J. Environ. Res. Public Health 15, 1000. doi: 10.3390/ijerph15051000
Gaskin, J. W., Risse, L. M., Kastner, J. R., and McLaurin, W. J. (2005). Evaluating onsite beneficial reuse of ground engineered wood wastes from residential construction. Trans. Am. Soc. Agric. Eng. 48, 1731–1738. doi: 10.13031/2013.20007
Ginga, C. P., Ongpeng, J. M. C., and Daly, M. K. M. (2020). Circular economy on construction and demolition waste: a literature review on material recovery and production. Materials 13, 1–18. doi: 10.3390/ma13132970
Gottesfeld, P., Kuepouo, G., Tetsopgang, S., and Durand, K. (2013). Lead concentrations and labeling of new paint in Cameroon. J. Occup. Environ. Hyg. 10, 243–249. doi: 10.1080/15459624.2013.768934
Gürcanli, G. E., and Müngen, U. (2013). Analysis of construction accidents in turkey and responsible parties. Ind. Health 51, 581–595. doi: 10.2486/indhealth.2012-0139
Hamby, D. M. (1995). A comparison of sensitivity analysis techniques. Health Phys. 68, 195–204. doi: 10.1097/00004032-199502000-00005
Health Safety Executive (2009). The control of lead at work regulations 1998. Health and Safety Executive. Available online at: https://www.hse.gov.uk/foi/internalops/ocs/200-299/298_15.htm#para-7 (accessed June 5, 2020).
Health and Safety Executive (2020a). EH40/2005 Workplace exposure limits: Containing the list of workplace exposure limits for use with the Control of Substances Hazardous to Health Regulations 2002 (as amended) (UK).
Health Safety Executive (2020b). Information and services. Health and Safety Executive. Available online at: https://www.hse.gov.uk/ (accessed October 13, 2020).
Henderson, D. W., and Leigh, J. (2011). “The history of asbestos utilization and recognition of asbestos-induced diseases,” in Asbestos: Risk Assessment, Epidemiology, and Health Effects, eds. R. F. Dodson and S. P. Hammar (Boca Raton, FL: Taylor & Francis Group), 1–22. doi: 10.1201/b10958-2
Huang, S., Zhao, X., Sun, Y., Ma, J., Gao, X., Xie, T., et al. (2016). Spatial distribution of organic pollutants in industrial construction and demolition waste and their mutual interaction on an abandoned pesticide manufacturing plant. Environ. Sci. Processes Impacts 18, 482–492. doi: 10.1039/C6EM00019C
Hunter, P. R., Payment, P., Ashbolt, N., and Bartram, J. (2003). “Assessment of risk,” in Assessing Microbial Safety of Drinking Water: Improving Approaches and Methods. (Paris: Organisation for Economic Cooperation and Development and World Health Organisation), 79–109.
IARC Working Group on the Evaluation of Carcinogenic Risks to Humans (2012). “Arsenic, metals, fibres, and dusts: IARC monographs on the evaluation of carcinogenic risks to humans volume 100C,” in IARC Monographs on the Evalauation of Carcionogenic Risks to Humans (Lyon: World Health Organization).
International Chrysotile Association (nd). For environmental occupational health safe responsible use: Products. International Chrysotile Association. Available online at: https://chrysotileassociation.com/chrysotile/products/default.php (accessed May 28 2020).
International Labour Organization (2020a). “Cases of fatal occupational injury by economic activity: Annual,” in Occupational Safety and Health Statistics (OSH). 04/10/2020 06:19:58 ed. Available online at: https://www.ilo.org/ilostat-files/Documents/Excel/INDICATOR/INJ_FATL_ECO_NB_A_EN.xlsx
International Labour Organization (2020b). ILOSTAT: The leading source of labour statistics. International Labour Organization. Available online at: https://ilostat.ilo.org/ (accessed October 13, 2020).
Jacobs, D. E. (1998). Occupational exposures to lead-based paint in structural steel demolition and residential renovation work. Int. J. Environ. Pollut. 9, 126–139.
Jadhav, A. V., and Gawde, N. C. (2019). Current asbestos exposure and future need for palliative care in India. Indian J. Palliat. Care 25, 587–591. doi: 10.4103/IJPC.IJPC_51_19
Jambeck, J. R., Townsend, T. G., and Solo-Gabriele, H. M. (2008). Landfill disposal of CCA-treated wood with construction and demolition (CandD) debris: arsenic, chromium, and copper concentrations in leachate. Environ. Sci. Technol. 42, 5740–5745. doi: 10.1021/es800364n
Jewell, C., Flanagan, R., and Cattell, K. (2005). “The Effects of the Informal Sector on Construction,” in Construction Research Congress 2005: Broadening Perspectives (San Diego, CA: American Society of Civil Engineers).
Jiménez-Rivero, A., and García-Navarro, J. (2017). Best practices for the management of end-of-life gypsum in a circular economy. J. Clean. Prod. 167, 1335–1344. doi: 10.1016/j.jclepro.2017.05.068
Kaya, G. K., Ward, J. R., and Clarkson, P. J. (2018). A framework to support risk assessment in hospitals. Int. J. Qual. Health Care 31, 393–401. doi: 10.1093/intqhc/mzy194
Kazan-Allen, L. (2019a). Chronology of Asbestos Bans and Restrictions. International Ban Asbestos Secretariat. Available online at: http://www.ibasecretariat.org/chron_ban_list.php (accessed May 28, 2020).
Kazan-Allen, L. (2019b). Current Asbestos Bans: National Asbestos Bans. International Ban Asbestos Secretariat. Available online at: http://www.ibasecretariat.org/alpha_ban_list.php (accessed May 29, 2020).
Kitazaki, S., Xiao, K., Nakagawa, K., Kindaichi, T., and Asakura, H. (2014). Hydrogen sulfide generation suppression by nitrate addition – application to solid waste landfill site. Am. J. Environ. Prot. 3, 267–274. doi: 10.11648/j.ajep.20140305.20
Kodros, J. K., Wiedinmyer, C., Ford, B., Cucinotta, R., Gan, R., Magzamen, S., et al. (2016). Global burden of mortalities due to chronic exposure to ambient PM2.5 from open combustion of domestic waste. Environ. Res. Lett. 11, 124022. doi: 10.1088/1748-9326/11/12/124022
Koyano, S., Ueno, D., Yamamoto, T., and Kajiwara, N. (2019). Concentrations of POPs based wood preservatives in waste timber from demolished buildings and its recycled products in Japan. Waste Manag. 85, 445–451. doi: 10.1016/j.wasman.2018.12.039
Kumar, P., and Morawska, L. (2014). Recycling concrete: an undiscovered source of ultrafine particles. Atmos. Environ. 90, 51–58. doi: 10.1016/j.atmosenv.2014.03.035
Kumar, P., Mulheron, M., and Som, C. (2012). Release of ultrafine particles from three simulated building processes. J. Nanoparticle Res. 14, 771. doi: 10.1007/s11051-012-0771-2
LaDou, J., London, L., and Watterson, A. (2018). Occupational health: a world of false promises. Environmental Health 17, 81. doi: 10.1186/s12940-018-0422-x
Landrigan Philip, J., Lioy Paul, J., Thurston, G., Berkowitz, G., Chen, L. C., Chillrud Steven, N., et al. (2004). Health and environmental consequences of the world trade center disaster. Environ. Health Perspect. 112, 731–739. doi: 10.1289/ehp.6702
Lange, J. H., and Thomulka, K. W. (2000). Effectiveness of engineering controls for airborne lead exposure during renovation/demolition of a commercial building. Indoor Built Environ. 9, 207–215. doi: 10.1177/1420326X0000900310
Lee, S., Xu, Q., Booth, M., Townsend, T. G., Chadik, P., and Bitton, G. (2006). Reduced sulfur compounds in gas from construction and demolition debris landfills. Waste Manag. 26, 526–533. doi: 10.1016/j.wasman.2005.10.010
Lemieux, P. M., Lutes, C. C., and Santoianni, D. A. (2004). Emissions of organic air toxics from open burning: a comprehensive review. Progress Energy Combust. Sci. 30, 1–32. doi: 10.1016/j.pecs.2003.08.001
Leung, C. C., Yu, I. T. S., and Chen, W. (2012). Silicosis. Lancet 379, 2008–2018. doi: 10.1016/S0140-6736(12)60235-9
Li, J., Dong, Q., Yu, K., and Liu, L. (2014). Asbestos and asbestos waste management in the Asian-Pacific region: trends, challenges and solutions. J. Clean. Prod. 81, 218–226. doi: 10.1016/j.jclepro.2014.06.022
Lippmann, M., Cohen, M. D., and Chen, L.-C. (2015). Health effects of World Trade Center (WTC) dust: an unprecedented disaster with inadequate risk management. Crit. Rev. Toxicol. 45, 492–530. doi: 10.3109/10408444.2015.1044601
Liu, W., Tang, P. T., Li, K., and Jiang, T. (2019). Demolition dust formation, diffusion mechanism and monitoring quantitative research on demolition of existing buildings. Appl. Ecol. Environ. Res. 17, 14543–14559. doi: 10.15666/aeer/1706_1454314559
López, A., and Lobo, A. (2014). Emissions of CandD refuse in landfills: a European case. Waste Manag. 34, 1446–1454. doi: 10.1016/j.wasman.2014.04.004
Maeda, H., Fujita, M. Q., Zhu, B.-L., Quan, L., Kamikodai, Y., Tsuda, K., et al. (2003). Labor-related fatalities in forensic postmortem investigations during the past 6 years in the southern half of Osaka city and surrounding areas. Leg. Med. 5, S325–S327. doi: 10.1016/S1344-6223(02)00162-1
McGee John, K., Chen Lung, C., Cohen Mitchell, D., Chee Glen, R., Prophete Colette, M., Haykal-Coates, N., et al. (2003). Chemical analysis of World Trade Center fine particulate matter for use in toxicologic assessment. Environ. Health Perspect. 111, 972–980. doi: 10.1289/ehp.5930
Molla, A. S., Tang, P., Sher, W., and Bekele, D. N. (2021). Chemicals of concern in construction and demolition waste fine residues: a systematic literature review. J. Environ. Manage. 299, 113654. doi: 10.1016/j.jenvman.2021.113654
Montero, A., Tojo, Y., Matsuo, T., Matsuto, T., Yamada, M., Asakura, H., et al. (2010). Gypsum and organic matter distribution in a mixed construction and demolition waste sorting process and their possible removal from outputs. J. Hazard. Mater. 175, 747–753. doi: 10.1016/j.jhazmat.2009.10.072
Morrell, J. J. (2006). “Chromated copper arsenate as a wood preservative,” in Environmental Impacts of Treated Wood, eds. T. G. Townsend and H. Solo-Gabriele, 1st ed (Boca Raton, FL: Taylor & Francis), 1–14. doi: 10.1201/9781420006216.sec1
National Minerals Information Center. (2018). Asbestos Statistics and Information: Minerals Yearbook: Advance Data Release of the 2018 Annual Tables Available online at: https://prd-wret.s3.us-west-2.amazonaws.com/assets/palladium/production/atoms/files/myb1-2018-asbes-adv.xlsx (accessed May 28, 2020).
Nie, Z., Yang, Z., Fang, Y., Yang, Y., Tang, Z., Wang, X., et al. (2015). Environmental risks of HBCDD from construction and demolition waste: a contemporary and future issue. Environ. Sci. Pollut. Res. 22, 17249–17252. doi: 10.1007/s11356-015-5487-2
Normohammadi, M., Kakooei, H., Omidi, L., Yari, S., and Alimi, R. (2016). Risk assessment of exposure to silica dust in building demolition sites. Saf. Health Work 7, 251–255. doi: 10.1016/j.shaw.2015.12.006
Odgerel, C. O., Takahashi, K., Sorahan, T., Driscoll, T., Fitzmaurice, C., Yoko, O. M., et al. (2017). Estimation of the global burden of mesothelioma deaths from incomplete national mortality data. Occup. Environ. Med. 74, 851–858. doi: 10.1136/oemed-2017-104298
Ogunmakinde, O., Sher, W., and Maund, K. (2019). An assessment of material waste disposal methods in the nigerian construction industry. Recycling 4, 13. doi: 10.3390/recycling4010013
Ogunseitan, O. A. (2015). The asbestos paradox: global gaps in the translational science of disease prevention. Bull. World Health Organ 93, 359–360. doi: 10.2471/BLT.14.142307
Peters, M. D. J., Marnie, C., Tricco, A. C., Pollock, D., Munn, Z., Alexander, L., et al. (2020). Updated methodological guidance for the conduct of scoping reviews. JBI Evid. Synth. 18, 2119–2126. doi: 10.11124/JBIES-20-00167
PubChem (1998). Parathion. National Library of Medicine: National Center for Biotechnology Information. Available online at: https://pubchem.ncbi.nlm.nih.gov/source/hsdb/197#section=EPA-Hazardous-Waste-Number (accessed February 24, 2022).
Puthussery, J. V., Kumar, R., and Garg, A. (2017). Evaluation of recycled concrete aggregates for their suitability in construction activities: an experimental study. Waste Manag. 60, 270–276. doi: 10.1016/j.wasman.2016.06.008
Rodríguez-Quijano, M., Jiménez-Rivero, A., de Guzmán-Báez, A., and García-Navarro, J. (2015). Gypsum Plasterboard Deconstruction to Recycling Economic Study in Europe, 665–674.
Saca, N., Dimache, A., Radu, L. R., and Iancu, I. (2017). Leaching behavior of some demolition wastes. J. Mater. Cycles Waste Manag. 19, 623–630. doi: 10.1007/s10163-015-0459-7
Schiopu, N., and Tiruta-Barna, L. (2012). “Wood preservatives,” in Toxicity of Building Materials, eds F. Pacheco-Torgal, S. Jalali and A. Fucic (Cambridge, UK: Woodhead Publishing Limited), 138–165. doi: 10.1533/9780857096357.138
Scholz, P. F., Materna, B. L., Harrington, D., and Uratsu, C. (2002). Residential and commercial painters' exposure to lead during surface preparation. Am. Ind. Hyg. Assoc. J. 63, 22–28. doi: 10.1080/15428110208984687
Secretariat of the Basel Convention (2017a). Technical guidelines on the environmentally sound management of wastes consisting of, containing or contaminated with pentachlorophenol and its salts and esters (PCP) (Geneva).
Secretariat of the Basel Convention (2017b). Technical guidelines on the environmentally sound management of wastes consisting of, containing or contaminated with polychlorinated biphenyls, polychlorinated terphenyls, polychlorinated naphthalenes or polybrominated biphenyls including hexabromobiphenyl (PCBs, PCTs, PCNs or PBBs, including HBB) (Geneva).
Secretariat of the Basel Convention (2017c). Technical guidelines: technical guidelines on the environmentally sound management of wastes consisting of, containing or contaminated with the pesticides aldrin, alpha hexachlorocyclohexane, beta hexachlorocyclohexane, chlordane, chlordecone, dieldrin, endrin, heptachlor, hexachlorobenzene, hexachlorobutadiene, lindane, mirex, pentachlorobenzene, pentachlorophenol and its salts, perfluorooctane sulfonic acid, technical endosulfan and its related isomers or toxaphene or with hexachlorobenzene as an industrial chemical (POP Pesticides) (Geneva).
Secretariat of the Basel Convention (nd). Framework for the Environmentally Sound Management of Hazardous Wastes Other Wastes. Geneva: United Nations Environment Programme. Available online at: http://www.basel.int/Implementation/CountryLedInitiative/EnvironmentallySoundManagement/ESMFramework/tabid/3616/Default.aspx (accessed October 7 2020).
Secretariat of the Stockholm Convention (nd). Hexabromocyclododecane. United Nations Environment Program (UNEP). Available online at: http://chm.pops.int/Implementation/Alternatives/AlternativestoPOPs/ChemicalslistedinAnnexA/HBCD/tabid/5861/Default.aspx (accessed June 4 2020).
Shim, Y., Jeong, J., Jeong, J., Lee, J., and Kim, Y. (2022). Comparative analysis of the national fatality rate in construction industry using time-series approach and equivalent evaluation conditions. Int. J. Environ. Res. Public Health 19, 195–204. doi: 10.3390/ijerph19042312
Shoji, T., and Egawa, Y. (2006). The structure of safety climates and its effects on workersandapos; attitudes and work safety at Japanese construction work sites. J. UOEH 28, 29–43. doi: 10.7888/juoeh.28.29
Singh, A., and Ladusingh, L. (2013). Increasing life expectancy and convergence of age at death in India. Genus 69, 83–99. Available online at: https://www.jstor.org/stable/genus.69.1.83
Sirrs, C. (2016). Accidents and apathy: the construction of the ‘Robens Philosophy' of occupational safety and health regulation in Britain, 1961–1974. Soc. Hist. Med. 29, 66–88. doi: 10.1093/shm/hkv068
Soltaninejad, K., and Shadnia, S. (2014). “History of the use and epidemiology of organophosphorus poisoning,” in Basic and Clinical Toxicology of Organophosphorus Compounds, eds M. Balali-Mood and M. Abdollahi (London: Springer London), 25–43. doi: 10.1007/978-1-4471-5625-3_2
Spasiano, D., and Pirozzi, F. (2017). Treatments of asbestos containing wastes. J. Environ. Manage 204, 82–91. doi: 10.1016/j.jenvman.2017.08.038
Stacey, P., Thorpe, A., and Roberts, P. (2011). Levels of Respirable Dust and Respirable Crystalline Silica at Construction Sites (UK).
Stayner, L., Welch, L. S., and Lemen, R. (2013). The worldwide pandemic of asbestos-related diseases. Annu. Rev. Public Health 34, 205–216. doi: 10.1146/annurev-publhealth-031811-124704
Stevulova, N., Estokova, A., Holub, M., Singovszka, E., and Csach, K. (2020). Characterization of demolition construction waste containing asbestos, and the release of fibrous dust particles. Appl. Sci. 10, 4048. doi: 10.3390/app10114048
t Mannetje, A., Steenland, K., Attfield, M., Boffetta, P., Checkoway, H., DeKlerk, N., et al. (2002). Exposure-response analysis and risk assessment for silica and silicosis mortality in a pooled analysis of six cohorts. Occup. Environ. Med. 59, 723. doi: 10.1136/oem.59.11.723
Takahashi, H. (2019). “An investigation of fatal accidents in demolition work of buildings,” in Tenth International Structural Engineering and Construction Conference (Chicago, IL: ISEC Press).
Tame, N. W., Dlugogorski, B. Z., and Kennedy, E. M. (2007). Formation of dioxins and furans during combustion of treated wood. Progress Energy Combust. Sci. 33, 384–408. doi: 10.1016/j.pecs.2007.01.001
The British Standards Institution (BS 8500-2:2015+A2:2019). Concrete—Complementary British Standard to BS EN 206 - Part 2: Specification for Constituent Materials and Concrete. London UK: The British Standards Institution.
The Construction (Design and Management) Regulations (1994). The Construction (Design and Management) Regulations.
The World Bank (2020). World Bank Open Data: Free and Open Access to Global Development Data. Available online at: https://data.worldbank.org/ (accessed December 14, 2020).
The World Bank. (nd). World Bank Country Lending Groups. The World Bank. Available online at: https://datahelpdesk.worldbank.org/knowledgebase/articles/906519-world-bank-country-and-lending-groups (accessed June 3 2020).
Townsend, T., Chadik, P., Bitton, G., Booth, M., Lee, S., and Yang, K. (2000). Gypsum Drywall Impact on Odor Production at Landfills: Science and Control Strategies (Gainesville, VA).
Tricco, A. C., Lillie, E., Zarin, W., O'Brien, K. K., Colquhoun, H., Levac, D., et al. (2018). PRISMA Extension for Scoping Reviews (PRISMA-ScR): checklist and explanation. Ann. Intern. Med. 169, 467–473. doi: 10.7326/M18-0850
Turner, A., and Solman, K. R. (2016). Lead in exterior paints from the urban and suburban environs of Plymouth, south west England. Sci. Total Environ. 547, 132–136. doi: 10.1016/j.scitotenv.2015.12.125
United States Environmental Protection Agency (2000). Effluent Limitations Guidelines, Pretreatment Standards, and New Source Performance Standards for the Landfills Point Source Category.
Van Praagh, M., and Modin, H. (2016). Leaching of chloride, sulphate, heavy metals, dissolved organic carbon and phenolic organic pesticides from contaminated concrete. Waste Manag. 56, 352–358. doi: 10.1016/j.wasman.2016.07.009
Velis, C. A., and Cook, E. (2021). Mismanagement of plastic waste through open burning with emphasis on the global south: a systematic review of risks to occupational and public health. Environ. Sci. Technol. 55, 7186–7207. doi: 10.1021/acs.est.0c08536
Vodyanitskii, Y. N. (2016). Biochemical processes in soil and groundwater contaminated by leachates from municipal landfills (mini review). Ann. Agrar. Sci. 14, 249–256. doi: 10.1016/j.aasci.2016.07.009
Wagner, A. C., Bergen, A., Brilke, S., Bühner, B., Ebert, M., Haunold, W., et al. (2017). Characterization of aerosol particles produced by a skyscraper demolition by blasting. J. Aerosol Sci. 112, 11–18. doi: 10.1016/j.jaerosci.2017.06.007
Wahab, A. B., and Lawal, A. F. (2011). An evaluation of waste control measures in construction industry in Nigeria. Afr. J. Environ. Sci. Technol. 5,246–254. Available online at: https://academicjournals.org/journal/AJEST/article-abstract/A67F57114037
Wasson, S. J., Linak, W. P., Gullett, B. K., King, C. J., Touati, A., Huggins, F. E., et al. (2005). Emissions of chromium, copper, arsenic, and PCDDs/Fs from open burning of CCA-treated wood. Environ. Sci. Technol. 39, 8865–8876. doi: 10.1021/es050891g
Wiedinmyer, C., Yokelson, R. J., and Gullett, B. K. (2014). Global emissions of trace gases, particulate matter, and hazardous air pollutants from open burning of domestic waste. Environ. Sci. Technol. 48, 9523–9530. doi: 10.1021/es502250z
Wielgosiński, G. (2011). The reduction of dioxin emissions from the processes of heat and power generation. J. Air Waste Manag. Assoc. 61, 511–526. doi: 10.3155/1047-3289.61.5.511
Wilson, D. C., Rodic, L., Modak, P., Soos, R., Carpintero, A., Velis, K., et al. (2015). Global Waste Management Outlook (Nairobi, Kenya: United Nations Environment Programme).
World Health Organization (1999). “hazard prevention and control in the work environment: airborne dust,” in Protection of the Human Environment Occupational and Environmental Health Series (Geneva: World Health Organization).
World Health Organization (2012). Rapid Risk Assessment of Acute Public Health Events. Geneva: World Health Organization.
World Health Organization (2019). “Download the raw data files of the WHO mortality database,” in Health Statistics and Information Systems. 15 December 2019 ed. (World Health Organization). Available online at: https://web.archive.org/web/20200714065349/https://www.who.int/healthinfo/statistics/mortality_rawdata/en/
World Health Organization (2020). World Health Data Platform. World Health Organization. Available online at: https://www.who.int/data (accessed October 13, 2020).
Xu, Q., Townsend, T., and Bitton, G. (2011). Inhibition of hydrogen sulfide generation from disposed gypsum drywall using chemical inhibitors. J. Hazard. Mater. 191, 204–211. doi: 10.1016/j.jhazmat.2011.04.063
Yang, K., Xu, Q., Townsend, T. G., Chadik, P., Bitton, G., and Booth, M. (2006). Hydrogen sulfide generation in simulated construction and demolition debris landfills: impact of waste composition. J. Air Waste Manag. Assoc. 56, 1130–1138. doi: 10.1080/10473289.2006.10464544
Zaharuddin, W., Paraskevas, I., and Liu, C. (2009). “Accident avoidance importance for building demolition,” in CIB W099 Working Commission on Construction Safety and Health, eds H. Lingard, T. Cooke, and M. Turner (Melbourne, VIC:. RMIT University).
Keywords: solid waste (MSW), informal recycling sector (IRS), circular economy, hazardous waste, accidents and causes, construction and demolition waste, occupational and public health and safety, open burning of solid waste
Citation: Cook E, Velis CA and Black L (2022) Construction and Demolition Waste Management: A Systematic Scoping Review of Risks to Occupational and Public Health. Front. Sustain. 3:924926. doi: 10.3389/frsus.2022.924926
Received: 20 April 2022; Accepted: 13 May 2022;
Published: 30 June 2022.
Edited by:
Maria Alzira Pimenta Dinis, Fernando Pessoa University, PortugalReviewed by:
Lubaba Sadaf, National University of Sciences and Technology (NUST), PakistanZujaja Wahaj, National University of Sciences and Technology, Pakistan
Copyright © 2022 Cook, Velis and Black. This is an open-access article distributed under the terms of the Creative Commons Attribution License (CC BY). The use, distribution or reproduction in other forums is permitted, provided the original author(s) and the copyright owner(s) are credited and that the original publication in this journal is cited, in accordance with accepted academic practice. No use, distribution or reproduction is permitted which does not comply with these terms.
*Correspondence: Costas A. Velis, c.velis@leeds.ac.uk