- 1Department of Nutrition and Food Science, Texas A&M University, College Station, TX, United States
- 2Department of Horticultural Sciences, Texas A&M University, College Station, TX, United States
- 3Department of Animal Science, Texas A&M University, College Station, TX, United States
- 4Artie McFerrin Department of Chemical Engineering, Texas A&M University, College Station, TX, United States
Postharvest cross-contamination of produce with bacterial human pathogens reduces the usefulness of produce as a source of wholesome human food. The application of nanoencapsulated natural antimicrobials, including essential oil components of plants, can help protect produce safety by decontaminating produce from microbial pathogens. The primary objective of this research was to determine the efficacy of antimicrobial interventions on pathogen-inoculated spinach samples stored refrigerated (5°C) for up to 10 days, or refrigerated (5°C) for 5 days with subsequent moderate (15°C) or severe (25°C) temperature abuse thereafter until a total of 10 days had elapsed. The secondary objective of the study was to determine the capacity of antimicrobial interventions to inhibit and/or reduce pathogen numbers on spinach when inoculation followed intervention treatment, simulating cross-contamination of spinach following postharvest sanitizing of spinach. Rinsed spinach was portioned into samples and inoculated with cocktailed antibiotic-resistant pathogens to 6.1 ± 0.1 log10 CFU/cm2 for each pathogen. Treatments of geraniol-loaded polymeric nanoparticles (0.5 wt.% geraniol), unencapsulated geraniol (0.5 wt.%), and 200 ppm free and available chlorine (FAC; pH 7.0) were applied to pathogens-inoculated or non-inoculated spinach samples. Following treatment, samples were collected for determining pathogen survival or were covered and held to determine changes in microbial populations (inoculated pathogens and naturally contaminating microbial hygiene indicators) during posttreatment simulated refrigerated and/or temperature-abuse storage. Changes in pathogen numbers were assessed periodically over 10 days for refrigerated (5°C), moderately (15°C), or severely (25°C) temperature-abused spinach. Immediately following treatment by FAC, unencapsulated or nanoparticle-entrapped geraniol, Escherichia coli O157:H7 and Salmonella counts on spinach ranged from 1.5 to 6.1 log10 CFU/cm2. Geraniol-loaded nanoparticles reduced pathogens to non-detectable numbers (detection limit: 0.5 log10 CFU/cm2) on refrigerated and moderately temperature-abused samples (15°C) within 7 days of storage posttreatment, and after 10 days of severe thermo-abuse storage (25°C). Conversely, FAC treatment did not reduce pathogens to non-detectable numbers for refrigerated spinach, and was not observed to inhibit pathogen growth in temperature-abused samples. Application of encapsulated geraniol can reduce pathogens on spinach surfaces, preventing their transmission to consumers, aiding the retention of produce wholesomeness, and utility for human consumption and nutrition.
Introduction
Microbial pathogens have been associated with a variety of produce-borne human disease outbreaks, with an apparent increase in the frequency of outbreaks in recent years (CDC, 2012a,b; Gould et al., 2013; Slayton et al., 2013; Jung et al., 2014; Angelo et al., 2015). Painter et al. (2013) reported leafy green produce commodities were the most frequently implicated pathogen transmission vehicles in US human foodborne disease cases, as well as the food vehicle type second most frequently linked to hospitalizations resulting from foodborne disease. The US Food and Drug Administration (FDA) reported microbial pathogens may cross-contaminate fresh produce during production and/or harvest, as well as during postharvest handling and packing. There is a need for effective antimicrobial interventions to decontaminate produce from pathogens in order to protect consumer safety, commodity wholesomeness, and to support the sustainability of production of food crops (FDA, 2008; Nguyen-The et al., 2016).
The enteric bacterial pathogens Escherichia coli O157:H7 and Salmonella enterica have previously been identified to cause human disease following consumption of pathogen-contaminated leafy green vegetables. Consumption of E. coli O157:H7-contaminated spinach grown in the Salinas Valley region of California in 2006 resulted in over 200 cases of human disease across 26 US states (CDC, 2006; Lynch et al., 2009). Recent outbreaks of human disease have also resulted from consumption of pathogen-contaminated lettuce and mixed salad vegetables (Painter et al., 2013). Herman et al. (2015) reported Salmonella enterica was the disease-causing agent in 11% of US human disease outbreaks attributable to consumption of contaminated leafy vegetables occurring from 1973 to 2012. While proper refrigeration is known to inhibit the growth of these pathogens on several foods, multiple research groups have reported the capability of E. coli O157:H7 and Salmonella to replicate on both intact and damaged leafy green vegetables, indicating a need for advanced decontamination technologies to reduce the risk of microbial pathogen transmission to consumers (Roever, 1998; Lang et al., 2004; Kroupitski et al., 2009; Khalil and Frank, 2010; Neal et al., 2012; Sant’Ana et al., 2012).
Plant-derived essential oil components (EOCs) are small, often volatile, compounds possessing antioxidant, and in some instances, antimicrobial properties. This makes them attractive candidates for use in food safety protection (Davidson et al., 2013). Due in part to their hydrophobicity and volatility, their utility in aqueous foods or in produce washing systems is limited. Nevertheless, encapsulation of EOCs with water-dispersible compounds has been reported by multiple researchers to assist in delivering plant EOCs to microbes in liquid and solid foods (Gaysinsky et al., 2007; Zhang et al., 2008, 2014; Donsì et al., 2011; Gomes et al., 2011; Casco et al., 2015). Our research group recently developed and characterized polymeric nanoparticles formed from the tri-block copolymer Pluronic® F-127 loaded with the rose oil component geraniol at 0.2 wt.%; geraniol-loaded polymeric nanoparticles (GPNs) were inhibitory to E. coli O157:H7 and Salmonella growth in liquid medium incubated at 35°C. Geraniol is identified by the FDA as generally recognized as safe for use in foods (FDA, 2016a,b). Additionally, Pluronic® F-127 has gained FDA approval for biomedical applications (FDA, 2011). Nanoparticles possessed a sustained release profile, with enhanced delivery of the hydrophobic EOC into the outer membranes of targeted pathogens versus unencapsulated geraniol (Yegin et al., 2016).
The potential for fresh and fresh-cut produce, including leafy vegetables, to be cross-contaminated with human microbial pathogens has spurred much research into antimicrobial interventions designed to reduce pathogens on produce when applied following harvest. The US FDA (2008) previously identified methods for minimizing cross-contamination during postharvest handling, including application of produce-contacting sanitizers. Nevertheless, the capacity of antimicrobial interventions to inhibit pathogens cross-contaminating produce surfaces following antimicrobial intervention application remains unknown due in large part to a lack of research.
The primary objective of this research was to determine the antimicrobial activity of interventions on pathogen-inoculated spinach stored refrigerated (5°C) for up to 10 days, or refrigerated (5°C) for 5 days with subsequent moderate (15°C) or severe (25°C) temperature abuse thereafter until a total of 10 days had elapsed. The testing of moderate or severe temperature abuse following 5 days of refrigerated storage was implemented to simulate potential abuse holding during retail display for spinach and the ability of antimicrobial treatments to inhibit pathogen growth therein. The secondary objective of the study was to determine the capacity of antimicrobial interventions to inhibit and/or reduce pathogen numbers on spinach when inoculation followed intervention treatment, simulating cross-contamination of spinach following postharvest sanitizing of spinach. Finally, the impacts of antimicrobial treatments on populations of differing microbial hygiene indicator groupings [aerobic bacteria, lactic acid bacteria (LAB), coliforms] were determined for spinach treated and then refrigerated (5°C), or initially refrigerated and then moved to moderate (15°C) or severe (25°C) temperature abuse conditions.
Materials and Methods
Preparation of GPNs
Geraniol (trans-3,7-dimethyl-2,6-octadien-1-ol; > 96.0%; CAS #1-6-24-1; TCl America, Portland, OR, USA) and the amphiphilic tri-block copolymer Pluronic® F-127 (CAS #9003-11-6; Sigma-Aldrich Co., St. Louis, MO, USA) were dissolved in tetrahydrofuran (THF; CAS #109-99-9; Sigma-Aldrich Co.), each to 0.5 wt.%. Solutions were prepared in order to account for an expected 57% encapsulation efficiency for GPNs, previously reported for identically made nanoparticles (Yegin et al., 2016). This concentration was chosen based on data demonstrating pathogen minimum inhibitory concentration (MIC) and minimum bactericidal concentration (Yegin et al., 2016). Following mixing, the reaction vessel was placed in a fume hood to evaporate THF off for 7.0 h, after which GPNs were suspended in one volume of sterile distilled water.
Preparation of Microorganisms for Inoculation onto Spinach Samples
Salmonella enterica serovar Typhimurium LT2, American Type Culture Collection (ATCC; Manassas, VA, USA) #700720, and E. coli O157:H7 ATCC #700728, both resistant to rifampicin (100.0 µg/mL; RifR) were obtained from the Food Microbiology Laboratory culture collection (Department of Animal Science, Texas A&M University, College Station, TX, USA). Isolates were revived from cryo-storage by previously published methods (Perez et al., 2012). Briefly, working cultures were obtained by aseptically transferring loop-fulls of cultures (~10.0 μL) from slants of tryptic soy agar (TSA; Becton, Dickinson and Co., Sparks, MD, USA) into sterile 9.0 mL volumes of tryptic soy broth (TSB; Becton, Dickinson and Co.), followed by incubation for 24 h at 35°C. A second passage was completed in identical fashion in order to fully revive cultures for inoculum preparation.
A pathogen inoculum fluid was prepared by first centrifuging pathogen isolates for 15 min at 22°C, 2191 × g. Resulting supernatants were carefully poured off; pellets were then vortexed in 10 mL sterile phosphate-buffered saline (PBS; Thermo-Fisher Scientific, Waltham, MA, USA) to wash bacterial cells. Centrifugation and washing procedures were completed a total of three times in identical fashion. After the final washing, both microbes were blended together and serially diluted in 0.1% peptone buffer (Becton, Dickinson and Co.) to achieve an inoculum of approximately 8.0 log10 CFU/mL. Inoculum fluid concentrations of E. coli O157:H7 and Salmonella were determined through preparation of serial dilutions in 0.1% (w/v) peptone and plating on surfaces of lactose-sulfite-phenol red-rifampicin (LSPR) agar supplemented with 100.0 µg/mL rifampicin-loaded Petri dishes (Perez et al., 2012; Ruengvisesh et al., 2015). Colonies of pathogens were differentially counted after 24 h incubation at 35°C. Salmonella enterica colonies are distinguished from E. coli O157:H7 colonies through appearance of blackened centers resulting from sulfite reduction, as well as through E. coli fermentation of lactose, producing yellow-tinted haloes (Castillo et al., 1998).
Preparation and Inoculation of Spinach Samples with Pathogens
Spinach was purchased from a College Station, TX, fresh produce retailer and returned to the Food Microbiology Laboratory. Spinach leaves were rinsed in sterile distilled water, allowed to drip dry on sterilized grills for approximately 1.0 h, and then portioned into 10 cm2 circular pieces through use of flame-sterilized boring device and forceps. Spinach pieces were prepared to determine antimicrobial treatment efficacy on physically damaged spinach, a risk factor for pathogen growth (Harris et al., 2003; Koukkidis et al., 2017). Each sample consisted of three circular pieces, giving a total 30 cm2 surface area for inoculation and treatment. Samples were spot-inoculated by applying ten 10 µL volumes of inoculum onto adaxial surfaces of spinach leaf samples (abaxial sides were placed face down and not inoculated) by pipette. Samples were then held at 25°C for 1.0 h to allow pathogen inoculum attachment to spinach surfaces prior to antimicrobial treatment.
Antimicrobial Treatments and Posttreatment Handling of Spinach
Efficacy of Antimicrobial Interventions on Spinach Held at Refrigeration and Spinach Held at Refrigeration with Subsequent Moderate or Severe Temperature Abuse
Antimicrobial treatments were applied to inoculated spinach by submerging in intervention treatment-containing sterile distilled water for 2 min, in order to simulate immersion washing postharvest. Treatments were: GPNs containing 0.5 wt.% geraniol, unencapsulated geraniol (0.5 wt.%) in sterile milli-Q water, 200 ppm free/available chlorine [FAC; adjusted to pH 7.0 by use of 1.0 N HCl; FAC content was confirmed by free/total chlorine test strip (Hach Company, Loveland, CO, USA)], and an untreated inoculated control. Chlorine treatment was prepared by diluting 6.25% NaOCl (Clorox Co., Oakland, CA, USA) in sterile distilled water prior to pH adjustment. Following antimicrobial treatment, samples destined for storage were removed from treatment, allowed to drip dry for 5–15 min on a single use sanitary paper towel, and randomly assigned to a storage period (3, 5, 7, or 10 days) and incubation schedule (refrigeration, refrigeration + moderate abuse, refrigeration + severe abuse), covered and stored aerobically in a refrigerated (5°C) incubator. Remaining samples were aseptically loaded into stomacher pouches and diluted in 100 mL 0.1% (w/v) peptone (Becton, Dickinson and Co.) diluent. Decimal dilutions were prepared in 0.1% peptone buffer and surviving pathogens were spread on surfaces of LSPR agar. Inoculated Petri dishes were incubated and differentially counted as described above (see Preparation of Microorganisms for Inoculation onto Spinach Samples).
For those samples assigned to either refrigeration + moderate abuse or refrigeration + severe abuse, samples were moved after 5 days’ refrigerated storage to either 15 or 25°C incubation, respectively. These samples were not subjected to microbiological analysis prior to the day 7 point, as all samples were prepared using the same inoculum fluid and methods. Preliminary experiments conducted prior to initiation of experimental procedures demonstrated uniform inoculum application across samples was achieved using the procedure described (see Preparation and Inoculation of Spinach Samples with Pathogens) (data not shown). Experimental samples were collected at 7 and 10 days of storage, and surviving pathogens were quantified using the same procedure described in Section “Preparation of Microorganisms for Inoculation onto Spinach Samples.”
Ability of Antimicrobial Treatments to Inhibit Pathogen Growth on Spinach Samples When Inoculation Follows Treatment Application for Samples Held at Refrigeration or at Refrigeration with Subsequent Moderate or Severe Temperature Abuse
A separate experiment was designed to determine the potential for antimicrobial treatments to inhibit pathogen growth on sample surfaces when decontamination treatment occurred prior to pathogen inoculation. Samples were prepared and treated with antimicrobial interventions in identical fashion as described in Section “Efficacy of Antimicrobial Interventions on Spinach Held at Refrigeration and Spinach Held at Refrigeration with Subsequent Moderate or Severe Temperature Abuse.” Samples were allowed to rest for 5 min following treatment, randomly assigned to a storage period (3, 5, 7, or 10 days) and incubation process (refrigeration, refrigeration + moderate abuse, refrigeration + severe abuse). They were covered and stored aerobically in a refrigerated (5°C) incubator, and held undisturbed for three days prior to inoculation. Inoculum fluid was prepared and applied as described in Sections “Preparation of Microorganisms for Inoculation onto Spinach Samples” and “Preparation and Inoculation of Spinach Samples with Pathogens,” except that it was diluted to a target of 7.0 log10 CFU/mL. Preliminary experiments indicated an approximate 1.0 log10 CFU/cm2 decline in numbers of pathogens (E. coli O157:H7, Salmonella) in inoculum occurring between days 0 and 3 (data not shown). This, in combination with the suggestion that postharvest cross-contamination of produce would not likely occur to levels as high as those occurring in production fields even in instances of gross postharvest sanitation failure, led researchers to employ a lower inoculated pathogen count (Harris et al., 2003). Following inoculation, E. coli O157:H7 and S. Typhimurium colonies were counted on LSPR agar surfaces at 5, 7, and 10 days of storage at 5°C.
In similar fashion to experiments detailed in Section “Efficacy of Antimicrobial Interventions on Spinach Held at Refrigeration and Spinach Held at Refrigeration with Subsequent Moderate or Severe Temperature Abuse,” two additional sets of samples were prepared and treated in identical fashion, then inoculated with pathogens after 3 days’ refrigerated storage (see Preparation and Inoculation of Spinach Samples with Pathogens and Efficacy of Antimicrobial Interventions on Spinach Held at Refrigeration and Spinach Held at Refrigeration with Subsequent Moderate or Severe Temperature Abuse). After inoculation, these samples were returned to refrigeration (5°C) for an additional two days prior to being moved to either 15°C (refrigeration + moderate abuse) or 25°C (refrigeration + severe abuse) incubation. Samples were retrieved for pathogen survival determination at 7 and 10 days of storage, using procedures described in Section “Preparation of Microorganisms for Inoculation onto Spinach Samples.”
Ability of Antimicrobial Treatments to Reduce Numbers of Microbial Hygiene Indicators on Non-Inoculated Spinach Held at Refrigeration or at Refrigeration with Subsequent Moderate or Severe Temperature Abuse
In addition to experiments using pathogen-inoculated spinach, a set of samples was prepared in identical fashion as in Section “Preparation and Inoculation of Spinach Samples with Pathogens,” except without pathogen inoculation. Spinach samples were treated by antimicrobial intervention treatments in identical fashion as procedures described in Section “Efficacy of Antimicrobial Interventions on Spinach Held at Refrigeration and Spinach Held at Refrigeration with Subsequent Moderate or Severe Temperature Abuse.” Following treatment, samples were placed at 5°C and evaluated for numbers of aerobic bacteria, LAB, and coliforms at 0, 3, 5, 7, and 10 days of storage. In addition to refrigerated samples, two sets of samples were prepared and treated by antimicrobial interventions. After 5 days’ refrigerated storage, these were moved to either 15°C (refrigeration + moderate abuse) or 25°C (refrigeration + severe abuse). Samples from these sets were collected for survivor determination at 7 and 10 days of total storage. Decimal dilutions of spinach samples were prepared in 0.1% (w/v) peptone diluent. Aerobic bacteria colonies were counted on 3 M™ Petrifilm™ Aerobic Count Plate films following incubation at 35°C for 48 h. Lactic acid bacteria were counted on 3 M™ Petrifilm™ Aerobic Count Plate using decimal dilutions of spinach samples prepared in de Man, Rogosa, and Sharpe Lactobacilli (MRS) broth (Becton, Dickinson and Co.) following 48 h anaerobic incubation at 35°C. Finally, coliforms were enumerated on 3 M™ Petrifilm™ E. coli/Coliform Count Plates following incubation (48 h, 35°C). Additionally, photographs were collected from treated samples subjected to handling conditions described for determination of changes in spinach appearance as a function of treatment and handling.
Experimental Replication and Data Analysis
All experiments were replicated three times each in identical fashion (N = 3). All data were transformed to log10 CFU/cm2 prior to statistical analysis. Significant differences between means of differing treatments were identified by analysis of variance (AOV) and separated at p = 0.05 by Tukey’s Honestly Significant Differences (HSD) multiple comparisons test via JMP® Pro v12.2.0 (SAS Institute, Inc., Cary, NC, USA). Main fixed effects were days of storage, antimicrobial intervention treatment, and the interaction of treatment by storage days. Separate analyses were conducted for each pathogen, and for each incubation temperature since independent pathogen or indicator counts were not collected for samples subjected to abuse storage at days 0, 3, or 5. Depending on the experiment, mean counts of pathogens at days 7 and 10 from samples stored under moderate or severe temperature abuse were not compared to means of pathogen counts from days 0, 3, or 5 of refrigerated storage. This is because temperature abuse conditions were not introduced prior to 3 days of refrigerated sample holding. The raw data supporting the conclusions of this manuscript will be made available by the authors, without undue reservation, to any qualified researcher.
Results
Reduction of Pathogens by Antimicrobial Interventions on Spinach Samples Held at Refrigeration and on Samples Held at Refrigeration with Subsequent Moderate or Severe Temperature Abuse
Inoculation of spinach with bacterial pathogens resulted in 6.1 ± 0.1 log10 CFU/cm2 E. coli O157:H7 and 6.1 ± 0.1 log10 CFU/cm2 S. Typhimurium LT2 attaching to spinach surfaces prior to treatment application. Analysis of data from objective 1 experiments (see Efficacy of Antimicrobial Interventions on Spinach Held at Refrigeration and Spinach Held at Refrigeration with Subsequent Moderate or Severe Temperature Abuse) indicated that although main fixed effects (storage duration, antimicrobial treatment) were highly significant (p < 0.01), the interaction of these effects did not significantly influence pathogen survival on treated, refrigerated spinach (Table 1). Antimicrobial treatments produced rapid declines in pathogen numbers for both E. coli O157:H7 and S. Typhimurium on day 0; counts of both pathogens continued to decrease over 10 days of storage at 5°C. Geraniol-loaded nanoparticles produced the greatest numerical reductions in both pathogens (non-detection throughout the storage period) of the applied treatments, although pathogen counts following treatment did not statistically differ from those on UG-treated sample surfaces. Unencapsulated geraniol produced greater reductions in pathogen numbers vs. FAC, and as expected, numbers of pathogens on control (untreated) samples remained the highest for all treatments through the experiment.
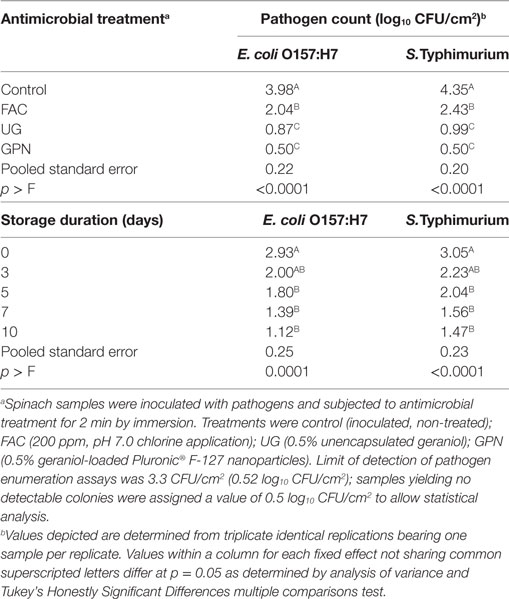
Table 1. Least squares means of Escherichia coli O157:H7 and Salmonella Typhimurium LT2 by antimicrobial treatment and storage duration on spinach held for 10 days at 5°C.
For all treatments, including the control, maintenance of refrigeration (5°C) on inoculated spinach resulted in pathogen numbers declining during the experimental storage period. After the application of antimicrobial treatments on day 0, E. coli O157:H7 numbers were 1.5 ± 1.6 and 3.7 ± 1.5 log10 CFU/cm2 for unencapsulated geraniol-treated and FAC-treated spinach, respectively (data not shown). In the case of E. coli O157:H7 treated by unencapsulated geraniol, 2/3 samples yielded non-detectable counts of the pathogen following treatment on day 0, while sample 3 yielded a count of 3.3 log10 CFU/cm2, failing to produce rapid pathogen inactivation. Similar results were obtained for S. Typhimurium LT2 on surfaces of spinach, where 2.0 ± 1.4 and 3.5 ± 1.5 log10 CFU/cm2 for unencapsulated geraniol-treated and FAC-treated samples, respectively, were obtained posttreatment (data not shown). There was again significant variability in the observed efficacy of the interventions to reduce Salmonella numbers postapplication. Nanoencapsulated geraniol produced rapid declines to non-detectable counts of both pathogens; combined with refrigeration GPNs inhibited pathogens over 10 days (Table 1). Pathogens on control, FAC, and UG samples also declined over the 10-day storage period. At day 10, populations of both pathogens on the surfaces of spinach treated with unencapsulated geraniol were, as with GPN-treated samples, below the assay detection limit (0.5 log10 CFU/cm2).
Similar to results obtained on refrigerated samples, statistical analysis of pathogen-specific mean counts on antimicrobial-treated samples at 15°C indicated both storage days and antimicrobial treatment both significantly influenced pathogen survival and growth (Table 2). Nevertheless, only for S. Typhimurium was the interaction of storage day × treatment statistically significant with respect to pathogen survival (p = 0.045). After moving treated spinach samples from 5 to 15°C, simulating moderate temperature abuse posthandling/packing, numbers of E. coli O157:H7 and S. Typhimurium at day 7 were highest on controls, and in descending order, lower on FAC, UG, and GPN-treated spinach samples. Likewise, means of pathogen counts declined from day 7 to day 10 (Table 2). In the case of Salmonella, while numbers on both control- and FAC-treated spinach declined from days 7 to 10 at 15°C, pathogen counts remained at non-detectable levels for both UG and GPN-treated spinach (Table 2). This was not the case for E. coli O157:H7; pathogen counts declined from days 7 to 10 but were nonetheless detectable at day 7 for UG-treated spinach (Table 2).
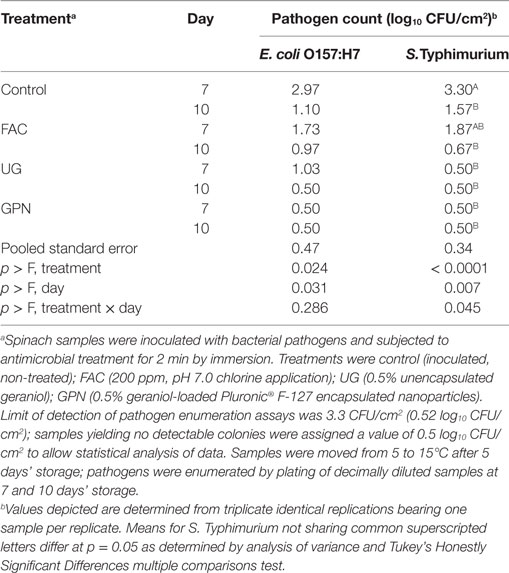
Table 2. Least squares means of Escherichia coli O157:H7 and Salmonella Typhimurium LT2 by antimicrobial treatment × storage day on spinach at 15°C.
For severely temperature-abused spinach, only the antimicrobial treatment effect was statistically significant with respect to impacts on pathogen numbers (p < 0.05) (Table 3). As was observed with spinach held under the other incubation conditions, control samples bore the highest numbers of both pathogens, followed by FAC, UG, and GPN treatments, respectively. The GPNs produced reductions of both pathogens to non-detectable numbers throughout the experimental period, demonstrating pathogen inhibition by the encapsulated plant oil without requirement for continued refrigeration (Table 3). Conversely, counts of E. coli O157:H7 and Salmonella Typhimurium-inoculated controls did not differ from those on FAC-treated samples. Numbers of pathogens for UG and GPN-treated spinach samples did not differ from one another (Table 3). Given the loss of pathogen detection for GPN- versus UG-treated samples, failure to identify statistically significant differences between means of pathogens likely arose from variability in sample data for the UG samples. This would indicate reduced reliability of pathogen reduction by UG treatment for food safety control/protection.
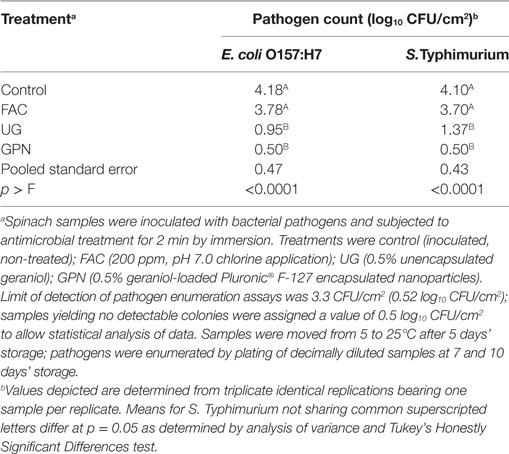
Table 3. Least squares means of Escherichia coli O157:H7 and Salmonella Typhimurium LT2 by antimicrobial treatment on spinach at 25°C.
Antimicrobial Treatments Applied Prior to Pathogen Inoculation on Spinach Samples Held at Refrigeration with or without Subsequent Moderate or Severe Temperature Abuse
Experiments were completed to determine whether antimicrobial treatments on samples would inhibit pathogens inoculated onto samples following treatment and 3 days of refrigerated storage, simulating postwashing cross-contamination (see Ability of Antimicrobial Treatments to Inhibit Pathogen Growth on Spinach Samples When Inoculation Follows Treatment Application for Samples Held at Refrigeration or at Refrigeration with Subsequent Moderate or Severe Temperature Abuse). The impacts of antimicrobial treatment on spinach on numbers of E. coli O157:H7 and S. Typhimurium inoculated three days posttreatment and stored at 5°C are depicted in Table 4. Similar to experiments in which pathogens were inoculated prior to spinach treatment (see Reduction of Pathogens by Antimicrobial Interventions on Spinach Samples Held at Refrigeration and on Samples Held at Refrigeration with Subsequent Moderate or Severe Temperature Abuse), numbers of both pathogens were highest on untreated spinach (controls), followed by FAC, UG, and GPN treatments. For E. coli O157:H7, UG and GPN treatments produced rapid declines in numbers of surviving pathogens versus FAC treatment, though UG-treated E. coli O157:H7 counts did not differ from those on FAC or control samples. With respect to antimicrobial treatment of spinach samples and impacts on S. Typhimurium applied to samples posttreatment, trends in pathogen declines during refrigerated storage were comparable to those observed for E. coli O157:H7 (Table 4). For both UG and GPN-treated spinach the pathogen was not detected by plating by day 7 (Table 4). Declines in Salmonella Typhimurium numbers on control and FAC-treated spinach mirrored those for E. coli O157:H7, with control spinach Salmonella counts decreasing by ~2.6 log10 CFU/cm2 from day 3 to day 10 (~3.0 log10 CFU/cm2 decline for E. coli O157:H7 in like samples). For both pathogens, antimicrobial treatments incorporating the plant EOC out-performed FAC treatment at producing longer lasting pathogen inhibition, in combination with the maintenance of proper refrigeration conditions (Table 4).
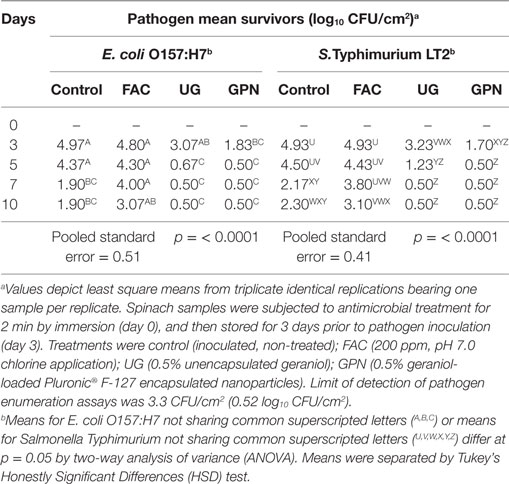
Table 4. Least squares means of Escherichia coli O157:H7 and Salmonella Typhimurium LT2 by antimicrobial treatment × storage period inoculated onto spinach posttreatment and 3 days’ storage at 5°C.
For spinach subjected to antimicrobial treatment, inoculated with pathogens after 3 days of refrigeration, and finally moved to 15°C after 5 days of storage, numbers of pathogens were nearly identical when compared for each treatment (Table 5). Pathogens were unable to recover from treatment by GPNs, with numbers of both E. coli O157:H7 and Salmonella remaining below the limit of detection throughout the experimental period. There were numerical increases in numbers of pathogens exposed to free geraniol following transfer to moderate temperature abuse condition versus those for pathogens kept at refrigeration for the entire experimental period. Surprisingly, pathogens on control and FAC samples remained numerically lower for moderately-abused spinach than means for refrigerated product (Tables 4 and 5). This may be the result of lingering effects of refrigeration in addition to antimicrobial intervention, particularly for FAC-treated samples, but the reasons for continued apparent decline in pathogens even when placed at a temperature otherwise allowing their growth are unclear. Nonetheless, data demonstrate the utility of the plant-derived EOC to exhibit pathogen growth inhibition on physically damaged spinach samples, even under conditions suitable for pathogen growth.
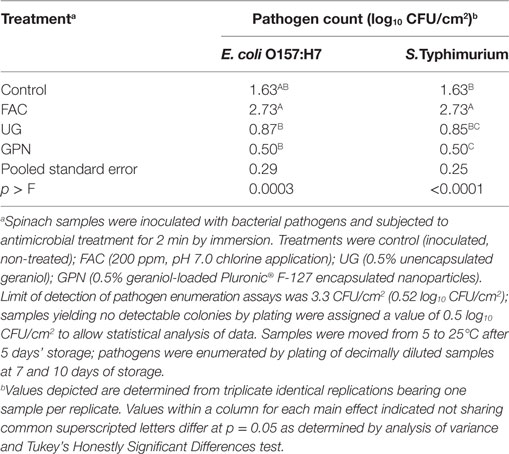
Table 5. Least squares means of Escherichia coli O157:H7 and Salmonella Typhimurium LT2 by antimicrobial treatment on posttreatment inoculated spinach at 15°C.
Similar trends for both pathogen counts on samples treated by antimicrobial intervention, inoculated 3 days later, and then moved to severe temperature abuse (25°C) after day 5 of storage were observed to occur (Table 6). As with moderately abused spinach (Table 5), FAC treatment on day 0 had no apparent effect on long-term pathogen suppression, particularly during the latter half of the experimental period in which the spinach was abusively held (Table 6). Numerical counts of pathogens on non-treated (control) samples at 25°C were higher than corresponding counts of pathogens on control samples held at 15°C (Tables 5 and 6), indicating enhanced growth opportunity under the warmer incubation condition. For both E. coli O157:H7 and S. Typhimurium, numbers of pathogens on control samples were approximately 1.0 log10 CFU/cm2 lower than numbers of pathogens on FAC-treated spinach, also observed in moderately abused spinach (Table 5) but not refrigerated spinach (Table 4). This may have occurred due to FAC reducing background microbes that would have otherwise competed for nutrients, a possible occurrence that would have been masked on refrigerated samples due to the growth-inhibiting effects of cold storage (Table 4). As compared to 15°C-stored spinach, pathogen sensitivity to UG and GPN treatments appeared to differ during severe temperature abuse holding, though for each pathogen the UG and GPN treatments again produced non-differing counts of the pathogen (P ≥ 0.05) (Table 6). Whereas E. coli O157:H7 remained at non-detectable numbers on both UG and GPN-treated spinach held at 25°C, Salmonella numbers were low but detectable (Table 6). This may have resulted from differing sensitivities of the two enteric pathogens to the EOC. This may also have been the result of greater numbers of Salmonella remaining on UG and/or GPN-treated spinach at the point of transition to severe abuse condition (day 5), allowing for greater opportunity for Salmonella detection despite continued inhibition by the antimicrobial.
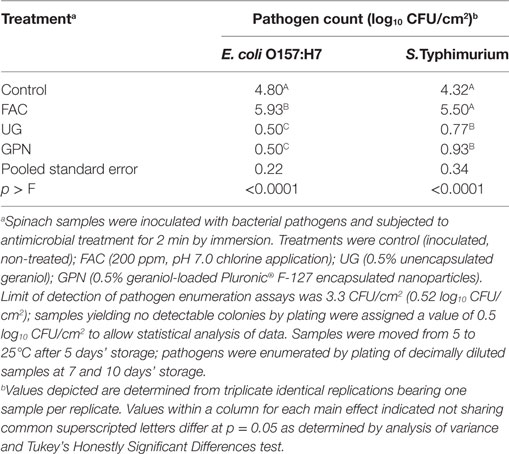
Table 6. Least squares means of Escherichia coli O157:H7 and Salmonella Typhimurium LT2 by antimicrobial treatment on posttreatment inoculated spinach at 25°C.
Impact of Antimicrobial Treatment on Numbers of Microbial Hygiene Indicators and Visual Appearance for Spinach Samples Refrigerated and Samples Initially Refrigerated with Subsequent Moderate or Severe Temperature Abuse
Initial numbers of aerobic bacteria, LAB, and coliforms were 4.7 ± 0.4, 4.3 ± 0.7, and 2.1 ± 1.4 log10 CFU/cm2 at day 0, respectively, prior to refrigerated storage of treated spinach. Immediately following the application of GPNs, counts of aerobic bacteria and LAB were reduced to 1.2 ± 0.6 and 0.7 ± 0.3 log10 CFU/cm2, respectively (Figures 1A,B). Microbial indicator mean counts were significantly impacted by antimicrobial treatment (p < 0.05); storage period and the interaction of treatment x storage period did not influence resulting microbial counts (Figure 1). With respect to counts of aerobic bacteria, UG and GPN treatments produced significantly lower counts as compared to control or FAC-treated samples when refrigerated posttreatment. Conversely, counts of aerobic bacteria did not differ for the control-, FAC-, and UG-treated spinach samples subjected to moderate temperature abuse (15°C) following antimicrobial treatment and 5 days’ refrigeration (Figure 1A). Even in severely abused spinach, unencapsulated and nanoparticle-entrapped geraniol application produced numerically lower aerobic plate counts versus FAC or controls, though means did not statistically differ (p = 0.062).
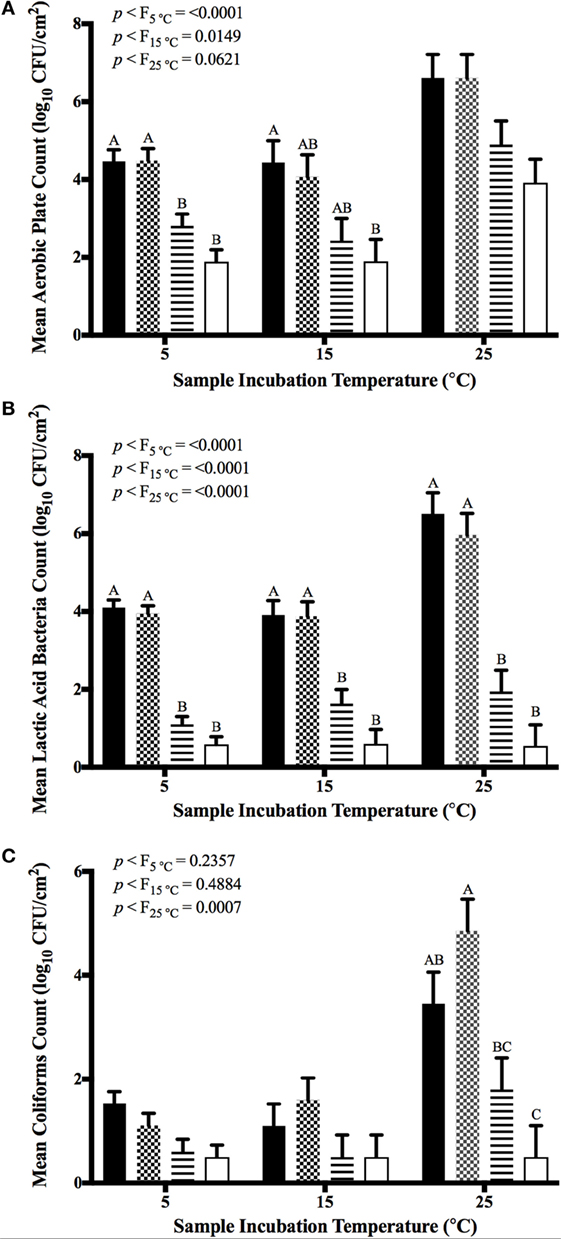
Figure 1. Mean aerobic plate counts (A), lactic acid bacteria (B), and total coliform (C) counts on antimicrobial intervention-treated non-inoculated spinach at 5, 15, or 25°C. Columns depict least squares means of microbes; error bars indicate 1 SE about the mean. Values within a column for each main effect not sharing common letters (A,B,C) differ at p = 0.05 as determined by analysis of variance and Tukey’s Honestly Significant Differences test. Sets of columns not depicting statistical difference indicators do not show statistically significant differences between means (p > 0.05). Treatments were control (inoculated, non-treated; filled bars); FAC (200 ppm, pH 7.0; checkered bars); UG (0.5% unencapsulated geraniol; horizontally hashed bars); GPN (0.5% geraniol-loaded Pluronic® F-127 nanoparticles; open bars).
Similar to aerobic bacteria results, LAB counts differed significantly by antimicrobial treatment for each incubation temperature process (refrigeration, refrigeration + moderate abuse, refrigeration + severe abuse; p < 0.0001), but not by storage period (Figure 1B). In contrast to trends identified for aerobic bacteria, LAB counts from control and FAC treatments did not differ from one another, but did differ from those of UG and GPN-treated samples. For all three storage procedures, LAB from GPN-treated spinach declined to non-detectable levels (detection limit: 0.5 log10 CFU/cm2), whereas countable LAB were detected with other antimicrobial treatments (Figure 1B). As compared to the other two groups of microbial hygiene indicator bacteria, coliform loads on spinach were lower at the outset of the experiment (Figure 1C). Coliform numbers on refrigerated and refrigerated + moderately abused samples remained low during the experiment, and yielded no statistically significant differences between means as a function of antimicrobial treatment. Only in refrigerated + severely abused samples were coliform counts determined to differ, and only differed by treatment. A higher count of coliforms on FAC-treated samples was identified, possibly the result of chlorine’s inactivation of other microbes that would otherwise out-compete coliforms for useful nutrients and/or attachment sites (Figure 1C).
Figures 2–5 depict the changes in spinach samples’ visual appearance as a function of antimicrobial treatment, storage condition(s) and days of storage for treated samples. All spinach samples experienced changes in visual appearance characteristics during posttreatment storage (dehydration, wilting of leaves, discoloration, etc.). Samples treated with GPNs and held at 5°C maintained superior visual appearance (Figure 2) as compared to samples treated by UG, FAC, or control (untreated, inoculated) (Figures 3–5, respectively). Predictably, the preservation of refrigerated storage for spinach better protected visual appearance of samples as compared to spinach subjected to moderate (15°C) or severe (25°C) temperature abuse, across all treatments.
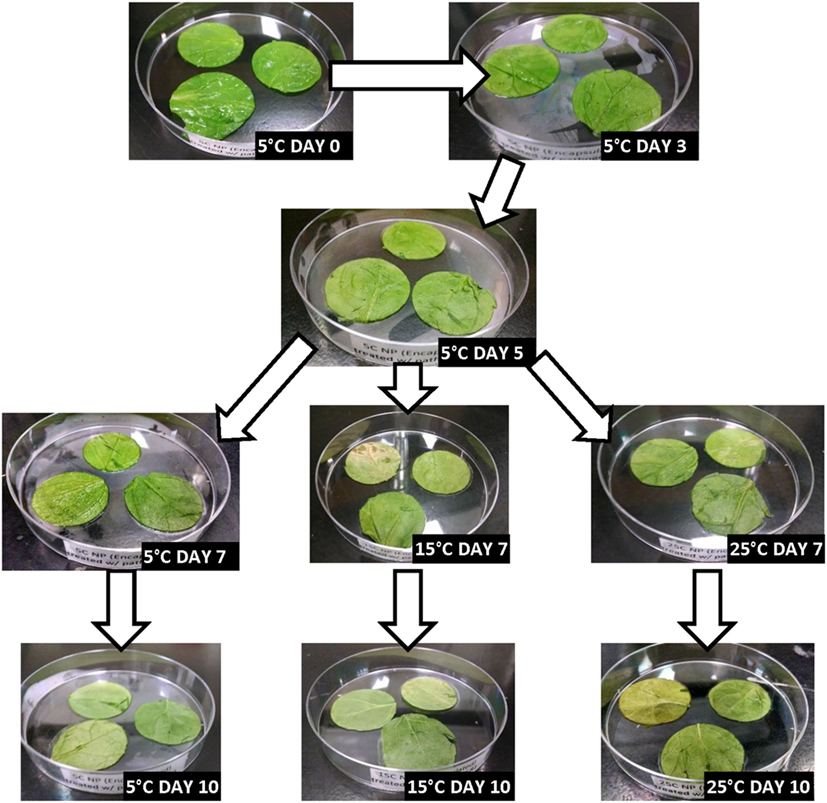
Figure 2. Visual appearance changes of spinach during storage following treatment with geraniol-loaded nanoparticles containing 0.5 wt.% geraniol and stored under refrigerated (5°C), refrigerated + moderate temperature abuse (5 + 15°C), or refrigerated + severe temperature abuse (5 + 25°C) conditions.
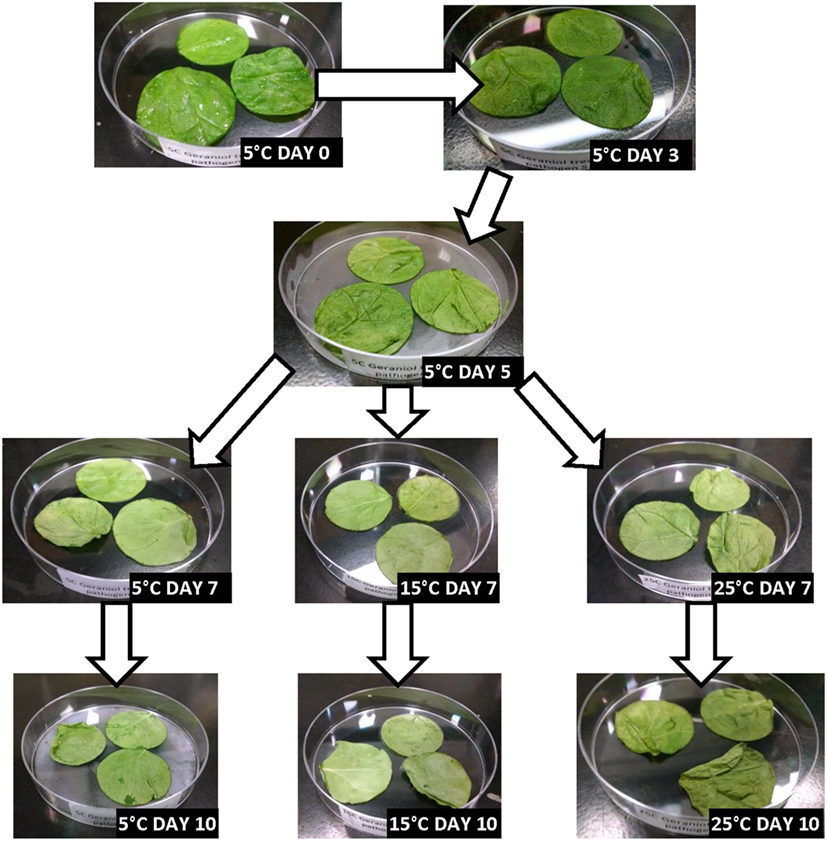
Figure 3. Visual appearance changes of spinach during storage following treatment with unencapsulated 0.5 wt.% geraniol (UG) and stored under refrigerated (5°C), refrigerated + moderate temperature abuse (5 + 15°C), or refrigerated + severe temperature abuse (5 + 25°C) conditions.
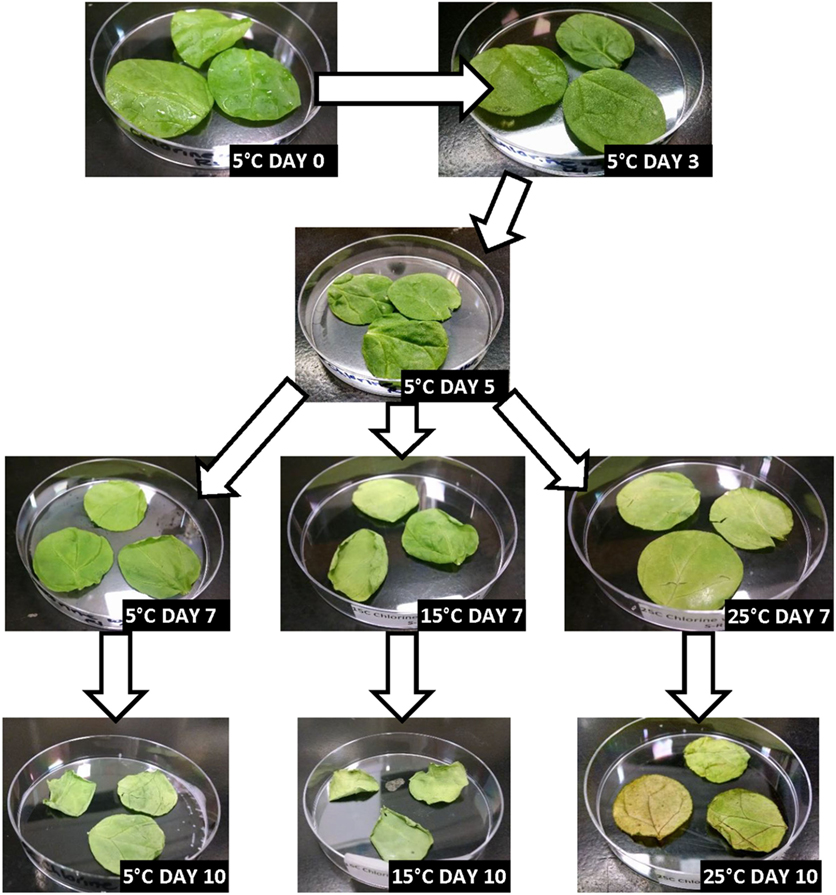
Figure 4. Visual appearance changes of spinach during storage following treatment with 200 ppm (pH 7.5) chlorine (free and available chlorine) and stored under refrigerated (5°C), refrigerated + moderate temperature abuse (5 + 15°C), or refrigerated + severe temperature abuse (5 + 25°C) conditions.
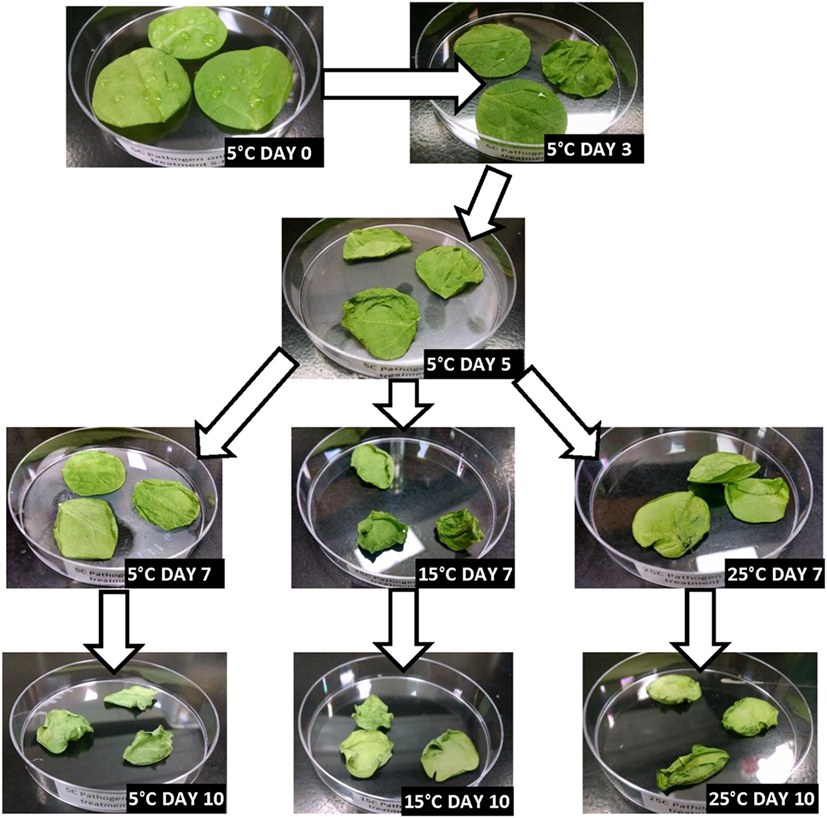
Figure 5. Visual appearance changes during storage of non-treated (Control) spinach stored under refrigerated (5°C), refrigerated + moderate temperature abuse (5 + 15°C), or refrigerated + severe temperature abuse (5 + 25°C) conditions.
Discussion
Reductions of Pathogens by Antimicrobial Interventions on Spinach Held at Refrigeration with or without Subsequent Moderate or Severe Temperature Abuse
Reductions in pathogens observed in the current study are similar to findings from previous research exploring the capacity of plant-derived antimicrobials to decontaminate inoculated produce. Researchers previously reported the application of 0.5% trans-cinnamaldehyde dispersed in TSB produced a 3.3 log10-cycle reduction in E. coli O157:H7 numbers on baby spinach leaves following 10 min exposure at 23°C (Viazis et al., 2011). Baskaran et al. (2013) reported application of 0.15% and 0.35% trans-cinnamaldehyde, 0.15% and 0.30% carvacrol, and 0.5% and 1% β-resorcyclic acid as wash treatments (applied separately) for apples were effective in reducing E. coli O157:H7 compared to a water wash treatment, reducing pathogens by 4.0–5.0 log10 CFU/apple in 5 min.
Analysis of pathogen numbers on spinach sample surfaces as affected by antimicrobial treatments indicated significantly lower numbers of E. coli O157:H7 and S. Typhimurium organisms throughout the 5°C storage period when treated by GPNs and UG as compared to control or FAC-treated spinach (p < 0.05). Pathogen numbers declined throughout the 5°C storage period (p < 0.05). Others have reported encapsulation of plant-derived EOCs within polymeric and/or liposomal nanoparticles enhanced the interaction of the antimicrobial payload with microbial pathogens. These results were reportedly achieved through greater delivery of plant compounds into aqueous media, while limiting interactions with food matrix components otherwise degrading antimicrobial activity (Mourtzinos et al., 2008; Liolios et al., 2009; Hill et al., 2013a). We previously demonstrated the entrapment of geraniol into polymeric nanoparticles resulted in cotransport of the lipophilic dye Nile Red and geraniol into E. coli O157:H7 membranes (Yegin et al., 2016). While free geraniol in the current experiment was able to inhibit the growth of pathogens, entrapment of plant EOCs provides for enhanced delivery of antimicrobial(s) to bacterial pathogenic and/or spoilage microbes for produce disinfection (Hill et al., 2013b; Zhang et al., 2014).
In combination with application of antimicrobial intervention treatments, preservation of spinach under refrigeration predictably limited pathogen growth in the current experiment (Table 1). Conversely, the transition of inoculated and treated spinach samples to 15°C after 5 days of refrigerated storage produced increased mean E. coli O157:H7 and S. Typhimurium counts on control (untreated) spinach at day 7 of the experiment versus pathogen counts on samples stored 7 days continually at 5°C (Tables 1 and 2; data not compared statistically). Likewise, shifting spinach samples from refrigeration to 25°C produced higher numerical means on control, FAC, and UG-treated spinach versus means of pathogens on 5°C-stored spinach treated similarly (Tables 1 and 3; data not statistically compared). Recent research describing the growth of both E. coli O157:H7 and Salmonella on spinach indicated increased growth rates of both organisms on the produce commodity when transitioned from refrigeration (5°C) to elevated/abusive storage temperatures, with lag phases of at least 10 h when ambient temperature was ≤21°C (Mishra et al., 2017). Research conducted on presterilized spinach extract indicated similar trends, with more rapid growth occurring when spinach was held at 16 or 20°C versus 8 or 10°C (Posada-Izquierdo et al., 2016). The FDA recommends the preservation of refrigeration conditions for storage of fresh produce during postharvest handling to prevent/slow growth of microbial pathogens (FDA, 2009, 2013). Hence, while the combination of refrigerated storage and antimicrobial intervention hurdles produces optimal opportunity for pathogen control on spinach, experimental data indicate GPN treatment is capable of slowing or halting enteric pathogens from growth even under conditions of moderate or severe thermal abuse of spinach (Tables 2 and 3).
Inhibition of Pathogens by Antimicrobial Interventions on Spinach Held at Refrigeration with or without Subsequent Moderate or Severe Temperature Abuse Antimicrobial Treatment Preceded Pathogen Application
While extensive research has been reported detailing the antimicrobial utility of chemical sanitizers for the decontamination of fresh and minimally processed produce when pathogens were inoculated onto produce surfaces prior to sanitizing treatment, the current study represents the first to authors’ knowledge to determine the utility of conventional and novel sanitizers to reduce pathogens when applied prior to contamination. The US FDA (2016a,b) has implemented requirements mandating postharvest handling of some produce commodities, including leafy vegetables, be completed in a sanitary fashion to prevent postharvest cross-contamination. Hence, the utilization of sanitizing technologies exhibiting posttreatment residual antimicrobial activity would greatly assist produce packing establishments and/or mixed-type farm facilities in preserving microbiological safety of produce during postharvest handling, packing, and chilling activities. Similar to observations made for spinach inoculated with pathogens, treated with antimicrobial treatments, and held at refrigeration for up to 10 days, spinach samples treated on day 0 and theninoculated on day 3 exhibited differences in pathogen survival as a function of treatment and days of storage postinoculation (Table 4). Whereas numbers of both pathogens on control samples declined from day 3 until day 10, pathogens on FAC-treated samples also declined but to a lesser degree. Significant differences were observed in loads of pathogens on day 7 between the treatments for each pathogen (Table 4). Greater numerical reductions in pathogen numbers were observed for UG and GPN-treated spinach during the experimental period. GPN treatment produced a reduction in both pathogens to non-detectable levels rapidly postinoculation, and both pathogens remained at non-detectable counts throughout the remainder of the experiment. Unencapsulated geraniol (UG) treatment was also able to produce reductions in pathogens to non-detectable levels, though loss of detection was only observed at 7 and 10 days’ refrigerated storage (Table 4).
For spinach treated, inoculated on day 3, and then transitioned to 15°C after 5 days of refrigeration, numbers on control samples (1.6 log10 CFU/cm2) approximated those of control samples stored at 5°C (1.9 log10 CFU/cm2; Tables 4 and 5). Surprisingly, numbers of both pathogens on FAC-treated spinach samples were approximately 1.0 log10-cycle higher than control samples, differing from previous research detailing the antimicrobial properties of FAC under similar storage condition (Gil et al., 2009, 2015; Brown et al., 2011). Nonetheless, application of unencapsulated and GPN-deployed geraniol both produced numerical reductions in pathogens versus FAC-treated samples. Furthermore, GPNs reduced pathogens to non-detectable counts (Table 5), indicating opportunity for nanoentrapped plant EOC to retain enhanced pathogen suppression capability versus FAC or unencapsulated plant antimicrobial through extended antimicrobial release (Gaysinsky et al., 2005, 2007; Yegin et al., 2016; Sarkar et al., 2017).
Transitioning of spinach to severe abuse conditions resulted in similar reductions to pathogens, though reductions were smaller, held at 15°C (Table 6). Previous research has indicated storage of vegetables at ambient conditions allowed for rapid pathogen growth (Hoelzer et al., 2012; Posada-Izquierdo et al., 2016; Mishra et al., 2017). As compared to results from 15°C-stored samples, UG and GPNs treatments yielded non-detectable numbers of E. coli O157:H7. Salmonella reductions were less severe, indicating pathogen-specific differences in sensitivity to the EOC. Previous research has reported pathogen-specific differences in sensitivity to plant-derived EOC antimicrobials, both unencapsulated and encapsulated (Oroojalian et al., 2010; Yegin et al., 2016). The application of antimicrobial-bearing nanoparticles to fresh postharvest leafy vegetable surfaces can assist produce growers and packers in preserving the microbiological safety during postwashing handling (i.e., chilling, transportation, retail display).
Reductions of Microbial Hygiene Indicators by Antimicrobial Interventions on Spinach Held at Refrigeration with or without Subsequent Moderate or Severe Temperature Abuse
Numerical reductions in naturally contaminating indicator microbiota by FAC, UG, and GPN treatments at all experimental incubation temperatures were observed to occur in a manner similar to findings from other research. Orue et al. (2013) reported initial reductions in numbers of aerobic mesophiles upon application of 200 ppm chlorine to spinach and parsley on the day of application, reducing the numbers of aerobes by 1.5–2.0 log10 CFU/g. Recent research has indicated that encapsulation of other plant EOCs when applied to minimally processed leafy vegetables is capable of reducing numbers of aerobic mesophiles on produce surfaces, similar to reductions demonstrated here (Sow et al., 2017). For control-treated samples, shifting spinach to moderate temperature abuse did not result in differences in aerobic mesophiles and LAB, differing from previous results where numbers of both groups of organisms increased upon moving to 15°C (Sant’Ana et al., 2012). Whereas the numbers of coliforms on spinach were not significantly impacted by antimicrobial treatment on refrigerated or moderately abused spinach, it is likely the failure to detect differences occurred due to low initial numbers of coliforms in combination with antimicrobial treatment and reduced temperature storage. When storage environment was shifted to 25°C, the application of GPNs produced significant differences in coliforms versus the control and FAC-treated spinach. As discussed elsewhere, while FAC treatment may reduce numbers of microbes initially, loss of cold storage and inactivation of chlorine activity by increased organic load would be expected to support their regrowth (Beuchat et al., 2005; Alvarado-Casillas et al., 2007; Van Haute et al., 2013). Nonetheless, as already discussed, the utilization of plant EOC encapsulates with a sustained release kinetic even at temperatures similar to those used in the current study may be expected to produce a longer-lasting antimicrobial pressure (Ruengvisesh et al., 2015; Yegin et al., 2016). These data indicate, in addition to pathogen suppression, that epiphytic microbiota capable of causing spoilage of fresh spinach may be inhibited during posttreatment handling, thus preserving spinach shelf-life and quality.
In addition to the reduction in numbers of pathogens on inoculated spinach via GPNs and other antimicrobial intervention treatments, results indicate GPNs may better protect the visual appearance of spinach during posttreatment refrigerated handling and/or retail display versus treatment with unencapsulated plant-derived essential oil components or FAC (Figures 2–4). Yossa et al. (2013) reported similar findings from studies applying free 800 ppm cinnamaldehyde to lettuce leaves, describing increased force to break romaine lettuce leaves versus untreated controls, as well as reduced lettuce color loss over 14 days of refrigerated (4°C) storage versus untreated controls. Additionally, recent research has indicated no statistically significant differences in overall acceptability of spinach samples treated with geranium oil versus non-treated control samples (Rafiq et al., 2016). Likewise, application of lavender oil onto endive leaves at concentrations ranging from 0.001 to 0.08% for 5 and 10 min did not result in detection of off-odor by the majority of panelists (Xylia et al., 2017).
Study Limitations
The utility of research data from the current study may be limited by two key study design variables: (i) the use of physically damaged spinach leaf samples and (ii) the experimental design which disallowed direct statistical comparisons between all treatments and all incubation conditions for each pathogen. All pathogen count raw data were in the current study transformed so as to report pathogen numbers in units of log10 CFU/cm2. At the outset, it was decided to prepare samples with known surface area (10 cm2) by physical sample cutting and preparation so as to facilitate easier produce preparation. Additionally, as has been described elsewhere, the physical damage of produce tends to facilitate greater microbial growth on many produce commodities. Hence, a “worst-case”-type scenario would be expected to occur in the present study, wherein antimicrobial interventions were applied to decontaminate produce samples were handicapped by researchers, in order to give pathogens better opportunity to survive. The occurrence of significant pathogen reduction would then indicate to researchers the likelihood that interventions would perform effectively on non-damaged produce as well. Nevertheless, beyond stem cutting, spinach is not routinely cut/chopped prior to retail, and thus data are not completely representative of current industry practices/needs.
Second, experiments detailing the initial storage of samples at refrigeration condition with a subsequent shift to moderate or severe abuse conditions were integrated into experiments where samples were stored refrigerated for the entire experimental period. Independent samples were not created for all storage day × treatment × temperature condition combinations and so means of pathogens could not be properly compared across all combinations of treatments. Nevertheless, when broken by storage day and pathogen, effects of antimicrobial treatments on pathogens were discernible through statistical analysis. Additionally, was described in Section “Reduction of Pathogens by Antimicrobial Interventions on Spinach Samples Held at Refrigeration and on Samples Held at Refrigeration with Subsequent Moderate or Severe Temperature Abuse,” pathogen loads did not differ numerically from one another following inoculation onto spinach, indicating successful and consistent inoculation was achieved.
Researchers in the current study selected one isolate of each pathogen, rather than opting for multiple isolates of each pathogen. Both isolates used in the current study were reported to bear reduced pathogenesis capacity, through loss of genetically encoded invasion or toxin-production systems. These isolates were chosen for researcher biosafety protection while also producing known pathogen-appropriate colony morphologies on the plating medium. In previous research our group identified MICs of GPNs and UG treatments against pathogen isolates used in the current study (Yegin et al., 2016). MICs of E. coli O157:H7 and Salmonella isolates in the current study were 0.4 and 0.7 wt.%. Other researchers have reported similar MICs for other isolates of E. coli O157:H7 and Salmonella enterica of free geraniol (0.5%) (Kim et al., 1995; Nazer et al., 2005). The use of single isolates versus mixed isolate inocula (i.e., cocktails) may reduce the application of study data to commercial systems by limiting evaluation of each pathogen’s tolerance to differing decontamination treatments (Beuchat et al., 2001). Additionally, while some experiments were conducted during spinach growing periods in Texas and the south central US, not all experimental procedures were able to be completed within a single growing season, disallowing the use of freshly harvested non-treated spinach. The choice to use retail-purchased spinach was made to facilitate study completion in a timely manner while reducing the overall costs associated with spinach procurement.
Innovativeness of Current Study, Conclusions, and Recommended Future Research
In conclusion, the application of free or encapsulated geraniol resulted in enhanced antimicrobial activity against the enteric pathogens S. Typhimurium LT2 and E. coli O157:H7 compared to FAC or control treatment on physically damaged spinach leaf sample surfaces, both under conditions of refrigeration and temperature abuse. The current study addresses fresh produce safety through two key innovations: (i) the application of nanoentrapped plant-derived antimicrobial, a technology only sparingly reported in the available literature and (ii) the determination of sanitizer capacity to provide produce decontamination when antimicrobial treatment precedes pathogen contamination, an event that may occur during postwashing handling of produce but remains under-researched. Nanoencapsulation likely increased bioavailability and transportation of geraniol, observed through lower numbers of surviving pathogens on surfaces of spinach leaves as compared to UG and FAC treatments. Though a great deal of research has been completed detailing the efficacy of plant-derived EOCs to inhibit the growth of foodborne bacterial pathogens, studies detailing their utility on fresh produce after encapsulation in food-grade encapsulating materials are novel, as are those demonstrating the residual utility of antimicrobial encapsulates applied prior to pathogen cross-contamination. These data may be useful in assisting produce packers to decontaminate harvested leafy vegetables from enteric pathogens in combination with sanitary postharvest handling and transportation prior to consumption.
Future research should be designed to align with the current study and other similar studies by evaluating geraniol and other plant-derived antimicrobials on intact spinach leaves (not physically damaged) applied either in field (preharvest foliar spray) or as a postharvest sanitizing treatment. Whereas in the current study only single isolates of each pathogen were utilized, studies utilizing multiple isolates of pathogens would enhance the opportunity for researchers to recover isolates demonstrating greater tolerance to antimicrobial interventions. This would indicate potential for variability in pathogen inhibition during commercial use of interventions. Research evaluating the utility of antimicrobial interventions testing nanoencapsulated EOCs against other microbial pathogens, such as Listeria monocytogenes, members of the Campylobacteriaceae, Shigella spp., or even viral pathogens (e.g., Human Norovirus), should be completed to more broadly determine the utility of antimicrobial interventions similar to those studied here. Future studies, in order to provide comprehensive characterization of the utility of these antimicrobial interventions for produce safety, should investigate the environmental decomposition of intervention treatments in spent produce washing waters as well as verify lack of acute toxicity of GPNs.
Author Contributions
All authors have, by virtue of assisting the drafting of this manuscript, its revision in order to prepare its submission, as well as through communication of their approval to the corresponding author, approved of this manuscript’s submission in its current form. KP-L led the sample preparation, inoculum preparation and application, and microbiological analysis of all samples described. YY and MA oversaw the preparation of geraniol-loaded nanoparticles; YY collaborated on antimicrobial treatment application to inoculated samples with KP-L. AC and LC-Z advised experimental conditions and trials in collaboration with author TT. LC-Z provided input on incorporation of photographic evaluation/data collection for assessing antimicrobial treatment impact on spinach. CK supervised the statistical analysis of all numerical data, collaborating with TT on recommended analysis procedures. TT led efforts to procure research funding, functioned as KP-L’s graduate degree program supervisor, and oversaw all major decisions related to study design and execution, collaborating with all other authors throughout the study completion, and manuscript preparation.
Conflict of Interest Statement
The authors declare that the research was conducted in the absence of any commercial or financial relationships that could be construed as a potential conflict of interest.
Acknowledgments
Authors thank Ming Zhang, PhD, Artie McFerrin Department of Chemical Engineering, Texas A&M University, College Station, TX, USA, for technical assistance provided in nanoparticle design/fabrication.
Funding
This work is supported by Agriculture and Food Research Initiative [grant no. 2011-67017-30028] from the USDA National Institute of Food and Agriculture. KP-L and YY received graduate assistantship support from Texas A&M University. Research sponsor did not have any influence over study design, experimental data analysis, manuscript preparation, journal selection, or manuscript submission for publication. The open access publishing fees for this article have been covered by the Texas A&M University Open Access to Knowledge Fund (OAKFund), supported by the University Libraries and the Office of the Vice President for Research.
References
Alvarado-Casillas, S., Ibarra-Sánchez, S., Rodríguez-García, O., Martínez-Gonzáles, N., and Castillo, A. (2007). Comparison of rinsing and sanitizing procedures for reducing bacterial pathogens on fresh cantaloupes and bell peppers. J. Food Prot. 70, 655–660. doi: 10.4315/0362-028X-70.3.655
Angelo, K. M., Chu, A., Anand, M., Nguyen, T.-A., Bottichio, L., Wise, M., et al. (2015). Outbreak of Salmonella newport infections linked to cucumbers – United States, 2014. Morb. Mort. Wkly. Rep. 64, 144–147.
Baskaran, S. A., Upadhyay, A., Kollanoor-Johny, A., Upadhyaya, I., Mooyottu, S., and Roshni Amalaradjou, M. A. (2013). Efficacy of plant-derived antimicrobials as antimicrobial wash treatments for reducing enterohemorrhagic Escherichia coli O157:H7 on apples. J. Food Sci. 78, M1399–M1404. doi:10.1111/1750-3841.12174
Beuchat, L. R., Farber, J. M., Garrett, E. H., Harris, L. J., Parish, M. E., and Suslow, T. V. (2001). Standardization of a method to determine the efficacy of sanitizers in inactivating human pathogenic microorganisms on raw fruits and vegetables. J. Food Prot. 64, 1079–1084. doi:10.4315/0362-028X-64.7.1079
Beuchat, L. R., Pettigrew, C. A., Tremblay, M. E., Roselle, B. J., and Scouten, A. J. (2005). Lethality of chlorine, chlorine dioxide, and a commercial fruit and vegetable sanitizer to vegetative cells and spores of Bacillus cereus and spores of Bacillus thuringiensis. J. Ind. Microbiol. Biotechnol. 32, 301–308. doi:10.1007/s10295-005-0212-7
Brown, A. L., Brooks, J. C., Karunasena, E., Echeverry, A., Laury, A., and Brashears, M. M. (2011). Inhibition of Escherichia coli O157:H7 and Clostridium sporogenes in spinach packaged in modified atmospheres after treatment combined with chlorine and lactic acid bacteria. J. Food Sci. 76, M427–M432. doi:10.1111/j.1750-3841.2011.02260.x
Casco, G., Taylor, T. M., and Alvarado, C. (2015). Evaluation of novel micronized encapsulated essential oil-containing phosphate and lactate blends for growth inhibition of Listeria monocytogenes and Salmonella on poultry bologna, pork ham, and roast beef ready-to-eat deli loaves. J. Food Prot. 78, 698–706. doi:10.4315/0362-028X.JFP-14-273
Castillo, A., Lucia, L. M., Goodson, K. J., Savell, J. W., and Acuff, G. R. (1998). Use of hot water for beef carcass decontamination. J. Food Prot. 61, 19–25. doi:10.4315/0362-028X-61.1.19
CDC. (2006). Multistate Outbreak of E. coli O157:H7 Infections Linked to Fresh Spinach (Final Update). Available from: https://www.cdc.gov/ecoli/2006/spinach-10-2006.html
CDC. (2012a). Multistate Outbreak of Salmonella braenderup Infections Associated with Mangoes (Final Update). Available from: https://www.cdc.gov/salmonella/braenderup-08-12/
CDC. (2012b). Multistate Outbreak of Salmonella typhimurium and Salmonella Newport Infections Linked to Cantaloupe (Final Update). Available from: https://www.cdc.gov/salmonella/typhimurium-cantaloupe-08-12/
Davidson, P. M., Critzer, F. J., and Taylor, T. M. (2013). Naturally occurring antimicrobials for minimally processed foods. Annu. Rev. Food Sci. Technol. 4, 163–190. doi:10.1146/annurev-food-030212-182535
Donsì, F., Annunziata, M., Sessa, M., and Ferrari, G. (2011). Nanoencapsulation of essential oils to enhance their antimicrobial activity in foods. Food Sci. Technol. 44, 1908–1914. doi:10.1016/j.lwt.2011.03.003
FDA. (2008). Guidance for Industry: Guide to Minimize Microbial Food Safety Hazards of Fresh-Cut Fruits and Vegetables. Available from: http://www.fda.gov/Food/GuidanceRegulation/GuidanceDocumentsRegulatoryInformation/ProducePlantProducts/ucm064458.htm
FDA. (2009). Draft Guidance for Industry: Guide to Minimize Microbial Food Safety Hazards of Melons. Available from: https://www.fda.gov/Food/GuidanceRegulation/GuidanceDocumentsRegulatoryInformationucm174171.htm
FDA. (2011). PMA P110003: Summary of Safety and Effectiveness (SSED): LeGoo®. Available from: https://www.accessdata.fda.gov/cdrh_docs/pdf11/P110003b.pdf
FDA. (2013). Food Code, 2013 Rev. Available from: https://www.fda.gov/Food/GuidanceRegulation/RetailFoodProtection/FoodCode/
FDA. (2016a). Title 21 CFR §112: Standards for the Growing, Harvesting, Packing, and Holding of Produce for Human Consumption. Available from: https://www.ecfr.gov/cgi-bin/text-idx?SID=25a4b17516cbd776c8bd574425ec88df&mc=true&node=pt21.2.112&rgn=div5
FDA. (2016b). Title 21 CFR §182: Substance Generally Recognized as Safe. Available from: https://www.ecfr.gov/cgi-bin/text-idx?SID=d0326695d7a37d94ba8b7691911a1006&mc=true&node=pt21.3.182&rgn=div5#se21.3.182_160
Gaysinsky, S., Davidson, P. M., Bruce, B. D., and Weiss, J. (2005). Stability and antimicrobial efficiency of eugenol encapsulated in surfactant micelles as affected by temperature and pH. J. Food Prot. 68, 1359–1366. doi:10.4315/0362-028X-68.7.1359
Gaysinsky, S., Taylor, T. M., Davidson, P. M., Bruce, B. D., and Weiss, J. (2007). Antimicrobial efficacy of eugenol microemulsions in milk against Listeria monocytogenes and Escherichia coli O157:H7. J. Food Prot. 70, 2631–2637. doi:10.4315/0362-028X-70.11.2631
Gil, M. I., Selma, M. V., López-Gálvez, F., and Allende, A. (2009). Fresh-cut product sanitation and wash water disinfection: problems and solutions. Int. J. Food Microbiol. 134, 37–45. doi:10.1016/j.ijfoodmicro.2009.05.021
Gil, M. I., Selma, M. V., Suslow, T., Jacxsens, L., Uyttendaele, M., and Allende, A. (2015). Pre- and postharvest preventive measures and intervention strategies to control microbial food safety hazards of fresh leafy vegetables. Crit. Rev. Food Sci. Nutr. 55, 453–468. doi:10.1080/10408398.2012.657808
Gomes, C., Moreira, R. G., and Castell-Perez, E. (2011). Microencapsulated antimicrobial compounds as a means to enhance electron beam irradiation treatment for inactivation of pathogens on fresh spinach leaves. J. Food Sci. 76, E479–E488. doi:10.1111/j.1750-3841.2011.02264.x
Gould, L. H., Walsh, K. A., Vieira, A. R., Herman, K., Williams, I. T., Hall, A. J., et al. (2013). Surveillance for foodborne disease outbreaks – United States, 1998-2008. MMWR Surveill. Summ. 62, 1–34.
Harris, L. J., Farber, J. N., Beuchat, L. R., Parish, M. E., Suslow, T. V., Garrett, E. H., et al. (2003). Outbreaks associated with fresh produce: incidence, growth, and survival of pathogens in fresh and fresh-cut produce. Comp. Rev. Food Sci. Food Safety. 2, 78–141. doi:10.1111/j.1541-4337.2003.tb00031.x
Herman, K. M., Hall, A. J., and Gould, L. H. (2015). Outbreaks attributed to fresh leafy vegetables, United States, 1973-2012. Epidemiol. Infect. 143, 3011–3021. doi:10.1017/S0950268815000047
Hill, L. E., Gomes, C., and Taylor, T. M. (2013a). Characterization of beta-cyclodextrin inclusion complexes containing essential oils (trans-cinnamaldehyde, eugenol, cinnamon bark, and clove bud extracts) for antimicrobial delivery applications. Food Sci. Technol. 51, 86–93. doi:10.1016/j.lwt.2012.11.011
Hill, L. E., Taylor, T. M., and Gomes, C. (2013b). Antimicrobial efficacy of poly (DL-lactide-co-glycolide) (PLGA) nanoparticles with entrapped cinnamon bark extract against Listeria monocytogenes and Salmonella typhimurium. J. Food Sci. 78, N626–N632. doi:10.1111/1750-3841.12069
Hoelzer, K., Pouillot, R., and Dennis, S. (2012). Listeria monocytogenes growth dynamics on produce: a review of the available data for predictive modeling. Foodborne Pathog. Dis. 9, 661–673. doi:10.1089/fpd.2011.1087
Jung, Y., Jang, H., and Matthews, K. R. (2014). Effect of the food production chain from farm practices to vegetable processing on outbreak incidence. Microb. Biotechnol. 7, 517–527. doi:10.1111/1751-7915.12178
Khalil, R. K., and Frank, J. F. (2010). Behavior of Escherichia coli O157: H7 on damaged leaves of spinach, lettuce, cilantro, and parsley stored at abusive temperatures. J. Food Prot. 73, 212–220. doi:10.4315/0362-028X-73.2.212
Kim, J. M., Marshall, M. R., and Wei, C. (1995). Antibacterial activity of some essential oil components against five foodborne pathogens. J. Agric. Food Chem. 43, 2839–2845. doi:10.1021/jf00059a013
Koukkidis, G., Haigh, R., Allcock, N., Jordan, S., and Freestone, P. (2017). Salad leaf juices enhance Salmonella growth, colonization of fresh produce, and virulence. Appl. Environ. Microbiol. 83, e–02416–16. doi:10.1128/AEM.02416-16
Kroupitski, Y., Pinto, R., Brandl, M. T., Belausov, E., and Sela, S. (2009). Interactions of Salmonella enterica with lettuce leaves. J. Appl. Microbiol. 109, 1876–1885. doi:10.1111/j.1365-2672.2009.04152.x
Lang, M. M., Harris, L. J., and Beuchat, L. R. (2004). Survival and recovery of Escherichia coli O157:H7, Salmonella, and Listeria monocytogenes on lettuce and parsley as affected by method of inoculation, time between inoculation and analysis, and treatment with chlorinated water. J. Food Prot. 67, 1092–1103. doi:10.4315/0362-028X-67.6.1092
Liolios, C. C., Gortzi, O., Lalas, S., Tsaknis, J., and Chinou, I. (2009). Liposomal incorporation of carvacrol and thymol isolated from the essential oil of Origanum dictamnus L. and in vitro antimicrobial activity. Food Chem. 112, 77–83. doi:10.1016/j.foodchem.2008.05.060
Lynch, M. F., Tauxe, R. V., and Hedberg, C. W. (2009). The growing burden of foodborne outbreaks due to contaminated fresh produce: risks and opportunities. Epidemiol. Infect. 137, 307–315. doi:10.1017/S0950268808001969
Mishra, A., Guo, M., Buchanan, R. L., Schaffner, D. W., and Pradhan, A. K. (2017). Prediction of Escherichia coli O157:H7, Salmonella, and Listeria monocytogenes growth in leafy greens without temperature control. J. Food Prot. 80, 68–73. doi:10.4315/0362-028X.JFP-16-153
Mourtzinos, I., Kalogeropoulos, N., Papadakis, S. E., Konstantinou, K., and Karathanos, V. T. (2008). Encapsulation of nutraceutical monoterpenes in β-cyclodextrin and modified starch. J. Food Sci. 73, S89–S94. doi:10.1111/j.1750-3841.2007.00609.x
Nazer, A. I., Kobilinsky, A., Tholozan, J.-L., and Dubois-Brissonnet, F. (2005). Combinations of food antimicrobials at low levels to inhibit the growth of Salmonella typhimurium: a synergistic effect? Food Microbiol. 22, 391–398. doi:10.1016/j.fm.2004.10.003
Neal, J. A., Cabrera-Diaz, E., and Castillo, A. (2012). Attachment of Escherichia coli O157:H7 and Salmonella on spinach (Spinacia oleracea) using confocal microscopy. Agric. Food Anal. Bacteriol. 2, 275–279.
Nguyen-The, C., Bardin, M., Berard, A., Berge, O., Brillard, J., Broussolle, V., et al. (2016). Agrifood systems and the microbial safety of fresh produce: trade-offs in the wake of increased sustainability. Sci. Total Environ. 562, 751–759. doi:10.1016/j.scitotenv.2016.03.241
Oroojalian, F., Kasra-Kermanshahi, R., Azizi, M., and Bassami, M. R. (2010). Phytochemical composition of the essential oils from three Apiaceae species and their antibacterial effects on food-borne pathogens. Food Chem. 120, 765–770. doi:10.1016/j.foodchem.2009.11.008
Orue, N., García, S., Feng, P., and Heredia, N. (2013). Decontamination of Salmonella, Shigella, and Escherichia coli O157:H7 from leafy green vegetables using edible plant extracts. J. Food Sci. 78, M290–M296. doi:10.1111/1750-3841.12016
Painter, J. A., Hoekstra, R. M., Ayers, T., Tauxe, R. V., Braden, C. R., Angulo, F. J., et al. (2013). Attribution of foodborne illnesses, hospitalizations, and deaths to food commodities by using outbreak data, United States, 1998-2008. Emerg. Infect. Dis. 19, 407–415. doi:10.3201/eid1903.111866
Perez, K. L., Lucia, L. M., Cisneros-Zevallos, L., Castillo, A., and Taylor, T. M. (2012). Efficacy of antimicrobials for the disinfection of pathogen contaminated green bell pepper and of consumer cleaning methods for the decontamination of knives. Int. J. Food Microbiol. 156, 76–82. doi:10.1016/j.ijfoodmicro.2012.03.012
Posada-Izquierdo, G., Del Rosal, S., Valero, A., Zurera, G., Sant’Ana, A. S., Alvarenga, V. O., et al. (2016). Assessing the growth of Escherichia coli O157:H7 and Salmonella in spinach, lettuce, parsley and chard extracts at different storage temperatures. J. Appl. Microbiol. 120, 1701–1710. doi:10.1111/jam.13122
Rafiq, R., Hayek, S. A., Anyanwu, U., Hardy, B. I., Giddings, V. L., Ibrahim, S. A., et al. (2016). Antibacterial and antioxidant activities of essential oils from Artemisia herba-alba Asso., Pelargonium capitatum x radens and Laurus nobilis L. Foods 5, 12. doi:10.3390/foods5020028
Roever, C. D. (1998). Microbiological safety evaluations and recommendations on fresh produce. Food Cont. 69, 321–347. doi:10.1016/S0956-7135(98)00022-X
Ruengvisesh, S., Loquercio, A., Castell-Perez, E., and Taylor, T. M. (2015). Inhibition of bacterial pathogens in medium and on spinach leaf surfaces using plant-derived antimicrobials loaded in surfactant micelles. J. Food Sci. 80, M2522–M2529. doi:10.1111/1750-3841.130895
Sant’Ana, A. S., Barbosa, M. S., Destro, M. T., Landgraf, M., and Franco, B. D. (2012). Growth potential of Salmonella spp. and Listeria monocytogenes in nine types of ready-to-eat vegetables stored at variable temperature conditions during shelf-life. Int. J. Food Microbiol. 157, 52–58. doi:10.1016/j.ijfoodmicro.2012.04.011
Sarkar, P., Bhunia, A. K., and Yao, Y. (2017). Impact of starch-based emulsions on the antibacterial efficacies of nisin and thymol in cantaloupe juice. Food Chem. 217, 155–162. doi:10.1016/j.foodchem.2016.08.071
Slayton, R. B., Turabelidze, G., Bennett, S. D., Schwensohn, C. A., Yaffee, A. Q., Khan, F., et al. (2013). Outbreak of Shiga toxin-producing Escherichia coli (STEC) O157:H7 associated with romaine lettuce consumption, 2011. PLoS ONE 8:e55300. doi:10.1371/journal.pone.0055300
Sow, L. C., Tirtawinata, F., Yang, H., Shao, Q., and Wang, S. (2017). Carvacrol nanoemulsion combined with acid electrolysed water to inactivate bacteria, yeast in vitro and native microflora on shredded cabbages. Food Cont. 76, 88–95. doi:10.1016/j.foodcont.2017.01.007
Van Haute, S., Sampers, I., Holvoet, K., and Uyttendaele, M. (2013). Physicochemical quality and chemical safety of chlorine as a reconditioning agent and wash water disinfectant for fresh-cut lettuce washing. Appl. Environ. Microbiol. 79, 2850–2861. doi:10.1128/AEM.03283-12
Viazis, S., Akhtar, M., Feirtag, J., and Diez-Gonzalez, F. (2011). Reduction of Escherichia coli O157:H7 viability on leafy green vegetables by treatment with a bacteriophage mixture and trans-cinnamaldehyde. Food Microbiol. 28, 149–157. doi:10.1016/j.fm.2010.09.009
Xylia, P., Chrysargyris, A., Botsaris, G., and Tzortzakis, N. (2017). Potential application of spearmint and lavender essential oils for assuring endive quality and safety. Crop Prot. 102, 94–103. doi:10.1016/j.cropro.2017.08.015
Yegin, Y., Perez-Lewis, K. L., Zhang, M., Akbulut, M., and Taylor, T. M. (2016). Development and characterization of geraniol-loaded polymeric nanoparticles with antimicrobial activity against foodborne bacterial pathogens. J. Food Eng. 170, 64–71. doi:10.1016/j.jfoodeng.2015.09.017
Yossa, N., Patel, J., Millner, P., Ravishankar, S., and Lo, Y. M. (2013). Antimicrobial activity of plant essential oils against Escherichia coli O157:H7 and Salmonella on lettuce. Foodborne Pathog. Dis. 10, 87–96. doi:10.1089/fpd.2012.1301
Zhang, H., Shen, Y., Bao, Y., He, Y., Feng, F., and Zheng, X. (2008). Characterization and synergistic antimicrobial activities of food-grade dilution-stable microemulsions against Bacillus subtilis. Food Res. Int. 41, 495–499. doi:10.1016/j.foodres.2008.02.006
Keywords: nanoparticles, Escherichia coli O157:H7, Salmonella, spinach, geraniol, plant-derived antimicrobial
Citation: Perez-Lewis KL, Yegin Y, Cisneros-Zevallos L, Castillo A, Kerth CR, Akbulut M and Taylor TM (2018) Geraniol-Loaded Polymeric Nanoparticles Inhibit Enteric Pathogens on Spinach during Posttreatment Refrigerated and Temperature Abuse Storage. Front. Sustain. Food Syst. 2:4. doi: 10.3389/fsufs.2018.00004
Received: 16 December 2017; Accepted: 31 January 2018;
Published: 16 February 2018
Edited by:
Joshua B. Gurtler, Agricultural Research Service (USDA), United StatesReviewed by:
Arun K. Bhunia, Purdue University, United StatesHyun-Gyun Yuk, Korea National University of Transportation, South Korea
Copyright: © 2018 Perez-Lewis, Yegin, Cisneros-Zevallos, Castillo, Kerth, Akbulut and Taylor. This is an open-access article distributed under the terms of the Creative Commons Attribution License (CC BY). The use, distribution or reproduction in other forums is permitted, provided the original author(s) and the copyright owner are credited and that the original publication in this journal is cited, in accordance with accepted academic practice. No use, distribution or reproduction is permitted which does not comply with these terms.
*Correspondence: Thomas Matthew Taylor, matt_taylor@tamu.edu