- 1Department of Biology, Arrowhead Pharmaceuticals, Madison, WI, United States
- 2Department of Chemistry, Arrowhead Pharmaceuticals, Madison, WI, United States
- 3Department of Nuclear Engineering and Radiological Sciences, University of Michigan, Ann Arbor, MI, United States
- 4Department of Human Oncology, University of Wisconsin, Madison, WI, United States
Previously, we presented a hypothesis on the Darwinian evolution of liposomes that relies only on natural and ever-present phenomena: solar UV radiation, day/night cycle, gravity, and the formation/release of amphiphiles in aqueous media. The new hypothesis comprises the necessary prerequisites for Darwinian evolution: adaptive traits and selective forces. The hypothesis suggests that amphiphilic molecules, introduced in the Archean water, inevitably form the Langmuir layer, bilayer, and liposomes at the water-air interface. The solar UV radiation could destroy the liposomes unless they acquire a crucial adaptive trait–the heavy content that sinks them to the bottom of the pool and facilitates protection from UV. The hypothesis makes two testable predictions: 1) ferric salts of Archean waters attenuate the UV, and 2) the attenuation protects the liposomes from solar UV destruction. The assumption of UV attenuation by ferric salt solutions has been tested by confirming that two salts, iron trichloride and ferric ammonium citrate, possess strong UV attenuation, reducing the UV intensity by a factor of 1,000 at a submersion depth of 6.3 mm and 7.4 mm, respectively. In the present study, we have tested the second conjecture by demonstrating that 10 mm of solutions, the ferric ammonium citrate or iron trichloride, completely protect UV-sensitive liposomes from UV damage. These results reinforce the proposed hypothesis and suggest that the Sun UV radiation and gravity could be the major selection forces for the abiogenesis.
Introduction
In our previous communication (Subbotin and Fiksel, 2023b), we considered the Archean water-air interface to be a spatial plane crucial for abiogenesis, in which the emergence of liposomes plays a critical role. At the same time, the formation and fate of liposomes can be strongly affected by interaction with solar UV, which can result in their destruction. Following the idea of Martin Rutten (Rutten, 1971), we suggested that some liposomes encapsulate a solute heavier than the surrounding media and, by descending from the water-air interface, are protected from UV because of the UV absorption in water. We also calculated the depth-vs-time dynamics of the submergence, which depends on known physical characteristics: liposomal size, specific gravity, and viscosity of the media (Subbotin and Fiksel, 2023b). However, the UV-shielding ability of the presumable Archean water strongly depends on its composition and remains a subject of experimental tests.
In the follow-up experiments (Subbotin and Fiksel, 2023a), we examined the UV-shielding ability of several ferric salts: FeCl2 (iron dichloride), FeCl3 (iron trichloride), Fe(NO3)3 (ferric nitride), NH4Fe(SO4)2 (ferric ammonium sulfate), and (NH4)5 [Fe(C6H4O7)2] (ferric ammonium citrate), which were suggested to be present in the Archean waters (Breslow et al., 2010; Gómez et al., 2007; Handschuh and Orgel, 1973; Hardy et al., 2015; Keefe and Miller, 1996; Kim et al., 2016; Miller and Urey, 1959; Patel et al., 2015; Sproul, 2015). The results showed that solutions of two salts, FeCl3 (iron trichloride) and (NH4)5 [Fe(C6H4O7)2] (ferric ammonium citrate), demonstrated very strong UV attenuation: the UV intensity drops by a factor of 1,000 at a submersion depth of 6.3 mm and 7.4 mm, respectively at a concentration of 2.5 g/L, the concentration previously suggested for the Archean waters (Gómez and others 2007).
In the present study, we investigated 1) what part of the UV spectrum produces a damaging effect on UV-sensitive liposomes and 2) whether the solutions of iron trichloride and ferric ammonium citrate can reduce or eliminate the UV damaging effect and protect liposomes.
Materials and methods
Liposomes
Although there are few publications utilizing UV-sensitive liposomes, (Liu et al., 2017; Sihorwala et al., 2023), the only well-characterized commercial source of UV-sensitive liposomes, to the best of our knowledge, appeared to be the Encapsula NanoSciences Company. We used liposomes that this company has already developed, which are large polymerizable UV-sensitive multilamellar micron-sized liposomes composed of Dibehenoyl-sn-glycero-3-phosphocholine (DBPC) and a 10,12-Pentacosadiynoic acid (PCDA) with DBPC: PCDA molar ratio as 80:20, at a total lipid concentration of 5 mM in a PBS buffer at a pH value of 7.4 (Encapsula NanoSciences, Brentwood, TN, USA). The PCDA is a polymerizable unsaturated fatty acid. PCDA lipids adjacent to each other polymerize upon UV radiation, changing their color from the original white to blue (Ahn et al., 2009). In the liposomes used, the PCDA lipids are organized into separate domains in the liposomal membrane using phase separation engineering (Mir, 2024) (i.e., not evenly distributed in the membrane). Additionally to color change, the PCDA domains’ polymerization breaks membrane integrity, causing liposomal disintegration into discs and micelles. The correlation of color change with the physical destruction of liposomes was tested and confirmed by the manufacturer using the QELS technique with Zetasizer Nano equipment (Mir, 2024). To destroy the liposomes, the manufacturer used the 20 W UV-C Lamp (245 nm) (Rexim, LLC, Bradenton, FL, USA). No other UV sources, including Solar UV, were tested.
In all our experiments, 100 µL of undiluted liposome suspension was used. Based on our measurements, this liposome size distribution does not appear to be very uniform, ranging from 5 to 13 microns. However, we did not account for the size distribution for this experiment. Therefore, we resorted to the manufacturer’s description as “micron-sized.”
In these experiments, the UV-sensitive liposomes were used only as a well-calibrated test system, to verify what part of the UV wavelength spectrum produces damage, and to check whether solutions of two ferric salts can protect these “model” liposomes from UV damage. Since liposomes were not supposed to sink or float in these experimental settings, the size discrimination was not crucial.
UV sources and measurement of UV intensity
One of the experimental aims was to determine the effect of the UV wavelength on the liposomal damage. Therefore, all the listed below UV sources were used to cover as wide as spectral range as possible, including the sunlight. For the latter, the cuvettes were filled with a solution and liposomes were exposed to bright sunlight for three and 6 minutes, following the same procedure as for the other UV sources.
We measured the UV intensity of all UV sources using two digital UV Light Meters, the UV512C with a spectrum range of 220–275 nm and the UV513AB with a spectrum range of 280–400 nm (General Tools, USA). The UV intensity of the used UV sources is shown below, with the UV512C Meter results marked (C), and the UV513AB Meter results marked (AB). The UV sources in this study and their spectral ranges, as provided by the manufacturers or literature, are listed below, as well as our measurements of UVC and UVAB intensities.
1. Spectroline Germicidal UV Sterilizing Lamp, 15 W (General Tools, United States), unfiltered 254, 9,500 μW/cm2 (C);
2. Analytik Jena 3 UV multi-wavelength lamp, 4 W (Thermo Fisher Scientific Inc., United States), 254 nm, 3,270 μW/cm2 (C);
3. Analytik Jena 3 UV multi-wavelength lamp, 4 W (Thermo Fisher Scientific Inc., United States), 302 nm, 7,451 μW/cm2 (AB);
4. Analytik Jena 3 UV multi-wavelength lamp, 4 W (Thermo Fisher Scientific Inc., United States), 365 nm, 6,215 μW/cm2 (AB);
5. Mercury HBO 50 W bulb (Zeiss, Germany), ∼315–400 nm (Growther, 2022), 14,327 μW/cm2 (AB);
6. CivilLaser deuterium bulb, 30W, with an optical UV-conducting quartz fiber interface output (Civil Laser Supplier, China), 190–400 nm, 32 μW/cm2 (C), 37 μW/cm2 (AB);
7. Solar UV (Madison, WI, 09-09-2024, 12:00), ∼315–400 nm (Herman et al., 1999; Seidlitz et al., 2001), 7,987 μW/cm2 (AB).
The UV lamps and the quartz fiber output of the deuterium bulb were placed 30 mm above the UVC Light Meter (Supplemental Material, Figure 1), while the Mercury HBO 50 W bulb was placed 100 mm above the UVAB Light Meter, as previously reported (Subbotin and Fiksel, 2023a). To ensure that changes in liposomes are not due to heating, the temperature of liposomes in wells was measured with a digital thermometer (Calibrated Scientific Thermistor with 1 mm probe, Cole-Parmer). A 6-min exposure to UV from a Spectroline lamp raised the temperature only by 1°C.
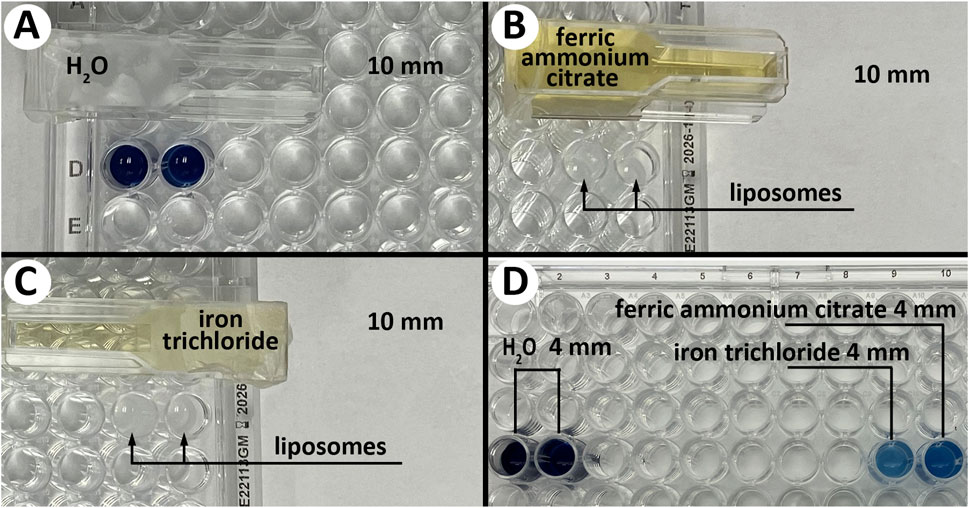
Figure 1. Visual assessment of color change during the liposome destruction by UV radiation. Liposome color is shown after 3 min of UV exposure (Spectroline Germicidal UV Sterilizing Lamp). (A) –completely destroyed liposomes (dark blue/black color) shielded by 10 mm distilled water. (B, C) - completely intact liposomes (original white color) shielded by 10 mm of ferric ammonium citrate or iron trichloride solutions. (D) (left) – completely destroyed liposomes (dark blue/black color) shielded by 4 mm distilled water, D (right) – partially destroyed liposomes (light/medium blue color) shielded by 4 mm of ferric ammonium citrate or iron trichloride solutions.
Assessment of the liposomal damage and protection
To characterize the color shift during liposome destruction, we used the light absorbance technique, which is widely utilized for studies of the chemical composition of various substances. In particular, the blue color of a substance is usually related to a preferable absorption of the red part of the visible spectrum. Accordingly, a strong absorption peak in the red area of spectra can quantitatively and qualitatively characterize the color change. In our experiment, the colorimetric shift was characterized by measuring the absorbance of the scanning light emitted by a BioTek Synergy Neo2 Hybrid Multimode Reader (Agilent, Santa Clara, CA, USA). The absorbance data was acquired by a Perkin Elmer Synergy Neo2 plate reader equipped with monochromator optics. We first conducted spectral scanning from 380 nm to 740 nm (5 nm steps) and indeed found 645 nm to be the wavelength of maximal light absorption. Subsequent pre- and post-UV exposures were done at that single wavelength. The measured absorption was closely correlated with the visual color change assessment.
Experimental setup
To study the liposome substance’s color shift, the samples were placed into wells of a 96-well plate and covered with disposable semi-micro UV cuvettes (GMBH, Germany) filled with (NH4)5 [Fe(C6H4O7)2] or FeCl3 solutions (2.5 g/L). For control experiments, the cuvettes were filled with distilled water. We also varied the thickness of the shielding solution. In particular, two thicknesses were used – 10 mm and 4 mm. The cuvettes hold 3.25 mL of fluid, are completely filled with solutions, and are hermetically sealed with a Parafilm-M sealing film. The cuvettes' material is transparent to UV, so the UV absorption was entirely due to the composition of the solutions. By design, the inside dimension of the upper part of the cuvette is 10 × 10 × 20 mm, and of the lower part 4 × 10 × 20 mm (Supplemental Material; Figures 2a,b, 3a,b), allowing complete coverage of a single well or two adjacent wells in a 96-well plate by 10 mm and 4 mm of the solutions. After that, the liposome samples were exposed to UV radiation, and the color change was assessed. In the experiments with liposome protection by ferric salt, the Spectroline UV Lamp (254) was used. In the experiments to study effects of different UV sources and various wavelengths on UV-sensitive liposomes, all seven UV sources listed above were used.
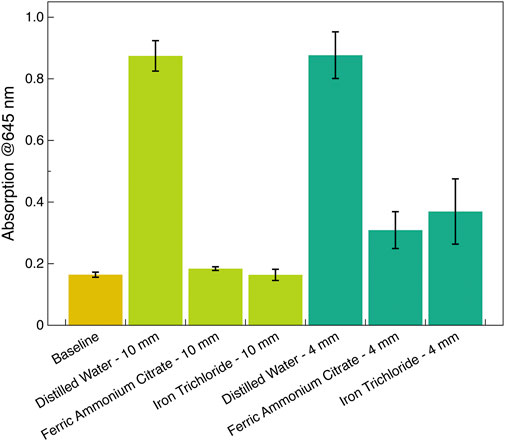
Figure 2. Quantification of the visual color assessment. The absorbance of the probing 645 nm after 3 min of UV exposure was acquired with a Perkin Elmer Synergy Neo2 plate reader equipped with monochromator optics. The baseline level was taken before UV exposure (Spectroline Germicidal UV Sterilizing Lamp). The data were averaged over three independent irradiation measurements, and the standard deviation is indicated by error bars.
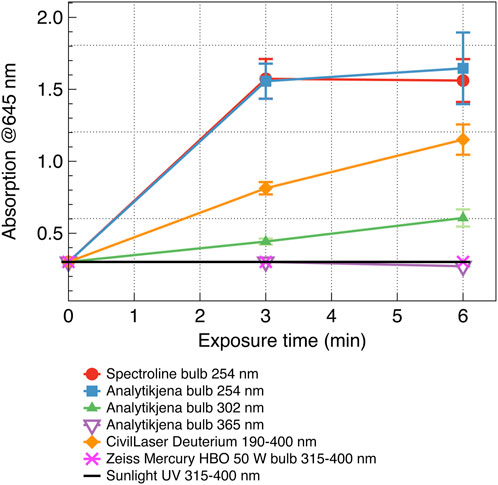
Figure 3. Temporal and spectral dependence of the probing 645 nm absorption by liposomes after radiation with different UV sources. Four/five-fold increased absorption of the scanning light at 645 nm corresponds to significant/complete destruction of the UV-radiated liposomes. The data were averaged over three consecutive measurements, and the standard deviation is indicated by error bars.
A single well or two adjacent wells in a 96-well plate filled with liposomes were analyzed with a Perkin Elmer Synergy Neo2 plate reader prior to UV radiation, then covered with shielding cuvettes holding certain solutions (iron salts or water) and exposed to UV and analyzed again. All experiments were repeated 3 times.
Results
Visual assessment of UV-protection of liposomes by iron trichloride and ferric ammonium citrate solutions
A visual assessment of the liposomes’ color change indicated complete destruction of liposomes shielded by 10 mm of distilled water—the color changed from white to dark blue/black. In comparison, liposomes shielded by a 10 mm solution of either iron trichloride or ferric ammonium citrate were completely intact—the color remained white—while a 4 mm solution of either iron salt showed only partial protection—the color changed to light/medium blue (Figure 1).
Quantitative assessment of UV-protection of liposomes by iron trichloride and ferric ammonium citrate solutions
Figure 2 quantifies the results of the visual color assessment. The liposomes were exposed to 254 nm UV radiation from the Spectroline 15 W UV lamp for this data. Using the experimental setup described earlier, the lamp was placed 30 mm above a 96-well plate containing liposomes. The plate was covered with UV-impermeable lead with openings above the designated wells. The lamp warm-up time was 1 min, and the radiation time was 3 min. The choice of irradiation time was based on the duration required for the absorption of the 645 nm probing light to reach a steady state. According to the liposome manufacturer, the dynamics of the liposome color change and the corresponding shifts in absorption are reliable indicators of liposome destruction. In particular, the radiation time required for liposome destruction (using a short-wavelength UV sterilization 20 W bulb, which is similar to the Spectroline Germicidal UV Sterilizing Lamp) is 3 min. Therefore, in experiments with liposome destruction/protection with the Spectroline Lamp, we used the same time duration of 3 min.
The probing 645 nm light absorption was the strongest for liposomes covered by cuvettes with distilled water, consistent with the visual assessment (dark blue/black color). On the contrary, liposomes covered by cuvettes with 10 mm of 2.5 g/L solutions of either (NH4)5 [Fe(C6H4O7)2] or FeCl3 exhibit low, baseline level absorption consisting of retaining of the original color (white). Protection from 4 mm-thick solutions was less pronounced, as indicated by the elevated absorption, consistent with the color change to light/medium blue (Figure 2).
The effects of different UV sources and various wavelengths on UV-sensitive liposomes
This section quantifies the effect of various UV wavelengths emitted by various UV sources. Since the output power of some other UV sources was less than that of the Spectroline Lamp, in the experiments to study the effect of various UV wavelengths emitted by various UV sources, we used a time duration of 3 and 6 min. The results show that at a 6-min exposure, the short-wave UV radiation (</ = 254 nm) destroyed all the liposomes regardless of the UV intensity (Spectroline, Analytikjena, and CivilLaser deuterium bulbs, UV intensity 9,500, 3,270, and 32 μW/cm2 correspondingly). The results also demonstrated that long-wave UV, either from filtered Sun UV or UV from bulb sources (Mercury HBO 50 W and Analytikjena bulbs 365 nm), have not affected the liposomes regardless of the UV intensity (Figure 3).
The UV absorbance spectra of the iron salt solutions
Finally, the wavelength dependence of the UV absorption was characterized for the two strongest absorbents used in this study–iron trichloride and ferric ammonium citrate. We used the Multicell Peltier Module G9889A for the Agilent Cary 3500 UV-Vis Spectrophotometer to measure the absorbance spectra. The high absorbance of the ferric salts in the short-wave range correlates with their excellent protection ability even in that highly destructive spectral range (Figure 4).
Summary and conclusions
Although this short communication does not intend to discuss in depth possible scenario of UV protection in evolution of early life, some comments on the subject should be mentioned. Notably, Carl Sagan and coauthors pointed out that short-wavelength, 240–290 nm, UV is lethal for pre- and biological organic matter, and suggested that the early life forms could be protected by absorbing layers of purines and pyrimidines (Ponnamperuma et al., 1963; Sagan, 1973). This suggestion was experimentally confirmed (Cleaves and Miller, 1998) and recently extended to nucleosides (Todd et al., 2021). On the other hand, Martin Rutten suggested that the natural composition of Archean water can constitute a protection for abiogenesis (Rutten, 1971). We are inclined to believe that a scenario in which the UV shielding of abiogenesis is facilitated by the salts naturally preexisted in Archean water is more feasible than a scenario in which the shielding presumed by complex organic molecules such as purines, pyrimidines, and nucleosides.
The results of this study using specially designed UV-sensitive liposomes demonstrated that 1) liposomes can be destroyed by short UV wavelengths (</ = 254 nm) after a radiation time as short as 6 min regardless of the UV intensities, 2) long-wave UV radiation (∼315–400 nm), including atmosphere-filtered Sun UV, do not destroy liposomes regardless of the UV intensities, and 3) a thin layer (10 mm) of ferric salt solutions, ferric ammonium citrate, and FeCl3, completely protect the liposomes from short-wave UV (</ = 254 nm) damage.
The experimental results confirmed our suggestion that the ferric ammonium citrate and iron trichloride solutions could constitute a protective media for life origin (Subbotin and Fiksel, 2023a) within the Lipid World scenario (Luisi et al., 1999; Segré et al., 2001). In addition, we showed that short-wave UV (220–275 nm) could also act as the selection force for the abiogenesis. It agrees with the idea suggested by A.I. Oparin and J.B.S Haldane that one of the main prerequisites for the origin of life on Earth is the reduced primitive Earth atmosphere, which allows the short-wave Sun UV to reach Archean water (Haldane, 1929; Oparin, 1924). The necessity of this condition for abiogenesis synthesis was also suggested by and experimentally confirmed by S.L. Miller and H.C. Urey (Miller, 1953; Miller and Urey, 1959). These results reinforce our hypothesis (Subbotin and Fiksel, 2023b) that the Sun UV radiation and gravity could be the major selection forces for the abiogenesis.
Data availability statement
The datasets presented in this article are not readily available because there are no such data. Requests to access the datasets should be directed to VS dnN1YmJvdGluQGFycm93aGVhZHBoYXJtYS5jb20=.
Author contributions
BT: Data curation, Formal Analysis, Investigation, Visualization, Writing – original draft, Writing – review and editing. KW: Investigation, Methodology, Visualization, Writing – original draft, Writing – review and editing. GF: Conceptualization, Formal Analysis, Methodology, Visualization, Writing – original draft, Writing – review and editing. VS: Conceptualization, Formal Analysis, Investigation, Supervision, Validation, Visualization, Writing – original draft, Writing – review and editing.
Funding
The author(s) declare that no financial support was received for the research and/or publication of this article.
Acknowledgments
The authors want to thank Drs. Sara Mir (Encapsula NanoSciences, Brentwood, TN), David Rozema (Empirico Inc., Madison, WI), Brian Davies, and Suping Zheng (Arrowhead Pharmaceuticals, Madison, WI) for valuable advice and technical support.
Conflict of interest
The authors declare that the research was conducted in the absence of any commercial or financial relationships that could be construed as a potential conflict of interest.
Generative AI statement
The author(s) declare that no Generative AI was used in the creation of this manuscript.
Publisher’s note
All claims expressed in this article are solely those of the authors and do not necessarily represent those of their affiliated organizations, or those of the publisher, the editors and the reviewers. Any product that may be evaluated in this article, or claim that may be made by its manufacturer, is not guaranteed or endorsed by the publisher.
Supplementary material
The Supplementary Material for this article can be found online at: https://www.frontiersin.org/articles/10.3389/fspas.2025.1566396/full#supplementary-material
References
Ahn, D. J., Lee, S., and Kim, J. M. (2009). Rational design of conjugated polymer supramolecules with tunable colorimetric responses. Adv. Funct. Mater. 19, 1483–1496. doi:10.1002/adfm.200801074
Breslow, R., Levine, M., and Cheng, Z.-L. (2010). Imitating prebiotic homochirality on earth. Orig. Life Evol. Biospheres 40, 11–26. doi:10.1007/s11084-009-9179-0
Cleaves, H. J., and Miller, S. L. (1998). Oceanic protection of prebiotic organic compounds from UV radiation. Proc. Natl. Acad. Sci. 95, 7260–7263. doi:10.1073/pnas.95.13.7260
Gómez, F., Aguilera, A., and Amils, R. (2007). Soluble ferric iron as an effective protective agent against UV radiation: implications for early life. Icarus 191, 352–359. doi:10.1016/j.icarus.2007.04.008
Growther, J. (2022). UV microscopy – zeiss 50w HBO lightsource modification. JMC Scientific Consulting Ltd.
Handschuh, G., and Orgel, L. (1973). Struvite and prebiotic phosphorylation. Science 179, 483–484. doi:10.1126/science.179.4072.483
Hardy, M. D., Yang, J., Selimkhanov, J., Cole, C. M., Tsimring, L. S., and Devaraj, N. K. (2015). Self-reproducing catalyst drives repeated phospholipid synthesis and membrane growth. Proc. Natl. Acad. Sci. 112, 8187–8192. doi:10.1073/pnas.1506704112
Herman, J., Krotkov, N., Celarier, E., Larko, D., and Labow, G. (1999). Distribution of UV radiation at the Earth's surface from TOMS-measured UV-backscattered radiances. J. Geophys. Res. Atmos. 104, 12059–12076. doi:10.1029/1999jd900062
Keefe, A. D., and Miller, S. L. (1996). Was ferrocyanide a prebiotic reagent? Orig. Life Evol. Biosphere 26, 111–129. doi:10.1007/bf01809851
Kim, H. J., Furukawa, Y., Kakegawa, T., Bita, A., Scorei, R., and Benner, S. A. (2016). Evaporite borate-containing mineral ensembles make phosphate available and regiospecifically phosphorylate ribonucleosides: borate as a multifaceted problem solver in prebiotic chemistry. Angew. Chem. 128, 16048–16052. doi:10.1002/ange.201608001
Liu, D., Wang, S., Xu, S., and Liu, H. (2017). Photocontrollable intermittent release of doxorubicin hydrochloride from liposomes embedded by azobenzene-contained glycolipid. Langmuir 33, 1004–1012. doi:10.1021/acs.langmuir.6b03051
Luisi, P. L., Walde, P., and Oberholzer, T. (1999). Lipid vesicles as possible intermediates in the origin of life. Curr. Opin. colloid & interface Sci. 4, 33–39. doi:10.1016/s1359-0294(99)00012-6
Miller, S. L. (1953). A production of amino acids under possible primitive earth conditions. Science 117, 528–529. doi:10.1126/science.117.3046.528
Miller, S. L., and Urey, H. C. (1959). Organic compound synthesis on the primitive earth. Science 130, 245–251. doi:10.1126/science.130.3370.245
Mir, S. (2024). Personal communication with lead scientist Dr. Sara Mir, the Encapsula NanoSciences Company.
Patel, B. H., Percivalle, C., Ritson, D. J., Duffy, C. D., and Sutherland, J. D. (2015). Common origins of RNA, protein and lipid precursors in a cyanosulfidic protometabolism. Nat. Chem. 7, 301–307. doi:10.1038/nchem.2202
Ponnamperuma, C., Sagan, C., and Mariner, R. (1963). Synthesis of adenosine triphosphate under possible primitive earth conditions. Nature 199, 222–226. doi:10.1038/199222a0
Sagan, C. (1973). Ultraviolet selection pressure on the earliest organisms. J. Theor. Biol. 39, 195–200. doi:10.1016/0022-5193(73)90216-6
Segré, D., Ben-Eli, D., Deamer, D. W., and Lancet, D. (2001). The lipid world. Orig. Life Evol. Biosphere 31, 119–145. doi:10.1023/a:1006746807104
Seidlitz, H. K., Thiel, S., Krins, A., and Mayer, H. (2001). Solar radiation at the Earth's surface. Compr. Ser. photosciences 3, 705–738. doi:10.1016/s1568-461x(01)80071-5
Sihorwala, A. Z., Lin, A. J., Stachowiak, J. C., and Belardi, B. (2023). Light-activated assembly of connexon nanopores in synthetic cells. J. Am. Chem. Soc. 145, 3561–3568. doi:10.1021/jacs.2c12491
Sproul, G. (2015). Abiogenic syntheses of lipoamino acids and lipopeptides and their prebiotic significance. Orig. Life Evol. Biospheres 45, 427–437. doi:10.1007/s11084-015-9451-4
Subbotin, V., and Fiksel, G. (2023a). Aquatic ferrous solutions of prebiotic mineral salts as strong UV Protectants and possible loci of life origin. Astrobiology 23, 741–745. doi:10.1089/ast.2023.0011
Subbotin, V., and Fiksel, G. (2023b). Exploring the Lipid World hypothesis: a novel scenario of self-sustained Darwinian evolution of the liposomes. Astrobiology 23, 344–357. doi:10.1089/ast.2021.0161
Keywords: origin of life, abiogenesis, adaptive traits and selective forces, solar UV, gravity
Citation: Turner B, Wu K, Fiksel G and Subbotin V (2025) Protection of liposomes by ferric salts against the UV damage and its implications for the origin of life. Front. Astron. Space Sci. 12:1566396. doi: 10.3389/fspas.2025.1566396
Received: 24 January 2025; Accepted: 09 April 2025;
Published: 01 May 2025.
Edited by:
Pasquale Stano, University of Salento, ItalyReviewed by:
Mauro S. Ferreira Santos, NASA Jet Propulsion Laboratory (JPL), United StatesMaría Colín-García, National Autonomous University of Mexico, Mexico
Copyright © 2025 Turner, Wu, Fiksel and Subbotin. This is an open-access article distributed under the terms of the Creative Commons Attribution License (CC BY). The use, distribution or reproduction in other forums is permitted, provided the original author(s) and the copyright owner(s) are credited and that the original publication in this journal is cited, in accordance with accepted academic practice. No use, distribution or reproduction is permitted which does not comply with these terms.
*Correspondence: Vladimir Subbotin, dnN1YmJvdGluQGFycm93aGVhZHBoYXJtYS5jb20=
Parts of this study were presented at: NASA/AGU Astrobiology Science Conference in Providence, RI on 5–10 May 2024, Elighting poster: B. Turner, G. Fiksel, and V. Subbotin. “Sunshine in Lipid world: Ferrous Salts of Archean Water as UV Protectants of Life Origin”.