- 1Space Science Centre, Institute of Climate Change, Universiti Kebangsaan Malaysia, Bangi, Malaysia
- 2Department of Electrical, Electronic and Systems Engineering, Faculty of Engineering and Built Environment, Universiti Kebangsaan Malaysia, Bangi, Malaysia
- 3Department of Applied Physics, Faculty of Science and Technology, Universiti Kebangsaan Malaysia, Bangi, Malaysia
- 4Department of Physics, Faculty of Science, Universiti Putra Malaysia, Seri Kembangan, Malaysia
The effectiveness and accuracy of earthquake precursors are measured by the ability of the precursors to predict the time, epicentral distance, and magnitude of the earthquake for short-term earthquake prediction. Past articles were reviewed to examine various studies of short-term earthquake precursor detection, particularly those that involved monitoring variations in total electron content (TEC). In this review, we examine recent studies that explore the use of the TEC parameter in the Lithospheric-Atmospheric-Ionospheric (LAI) interaction to forecast earthquake characteristics, including detection time, epicentral distance, and magnitude. This review characterizes anomalous observations of TEC parameters that may be linked to subsequent seismic events and investigates their correlation with earthquake properties. It has been conclusively demonstrated that TEC parameters show significant variations prior to earthquakes, and these results can be used in combination with other parameters to forecast earthquake properties.
1 Introduction
Earthquake is a geophysical phenomenon involving irregular, non-linear, and complicated processes (Akhoondzadeh et al., 2010). Short-term earthquake prediction typically relies on the detection of physical phenomena or precursors occurring within a specific time frame from a few days to a few weeks (Hayakawa, 2015). In his study, Uyeda (2013) highlighted several critical factors that must be identified to achieve successful earthquake prediction, such as the timing of occurrence, epicenter location, and magnitude of the impending earthquake. Pulinets et al. (2004) identified two main approaches to short-term earthquake prediction: deterministic analysis of precursor behavior and statistical pattern processing. Scientific methods for earthquake prediction include monitoring variations in the geomagnetic field, ionospheric TEC, and surface displacements. (Sasmal et al., 2021; Su and Sha, 2022). This study focused on the correlations of LAI interaction, specifically TEC, prior to earthquakes for earthquake precursor study.
To monitor ionospheric perturbations and delays to GPS signals, various approaches can be employed to acquire TEC measurements, including ground-based ionosondes and GPS measurements. Ionosondes can observe only the bottom layer of the ionosphere; in contrast, a ground-based GPS network enables effective ionosphere monitoring (Choi et al., 2012). Only decades ago, there was a lack of extensive ground observations to monitor ionospheric parameters in most areas. The emergence of GPS sensing technology has marked a paradigm shift in the field of remote sensing, providing unprecedented opportunities to study the ionosphere with remarkable precision and accuracy. An advantage of GPS satellite measurement is that it allows spatial and time-dependent information of the disturbed region to be obtained (Sharma et al., 2010). Furthermore, networks of GPS receivers can be utilized to monitor ionospheric TEC in real-time, allowing for continuous monitoring of space weather events and seismic activity. (Zhao et al., 2010; Hasbi et al., 2011). Based on Contadakis et al. (2008), TEC variations can provide insight into a wide range of ionospheric changes, including global effects such as variations in the geomagnetic field, and solar activity, as well as local factors like atmospheric conditions, underground explosions, volcanic eruptions, and extreme weather phenomena. Additionally, TEC variations have been shown to be associated with seismic activity, providing a potentially valuable tool for earthquake monitoring and early warning.
The application of TEC measurements has been further employed in earthquake study. Akhoondzadeh et al. (2010) hypothesized that crust displacement during earthquake preparation leads to energy transfer. Changes in the physical and chemical properties of the lithosphere, atmosphere, and ionosphere have been observed to occur as precursors to earthquakes, often appearing several hours to days prior to the seismic event. (Akhoondzadeh and Saradjian, 2011). Further discussion on potential mechanisms of the origin of seismo-ionospheric perturbations were explained in previous studies. Fujinawa and Takahashi (1990) and Pulinets et al. (2000) proposed that the irregularities of the electron density formation in the ionosphere are caused by the penetration of the anomalous vertical field within the radius of the earthquake area while another theory suggests that earthquakes can produce atmospheric gravity waves (AGW), which can perturb the ionosphere (Hayakawa, 1999; Liu et al., 2006; Saradjian and Akhoondzadeh, 2011). The detailed aspects of the physical mechanism of the ionospheric variability associated with the seismic activity are described in Pulinets and Boyarchuk (2004).
2 Established principles from previous research
Global Ionospheric Maps (GIM) provide grid data of the vertical TEC (VTEC), has been used extensively by scientists to explore ionospheric phenomena. However, VTEC obtained from permanent GPS ground-stations offers greater precision and temporal resolution. Summary of the satellite network information used for the TEC data in this study is listed in the Supplementary Material. The GIM is produced from the TEC data obtained from over 400 stations located worldwide with its temporal resolution ranges from 1 to 2 h, while its spatial resolution is typically determined by the data provider and is measured at 2.5 ° × 5 ° (latitude × longitude). TEC data, derived from GPS, is acquired, and extracted using dual-frequency GPS measurements to determine the predominant portion of the total ionospheric delay or slant TEC (STEC) in the GPS signal’s propagation path, expressed as follows:
where the variables
in this equation, R refers to the radius of the earth, and H signifies the altitude of the upper boundary of the ionosphere. Likewise, Z represents the elevation angle of the satellite (Dong et al., 2022).
Irregular variations detected in TEC measurements exceeding a statistical threshold may be considered as a signature of an underground seismic source as proposed by previous researchers. (Naaman et al., 2001). Zhao et al. (2010) reported that anomalous TEC fluctuations occur several days before earthquakes, either increasing or decreasing over the preparation zone. According to Pulinets et al. (2000) pre-earthquake anomalies observed in low latitudes are believed to be caused by a strong vertical electric field. The seismogenic electric field rises upward and interacts with the eastward electric field, resulting in ionospheric disturbances near the epicenter and deforming the shape of the equatorial anomaly. (Hasbi et al., 2011). Lin (2014) suggested that ionizing radiation radon gas release could explain TEC anomalous fluctuations through electron density variations. The variability is also thought to be caused by other factors, such as air electrization and electromagnetic coupling between the atmosphere and the ionosphere. (Pulinets et al., 2007).
However, anomalies caused by seismic activity during the earthquake preparation period may be concealed by changes in global ionization due to UV solar radiation and geomagnetic activity. The ionospheric response to these factors is complex and varies under different geomagnetic-solar conditions. In their study, Akhoondzadeh and Saradjian (2011), found that the period of quiet geomagnetic variation before the earthquake day is suitable for pre-seismic ionospheric studies. Several researchers have demonstrated the use of global geomagnetic indices such as Disturbance Storm-Time (Dst), Planetary K-index (Kp), and solar radio flux at 10.7 cm (F10.7). The results showed positive correlations of TEC anomalies with earthquakes (Pulinets et al., 2007; Afraimovich and Astafyeva, 2008; Akhoondzadeh et al., 2010; Sharma et al., 2010; Zhao et al., 2010; Hasbi et al., 2011; Choi et al., 2012; Zhu et al., 2013; Tao et al., 2017; Shi et al., 2019; Hu et al., 2021; Dong et al., 2022).
In the effort to further distinguish the TEC anomalies from the non-seismic effects, Afraimovich and Astafyeva (2008) suggested that statistical analysis of TEC changes that considers all the leading global factors that may influence the TEC anomalies be conducted to establish the occurrence of the earthquake precursor. Astafyeva and Heki (2011), in their previous study, also demonstrated the essence of determining the locality of the TEC anomalies to establish the connection of the anomalies with earthquakes using the application of GPS. There are several techniques used to analyze ionospheric anomalies and their relationship with seismic activity to better understand the processes that occur before earthquakes and to develop new methods for earthquake prediction and early warning. Basic statistical tools, such as relative deviation, time derivative analysis, IQR analysis, correlation analysis, TEC difference analysis, and ionospheric correction, are also used to investigate the effect of ionospheric disturbances on ionospheric parameters. (Karatay et al., 2010).
Although this study only focused on the TEC parameters, previous studies have demonstrated on the importance of conducting multi-parameters study for earthquake prediction. Xu et al. (2011) found that utilizing multiple parameters can reveal the existence of LAI coupling in association with strong earthquakes. Simha et al. (2020) support this conclusion and recommend incorporating additional precursory parameters and examining more earthquakes to enhance short-term forecasting models. Sasmal et al. (2021), in their study, analyzed different parameters including TEC and magnetic field and found that these parameters are successful in being a convincing pre-seismic signature but with significant differences in their precursory time frames.
3 Earthquake properties
Earthquakes can be classified based on their properties which include: (a) magnitudes, to define the severity of earthquakes which is categorized as light (M0.0–4.9), moderate (M5.0–5.9), strong (M6.0-M6.9), major (M7.0–7.9), and great (M8.0 and higher), as reported in the U.S. Geological Survey (2010); and (b) depth which is categorized as shallow-focus (focal depth lower than 70 km from earth’s surface), intermediate depth or mid-focus (focal depth between 70–300 km), and deep-focus (focal depth at a greater depth ranging from 300–700 km) as defined by Hayakawa (2015). This section discusses further on previous findings on the relationship between the earthquake properties with TEC changes in terms of the earliest time of precursor detection, the magnitude of the earthquake, the epicentral distance from the GPS receivers, and the hypocentral depth of the earthquake.
3.1 Time
An essential aspect of earthquake prediction is time of occurrence. Previous studies have shown that the earthquake precursors can be found in the TEC variation several days before the earthquake. Each of these studies has shown a different range of time when the precursor was detected, ranging from 6–7 h to 36 days before the earthquake occurrence. The earliest time of precursor detection was most frequently found to be 3–5 days before the earthquake (Figure 1). These results are crucial for selecting the observation period before the earthquake. Previous studies have shown that TEC anomalies can be found in less than 40 days. However, Astafyeva and Heki (2011) in their study had decided to analyze TEC variations of ∼160 days based on the justification that long periods could exclude any ionospheric TEC changes caused by planetary waves and other kinds of non-seismic variability. Meanwhile, Saradjian and Akhoondzadeh (2011) determined that the appropriate period to show normal behavior might be about 45 days before the events. This extended period is sufficient to detect both normal and abnormal signals. In contrast, the precursors identified by the latter study are expected to appear near the end of the period analyzed. The determination of the duration of the TEC observation period duration is very crucial to ensure there are no missed significant precursors before the earthquake event.
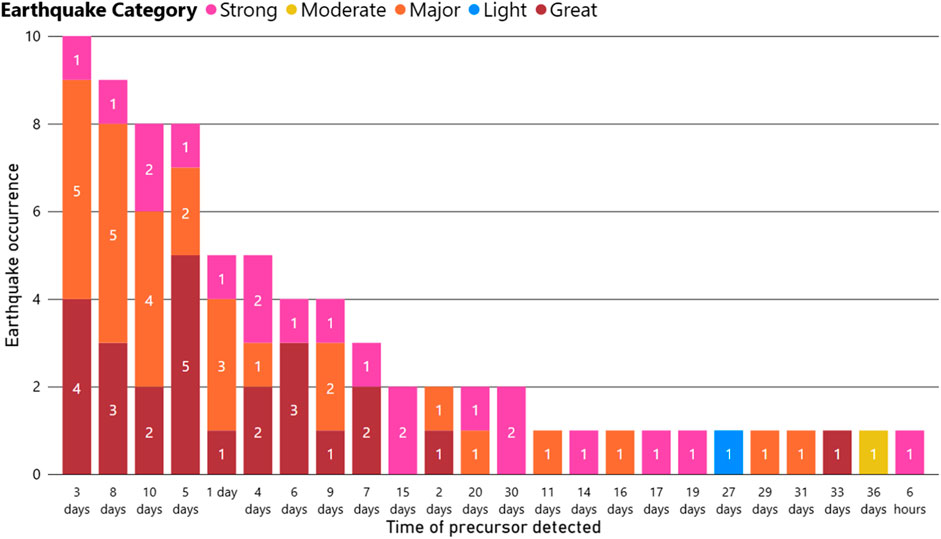
FIGURE 1. The number of earthquakes with precursors in relationship with the earliest time it was detected before earthquake, and size of magnitude based on previous studies (Naaman et al., 2001; Pulinets et al., 2004; Pulinets et al., 2007; Afraimovich and Astafyeva, 2008; Akhoondzadeh et al., 2010; Sharma et al., 2010; Zhao et al., 2010; Akhoondzadeh and Saradjian, 2011; Hasbi et al., 2011; Kon et al., 2011; Saradjian and Akhoondzadeh, 2011; Xu et al., 2011; Zolotov et al., 2011; Akhoondzadeh, 2012; 2013; Choi et al., 2012; Zhu et al., 2013; Lin, 2014; Tang et al., 2015; Daneshvar and Freund, 2016; Oikonomou et al., 2016; Jiang et al., 2017; Sharma et al., 2017; Tao et al., 2017; Akpan et al., 2019; Li et al., 2019; Shi et al., 2019; Yang et al., 2019; Simha et al., 2020; Tachema and Nadji, 2020; Chen et al., 2021; Mahmoudian and Ghayour, 2021; Mehdi et al., 2021; Sasmal et al., 2021; Dong et al., 2022).
3.2 Magnitude
As described by Pulinets et al. (2007), the magnitude of an earthquake exhibits the exponential change in the amount of energy emitted throughout the seismic event. Therefore, the size of the earthquake preparation area discussed in Section 3.2 was established based on the magnitude of the earthquake. Based on the number of earthquakes with precursor presence detected in previous studies, major earthquakes showed the highest percentage at 38%, which is then followed by 33% for great earthquakes and 27% for strong earthquakes. Both light and moderate earthquakes showed only 2% occurrences each in terms of total precursors found (Figure 1).
Kon et al. (2011) and Zhu and Jiang (2020) concur that the frequency of ionospheric TEC disturbances is directly proportional to the magnitude of the earthquake. Hence, as the magnitude of the earthquake increases, any slight rise in the occurrence rates of TEC disturbances preceding the event is more likely to be associated with the impending seismic activity (Pulinets et al., 2003). Akhoondzadeh and Saradjian (2011) highlighted the importance of using specific bounds or threshold values for earthquake precursor detection, depending on the magnitude of the earthquake. They noted that lower magnitude earthquakes (M < 6.0) exhibit weaker anomalies, which can result in inaccurate results if the same bounds or thresholds are used for stronger earthquakes. Therefore, it is necessary to conduct an empirical evaluation to select appropriate bounds or thresholds for each case study. By categorizing earthquakes into different magnitude ranges, the potential bias in the analysis can be minimized.
3.3 Epicentral and hypocentral distance
The receiver’s distance from the earthquake epicenter is a significant characteristic to be considered before analyzing the TEC variations for earthquake precursor. According to Pulinets et al. (2007), the amplitude of earthquake precursors decreases as the distance from the epicenter increases. This is because the ionospheric anomalies are believed to be generated by the seismogenic electric field, which has a stronger effect closer to the epicenter and weaker effect farther away. The study by Sharma et al. (2017) demonstrated that the TEC concentration increases when the distance from the epicenter decreases. Due to these theories, many researchers have applied the concept of earthquake preparation area that was initially introduced by Dobrovolsky et al. (1979) to calculate the effective radius of the seismogenic zone, using the elastic deformation calculations, where R and M are the radius of the effective seismogenic zone and magnitude of the earthquake, respectively (Pulinets et al., 2004; Sharma et al., 2010; Akhoondzadeh and Saradjian, 2011; Hasbi, et al., 2011; Akhoondzadeh, 2012; Choi et al., 2012; Oikonomou et al., 2016; Sharma et al., 2017; Tao et al., 2017; Simha et al., 2020).
In our review, only one study that applied the updated Dobrovolsky formula was found, namely, in Saradjian and Akhoondzadeh (2011), where R and M are the radius of the effective seismogenic zone and magnitude of the earthquake, respectively (Dobrovolsky et al., 1989).
Previous research has demonstrated that the ionospheric variability exhibits distinct characteristics within the seismogenic zone compared to the irregularities outside the area (Pulinets et al., 2007). As shown in Figure 2, most of the precursors were found within the earthquake preparation zone (Pulinets et al., 2004; Sharma et al., 2010; Akhoondzadeh and Saradjian, 2011; Hasbi, et al., 2011; Akhoondzadeh, 2012; Choi et al., 2012; Oikonomou et al., 2016; Sharma et al., 2017; Tao et al., 2017; Simha et al., 2020) (Figure 2). However, Pulinets et al. (2004) cautioned that the ionospheric irregularity does not necessarily only appear within the epicenter area as it could be displaced along the geomagnetic field lines towards the magnetic equator, which lies equatorward of the vertical projection of the epicenter of the forthcoming seismic activity on the ionosphere. Thus, there is a need for further study in determining the maximum area in which the shifted ionospheric irregularity will occur. In terms of the hypocenter depth, most precursors were found in shallow and intermediate depths with only one study finding precursors in a deep-focus earthquake of 378.8 km depth (Lin, 2014).
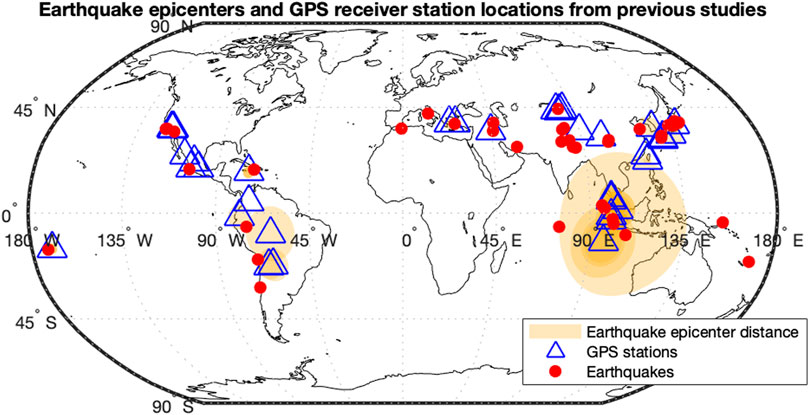
FIGURE 2. Locations of earthquakes studied by previous researchers in connection with the GPS stations. The brown circle indicates earthquake precursors presence within the radius of the epicentral distance from the GPS stations (Naaman et al., 2001; Pulinets et al., 2004; 2007; Afraimovich and Astafyeva, 2008; Akhoondzadeh et al., 2010; Sharma et al., 2010; Zhao et al., 2010; Akhoondzadeh and Saradjian, 2011; Hasbi et al., 2011; Kon et al., 2011; Saradjian and Akhoondzadeh, 2011; Xu et al., 2011; Zolotov et al., 2011; Akhoondzadeh, 2012; 2013; Choi et al., 2012; Zhu et al., 2013; Lin, 2014; Tang et al., 2015; Daneshvar and Freund, 2016; Oikonomou et al., 2016; Jiang et al., 2017; Sharma et al., 2017; Tao et al., 2017; Akpan et al., 2019; Li et al., 2019; Shi et al., 2019; Yang et al., 2019; Simha et al., 2020; Tachema and Nadji, 2020; Chen et al., 2021; Mahmoudian and Ghayour, 2021; Mehdi et al., 2021; Sasmal et al., 2021; Dong et al., 2022).
4 Highlights of future directions
Earthquake properties in terms of time, magnitude, and hypocentral depth have been discussed in Section 3 of this paper. Previous studies on the seismo-ionospheric relationship were mostly conducted on a case study basis. This study has revealed the similarities and differences of each study to provide a systematic view on the findings of TEC anomalies as the earthquake precursors. Despite numerous studies on pre-earthquake ionospheric anomalies, this field remains incompletely understood. In addition, ionospheric perturbations are vulnerable to other dynamic processes (Xia et al., 2011). Another interesting factor or effect that has not been considered by many in the ionospheric study is the effect of the faulting mechanism on the TEC variations. For instance, Naaman et al. (2001) only associated atmospheric waves propagating upward with earthquakes that are caused by strong vertical movement of the earth’s surface, instead of earthquakes caused by horizontal tectonic motion. Conducting studies on this effect is therefore crucial to ascertain the effect of seismic activities on TEC changes.
The variability of ionospheric variations makes it difficult to distinguish the anomalies caused by enhanced pre-seismic activity from those caused by global and local effects. The critical question is to ascertain whether the ionospheric anomalies before the earthquake are seismic related. This is a rather controversial issue within the geophysics and space physics research community as some believe it could be a precursor, while some believe it is related to other global or local effects. Due to the controversy and uncertainty surrounding the detection of earthquake precursors and their validity in influencing the ionosphere, it is important to exercise caution before asserting the detection of ionospheric precursors to seismic activity (Masci, 2012; Masci, 2013).
In making further recommendations on seismo-ionospheric relationship study, Zhao et al. (2010) suggested that any effort to find abnormal electromagnetic signals prior to the earthquake must be made following the TEC variation. A future study integrating local geomagnetic disturbances and ionospheric irregularities would be very interesting since seismic activities were originated from regional source. Another significant recommendation in this study is to establish a denser network of GNSS stations for better TEC data acquisition since it has been implied that even the low-magnitude events can be monitored if the location of the GNSS receivers located close to the epicenter (Sharma et al., 2017). In addition, it is important to conduct various statistical analyses as it can provide valuable insights into the relationship between earthquakes and ionospheric perturbations (Xia et al., 2011). The findings of this research provide insights for future studies and could serve as a base in monitoring the ionospheric responses particularly TEC changes to seismic activities.
5 Key concepts
GPS advancement. The advent of GPS and its benefit to ionospheric study.
LAI interaction with earthquakes. The interaction of different layer of earth (lithosphere, atmosphere, and ionosphere) with seismic activities.
TEC as earthquake precursor. Irregular variations detected in TEC measurements exceeding a statistical threshold might be considered as a signature of an underground seismic source by previous researchers.
Multi-parameters study for earthquake study. The application of different indicators such as TEC variations, geomagnetic variations, and atmospheric parameters in earthquake study.
Author contributions
NFIZ: Developing concept of the work; the acquisition, analysis, and interpretation of data for the work and drafting the work. NSAH, KAY, and SAB: Revising it critically for important intellectual content. MA: Final approval of the version to be published; being accountable for all aspects of the work in ensuring that questions related to the accuracy or integrity of any part of the work are appropriately investigated and resolved. All authors contributed to the article and approved the submitted version.
Funding
The research was funded by the Ministry of Higher Education (MOHE), Malaysia through the Fundamental Research Grant Scheme under the grant number FRGS/1/2020/TK0/UKM/01/1 through Universiti Kebangsaan Malaysia.
Conflict of interest
The authors declare that the research was conducted in the absence of any commercial or financial relationships that could be construed as a potential conflict of interest.
Publisher’s note
All claims expressed in this article are solely those of the authors and do not necessarily represent those of their affiliated organizations, or those of the publisher, the editors and the reviewers. Any product that may be evaluated in this article, or claim that may be made by its manufacturer, is not guaranteed or endorsed by the publisher.
Supplementary material
The Supplementary Material for this article can be found online at: https://www.frontiersin.org/articles/10.3389/fspas.2023.1166394/full#supplementary-material
References
Afraimovich, E. L., and Astafyeva, E. (2008). TEC anomalies-local TEC changes prior to earthquakes or TEC response to solar and geomagnetic activity changes? Earth Planets Space 60, 961–966. doi:10.1186/bf03352851
Akhoondzadeh, M. (2012). Anomalous TEC variations associated with the powerful tohoku earthquake of 11 march 2011. Nat. Hazards Earth Syst. Sci. 12 (5), 1453–1462. doi:10.5194/nhess-12-1453-2012
Akhoondzadeh, M., Parrot, M., and Saradjian, M. R. (2010). Electron and ion density variations before strong earthquakes (M >6.0) using DEMETER and GPS data. Nat. Hazards Earth Syst. Sci. 10, 7–18. doi:10.5194/nhess-10-7-2010
Akhoondzadeh, M., and Saradjian, M. R. (2011). TEC variations analysis concerning Haiti (january 12, 2010) and Samoa (september 29, 2009) earthquakes. Adv. Space Res. 47 (1), 94–104. doi:10.1016/j.asr.2010.07.024
Akhoondzadeh, M. (2013). Support vector machines for TEC seismo-ionospheric anomalies detection. Ann. Geophys. 31 (2), 173–186. doi:10.5194/angeo-31-173-2013
Akpan, A. E., Ibanga, J. I., George, N. J., and Ekanem, A. M. (2019). Assessing seismo-ionospheric disturbances using Vanuatu and honshu earthquakes of march 25, 2007, employing DEMETER and GPS data. Int. J. Environ. Sci. Technol. 16 (11), 7187–7196. doi:10.1007/s13762-019-02339-x
Astafyeva, E., and Heki, K. (2011). Vertical TEC over seismically active region during low solar activity. J. Atmos. Solar-Terrestrial Phys. 73 (13), 1643–1652. doi:10.1016/j.jastp.2011.02.020
Chen, H., Miao, M., Chang, Y., Wang, Q., Shen, X., Hattori, K., et al. (2021). Singular spectrum analysis of the total electron content changes prior to M ≥ 6.0 earthquakes in the Chinese mainland during 1998–2013. Front. Earth Sci. 9, 1–12. doi:10.3389/feart.2021.677163
Choi, B. K., Park, J. U., and Lee, S. J. (2012). Ionospheric anomalies observed over South Korea preceding the great tohoku earthquake of 2011. Adv. Space Res. 50 (3), 311–317. doi:10.1016/j.asr.2012.04.011
Contadakis, M. E., Arabelos, D. N., Asteriadis, G., Spatalas, S. D., and Pikridas, C. (2008). TEC variations over the Mediterranean during the seismic activity period of the last quarter of 2005 in the area of Greece. Hazards Earth Syst. Sci. 8, 1267–1276. doi:10.5194/nhess-8-1267-2008
Daneshvar, M. R. M., and Freund, F. T. (2016). Remote sensing of atmospheric and ionospheric signals prior to the mw 8.3 illapel earthquake, Chile 2015. Pure Appl. Geophys. 174 (1), 11–45. doi:10.1007/s00024-016-1366-0
Dobrovolsky, I. P., Gershenzon, N. J., and Gokhberg, M. B. (1989). Theory of electrokinetic effects occurring at the final stage in the preparation of a tectonic earthquake. Phys. Earth Planet. Interiors 57, 144–156. doi:10.1016/0031-9201(89)90224-0
Dobrovolsky, I. P., Zubkov, S. I., and Miachkin, V. I. (1979). Estimation of the size of earthquake preparation zones. Pageoph 117, 1025–1044. doi:10.1007/bf00876083
Dong, Y., Gao, C., Long, F., and Yan, Y. (2022). Suspected seismo-ionospheric anomalies before three major earthquakes detected by gims and gps tec of permanent stations. Remote Sens. 14 (1), 20. doi:10.3390/rs14010020
Fujinawa, Y., and Takahashi, K. (1990). Emission of electromagnetic radiation preceding the ito seismic swarm of 1989. Nature 347, 376–378. doi:10.1038/347376a0
Hasbi, A. M., Mohd Ali, M. A., and Misran, N. (2011). Ionospheric variations before some large earthquakes over sumatra. Nat. Hazards Earth Syst. Sci. 11 (2), 597–611. doi:10.5194/nhess-11-597-2011
Hayakawa, M. (1999). Atmospheric and ionospheric electromagnetic phenomena with earthquakes. Tokyo: Terra Scientific Publishing.
Hayakawa, M. (2015). Earthquake prediction with radio techniques. Hoboken: Wiley. doi:10.1002/9781118770368
Hu, T., Yao, Y., and Kong, J. (2021). Study of spatial and temporal variations of ionospheric total electron content in Japan, during 2014–2019 and the 2016 kumamoto earthquake. Sensors 21 (6), 2156. doi:10.3390/s21062156
Jiang, W., Ma, Y., Zhou, X., Zhao, L., An, X., and Wang, K. (2017). Analysis of ionospheric vertical total electron content before the 1 april 2014 Mw 8.2 Chile earthquake. J. Seismol. 21 (6), 1599–1612. doi:10.1007/s10950-017-9684-y
Karatay, S., Arikan, F., and Arikan, O. (2010). Investigation of total electron content variability due to seismic and geomagnetic disturbances in the ionosphere. Radio Sci. 45 (5), 1–12. doi:10.1029/2009RS004313
Kon, S., Nishihashi, M., and Hattori, K. (2011). Ionospheric anomalies possibly associated with M 6.0 earthquakes in the Japan area during 1998-2010: Case studies and statistical study. J. Asian Earth Sci. 41 (4–5), 410–420. doi:10.1016/j.jseaes.2010.10.005
Li, M., Lu, J., Zhang, X., and Shen, X. (2019). Indications of ground-based electromagnetic observations to a possible lithosphere- atmosphere-ionosphere electromagnetic coupling before the 12 may 2008 wenchuan MS 8.0 earthquake. Atmosphere 10 (7), 355. doi:10.3390/atmos10070355
Lin, J. W. (2014). Ionospheric precursor for a deep earthquake (∼378 km) near Papua New Guinea occurred on 7 July 2013,Mw= 7.2 in the environment of geomagnetic storm using two-dimensional principal component analysis. Georisk 8 (2), 141–146. doi:10.1080/17499518.2014.880853
Liu, J. Y., Chen, Y. I., Chuo, Y. J., and Chen, C. S. (2006). A statistical investigation of preearthquake ionospheric anomaly. J. Geophys. Res. Space Phys. 111 (5), A05304–A05305. doi:10.1029/2005JA011333
Mahmoudian, A., and Ghayour, A. S. (2021). Study of local ionospheric plasma perturbation induced by pre-seismic activities. Acta Geophys. 69 (5), 1585–1595. doi:10.1007/s11600-021-00633-2
Masci, F. (2013). Brief communication: Further comments on the ionospheric precursor of the 1999 hector mine earthquake. Nat. Hazards Earth Syst. Sci. 13 (1), 193–196. doi:10.5194/nhess-13-193-2013
Masci, F. (2012). The Study of Ionospheric Anomalies in Japan Area during 1998-2010 by Kon et al.: An Inaccurate Claim of Earthquake-Related Signatures? J. Asian Earth Sci. 57, 1–5. doi:10.1016/j.jseaes.2012.06.009
Mehdi, S., Shah, M., and Naqvi, N. A. (2021). Lithosphere atmosphere ionosphere coupling associated with the 2019 Mw 7.1 California earthquake using GNSS and multiple satellites. Environ. Monit. Assess. 193 (8), 501. doi:10.1007/s10661-021-09278-6
Naaman, S., Alperovich, L. S., Wdowinski, S., Hayakawa, M., and Calais, E. (2001). Comparison of simultaneous variations of the ionospheric total electron content and geomagnetic field associated with strong earthquakes. Nat. Hazards Earth Syst. Sci. 1, 53–59. doi:10.5194/nhess-1-53-2001
Oikonomou, C., Haralambous, H., and Muslim, B. (2016). Investigation of ionospheric TEC precursors related to the M7.8 Nepal and M8.3 Chile earthquakes in 2015 based on spectral and statistical analysis. Nat. Hazards 83, 97–116. doi:10.1007/s11069-016-2409-7
Pulinets, S. A., Gaivoronska, T. B., Contreras, A. L., and Ciraolo, L. (2004). Correlation analysis technique revealing ionospheric precursors of earthquakes. Nat. Hazards Earth Syst. Sci. 4, 697–702. doi:10.5194/nhess-4-697-2004
Pulinets, S. A., Boyarchuk, K. A., Hegai, V. V., Kim, V. P., and Lomonosov, A. M. (2000). Quasielectrostatic model of atmosphere-thermosphere-ionosphere coupling. Adv. Space Res. 26, 1209–1218. doi:10.1016/s0273-1177(99)01223-5
Pulinets, S. A., Kotsarenko, A. N., Ciraolo, L., and Pulinets, I. A. (2007). Special case of ionospheric day-to-day variability associated with earthquake preparation. Adv. Space Res. 39 (5), 970–977. doi:10.1016/j.asr.2006.04.032
Pulinets, S. A., Legen’ka, A. D., Gaivoronskaya, T. v., and Kh Depuev, V. (2003). Main phenomenological features of ionospheric precursors of strong earthquakes. J. Atmos. Solar-Terrestrial Phys. 65 (16–18), 1337–1347. doi:10.1016/j.jastp.2003.07.011
Saradjian, M. R., and Akhoondzadeh, M. (2011). Prediction of the date, magnitude and affected area of impending strong earthquakes using integration of multi precursors earthquake parameters. Nat. Hazards Earth Syst. Sci. 11 (4), 1109–1119. doi:10.5194/nhess-11-1109-2011
Sasmal, S., Chowdhury, S., Kundu, S., Politis, D. Z., Potirakis, S. M., Balasis, G., et al. (2021). Pre-seismic irregularities during the 2020 samos (Greece) earthquake (m = 6.9) as investigated from multi-parameter approach by ground and space-based techniques. Atmosphere 12 (8), 1059. doi:10.3390/atmos12081059
Sharma, G., Champati ray, P. K., Mohanty, S., and Suresh, K. (2017). Ionospheric TEC modelling for earthquakes precursors from GNSS data. Quat. Int. 462, 65–74. doi:10.1016/j.quaint.2017.05.007
Sharma, K., Dabas, R. S., Sarkar, S. K., Das, R. M., Ravindran, S., and Gwal, A. K. (2010). Anomalous enhancement of ionospheric F2 layer critical frequency and total electron content over low latitudes before three recent major earthquakes in China. J. Geophys. Res. 115. doi:10.1029/2009ja014842
Shi, K., Liu, X., Guo, J., Liu, L., You, X., and Wang, F. (2019). Pre-earthquake and coseismic ionosphere disturbances of the mw 6.6 lushan earthquake on 20 april 2013 monitored by CMONOC. Atmosphere 10 (4), 216–220. doi:10.3390/ATMOS10040216
Simha, C. P., Natarajan, V., and Madhusudhana Rao, K. (2020). Pre-earthquake atmospheric and ionospheric anomalies before taiwan earthquakes (M 6.1 and M 6.4) on february (4th and 6th), 2018. Geomagnetism Aeronomy 60 (5), 644–660. doi:10.1134/S0016793220050151
Su, Y. C., and Sha, J. (2022). A study of possible correlations between seismo-ionospheric anomalies of GNSS total electron content and earthquake energy. Remote Sens. 14 (5), 1155. doi:10.3390/rs14051155
Tachema, A., and Nadji, A. (2020). Contribution of ionospheric TEC anomalies to detecting the seismic precursors related to the 2008 oran-Algeria event. Adv. Space Res. 65 (11), 2559–2572. doi:10.1016/j.asr.2020.03.007
Tang, J., Yao, Y., and Zhang, L. (2015). Temporal and spatial ionospheric variations of 20 april 2013 earthquake in yaan, China. IEEE Geoscience Remote Sens. Lett. 12 (11), 2242–2246. doi:10.1109/lgrs.2015.2463081
Tao, D., Cao, J., Battiston, R., Li, L., Ma, Y., Liu, W., et al. (2017). Seismo-ionospheric anomalies in ionospheric TEC and plasma density before the 17 July 2006 M 7.7 south of Java earthquake. Ann. Geophys. 35 (3), 589–598. doi:10.5194/angeo-35-589-2017
U.S. Geological Survey (2010). Earthquake facts and statistics 2010. Avaialable at: https://web.archive.org/web/20100524161817/http://earthquake.usgs.gov/earthquakes/eqarchives/year/eqstats.php.
Uyeda, S. (2013). On earthquake prediction in Japan. Proc. Jpn. Acad. Ser. B Phys. Biol. Sci. 89 (9), 391–400. doi:10.2183/pjab.89.391
Xia, C., Wang, Q., Tao, Y., Xu, G., and Yang, S. (2011). Variations of ionospheric total electron content before three strong earthquakes in the qinghai-tibet region. Adv. Space Res. 47 (3), 506–514. doi:10.1016/j.asr.2010.09.006
Xu, T., Chen, Z., Li, C., Wu, J., Hu, Y., and Wu, Z. (2011). GPS total electron content and surface latent heat flux variations before the 11 march 2011 M9.0 sendai earthquake. Adv. Space Res. 48 (8), 1311–1317. doi:10.1016/j.asr.2011.06.024
Yang, J., Zhou, Y., Lin, J., Su, F., and Shen, W. (2019). Possible seismo-ionospheric precursor of the 12 february 2014 yutian earthquake in China. J. Earth Syst. Sci. 128 (3), 53. doi:10.1007/s12040-019-1069-x
Zhao, B., Wang, M., Tao, Y., Xu, G., Wan, W., and Liu, L. (2010). Ionospheric total electron content variations prior to the 2008 wenchuan earthquake. Int. J. Remote Sens. 31 (13), 3545–3557. doi:10.1080/01431161003727622
Zhu, F., and Jiang, Y. (2020). Investigation of GIM-TEC disturbances before M ≥ 6.0 inland earthquakes during 2003–2017. Sci. Rep. 10 (1), 18038. doi:10.1038/s41598-020-74995-w
Zhu, F., Zhou, Y., and Wu, Y. (2013). Anomalous variation in GPS TEC prior to the 11 april 2012 sumatra earthquake. Astrophysics Space Sci. 345 (2), 231–237. doi:10.1007/s10509-013-1389-2
Keywords: ionosphere, geomagnetic, total electron content (TEC), earthquake, global positioning system (GPS)-TEC
Citation: Zulhamidi NFI, Abdullah M, Abdul Hamid NS, Yusof KA and Bahari SA (2023) Investigating short-term earthquake precursors detection through monitoring of total electron content variation in ionosphere. Front. Astron. Space Sci. 10:1166394. doi: 10.3389/fspas.2023.1166394
Received: 15 February 2023; Accepted: 04 May 2023;
Published: 05 June 2023.
Edited by:
Erman Şentürk, Kocaeli University, TürkiyeReviewed by:
Sampad Kumar Panda, KL University, IndiaCopyright © 2023 Zulhamidi, Abdullah, Abdul Hamid, Yusof and Bahari. This is an open-access article distributed under the terms of the Creative Commons Attribution License (CC BY). The use, distribution or reproduction in other forums is permitted, provided the original author(s) and the copyright owner(s) are credited and that the original publication in this journal is cited, in accordance with accepted academic practice. No use, distribution or reproduction is permitted which does not comply with these terms.
*Correspondence: Mardina Abdullah, bWFyZGluYUB1a20uZWR1Lm15